- China General Microbiological Culture Collection Center (CGMCC), Institute of Microbiology, Chinese Academy of Sciences, Beijing, China
The bacterial genus Cryobacterium includes at present 14 species that live in cryospheric environments. In this study, we analyzed 101 genomes of Cryobacterium with pure cultures obtained from GenBank. They could be classified into 44 species based on average nucleotide identity (ANI) analysis, showing the diversity of Cryobacterium. Among these, 19 strains in our laboratory were isolated from the glacier samples in China. The pairwise ANI values of these 19 strains and known species were <95%, indicating that they represented 19 novel species. The comparative genomic analysis showed significant differences in gene content between the two groups with a maximum growth temperature (Tmax) of ≤ 20°C and a Tmax of >20°C. A comprehensive and robust phylogenetic tree, including 14 known species and 19 novel species, was constructed and showed five phylogenetic branches based on 265 concatenated single-copy gene sequences. The Tmax parameter had a strong phylogenetic signal, indicating that the temperature adaptation of Cryobacterium was largely through vertical transfer rather than horizontal gene transfer and was affected by selection. Furthermore, using polyphasic taxonomy combined with phylogenomic analysis, we proposed 19 novel species of the genus Cryobacterium by the following 19 names: Cryobacterium serini sp. nov., Cryobacterium lactosi sp. nov., Cryobacterium gelidum sp. nov., Cryobacterium suzukii sp. nov., Cryobacterium fucosi sp. nov., Cryobacterium frigoriphilum sp. nov., Cryobacterium cryoconiti sp. nov., Cryobacterium lyxosi sp. nov., Cryobacterium sinapicolor sp. nov., Cryobacterium sandaracinum sp. nov., Cryobacterium cheniae sp. nov., Cryobacterium shii sp. nov., Cryobacterium glucosi sp. nov., Cryobacterium algoritolerans sp. nov., Cryobacterium mannosilyticum sp. nov., Cryobacterium adonitolivorans sp. nov., Cryobacterium algoricola sp. nov., Cryobacterium tagatosivorans sp. nov., and Cryobacterium glaciale sp. nov. Overall, the taxonomy and genomic analysis can improve our knowledge of phenotypic diversity, genetic diversity, and evolutionary characteristics of Cryobacterium.
Introduction
Glaciers represent an important low-temperature ecosystem. The glacier surface encompasses a variety of habitats for cold-adapted microorganisms, including uppermost ice and snow, surface streams, ponds, cryoconite holes, and moraines (Boenigk et al., 2006). These habitats constitute an important ecological area in the glacier, known as the supraglacial ecological zone (Hotaling et al., 2017). Microorganisms living in the supraglacial ecological zone have attracted increasing attention, and many studies have deepened our understanding of their diversity, speciation, and evolution (Liu et al., 2017, 2019b; Nicholes et al., 2019). DNA sequencing technology has provided insights into the composition of the supraglacial microbial community mainly composed of algae, Cyanobacteria, fungi, bacteria, viruses, and protozoa (Anesio et al., 2017). Though inhabited by a variety of prokaryotic and eukaryotic microorganisms, the supraglacial community contains predominantly autotrophic and heterotrophic bacteria, which are exposed to oligotrophic, solar radiation, and periodic freezing–thawing effects (Hotaling et al., 2017).
The recent focus has been on unknown extremophilic microorganisms and their metabolic characteristics (Silva et al., 2021). Therefore, obtaining pure cultures of extreme microorganisms for further classification and physiological and evolutionary studies is essential. In the last decade, we isolated thousands of heterotrophic bacterial strains from the surface samples of glaciers in Western China, including some common taxa in the cryosphere, such as Arthrobacter, Cryobacterium, Flavobacterium, Polaromonas, Sphingomonas, and Janthinobacterium (Liu et al., 2015). Studies investigating these strains have promoted not only the discovery and validation of new species (Liu et al., 2019a, 2020b, 2021b; Zhang et al., 2020; Yang et al., 2021) but also the understanding of novel functions (Liu et al., 2021a) and evolutionary characteristics (Liu et al., 2020a) of cold-adapted bacteria.
The genus Cryobacterium represents a group specific to low-temperature environments. It was proposed by Suzuki et al. (1997) with Cryobacterium psychrophilum as the type species. It belongs to the class Actinobacteria and the family Microbacteriaceae. Most strains of Cryobacterium are cold tolerant, and some of them are psychrophilic, growing at ≤ 20°C (Liu et al., 2020a). At the time of writing this manuscript, Cryobacterium included 14 species with validly published names (Parte et al., 2020), of which 12 species were isolated from the cryosphere. All type strains, except Cryobacterium tepidiphilum NEAU-85T, grew at a temperature of < 10°C (Wang et al., 2019). The maximum growth temperature (Tmax) of nine species was less than 20°C (Suzuki et al., 1997; Reddy et al., 2010; Liu et al., 2012, 2013, 2018a, 2019c, 2020b). Furthermore, the other five species had a Tmax range of 26–30°C and could grow well not only at < 20°C but also at >20°C, indicating a broader temperature tolerance range compared with the psychrophilic species. Because few pure culture strains are available, the investigations related to its phylogeny and biodiversity are comparatively limited. Liu et al. (2020a) conducted an adaptive evolution analysis based on the genomic sequences of 78 Cryobacterium strains and found that these strains showed thermotolerance divergence with phylogenetic cohesion, which was closely related to the GC3 content. These findings indicated that Cryobacterium was a valuable bacterial genus for studying adaptive evolution under temperature stress. Therefore, it is important to isolate additional Cryobacterium strains from the cryosphere and identify novel species.
Our previous studies showed that the pure cultures of Cryobacterium, including strains isolated in our laboratory and type strains of known species, could be classified into at least more than 30 species with high genetic diversity (Liu et al., 2020a). In this study, we analyzed more than 100 genome sequences of Cryobacterium strains in GenBank. We inferred that at least 44 species of the genus Cryobacterium existed with pure cultures. Therefore, many novel species remain to be classified, identified, and validly published. We described 19 strains, which we isolated from glaciers, and proposed 19 novel species using a polyphasic approach. Furthermore, we divided 79 Cryobacterium strains with available Tmax traits into two groups based on their ability to grow at a temperature of more than 20°C and conducted a genome analysis. This study provided new insights into the cold-adaptation mechanisms and ecological diversification of the genus Cryobacterium.
Materials and methods
Genome information, annotation, and ANI calculation
The whole-genome sequences of the genus Cryobacterium were downloaded from the National Center for Biotechnology Information (NCBI) genome database using the BioSAK program (https://github.com/songweizhi/BioSAK). The genomes were classified using the Genome Taxonomy Database Toolkit (GTDB-Tk) v.2.1.0 (Chaumeil et al., 2019) based on the GTDB Release R207_v2 database (Parks et al., 2022). The CheckM v.1.1.3 program (Parks et al., 2015) was used to check the completeness and contamination values of the genomes. The average nucleotide identity (ANI) values were calculated using the FastANI program (Jain et al., 2018). The symmetric pairwise ANI dissimilarity matrix was clustered using the average linkage hierarchical clustering method with the “bactaxR” package (Carroll et al., 2020) in R 4.0.3. The genomes were annotated using Prokka software (Seemann, 2014). The protein sequence was also functionally annotated and classified into Cluster of Orthologous Groups (COG) categories using the BLAST+ program (Camacho et al., 2009) based on the latest COG database (Galperin et al., 2021). Carbohydrate-active enzymes (CAZymes) were identified using the CAZy database V11 (Drula et al., 2022). The growth temperature data were obtained from the studies by Wang et al. (2019), Gong et al. (2020), and Liu et al. (2020a). The strains with available Tmax were divided into two groups: strictly psychrophilic (SP, Tmax ≤ 20°C) and psychrotolerant (PT, Tmax > 20°C).
Strains and culture conditions
In total, 19 Cryobacterium strains, which were selected for polyphasic taxonomic analysis, were isolated from ice, cryoconite, and meltwater samples in the supraglacial zone of Xinjiang No. 1, Toumingmengke, Hailuogou, and Midui glaciers of China, as listed in Table 1 and described by Liu et al. (2020a). All strains were cultivated in a peptone, yeast extract, and glucose (PYG) medium (Liu et al., 2012) and routinely incubated at 15°C.
Phylogenetic and phylogenomic analyses
The 16S rRNA gene sequences of the aforementioned strains were retrieved from GenBank using accession numbers (Table 1; Liu et al., 2020a) and compared with the latest GenBank database using BLAST+ software (Camacho et al., 2009) to search their close phylogenetic neighbors. The complete 16S rRNA gene sequences were also retrieved from the genome sequences using RNAmmer (Lagesen et al., 2007) for phylogenetic analysis. After multiple sequence alignment using ClustalW (Thompson et al., 1994), the neighbor-joining tree was built with 1,000 bootstrap replicates using the Molecular Evolutionary Genetics Analysis V.5.2 software (Tamura et al., 2011). Kimura's two-parameter model was used to calculate the genetic distances (Kimura, 1980).
Single-copy core genes were extracted from the genome sequences using the GET_HOMOLOGUES program (Contreras-Moreira and Vinuesa, 2013). The phylogenetic markers were selected using the GET_PHYLOMARKERS program for constructing species trees (Vinuesa et al., 2018). The concatenated sequences of the top-ranking phylogenetic marker genes were aligned using Clustal Omega software (Sievers and Higgins, 2014). The phylogenomic tree was constructed using the maximum-likelihood algorithm with a GTR+F+I+G4 model in the IQ-TREE software (Nguyen et al., 2015) based on the concatenated gene sequences with 1,000 bootstrap replicates. Blomberg's K (Blomberg et al., 2003) was measured based on 1,000 randomizations using the “phytools” package in R 4.0.3 to test the phylogenetic signal of the Tmax trait. Blomberg's K values were tested for the null hypothesis that the trait values were randomly distributed in the phylogenetic tree.
Physiology and chemotaxonomy
The 19 Cryobacterium strains were subjected to polyphasic taxonomic analysis. The morphology of the colonies was determined after culturing on PYG agar for 7 days. The cellular morphology was examined via transmission electron microscopy using a JEM-1400 transmission electron microscope (JEOL Ltd., Tokyo, Japan). The growth at various NaCl concentrations [0–4.0% (w/v) at 0.5% intervals] and pH levels (ranging from pH 5.0 to 10.0 at intervals of 1 pH unit) were tested in the presence of PYG broth for 10 days. The hydrolysis of casein, starch, and Tween 80 was performed according to Smibert and Krieg (1994). The agar plates for the starch hydrolysis test were flooded with iodine solution after growth. The colorless areas around the colonies were observed for determining casein and starch hydrolysis. Lipolysis was tested on PYG agar with 1% (v/v) Tween 80 by observing the formation of an oily iridescent sheen and its vicinity on the surface of the medium. The use of a sole carbon source was tested with a GEN III MicroStation (Biolog). The enzyme activities and other biochemical tests were performed using the API 20E, 20NE, and ZYM strips (bioMérieux; Marcy-l'Étoile, France) following the manufacturer's protocols.
For analyzing the cellular fatty acid composition, the cells of the tested strains were harvested from colonies on the same sectors of the PYG plates after incubation at 15°C. The saponified and methylated fatty acids were extracted according to the MIDI 6.0 protocol (Sasser, 1990). The samples were analyzed using an Agilent 6890N gas chromatography system (Agilent Technologies, CA, USA) equipped with an HP-ULTRA two-capillary column (25 m × 0.2 mm × 0.33 μm; Agilent) and a flame ionization detector (Agilent) and identified using the TSBA6 database of the Microbial Identification System (Sherlock version 6.0B). The cells were harvested from PYG broth after incubation at 15°C for 4 days and freeze-dried for analyzing menaquinones and polar lipids. Menaquinones were extracted and purified based on the method of Collins (1985) and analyzed by HPLC using a YMC-Pack ODS-A column (150 × 4.6 mm2) with the elution of methanol/isopropyl ether (3:1, v/v) at a flow rate of 1 ml/min, followed by the detection of ultraviolet absorbance at a wavelength of 275 nm. Polar lipids were extracted and separated on silica gel 60 plates (Merck 1.05553) via two-dimensional thin-layer chromatography (Komagata and Suzuki, 1988).
Results
Diversity of Cryobacterium
At present, 120 genome sequences are temporarily classified into the genus Cryobacterium in GenBank. After removing duplicate strains and metagenome assembly samples, 103 genomes were downloaded using the BioSAK tool (Supplementary Table 1). The completeness of all the genome sequences was above 96%, of which, the completeness of 102 genomes was above 98%. Based on the GTDB Release R207_v2 database, 101 strains were classified into the genus Cryobacterium, and the other two strains BB307 and BB736 were classified into the genus Terrimesophilobacter (Supplementary Table 1). The 101 strains could be classified into 44 species according to the threshold for species delineation of 96% (Richter and Rosselló-Móra, 2009) and the FigANI value cluster analysis (Figure 1). It indicates that at least 44 species of Cryobacterium have been isolated and cultured to date. As only 14 species with validly published names exist at present, at least 30 new species need to be classified and described. In addition, the cluster diagram of ANI values showed higher phylogenetic diversity of the genus Cryobacterium than in a previous study by Liu et al. (2020a); some species showed intraspecific diversity. For example, one unknown species contained 15 strains; Cryobacterium zongtaii contained 11 strains, which were clustered into three branches; and Cryobacterium levitaulinum contained seven strains and was divided into three branches (Figure 1).
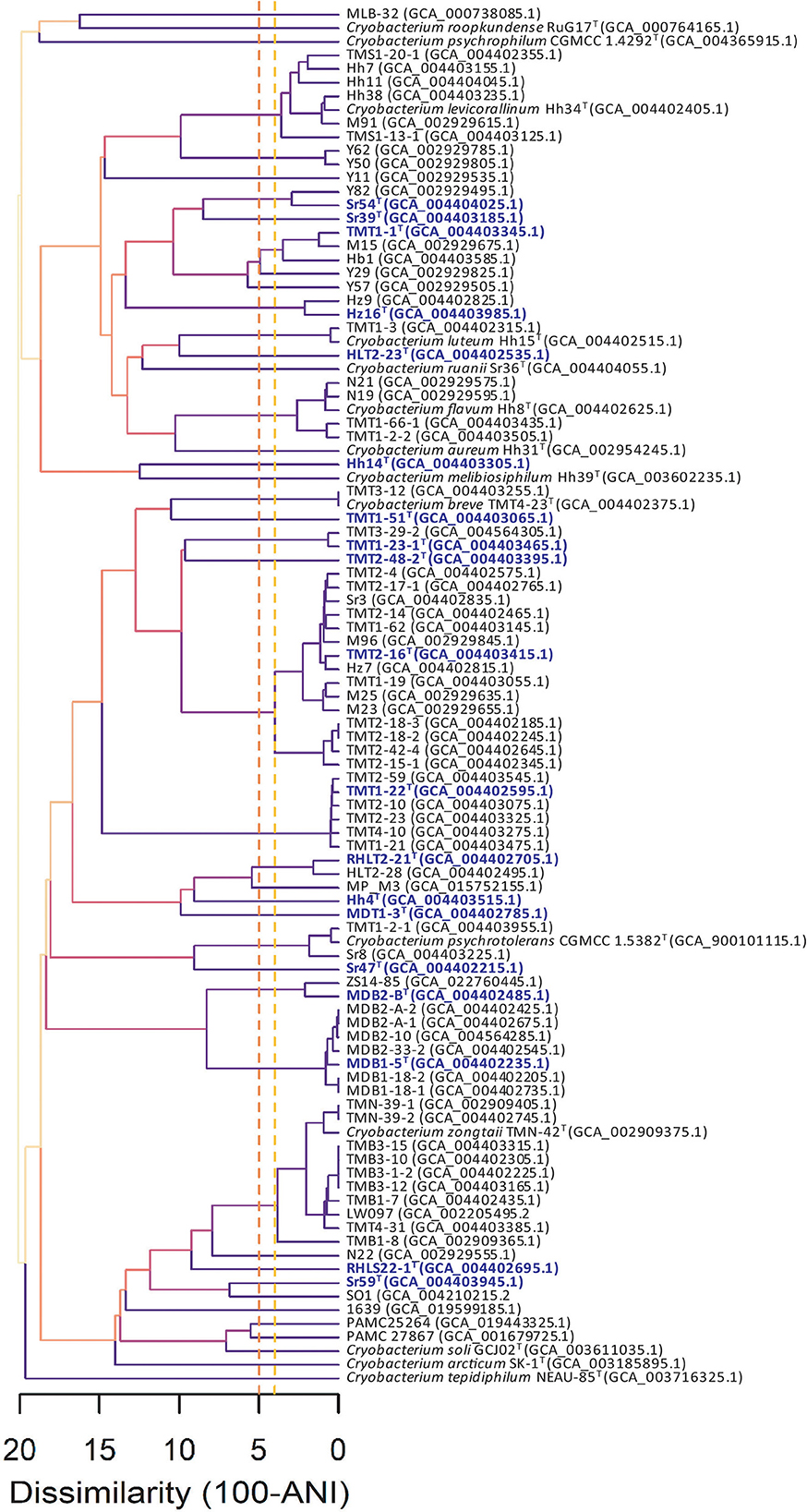
Figure 1. Cluster analysis based on pairwise ANI values of 101 Cryobacterium strains. Accession numbers of the genomic sequences are given in parentheses. Nineteen strains representing the novel species described in this study are in purple color.
Comparative genomic analysis
Among the 101 strains, the Tmax trait of 79 strains was available. These strains were divided into SP and PT groups for comparative genomic analysis based on Tmax (Supplementary Table 1). The gene content showed differences between the two groups (Figure 2). Compared with the PT group, the proportions in the SP group related to “amino acid transport and metabolism,” “lipid transport and metabolism,” “energy production and conversion,” “secondary metabolite biosynthesis, transport, and catabolism,” “intracellular trafficking, secretion, and vesicular transport,” “extracellular structures,” “mobilome: prophages and transposons,” “cell motility,” and “cell cycle control, cell division, and chromosome partitioning” were significantly higher (P < 0.05); however, the proportions of “carbohydrate transport and metabolism,” “general function prediction only,” “signal transduction mechanisms,” “coenzyme transport and metabolism,” “transcription,” and “function unknown” were significantly lower (P < 0.05).
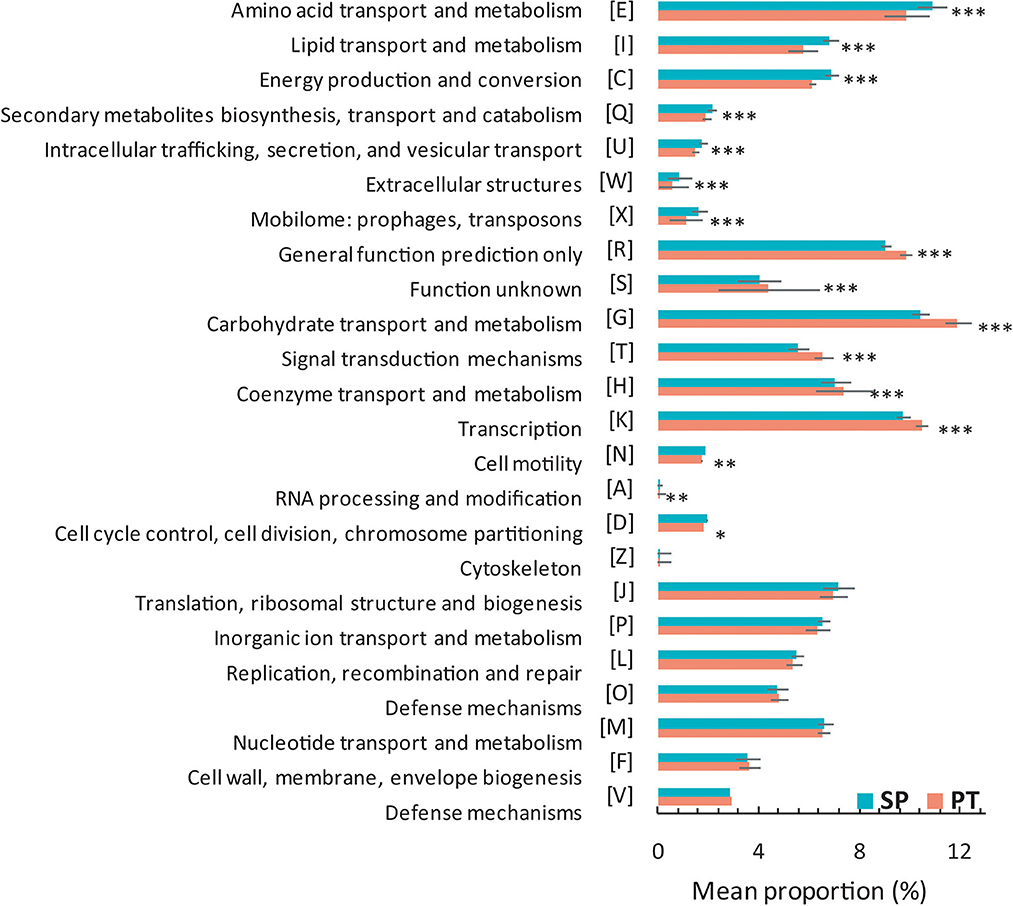
Figure 2. Proportional differences of COG category between the SP and PT groups. Two groups were defined by the strains with Tmax ≤ 20°C (SP) and Tmax > 20°C (PT). P-values were obtained using the Student's t-test. ***P < 0.005; **P = 0.005–0.01; *P = 0.01–0.05.
We compared the numbers of genes of CAZyme families using the CAZy database to estimate the ability to assemble, modify, and break down carbohydrates and glycoconjugates between SP and PT groups. The differences in the predicted CAZyme profiles were found between SP and PT groups (Figure 3). The number of enzymes involved in glycoside hydrolase (GH) and carbohydrate esterase (CE) was significantly lower in the SP group compared with the PT group (P < 0.005); meanwhile, the number of enzymes involved in auxiliary activities (AAs) and carbohydrate-binding modules (CBMs) was also significantly lower (P < 0.05).
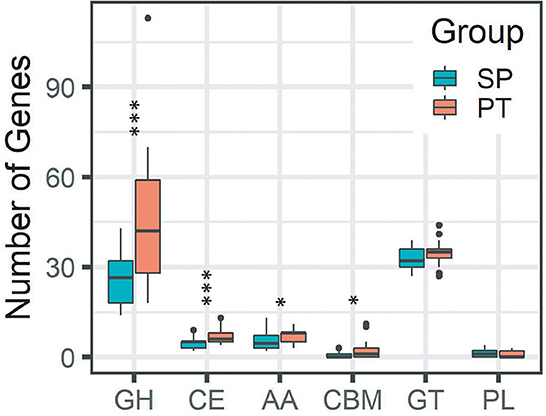
Figure 3. Differences in the predicted CAZyme profiles between the SP and PT groups. Two groups were defined by the strains with Tmax ≤ 20°C (SP) and Tmax > 20°C (PT). ***P < 0.005; *P = 0.01–0.05. Enzyme classes: (GH), glycoside hydrolases; (CE), carbohydrate esterases; (AA), auxiliary activities; (CBM),carbohydrate-binding modules; glycosyltransferases, and (PL), polysaccharide lyases.
Phylogenetic and phylogenomic analyses
Among the 30 new species found in this study, 19 were isolated from the glacier samples in Western China. Furthermore, 19 Cryobacterium strains were selected as type strains and deposited into two publicly accessible service collections for further phylogenetic and phylogenomic studies (Table 1). The symmetric pairwise ANI values between the 19 new type strains and 14 type strains of the known species ranged from 78.58 to 91.76%. Therefore, they represented 19 novel species belonging to the genus Cryobacterium.
The complete 16S rRNA gene sequences of the 33 type strains were also retrieved from the genome sequences. All Cryobacterium strains contained one copy of the 16S rRNA gene, expect for Cryobacterium arcticum SK-1T, which contained three copies. The phylogenetic analysis based on 16S rRNA gene sequences showed that all of the 19 new type strains were members of the genus Cryobacterium (Supplementary Figure 1). The sequence similarities of these strains with their respective closest type strains ranged from 98.76 to 100% (Supplementary Table 2). Obviously, it was difficult to differentiate them from their closely related strains using the 16S rRNA gene sequence, as they shared high levels of similarity, exceeding the 98.65% cutoff for species distinction suggested by Kim et al. (2014).
A phylogenomic analysis of the aforementioned strains was carried out to determine their phylogenetic relationship. Furthermore, 479 single-copy core genes were extracted and 265 top-ranking phylogenetic markers were selected from the genomes. Following sequence alignment, the concatenated sequences of phylogenetic markers were used to construct the species tree using the maximum-likelihood method. A robust tree with strong bootstrap support was obtained (Figure 4). The 33 strains of Cryobacterium were clearly separated from each other and grouped into five clusters based on phylogenetic similarity.
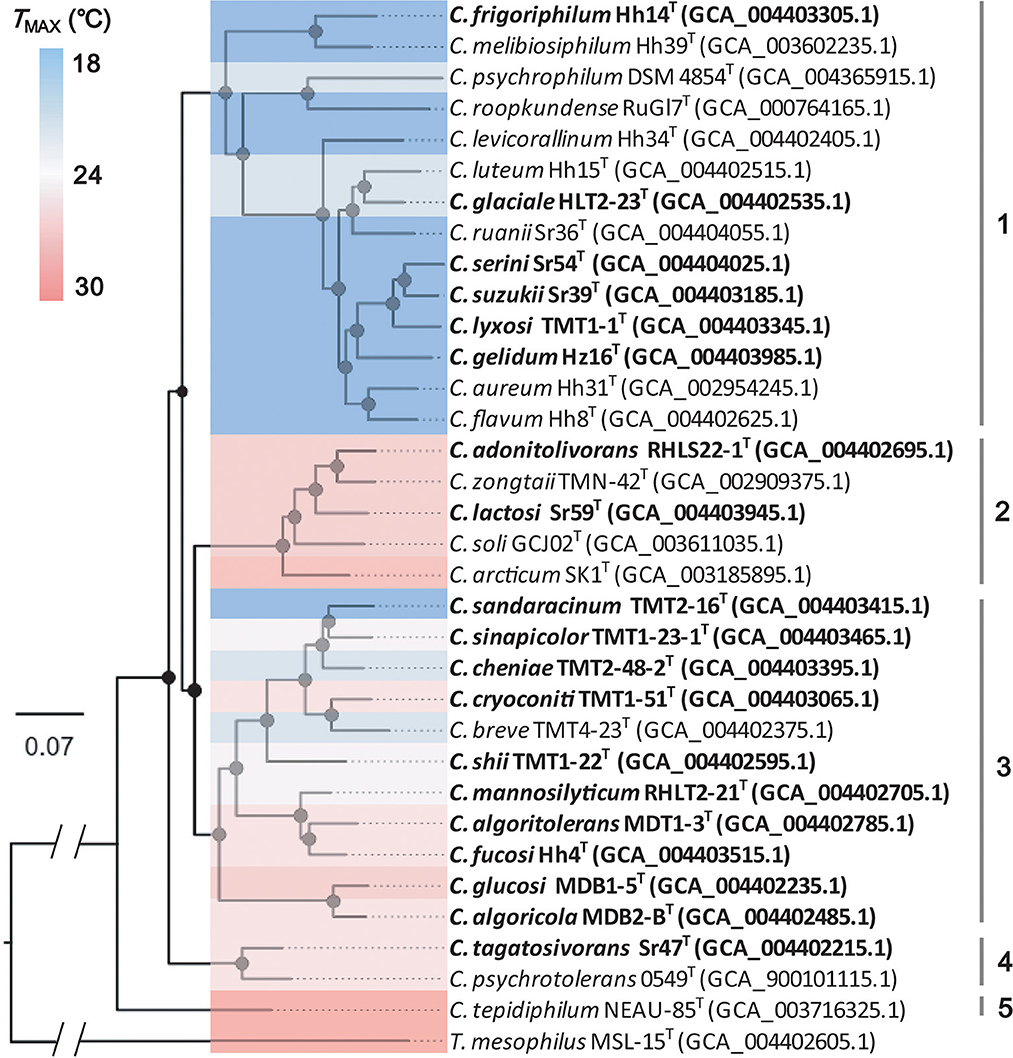
Figure 4. Phylogeny of the genus Cryobacterium using the maximum-likelihood algorithm based on the 265 concatenated single-copy gene sequences. Accession numbers of the genomic sequences are given in parentheses. Bootstrap values >70% are marked as black dots on the internal nodes. The bar represents 0.07 nt substitutions per site.
Cluster 1 consisted of 14 strains, including Hh14T, HLT2-23T, Sr54T, Sr39T, TMT1-1T, Hz16T, and eight type strains of known species with validly published names: Cryobacterium melibiosiphilum Hh39T, Cryobacterium roopkundense RuGl7T, Cryobacterium psychrophilum DSM 4854T, Cryobacterium levicorallinum Hh34T, Cryobacterium luteum Hh15T, Cryobacterium ruanii Sr36T, Cryobacterium aureum Hh31T, and Cryobacterium flavum Hh8T. In total, 11 of these strains were isolated from Xinjiang's No. 1 glacier in China. Strains RHLS22-1T, Sr59T, Cryobacterium zongtaii TMN-42TT, Cryobacterium soli GCJ02T, and C. arcticum SK-1T formed cluster 2. Cluster 3 comprised 11 strains, including TMT2-16T, TMT1-23-1T, TMT2-48-2T, TMT1-51T, TMT1-22T, RHLT2-21T, MDT1-3T, Hh4T, MDB1-5T, MDB2-BT, and Cryobacterium breve TMT4-23T. Strains Sr47T and Cryobacterium psychrotolerans 0549T clustered together and formed cluster 4. Strain C. tepidiphilum NEAU-85T was separated from other clusters and formed a distinct and independent branch. The species tree based on the 265 single-copy core genes yielded a comprehensive phylogenetic structure of the genus Cryobacterium. All strains in the species tree represented different species, which greatly expanded the species diversity of the genus Cryobacterium. Therefore, we proposed 19 novel species under the genus Cryobacterium based on pairwise ANI values and phylogenetic analysis. The proposed names are listed in Table 1.
The Tmax trait of Cryobacterium species showed large differences between different phylogenetic branches (Figure 4). The psychrophilic species were mainly distributed in cluster 1. We used Blomberg's K to measure the strength of the signal relative to the Brownian motion (BM) model of the Tmax trait to test the evolution of Cryobacterium to temperature. For the Tmax trait, the closely related species tended to exhibit similar phylogenetic signals (Blomberg's K = 3.10; P-value = 0.001). The value of Blomberg's K > 1 indicated that the similarity between closely related species was higher than expected under the BM model (Blomberg et al., 2003), showing the phylogenetic conservation of the Tmax trait and implying that selection played a role in the Tmax trait evolution. This result was consistent with our previous finding related to the adaptive evolution of Cryobacterium strains (Liu et al., 2020a).
Phenotypic and chemotaxonomic characteristics
The phenotypic and chemotaxonomic characteristics of 19 novel species of the genus Cryobacterium were evaluated and showed a phenotypic diversity (Supplementary Tables 3, 4). These strains formed colorful colonies and were found to be Gram-positive, rod-shaped, and catalase-positive bacteria. Cells of 15 strains carried a single flagellum, while no flagellum was detected in the cells of the other four strains (Supplementary Figure 2). They showed growth in a pH range of 6.0/7.0–9.0/10.0, varying with different strains. They could all grow at 0% NaCl concentration, while the maximum NaCl tolerance concentration varied greatly from 1 to 5% (Supplementary Table 3). Several differences were observed in their ability to use a sole carbon source (Supplementary Table 4). The number of carbon sources that these strains could use showed a correlation with their Tmax (R2 = 0.69 and P = 0.001). The strains with higher Tmax could use more carbon sources.
The cellular fatty acid composition of novel strains is shown in Table 2. All strains contained anteiso-C15:0 as the predominant fatty acids, which was consistent with the other members of the genus Cryobacterium (Liu et al., 2018b). Different strains carried different secondary dominant components, such as iso-C15:0, iso-C16:0, anteiso-C17:0, and iso-C17:1 ω5c. Their polar lipid profile included diphosphatidylglycerol, phosphatidylglycerol, one unidentified glycolipid, and one unidentified lipid, which was consistent with the description of the genus Cryobacterium (Liu et al., 2018b). All strains contained multiple menaquinone components. The dominant components of most strains included MK-10 and/or MK-9, and MK-11. However, strains MDT1-3T, RHLT2-21T, and Sr47T contained MK-11 and MK-12 as the primary menaquinones with minor amounts of MK-10. Other detailed phenotypic characteristics of these strains are listed in the species description.
Discussion
Temperature is a crucial ecological factor for microorganisms. Microorganisms have a specific range of temperature tolerance and are constantly affected by changes in the external ambient temperature. A continental-scale study of Cyanobacteria found that temperature was the main factor affecting cyanobacterial populations, and differences were observed in temperature tolerance among populations at different latitudes (Garcia-Pichel et al., 2013). The Spumella morphospecies in different climatic regions showed adaptation to different temperatures, and the temperatures for maximum and optimum growth were significantly correlated with habitat temperature (Boenigk et al., 2006, 2007). Based on the analysis of whole-genome single-nucleotide polymorphisms and chromosomal regions, Ellison et al. (2011) found that temperature at different latitudes led to the genomic divergence of two Neurospora crassa populations and differences in their temperature fitness. Therefore, temperature plays an important role not only in the structure and composition of microbial communities but also in genome variation and evolution.
Cryobacterium is a typical cold-active genus inhabiting the cryosphere. Taxonomic and comparative genomic studies could provide new insights into its adaptive evolution. In this study, we compared the gene content of two groups containing 79 Cryobacterium strains. Although no significant difference was observed in the genome size of these strains in the two groups, several differences in the gene content were observed between the groups. The higher number of genes involved in several COG categories (i.e., “amino acid transport and metabolism,” “lipid transport and metabolism,” “secondary metabolite biosynthesis, transport, and catabolism,” “intracellular trafficking, secretion, and vesicular transport,” “extracellular structures,” “mobilome: prophages and transposons,” “cell motility,” and “cell cycle control, cell division, and chromosome partitioning”) was found in the SP group than in the PT group, indicating that the acquisition of genes belonging to these functions might be related to their Tmax trait. Some genes (i.e., “general function prediction only” and “function unknown”) were less abundant in the SP group than in the PT group, which might not be necessary for survival. The loss of some nonessential genes in the SP group might help them save energy in a cold environment. As reported by Liu et al. (2020), gene gain/loss events occurred frequently in the genome of Cryobacterium. These two evolutionary processes might be proactive processes leading to the niche differentiation of Cryobacterium. Interestingly, the number of genes related to carbohydrate transport and metabolism was significantly lower in the SP group than in the PT group (Figure 2). Carbohydrate metabolism is central to whole biological metabolism, mainly including synthesis, decomposition, modification, and other processes, which require enzymes with different functions. The CAZymes classified into families GH and CE were involved in carbohydrate degradation (Wardman et al., 2022). The amounts of GH and CE in the SP group were significantly lower (Figure 3), suggesting that the ability of the SP group to metabolize carbohydrates might be reduced. This finding was consistent with the results of phenotypic experiments, which showed that the strains with lower Tmax could use fewer carbon sources. However, whether this difference in carbon source utilization capacity has a causal relationship with the Tmax remains to be further explored.
In this study, we also inferred that the genus Cryobacterium contains at least 44 species based on genome sequences of pure cultures in the NCBI database, including 19 species isolated from glaciers in Western China. We proposed them as 19 novel species using the polyphasic taxonomic method combined with phylogenomic analysis. Therefore, the number of species with validly published names under this genus was expanded from 14 to 33, which significantly increased our understanding of the species diversity of Cryobacterium. The species in the genus Cryobacterium showed phenotypic diversity, such as the difference in their maximum NaCl tolerance concentrations. The salt concentration in the microenvironment changed during the freeze–thaw cycles in the supraglacial zone, resulting in variations in osmotic pressure. Cryobacterium achieved the adaptation ability at different NaCl concentrations perhaps for this reason. The Tmax of Cryobacterium species also showed large-scale differences among different species. All species collected from the cryosphere (32 species) showed strong cold adaptability (growing at 0°C), which included 16 species with a Tmax of ≤ 20°C and other species that failed to grow at a temperature of more than 26°C. Blomberg's K values were tested for the null hypothesis of the absence of the phylogenetic signal, which fit the model of neutral evolution. According to Blomberg et al. (2003), K values >1 indicated that the related species resembled each other more than expected under BM, implying that the Tmax trait was phylogenetic conservation and affected by selection rather than by neutral drift. The codon usage bias (Liu et al., 2020a) and gene content changed under low-temperature selection pressure, resulting in the diversification of species and differences in temperature adaptability. Therefore, we inferred that under the selective pressure of low-temperature conditions in the cryosphere, Cryobacterium acquired strong adaptability to low temperatures but lost the ability to survive in the external environment at higher temperatures. The dispersal barrier was finally formed due to the ecological differentiation between the cryosphere and the external environment, resulting in Cryobacterium as a specific group in the cryosphere. Hence, Cryobacterium occupied specific ecological niches and formed several novel and distinct species with diverse phenotypes in long-term cold habitats.
Most psychrophilic species were present in cluster 1 of the species tree of the genus Cryobacterium, while the other three appeared in cluster 3. The Cryobacterium species with different Tmax values could be found in the same glacier sample since both the SP and PT groups were well adapted at low temperatures. Therefore, the genome-wide selective sweep (Polz et al., 2013) was less likely to occur in the case of Cryobacterium. However, the low r/m and ρ/θ values within the genus Cryobacterium calculated by Liu et al. (2020a) indicated a low rate of interspecies recombination, suggesting that gene-specific sweeps (Cohan and Koeppel, 2008) also could not explain the cold-adapted evolution of Cryobacterium. The phylogenetic conservation of the Tmax trait implied that the temperature adaptation was mainly through vertical transfer rather than horizontal transfer. Previous studies reported that the adaptation of bacteria to temperature changes involved multiple genes, such as the genes related to the fluidity of the cellular membrane, osmotic and oxidative stress, polysaccharide transporter, ice-binding protein, cold-shock protein, and carotenoid biosynthesis (Casanueva et al., 2010). Therefore, as a multigene trait, temperature adaptation may not be easily acquired through horizontal gene transfer. Hence, we inferred that the transmission of temperature adaptation of Cryobacterium was largely via vertical transfer, resulting in the formation of phylogenetic clustering of temperature adaptation.
Conclusion
In this study, 19 Cryobacterium strains originally isolated from the glaciers in China were classified into 19 novel species using polyphasic taxonomy combined with phylogenomic analysis. Based on 265 single-copy core genes, a comprehensive and robust phylogenetic tree including 33 species belonging to the genus Cryobacterium was constructed with five phylogenetic branches. A comparative genomics analysis of the genus Cryobacterium was performed, indicating significant differences in the gene content between the groups with different Tmax values (≤ 20°C and >20°C). Furthermore, a strong phylogenetic signal of the Tmax trait was detected, suggesting that the temperature adaptation of Cryobacterium was largely via vertical transfer rather than via horizontal transfer and was affected by selection. Hence, the proposal and description of 19 novel species and the comparative genomic analysis enhanced our understanding of the phenotype and genetic diversity of the genus Cryobacterium and also provided some insights into the ecosystem of the supraglacial zone in glaciers.
Description of Cryobacterium serini sp. nov.
Cryobacterium serini (se.ri'ni. N.L. gen. n. serini, of serine, referring to the utilization of D-serine).
Cells are Gram-positive, aerobic, non-motile, rod-shaped, and 1.5–1.7 × 0.7–0.8 μm in size. Cells do not carry any flagella. Colonies are gold-colored, convex, round, and 1.0 mm in diameter after 7 days of incubation on PYG plates at 14°C. Growth occurs at 0–18°C, pH 6.0–10.0, and in the presence of 0–3.0% (w/v) NaCl. The optimum growth temperature is 10–14°C. Positive for catalase, but negative for oxidase. Reduces nitrate to nitrite. Does not hydrolyze Tween 80, gelatin, casein, and starch. Hydrolyze esculine. Indole and H2S are not formed. Positive for alkaline phosphatase, leucine arylamidase, citrate utilization, Voges–Proskauer test, and β-galactosidase. Utilizes the following substances as carbon sources: D-maltose, D-trehalose, D-cellobiose, sucrose, stachyose, α-D-glucose, D-mannose, D-galactose, D-sorbitol, D-arabitol, glycerol, D-serine, gelatin, glycyl-L-proline, L-alanine, L-arginine, L-serine, pectin, D-galacturonic acid, D-gluconic acid, mucic acid, quinic acid, D-saccharic acid, L-lactic acid, citric acid, D-malic acid, L-malic acid, Tween 40, γ-aminobutyric acid, α-hydroxybutyric acid, β-hydroxy-D,L-butyric acid, acetoacetic acid, and acetic acid. Acids are produced from glycerol, fructose, esculine, starch, and D-arabitol. The major fatty acids are anteiso-C15:0, iso-C16:0, iso-C15:0, anteiso-C17:0, and anteiso-C15:1. The predominant menaquinones include MK-10 and MK-9, with minor amounts of MK-8. The polar lipids are diphosphatidylglycerol, phosphatidylglycerol, one unidentified glycolipid, and one unidentified lipid. The DNA G+C content of the type strain is 63.79 %.
The type strain, Sr54T (=CGMCC 1.9249T = NBRC 114034T), was isolated from the ice sample obtained from the supraglacial zone of No. 1 glacier in Xinjiang Uygur Autonomous Region, China. The NCBI accession numbers for the 16S rRNA gene and genome sequences are JX949302 and GCA_004404025.1, respectively.
Description of Cryobacterium lactosi sp. nov.
Cryobacterium lactosi (lac.to'si. N.L. gen. n. lactosi, of lactose).
Cells are Gram-positive, aerobic, motile with a single flagellum, rod-shaped, and 1.3–1.9 × 0.7–0.8 μm in size. Colonies are citrine-colored, convex, round, and 1.0 mm in diameter after 7 days of incubation on PYG plates at 14°C. Growth occurs at 0–26°C, pH 6.0–10.0, and in the presence of 0–5.0% (w/v) NaCl. The optimum growth temperature is 14–20°C. Positive for catalase, but negative for oxidase. Does not reduce nitrate to nitrite. Does not hydrolyze Tween 80, gelatin, casein, and starch. Hydrolyze esculine. Indole and H2S are not formed. Positive for leucine arylamidase, β-galactosidase, α-glucosidase, β-glucosidase, and Voges–Proskauer test. Utilizes the following substances as carbon sources: dextrin, D-maltose, D-trehalose, D-cellobiose, gentiobiose, sucrose, D-turanose, stachyose, D-raffinose, α-D-lactose, D-melibiose, β-methyl-D-glucoside, D-salicin, N-acetyl-D-glucosamine, N-acetyl-β-D-mannosamine, N-acetyl-D-galactosamine, N-acetyl neuraminic acid, α-D-glucose, D-mannose, D-fructose, D-galactose, D-fucose, L-rhamnose, inosine, D-sorbitol, D-mannitol, glycerol, glycyl-L-proline, L-alanine, L-aspartic acid, L-glutamic acid, L-histidine, pectin, D-galacturonic acid, L-galactonic acid lactone, D-gluconic acid, D-glucuronic acid, methyl pyruvate, L-lactic acid, citric acid, D-malic acid, L-malic acid, bromosuccinic acid, Tween 40, α-hydroxybutyric acid, β-hydroxy-D,L-butyric acid, α-keto-butyric acid, propionic acid, acetic acid, and formic acid. Acids are produced from glycerol, L-arabinose, D-ribose, D-xylose, galactose, glucose, fructose, mannose, mannitol, methyl-α-D-glucopyranoside, N-acetylglucosamine, esculine, D-cellobiose, D-maltose, D-lactose, D-melibiose, D-saccharose, D-trehalose, D-melezitose, D-raffinose, D-fucose, and L-arabitol. The major fatty acids are anteiso-C15:0, iso-C16:0, anteiso-C15:1, and anteiso-C17:0. The predominant menaquinone includes MK-10, with minor amounts of MK-9 and MK-11. The polar lipids are diphosphatidylglycerol, phosphatidylglycerol, one unidentified glycolipid, and one unidentified lipid. The DNA G+C content of the type strain is 67.55%.
The type strain, Sr59T (=CGMCC 1.9254T = NBRC 114035T), was isolated from the ice sample obtained from the supraglacial zone of No. 1 glacier in Xinjiang Uygur Autonomous Region, China. The NCBI accession numbers for the 16S rRNA gene and genome sequences are JX949303 and GCA_004403945.1, respectively.
Description of Cryobacterium gelidum sp. nov.
Cryobacterium gelidum (ge'li.dum. L. neut. adj. gelidum, icy cold, very cold).
Cells are Gram-positive, aerobic, motile with a single flagellum, rod-shaped, and 1.1–1.7 × 0.7–0.9 μm in size. Colonies are golden poppy-colored, convex, round, and 2.0 mm in diameter after 7 days of incubation on PYG plates at 14°C. Growth occurs at 0–18°C, pH 7.0–9.0, and in the presence of 0–1.0% (w/v) NaCl. The optimum growth temperature is 10–14°C. Positive for catalase, but negative for oxidase. Does not reduce nitrate to nitrite. Does not hydrolyze Tween 80, gelatin, casein, and starch. Hydrolyze esculine. Indole and H2S are not formed. Positive for alkaline phosphatase, leucine arylamidase, β-galactosidase, and Voges–Proskauer test. Utilizes the following substances as carbon sources: D-maltose, D-cellobiose, sucrose, D-turanose, D-salicin, α-D-glucose, D-mannose, D-fructose, D-galactose, L-fucose, L-rhamnose, inosine, D-mannitol, D-arabitol, myo-inositol, glycerol, glycyl-L-proline, L-alanine, L-arginine, L-glutamic acid, L-pyroglutamic acid, D-gluconic acid, L-lactic acid, D-malic acid, L-malic acid, bromosuccinic acid, γ-aminobutyric acid, β-hydroxy-D,L-butyric acid, α-keto-butyric acid, acetoacetic acid, propionic acid, acetic acid, and formic acid. Acids are produced from L-arabinose, D-ribose, L-xylose, glucose, mannose, rhamnose, and esculine. The major fatty acids are anteiso-C15:0, iso-C16:0, anteiso-C17:0, iso-C15:0, and anteiso-C15:1. The predominant menaquinones include MK-9 and MK-10, with minor amounts of MK-8. The polar lipids are diphosphatidylglycerol, phosphatidylglycerol, one unidentified glycolipid, and one unidentified lipid. The DNA G+C content of the type strain is 64.52%.
The type strain, Hz16T (=CGMCC 1.9272T = NBRC 114048T), was isolated from the ice sample obtained from the supraglacial zone of No. 1 glacier in Xinjiang Uygur Autonomous Region, China. The NCBI accession numbers for the 16S rRNA gene and genome sequences are JX949290 and GCA_004403985.1, respectively.
Description of Cryobacterium suzukii sp. nov.
Cryobacterium suzukii (su.zu'ki.i, N.L. gen. masc .n. suzukii of Suzuki, to honor Professor Ken-ichiro Suzuki who proposed the genus Cryobacterium).
Cells are Gram-positive, aerobic, non-motile, rod-shaped, and 1.1–1.6 × 0.5–0.6 μm in size. Cells do not carry any flagella. Colonies are citrine-colored, convex, round, and 1.0 mm in diameter after 7 days of incubation on PYG plates at 14°C. Growth occurs at 0–18°C, pH 7.0–10.0, and in the presence of 0–3.0% (w/v) NaCl. The optimum growth temperature is 10–14°C. Positive for catalase, but negative for oxidase. Reduces nitrate to nitrite. Does not hydrolyze Tween 80, gelatin, casein, and starch. Hydrolyze esculine. Indole and H2S are not formed. Positive for alkaline phosphatase, leucine arylamidase, Voges–Proskauer test, and β-galactosidase. Utilizes the following substances as carbon sources: D-cellobiose, sucrose, N-acetyl-D-glucosamine, α-D-glucose, D-fructose, glycerol, glycyl-L-proline, L-alanine, L-arginine, L-glutamic acid, L-pyroglutamic acid, D-gluconic acid, L-lactic acid, D-malic acid, L-malic acid, α-hydroxybutyric acid, β-hydroxy-D,L-butyric acid, α-keto-butyric acid, propionic acid, acetic acid, and formic acid. Acids are produced from L-arabinose, D-ribose, D-xylose, galactose, glucose, fructose, mannose, N-acetylglucosamine, esculine, D-cellobiose, D-maltose, D-saccharose, D-trehalose, and D-turanose. The major fatty acids are anteiso-C15:0, anteiso-C17:0, iso-C16:0, anteiso-C15:1, and iso-C15:0. The predominant menaquinones include MK-10 and MK-9, with minor amounts of MK-8. The polar lipids are diphosphatidylglycerol, phosphatidylglycerol, one unidentified glycolipid, and one unidentified lipid. The DNA G+C content of the type strain is 64.05%.
The type strain, Sr39T (=CGMCC 1.9276T = NBRC 114032T), was isolated from the ice sample obtained from the supraglacial zone of No. 1 glacier in Xinjiang Uygur Autonomous Region, China. The NCBI accession numbers for the 16S rRNA gene and genome sequences are JX949299 and GCA_004403185.1, respectively.
Description of Cryobacterium fucosi sp. nov.
Cryobacterium fucosi (fu.co'si. N.L. gen. n. fucosi, of fucose).
Cells are Gram-positive, aerobic, motile with a single flagellum, rod-shaped, and 1.0–1.7 × 0.4–0.6 μm in size. Colonies are citrine-colored, convex, round, and 2.0 mm in diameter after 7 days of incubation on PYG plates at 14°C. Growth occurs at 0–24°C, pH 6.0–10.0, and in the presence of 0–2.0% (w/v) NaCl. The optimum growth temperature is 14–20°C. Positive for catalase, but negative for oxidase. Reduces nitrate to nitrite. Does not hydrolyze Tween 80, gelatin, casein, and starch. Hydrolyze esculine. Indole and H2S are not formed. Positive for leucine arylamidase, α-glucosidase, arginine dihydrolase, Voges–Proskauer test, and β-galactosidase. Utilizes the following substances as carbon sources: dextrin, D-maltose, D-trehalose, D-cellobiose, gentiobiose, sucrose, D-turanose, α-D-lactose, D-melibiose, D-salicin, N-acetyl-D-glucosamine, α-D-glucose, D-mannose, D-fructose, D-galactose, inosine, D-mannitol, glycerol, D-fructose-6-PO4, L-alanine, L-arginine, L-glutamic acid, L-histidine, L-serine, D-gluconic acid, p-hydroxyphenylacetic acid, L-lactic acid, Tween 40, γ-aminobutyric acid, α-hydroxybutyric acid, propionic acid, and acetic acid. Acids are produced from L-arabinose, D-ribose, D-xylose, galactose, glucose, fructose, mannose, mannitol, N-acetylglucosamine, D-cellobiose, D-maltose, D-saccharose, D-trehalose, starch, glycogene, and gentiobiose. The major fatty acids are anteiso-C15:0, iso-C16:0, anteiso-C15:1, and anteiso-C17:0. The predominant menaquinones include MK-11, MK-12, and MK-10. The polar lipids are diphosphatidylglycerol, phosphatidylglycerol, one unidentified glycolipid, and one unidentified lipid. The DNA G+C content of the type strain is 68.04%.
The type strain, Hh4T (=CGMCC 1.9290T = NBRC 114036T), was isolated from the ice sample obtained from the supraglacial zone of No. 1 glacier in Xinjiang Uygur Autonomous Region, China. The NCBI accession numbers for the 16S rRNA gene and genome sequences are JX949272 and GCA_004403515.1, respectively.
Description of Cryobacterium frigoriphilum sp. nov.
Cryobacterium frigoriphilum (fri.go.ri'phi.lum. L. neut. n. frigor, the cold; N.L. masc. adj. philus (from Gr. masc. adj. philos), loving; N.L. neut. adj. frigoriphilum, cold-loving).
Cells are Gram-positive, aerobic, non-motile, rod-shaped, and 1.5–1.8 × 0.7–0.9 μm in size. Cells do not carry any flagella. Colonies are naples yellow-colored, convex, round, and 2.0 mm in diameter after 7 days of incubation on PYG plates at 14°C. Growth occurs at 0–18°C, pH 7.0–10.0, and in the presence of 0–3.0% (w/v) NaCl. The optimum growth temperature is 10–14°C. Positive for catalase and oxidase. Reduces nitrate to nitrite. Does not hydrolyze Tween 80, gelatin, casein, and starch. Hydrolyze esculine. Indole and H2S are not formed. Positive for alkaline phosphatase, esterase lipase (C8), leucine arylamidase, acid phosphatase, α-glucosidase, β-glucosidase, Voges–Proskauer test, and β-galactosidase. Utilizes the following substances as carbon sources: D-cellobiose, gentiobiose, β-methyl-D-glucoside, D-salicin, α-D-glucose, D-mannose, D-fructose, D-galactose, inosine, D-sorbitol, D-mannitol, D-arabitol, myo-inositol, glycerol, L-alanine, L-pyroglutamic acid, D-galacturonic acid, L-galactonic acid lactone, D-gluconic acid, D-glucuronic acid, L-lactic acid, citric acid, L-malic acid, α-hydroxybutyric acid, β-hydroxy-D,L-butyric acid, propionic acid, and acetic acid. Acids are produced from galactose, mannose, and esculine. The major fatty acids are anteiso-C15:0, iso-C16:0, iso-C15:0, anteiso-C17:0, and anteiso-C15:1. The predominant menaquinone includes MK-10, with minor amounts of MK-9 and MK-11. The polar lipids are diphosphatidylglycerol, phosphatidylglycerol, one unidentified glycolipid, and one unidentified lipid. The DNA G+C content of the type strain is 66.74%.
The type strain, Hh14T (=CGMCC 1.9297T = NBRC 114037T), was isolated from the ice sample obtained from the supraglacial zone of No. 1 glacier in Xinjiang Uygur Autonomous Region, China. The NCBI accession numbers for the 16S rRNA gene and genome sequences are JX949277 and GCA_004403305.1, respectively.
Description of Cryobacterium cryoconiti sp. nov.
Cryobacterium cryoconiti (cry.o.co.ni'ti. N.L. gen. n. cryoconiti, isolated from cryoconite).
Cells are Gram-positive, aerobic, motile with a single flagellum, rod-shaped, and 1.5–2.1 × 0.6–0.8 μm in size. Colonies are yellow-colored, convex, round, and 1.0 mm in diameter after 7 days of incubation on PYG plates at 14°C. Growth occurs at 0–24°C, pH 7.0–10.0, and in the presence of 0–4.0% (w/v) NaCl. The optimum growth temperature is 14–20°C. Positive for catalase, but negative for oxidase. Reduces nitrate to nitrite. Does not hydrolyze Tween 80, gelatin, casein, and starch. Hydrolyze esculine. Indole and H2S are not formed. Positive for leucine arylamidase, α-glucosidase, Voges–Proskauer test, and β-galactosidase. Utilizes the following substances as carbon sources: dextrin, D-maltose, D-trehalose, D-cellobiose, gentiobiose, sucrose, D-turanose, stachyose, D-raffinose, α-D-lactose, D-melibiose, α-D-glucose, D-mannose, D-fructose, D-galactose, inosine, glycerol, L-alanine, L-arginine, L-glutamic acid, L-histidine, D-gluconic acid, p-hydroxyphenylacetic acid, L-lactic acid, L-malic acid, bromosuccinic acid, Tween 40, γ-aminobutyric acid, α-hydroxybutyric acid, β-hydroxy-D,L-butyric acid, propionic acid, acetic acid, and formic acid. Acids are produced from galactose, glucose, fructose, mannose, D-maltose, D-melibiose, D-saccharose, and D-raffinose. The major fatty acids are anteiso-C15:0, iso-C16:0, C16:0, anteiso-C15:1, anteiso-C17:0, and iso-C15:1. The predominant menaquinones include MK-10 and MK-11. The polar lipids are diphosphatidylglycerol, phosphatidylglycerol, one unidentified glycolipid, and one unidentified lipid. The DNA G+C content of the type strain is 67.76%.
The type strain, TMT1-51T (=CGMCC 1.9350T = NBRC 114038T), was isolated from the cryoconite sample obtained from the supraglacial zone of Toumingmengke glacier in Gansu province, China. The NCBI accession numbers for the 16S rRNA gene and genome sequences are JX949903 and GCA_004403065.1, respectively.
Description of Cryobacterium lyxosi sp. nov.
Cryobacterium lyxosi (ly.xo'si. N.L. gen. n. lyxosi, of lyxose).
Cells are Gram-positive, aerobic, motile with a single flagellum, rod-shaped, and 1.2–2.0 × 0.5–0.7 μm in size. Colonies are gold yellow-colored, convex, round, and 1.0 mm in diameter after 7 days of incubation on PYG plates at 14°C. Growth occurs at 0–18°C, pH 7.0–9.0, and in the presence of 0–2.0% (w/v) NaCl. The optimum growth temperature is 10–14°C. Positive for catalase, but negative for oxidase. Does not reduce nitrate to nitrite. Does not hydrolyze Tween 80, gelatin, casein, and starch. Hydrolyze esculine. Indole and H2S are not formed. Positive for alkaline phosphatase, leucine arylamidase, α-glucosidase, Voges–Proskauer test, and β-galactosidase. Utilizes the following substances as carbon sources: dextrin, D-maltose, D-trehalose, D-salicin, α-D-glucose, D-mannose, D-fructose, D-galactose, inosine, D-mannitol, D-arabitol, L-pyroglutamic acid, D-gluconic acid, D-saccharic acid, L-lactic acid, D-malic acid, L-malic acid, bromosuccinic acid, acetoacetic acid, propionic acid, acetic acid, and formic acid. Acids are produced from glycerol, glucose, fructose, mannitol, esculine, D-cellobiose, D-saccharose, D-turanose, and D-lyxose. The major fatty acids are anteiso-C15:0, iso-C16:0, anteiso-C17:0, and anteiso-C15:1. The predominant menaquinone includes MK-10, with minor amounts of MK-11 and MK-9. The polar lipids are diphosphatidylglycerol, phosphatidylglycerol, one unidentified glycolipid, and one unidentified lipid. The DNA G+C content of the type strain is 63.51%.
The type strain, TMT1-1T (=CGMCC 1.9465T = NBRC 113798T), was isolated from the cryoconite sample obtained from the supraglacial zone of Toumingmengke glacier in Gansu province, China. The NCBI accession numbers for the 16S rRNA gene and genome sequences are JX949920 and GCA_004403345.1, respectively.
Description of Cryobacterium sinapicolor sp. nov.
Cryobacterium sinapicolor (si.na.pi'co.lor. L. fem. n. sinapis, mustard; L. masc. n. color, color; N.L. neut. adj. sinapicolor, mustard-colored).
Cells are Gram-positive, aerobic, non-motile, rod-shaped, and 1.2–1.8 × 0.6–0.8 μm in size. Cells do not carry any flagella. Colonies are mustard-colored, convex, round, and 1.0 mm in diameter after 7 days of incubation on PYG plates at 14°C. Growth occurs at 0–22°C, pH 7.0–10.0, and in the presence of 0–3.0% (w/v) NaCl. The optimum growth temperature is 10–14°C. Positive for catalase, but negative for oxidase. Reduces nitrate to nitrite. Does not hydrolyze Tween 80, gelatin, casein, and starch. Hydrolyze esculine. Indole and H2S are not formed. Positive for leucine arylamidase, arginine dihydrolase, Voges–Proskauer test, and β-galactosidase. Utilizes the following substances as carbon sources: dextrin, D-maltose, D-trehalose, D-cellobiose, gentiobiose, sucrose, α-D-lactose, β-methyl-D-glucoside, D-salicin, N-acetyl-D-glucosamine, N-acetyl-β-D-mannosamine, α-D-glucose, D-mannose, D-fructose, D-galactose, L-rhamnose, inosine, glycerol, L-alanine, L-arginine, L-glutamic acid, L-histidine, L-serine, p-hydroxyphenylacetic acid, methyl pyruvate, L-lactic acid, D-malic acid, L-malic acid, bromosuccinic acid, Tween 40, γ-aminobutyric acid, α-hydroxybutyric acid, acetoacetic acid, propionic acid, and acetic acid. Acids are produced from galactose, glucose, fructose, mannose, rhamnose, esculine, D-cellobiose, D-maltose, starch, and glycogene. The major fatty acids are anteiso-C15:0, iso-C16:0, iso-C17:1 ω5c, anteiso-C17:0, anteiso-C15:1, and anteiso-C16:0. The predominant menaquinone includes MK-10, with minor amounts of MK-11 and MK-9. The polar lipids are diphosphatidylglycerol, phosphatidylglycerol, one unidentified glycolipid, and one unidentified lipid. The DNA G+C content of the type strain is 67.20%.
The type strain, TMT1-23-1T (=CGMCC 1.9483T = NBRC 113799T), was isolated from the cryoconite sample obtained from the supraglacial zone of Toumingmengke glacier in Gansu province, China. The NCBI accession numbers for the 16S rRNA gene and genome sequences are JX949927 and GCA_004403465.1, respectively.
Description of Cryobacterium sandaracinum sp. nov.
Cryobacterium sandaracinum (san.da.ra.ci'num. Gr. masc. adj. sandarakinos, orange; N.L. neut. adj. sandarakinum, orange).
Cells are Gram-positive, aerobic, motile with a single flagellum, rod-shaped, and 1.3–2.1 × 0.6–0.8 μm in size. Colonies are peach orange-colored, convex, round, and 1.0 mm in diameter after 7 days of incubation on PYG plates at 14°C. Growth occurs at 0–18°C, pH 7.0–10.0, and in the presence of 0–3.0% (w/v) NaCl. The optimum growth temperature is 10–14°C. Positive for catalase, but negative for oxidase. Reduces nitrate to nitrite. Does not hydrolyze Tween 80, gelatin, casein, and starch. Hydrolyze esculine. Indole and H2S are not formed. Positive for leucine arylamidase, arginine dihydrolase, citrate utilization, Voges–Proskauer test, and β-galactosidase. Utilizes the following substances as carbon sources: dextrin, D-maltose, D-trehalose, D-cellobiose, sucrose, D-turanose, N-acetyl-D-glucosamine, α-D-glucose, D-mannose, D-fructose, D-galactose, 3-methyl glucose, inosine, D-mannitol, glycerol, L-alanine, L-arginine, L-glutamic acid, D-gluconic acid, p-hydroxyphenylacetic acid, methyl pyruvate, L-lactic acid, L-malic acid, bromosuccinic acid, Tween 40, γ-aminobutyric acid, α-hydroxybutyric acid, acetoacetic acid, and propionic acid. Acids are produced from D-ribose, galactose, glucose, fructose, mannose, methyl-α-D-mannopyranoside, N-acetylglucosamine, and esculine. The major fatty acids are anteiso-C15:0, iso-C16:0, anteiso-C17:0, anteiso-C15:1, iso-C17:1 ω5c, iso-C15:1, and iso-C16:0. The predominant menaquinone includes MK-10, with minor amounts of MK-11 and MK-9. The polar lipids are diphosphatidylglycerol, phosphatidylglycerol, one unidentified glycolipid, and one unidentified lipid. The DNA G+C content of the type strain is 66.75%.
The type strain, TMT2-16T (=CGMCC 1.9503T = NBRC 114039T), was isolated from the cryoconite sample obtained from the supraglacial zone of Toumingmengke glacier in Gansu province, China. The NCBI accession numbers for the 16S rRNA gene and genome sequences are JX949884 and GCA_004403415.1, respectively.
Description of Cryobacterium cheniae sp. nov.
Cryobacterium cheniae (chen'i.ae. N.L. gen. fem. n. cheniae, of Chen, to honor Professor Wen-Xin Chen, a respected microbiologist, for her great contributions to the investigation and development of Rhizobia resources in China).
Cells are Gram-positive, aerobic, motile with a single flagellum, rod-shaped, and 1.2–2.1 × 0.7–0.8 μm in size. Colonies are yellow-colored, convex, round, and 1.0 mm in diameter after 7 days of incubation on PYG plates at 14°C. Growth occurs at 0–20°C, pH 7.0–9.0, and in the presence of 0–3.0% (w/v) NaCl. The optimum growth temperature is 10–14°C. Positive for catalase, but negative for oxidase. Reduces nitrate to nitrite. Does not hydrolyze Tween 80, gelatin, casein, and starch. Hydrolyze esculine. Indole and H2S are not formed. Positive for leucine arylamidase, α-glucosidase, Voges–Proskauer test, and β-galactosidase. Utilizes the following substances as carbon sources: dextrin, D-maltose, D-trehalose, D-cellobiose, sucrose, D-turanose, α-D-lactose, D-salicin, N-acetyl-D-glucosamine, α-D-glucose, D-mannose, D-fructose, D-galactose, inosine, D-sorbitol, D-mannitol, glycerol, L-alanine, L-arginine, L-glutamic acid, L-histidine, L-serine, pectin, D-gluconic acid, p-hydroxyphenylacetic acid, methyl pyruvate, L-lactic acid, L-malic acid, bromosuccinic acid, Tween 40, γ-aminobutyric acid, α-hydroxybutyric acid, α-keto-butyric acid, acetoacetic acid, propionic acid, acetic acid, and formic acid. Acids are produced from galactose, glucose, fructose, mannose, mannitol, sorbitol, N-acetylglucosamine, D-maltose, D-saccharose, and glycogene. The major fatty acids are anteiso-C15:0, iso-C16:0, anteiso-C17:0, iso-C15:1, anteiso-C15:1, and anteiso-C16:0. The predominant menaquinones include MK-10 and MK-11. The polar lipids are diphosphatidylglycerol, phosphatidylglycerol, one unidentified glycolipid, and one unidentified lipid. The DNA G+C content of the type strain is 67.75%.
The type strain, TMT2-48-2T (=CGMCC 1.9517T = NBRC 114040T), was isolated from the cryoconite sample obtained from the supraglacial zone of Toumingmengke glacier in Gansu province, China. The NCBI accession numbers for the 16S rRNA gene and genome sequences are JX949892 and GCA_004403395.1, respectively.
Description of Cryobacterium shii sp. nov.
Cryobacterium shii (sh.i'i. N.L. gen. n. shii, of Shi, to honor Mr. Ya-Feng Shi, a great geographer and glaciologist, the founder of Glaciology in China).
Cells are Gram-positive, aerobic, motile with a single flagellum, rod-shaped, and 1.2–2.1 × 0.8–0.9 μm in size. Colonies are lemon-colored, convex, round, and 2.0 mm in diameter after 7 days of incubation on PYG plates at 14°C. Growth occurs at 0–22°C, pH 6.0–9.0, and in the presence of 0–3.0 % (w/v) NaCl. The optimum growth temperature is 10–14°C. Positive for catalase, but negative for oxidase. Reduces nitrate to nitrite. Does not hydrolyze Tween 80, gelatin, casein, and starch. Hydrolyze esculine. Indole and H2S are not formed. Positive for leucine arylamidase, acid phosphatase, naphthol-AS-BI-phosphohydrolase, arginine dihydrolase, Voges–Proskauer test, and β-galactosidase. Utilizes the following substances as carbon sources: dextrin, D-maltose, D-trehalose, D-cellobiose, sucrose, D-turanose, α-D-glucose, D-mannose, D-fructose, D-galactose, inosine, D-glucose-6-PO4, D-fructose-6-PO4, L-alanine, L-arginine, L-glutamic acid, L-histidine, L-serine, pectin, D-gluconic acid, p-hydroxyphenylacetic acid, methyl pyruvate, D-lactic acid methyl ester, L-lactic acid, D-malic acid, L-malic acid, bromosuccinic acid, Tween 40, γ-aminobutyric acid, α-hydroxybutyric acid, β-hydroxy-D,L-butyric acid, α-keto-butyric acid, acetoacetic acid, propionic acid, acetic acid, and formic acid. Acids are produced from L-arabinose, galactose, glucose, fructose, mannose, esculine, D-maltose, and D-saccharose. The major fatty acids are anteiso-C15:0, iso-C16:0, anteiso-C15:1, anteiso-C17:0, iso-C15:1, and iso-C16:0. The predominant menaquinones include MK-10 and MK-11. The polar lipids are diphosphatidylglycerol, phosphatidylglycerol, one unidentified glycolipid, and one unidentified lipid. The DNA G+C content of the type strain is 68.14%.
The type strain, TMT1-22T (=CGMCC 1.9687T = NBRC 114041T), was isolated from the cryoconite sample obtained from the supraglacial zone of Toumingmengke glacier in Gansu province, China. The NCBI accession numbers for the 16S rRNA gene and genome sequences are JX949935 and GCA_004402595.1, respectively.
Description of Cryobacterium glucosi sp. nov.
Cryobacterium glucosi (glu.co'si. N.L. gen. n. glucosi, of glucose).
Cells are Gram-positive, aerobic, motile with a single flagellum, rod-shaped, and 1.4–2.0 × 0.5–0.6 μm in size. Colonies are cream-colored, convex, round, and 1.0 mm in diameter after 7 days of incubation on PYG plates at 14°C. Growth occurs at 0–26°C, pH 5.0–8.0, and in the presence of 0–3.0% (w/v) NaCl. The optimum growth temperature is 14–20°C. Positive for catalase, but negative for oxidase. Reduces nitrate to nitrite. Does not hydrolyze Tween 80, gelatin, casein, and starch. Hydrolyze esculine. Indole and H2S are not formed. Positive for alkaline phosphatase, leucine arylamidase, acid phosphatase, α-galactosidase, β-galactosidase, α-glucosidase, β-glucosidase, arginine dihydrolase, Voges–Proskauer test, and fermentation of glucose. Utilizes the following substances as carbon sources: dextrin, D-maltose, D-trehalose, D-cellobiose, sucrose, D-turanose, stachyose, D-raffinose, D-melibiose, β-methyl-D-glucoside, D-salicin, N-acetyl-D-glucosamine, N-acetyl-D-galactosamine, α-D-glucose, D-mannose, D-fructose, D-galactose, L-rhamnose, inosine, D-sorbitol, D-mannitol, glycerol, glycyl-L-proline, L-alanine, L-arginine, L-glutamic acid, L-histidine, pectin, D-galacturonic acid, L-galactonic acid lactone, D-gluconic acid, D-glucuronic acid, p-hydroxyphenylacetic acid, D-lactic acid methyl ester, L-lactic acid, α-ketoglutaric acid, D-malic acid, L-malic acid, Tween 40, γ-aminobutyric acid, α-hydroxybutyric acid, β-hydroxy-D,L-butyric acid, α-keto-butyric acid, acetoacetic acid, propionic acid, and acetic acid. Acids are produced from glycerol, L-arabinose, D-ribose, D-xylose, methyl-β-D-xylopyranoside, galactose, glucose, fructose, mannose, rhamnose, mannitol, sorbitol, N-acetylglucosamine, amygdaline, arbutin, esculine, salicin, D-cellobiose, D-maltose, D-melibiose, D-saccharose, D-trehalose, D-raffinose, and potassium gluconate. The major fatty acids are anteiso-C15:0, anteiso-C17:0, and iso-C16:0. The predominant menaquinones include MK-10 and MK-11, with minor amounts of MK-9. The polar lipids are diphosphatidylglycerol, phosphatidylglycerol, one unidentified glycolipid, and one unidentified lipid. The DNA G+C content of the type strain is 67.68%.
The type strain, MDB1-5T (=CGMCC 1.9741T = NBRC 114042T), was isolated from the ice sample obtained from the supraglacial zone of Midui glacier on the Tibetan Plateau, China. The NCBI accession numbers for the 16S rRNA gene and genome sequences are JX949731 and GCA_004402235.1, respectively.
Description of Cryobacterium algoritolerans sp. nov.
Cryobacterium algoritolerans (al.go.ri.to'le.rans. L. masc. n. algor, the cold; L. pres. part. tolerans, tolerating; N.L. part. adj. algoritolerans, cold tolerating).
Cells are Gram-positive, aerobic, motile with a single flagellum, rod-shaped, and 1.4–3.0 × 0.5–0.6 μm in size. Colonies are tangerine yellow-colored, convex, round, and 2.0 mm in diameter after 7 days of incubation on PYG plates at 14°C. Growth occurs at 0–24°C, pH 6.0–8.0, and in the presence of 0–3.0% (w/v) NaCl. The optimum growth temperature is 14–20°C. Positive for catalase, but negative for oxidase. Reduces nitrate to nitrite. Does not hydrolyze Tween 80, gelatin, and casein. Hydrolyze starch and esculine. Indole and H2S are not formed. Positive for leucine arylamidase, Voges–Proskauer test, and β-galactosidase. Utilizes the following substances as carbon sources: dextrin, D-maltose, D-trehalose, D-cellobiose, gentiobiose, sucrose, D-turanose, D-raffinose, α-D-lactose, D-melibiose, D-salicin, N-acetyl-D-glucosamine, α-D-glucose, D-mannose, D-fructose, D-galactose, inosine, D-mannitol, D-arabitol, glycerol, glycyl-L-proline, L-alanine, L-arginine, L-glutamic acid, L-histidine, L-serine, pectin, D-gluconic acid, p-hydroxyphenylacetic acid, methyl pyruvate, L-lactic acid, L-malic acid, bromosuccinic acid, Tween 40, α-hydroxybutyric acid, β-hydroxy-D,L-butyric acid, α-keto-butyric acid, propionic acid, acetic acid, and formic acid. Acids are produced from glycerol, L-arabinose, D-ribose, galactose, glucose, fructose, mannose, mannitol, N-acetylglucosamine, esculine, D-maltose, D-saccharose, starch, and glycogene. The major fatty acids are anteiso-C15:0, iso-C16:0, anteiso-C17:0, anteiso-C15:1, iso-C15:1, and iso-C16:0. The predominant menaquinones include MK-11 and MK-12, with minor amounts of MK-10. The polar lipids are diphosphatidylglycerol, phosphatidylglycerol, one unidentified glycolipid, and one unidentified lipid. The DNA G+C content of the type strain is 67.01%.
The type strain, MDT1-3T (=CGMCC 1.9782T = NBRC 114043T), was isolated from the cryoconite sample obtained from the supraglacial zone of Midui glacier on the Tibetan Plateau, China. The NCBI accession numbers for the 16S rRNA gene and genome sequences are JX949739 and GCA_004402785.1, respectively.
Description of Cryobacterium mannosilyticum sp. nov.
Cryobacterium mannosilyticum (man.no.si.ly'ti.cum. N.L. neut. n. mannosum, mannose; N.L. masc. adj. lyticus (from Gr. masc. adj. lytikos) dissolving; N.L. neut. adj. mannosilyticum, mannose dissolving).
Cells are Gram-positive, aerobic, motile with a single flagellum, rod-shaped, and 1.1–3.4 × 0.5–0.7 μm in size. Colonies are yellow-colored, convex, round, and 2.0 mm in diameter after 7 days of incubation on PYG plates at 14°C. Growth occurs at 0–22°C, pH 5.0–9.0, and in the presence of 0–3.0 % (w/v) NaCl. The optimum growth temperature is 10–14°C. Positive for catalase, but negative for oxidase. Reduces nitrate to nitrite. Does not hydrolyze Tween 80, gelatin, casein, and starch. Hydrolyze esculine. Indole and H2S are not formed. Positive for alkaline phosphatase, leucine arylamidase, acid phosphatase, α-glucosidase, α-mannosidase, β-galactosidase, and Voges–Proskauer test. Utilizes the following substances as carbon sources: dextrin, D-maltose, D-trehalose, D-cellobiose, gentiobiose, sucrose, D-turanose, N-acetyl-D-glucosamine, α-D-glucose, D-mannose, D-fructose, D-galactose, inosine, D-sorbitol, glycerol, L-arginine, L-glutamic acid, D-gluconic acid, L-lactic acid, L-malic acid, β-hydroxy-D,L-butyric acid, propionic acid, and acetic acid. Acids are produced from glycerol, L-arabinose, D-ribose, D-xylose, galactose, glucose, fructose, mannose, sorbitol, methyl-α-D-mannopyranoside, esculine, D-maltose, D-saccharose, and D-trehalose. The major fatty acids are anteiso-C15:0, iso-C16:0, anteiso-C17:0, anteiso-C16:0, and anteiso-C15:1. The predominant menaquinones include MK-11 and MK-12, with minor amounts of MK-10. The polar lipids are diphosphatidylglycerol, phosphatidylglycerol, one unidentified glycolipid, and one unidentified lipid. The DNA G+C content of the type strain is 68.63%.
The type strain, RHLT2-21T (=CGMCC 1.10060T = NBRC 114044T), was isolated from the cryoconite sample obtained from the supraglacial zone of Hailuogou glacier in Sichuan province, China. The NCBI accession numbers for the 16S rRNA gene and genome sequences are JX949475 and GCA_004402705.1, respectively.
Description of Cryobacterium adonitolivorans sp. nov.
Cryobacterium adonitolivorans (a.do.ni.to.li.vo'rans. N.L. neut. n. adonitol, adonitol; L. pres. part. vorans, devouring; N.L. part. adj. adonitolivorans, adonitol-devouring).
Cells are Gram-positive, aerobic, motile with a single flagellum, rod-shaped, and 0.9–1.7 × 0.7–0.8 μm in size. Colonies are lemon yellow-colored, convex, round, and 2.0 mm in diameter after 7 days of incubation on PYG plates at 14°C. Growth occurs at 0–26°C, pH 6.0–10.0, and in the presence of 0–5.0% (w/v) NaCl. The optimum growth temperature is 14–20°C. Positive for catalase, but negative for oxidase. Reduces nitrate to nitrite. Does not hydrolyze Tween 80, gelatin, casein, and starch. Hydrolyze esculine. Indole and H2S are not formed. Positive for alkaline phosphatase, leucine arylamidase, α-glucosidase, β-glucosidase, β-galactosidase, and Voges–Proskauer test. Utilizes the following substances as carbon sources: dextrin, D-maltose, D-trehalose, D-cellobiose, gentiobiose, sucrose, D-turanose, D-salicin, N-acetyl-D-glucosamine, N-acetyl-β-D-mannosamine, α-D-glucose, D-mannose, D-fructose, D-galactose, inosine, D-sorbitol, D-arabitol, glycerol, D-gluconic acid, methyl pyruvate, citric acid, L-malic acid, bromosuccinic acid, propionic acid, and acetic acid. Acids are produced from glycerol, L-arabinose, D-ribose, D-xylose, D-adonitol, galactose, glucose, mannose, mannitol, sorbitol, esculine, D-cellobiose, D-maltose, and D-arabitol. The major fatty acids are anteiso-C15:0, iso-C16:0, anteiso-C17:0, iso-C17:1 ω5c, anteiso-C15:1, and anteiso-C16:0. The predominant menaquinones include MK-10 and MK-9, with minor amounts of MK-11. The polar lipids are diphosphatidylglycerol, phosphatidylglycerol, one unidentified glycolipid, and one unidentified lipid. The DNA G+C content of the type strain is 67.61%.
The type strain, RHLS22-1T (=CGMCC 1.10101T = NBRC 114045T), was isolated from the melt water sample obtained from the supraglacial zone of Hailuogou glacier in Sichuan province, China. The NCBI accession numbers for the 16S rRNA gene and genome sequences are JX949476 and GCA_004402695.1, respectively.
Description of Cryobacterium algoricola sp. nov.
Cryobacterium algoricola (al.go.ri'co.la. L. masc. n. algor, the cold; L. masc./fem. suff. -cola, inhabitant, dweller; from L. masc./fem. n. incola, dweller; N.L. masc./fem. n. algoricola, cold-dweller).
Cells are Gram-positive, aerobic, motile with a single flagellum, rod-shaped, and 0.8–2.3 × 0.5–0.6 μm in size. Colonies are creamy yellow-colored, convex, round, and 1.0 mm in diameter after 7 days of incubation on PYG plates at 14°C. Growth occurs at 0–24°C, pH 6.0–8.0, and in the presence of 0–2.0% (w/v) NaCl. The optimum growth temperature is 14–20°C. Positive for catalase and oxidase. Reduces nitrate to nitrite. Does not hydrolyze Tween 80, gelatin, casein, and starch. Hydrolyze esculine. Indole and H2S are not formed. Positive for leucine arylamidase, acid phosphatase, β-galactosidase, α-glucosidase, β-glucosidase, and Voges–Proskauer test. Utilizes the following substances as carbon sources: D-maltose, D-trehalose, D-cellobiose, sucrose, D-turanose, stachyose, D-raffinose, D-melibiose, β-methyl-D-glucoside, D-salicin, N-acetyl-D-glucosamine, α-D-glucose, D-mannose, D-fructose, D-galactose, L-rhamnose, D-mannitol, glycerol, D-gluconic acid, L-lactic acid, L-malic acid, β-hydroxy-D,L-butyric acid, propionic acid, and acetic acid. Acids are produced from L-arabinose, D-xylose, galactose, glucose, fructose, mannose, rhamnose, mannitol, N-acetylglucosamine, arbutin, esculine, salicin, D-cellobiose, D-maltose, D-melibiose, D-saccharose, D-trehalose, and D-raffinose. The major fatty acids are anteiso-C15:0, iso-C16:0, anteiso-C15:1, and anteiso-C17:0. The predominant menaquinones include MK-10 and MK-11, with minor amounts of MK-9. The polar lipids are diphosphatidylglycerol, phosphatidylglycerol, one unidentified glycolipid, and one unidentified lipid. The DNA G+C content of the type strain is 67.90%.
The type strain, MDB2-BT (=CGMCC 1.11135T = NBRC 114047T), was isolated from the ice sample obtained from the supraglacial zone of Midui glacier on the Tibetan Plateau, China. The NCBI accession numbers for the 16S rRNA gene and genome sequences are JX949747 and GCA_004402485.1, respectively.
Description of Cryobacterium tagatosivorans sp. nov.
Cryobacterium tagatosivorans (ta.ga.to.si.vo'rans. N.L. neut. adj. tagatosum, tagatose; L. pres. part. vorans, eating; N.L. part. adj. tagatosivorans, eating tagatose).
Cells are Gram-positive, aerobic, motile with a single flagellum, rod-shaped, and 1.4–3.0 × 0.5–0.6 μm in size. Colonies are creamy yellow-colored, convex, round, and 2.0 mm in diameter after 7 days of incubation on PYG plates at 14°C. Growth occurs at 0–24°C, pH 7.0–10.0, and in the presence of 0–3.0% (w/v) NaCl. The optimum growth temperature is 14–20°C. Positive for catalase and oxidase. Reduces nitrate to nitrite. Does not hydrolyze Tween 80, gelatin, casein, and starch. Hydrolyze esculine. Indole and H2S are not formed. Positive for leucine arylamidase, α-glucosidase, Voges–Proskauer test, and β-galactosidase. Utilizes the following substances as carbon sources: D-maltose, D-cellobiose, stachyose, D-raffinose, D-melibiose, N-acetyl-D-glucosamine, N-acetyl-D-galactosamine, α-D-glucose, D-mannose, D-fructose, D-galactose, L-fucose, L-rhamnose, D-sorbitol, D-mannitol, D-arabitol, glycerol, L-alanine, L-arginine, L-glutamic acid, L-histidine, L-pyroglutamic acid, L-serine, D-gluconic acid, L-lactic acid, D-malic acid, L-malic acid, γ-aminobutyric acid, β-hydroxy-D,L-butyric acid, propionic acid, and acetic acid. Acids are produced from glycerol, glucose, fructose, rhamnose, mannitol, sorbitol, esculine, D-cellobiose, D-maltose, D-lyxose, D-tagatose, L-fucose, and D-arabitol. The major fatty acids are anteiso-C15:0, anteiso-C15:1, iso-C15:0, iso-C16:0, and anteiso-C17:0. The predominant menaquinones include MK-11 and MK-12, with minor amounts of MK-10. The polar lipids are diphosphatidylglycerol, phosphatidylglycerol, one unidentified glycolipid, and one unidentified lipid. The DNA G+C content of the type strain is 68.28%.
The type strain, Sr47T (=CGMCC 1.11221T = NBRC 114033T), was isolated from the ice sample obtained from the supraglacial zone of No. 1 glacier in Xinjiang Uygur Autonomous Region, China. The NCBI accession numbers for the 16S rRNA gene and genome sequences are JX949300 and GCA_004402215.1, respectively.
Description of Cryobacterium glaciale sp. nov.
Cryobacterium glaciale (gla.cia'le. L. neut. adj. glaciale, icy).
Cells are Gram-positive, aerobic, motile with a single flagellum, rod-shaped, and 1.4–3.3 × 0.6–0.7 μm in size. Colonies are creamy yellow-colored, convex, round, and 2.0 mm in diameter after 7 days of incubation on PYG plates at 14°C. Growth occurs at 0–20°C, pH 6.0–10.0, and in the presence of 0–4.0% (w/v) NaCl. The optimum growth temperature is 10–14°C. Positive for catalase, but negative for oxidase. Reduces nitrate to nitrite. Does not hydrolyze Tween 80, gelatin, casein, and starch. Hydrolyze esculine. Indole and H2S are not formed. Positive for alkaline phosphatase, leucine arylamidase, acid phosphatase, α-glucosidase, β-galactosidase, arginine dihydrolase, citrate utilization, and Voges–Proskauer test. Utilizes the following substances as carbon sources: D-maltose, D-trehalose, D-cellobiose, gentiobiose, sucrose, D-turanose, stachyose, β-methyl-D-glucoside, D-salicin, α-D-glucose, D-mannose, D-fructose, L-rhamnose, inosine, D-sorbitol, D-mannitol, D-arabitol, myo-inositol, glycerol, L-alanine, L-arginine, pectin, D-galacturonic acid, L-galactonic acid lactone, D-gluconic acid, D-glucuronic acid, L-lactic acid, L-malic acid, Tween 40, γ-aminobutyric acid, α-hydroxybutyric acid, acetoacetic acid, propionic acid, and acetic acid. Acids are produced from glycerol, glucose, fructose, mannose, rhamnose, esculine, and D-cellobiose. The major fatty acids are anteiso-C15:0, iso-C16:0, anteiso-C17:0, iso-C15:0, and anteiso-C15:1. The predominant menaquinones include MK-10 and MK-9, with minor amounts of MK-11. The polar lipids are diphosphatidylglycerol, phosphatidylglycerol, one unidentified glycolipid, and one unidentified lipid. The DNA G+C content of the type strain is 65.39%.
The type strain, HLT2-23T (=CGMCC 1.11085T = NBRC 114046T), was isolated from the ice sample obtained from the supraglacial zone of Hailuogou glacier in Sichuan province, China. The NCBI accession numbers for the 16S rRNA gene and genome sequences are JX949477 and GCA_004402535.1, respectively.
Data availability statement
The datasets presented in this study can be found in online repositories. The names of the repository/repositories and accession number(s) can be found in the article/Supplementary material.
Author contributions
Y-HX and QL designed the project, analyzed the data, collected and purified the strains, and wrote the manuscript. QL and L-LY performed bioinformatic analysis and experiments on phenotypic and chemotaxonomic characteristics. QL performed fatty acids analysis. All authors contributed to the article and approved the submitted version.
Funding
This study was supported by grant nos. 32170007 and 31600007 from the National Natural Science Foundation of China (NSFC) and the Biological Resources Programme and Chinese Academy of Sciences (grant no. KFJ-BRP-017-05).
Acknowledgments
We thank Aharon Oren (Hebrew University of Jerusalem, Israel) for the proposed names and etymologies of the new species. We thank Jing-Nan Liang of the Institute of Microbiology, Chinese Academy of Sciences (IMCAS), for her help in the use of transmission electron microscopes.
Conflict of interest
The authors declare that the research was conducted in the absence of any commercial or financial relationships that could be construed as a potential conflict of interest.
Publisher's note
All claims expressed in this article are solely those of the authors and do not necessarily represent those of their affiliated organizations, or those of the publisher, the editors and the reviewers. Any product that may be evaluated in this article, or claim that may be made by its manufacturer, is not guaranteed or endorsed by the publisher.
Supplementary material
The Supplementary Material for this article can be found online at: https://www.frontiersin.org/articles/10.3389/fmicb.2023.1115168/full#supplementary-material
References
Anesio, A. M., Lutz, S., Chrismas, N. A. M., and Benning, L. G. (2017). The microbiome of glaciers and ice sheets. NPJ Biofilms Microbiomes 3, 10. doi: 10.1038/s41522-017-0019-0
Blomberg, S. P., Garland, T., and Ives, A. R. (2003). Testing for phylogenetic signal in comparative data: behavioral traits are more labile. Evolution 57, 717–745. doi: 10.1111/j.0014-3820.2003.tb00285.x
Boenigk, J., Jost, S., Stoeck, T., and Garstecki, T. (2007). Differential thermal adaptation of clonal strains of a protist morphospecies originating from different climatic zones. Environ. Microbiol. 9, 593–602. doi: 10.1111/j.1462-2920.2006.01175.x
Boenigk, J., Pfandl, K., Garstecki, T., Harms, H., Novarino, G., Chatzinotas, A., et al. (2006). Evidence for geographic isolation and signs of endemism within a protistan morphospecies. Appl. Environ. Microbiol. 72, 5159–64. doi: 10.1128/AEM.00601-06
Camacho, C., Coulouris, G., Avagyan, V., Ma, N., Papadopoulos, J., Bealer, K., et al. (2009). BLAST+: architecture and applications. BMC Bioinformatics 10, 421. doi: 10.1186/1471-2105-10-421
Carroll, L. M., Wiedmann, M., and Kovac, J. (2020). Proposal of a taxonomic nomenclature for the Bacillus cereus group which reconciles genomic definitions of bacterial species with clinical and industrial phenotypes MBio 11, e0003-20. doi: 10.1128/mBio.00034-20
Casanueva, A., Tuffin, M., Cary, C., and Cowan, D. A. (2010). Molecular adaptations to psychrophily: the impact of 'omic' technologies. Trends Microbiol. 18, 374–81. doi: 10.1016/j.tim.2010.05.002
Chaumeil, P. A., Mussig, A. J., Hugenholtz, P., and Parks, D. H. (2019). GTDB-Tk: a toolkit to classify genomes with the genome taxonomy database. Bioinformatics 36, 1925–1927. doi: 10.1093/bioinformatics/btz848
Cohan, F. M., and Koeppel, A. F. (2008). The origins of ecological diversity in prokaryotes. Curr. Biol. 18, R1024–1034. doi: 10.1016/j.cub.2008.09.014
Collins, M. D. (1985). “Isoprenoidquinone analysis in classification and identification,” in Chemical Methods in Bacterial Systematics, eds M. Goodfellow, and D. E. Minnikin (London: Academic Press), 267–287.
Contreras-Moreira, B., and Vinuesa, P. (2013). GET_HOMOLOGUES, a versatile software package for scalable and robust microbial pangenome analysis. Appl. Environ. Microbiol. 79, 7696–7701. doi: 10.1128/AEM.02411-13
Drula, E., Garron, M. L., Dogan, S., Lombard, V., Henrissat, B., Terrapon, N., et al. (2022). The carbohydrate-active enzyme database: functions and literature. Nucleic Acids Res. 50(D1), D571–D577. doi: 10.1093/nar/gkab1045
Ellison, C. E., Hall, C., Kowbel, D., Welch, J., Brem, R. B., Glass, N. L., et al. (2011). Population genomics and local adaptation in wild isolates of a model microbial eukaryote. Proc. Natl. Acad. Sci. U. S. A. 108, 2831–2836. doi: 10.1073/pnas.1014971108
Galperin, M. Y., Wolf, Y. I., Makarova, K. S., Vera Alvarez, R., Landsman, D., Koonin, E. V., et al. (2021). COG database update: focus on microbial diversity, model organisms, and widespread pathogens. Nucleic Acids Res. 49(D1), D274–D281. doi: 10.1093/nar/gkaa1018
Garcia-Pichel, F., Loza, V., Marusenko, Y., Mateo, P., and Potrafka, R. M. (2013). Temperature drives the continental-scale distribution of key microbes in topsoil communities. Science 340, 1574–1577. doi: 10.1126/science.1236404
Gong, C., Lai, Q., Cai, H., Jiang, Y., Liao, H., Liu, Y., et al. (2020). Cryobacterium soli sp. nov., isolated from forest soil. Int. J. Syst. Evol. Microbiol. 70, 675–679. doi: 10.1099/ijsem.0.003820
Hotaling, S., Hood, E., and Hamilton, T. L. (2017). Microbial ecology of mountain glacier ecosystems: biodiversity, ecological connections and implications of a warming climate. Environ. Microbiol. 19, 2935–2948. doi: 10.1111/1462-2920.13766
Jain, C., Rodriguez-R, L. M., Phillippy, A. M., Konstantinidis, K. T., and Aluru, S. (2018). High throughput ANI analysis of 90K prokaryotic genomes reveals clear species boundaries. Nat. Commun. 9, 5114. doi: 10.1038/s41467-018-07641-9
Kim, M., Oh, H. S., Park, S. C., and Chun, J. (2014). Towards a taxonomic coherence between average nucleotide identity and 16S rRNA gene sequence similarity for species demarcation of prokaryotes. Int. J. Syst. Evol. Microbiol. 64, 346–351. doi: 10.1099/ijs.0.059774-0
Kimura, M. (1980). A simple method for estimating evolutionary rates of base substitutions through comparative studies of nucleotide sequences. J. Mol. Evol. 16, 111–120. doi: 10.1007/BF01731581
Komagata, K., and Suzuki, K. (1988). 4. Lipid and cell-wall analysis in bacterial systematics. Method Microbiol. 19, 161–207. doi: 10.1016/S0580-9517(08)70410-0
Lagesen, K., Hallin, P., Rødland, E. A., Staerfeldt, H. H., Rognes, T., Ussery, D. W., et al. (2007). RNAmmer: consistent and rapid annotation of ribosomal RNA genes. Nucleic Acids Res. 35, 3100–3108. doi: 10.1093/nar/gkm160
Liu, Q., Li, W., Liu, D., Li, L., Li, J., Lv, N., et al. (2021a). Light stimulates anoxic and oligotrophic growth of glacial Flavobacterium strains that produce zeaxanthin. ISME J. 15, 1844–1857. doi: 10.1038/s41396-020-00891-w
Liu, Q., Liu, H. C., Wen, Y., Zhou, Y. G., and Xin, Y. H. (2012). Cryobacterium flavum sp. nov. and Cryobacterium luteum sp. nov., isolated from glacier ice. Int. J. Syst. Evol. Microbiol. 62, 1296–1299. doi: 10.1099/ijs.0.033738-0
Liu, Q., Liu, H. C., Zhang, J. L., Zhou, Y. G., and Xin, Y. H. (2013). Cryobacterium levicorallinum sp. nov., a psychrophilic bacterium isolated from glacier ice. Int. J. Syst. Evol. Microbiol. 63, 2819–2822. doi: 10.1099/ijs.0.046896-0
Liu, Q., Liu, H. C., Zhou, Y. G., and Xin, Y. H. (2019a). Genetic diversity of glacier-inhabiting Cryobacterium bacteria in China and description of Cryobacterium zongtaii sp. nov. and Arthrobacter glacialis sp. nov. Syst. Appl. Microbiol. 42, 168–177. doi: 10.1016/j.syapm.2018.10.005
Liu, Q., Liu, H. C., Zhou, Y. G., and Xin, Y. H. (2019b). Microevolution and adaptive strategy of psychrophilic species Flavobacterium bomense sp. nov. isolated from glaciers. Front. Microbiol. 22, 10.1069. doi: 10.3389/fmicb.2019.01069
Liu, Q., Song, W. Z., Zhou, Y. G., Dong, X. Z., and Xin, Y. H. (2020a). Phenotypic divergence of thermotolerance: molecular basis and cold adaptive evolution related to intrinsic DNA flexibility of glacier-inhabiting Cryobacterium strains. Environ. Microbiol. 22, 1409–1420. doi: 10.1111/1462-2920.14957
Liu, Q., Tian, J. H., Liu, H. C., Zhou, Y. G., and Xin, Y. H. (2019c). Cryobacterium melibiosiphilum sp. nov., a psychrophilic bacterium isolated from glacier ice. Int. J. Syst. Evol. Microbiol. 69, 3276–3280. doi: 10.1099/ijsem.0.003620
Liu, Q., Tian, J. H., Liu, H. C., Zhou, Y. G., and Xin, Y. H. (2020b). Cryobacterium ruanii sp. nov. and Cryobacterium breve sp. nov., isolated from glaciers. Int. J. Syst. Evol. Microbiol. 70, 1918–1923. doi: 10.1099/ijsem.0.003994
Liu, Q., Xin, Y. H., Chen, X. L., Liu, H. C., Zhou, Y. G., Chen, W. X., et al. (2018a). Cryobacterium aureum sp. nov., a psychrophilic bacterium isolated from glacier ice collected from the ice tongue surface. Int. J. Syst. Evol. Microbiol. 68, 1173–1176. doi: 10.1099/ijsem.0.002647
Liu, Q., Yang, L. L., Liu, H. C., Zhang, G. Q., and Xin, Y. H. (2021b). Glacieibacterium frigidum gen. nov., sp. nov., a novel member of the family Sphingosinicellaceae isolated from a glacier. Int. J. Syst. Evol. Microbiol. 71, 004886. doi: 10.1099/ijsem.0.004886
Liu, Q., Zhou, Y. G., and Xin, Y. H. (2015). High diversity and distinctive community structure of bacteria on glaciers in China revealed by 454 pyrosequencing. Syst. Appl. Microbiol. 38, 578–585. doi: 10.1016/j.syapm.2015.09.005
Liu, Q., Zhou, Y. G., and Xin, Y. H. (2018b). “Cryobacterium,” in Bergey's Manual of systematics of Archaea and Bacteria, eds W. B. Whitman, and F. Rainey (New York, NY: John Wiley and Sons).
Liu, Y., Shen, L., Zeng, Y., Xing, T., Xu, B., and Wang, N. (2020). Genomic insights of cryobacterium isolated from ice core reveal genome dynamics for adaptation in glacier. Front. Microbiol. 11, 1530. doi: 10.3389/fmicb.2020.01530
Liu, Y., Vick-Majors, T. J., Priscu, J. C., Yao, T., Kang, S., Liu, K., et al. (2017). Biogeography of cryoconite bacterial communities on glaciers of the Tibetan Plateau. FEMS Microbiol. Ecol. 93. doi: 10.1093/femsec/fix072
Nguyen, L. T., Schmidt, H. A., von Haeseler, A., and Minh, B. Q. (2015). IQ-TREE: a fast and effective stochastic algorithm for estimating maximum-likelihood phylogenies. Mol. Biol. Evol. 32, 268–274. doi: 10.1093/molbev/msu300
Nicholes, M. J., Williamson, C. J., Tranter, M., Holland, A., Poniecka, E., Yallop, M. L., et al. (2019). Bacterial dynamics in supraglacial habitats of the greenland ice sheet. Front. Microbiol. 10, 1366. doi: 10.3389/fmicb.2019.01366
Parks, D. H., Chuvochina, M., Rinke, C., Mussig, A. J., Chaumeil, P. A., Hugenholtz, P., et al. (2022). GTDB: an ongoing census of bacterial and archaeal diversity through a phylogenetically consistent, rank normalized and complete genome-based taxonomy. Nucleic Acids Res. 50, D785–D794. doi: 10.1093/nar/gkab776
Parks, D. H., Imelfort, M., Skennerton, C. T., Hugenholtz, P., and Tyson, G. W. (2015). CheckM: Assessing the quality of microbial genomes recovered from isolates, single cells, and metagenomes. Genome Res. 25, 1043–1055. doi: 10.1101/gr.186072.114
Parte, A. C., Sardà Carbasse, J., Meier-Kolthoff, J. P., Reimer, L. C., and Göker, M. (2020). List of Prokaryotic names with Standing in Nomenclature (LPSN) moves to the DSMZ. Int. J. Syst. Evol. Microbiol. 70, 5607–5612. doi: 10.1099/ijsem.0.004332
Polz, M. F., Alm, E. J., and Hanage, W. P. (2013). Horizontal gene transfer and the evolution of bacterial and archaeal population structure. Trends Genet. 29, 170–175. doi: 10.1016/j.tig.2012.12.006
Reddy, G. S., Pradhan, S., Manorama, R., and Shivaji, S. (2010). Cryobacterium roopkundense sp. nov., a psychrophilic bacterium isolated from glacial soil. Int. J. Syst. Evol. Microbiol. 60, 866–870. doi: 10.1099/ijs.0.011775-0
Richter, M., and Rosselló-Móra, R. (2009). Shifting the genomic gold standard for the prokaryotic species definition. Proc. Natl. Acad. Sci. USA. 106, 19126–19131. doi: 10.1073/pnas.0906412106
Sasser, M. (1990). Identification of Bacteria by Gas Chromatography of Cellular Fatty Acids, MIDI Technical Note 101. Newark, DE: MIDI Inc.
Seemann, T. (2014). Prokka: rapid prokaryotic genome annotation. Bioinformatics 30, 2068–2069. doi: 10.1093/bioinformatics/btu153
Sievers, F., and Higgins, D. G. (2014). Clustal Omega, accurate alignment of very large numbers of sequences. Methods Mol. Biol. 1079, 105–116. doi: 10.1007/978-1-62703-646-7_6
Silva, B., Antunes, C., Andrade, F., and Ferreira, d. a. Silva E, Grande JA, Luís AT. (2021). Prokaryotic and eukaryotic diversity in hydrothermal continental systems. Arch. Microbiol. 203, 3751–3766. doi: 10.1007/s00203-021-02416-1
Smibert, R. M., and Krieg, N. R. (1994). “Phenotypic characterization,” in Methods for General and Molecular Bacteriology, eds P. Gerhardt, R. G. E. Murray, W. A. Wood, and N. R. Krieg (Washington, DC: ASM Press), 607–54.
Suzuki, K., Sasaki, J., Uramoto, M., Nakase, T., and Komagata, K. (1997). Cryobacterium psychrophilum gen. nov., sp. nov., nom. rev., comb. nov., an obligately psychrophilic actinomycete to accommodate “Curtobacterium psychrophilum” Inoue and Komagata 1976. Int. J. Syst. Bacteriol. 47, 474–478. doi: 10.1099/00207713-47-2-474
Tamura, K., Peterson, D., Peterson, N., Stecher, G., Nei, M., Kumar, S., et al. (2011). MEGA5: molecular evolutionary genetics analysis using maximum likelihood, evolutionary distance, and maximum parsimony methods. Mol. Biol. Evol. 28, 2731–2739. doi: 10.1093/molbev/msr121
Thompson, J. D., Higgins, D. G., and Gibson, T. J. (1994). CLUSTAL W: improving the sensitivity of progressive multiple sequence alignment through sequence weighting, position-specific gap penalties and weight matrix choice. Nucleic Acids Res. 22, 4673–4680. doi: 10.1093/nar/22.22.4673
Vinuesa, P., Ochoa-Sánchez, L. E., and Contreras-Moreira, B. (2018). GET_PHYLOMARKERS, a software package to select optimal orthologous clusters for phylogenomics and inferring pan-genome phylogenies, used for a critical geno-taxonomic revision of the genus Stenotrophomonas. Front. Microbial. 9, 711. doi: 10.3389/fmicb.2018.00771
Wang, Y., Cao, P., Sun, P., Zhao, J., Sun, X., Zhang, J., et al. (2019). Cryobacterium tepidiphilum sp. nov., isolated from rhizosphere soil of lettuce (var. ramosa Hort.). Antonie Van Leeuwenhoek 112, 1611–1621. doi: 10.1007/s10482-019-01287-3
Wardman, J. F., Bains, R. K., Rahfeld, P., and Withers, S. G. (2022). Carbohydrate-active enzymes (CAZymes) in the gut microbiome. Nat. Rev. Microbiol. 20, 542–556. doi: 10.1038/s41579-022-00712-1
Yang, L. L., Liu, H. C., Liu, Q., and Xin, Y. H. (2021). Arthrobacter cheniae and Arthrobacter frigidicola sp. nov., isolated from a glacier. Int. J. Syst. Evol. Microbiol. 71, 005177. doi: 10.1099/ijsem.0.005177
Keywords: Cryobacterium, glacier-inhabiting bacteria, cold adaptation, psychrophilic, taxonomy
Citation: Liu Q, Yang L-L and Xin Y-H (2023) Diversity of the genus Cryobacterium and proposal of 19 novel species isolated from glaciers. Front. Microbiol. 14:1115168. doi: 10.3389/fmicb.2023.1115168
Received: 03 December 2022; Accepted: 27 February 2023;
Published: 20 March 2023.
Edited by:
Brian D. Lanoil, University of Alberta, CanadaReviewed by:
Eric M. Bottos, Thompson Rivers University, CanadaBao-zhu Fang, Xinjiang Institute of Ecology and Geography (CAS), China
Copyright © 2023 Liu, Yang and Xin. This is an open-access article distributed under the terms of the Creative Commons Attribution License (CC BY). The use, distribution or reproduction in other forums is permitted, provided the original author(s) and the copyright owner(s) are credited and that the original publication in this journal is cited, in accordance with accepted academic practice. No use, distribution or reproduction is permitted which does not comply with these terms.
*Correspondence: Yu-Hua Xin, xinyh@im.ac.cn; Lei-Lei Yang, yangll@im.ac.cn