- 1School of Karst Science, Guizhou Normal University, Guiyang, China
- 2State Engineering Technology Institute for Karst Desertification Control, Guizhou Normal University, Guiyang, China
- 3School of Life Science, Guizhou Normal University, Guiyang, China
As important components of the biological soil crusts (BSCs) and of the primary stage of crust succession, cyanobacterial communities occupy an important ecological niche and play an important ecological role in desertification areas. In this study, we focused on the karst desertification area, which also belongs to the same category of desertification, and selected three study areas, Guanling-Zhenfeng Huajiang (HJ), Bijie Salaxi (SLX), and Shibing (SB), in the Guizhou Plateau, which represents the overall ecological environment of South China karst, to conduct surveys on the diversity of BSC species and soil properties. Analysis of the cyanobacterial communities and physicochemical properties using the Shannon-Wiener diversity index, principal component analysis, and redundancy analysis revealed that: (1) The three study areas had common cyanobacterial species, with a total of 200 species distributed across 22 genera, 2 classes, 5 orders, and 6 families belonging to the Oscillatoriales (39%), Scytonematales (24.5%), Chroococcales (23%), Nostocales (11.5%), and Rivulariales (2%), (2) The number of species increased with the intensity of karst desertification—while Oscillatoriaceae was the dominant family in HJ and moderate–severe desertification areas, Chroococcaceae and Scytonemataceae were dominant in the mild and potential desertification areas SLX and SB, (3) The Shannon-Wiener diversity indices followed the trend: SLX (3.56) > SB (3.08) > HJ (3.01), indicating that the species were more evenly distributed in mild desertification, (4) In the carbonate background, shrubland harbored the largest number of cyanobacterial species compared to grassland, bare land, and arbor woodland; however, the highest number was documented in arbor woodland in dolomite karst, (5) The soil is weathered limestone or yellow soil in all three areas, with pH ranging from 5.73 to 6.85, fine sand dominated, and soil nutrients increased with the intensity of desertification, and (6) Redundancy analysis showed that organic carbon, soil moisture content (0–5 cm), and total nitrogen substantially influenced cyanobacterial diversity. These results reveal that differences in soil nutrient content play an important role in regulating the cyanobacterial diversity and composition, thereby establishing a foundation for further research and application of soil ecological restoration of cyanobacteria in BSCs of karst desertification areas.
1. Introduction
BSCs are an important component of desert ecosystems that have received widespread attention in desertification areas, it refers to the plant–soil complexes formed by different proportions of cyanobacteria, algae, lichens, mosses, and microorganisms cementing soil particles (Belnap and Lange, 2003; Belnap and Miller, 2004). It covers 12% of the global land area, with some arid regions covering more than 70% (Belnap and Lange, 2001). Cyanobacteria-dominated crusts have harsh environmental adaptations and are, therefore, dominant in extreme environments (Belnap et al., 2016). Research results from many different global habitats, including deserts, plateaus, mountains, savannas, and even polar regions, revealed that cyanobacterial crusts provide important ecosystem services, such as responding to climate change, dust cycling (Rodriguez-Caballero et al., 2022), hydrological change (Li et al., 2018), and enhancement of global carbon sink capacity (Zou et al., 2022). In soil ecosystems, cyanobacteria are usually the pioneers of community succession, providing carbon and nitrogen to the soil (Belnap, 2002; Housman et al., 2006) and promoting soil nutrient cycling. It also prevents soil erosion and reduces water evaporation (Felde et al., 2018), indirectly enhancing soil stability and improving ecosystem quality (Hagemann et al., 2015; Belnap et al., 2016; Chamizo et al., 2016). Soil properties reflect regional climate, bedrock, geological features, and biodiversity. Research has been conducted on the co-changes in cyanobacteria organisms and soil. Cano-Díaz et al. explored cyanobacterial diversity in gypsum soils in central Spain and revealed that gypsum soils are dominated by filamentous cyanobacteria and have lower abundance and diversity (Cano-Díaz et al., 2018). In the mountainous regions of the northern Urals, where weathering is intense, coarse sandy soils dominate steep slopes and are dominated by spherical cyanobacteria, whereas soils in depressions and gentle areas are fine-textured and dominated by filamentous cyanobacteria (Patova et al., 2018). Temraleeva (2018) explored cyanobacteria species diversity in Russian arid and desert steppe semi-desert soils, meadow soils, and chestnut calcareous soils. Roncero-Ramos et al. (2019) explored the structural diversity of cyanobacterial communities developed from clay, silty, and sandy loam of calcareous sandstones and calcareous mudstones in southeastern Spain and revealed the differences in cyanobacterial composition in soils from different geographical regions. However, more studies based on the diversity of BSCs cyanobacteria in different soil matrices are yet to be conducted.
The composition and dominance of cyanobacterial species corresponds to different soil environments. Microcoleus, Scytonema, Phormidium, Trichocoleus, Leptolyngbya, and Tychonema have been widely reported in the desert, with Microcoleus vaginatus being overwhelmingly dominant (Hagemann et al., 2015; Zhang et al., 2015; Etemadi-Khah et al., 2017; Fernandes et al., 2018; Zhang et al., 2021; Sosa-Quintero et al., 2022). In temperate arid steppe and dryland ecosystems, cyanobacterial crusts mainly consist of Nostocales, Oscillatoriales, Synechoccocales, and the dominant species beside M. vaginatus, including Symplocastrum purpurascens, Scytonema sp., Nostoc commune, Phormidium sp., with the biomass of cyanobacteria increasing with light intensity (Büdel et al., 2009; Williams et al., 2016; Büdel et al., 2018; Temraleeva, 2018; Roncero-Ramos et al., 2019). In the mountains of northeastern Europe, cyanobacteria mainly consist of Phormidium, Leptolyngbya, and Nostoc, with Leptolyngbya voronichiniana, Leptolyngbya foveolarum, Trichocoleus hospitus dominating (Gaysina et al., 2018; Novakovskaya et al., 2022). The main cyanobacteria in Brazil’s tropical savanna include Microcoleus, Nostoc, Leptolyngbya, Porphyrosiphon, and Pycnacronema (Machado de Lima et al., 2019, 2021). In cold polar environments, Chroococcales, Pseudanabanales, and Oscillatoriales are the main cyanobacterial constituent groups but lack genera such as Oculatella and Hassallia, which are common in temperate and tropical regions and where humidity is high, and are usually covered with thicker Nostoc spp. with enhanced nitrogen fixation capacity (Pushkareva et al., 2015, 2016, 2018). Although there are certain similarities in genus composition, the species are different. However, whether there are differences in ecological functions between different cyanobacterial crusts remains unclear because of the lack of taxonomic information on cyanobacteria from different areas. To more accurately interpret their ecological functions from global patterns and apply their restoration value for degraded ecosystems, more attention should be paid to cyanobacteria taxonomy in karst critical zones.
Karst is the sum of the binary three-dimensional structure of landscapes and phenomena between the surface and the ground formed by erosion, dissolution, collapse, and accumulation of the affection by flowing water to soluble rocks. Karst desertification is an extreme process of ecosystem degradation (Ravbar and Šebela, 2015). South China karst is the most comprehensive, complex, and longest-developed karst landscape in the world and is one of the three largest continuous carbonate rock distribution areas worldwide (Chen et al., 2019). The expansion of rock desertification is exacerbated by the uneven spatial and temporal distribution of seasonal precipitation and overloaded economic activities due to high population loads, resulting in shallow and infertile soils, low vegetation coverage, soil erosion, and high rock exposure rates, leading to the degradation of the ecosystem and eventually presenting a karst desertification landscape such as desertification. In the desertification area in South China karst, there are completely different climatic, zonal, and non-zonal soil-forming conditions from the above desertification and temperate areas, with the soil dominated by limestone, dolomite, and other carbonate rocks and zonal yellow and brown soil (Sheng et al., 2016). In this particular context, studies based on the synergistic evolution of cyanobacteria crust species diversity and soil properties in karst desertification are still lacking. This study examined the diversity and composition of the extant cyanobacteria crust species in the three karst desertification study areas. The synergistic evolutionary relationship with cyanobacteria is discussed in terms of different habitats and soil physicochemical properties, which in turn provides data support for the study of cyanobacterial crust soils in karst desertification areas, a reference for ecological restoration of karst desertification soils, and a case study of karst desertification ecological restoration for global desertification.
2. Materials and methods
2.1. Study area
The Guizhou Plateau was selected as the study area, as it represents the overall ecological structure of South China karst, and chose the Guanling-Zhenfeng Huajiang (HJ) study area with moderate–severe desertification, the Bijie Salaxi (SLX) study area with potential-mild desertification, and the Shibing (SB) study area with no-potential desertification (Figure 1). These areas are dominated by a subtropical humid monsoon climate with rain and heat during the same period but retain differences in lithology, soils, and vegetation. HJ is a typical plateau canyon area with an altitude of 450–1,450 m, an average annual temperature of 18°C, and an average annual precipitation of over 1,200 mm (Zhang et al., 2019). The vegetation type is mainly scrub, broad-leaved forest, and mixed coniferous forest. The altitude of SLX ranges from 1,410 to 1780 m, the average annual temperature is 12°C, the average annual precipitation is above 984.40 mm, and the vegetation type is dominated by scrubs, broad-leaved forests, and coniferous forests. SB is a typical dolomite karst area with an altitude of 526–1,576 m, an average annual temperature of 16°C, an average annual precipitation of 1,110 mm or more, and a vegetation type mainly comprised of subtropical evergreen broad-leaved forests (Wang et al., 2019); the differences in soil properties are shown in Table 1.
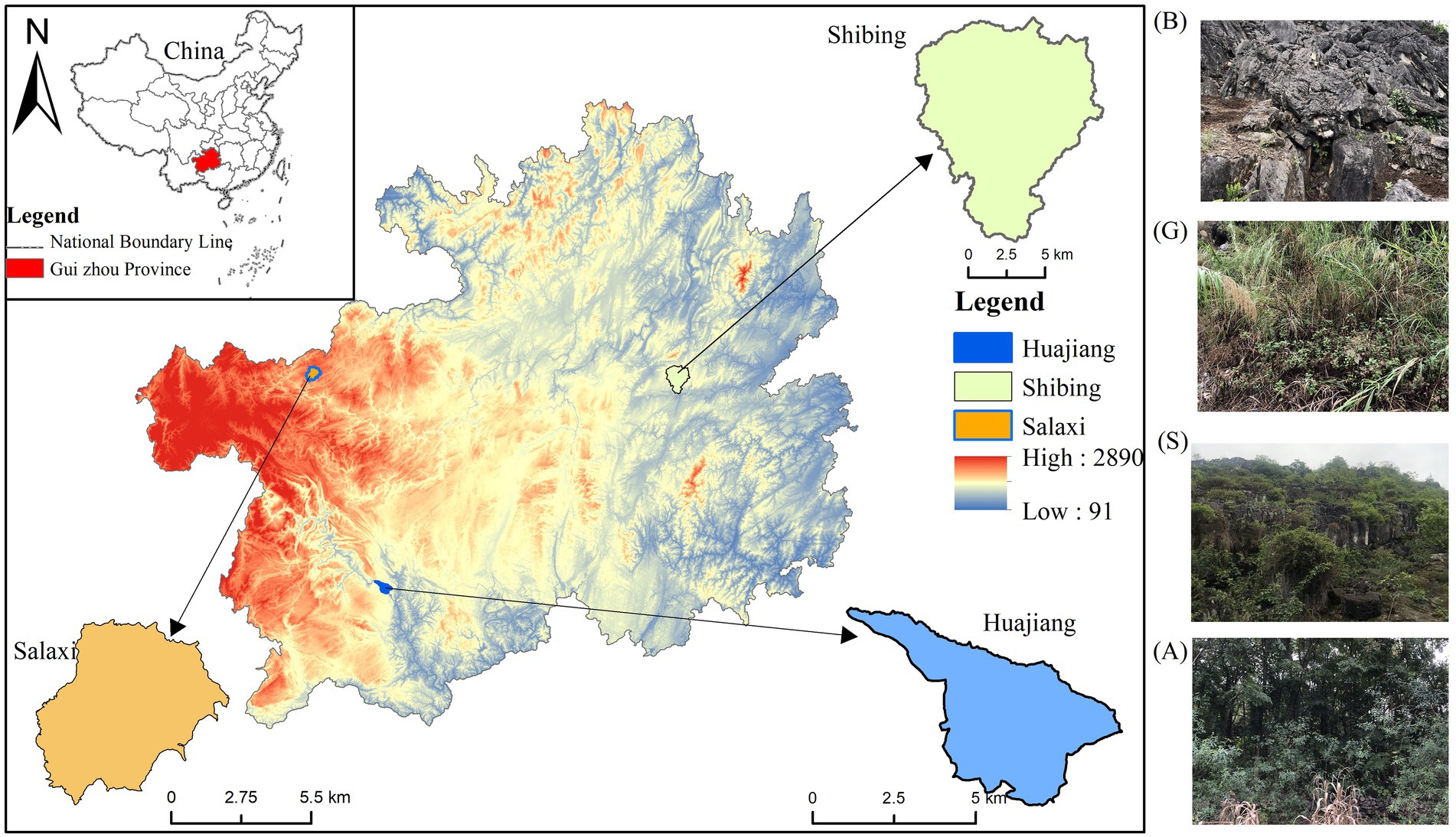
Figure 1. Study area and sampling community. B-Bare land; G-Grassland; S-Shrub land; A-Arbor woodland, same as below.
2.2. Sample sites
Sampling sites were identified in the three study areas, and the vegetation composition of bare land, grassland, shrubland, and arbor woodland, as well as information on key species, latitude, longitude, elevation, coverage, etc., were investigated. The major species and environmental factors of sampling sites of the same community type should be as similar as possible, with a sampling quadrat of 10 m × 10 m for bare land, grassland, and shrubland and 30 m × 30 m for arbor woodland, specific sampling quadrats information are shown in Table 2.
2.3. Crust sample collection and procession
2.3.1. Sample collection
In April 2021, BSCs were collected from different communities in the three study areas, 1 cm thickness of cyanobacteria-dominated BSCs was collected extensively and randomly with small shovels in the sampling quadrat, mixed into one sample, and placed in plastic bags. Three mixed samples were collected from each of the four community types, and 12 mixed samples were collected from one study area, resulting in a total of 36 mixed BSCs samples from the three study areas, which were placed in plastic bags and taken back to the laboratory as soon as possible.
2.3.2. Sample preservation and identification
The field-collected BSCs were placed in specimen bottles containing propanetriol-formaldehyde-water solution (1:1:8) fixative and stored at room temperature in the laboratory of the School of Karst Science, Guizhou Normal University, where cyanobacteria species identification, dominant species recording and micrographs were taken using an OLYMPUS-CX41 trinocular microscope (Yan et al., 2021).
Dominant species: a small piece of BSCs was removed with small forceps to make a temporary water mount. Three temporary slices of each sample were taken, and 10 fields of view were observed for each mount to determine the dominant cyanobacteria species based on the frequency of occurrence (Zhang et al., 2008).
2.4. Soil sample collection and procession
2.4.1. Sample collection
In each community quadrate, an S-shaped random sampling method was used to collect the physical and chemical properties of different analysis targets; the ring knife sampling method was used for the physical properties of the soil, the soil with two layers of 0–5 cm and 5–10 cm was selected, and chemical properties selected 0–10 cm mixed soil. The soils were taken back to the laboratory to dry naturally, ground, and sieved through 2 and 0.15 mm soil sieves and stored in plastic bags at room temperature for later determination of indicators.
2.4.2. Methods for the determination of physical and chemical indicators
Soil moisture content was determined by the ring-knife and drying methods; pH was determined using the 1:2.5 soil/water ratio centrifugal sedimentation method and a Leici PHS-25 acidity meter; soil texture was determined by the international classification standard, which classifies soil particle size into clay (<0.002 mm), silt (0.002–0.02 mm), fine sand (0.02 ~ 0.2 mm), and coarse sand (0.2 ~ 2 mm), using the hydrometer method; soil organic carbon was determined by the potassium dichromate oxidation-external heating method; soil total nitrogen was determined by sulfuric acid-catalyst digestion and the Kjeldahl nitrogen determination method; available nitrogen was determined by the alkali-hydrolysis diffusion method; soil total phosphorus was determined by the molybdenum antimony spectrophotometric method; soil available phosphorus was determined by sodium fluoride hydrochloric acid leaching and the molybdenum antimony anti-colorimetric method (Bao, 2000).
2.5. Data statistics and analysis
2.5.1. Cyanobacterial diversity index
Using the Shannon-Wiener diversity index:
where S is the number of species in cyanobacteria, is the proportion of individuals of the species among all individuals, and H is the diversity index.
2.5.2. Data analysis
The data were processed and analyzed using Excel 2013 and SPSS 22.0. One-way analysis of variance (ANOVA) and principal component analysis were used to analyze and test the soil physicochemical properties and cyanobacterial diversity using Canoco 5 software for redundancy analysis and plotting, and the remaining graphs were plotted using the Origin 2021 software.
3. Results
3.1. Taxa and diversity characteristics of cyanobacteria in karst desertification areas
3.1.1. Cyanobacteria taxa
There are 200 species of cyanobacteria in two classes, five orders, six families, and 22 genera in the three karst desertification study areas, with filamentous cyanobacteria accounting for 77.4% of the total number of cyanobacteria. Oscillatoriales (39%) accounted for the largest proportion, followed by Scytonematales (24.5%), Chroococcales (23%), Nostocales (11.5%), and Rivulariales (2%). Oscillatoriaceae and Chroococcaceae were the dominant families and only three genera of Scytonemataceae, but the number of species was second only to that of Chroococcaceae, accounting for 24.5% (Table 3). The dominant genera were Scytonema, Oscillatoria, Lyngbya, Nostoc, and Gloeocapsa, with filamentous cyanobacteria being overwhelmingly dominant (Figure 2).
3.1.2. Composition and distribution for each study area
The distribution and dominance of cyanobacteria families and genera differed among the different karst desertification study areas (Table 4). A total of 94 species of cyanobacteria from 5 families and 12 genera were found in HJ, with Oscillatoriaceae, the most dominant family, accounting for 54.25% of the total number of species, and Scytonemataceae, the subdominant family, accounting for 23.40%. The dominant genera were Oscillatoria (23), Lyngbya (16), and Scytonema (15). A total of 77 species of cyanobacteria from 5 families and 17 genera were found in SLX, with Chroococcaceae being the most dominant, accounting for 37.66% of the total number of species. Oscillatoriaceae accounted for 36.36% of the total number of species. The dominant genera were Oscillatoria (15), Gloeocapsa (10), and Nostoc (9). There were 80 species of cyanobacteria in 5 families and 14 genera in SB, with Scytonemataceae being the most dominant, accounting for 33.75% of the total number of species, and Chroococcaceae being subdominant, accounting for 27.50%. The dominant genera were Scytonema (23), Gloeocapsa (16), and Nostoc (11) (Figure 3).
3.1.3. Number of cyanobacteria species
Under the four community habitat types in the three different desertification study areas (HJ, SLX, and SB), there were significant differences in the number of species and genera as the communities evolved from bare land, grassland, shrubland, and arbor woodland (Figure 4). HJ and SLX had the highest number of species in shrubland, with an average cover of over 85% of the community vegetation and 65% of the arbor woodland. The highest number of species was found in the arbor woodland in SB, with an average vegetation cover of 95%, whereas the other communities did not differ significantly. The number of cyanobacteria genera was highest in HJ and SB arbor woodlands and highest in SLX shrubs (Table 4).
3.1.4. Cyanobacterial diversity
The Shannon-Wiener diversity index of cyanobacteria differed among the three study areas (Figure 5), with SLX having the highest diversity index, followed by SB and HJ having the lowest, at 3.56, 3.08, and 3.01, respectively. Second, the diversity index also differed among the different types of communities in the three study areas, with HJ having the highest diversity in arbor woodland (3.19), SLX having the highest diversity in shrubland (3.54), and SB having the highest diversity in bare land (2.93).
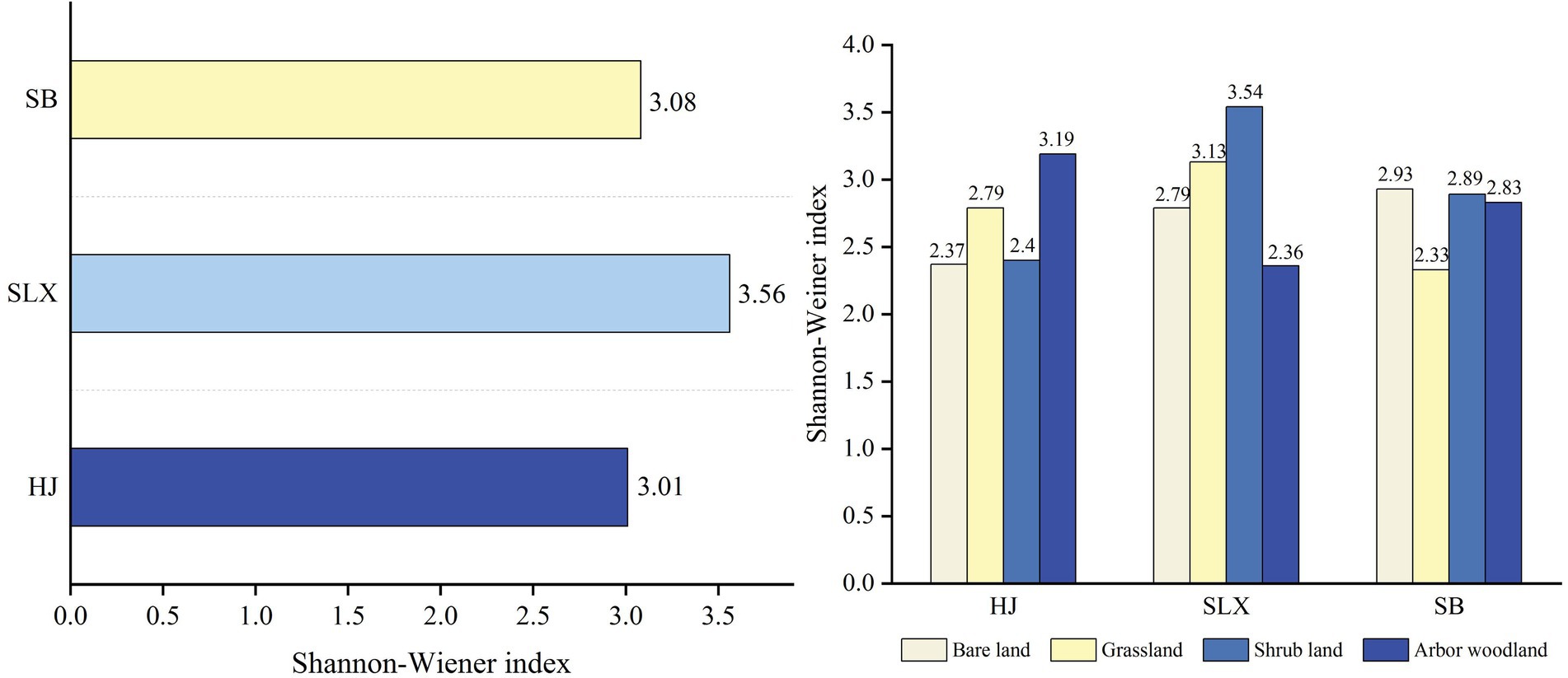
Figure 5. Shannon-Wiener diversity index of cyanobacteria in the study area and different community type.
3.1.5. Dominant species
The dominant species differed among the three study areas and the different community types, with a total of 15 dominant cyanobacteria species identified (Table 5). The dominant species in the three study areas belonged to the genera Oscillatoria, Scytonema, and Gloeocapsa; in HJ, the dominant species were Scytonema javanicum and Lyngbya gracilis; in SLX, the dominant species were Lyngbya contorta and Gloeocapsa montana, and in SB, the dominant species were Scytonema hofmanni and Gloeocapsa montana.
The dominant cyanobacteria in all communities were M. vaginatus and Nostoc commune, Scytonema was dominant in the arbor woodlands of all three study areas, Gloeocapsa was dominant in the shrublands of SLX and SB, and Lyngbya was dominant in the shrubland of HJ, with different dominant species in the grassland and bare land.
3.2. Physicochemical properties of soils
3.2.1. Soil moisture content
There were significant differences (p < 0.05) in the soil physicochemical indicators between the different desertification study areas, as shown in Table 6. In the limestone-dominated study areas of HJ and SLX, the overall soil moisture content of SLX was greater than that of HJ, and the surface moisture content (0–5 cm) was greater than that of the lower layer (5–10 cm). The difference in soil moisture content between the four different community habitats was not significant, with SLX having the highest moisture content in grassland and HJ having the highest moisture content in shrubland. The dolomite-dominated study area of SB showed that the surface moisture content (0–5 cm) was less than the lower (5–10 cm) surface moisture content, with significant differences in surface moisture content and non-significant differences in lower moisture content in the community habitats, with the highest moisture content in the arbor woodland.
3.2.2. pH and soil texture
The pH of the selected karst desertification study area was neutral to acidic, with overall non-significant differences ranging from 5.93 to 6.75. The soil particles were mainly composed of sand, with the proportion of fine sand content being the largest among the soil particles in all three study areas, with an average content of HJ (66.49%) > SB (53.29%) > SLX (45.57%), with significant differences in the SLX and SB community habitats. The coarse sand content averaged 14% in the three study areas, showing SLX (23.12%) > SB (13.90%) > HJ (4.99%), with no significant differences between the HJ and SB community habitats. There was no significant difference in silt particles in the HJ community habitat, but there were significant differences in the SLX and SB community habitats, with the largest content in the SB arbor woodland and the lowest in the HJ shrubland. The clay content was below 10% in all the study areas.
3.2.3. Organic carbon, phosphorus, and nitrogen
In terms of soil nutrients, the average content of organic carbon, total phosphorus, and total nitrogen in all three study areas showed a maximum in HJ, followed by SLX, and a minimum in SB. The available nitrogen content was generally uniform with little variation. The organic carbon and total nitrogen contents did not differ significantly among the communities in the three study areas, and the average content was more uniform within the community habitats. The differences in total phosphorus content were significant in the SB communities but not in the HJ and SLX communities. The differences in available phosphorus were not significant in the communities of SLX and SB but significant in the communities of HJ, with the highest value in the arbor woodland of HJ and the lowest value in the shrubland of SB, whereas the differences in available nitrogen were not significant in the communities of SLX and SB.
3.3. Cyanobacteria species diversity and soil properties
3.3.1. Soil property factors affecting the cyanobacteria species diversity
Principal component analysis (PCA) was conducted on 12 soil property factors for each community in the three study areas, with the aim of screening out the principal components that may influence cyanobacteria species diversity. The results of the PCA analysis of the soil property factors in the study areas showed that PC1 explained 34.4% of the soil property factors and PC2 explained 23.8% of the soil property factors, with differences between the soil property factors in the three study areas (Figure 6). The first principal component explained the soil property factors affecting cyanobacteria species diversity as organic carbon, total nitrogen, total phosphorus, available nitrogen, and available phosphorus, whereas the second principal component explained soil moisture content (0–5 cm, 5–10 cm) and coarse and silt in the soil particle composition, thus excluding the three factors of fine sand, clay, and pH.
3.3.2. Redundancy analysis of cyanobacterial diversity and soil properties
To clarify the relationship between cyanobacterial diversity and soil properties, a redundancy analysis was conducted by combining the cyanobacterial diversity index and the number of species with nine soil property factors (Figure 7). The results showed that Axis-1 explained 56.8% of the results, with organic carbon, total nitrogen, available nitrogen, soil moisture content (0–5 cm), and soil moisture content (5–10 cm) all pointing to Axis-1. There were positive correlations (number and index) between the response factors, with the number of cyanobacteria species mainly influenced by organic carbon (contribution of 27.9%), with a significant positive correlation with organic carbon (p < =0.03). The diversity index was mainly influenced by soil moisture content (0–5 cm) (contribution of 23%), with a significant positive correlation with soil water content (0–5 cm) (p < =0.012), and the diversity index was also influenced by total nitrogen (contribution of 19.6%), with a significant positive correlation with total nitrogen (p < =0.016). Although soil moisture content (5–10 cm) and moisture nitrogen also pointed to the same axis, indicating that there may also be an effect on cyanobacteria species diversity, they did not show statistical significance in the analyzes performed (p > 0.05).
4. Discussion
4.1. Similarities and differences in cyanobacterial diversity between karst desertification areas and desertification areas
The BSCs cyanobacteria in the karst desertification study areas were dominated by filamentous cyanobacteria, which accounted for 77.4% of the total. The main families and genera of cyanobacteria are Oscillatoriaceae (Oscillatoria, Lyngbya), Scytonemataceae (Scytonema), Nostocaceae (Nostoc), and Chroococcaceae (Gloeocapsa), the most common of which is M. vaginatus (Garcia-Pichel et al., 2001; Belnap and Lange, 2003; Zhang et al., 2015). In our study, M. vaginatus occurred at all sampling sites, which was generally consistent with the composition of cyanobacteria in most other types of desertification areas (Zhang et al., 2005a,b; Büdel et al., 2009; Etemadi-Khah et al., 2017; Cano-Díaz et al., 2018; Roncero-Ramos et al., 2019), indicating that M. vaginatus is the main cyanobacteria that make up the BSCs and plays an important ecological role, while N. commune also occurred in almost all sample sites and has been shown to occur most frequently among cyanobacteria (Starks et al., 1981). The cyanobacteria in this study are common to the desert ecological zone and have a wide ecological range, which again supports the fact that they are an important genus of cyanobacteria that form BSCs in the desert ecological zone, and also reflects the similarity in the cyanobacterial composition of BSCs in the karst desertification areas as a degraded desert ecological zone. There are also differences, with the genera unique to karst desertification areas being Cyanostylon and Symploca, with also differences in species, which may be related to differences in regional microhabitats and the adaptation of different cyanobacteria. Desert ecosystems are often considered to be harsh and lifeless habitats, and cyanobacteria can adapt to such extreme environmental conditions and occupy a unique ecological niche (Whitton and Potts, 2007; Perera et al., 2018), as opposed to harsh desertification areas with strong solar radiation, sandy winds, and lack of water. The warm and humid conditions in karst desertification areas provide good conditions for the growth and reproduction of filamentous cyanobacteria, and filamentous cyanobacteria are dominant in cementing fixed soil particles, which can slow down the soil loss caused by the special geomorphic structure in karst areas, improve soil stability, and have important functions in karst soil ecological restoration.
Although the species and numbers of cyanobacteria identified in the three karst desertification study areas are more convincing in this study, the classification of cyanobacteria is based only on morphological microscopic identification. It has been pointed out that the taxonomy of cyanobacteria traditionally relies heavily on morphological data, but the evolutionary relationships of cyanobacteria are more complex, and the true diversity of cyanobacteria is likely to be underestimated (Dvořák et al., 2017). The existing methods also include microscopic identification combined with culture identification, but there are cases where some cyanobacteria are not viable in culture (Dvořák et al., 2018). With the expansion of cyanobacterial gene pools and rapid development of molecular techniques, studies have shown that comprehensive evaluation of cyanobacteria in BSCs requires a combination of multiple molecular techniques, such as DNA extraction, PCR amplification, and sequencing. Molecular tools are often heavily applied to desert BSCs cyanobacteria, and some scholars have used phylogenies to study the global distribution of desert cyanobacteria, concluding that the global distribution of desert cyanobacteria is not the result of widespread dispersal but rather an ancient genetic evolution (Bahl et al., 2011). Other scholars have also argued that cyanobacterial diversity and distribution are determined by complex interactions with multiple abiotic stressors (Lacap-Bugler et al., 2017). However, it is also important to combine a multiphase approach to species description with morphology, as gene sequences can also appear to be improperly assigned to genera (Muhlsteinova et al., 2014), even though the multiphase approach does not guarantee that all cyanobacteria will be identified (Redfield et al., 2002). Therefore, in future research on karst cyanobacteria, the three karst desertification regions also need to combine a multiphase approach to further illustrate the cyanobacterial diversity of the three sites and their intrinsic connections at the geographic scale and phylogeny.
4.2. Differences in cyanobacteria species diversity in different karst desertification areas and community habitats
In the three karst desertification study areas, there were differences in the abundance, diversity, and species composition of cyanobacteria. The overall number of species was HJ > SB > SLX, and the diversity index was SLX > SB > HJ. In the same limestone lithological background, the number of species was HJ > SLX, and the diversity index was SLX > HJ. Scholars have shown that cyanobacterial community structure is associated with different physicochemical properties of the colonized mineral matrix and petrographic properties (Khomutovska et al., 2020). The number of cyanobacteria was the highest, and the diversity index was the lowest in the HJ moderate–severe desertification study area, with the largest proportion of Oscillatoriaceae, and the species composition was relatively simple, which is consistent with the conclusion of Yang et al. (2013), and may be related to the hydrothermal combination conditions caused by the microclimate in the region since the study area of HJ in moderate–severe desertification is located in a dry and hot valley with high annual rainfall and evaporation, and high temperature, hence it is dominated by Oscillatoriaceae which is non-heterocystic, swimmable and can grow and develop while avoiding the strong sunlight and dry environment (Belnap et al., 1993). The largest number of cyanobacteria species and the highest diversity index in the SLX potential-mild desertification study area were dominated by Chroococcaceae because of the predominance of spherical cyanobacteria species with greater resilience in SLX at an average altitude of more than 1,000 m, with low mean annual temperatures and a wet and cold environment (Pushkareva et al., 2015). In the background of dolomite lithology, the number of species in the SB study area was second to that of HJ, and the diversity index was lower than that of SLX and dominated by Scytonemataceae, which is consistent with the conclusion of Yan et al. (2021) that the terrestrial algae of the dolomite karst of SB are dominated by Scytonemataceae, reflecting the richness in calcium and magnesium and poverty in nutrients of the dolomite karst and supporting the view that dolomite dominates the growth and development of heteromorphic filamentous cyanobacteria (Hu et al., 2002).
In the four community habitats of three different karst desertification study areas, in terms of species numbers, the number of species in the HJ study area was shrubland > arbor woodland > bare land > grassland; in the SLX study area, shrubland > grassland > bare land > arbor woodland, and the trends were basically the same in the two desertification study areas of the same lithology, except for the opposite positions in the number of arbor woodland and grassland. The number of species in the arbor woodland was the highest in the SB study area, whereas the rest of the communities showed little difference. In terms of diversity indices, HJ had the highest diversity in grassland and woodland, SLX had the highest diversity in grassland and shrubland, and SB had the highest diversity in bare land and shrubland. In summary, it is clear that the number of species in the study area is highest in shrubland and highest in grassland in the lithologically identical HJ and SLX study areas. The highest number of species in the dolomite study area of SB is in the arbor woodland, and the highest diversity is in the bare land, which is not only influenced by lithology, general environment, and soils but also by other factors. It has been suggested that grassland has a greater capacity to trap surface water and that vegetation coverage also affects light intensity and, thus, cyanobacterial diversity (Vijayan and Ray, 2015), combined with the community coverage information in this study.
4.3. The influence of karst desertification soil properties on cyanobacterial diversity and species composition
Soil physicochemical properties are influenced by topographic and climatic conditions, bedrock properties, land use, community vegetation, and habitat degradation, resulting in the spatial heterogeneity of regional soil properties (Quesada et al., 1998; Řeháková et al., 2011; Hakkoum et al., 2020). Karst desertification succession is considered to be an extreme land degradation process; however, in our study, soil nutrient content did not decrease with increasing desertification, in agreement with the conclusions reached by Sheng et al. (2016). The organic carbon, total nitrogen, and total phosphorus contents were greatest in the study area of moderate–severe desertification in HJ, followed by SLX, and lowest in SB, due to the increase in the rate of rock exposure as the degree of rock desertification deepened; the nutrients from atmospheric deposition, rock dissolution, and plant apoptosis cannot be absorbed and preserved by the exposed rocks and can only be washed by rain or blown by the wind into thxe surrounding soil, so the soil nutrient content increases, the soil that can be lost is limited too, and nutrient losses are minimal. The results of our study show that the soil properties that affect the cyanobacteria species diversity in the three karst desertification study areas are soil moisture content (0–5 cm), organic carbon, and total nitrogen. Organic carbon is not only positive for the growth and development of cyanobacterial crusts, but also a key factor in promoting the formation of cyanobacterial communities. Some scholars have studied the structure of Arctic cyanobacterial communities and their bearing soil substrates, showing a significant positive correlation between organic carbon and cyanobacteria abundance (Pushkareva et al., 2016). In a study of the community structure of BSCs cyanobacteria in the Tengger East Desert, Zhang et al. (2021) concluded that total nitrogen and organic carbon were the factors that significantly influenced cyanobacterial diversity and abundance, and that the highest organic carbon content and the highest number of cyanobacteria species were found in the arbor woodland of SB, while the highest diversity and total nitrogen content were found in the bare land, thus supporting this conclusion. In studying the relationship between cyanobacterial abundance and rainfall in the Atacama Desert, researchers have concluded that cyanobacterial abundance decreases with decreasing rainfall (Warren-Rhodes et al., 2006). Zhang et al. (2009) concluded that precipitation is the main factor influencing the distribution of cyanobacteria and that the higher the precipitation, the higher the soil moisture content, resulting in a finer soil texture that can support the growth and development of more cyanobacterial communities. In a study of cyanobacteria in BSCs in the Ural Mountains, researchers pointed out that soil moisture content and total nitrogen were important soil property factors affecting cyanobacteria species diversity (Patova et al., 2018), which is generally consistent with the findings of this study. Others have shown that cyanobacterial diversity is most influenced by soil moisture content and less related to soil chemical composition (Hagemann et al., 2015), which is generally consistent with the results of this study. SLX had a significantly higher soil moisture content in all communities than the other two study areas, which, based on the analytical discussion, could be used as an explanation for the highest diversity index in SLX.
4.4. Synergistic evolution of cyanobacteria species composition and soil properties in karst desertification areas
The specific physiological and ecological characteristics of cyanobacteria allow them to occupy different ecological niches, and the dominance of cyanobacteria varies depending on habitat and soil conditions (Loza et al., 2014). A study of the cyanobacteria species composition of BSCs in the Saharan desert indicated that Microcoleus spp. were significantly dominant in low salinity soils, filamentous heterocystic cyanobacteria and Acaryochloris were dominant in high salinity soils, and non-heterocystic cyanobacteria were dominant in dry soils (Mehda et al., 2021), which is consistent with the results obtained by HJ in this study. Cyanobacteria are alkaline lovers that grow best in neutral to slightly alkaline soils (Belnap and Lange, 2003). The soil of the selected communities in karst desertification areas is neutral to acidic, but cyanobacteria species are still abundant, indicating that cyanobacteria can grow and reproduce well in acidic environments. From the analysis of our paper, it can be seen that the soils of HJ are characterized by low soil moisture content (about 7–15%), and sandy loam; the average content of organic carbon is 25.09 and total nitrogen is 3.49; the content of clay is 7.35%, resulting in low soil moisture content supporting the growth of cyanobacteria; SLX soils are characterized by rich soil moisture content (over 17%); the coarse sand content is larger than the other two study areas, and the content of clay is 7.98%, rich in organic carbon and nitrogen, and the soils of SB are characterized by low soil moisture content, similar to HJ, and relatively poor in soil nutrients. The highest organic carbon and total nitrogen content of HJ were dominated by Oscillatoria and Lyngbya, such as the unique Oscillatoria animalis and Oscillatoria limosa, etc. In addition, a unique genus Raphidiopsis exists. Similarly, the organic carbon and total nitrogen content of SLX was second only to that of HJ, and Oscillatoria was also dominant, with the unique species Oscillatoria prolifica and Oscillatoria agardhii, etc. It can be seen that soils rich in organic carbon and total nitrogen are dominated by cyanobacterial crusts of filamentous non-heterocystic Oscillatoria. In contrast, soils with high moisture content and coarse texture were dominated by cyanobacterial crusts of Gloeocapsa, such as Gloeocapsa montana Kütz and Gloeocapsa montana in SLX, in agreement with the conclusions reached by Patova et al. (2018), and the unique genera Plectonema, Anabaena, Cyanostylon, etc. The temperature of SB is suitable, the soil texture is fine and relatively poor in soil moisture and nutrients, and is dominated by cyanobacterial crusts of filamentous heteromorphic Scytonema, such as Scytonema hofmanni and Scytonema julianum, etc. In addition, there also exists the unique Symploca and Calothrix species.
5. Conclusion
1. A total of 200 species of cyanobacteria in two classes, five orders, six families, and 22 genera were identified in the three karst desertification study areas: the species composition of cyanobacteria is similar to that of desertification areas, all of which are common species, with Oscillatoriaceae, Chroococcaceae, Scytonemataceae, and Nostocaceae as common families, among which M. vaginatus and N. commune are generally dominant, which is related to the wide ecological range of generalist cyanobacteria; the dominant family and genus in the three desertification study areas were different, with Oscillatoriaceae being the dominant family in the moderate–severe desertification study area of HJ, Chroococcaceae in the potential-mild desertification study area of SLX, and Scytonemataceae in the no-potential desertification study area of SB.
2. The diversity of cyanobacteria in the karst desertification study area is related to three soil properties: soil moisture content (0–5 cm), organic carbon, and total nitrogen, but other soil properties affecting the cyanobacterial diversity need to be explored in combination with more soil properties in karst desertification areas. Cyanobacterial diversity varies between community habitats, with the highest number of species in both the HJ and SLX study areas of limestone lithology in shrubland and the highest diversity in grassland. The number of species was highest in arbor woodland, and the diversity was highest in bare land in the dolomite study area of SB. In addition to lithology and soil properties, this is also related to the increase in soil nutrients due to vegetation litter, trapping of surface water, and light intensity directly influenced by vegetation cover.
3. There is a synergistic evolution of suitable cyanobacteria in the karst desertification study area in response to changes in the soil properties. Cyanobacterial crusts of dry and hot soils (7–15% moisture content), and soils rich in organic carbon and total nitrogen, are dominated by Oscillatoria, reflecting the physiological adaptability of Oscillatoria to dry and hot environments and its carbon and nitrogen-loving properties. Cyanobacterial crusts of Gloeocapsa are dominant in soils with high moisture content (above 17%) and coarse texture, reflecting the resistance of Gloeocapsa to cold and wet conditions and influenced by coarse soil particles. Scytonema is influenced by dolomite lithology and is tolerant of infertile soils at suitable temperatures, so poor nutrients and low moisture content are dominated by Scytonema as the dominant cyanobacterial crust.
Data availability statement
The original contributions presented in the study are included in the article/supplementary material, further inquiries can be directed to the corresponding author.
Author contributions
QC and NY conceived the work, conducted data sorting, and analysis. NY, QC, and JZ collected the biocrust samples and soil samples. QC performed the microscopic study for cyanobacteria phenotypic characterization. NY performed the soil physicochemical analysis. JZ collected literature. QC wrote the manuscript and NY revised it. KX provided financial support and summarized manuscripts. All authors contributed to the article and approved the submitted version.
Funding
This research was financially supported by the Key Project of Science and Technology Program of Guizhou Province (No. 5411 2017 Qiankehe Pingtai Rencai); the China Overseas Expertise Introduction Program for Discipline Innovation (No. D17016); the National Major Research and Development Program of China (2016YFC0502607).
Conflict of interest
The authors declare that the research was conducted in the absence of any commercial or financial relationships that could be construed as a potential conflict of interest.
Publisher’s note
All claims expressed in this article are solely those of the authors and do not necessarily represent those of their affiliated organizations, or those of the publisher, the editors and the reviewers. Any product that may be evaluated in this article, or claim that may be made by its manufacturer, is not guaranteed or endorsed by the publisher.
References
Bahl, J., Lau, M. C., Smith, G. J., Vijaykrishna, D., Cary, S. C., Lacap, D. C., et al. (2011). Ancient origins determine global biogeography of hot and cold desert cyanobacteria. Nat. Commun. 2, 163–166. doi: 10.1038/ncomms1167
Belnap, J. (2002). Nitrogen fixation in biological soil crusts from Southeast Utah. USA. Biol. Fertil. Soils. 35, 128–135. doi: 10.1007/s00374-002-0452-x
Belnap, J., Harper, K., and Warren, S. (1993). Surface disturbance of cryptobiotic soil crusts: Nitrogenase activity, chlorophyll content, and chlorophyll degradation. Arid Land Res. Manag. 8, 1–8. doi: 10.1080/15324989309381373
Belnap, J., and Lange, O. L. (2001). Structure and functioning of biological soil crusts: a synthesis. Biol. soil crusts: struct, function, Manag. 150, 471–479. doi: 10.1007/978-3-642-56475-8_33
Belnap, J., and Lange, O. L. (2003) Biological soil crusts: structure, function, and management. Ecol. stud. 150.
Belnap, J., and Miller, P. (2004). Response of desert biological soil crusts to alterations in precipitation frequency. Oecologia 141, 306–316. doi: 10.1007/s00442-003-1438-6
Belnap, J., Weber, B., and Büdel, B. (2016). Biological soil crusts: an organizing principle in drylands. Ecol. Studies 226, 3–13. doi: 10.1007/978-3-319-30214-0
Büdel, B., Darienko, T., Deutschewitz, K., Mohr, K. I., and Weber, B. (2009). Southern African biological soil crusts are ubiquitous and highly diverse in drylands, being restricted by rainfall frequency. Microb. Ecol. 57, 229–247. doi: 10.1007/S00248-008-9449
Büdel, B., Williams, W. J., and Reichenberger, H. (2018). Annual net primary productivity of a cyanobacteria-dominated biological soil crust in the Gulf Savannah, Queensland. Aust. Biogeosci. 15, 491–505. doi: 10.5194/bg-15-491-2018
Cano-Díaz, C., Mateo, P., Muoz-Martín, M. A., and Maestre, F. T. (2018). Diversity of biocrust-forming cyanobacteria in a semiarid gypsiferous site from Central Spain. J. Arid Environ. 151, 83–89. doi: 10.1016/j.jaridenv.2017.11.008
Chamizo, S., Cantón, Y., Rodríguez-Caballero, E., and Domingo, F. (2016). Biocrusts positively affect the soil water balance in semiarid ecosystems. Ecohydrology 9, 1208–1221. doi: 10.1002/eco.1719
Chen, Y. B., Xiong, K. N., and Chi, Y. K. (2019). Process of rocky desertification control in karst area of southern China. Jiangsu. J. Agric. Sci. 47, 17–21. doi: 10.15889/j.issn.1002-1302.2019.01.004
Dvořák, P., Casamatta, D. A., Hašler, P., Jahodářová, E., Norwich, A. R., and Poulíčková, A. (2017). “Diversity of the cyanobacteria” in Modern Topics in the Phototrophic Prokaryotes (Cham: Springer), 3–46.
Dvořák, P., Jahodářová, E., Casamatta, D. A., Hašler, P., and Poulíčková, A. (2018). Difference without distinction? Gaps in cyanobacterial systematics; when more is just too much. J. Czech. Phycol. Soc. 18, 130–136. doi: 10.5507/fot.2017.023
Etemadi-Khah, A., Pourbabaee, A. A., Alikhani, H. A., Noroozi, M., and Bruno, L. (2017). Biodiversity of isolated cyanobacteria from desert soils in Iran. Geomicrobiol J. 34, 784–794. doi: 10.1080/01490451.2016.1271064
Felde, V. J. M. N. L., Chamizo, S., Felix-Henningsen, P., and Drahorad, S. L. (2018). What stabilizes biological soil crusts in the Negev Desert? Plant Sci. 429, 9–18. doi: 10.1007/s11104-017-3459-7
Fernandes, V. M. C., Machado de Lima, N. M., Roush, D., Rudgers, J., Collins, S. L., and Garcia-Pichel, F. (2018). Exposure to predicted precipitation patterns decreases population size and alters community structure of cyanobacteria in biological soil crusts from the Chihuahuan Desert. Environ. Microbiol. 20, 259–269. doi: 10.1111/1462-2920.13983
Garcia-Pichel, F., Lopez-Cortes, A., and Nubel, U. (2001). Phylogenetic and morphological diversity of cyanobacteria in soil desert crusts from the Colorado plateau. Appl. Environ. Microbiol. 67, 1902–1910. doi: 10.1128/aem.67.4.1902-1910.2001
Gaysina, L. A., Bohunicka, M., Hazukova, V., and Johansen, J. R. (2018). Biodiversity of terrestrial cyanobacteria of the South Ural region. Cryptogam. Algol. 39, 167–198. doi: 10.7872/crya/v39.iss2.2018.167
Hagemann, M., Henneberg, M., Felde, V. J. M. N. L., Drahorad, S. L., Berkowicz, S. M., Felix-Henningsen, P., et al. (2015). Cyanobacterial diversity in biological soil crusts along a precipitation gradient, Northwest Negev Desert. Israel. Microb. Ecol. 70, 219–230. doi: 10.1007/s00248-014-0533-z
Hakkoum, Z., Minaoui, F., Douma, M., Mouhri, K., and Loudiki, M. (2020). Diversity and spatial distribution of soil cyanobacteria along an altitudinal gradient in Marrakesh area (Morocco). Appl. Ecol. Environ. Res. 18, 5527–5545. doi: 10.15666/aeer/1804_55275545
Housman, D. C., Powers, H. H., Collins, A. D., and Belnap, J. (2006). Carbon and nitrogen fixation differ between successional stages of biological soil crusts in the Colorado plate au and Chihuahuan Desert. J. Arid Environ. 66, 620–634. doi: 10.1016/j.jaridenv.2005.11.014
Hu, C. X., Liu, Y. D., and Song, L. R. (2002). New development of soil algae research. Acta. Hydrobiol. Sin. 26, 521–528. doi: 10.3321/j.issn:1000-3207.2002.05.018
Khomutovska, N., de Los Ríos, A., and Jasser, I. (2020). Diversity and colonization strategies of endolithic cyanobacteria in the cold mountain desert of Pamir. Microorganisms. 9:6. doi: 10.3390/microorganisms9010006
Lacap-Bugler, D. C., Lee, K. K., Archer, S., Gillman, L. N., Lau, M. C., Leuzinger, S., et al. (2017). Global diversity of desert hypolithic cyanobacteria. Front. Microbiol. 8:867. doi: 10.3389/fmicb.2017.00867
Li, X. R., Jia, R. L., Zhang, Z. S., Zhang, P., and Hui, R. (2018). Glob. Change. Biol. 24:Hydrological response ofbiological soil crusts to global warming: A ten-year simulative study, 4960–4971. doi: 10.1111/gcb.14378
Loza, V., Perona, E., and Mateo, P. (2014). Specific responses to nitrogen and phosphorus enrichment in cyanobacteria: factors influencing changes in species dominance along eutrophic gradients. Water Res. 48, 622–631. doi: 10.1016/j.watres.2013.10.014
Machado de Lima, N. M., Fernandes, V. M. C., Roush, D., Velasco Ayuso, S., Rigonato, J., Garcia-Pichel, F., et al. (2019). The compositionally distinct cyanobacterial biocrusts from Brazilian savanna and their environmental drivers of community diversity. Front. Microbiol. 10:2798. doi: 10.3389/fmicb.2019.02798
Machado de Lima, N. M., Muñoz-Rojas, M., Vázquez-Campos, X., and Branco, L. H. Z. (2021). Biocrust cyanobacterial composition, diversity, and environmental drivers in two contrasting climatic regionsin Brazil. Geoderma 386:114914. doi: 10.1016/j.geoderma.2020.114914
Mehda, S., Muñoz-Martin, M. Á., Oustani, M., Hamdi-Aïssa, B., Perona, E., and Mateo, P. (2021). Microenvironmental conditions drive the differential cyanobacterial community composition of biocrusts from the Sahara Desert. Microorganisms 9:487. doi: 10.3390/microorganisms9030487
Muhlsteinova, R., Johansen, J. R., Pietrasiak, N., Martin, M. P., Osorio-Santos, K., and Warren, S. D. (2014). Polyphasic characterization of Trichocoleus desertorum sp. nov.(Pseudanabaenales, cyanobacteria) from desert soils and phylogenetic placement of the genus Trichocoleus. Phytotaxa. 163, 241–261. doi: 10.11646/phytotaxa.163.5.1
Novakovskaya, I. V., Patova, E. N., Dubrovskiy, Y. A., Novakovskiy, A. B., and Kulyugina, E. E. (2022). Distribution of algae and cyanobacteria of biological soil crusts along the elevation gradient in mountain plant communities at the northern Urals(Russian European northeast). J. Mt. Sci. 19, 637–646. doi: 10.1007/s11629-021-6952-7
Patova, E. N., Novakovskaya, I. V., and Deneva, S. V. (2018). The influence of edaphic and orographic factors on algal diversity in biological soil crusts on bare spots in the polar and subpolar Urals. Eurasian Soil Sci. 51, 309–320. doi: 10.1134/s1064229318030109
Perera, I., Subashchandrabose, S. R., Venkateswarlu, K., Naidu, R., and Megharaj, M. (2018). Consortia of cyanobacteria/microalgae and bacteria in desert soils: an underexplored microbiota. Appl. Microbiol. Biotechnol. 102, 7351–7363. doi: 10.1007/s00253-018-9192-1
Pushkareva, E., Johansen, J. R., and Elster, J. (2016). A review of the ecology, ecophysiology and biodiversity of microalgae in Arctic soil crusts. Polar Biol. 39, 2227–2240. doi: 10.1007/s00300-016-1902-5
Pushkareva, E., Pessi, I. S., Namsaraev, Z., Mano, M. J., Elster, J., and Wilmotte, A. (2018). Cyanobacteria inhabiting biological soil crusts of a polar desert: Sør Rondane Mountains. Antarct. Syst. Appl. Microbiol. 41, 363–373. doi: 10.1016/j.syapm.2018.01.006
Pushkareva, E., Pessi, I. S., Wilmotte, A., and Elster, J. (2015). Cyanobacterial community composition in Arctic soil crusts at different stages of development. FEMS Microbiol. Ecol. 91:fiv143. doi: 10.1093/femsec/fiv143
Quesada, A., Nieva, M., Leganés, F., Ucha, A., Martín, M., Prosperi, C., et al. (1998). Acclimation of Cyanophytal communities in rice fields and response of nitrogenase acti vity to light regime. Microb. Ecol. 35, 147–155. doi: 10.1007/s002489900069
Roncero-Ramos, B., Muñoz-Martín, M. Á., Chamizo, S., Fernández-Valbuena, L., Mendoza, D., Perona, E., et al. (2019). Polyphasic evaluation of key cyanobacteria in biocrusts from the most arid region in Europe. PeerJ. 7:e6169. doi: 10.7717/peerj.6169
Ravbar, N., and Šebela, S. (2015). The effectiveness of protection policies and legislative framework with special regard to karst landscapes: insights from Slovenia. Environ. Sci. Pol. 51, 106–116. doi: 10.1016/j.envsci.2015.02.013
Redfield, E., Barns, S. M., Belnap, J., Daane, L. L., and Kuske, C. R. (2002). Comparative diversity and composition of cyanobacteria in three predominant soil crusts of the Colorado plateau. FEMS Microbiol. Ecol. 40, 55–63. doi: 10.1111/j.1574-6941.2002.tb00936.x
Řeháková, K., Chlumská, Z., and Doležal, J. (2011). Soil Cyanobacterial and microalgal diversity in dry mountains of Ladakh, NW Himalaya, as related to site, altitude, and vegetation. Microb. Ecol. 62, 337–346. doi: 10.1007/s00248-011-9878-8
Rodriguez-Caballero, E., Stanelle, T., Egerer, S., Cheng, Y., Su, H., Canton, Y., et al. (2022). Global cycling and climate effects of aeolian dust controlled by biological soil crusts. Nat. Geosci. 15:458-+. doi: 10.1038/s41561-022-00942-1
Sheng, M. Y., Xiong, K. N., Wang, L. J., Li, X. N., Li, R., and Tian, X. J. (2016). Response of soil physical and chemical properties to rocky desertification succession in South China karst. Carbonates Evaporites 33, 15–28. doi: 10.1007/s13146-016-0295-4
Sosa-Quintero, J., Godínez-Alvarez, H., Camargo-Ricalde, S. L., Gutiérrez-Gutiérrez, M., Huber-Sannwald, E., Jiménez-Aguilar, A., et al. (2022). Biocrusts in Mexican deserts and semideserts: a review of their species composition, ecology, and ecosystem function. J. Arid Environ. 199:104712. doi: 10.1016/j.jaridenv.2022.104712
Starks, T. L., Shubert, L. E., and Trainor, F. R. (1981). Ecology of soil algae: a review. Phycologia 20, 65–80. doi: 10.2216/i0031-8884-20-1-65.1
Temraleeva, A. D. (2018). Cyanobacterial diversity in the soils of Russian dry steppesand semideserts. Microbiology 87, 249–260. doi: 10.1134/s0026261718020169
Vijayan, D., and Ray, J. G. (2015). Ecology and diversity of cyanobacteria in KuttanaduPaddy wetlands, Kerala. India. Am. J. Plant. Sci. 6, 2924–2938. doi: 10.4236/ajps.2015.618288
Wang, S., Gao, E. G., Luo, X. J., Xu, Y., and Liu, Y. L. (2019). Characteristics of forest communities of typical evergreen broad-leaved forest in Shibing. Mol. Plant. Breed. 17, 3432–3441. doi: 10.13271/j.mpb.017.003432
Warren-Rhodes, K. A., Rhodes, K. L., Pointing, S. B., Ewing, S. A., Lacap, D. C., Gomez-Silva, B., et al. (2006). Hypolithic cyanobacteria, dry limit of photosynthesis, and microbial ecology in the hyperarid Atacama Desert. Microb. Ecol. 52, 389–398. doi: 10.2307/25153391
Whitton, B. A., and Potts, M. (Eds.) (2007). The Ecology of Cyanobacteria: Their Diversity in Time and Space Springer Science & Business Media.
Williams, L., Loewen-Schneider, K., Maier, S., and Büdel, B. (2016). Cyanobacterial diversity of western European biological soil crusts along a latitudinal gradient. FEMS Microbiol. Ecol. 92:fiw157. doi: 10.1093/femsec/fiw157
Yan, N., Zhang, T., Xiong, K. N., Chen, Q., Guo, D. L., and Liu, Z. W. (2021). Terrestrial algae diversity of the two world natural heritage sites in China. Acta Ecol. Sin. 41, 9593–9603.
Yang, H. Y., Liang, Y. H., Fan, Y. L., Zhang, F. T., and Luo, X. Q. (2013). Characteristics of soil algae community structure in Huajiang karst area of Guizhou. Guizhou Agric. Sci. 41, 102–105. doi: 10.3969/j.issn.1001-3601.2013.11.027
Zhang, X. C., Li, J. Y., Liu, J. L., Yuan, C. X., Li, Y. N., Liu, B. R., et al. (2021). Temporal shifts in cyanobacterial diversity and their relationships to different types of biological soil crust in the southeastern Tengger Desert. Rhizosphere. 17:100322. doi: 10.1016/j.rhisph.2021.100322
Zhang, B., Li, R., Xiao, P., Su, Y., and Zhang, Y. (2015). Cyanobacterial composition and spatial distribution based on pyrosequencing data in the Gurbantunggut Desert, northwestern China. J. Basic Microbiol. 56, 308–320. doi: 10.1002/jobm.201500226
Zhang, N., Ye, C., An, M. T., Chen, L., and Ran, X. Z. (2019). Study on plant diversity during natural restoration of karst vegetation in Huajiang grand canyo. Seed. 38:64-67+71. doi: 10.16590/j.cnki.1001-4705.2019.12.064
Zhang, B. C., Zhang, Y. M., and Zhao, J. C. (2005a). Composition and ecological distribution of the algae living in the Gurbantonggut desert of Xinjiang. Acta. Bot. Boreali-Occident. Sin. 25, 2048–2055. doi: 10.3321/j.issn:1000-4025.2005.10.020
Zhang, B. C., Zhang, Y. M., Zhao, J. C., Chen, R. Y., and Zheng, Y. P. (2009). Variation in algal composition among different developmental stages of biological soil crusts in Gurbantunggut Desert. Acta Ecol. Sin. 29, 9–17. doi: 10.3321/j.issn:1000-0933.2009.01.002
Zhang, B. C., Zhang, Y. M., Zhao, J. C., and Zhang, R. C. (2005b). Study on cyanobacteria of biological soil crusts in Guerbantunggut desert, Zhungar basin, sinkiang. Geogr. Geo-Infor. Sci. 21, 107–109. doi: 10.10007/978-3-642-56475-8
Zhang, B. C., Zhao, J. C., Zhang, Y. M., Li, M., and Zhang, J. (2008). Vertical distribution of algae in different locations of sand dunes in the Gurbantunggut Desert, Xinjiang. China. Chin. J. Plant. Ecol. 32, 456–464. doi: 10.3773/j.issn.1005-264x.2008.02.025
Keywords: biological soil crusts, cyanobacteria, species diversity, soil properties, karst desertification
Citation: Chen Q, Yan N, Xiong K and Zhao J (2023) Cyanobacterial diversity of biological soil crusts and soil properties in karst desertification area. Front. Microbiol. 14:1113707. doi: 10.3389/fmicb.2023.1113707
Edited by:
Xiangyu Guan, China University of Geosciences, ChinaCopyright © 2023 Chen, Yan, Xiong and Zhao. This is an open-access article distributed under the terms of the Creative Commons Attribution License (CC BY). The use, distribution or reproduction in other forums is permitted, provided the original author(s) and the copyright owner(s) are credited and that the original publication in this journal is cited, in accordance with accepted academic practice. No use, distribution or reproduction is permitted which does not comply with these terms.
*Correspondence: Kangning Xiong, xiongkn@163.com
†These authors have contributed equally to this work and share first authorship