- 1Department of Genomic Science, School of Biological Sciences, Central University of Kerala, Kerala, India
- 2Department of Microbiology, School of Life Sciences, Pondicherry University, Puducherry, India
- 3Department of Biochemistry and Molecular Biology, School of Life Sciences, Pondicherry University, Puducherry, India
- 4Biochemistry, Molecular Biology, Edaphology and Agricultural Chemistry Department, Faculty of Sciences, University of Alicante, Alicante, Spain
- 5Multidisciplinary Institute for Environmental Studies “Ramón Margalef”, University of Alicante, Alicante, Spain
- 6Department of Biotechnology, School of Life Sciences, Pondicherry University, Puducherry, India
Marine environments and salty inland ecosystems encompass various environmental conditions, such as extremes of temperature, salinity, pH, pressure, altitude, dry conditions, and nutrient scarcity. The extremely halophilic archaea (also called haloarchaea) are a group of microorganisms requiring high salt concentrations (2–6 M NaCl) for optimal growth. Haloarchaea have different metabolic adaptations to withstand these extreme conditions. Among the adaptations, several vesicles, granules, primary and secondary metabolites are produced that are highly significant in biotechnology, such as carotenoids, halocins, enzymes, and granules of polyhydroxyalkanoates (PHAs). Among halophilic enzymes, reductases play a significant role in the textile industry and the degradation of hydrocarbon compounds. Enzymes like dehydrogenases, glycosyl hydrolases, lipases, esterases, and proteases can also be used in several industrial procedures. More recently, several studies stated that carotenoids, gas vacuoles, and liposomes produced by haloarchaea have specific applications in medicine and pharmacy. Additionally, the production of biodegradable and biocompatible polymers by haloarchaea to store carbon makes them potent candidates to be used as cell factories in the industrial production of bioplastics. Furthermore, some haloarchaeal species can synthesize nanoparticles during heavy metal detoxification, thus shedding light on a new approach to producing nanoparticles on a large scale. Recent studies also highlight that exopolysaccharides from haloarchaea can bind the SARS-CoV-2 spike protein. This review explores the potential of haloarchaea in the industry and biotechnology as cellular factories to upscale the production of diverse bioactive compounds.
1. Introduction: Haloarchaeal diversity and ecology
Microorganisms possess several mechanisms to acclimatize to stress conditions that influence growth and survival in saline environments. Halophiles are microbes that can survive such saline conditions from low to high saturation points. There are different stress proteins and strategies that halophiles adapt to counteract stressful factors such as ions, temperature, pH, and UV radiation. Prokaryotic halophiles have attracted the attention of researchers worldwide because of their distinctive features, ease of manipulation, lesser space requirements for cultivation, and the production of diverse metabolites compared to plants or eukaryotic algae (Torregrosa-Crespo et al., 2017; Dutta and Bandopadhyay, 2022). A significant group of halophilic archaea, represented under the halobacteria class, are tolerant to extreme saline environments. These environments include salt lakes, estuaries, rivers, mangrove swamps, open seawater, coastal waters, salt lakes, estuaries, and salt deserts. Halobacterial class constitutes a wide range of genera (Figure 1) – Salarchaeum, Halobiforma, Natronolimnobius, Halopelagius, Halogranum, Halonotius, Haladaptatus, Natronococcus, Haloferax, Halococcus, Haloalcalophilium, Halorubrum, Halorhabdus, Halorussus, Halopiger, Halomarina, Natronoarchaeum, Halobellus, Natrialba Halobaculum, Haloplanus, Halostagnicola, Halorientalis, Natronomonas, Natrialba, Natronobacterium, Natronorubrum, Haloarcula, Halobacterium, Haloterrigena, Natrinema, Halogeometricum, Halalkalicoccus, Haloquadratum, Halogeometricum, Natronorubrum, Halomicrobium, Halolamina, Halovivax, Halarchaeum, and Halosimplex (Cui and Dyall-Smith, 2021).
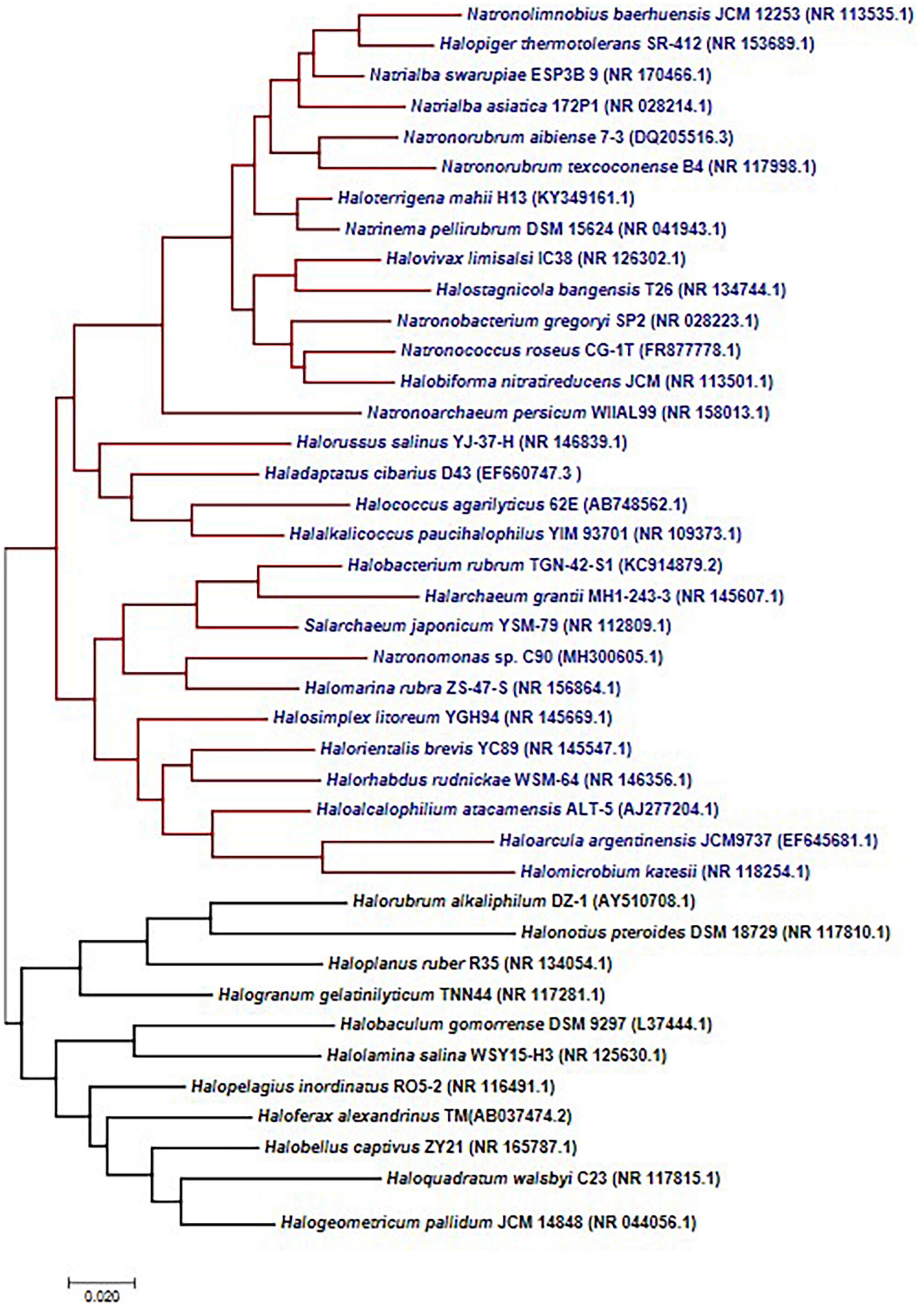
Figure 1. Haloarchaeal 16s RNA gene diversity. Phylogenetic tree constructed using the maximum likelihood methods suggests two separate clades, and most of the organisms are represented under a single clade.
Considering the difficulties found by researchers to obtain pure cultures of extremophilic microorganisms (including halophilic archaea) from environmental samples, and consequently to know the microbial biodiversity in those samples, recent advances in omic-based approaches have contributed to a better understanding of haloarchaeal biodiversity. Particularly, metagenomic analysis has been used to overcome this limitation. Metagenomic analysis of environmental samples from the Dead Sea, RedSea, Gulf of Cambay, Mediterranean Sea, Sundarbans mangrove forest, Karak Salt Mine, and Pannonian Steppe, revealed the predominance of haloarchaeal genera in the natural environment, including Haloarcula, Halorubrum, and Halorhabdus (Oh et al., 2010; Rhodes et al., 2012; Somboonna et al., 2012; Fernández et al., 2014; Bhattacharyya et al., 2015; Keshri et al., 2015; Behzad et al., 2016; Szabó et al., 2017; Haldar and Nazareth, 2018; Osman et al., 2019; Cecil et al., 2020). Similarly, a global metagenomic meta-analysis revealed the dominance of Haloarcula and Haloquandratum sp., in the seacoast (Moopantakath et al., 2021). The biogeography of haloarchaea also varies based on the biosystems. For instance, the Haloferax genus is highly dominant in seashores and island samples and estuaries, while the Natrialba genus is predominant in rivers, mangroves, and lakes (Hegazy et al., 2020; Moopantakath et al., 2020; Cho et al., 2021). Thus, the prevalence of halophilic microorganisms in general, and particularly of haloarchaea, is highly dependent on the environment, geography, and physicochemical parameters like salinity, pH, oxygen availability and sun radiation, among other factors (Ventosa, 2006; Mani et al., 2020). As an example, recent whole genome sequencing studies revealed that autotrophic haloarchaea are highly abundant in coastal environments, probably due to salinity and direct sunlight exposure (Moopantakath et al., 2021). These environments display unique features and salt deposition phenomena that contribute to the increase of some haloarchaeal populations requiring extremely high concentrations for optimal growth.
Environmental degradation, such as the release of industrial chemicals into the coastal environment, is also a major factor in pollution and shoreline contamination (Lu et al., 2018) and in microbial biodiversity. Nonetheless, the microbial community plays a significant role in the polluted site, and the unique metabolic features of haloarchaea contribute to the homeostasis of these environments. Halorhabdus and Natrinema sp., have been reported to degrade xylan and produce halocin (antimicrobial peptide), respectively (Begemann et al., 2011; Besse et al., 2017). Similarly, recent advances in the metagenomic analysis have revealed that haloarchaea, such as Haloferax, Haladaptatus, Natrialba, etc., participate in maintaining the biogeochemical processes in coastal ecosystems (Osman et al., 2019). Some haloarchaea can carry out interesting metabolic processes from an industrial point of view; examples: sulfur reduction by Natranaeroarchaeum sulfidigenes (Sorokin et al., 2022), nitrification by Haloarcula, Halolamina, and Halobacterium (Wei et al., 2022), phosphorus solubilization by Haloarcula, Halobacterium, Halococcus, and Haloferax (Yadav et al., 2015) or denitrification by Haloarcula or Haloferax (Miralles-Robledillo et al., 2021).
In summary, haloarchaea exhibit diverse metabolic pathways and biological activities of interest for biotechnological purposes. They constitute predominant microbial communities in salty ecosystems, which are widespread worldwide. For example, Haloarcula, Haloferax, and Halogeometricum sp., can be isolated from saline sediments across the world, such as from Spanish coastal and inland salted ponds (Martínez et al., 2022), Algerian salt lakes, Indian salt pans, Verkhnekamsk deposit etc., and exhibit several biological activities, including the production of carotenoid pigments (Das et al., 2019; Sahli et al., 2022). Besides, many more ecosystems characterized by their high salt concentrations are far from known from a microbiological point of view (i.e., saline mines in Senegal). This review aims to summarize new advances in the knowledge of biological applications of halophilic archaea and the synthesis of secondary metabolites thus contributing to the design of new biotech processes low cost and environmentally friendly based on the use of haloarchaea as cellular factories.
1.1. Haloarchaeal strategies to cope with stress
Haloarchaea can survive in stress conditions such as salinity, ultraviolet (UV), high concentration of ions, high temperature, and extreme pH values. In addition, continuous heavy rain or change in temperature can lead to a dramatic shift in the salinity, causing significant pressure on haloarchaea and promoting the switching on of molecular machinery to be better adapted to these environmental changes (Griffiths and Philippot, 2013; Thombre et al., 2016). Halophiles inhabiting saline environments can exist at different salt concentrations, mainly above 1 M. Based on the optimum salinity requirements, halophiles can be classified into slight halophiles (0.34 to 0.85 M), moderate halophiles (0.85 to 3.4 M), and extreme halophiles (3.4 M to saturation point) (Abaramak et al., 2020). Haloarchaea requires ∼10 to 35% w/v (1.71 to 6 M) of salt concentration for optimum growth. Interestingly, haloarchaea are the dominant class of microbes when the salt concentration increases above 16% w/v (2.74 M) (Oren, 2002b; Andrei et al., 2012).
Haloarchaea evolved with several metabolic adaptations to survive different stresses among which salt stress is one of the most significant affecting the protein structures and therefore, their biological activities (Britton et al., 2006). Thus, most of their proteins are salt dependent for optimal enzymatic activity and stability. The unique feature of these proteins is due to the presence of acidic amino acids on the surface (Esclapez et al., 2007). The negatively charged acidic amino acids on the surface develop into a cluster form and interact with networks of hydrated ions. Consequently, this feature avoids the precipitation of haloarchaeal proteins under high KCl/NaCl concentrations. Also, proteins exhibit less hydrophobic interactions owing to the limited content of hydrophilic amino acids such as lysine. Hence, a lack of optimum salt concentration may cause the unfolding of proteins owing to the presence of negatively charged amino acids (Kennedy et al., 2001; Oren, 2002a; Zafrilla et al., 2010; Andrei et al., 2012).
Some haloarchaea are called polyextremophiles owing to their ability to respond to multiple extreme conditions (Das and Dash, 2018). They can adjust to osmotic stress and survive at low water activity and desiccation. The presence of high salt concentrations may reduce water activity from 1 to 0.75. Further, salt-in and low-salt-in are the two methods adopted by haloarchaea to resist osmotic stress. In the salt-in method, K+ is accumulated inside cells with the help of protein transport and ion pumps (Schäfer et al., 1996; Oren, 2013). Halobacterium sp., NRC-1 utilizes the salt-in strategy with the help of potassium transporters and sodium efflux pumps (Ng et al., 2000).
In the low-salt-in method, some organisms produce compatible and low molecular-weight solutes to adapt to osmotic stress (Grant et al., 2004). The compatible solutes such as amino acids, ectoines, thetines, polyols, betaines, derivatives of sugar, and glutamine amide are accumulated in low concentration in the cytoplasm to tolerate osmotic stress (Matarredona et al., 2020). For instance, solutes like 2-sulphotrehalose, and glycine produced by Natronobacterium and Natronococcus, respectively, help in the low-salt process (Grant et al., 2004; Matarredona et al., 2020). The square-shaped haloarchaea, Haloquadratum walsbyi, carries a unique protein, halomucin, which helps to survive desiccation. The protein is glycosylated and sulfated to develop as a water-rich capsule around the archaea. The water-rich cloud formed around the cell protects it from surrounding desiccation or the presence of low water activity (Bolhuis et al., 2006; Dyall-Smith et al., 2011; Zenke et al., 2015).
Regarding stress due to temperature changes, haloarchaea can tolerate different temperature variations in saline environments thanks to the presence of heat shock proteins (e.g., chaperones and chaperonins). The molecular chaperones are involved in the folding or unfolding of proteins at extreme temperatures (Fenderson, 2006; Shukla, 2006; Coker et al., 2007). The most common heat shock proteins observed in haloarchaea are Hsp60 and 70 (Macario et al., 1999). The expression and synthesis of cold shock proteins, polar lipids, and gas vesicles in cold temperatures help to maintain homeostasis (Coker et al., 2007). Some haloarchaea, for example, Haloferax, produce thermoprotectans, such as glycoside hydrolases, to withstand high temperatures by promoting protein stabilization (Amin et al., 2021).
The ecosystems inhabited by haloarchaea are exposed to high sun radiation doses that cause UV irradiation and the formation of photoproducts and pyrimidine dimers in DNA. The photoreactivation process can remove these lesions with the help of photolyase expressed by haloarchaea. Haloarchaea has unique compounds that include rhodopsin which has a phototaxis mechanism. On the other hand, gas vesicles play a crucial role in light regulation and responses to oxygen availability changes (Jones and Baxter, 2017; Miralles-Robledillo et al., 2021). Response to UV irradiation also includes the downregulation of genes involved in the gas vesicle production to sink the cells below the water surface (Kottemann et al., 2005). Some haloarchaea, such as Halorubrum lacusprofundi and Haloferax volcanii, can withstand a wide range of pH. They can exist in both low-pH environments like acidic lakes and alkaline lakes (Mormile et al., 2009). In the case of Halorubrum lacusprofundi, Haloferax volcanii, and Halobacterium sp., residing in alkaline pH conditions, it has been described that several stress genes such as hsp20 family, universal stress protein uspA, or groEL chaperone are upregulated. In contrast, H. lacusprofundi exhibited upregulation of hlac3059 and hlac3556 gene expression in acidic pH. Besides, they display dormancy-specific responses at acidic pH to survive in the environment (Moran-Reyna and Coker, 2014).
2. Biotechnological significance of haloarchaea
Several biotechnological-based processes can be benefited from the use of haloarchaea. Thus, the use of whole cells in wastewater treatments and bioremediation of brines and salty soils has been recently revealed as a promising tool. Hypersaline wastewater is a common byproduct of industrial processes. Hence, cost-effective treatment of hypersaline wastewater is necessary for sustainable development. Biological wastewater treatment has been considered a more economical approach, but mesophilic microorganisms used so far for this purpose can not be used in the biological treatment of polluted brines of wastewater containing high salt concentration. In this context, recent research findings revealed the role of haloarchaea in the treatment of salty wastewater and brine generated as final residues in water desalination plants (Li et al., 2021; Martínez et al., 2022). Haloarchaea can also degrade hydrocarbons; however, their degradation is more efficient for low molecular-weight hydrocarbons. The degradation of naphthalene, phenol, p-hydroxybenzoic acid, and 3-phenyl propionic acid, and oxychlorides like perchlorate or chlorate by haloarchaea also make it an attractive choice for wastewater treatment (Martínez-Espinosa et al., 2015; Mukherji et al., 2020; Li et al., 2021). Another interesting approach related to wastewater treatments is the removal of nitrogen to avoid eutrophication in the receiving water bodies and heavy metals to avoid global toxicity. Haloarchaea, such as Haloferax mediterranei, can use NO3– and NO2– as nitrogen sources for growth or as final electron acceptors instead of oxygen in an anaerobic respiratory process (denitrification). For example, Haloferax mediterranei encodes nitrate reductase (nas) and nitrite reductase (nir) that can perform assimilatory nitrate/nitrite reduction (Martínez-Espinosa et al., 2007), whilst Nar, NirK, Nor, and Nos encodes key enzymes in catalyzing the reactions involved in the process of denitrification (Bernabeu et al., 2021). Regarding heavy metals, several recent studies have demonstrated that some haloarchaeal species can grow in the presence of heavy metals at concentrations that are toxic for most living beings. In some cases, because of cellular growth, heavy metals are accumulated, modified or bioassimilated. Molecular machinery for the potential removal of cooper and cadmium has also been identified from many haloarchaeal species (Vera-Bernal and Martínez-Espinosa, 2021; Llorca and Martínez-Espinosa, 2022). In other cases, haloarchaea growing in the presence of heavy metals can also synthesize nanoparticles (NPs) from wastewater polluted with cadmium, arsenic, and zinc (Taran et al., 2017; Li et al., 2021; Gaonkar and Furtado, 2022). Haloarchaea have various mechanisms to tolerate the arsenate metals using minichromosomes/megaplasmids (arsADRC gene cluster) in the Halobacterium species (Wang et al., 2004; Voica et al., 2016). The zinc tolerance mechanism was found due to the presence of physical bioabsorption, ion exchange and intracellular accumulation which can be used for the various biological process inside the cell (Popescu and Dumitru, 2009; Williams et al., 2013; Salgaonkar et al., 2016). Similarly, cadmium also plays a crucial aid in intracellular metabolic functions so cadmium can be tolerated inside the cell without stress (Vera-Bernal and Martínez-Espinosa, 2021).
Another interesting biotechnological application is related to the enzymes from haloarchaea. They exhibit increased tolerance, not only to salinity but also to pH, pressure, temperature, etc. Haloenzymes have several advantages, such as minimum steps in purification, sterilization, and cost-effectiveness (Amoozegar et al., 2017). Haloenzymes such as lipase and alcohol dehydrogenase from Haloferax volcanii and Haloarcula sp., G41, respectively, have been immobilized successfully for increased activity (Alsafadi and Paradisi, 2014; Li and Yu, 2014). Saline-tolerant lipases and esterases like those described from Haloarcula marismortui and Natronococcus sp., TC6 are essential enzymes in several biotechnological applications such as biofuel, detergent, textile, etc (Camacho et al., 2009; Del Campo et al., 2015).
The haloarchaeal membrane protein bacteriorhodopsin, initially discovered from Halobacterium salinarum, is highly stable to thermal and photochemical stress. Bacteriorhodopsin can sense light and convert it into electrical signals (Singh and Singh, 2017). Bacteriorhodopsin from haloarchaea has also found applications in biosensors and artificial retinas (Figure 2; Amoozegar et al., 2017). The gas vesicles mentioned in section “1. Introduction: haloarchaeal diversity and ecology” have applications in drug delivery systems and vaccine development (Cánovas et al., 2021). Meanwhile, poly-β-hydroxy-alkanoates (PHAs) produced by haloarchaea, such as Haloferax mediterranei, are considered an alternative to plastics produced from petroleum and in medical applications owing to their biocompatibility (Salgaonkar et al., 2013; Li et al., 2021). Thus, PHA can be synthesized using these microorganisms as biofactories thanks to cheaper procedures in which the biomass downstream process as well as the purification process of the biopolymer can be done with single steps (Simó-Cabrera et al., 2021). PHA produced by various materials such as residues from food and agriculture products can be synthesized into PHA with a help of haloarchaea (Quillaguamán et al., 2010). Genomic insights of halophiles such as Halonotius terrestris sp., nov. and Halonotius roseus sp., nov. have been recently delineated and found to encode the complete biosynthetic genes for the biosynthesis of cobalamin (vitamin B12) (Durán-Viseras et al., 2019).
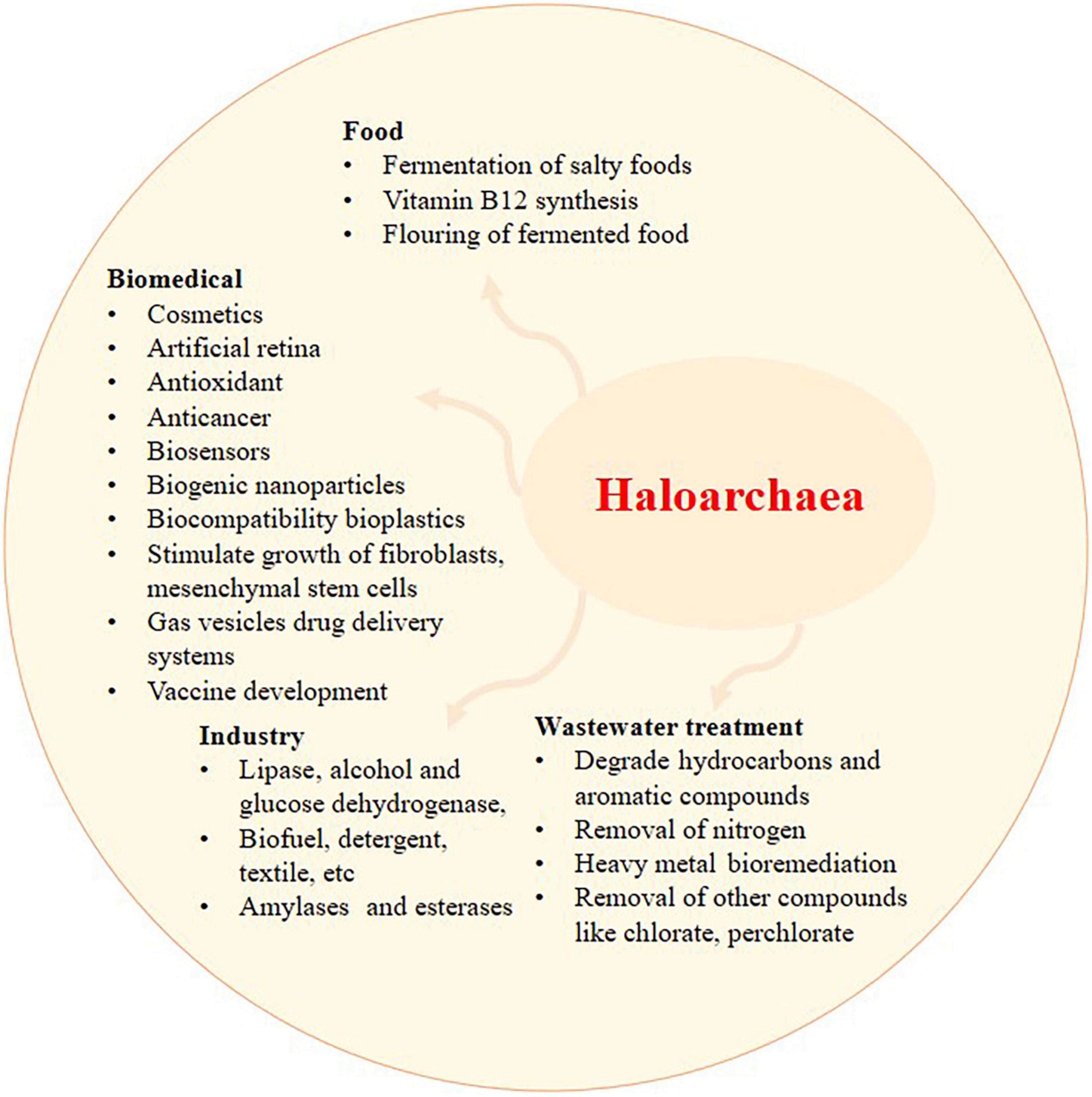
Figure 2. The application of haloarchaea and its metabolites. Recent exploration of haloarchaea and its metabolites has shed light on their potential applications in wastewater treatment, biomedical, food, and industrial sectors. Haloarchaea is a preferred source of haloenzymes since the growth and purification of enzymes involve minimal steps and are prone to less contamination.
Halophiles produce saline-tolerant proteins and metabolites, such as carotenoids, which assist in tolerance toward salinity. Haloarchaeal carotenoids have industrial interest due to their antioxidant, anticancer, antimicrobial, anti-inflammatory, food colorant, and several other biomedical applications (Verma et al., 2020). Bacterioruberin is also commonly used in cosmetics and drug encapsulation (Serrano et al., 2022). Haloarchaea have been extensively explored for bioactive metabolites with anticancer, antimicrobial, and antioxidant activities (Table 1). Among the several haloarchaeal secondary metabolites, carotenoids gained attention due to their multi-faced applications in cosmetics, food, and biomedical sectors (Serrano et al., 2022). Carotenoids have multiple roles in bacteria, plants, and archaea. Carotenoid helps photosynthetic plants and microbes to increase light absorption in the blue-green region through the singlet-singlet energy transfer (Hashimoto et al., 2015). It also has photoprotective effects against excessive light and reactive oxygen species through the triplet energy transfer of chlorophylls to carotenoids (Maoka, 2020). They are crucial in structural stabilization and render tolerance to hypersalinity.
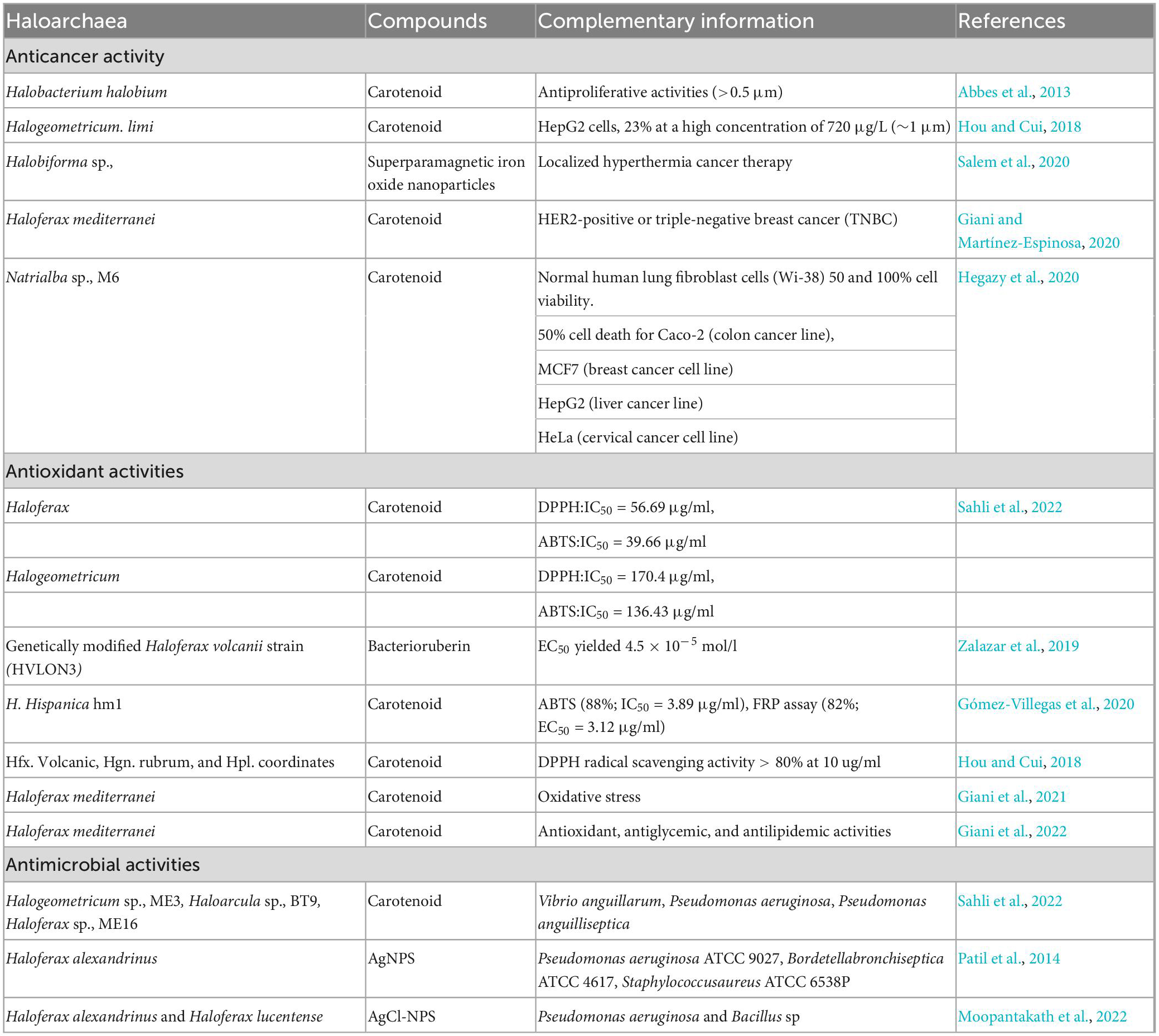
Table 1. Overview of the biological application of haloarchaea and their compounds: anticancer, antioxidant and antimicrobial activities of the metabolites derived from haloarchaea.
Due to the advancements in culture-based methods and metagenomics, the identification and discovery of novel species of haloarchaea become a continuous process. Recently, 12 haloarchaeal isolates were isolated from Tamil Nadu (India), out of which nine isolates were novel. Interestingly, all of the isolates produced carotenoids (Verma et al., 2020). Haloarchaea has an inherent molecule known as bacterioruberin, which is found in most haloarchaea (Nagar et al., 2022). Similarly, novel species under the genus Halorubrum, isolated from South Korea, produced C50 carotenoid (bacterioruberin), having a strong antioxidant activity (Hwang et al., 2022). Likewise, carotenoids from novel Haloarcula sp., and Halorubrum sp., strains were isolated from the Atacama Desert (Lizama et al., 2021). Large-scale whole genome (n = 68) analysis suggested that haloarchaea has a wide diversity of carotenoid biosynthetic genes (Serrano et al., 2022), suggesting that haloarchaeal species could be a reservoir of carotenoid derivatives.
2.1. Anticancer compounds
Haloarchaea and their metabolic products are getting more attention for the treatment of several cancers. Carotenoid pigment from Halobacterium halobium, isolated from a saltern in Tunisia, exhibited anticancer activity against the HepG2 cell line (Abbes et al., 2013). In addition, the carotenoid pigment of Halogeometricum limi, at a concentration of 720 μg/l, exhibited ∼23% anticancer activity against HepG2 cells (Hou and Cui, 2018). Similarly, high carotenoid-producing (0.98 g/l) haloarchaea i.e., Natrialba sp., M6, which thrives at 25% NaCl and a pH of 10.0, exhibited 50% anticancer activity against MCF-7, HepG2, and HeLa cells at low concentrations (<39 μg/ml) (Hegazy et al., 2020).
Compared to β-carotene, bacterioruberin has a superior antihemolytic and cytotoxic effect against HepG2 cells (Hou and Cui, 2018). Natrialba sp., M6, under the phylum Euryarchaeota, isolated from Egypt, produced C50 carotenoid as the predominant compound (Hegazy et al., 2020). The pigment had a higher selectivity toward cancer cells than the standard chemotherapeutic agent 5-fluorouracil. It is active against breast, liver, and colon cancer cells. On the other hand, C50 carotenoid bacterioruberin with dexamethasone reduced the release of TNF-α and IL-8, reversed the inflammation-induced morphological changes of macrophage, and had a potential role as an intestinal barrier repairing agent (Higa et al., 2020).
2.2. Antimicrobial compounds
Microorganisms causing infectious diseases evolve and acquire antimicrobial resistance continuously. Thus, alternative antimicrobials are required to meet emerging health challenges. Haloarchaeal carotenoids as antimicrobials have been explored less compared to other carotenoids. However, the potential of haloarchaeal carotenoids as antimicrobials was demonstrated against several pathogens (Gómez-Villegas et al., 2020). Recent reports have shown that carotenoids from Halogeometricum sp., ME3, Haloarcula sp., BT9 and Haloferax sp., ME16 have antimicrobial activity against Vibrio anguillarum, Pseudomonas aeruginosa, and Pseudomonas anguilliseptica, respectively (Sahli et al., 2022).
Haloarchaea produce halocins with potent antimicrobial activity. Halocin from the supernatant of Haloferax larsenii HA1 exhibits potent antimicrobial activity (Kumar and Tiwari, 2017). Previous works on halocines from haloarchaea suggested that the antimicrobial action of halocins could be related to processes of competition between different species for niche, food, or space. For instance, Halobacterium salinarum ETD5, isolated from the solar saltern of Sfax, Tunisia, exhibited antagonistic activity against haloarchaea of similar niches such as Halorubrum sp (strain ETD1, ETD2, ETD6, ETR7), Halorubrum chaoviator sp (strains ETD3, ETR14, and SS1R17), and Halobacterium salinarum ETD19 (Ghanmi et al., 2016). Furthermore, the C50 carotenoid pigment from Natrialba sp., M6 also exhibited promising potential in the elimination of hepatitis C virus (HCV) and hepatitis B virus (HBV) from human blood mononuclear cells suggesting its strong antiviral activity (Hegazy et al., 2020).
2.3. Antioxidant compounds
The human body produces free radicals during metabolic processes, which create oxidative stress and contribute to inflammation and lifestyle diseases. At present, haloarchaeal compounds have received significant attention due to their free radical scavenging properties at lower concentrations which are considerably more effective than the standard reference compounds like ascorbic acids. Bacterioruberin has a conjugated structure containing 13 C-C units and has a high free radical scavenging activity. The carotenoid from Haloferax sp., exhibited high antioxidative activity, confirmed with DPPH (2,2-diphenylpicrylhydrazyl) and ABTS (2,2’-azino-bis(3-ethylbenzothiazoline-6-sulfonic acid) assay. The IC50 values for the carotenoid compound were 56.69 and 39.66 μg/ml in DPPH and ABTS assay, respectively (Sahli et al., 2022). In contrast, carotenoid pigments isolated from the Halogeometricum sp., exhibited antioxidative activity with an IC50?170.4 μg/ml (DPPH assay) (Sahli et al., 2022). Similarly, acetone extracts from the H. hispanica HM1 showed 88% (ABTS) and 82% (Ferric ion reducing power) activity (Gómez-Villegas et al., 2020). Similarly, carotenoids from H. volcanii, Hgn. rubrum, and Hpl. Inordinatehave highlighted higher (80%) free radical scavenging properties at a concentration of 10 μg/ml (Hou and Cui, 2018). Zalazar et al. (2019) reported a genetically modified Haloferax volcanii strain (HVLON3) with high antioxidative activities (EC50 = 4.5 × 10–5 mol/l). Thus, haloarchaeal compounds can serve as a prominent source of antioxidant molecules in the future.
Bacterioruberin and β-carotene are the most desirable carotenoids for biological applications. Bacterioruberin, as an antioxidant molecule, can capture reactive oxygen species. Its exhibits antioxidant activity higher than the standard ascorbic acid (Sahli et al., 2022). Bacterioruberin has superior antioxidant properties compared to the β-carotene (Zalazar et al., 2019). Oxidative stress in the form of H2O2 increases the bacterioruberin production in Haloferax mediterranei strain R-4 up to 78% (Giani and Martínez-Espinosa, 2020). Furthermore, the antioxidant activity of haloarchaea varies between species. For instance, carotenoids from Haloferax sp., ME16 have a higher antioxidant activity than Halogeometricum and Haloarcula (Sahli et al., 2022).
3. Hydrolytic enzymes from haloarchaea
The wide range of enzymes produced by halophiles plays a vital role in biotechnology, including biosynthesis, food processing industries, and bioremediation methods. As the enzymes produced by haloarchaea are stable at high salt concentrations they can be utilized in several processes related to food or leather tanning. The most important hydrolytic enzymes produced by haloarchaea are proteases and lipases (Modern et al., 2000). The first hydrolase enzyme, a serine protease, was purified and studied from Halobacterium salinarum. This enzyme was active only at a high concentration of NaCl (more than 2 M), being an enzyme rich in negatively charged amino acids (Ventosa et al., 2005). Starch-degrading alpha-amylases are synthesized by halophiles such as Halobacterium salinarum, Haloferax mediterranei, Halomonas meridiana, and Natronococcus amylolyticus (Kumar et al., 2016). Besides amylases, pullulanase is produced by different archaea, such as Halorubrum sp., Ha25, which is already used in the starch industry (Siroosi et al., 2014). Cellulase-degrading cellulases and glycoside hydrolase gene homologs are present in Halorhabdus utahensis (Zhang et al., 2011), Haloarcula sp., (Ogan et al., 2012), Halorubrum lacusprofundi (Karan et al., 2013), Haloarcula vallismortis (Nercessian et al., 2015), Natronobiforma cellulositropha (Sorokin et al., 2018), Halalkalicoccus jeotgali (Anderson et al., 2011), and Haloferax sulfurifontis (Malik and Furtado, 2019). Table 2 displays different intracellular or extracellular hydrolytic enzymes produced by haloarchaea.
Amylase, one of the important industrial enzymes, was reported to synthesize by a new haloarchaeal strain isolated from salterns. High amylase activity was exhibited by the new strain Haloarcula sp., HS and found to be poly extremotolerant. The optimum enzyme yield was obtained at high salt concentrations (25%), 60°C, and was calcium-dependent. Amylases were found to be synthesized in extracellular and intracellular fractions and observed as 3 different types of enzymes. The extracted enzymes were tested on bakery waste. It was found that amylases degraded bakery waste efficiently at high salt concentrations (Gómez-Villegas et al., 2021). Interestingly, extracellular hydrolytic enzymes were produced by haloarchaeal strains obtained from hypersaline lakes. The two most abundant archaeal species, Natrinema and Halorubrum, produced cellulase, pectinase, amylase, lipase, and xylanase but not protease (Karray et al., 2018). In the study, alpha-amylase obtained from H. salinarum was immobilized in calcium alginate to enhance its stability (Patel et al., 1996). In another study, the tolerance of alcohol dehydrogenase enzyme, obtained from Haloferax volcanii, to organic solvents was reported to improve upon immobilization with sepabeads (Alsafadi and Paradisi, 2014). Immobilized lipase enzyme on anionic resin obtained from Haloarcula sp., was used to produce biodiesel (Li and Yu, 2014). Halobacterium salinarum NRC-1, Haloarcula japonica, H. salinarum CECT 395, and H. mediterranei can grow on chitin thanks to chitinases (García-Fraga et al., 2014; Hou et al., 2014). Haloarchaea degrades lignin through laccase and peroxidase enzymes. Some haloarchaea produces enzymes like esterases and lipases to degrade ester, ether, and glycosidic linkages. Menasria et al. (2018) have reported extensive bioprospecting of salt-stable and active hydrolytic enzymes from haloarchaea of arid and semi-arid wetlands. The major haloarchaea identified were from the class halobacteria such as Haloarcula, Halogeometricum, Halococcus, Haloterrigena, etc. Among the 68 isolates screened, 89.7% of isolates produced 2 halophilic enzymes, whereas 52.9% produced 3 hydrolytic enzymes. These isolates produced gelatinase, cellulase, esterase, and inulinase. Secondly, some isolates were profound in producing xylanase, pectinase, and nuclease. The study also reported that the high cellulase activity (35%) makes it a potential candidate in the food and textile industries (Menasria et al., 2018). Likewise, out of 300 isolates from a salt lake, 293 haloarchaea isolates were selected and studied for active hydrophilic hydrolytic enzymes.
The cellulase, xylanase, amylase, DNase, lipase, protease, pullulanase, chitinase, and inulinase were observed in 9 potential isolates. The most abundant enzymes produced by haloarchaeal isolates (Halorubrum and Haloarcula) were lipase, DNase, and amylase (Makhdoumi Kakhki et al., 2011).
4. Biodegradable and biocompatible polymers by haloarchaea
Search for eco-friendly biopolymers is one of the important research objectives worldwide to reduce global plastic pollution and in the production of biomedical devices (Simó-Cabrera et al., 2021). Regarding biomedicine, the desirable properties of biodegradable polymers vary based on their application, such as the high degradative potential for surgical mesh and low degradative potential for bioengineered skin. For such applications, biopolymers can be synthetically prepared, such as polylactic acid (PLA) and polyglycolic acid (PGA) (Han et al., 2015). Yet such synthetically prepared biopolymers are associated with setbacks such as biocompatibility and inflammation. Among natural biopolymers with interesting physicochemical properties to be used as bioplastic for packaging or biomedical applications, polyhydroxyalkanoates (PHAs) from haloarchaea are of research interest. The advantages of PHAs include their ease of mechanical customization, biodegradability, and biocompatibility (Han et al., 2015). Hence, PHAs have been under extensive research for application in medical implants, drug delivery, tissue replacement, etc. PHAs, composed of hydroxyalkanoate monomers, are stored as a source of carbon and energy under stress conditions in archaea and bacteria. The type of hydroxyalkanoate monomers determines the physical properties (rigid or elastic) of the PHAs. Among the PHAs, the most common ones are poly-3-hydroxybutyrate (PHB) and poly(3-hydroxybutyrate-co-3-hydroxyvalerate) (PHBHV). PHBHV comprises PHB and 3-hydroxyvalerate (3HV) monomer. PHBHV, upon degradation in the body, does not release toxic byproducts, has more outstanding biocompatibility and biodegradation, and helps in the growth of fibroblasts, mesenchymal stem cells, etc (Ahmed et al., 2010).
Archaea are considered promising cell factories and more cost-effective than bacteria for PHBHV production. For instance, Haloferax mediterranei produces PHBHV with a lower melting point than Hydrogenophaga pseudoflava (Koller et al., 2007). PHBHV produced by Halogranum amylolyticum has a higher hemocompatibility than Ralstonia eutropha (Zhao Y. et al., 2015). Furthermore, the entire process of biosynthesis is within the cell. PHAs are water-insoluble, degradable without oxygen, and increase their solubility in chlorinated solvents (Simó-Cabrera et al., 2021). Based on the monomers, PHAs are classified as homopolymers consisting of the same type of monomers (P4HB, P3HP, P3H4P, etc.), random copolymers consisting of multiple monomer types distributed random, and block copolymers where the distinct polymers are distributed in discrete blocks (Simó-Cabrera et al., 2021). Haloferax mediterranei can also produce varying types of PHBHV based on the concentration of valerate being fed (Han et al., 2015). These variations are of two main types, higher-order copolymers (O-PHBHV) composed of PHB and PHV with random PHBHV segments. R-PHBHV comprises random copolymers 3HB and 3HV (Han et al., 2015). PHAs are also produced by Halogranum amylolyticum TNN58 with poly (3-hydroxybutyrate-co-3-hydroxyvalerate) (PHBV) and 3-hydroxyvalerate (3HV) fraction when the carbon source is glucose (Zhao Y. X. et al., 2015). Genetic engineering, such as CRISPR-Cas technology, can enhance gene expression for PHAs production. A recent study showed a ∼165% increase in the output of PHA when citZ and gltA genes were downregulated by CRISPRi (Lin et al., 2021). Recently, robust methods have been developed for monitoring PHA granules in Haloferax mediterranei, such as through confocal fluorescence microscopy stained with Nile red and SYBR Green (Cánovas et al., 2021).
5. Synthesis and application of bioactive nanoparticles from haloarchaea
Nanobiotechnology is a boon to the field of medicine, which deals with the synthesis and application of a wide range of NPs for the treatment of diseases and targeted delivery of drugs (Patra et al., 2018; Dash et al., 2020). Synthesis of NPs using biological entities is always important due to their ease of production, eco-friendly approach, and bioavailability. The green approach in nanotechnology involves producing stable NPs capped with metabolites employed by organisms or plants (Singh et al., 2018). Several works have demonstrated that some haloarchaea can synthesize nanoparticles. However, the synthesis of NPs using haloarchaea needs to be better explored.
Recently, haloarchaea has been explored due to its ability to produce several NPs by detoxifying heavy metals. These organisms survive in the presence of heavy metals by employing enzymatic reduction of metals and sequestration methods to detoxify them (Voica et al., 2016). Table 3 shows different NPs synthesized using haloarchaea as well as their potential biological applications (Table 3). Additional factors such as salt, pH, temperature, and size affect the stability and efficiency of NPs (Dutta and Bandopadhyay, 2022).
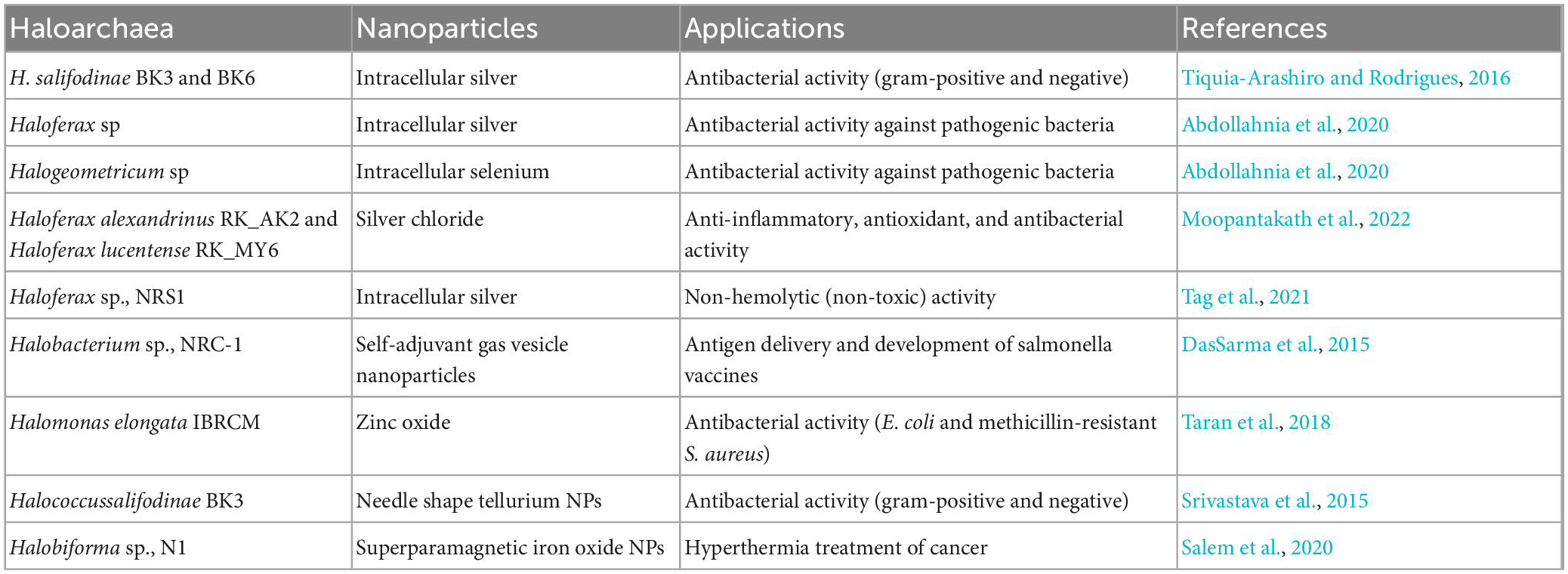
Table 3. Different types of nanoparticles synthesized using haloarchaea and their biological activities.
Abdollahnia et al. (2020) studied the synthesis of silver and selenium nanoparticles by haloarchaea isolated from solar salterns. Intracellular production of silver and selenium NPs were reported by Haloferax sp., and Halogeometricum sp., respectively (Abdollahnia et al., 2020; Nagar et al., 2022). The biosynthesized nanoparticles exhibited antibacterial activity against S. aureus, E. coli, B. subtilis, and P. aeruginosa (Abdollahnia et al., 2020). A haloarchaea, Haloferax sp., NRS1 screened from solar saltern found in Saudi Arabia showed promising potential in synthesizing silver NPs. The biogenic silver NPs showed non-hemolytic activity below 12.5 μg/ml concentration suggesting their thrombolysis property. The non-toxic/hemolytic property of synthesized NPs potentiates their application as nano drug carriers (Tag et al., 2021).
Similarly, silver NPs synthesized by another group exhibited broad antimicrobial activity. The archaea Halococcus salifodinae BK6, mediated synthesis of silver NPs, employs NADH-dependent nitro reductases to reduce metal to nanoparticles. They showed promising antibacterial activity against S. aureus and M. luteus (Gram-positive) and E. coli and P. aeruginosa (Gram-negative) (Srivastava et al., 2014b).
Srivastava et al. (2014a) discussed the synthesis of selenium NPs using H. salifodinae BK18. Similar to their previous work, nitro reductases reduced sodium selenite to NPs. The intracellular synthesized NPs exhibited antiproliferative properties against HeLa cancer cell lines. Also, the NPs were found to be non-toxic against normal cells suggesting their application as an anticancer agent (Srivastava et al., 2014a). Bioactive gold and silver NPs were synthesized using Haloferax volcanii. Significant antibacterial activities were observed with synthesized silver NPs against E. coli and P. putida (Costa et al., 2020). In addition, haloarchaeal nanoparticles hold promising antibacterial properties. Haloferax alexandrinus was used to synthesize silver nanoparticles with an average size of 18 nm and with an amide carbonyl group on the surface. This synthesized nanoparticle has shown antimicrobial activity against P. aeruginosa, Bordetella bronchiseptica, and S. aureus at 5 μg concentrations (Patil et al., 2014). Another study also highlights the synthesis of spherical-shaped silver chloride nanoparticles (MY6-NP and AK2-NPs) with a size of 30–50 nm using Haloferax Alexandrinus and Haloferax lucentense at high salt-saturated conditions. According to a study by Salem et al. (2020), hyperthermia therapy can be employed with the help of superparamagnetic iron oxide nanoparticles synthesized using Halobiforma sp., N1. The nanoparticles were monodispersed and presented growth inhibition activity against P. aeruginosa PAO1 and Bacillus sp., at 200 μg/ml concentration (Moopantakath et al., 2022).
6. Conclusion
Haloarchaea, or extremely halophilic archaea, are a group of microbes with genuine metabolic features. They inhabit and even predominate in various extreme geographical and ecological/environmental conditions, The number of new haloarchaeon discovered through culturable and non-culturable methods and techniques is increasing during the last decades. Metabolites synthesized by several haloarchaeal species are of high interest due to their potential applications in biotechnology Thus, carotenoids are highly efficient as antimicrobials, antioxidants, and food colorants. They have been reported to have diverse biological activities, including anticancer and antimicrobial activities. Among carotenoids, bacterioruberin, carotenoids almost produce by haloarchaea show higher antioxidant activity than most of the referenced carotenoids from plants, yeast or algae. Haloarchaea are also implemented in hypersaline wastewater treatment to degrade hydrocarbons, nitrogen removal, and heavy metal bioremediation and nanoparticle (NP) biosynthesis. On the other hand, enzymes from haloarchaea like amylase, chitinase, lipase, protease, and esterase show high activity at extreme conditions (in terms of pH, temperature etc.) which are of interest for industrial processes. The biosynthesis of nanoparticles by haloarchaea has also described being many of those nanoparticles effective against drug-resistant microbes. The haloarchaea, haloenzymes, and pigments are also widely used in the fermentation of salty foods, cosmetics, food, biomedical sectors, biocompatible bioplastics, and biosensors. Despite such an array of applications, their roles within the cells in natural environments as well as in industrial processes remain unexplored consequently, the research on the metabolites synthesized by these microorganisms must continue through more specialized approaches using high-tech equipments.
Author contributions
RK and SB designed the study. JM and VA prepared the figures. RK, SB, MI, JM, and VA wrote the manuscript. RK, SB, RM-E, and MD critically revised the manuscript. All authors have read and agreed to the submitted version of the manuscript.
Funding
This study was supported by the Science and Engineering Research Board (SERB) Government of India funds through the EEQ (EEQ/2018/001085) scheme. This study was also supported by Generalitat Valenciana, Spain (PROMETEO/2021/055), and VIGROB-309 (University of Alicante) to RM-E.
Acknowledgments
We thank the research facilities supported by the Pondicherry University and the Central University of Kerala. RK thanks SERB-EEQ (EEQ/2018/001085), Government of India. JM thanks ICMR-SRF, the Government of India. Financial support from the DBT-RA Programme in Biotechnology and Life Sciences is gratefully acknowledged by MI.
Conflict of interest
The authors declare that the research was conducted in the absence of any commercial or financial relationships that could be construed as a potential conflict of interest.
Publisher’s note
All claims expressed in this article are solely those of the authors and do not necessarily represent those of their affiliated organizations, or those of the publisher, the editors and the reviewers. Any product that may be evaluated in this article, or claim that may be made by its manufacturer, is not guaranteed or endorsed by the publisher.
References
Abaramak, G., Kırtel, O., and Öner, E. T. (2020). “Fructanogenic halophiles: a new perspective on extremophiles,” in Physiological and Biotechnological Aspects of Extremophiles, eds. R. Salwan and V. Sharma (London: Elsevier, Academic Press), 123–130. doi: 10.1016/B978-0-12-818322-9.00009-5
Abbes, M., Baati, H., Guermazi, S., Messina, C., Santulli, A., Gharsallah, N., et al. (2013). Biological properties of carotenoids extracted from Halobacterium halobium isolated from a Tunisian solar saltern. BMC Complement Altern. Med. 13:255. doi: 10.1186/1472-6882-13-255
Abdollahnia, M., Makhdoumi, A., Mashreghi, M., and Eshghi, H. (2020). Exploring the potentials of halophilic prokaryotes from a solar saltern for synthesizing nanoparticles: The case of silver and selenium. PLoS One 15:e0229886. doi: 10.1371/journal.pone.0229886
Ahmed, T., Marçal, H., Lawless, M., Wanandy, N. S., Chiu, A., and Foster, L. J. (2010). Polyhydroxybutyrate and its copolymer with polyhydroxy valerate as biomaterials: Influence on progression of stem cell cycle. Biomacromolecules 11, 2707–2715. doi: 10.1021/bm1007579
Akmoussi-Toumi, S., Khemili-Talbi, S., Ferioune, I., and Kebbouche-Gana, S. (2018). Purification and characterization of an organic solvent-tolerant and detergent-stable lipase from Haloferax mediterranei CNCMM 50101. Int. J. Biol. Macromol. 116, 817–830. doi: 10.1016/j.ijbiomac.2018.05.087
Alsafadi, D., and Paradisi, F. (2014). Covalent immobilization of alcohol dehydrogenase (ADH2) from Haloferax volcanii: How to maximize activity and optimize performance of halophilic enzymes. Mol. Biotechnol. 56, 240–247. doi: 10.1007/s12033-013-9701-5
Amin, K., Tranchimand, S., Benvegnu, T., Abdel-Razzak, Z., and Chamieh, H. (2021). Glycoside hydrolases and glycosyltransferases from hyperthermophilic archaea: Insights on their characteristics and applications in biotechnology. Biomolecules 11:1557. doi: 10.3390/biom11111557
Amoozegar, M. A., Siroosi, M., Atashgahi, S., Smidt, H., and Ventosa, A. (2017). Systematics of haloarchaea and biotechnological potential of their hydrolytic enzymes. Microbiology 163, 623–645. doi: 10.1099/mic.0.000463
Anderson, I., Scheuner, C., Göker, M., Mavromatis, K., Hooper, S. D., Porat, I., et al. (2011). Novel insights into the diversity of catabolic metabolism from ten haloarchaeal genomes. PLoS One 6:e20237. doi: 10.1371/journal.pone.0020237
Andrei, A. Ş, Banciu, H. L., and Oren, A. (2012). Living with salt: Metabolic and phylogenetic diversity of archaea inhabiting saline ecosystems. FEMS Microbiol. Lett. 330, 1–9. doi: 10.1111/j.1574-6968.2012.02526.x
Begemann, M. B., Mormile, M. R., Paul, V. G., and Vidt, D. J. (2011). “Potential enhancement of biofuel production through enzymatic biomass degradation activity and biodiesel production by halophilic microorganisms,” in Halophiles and hypersaline environments, eds A. Ventosa, A. Oren, and Y. Ma (Berlin: Springer), 341–357. doi: 10.1007/978-3-642-20198-1_18
Behzad, H., Ibarra, M. A., Mineta, K., and Gojobori, T. (2016). Metagenomic studies of the Red Sea. Gene 576, 717–723. doi: 10.1016/j.gene.2015.10.034
Bernabeu, E., Miralles-Robledillo, J., Giani, M., Valdés, E., Martínez-Espinosa, R., and Pire, C. (2021). In silico analysis of the enzymes involved in haloarchaeal denitrification. Biomolecules 11:1043. doi: 10.3390/biom11071043
Besse, A., Vandervennet, M., Goulard, C., Peduzzi, J., Isaac, S., Rebuffat, S., et al. (2017). Halocin C8: An antimicrobial peptide distributed among four halophilic archaeal genera: Natrinema, Haloterrigena, Haloferax, and Halobacterium. Extremophiles 21, 623–638. doi: 10.1007/s00792-017-0931-5
Bhattacharyya, A., Majumder, N. S., Basak, P., Mukherji, S., Roy, D., Nag, S., et al. (2015). Diversity and distribution of Archaea in the mangrove sediment of Sundarbans. Archaea 2015:968582. doi: 10.1155/2015/968582
Bolhuis, H., Palm, P., Wende, A., Falb, M., Rampp, M., Rodriguez-Valera, F., et al. (2006). The genome of the square archaeon Haloquadratum walsbyi: Life at the limits of water activity. BMC Genomics 7:169. doi: 10.1186/1471-2164-7-169
Britton, K. L., Baker, P. J., Fisher, M., Ruzheinikov, S., Gilmour, D. J., Bonete, M. J., et al. (2006). Analysis of protein solvent interactions in glucose dehydrogenase from the extreme halophile Haloferax mediterranei. Proc. Natl. Acad. Sci. U.S.A. 103, 4846–4851. doi: 10.1073/pnas.0508854103
Camacho, R. M., Mateos, J. C., González-Reynoso, O., Prado, L. A., and Córdova, J. (2009). Production and characterization of esterase and lipase from Haloarcula marismortui. J. Ind. Microbiol. Biotechnol. 36, 901–909. doi: 10.1007/s10295-009-0568-1
Cánovas, V., Garcia-Chumillas, S., Monzó, F., Simó-Cabrera, L., Fernández-Ayuso, C., Pire, C., et al. (2021). Analysis of polyhydroxyalkanoates granules in Haloferax mediterranei by double-fluorescence staining with Nile Red and SYBR Green by confocal fluorescence microscopy. Polymers 13:1582. doi: 10.3390/polym13101582
Cecil, L. M., DasSarma, S., Pecher, W., McDonald, R., AbdulSalam, M., and Hasan, F. (2020). Metagenomic insights into the diversity of halophilic microorganisms indigenous to the Karak Salt Mine. Pak. Front. Microbiol. 11:1567. doi: 10.3389/fmicb.2020.01567
Cho, E. S., Cha, I. T., Roh, S. W., and Seo, M. J. (2021). Haloferax litoreum sp. nov., Haloferax marinisediminis sp. nov., and Haloferax marinum sp. nov., low salt-tolerant haloarchaea isolated from seawater and sediment. Antonie van Leeuwenhoek 114, 2065–2082. doi: 10.1007/s10482-021-01661-0
Coker, J. A., DasSarma, P., Kumar, J., Müller, J. A., and DasSarma, S. (2007). Transcriptional profiling of the model Archaeon Halobacterium sp. NRC-1: Responses to changes in salinity and temperature. Saline Syst. 3:6. doi: 10.1186/1746-1448-3-6
Costa, M. I., Álvarez-Cerimedo, M. S., Urquiza, D., Ayude, M. A., Hoppe, C. E., Fasce, D. P., et al. (2020). Synthesis, characterization and kinetic study of silver and gold nanoparticles produced by the archaeon Haloferax volcanii. J. Appl. Microbiol. 129, 1297–1308. doi: 10.1111/jam.14726
Cui, H.-L., and Dyall-Smith, M. L. (2021). Cultivation of halophilic Archaea (class Halobacteria) from thalassohaline and athalassohaline environments. Mar. Life Sci. Technol. 3, 243–251. doi: 10.1007/s42995-020-00087-3
Das, D., Kalra, I., Mani, K., Salgaonkar, B. B., and Braganca, J. M. (2019). Characterization of extremely halophilic archaeal isolates from Indian salt pans and their screening for production of hydrolytic enzymes. Environ. Sustain. 2, 227–239. doi: 10.1007/s42398-019-00077-x
Das, S., and Dash, H. R. (2018). Microbial diversity in the genomic era. Cambridge, MA: Academic Press, doi: 10.1016/c2017-0-01759-7
Dash, D. K., Panik, R. K., Sahu, A. K., and Tripathi, V. (2020). “Role of nanobiotechnology in drug discovery, development and molecular diagnostic,” in Applications of nanobiotechnology, eds M. Stoytcheva and R. Zlatev (London: IntechOpen), 225–240. doi: 10.5772/intechopen.92796
DasSarma, P., Negi, V. D., Balakrishnan, A., Kim, J.-M., Karan, R., Chakravortty, D., et al. (2015). Haloarchaeal gas vesicle nanoparticles displaying Salmonella antigens as a novel approach to vaccine development. Procedia Vaccinol. 9, 16–23. doi: 10.1016/j.provac.2015.05.003
De Castro, R. E., Maupin-Furlow, J. A., Giménez, M. I., Herrera Seitz, M. K., and Sánchez, J. J. (2006). Haloarchaeal proteases and proteolytic systems. FEMS Microbiol. Rev. 30, 17–35. doi: 10.1111/j.1574-6976.2005.00003.x
Del Campo, M. M., Camacho, R. M., Mateos-Díaz, J. C., Müller-Santos, M., Córdova, J., and Rodríguez, J. A. (2015). Solid-state fermentation as a potential technique for esterase/lipase production by halophilic archaea. Extremophiles 19, 1121–1132.
Durán-Viseras, A., Andrei, A. S., Ghai, R., Sánchez-Porro, C., and Ventosa, A. (2019). New Halonotius species provide genomics-based insights into cobalamin synthesis in haloarchaea. Front. Microbiol. 27:1928. doi: 10.3389/fmicb.2019.01928
Dutta, B., and Bandopadhyay, R. (2022). Biotechnological potentials of halophilic microorganisms and their impact on mankind. Beni Suef Univ. J. Basic Appl. Sci. 11:75. doi: 10.1186/s43088-022-00252-w
Dyall-Smith, M. L., Pfeiffer, F., Klee, K., Palm, P., Gross, K., Schuster, S. C., et al. (2011). Haloquadratum walsbyi: Limited diversity in a global pond. PLoS One 6:e20968. doi: 10.1371/journal.pone.0020968
Esclapez, J., Pire, C., Bautista, V., Martínez-Espinosa, R. M., Ferrer, J., and Bonete, M. J. (2007). Analysis of acidic surface of Haloferax mediterranei glucose dehydrogenase by site-directed mutagenesis. FEBS Lett. 581, 837–842. doi: 10.1016/j.febslet.2007.01.054
Fenderson, B. A. (2006). Molecular chaperones and cell signalling. Shock 25:426. doi: 10.1097/01.shk.0000215322.52607.c3
Fernández, A. B., Vera-Gargallo, B., Sánchez-Porro, C., Ghai, R., Papke, R. T., Rodriguez-Valera, F., et al. (2014). Comparison of prokaryotic community structure from Mediterranean and Atlantic saltern concentrator ponds by a metagenomic approach. Front. Microbiol. 5:196. doi: 10.3389/fmicb.2014.00196
Fukushima, T., Mizuki, T., Echigo, A., Inoue, A., and Usami, R. (2005). Organic solvent tolerance of halophilic α-amylase from a Haloarchaeon, Haloarcula sp. strain S-1. Extremophiles 9, 85–89. doi: 10.1007/s00792-004-0423-2
Gaonkar, S. K., and Furtado, I. J. (2022). Biorefinery-fermentation of agro-wastes by Haloferax lucentensis GUBF-2 MG076878 to haloextremozymes for use as biofertilizer and biosynthesizer of AgNPs. Waste Biomass Valorization 13, 1117–1133. doi: 10.1007/S12649-021-01556-1/FIGURES/6
García-Fraga, B., Da Silva, A. F., López-Seijas, J., and Sieiro, C. (2014). Functional expression and characterization of a chitinase from the marine archaeon Halobacterium salinarum CECT 395 in Escherichia coli. Appl. Microbiol. Biotechnol. 98, 2133–2143. doi: 10.1007/s00253-013-5124-2
Ghanmi, F., Carré-Mlouka, A., Vandervennet, M., Boujelben, I., Frikha, D., Ayadi, H., et al. (2016). Antagonistic interactions and production of halocin antimicrobial peptides among extremely halophilic prokaryotes isolated from the solar saltern of Sfax, Tunisia. Extremophiles 20, 363–374. doi: 10.1007/s00792-016-0827-9
Giani, M., Gervasi, L., Loizzo, M., and Martínez-Espinosa, R. (2022). Carbon source influences antioxidant, antiglycemic, and antilipidemic activities of Haloferax mediterranei carotenoid extracts. Mar. Drugs 20:659. doi: 10.3390/md20110659
Giani, M., and Martínez-Espinosa, R. (2020). Carotenoids as a protection mechanism against oxidative stress in Haloferax mediterranei. Antioxidants 9:1060. doi: 10.3390/antiox9111060
Giani, M., Montoyo-Pujol, Y., Peiró, G., and Martínez-Espinosa, R. (2021). Halophilic carotenoids and breast cancer: From salt marshes to biomedicine. Mar. Drugs 19:594. doi: 10.3390/md19110594
Gómez-Villegas, P., Vigara, J., Romero, L., Gotor, C., Raposo, S., Gonçalves, B., et al. (2021). Biochemical characterization of the amylase activity from the new haloarchaeal strain Haloarcula sp. HS isolated in the Odiel Marshlands. Biology 10:337. doi: 10.3390/biology10040337
Gómez-Villegas, P., Vigara, J., Vila, M., Varela, J., Barreira, L., and Léon, R. (2020). Antioxidant, antimicrobial, and bioactive potential of two new haloarchaeal strains isolated from odiel salterns (Southwest Spain). Biology 9:298. doi: 10.3390/biology9090298
Grant, W. D., Danson, M. J., Scott, D. J., Halling, P. J., Engberts, J. B. F. N., Ho, M. W., et al. (2004). Life at low water activity. Philos. Trans. R. Soc. Lond. B Biol. Sci. 359, 1249–1267. doi: 10.1098/rstb.2004.1502
Griffiths, B. S., and Philippot, L. (2013). Insights into the resistance and resilience of the soil microbial community. FEMS Microbiol. Rev. 37, 112–129. doi: 10.1111/j.1574-6976.2012.00343.x
Haldar, S., and Nazareth, S. W. (2018). Taxonomic diversity of bacteria from mangrove sediments of Goa: Metagenomic and functional analysis. 3 Biotech 8:436. doi: 10.1007/s13205-018-1441-6
Han, J., Wu, L. P., Hou, J., Zhao, D., and Xiang, H. (2015). Biosynthesis, characterization, and hemostasis potential of tailor-made poly (3-hydroxybutyrate-co-3-hydroxyvalerate) produced by Haloferax mediterranei. Biomacromolecules 16, 578–588. doi: 10.1021/bm5016267
Hashimoto, H., Sugai, Y., Uragami, C., Gardiner, A. T., and Cogdell, R. J. (2015). Natural and artificial light-harvesting systems utilizing the functions of carotenoids. J. Photochem. Photobiol. C. 25, 46–70. doi: 10.1016/j.jphotochemrev.2015.07.004
Hegazy, G. E., Abu-Serie, M. M., Abo-Elela, G. M., Ghozlan, H., Sabry, S. A., Soliman, N. A., et al. (2020). In vitro dual (anticancer and antiviral) activity of the carotenoids produced by haloalkaliphilic archaeon Natrialba sp. M6. Sci. Rep. 10:5986. doi: 10.1038/s41598-020-62663-y
Higa, L. H., Schilrreff, P., Briski, A. M., Jerez, H. E., de Farias, M. A., Portugal, R. V., et al. (2020). Bacterioruberin from Haloarchaea plus dexamethasone in ultra-small macrophage-targeted nanoparticles as potential intestinal repairing agent. Colloids Surf. B. 191:110961. doi: 10.1016/j.colsurfb.2020.110961
Hou, J., and Cui, H. L. (2018). In vitro antioxidant, antihemolytic, and anticancer activity of the carotenoids from halophilic archaea. Curr. Microbiol. 75, 266–271. doi: 10.1007/s00284-017-1374-z
Hou, J., Han, J., Cai, L., Zhou, J., Lü, Y., Jin, C., et al. (2014). Characterization of genes for chitin catabolism in Haloferax mediterranei. Appl. Microbiol. Biotechnol. 8, 1185–1194. doi: 10.1007/s00253-013-4969-8
Hutcheon, G. W., Vasisht, N., and Bolhuis, A. (2005). Characterisation characterization of a highly stable α-amylase from the halophilic archaeon Haloarcula hispanica. Extremophiles 9, 487–495. doi: 10.1007/s00792-005-0471-2
Hwang, C. Y., Cho, E. S., Rhee, W. J., Kim, E., and Seo, M. J. (2022). Genomic and physiological analysis of C50 carotenoid-producing novel Halorubrumruber sp. nov. J. Microbiol. 26, 1007–1020. doi: 10.1007/s12275-022-2173-1
Jones, D. L., and Baxter, B. K. (2017). DNA repair and photoprotection: Mechanisms of overcoming environmental ultraviolet radiation exposure in halophilic archaea. Front. Microbiol. 8:1882. doi: 10.3389/fmicb.2017.01882
Karan, R., Capes, M. D., DasSarma, P., and DasSarma, S. (2013). Cloning, overexpression, purification, and characterization of a polyextremophilic β-galactosidase from the Antarctic haloarchaeon Halorubrum lacusprofundi. BMC Biotechnol. 13:3. doi: 10.1186/1472-6750-13-3
Karray, F., Ben Abdallah, M., Kallel, N., Hamza, M., Fakhfakh, M., and Sayadi, S. (2018). Extracellular hydrolytic enzymes produced by halophilic bacteria and archaea isolated from hypersaline lake. Mol. Biol. Rep. 45, 1297–1309. doi: 10.1007/s11033-018-4286-5
Kennedy, S. P., Ng, W. V., Salzberg, S. L., Hood, L., and DasSarma, S. (2001). Understanding the adaptation of Halobacterium species NRC-1 to its extreme environment through computational analysis of its genome sequence. Genome Res. 11, 1641–1650. doi: 10.1101/gr.190201
Keshri, J., Yousuf, B., Mishra, A., and Jha, B. (2015). The abundance of functional genes, cbbL, nifH, amoA and apsA, and bacterial community structure of intertidal soil from Arabian Sea. Microbiol. Res. 175, 57–66. doi: 10.1016/j.micres.2015.02.007
Koller, M., Hesse, P., Bona, R., Kutschera, C., Atliæ, A., and Braunegg, G. (2007). Potential of various archaea and eubacterial strains as industrial polyhydroxyalkanoate producers from whey. Macromol. Biosci. 7, 218–226. doi: 10.1002/mabi.200600211
Kottemann, M., Kish, A., Iloanusi, C., Bjork, S., and DiRuggiero, J. (2005). Physiological responses of the halophilic archaeon Halobacterium sp. strain NRC1 to desiccation and gamma irradiation. Extremophiles 9, 219–227. doi: 10.1007/s00792-005-0437-4
Kumar, S., Grewal, J., Sadaf, A., Hemamalini, R., and Khare, S. K. (2016). Halophiles as a source of polyextremophilic α-amylase for industrial applications. AIMS Microbiol. 2, 1–26. doi: 10.3934/Microbiol.2016.1.1
Kumar, V., and Tiwari, S. K. (2017). Halocin HA1: An archaeocin produced by the haloarchaeon Haloferax larsenii HA1. Process Biochem. 61, 202–208. doi: 10.1016/j.procbio.2017.06.010
Li, J., Gao, Y., Dong, H., and Sheng, G. P. (2021). Haloarchaea, excellent candidates for removing pollutants from hypersaline wastewater. Trends Biotechnol. 40, 226–239. doi: 10.1016/j.tisbtech.2021.06.006
Li, X., and Yu, H. Y. (2013). Halostable cellulase with organic solvent tolerance from Haloarcula sp. LLSG7 and its application in bioethanol fermentation using agricultural wastes. J. Ind. Microbiol. Biotechnol. 40, 1357–1365. doi: 10.1007/s10295-013-1340-0
Li, X., and Yu, H. Y. (2014). Characterization of an organic solvent-tolerant lipase from Haloarcula sp. G41 and its application for biodiesel production. Folia Microbiol. 59, 455–463. doi: 10.1007/s12223-014-0320-8
Lin, L., Chen, J., Mitra, R., Gao, Q., Cheng, F., Xu, T., et al. (2021). Optimising optimizing PHBV biopolymer production in haloarchaea via CRISPRi-mediated redirection of carbon flux. Commun. Biol. 4:1007. doi: 10.1038/s42003-021-02541-z
Lizama, C., Romero-Parra, J., Andrade, D., Riveros, F., Bórquez, J., Ahmed, S., et al. (2021). Analysis of carotenoids in haloarchaea species from atacama saline lakes by high resolution UHPLC-Q-orbitrap-mass spectrometry: Antioxidant potential and biological effect on cell viability. Antioxidants 10:1230. doi: 10.3390/antiox10081230
Llorca, M., and Martínez-Espinosa, R. (2022). Assessment of Haloferax mediterranei genome in search of copper-molecular machinery with potential applications for bioremediation. Front. Microbiol. 13:895296. doi: 10.3389/fmicb.2022.895296
Lu, Y., Yuan, J., Lu, X., Su, C., Zhang, Y., Wang, C., et al. (2018). Major threats of pollution and climate change to global coastal ecosystems and enhanced management for sustainability. Environ. Pollut. 239, 670–680. doi: 10.1016/j.envpol.2018.04.016
Macario, A. J. L., Lange, M., Ahring, B. K., and De Macario, E. C. (1999). Stress genes and proteins in the Archaea. Microbiol. Mol. Biol. Rev. 63, 923–967. doi: 10.1128/MMBR.63.4.923-967.1999
Makhdoumi Kakhki, A., Amoozegar, M. A., and Mahmodi Khaledi, E. (2011). Diversity of hydrolytic enzymes in haloarchaeal strains isolated from salt lake. Int. J. Environ. Sci. Technol. 8, 705–714. doi: 10.1007/bf03326255
Malik, A. D., and Furtado, I. J. (2019). Haloferax sulfurifontis GUMFAZ2 producing xylanase-free cellulase retrieved from Haliclona sp. inhabiting rocky shore of Anjuna, Goa-India. J. Basic Microbiol. 59, 692–700. doi: 10.1002/jobm.201800672
Mani, K., Taib, N., Hugoni, M., Bronner, G., Bragança, J. M., and Debroas, D. (2020). Transient dynamics of archaea and bacteria in sediments and brine across a salinity gradient in a solar saltern of Goa, India. Front. Microbiol. 11:1891. doi: 10.3389/fmicb.2020.01891
Maoka, T. (2020). Carotenoids as natural functional pigments. J. Nat. Med. 74, 1–6. doi: 10.1007/s11418-019-01364-x
Martin del Campo, M., Camacho, R. M., Mateos-Díaz, J. C., Müller-Santos, M., Córdova, J., and Rodríguez, J. A. (2015). Solid-state fermentation as a potential technique for esterase/lipase production by halophilic archaea. Extremophiles 19, 1121–1132. doi: 10.1007/s00792-015-0784-8
Martínez, G., Pire, C., and Martínez-Espinosa, R. (2022). Hypersaline environments as natural sources of microbes with potential applications in biotechnology: The case of solar evaporation systems to produce salt in Alicante County (Spain). Curr. Res. Microb. Sci. 3:100136. doi: 10.1016/j.crmicr.2022.100136
Martínez-Espinosa, R., Richardson, D., and Bonete, M. (2015). Characterisation Characterization of chlorate reduction in the haloarchaeon Haloferax mediterranei. Biochim. Biophys. Acta 1850, 587–594. doi: 10.1016/j.bbagen.2014.12.011
Martínez-Espinosa, R. M., Lledó, B., Marhuenda-Egea, F. C., and Bonete, M. J. (2007). The effect of ammonium on assimilatory nitrate reduction in the haloarchaeon Haloferax mediterranei. Extremophiles 11, 759–767. doi: 10.1007/s00792-007-0095-9
Matarredona, L., Camacho, M., Zafrilla, B., Bonete, M. J., and Esclapez, J. (2020). The role of stress proteins in haloarchaea and their adaptive response to environmental shifts. Biomolecules 10:1390. doi: 10.3390/biom10101390
Menasria, T., Aguilera, M., Hocine, H., Benammar, L., Ayachi, A., Si Bachir, A., et al. (2018). Diversity and bioprospecting of extremely halophilic archaea isolated from Algerian arid and semi-arid wetland ecosystems for halophilic-active hydrolytic enzymes. Microbiol. Res. 207, 289–298. doi: 10.1016/j.micres.2017.12.011
Miralles-Robledillo, J., Bernabeu, E., Giani, M., Martínez-Serna, E., Martínez-Espinosa, R., and Pire, C. (2021). Distribution of denitrification among haloarchaea: A comprehensive study. Microorganisms 9:1669. doi: 10.3390/microorganisms9081669
Modern, D., Ebel, C., and Zaccai, G. (2000). Halophilic adaptation of enzymes. Extremophiles 4, 91–98. doi: 10.1007/s007920050142
Moopantakath, J., Imchen, M., Kumavath, R., and Martínez-Espinosa, R. M. (2021). Ubiquitousness of Haloferax and carotenoid producing genes in Arabian sea coastal biosystems of India. Mar. Drugs 19:442. doi: 10.3390/md19080442
Moopantakath, J., Imchen, M., Siddhardha, B., and Kumavath, R. (2020). 16s rRNA metagenomic analysis reveals predominance of Crtl and CruF genes in Arabian Sea coast of India. Sci. Total Environ. 743:140699. doi: 10.1016/j.scitotenv.2020.140699
Moopantakath, J., Imchen, M., Sreevalsan, A., Siddhardha, B., Martínez-Espinosa, R. M., and Kumavath, R. (2022). Biosynthesis of silver chloride nanoparticles (AgCl-NPs) from extreme halophiles and evaluation of their biological applications. Curr. Microbiol. 79:266. doi: 10.1007/s00284-022-02970-x
Moran-Reyna, A., and Coker, J. A. (2014). The effects of extremes of pH on the growth and transcriptomic profiles of three haloarchaea. F1000Res. 3:168. doi: 10.12688/f1000research.4789.1
Mormile, M. R., Hong, B. Y., and Benison, K. C. (2009). Molecular analysis of the microbial communities of Mars analog lakes in Western Australia. Astrobiology 9, 919–930. doi: 10.1089/ast.2008.0293
Mukherji, S., Ghosh, A., Bhattacharyya, C., Mallick, I., Bhattacharyya, A., Mitra, S., et al. (2020). Molecular and culture-based surveys of metabolically active hydrocarbon-degrading archaeal communities in Sundarban mangrove sediments. Ecotoxicol. Environ. Saf. 195:110481. doi: 10.1016/j.ecoenv.2020.110481
Nagar, D. N., Ghosh, N. N., and Braganca, J. M. (2022). Green synthesis of selenium nanospheres and nanoneedles by halophilic archaea. Appl. Nanosci. 12, 3983–3994. doi: 10.1007/S13204-022-02665-6
Nercessian, D., Di Meglio, L., De Castro, R., and Paggi, R. (2015). Exploring the multiple biotechnological potential of halophilic microorganisms isolated from two Argentinean salterns. Extremophiles 19, 1133–1143. doi: 10.1007/s00792-015-0785-7
Ng, W. V., Kennedy, S. P., Mahairas, G. G., Berquist, B., Pan, M., Shukla, H. D., et al. (2000). Genome sequence of Halobacterium species NRC-1. Proc. Natl. Acad. Sci.U.S. A. 97, 12176–12181. doi: 10.1073/pnas.190337797
Ogan, A., Danis, O., Gozuacik, A., Cakmar, E., and Birbir, M. (2012). Production of cellulase by immobilized whole cells of Haloarcula. Appl. Biochem. Microbiol. 48, 440–443. doi: 10.1134/S0003683812050092
Oh, D., Porter, K., Russ, B., Burns, D., and Dyall-Smith, M. (2010). Diversity of Haloquadratum and other haloarchaea in three, geographically distant, Australian saltern crystallizer ponds. Extremophiles 14, 161–169. doi: 10.1007/s00792-009-0295-6
Oren, A. (2002b). Molecular ecology of extremely halophilic Archaea and Bacteria. FEMS Microbiol. Ecol. 39, 1–7. doi: 10.1016/S0168-6496(01)00200-8
Oren, A. (2002a). Halophilic microorganisms and their environments. Dordrecht: Springer, doi: 10.1007/0-306-48053-0
Oren, A. (2013). “Life at high salt concentrations,” in The prokaryotes: Prokaryotic communities and ecophysiology, eds E. Rosenberg, E. F. DeLong, S. Lory, E. Stackebrandt, and F. Thompson (Berlin: Springer), 421–440. doi: 10.1007/978-3-642-30123-0_57
Osman, J. R., Regeard, C., Badel, C., Fernandes, G., and DuBow, M. S. (2019). Variation of bacterial biodiversity from saline soils and estuary sediments present near the Mediterranean Sea coast of Camargue (France). Antonie Van Leeuwenhoek 112, 351–365. doi: 10.1007/s10482-018-1164-z
Patel, S., Bagai, R., and Madamwar, D. (1996). Stabilization of a Halophilic α-Amylase by Calcium Alginate Immobilization. Biocatal. Biotransformation 14, 147–155. doi: 10.3109/10242429609106882
Patil, S., Fernandes, J., Tangasali, R., and Furtado, I. (2014). Exploitation of Haloferax Alexandrinus for biogenic synthesis of silver nanoparticles antagonistic to human and lower mammalian pathogens. J. Clust. Sci. 25, 423–433. doi: 10.1007/s10876-013-0621-0
Patra, J. K., Das, G., Fraceto, L. F., Campos, E. V. R., Rodriguez-Torres, M., del, P., et al. (2018). Nano based drug delivery systems: Recent developments and future prospects. J. Nanobiotechnol. 16:71. doi: 10.1186/s12951-018-0392-8
Pérez-Pomares, F., Bautista, V., Ferrer, J., Pire, C., Marhuenda-Egea, F. C., and Bonete, M. J. (2003). α-Amylase activity from the halophilic archaeon Haloferax mediterranei. Extremophiles 7, 299–306. doi: 10.1007/s00792-003-0327-6
Popescu, G., and Dumitru, L. (2009). Biosorption of some heavy metals from media with high salt concentrations by halophilic Archaea. Biotechnol. Biotechnol. Equip. 23, 791–795. doi: 10.1080/13102818.2009.10818542
Quillaguamán, J., Guzmán, H., Van-Thuoc, D., and Hatti-Kaul, R. (2010). Synthesis and production of polyhydroxyalkanoates by halophiles: Current potential and future prospects. Appl. Microbiol. Biotechnol. 85, 1687–1696. doi: 10.1007/s00253-009-2397-6
Rhodes, M. E., Oren, A., and House, C. H. (2012). Dynamics and persistence of Dead Sea microbial populations as shown by high-throughput sequencing of rRNA. Appl. Environ. Microbiol. 78, 2489–2492. doi: 10.1128/AEM.06393-11
Sahli, K., Gomri, M. A., Esclapez, J., Gómez-Villegas, P., Bonete, M. J., León, R., et al. (2022). Characterization and biological activities of carotenoids produced by three haloarchaeal strains isolated from Algerian salt lakes. Arch. Microbiol. 204:6. doi: 10.1007/s00203-021-02611-0
Salem, N. F., Abouelkheir, S. S., Yousif, A. M., Meneses-Brassea, B. P., Sabry, S. A., Ghozlan, H. A., et al. (2020). Large scale production of superparamagnetic iron oxide nanoparticles by the haloarchaeon Halobiforma sp. N1 and their potential in localized hyperthermia cancer therapy. Nanotechnology 32:09LT01. doi: 10.1088/1361-6528/abc851
Salgaonkar, B. B., Das, D., and Bragança, J. M. (2016). Resistance of extremely halophilic archaea to zinc and zinc oxide nanoparticles. Appl. Nanosci. 6, 251–258. doi: 10.1007/s13204-015-0424-8
Salgaonkar, B. B., Mani, K., and Bragança, J. M. (2013). Accumulation of polyhydroxyalkanoates by halophilic archaea isolated from traditional solar salterns of India. Extremophiles 17, 787–795. doi: 10.1007/S00792-013-0561-5
Santorelli, M., Maurelli, L., Pocsfalvi, G., Fiume, I., Squillaci, G., La Cara, F., et al. (2016). Isolation and characterisation characterization of a novel alpha-amylase from the extreme haloarchaeon Haloterrigena turkmenica. Int. J. Biol. Macromol. 92, 174–184. doi: 10.1016/j.ijbiomac.2016.07.001
Schäfer, G., Purschke, W., and Schmidt, C. L. (1996). On the origin of respiration: Electron transport proteins from archaea to man. FEMS Microbiol. Rev. 5, 223–234. doi: 10.1016/0168-6445(96)00010-1
Serrano, S., Mendo, S., and Caetano, T. (2022). Haloarchaea have a high genomic diversity for the biosynthesis of carotenoids of biotechnological interest. Res. Microbiol. 173:103919. doi: 10.1016/j.resmic.2021.103919
Shukla, H. D. (2006). Proteomic analysis of acidic chaperones, and stress proteins in extreme halophile Halobacterium NRC-I: A comparative proteomic approach to study heat shock response. Proteome Sci. 4:6. doi: 10.1186/1477-5956-4-6
Simó-Cabrera, L., García-Chumillas, S., Hagagy, N., Saddiq, A., Tag, H., Selim, S., et al. (2021). Haloarchaea as cell factories to produce bioplastics. Mar. Drugs 19:159. doi: 10.3390/md19030159
Singh, A., and Singh, A. K. (2017). Haloarchaea: Worth exploring for their biotechnological potential. Biotechnol. Lett. 39, 1793–1800. doi: 10.1007/s10529-017-2434-y
Singh, J., Dutta, T., Kim, K. H., Rawat, M., Samddar, P., and Kumar, P. (2018). ‘Green’ synthesis of metals and their oxide nanoparticles: Applications for environmental remediation. J. Nanobiotechnol. 16:84. doi: 10.1186/s12951-018-0408-4
Siroosi, M., Amoozegar, M. A., Khajeh, K., Fazeli, M., and Habibi Rezaei, M. (2014). Purification and characterization of a novel extracellular halophilic and organic solvent-tolerant amylopullulanase from the haloarchaeon, Halorubrum sp. strain Ha25. Extremophiles 18, 25–33. doi: 10.1007/s00792-013-0589-6
Somboonna, N., Assawamakin, A., Wilantho, A., Tangphatsornruang, S., and Tongsima, S. (2012). Metagenomic profiles of free-living archaea, bacteria and small eukaryotes in coastal areas of Sichang island, Thailand. BMC Genomics 13(Suppl. 7):S29. doi: 10.1186/1471-2164-13-S7-S29
Sorokin, D. Y., Khijniak, T. V., Kostrikina, N. A., Elcheninov, A. G., Toshchakov, S. V., Bale, N. J., et al. (2018). Natronobiforma cellulositropha gen. nov., sp. nov., a novel haloalkaliphilic member of the family Natrialbaceae (class Halobacteria) from hypersaline alkaline lakes. Syst. Appl. Microbiol. 41, 355–362. doi: 10.1016/j.syapm.2018.04.002
Sorokin, D. Y., Toshchakov, S. V., Kolganova, T. V., and Kublanov, I. V. (2015). Halo(natrono)archaea isolated from hypersaline lakes utilize cellulose and chitin as growth substrates. Front. Microbiol. 6:942. doi: 10.3389/fmicb.2015.00942
Sorokin, D. Y., Yakimov, M., Messina, E., Merkel, A. Y., Koenen, M., Bale, N. J., et al. (2022). Natranaeroarchaeum sulfidigenes gen. nov., sp. nov., carbohydrate-utilizing sulfur-respiring haloarchaeon from hypersaline soda lakes, a member of a new family Natronoarchaeaceae fam. nov. in the order Halobacteriales. Syst. Appl Microbiol. 45:126356. doi: 10.1016/j.syapm.2022.126356
Srivastava, P., Braganca, J., Ramanan, S. R., and Kowshik, M. (2014b). Green Synthesis of Silver Nanoparticles by Haloarchaeon Halococcus salifodinae BK6. Adv. Mater. Res. 938, 236–241. doi: 10.4028/www.scientific.net/AMR.938.236
Srivastava, P., Braganca, J. M., and Kowshik, M. (2014a). In vivo synthesis of selenium nanoparticles by Halococcus salifodinae BK18 and their antiproliferative properties against HeLa cell line. Biotechnol. Prog. 30, 1480–1487. doi: 10.1002/btpr.1992
Srivastava, P., Nikhil, E. V. R., Bragança, J. M., and Kowshik, M. (2015). Anti-bacterial TeNPs biosynthesized by haloarcheaon Halococcus salifodinae BK3. Extremophiles 19, 875–884. doi: 10.1007/s00792-015-0767-9
Szabó, A., Korponai, K., Kerepesi, C., Somogyi, B., Vörös, L., and Bartha, D. (2017). Soda pans of the Pannonian steppe harbor unique bacterial communities adapted to multiple extreme conditions. Extremophiles 21, 639–649. doi: 10.1007/s00792-017-0932-4
Tag, H. M., Saddiq, A. A., Alkinani, M., and Hagagy, N. (2021). Biosynthesis of silver nanoparticles using Haloferax sp. NRS1: Image analysis, characterization, in vitro thrombolysis and cytotoxicity. AMB Express 11:75. doi: 10.1186/s13568-021-01235-3
Taran, M., Monazah, A., and Alavi, M. (2017). Using petrochemical wastewater for synthesis of cruxrhodopsin as an energy capturing nanoparticle by Haloarcula sp. IRU1. Prog. Biol. Sci. 6, 151–157. doi: 10.22059/PBS.2016.590017
Taran, M., Rad, M., and Alavi, M. (2018). Biosynthesis of TiO2 and ZnO nanoparticles by Halomonas elongata IBRC-M 10214 in different conditions of medium. BioImpacts 8, 81–89. doi: 10.15171/bi.2018.10
Thombre, R. S., Shinde, V. D., Oke, R. S., Dhar, S. K., and Shouche, Y. S. (2016). Biology and survival of extremely halophilic archaeon Haloarcula marismortui RR12 isolated from Mumbai salterns, India in response to salinity stress. Sci. Rep. 6:25642. doi: 10.1038/srep25642
Tiquia-Arashiro, S., and Rodrigues, D. (2016). “Halophiles in Nanotechnology,” in Extremophiles: applications in nanotechnology, eds. S. Tiquia-Arashiro and D. Rodrigues (Switzerland: Springer, Cham), 53–88. doi: 10.1007/978-3-319-45215-9_2
Torregrosa-Crespo, J., Pire Galiana, C., and Martínez-Espinosa, R. M. (2017). “Biocompounds from Haloarchaea and their uses in biotechnology,” in Archaea-new biocatalysts, novel pharmaceuticals and various biotechnological applications, eds. H. Sghaier, A. Najjar, and K. Ghedira (London: IntechOpen), 63–82. doi: 10.5772/intechopen.69944
Uthandi, S., Saad, B., Humbard, M. A., and Maupin-Furlow, J. A. (2010). LccA, an Archaeal Laccase Secreted as a Highly Stable Glycoprotein into the Extracellular Medium by Haloferax volcanii. Appl. Environ. Microbiol. 76, 733–743. doi: 10.1128/AEM.01757-09
Ventosa, A. (2006). “Unusual microorganisms from unusual habitats: Hypersaline environments,” in Prokaryotic diversity mechanisms and significance, eds N. A. Logan, H. M. Lappin-Scott, and P. C. F. Oyston (Cambridge: Cambridge University Press), 223–254. doi: 10.1017/CBO9780511754913.015
Ventosa, A., Sánchez-Porro, C., Martín, S., and Mellado, E. (2005). “Halophilic Archaea and Bacteria as a Source of Extracellular Hydrolytic Enzymes,” in Adaptation to life at high salt concentrations in archaea, bacteria, and eukarya, eds N. Gunde-Cimerman, A. Oren, and A. Plemenitaš (Berlin: Springer), 337–354. doi: 10.1007/1-4020-3633-7_23
Vera-Bernal, M., and Martínez-Espinosa, R. M. (2021). Insights on cadmium removal by bioremediation: The case of Haloarchaea. Microbiol. Res. 12, 354–375. doi: 10.3390/microbiolres12020024
Verma, D. K., Chaudhary, C., Singh, L., Sidhu, C., Siddhardha, B., Prasad, S. E., et al. (2020). Isolation and taxonomic characterization of novel haloarchaeal isolates from indian solar saltern: A brief review on distribution of bacteriorhodopsins and V-Type ATPases in haloarchaea. Front. Microbiol. 11:554927. doi: 10.3389/fmicb.2020.554927
Voica, D. M., Bartha, L., Banciu, H. L., and Oren, A. (2016). Heavy metal resistance in halophilic Bacteria and Archaea. FEMS Microbiol. Lett. 363:fnw146. doi: 10.1093/femsle/fnw146
Wang, G., Kennedy, S. P., Fasiludeen, S., Rensing, C., and DasSarma, S. (2004). Arsenic resistance in Halobacterium sp. strain NRC-1 examined by using an improved gene knockout system. J. Bacteriol. 186, 3187–3194. doi: 10.1128/JB.186.10.3187-3194.2004
Wei, W., Hu, X., Yang, S., Wang, K., Zeng, C., Hou, Z., et al. (2022). Denitrifying halophilic archaea derived from salt dominate the degradation of nitrite in salted radish during pickling. Int. Food Res. J. 152:110906. doi: 10.1016/j.foodres.2021.110906
Williams, G. P., Gnanadesigan, M., and Ravikumar, S. (2013). Biosorption and bio-kinetic properties of solar saltern halobacterial strains for managing Zn2+, As2+ and Cd2+ metals. Geomicrobiol. J. 30, 497–500. doi: 10.1080/01490451.2012.732663
Yadav, A. N., Sharma, D., Gulati, S., Singh, S., Dey, R., Pal, K. K., et al. (2015). Haloarchaea endowed with phosphorus solubilization attribute implicated in phosphorus cycle. Sci. Rep. 5:12293. doi: 10.1038/srep12293
Yatsunami, R., Sato, M., Orishimo, K., Hatori, Y., Zhang, Y., Takashina, T., et al. (2010). Gene expression and characterization of a novel GH family 18 chitinase from extremely halophilic archaeon Halobacterium salinarum NRC-1. J. Jpn. Soc. Extrem. 9, 19–24. doi: 10.3118/jjse.9.19
Zafrilla, B., Martínez-Espinosa, R. M., Alonso, M. A., and Bonete, M. J. (2010). Biodiversity of archaea and floral of two inland saltern ecosystems in the Alto Vinalopó Valley, Spain. Saline Syst. 6:10. doi: 10.1186/1746-1448-6-10
Zalazar, L., Pagola, P., Miró, M. V., Churio, M. S., Cerletti, M., Martínez, C., et al. (2019). Bacterioruberin extracts from a genetically modified hyperpigmented Haloferax volcanii strain: Antioxidant activity and bioactive properties on sperm cells. J. Appl. Microbiol. 126, 796–810. doi: 10.1111/jam.14160
Zenke, R., von Gronau, S., Bolhuis, H., Gruska, M., Pfeiffer, F., and Oesterhelt, D. (2015). Fluorescence microscopy visualization of halomucin, a secreted 927 kDa protein surrounding Haloquadratum walsbyi cells. Front. Microbiol. 6:249. doi: 10.3389/fmicb.2015.00249
Zhang, T., Datta, S., Eichler, J., Ivanova, N., Axen, S. D., Kerfeld, C. A., et al. (2011). Identification of a haloalkaliphilic and thermostable cellulase with improved ionic liquid tolerance. Green Chem. 13, 2083–2090. doi: 10.1039/c1gc15193b
Zhao, Y., Rao, Z., Xue, Y., Gong, P., Ji, Y., and Ma, Y. (2015). Biosynthesis, property comparison, and hemocompatibility of bacterial and haloarchaeal poly (3-hydroxybutyrate-co-3-hydroxyvalerate). Sci. Bull. 60, 1901–1910. doi: 10.1007/s11434-015-0923-8
Keywords: haloarchaea, nanoparticles, antimicrobial compound, anticancer, antioxidants, carotenoids, halocins
Citation: Moopantakath J, Imchen M, Anju VT, Busi S, Dyavaiah M, Martínez-Espinosa RM and Kumavath R (2023) Bioactive molecules from haloarchaea: Scope and prospects for industrial and therapeutic applications. Front. Microbiol. 14:1113540. doi: 10.3389/fmicb.2023.1113540
Received: 01 December 2022; Accepted: 14 March 2023;
Published: 31 March 2023.
Edited by:
Philippe M. Oger, UMR 5240 Microbiologie, Adaptation et Pathogenie (MAP), FranceReviewed by:
Salma Mukhtar, The Connecticut Agricultural Experiment Station, United StatesJudith Maria Braganca, Birla Institute of Technology and Science, India
Copyright © 2023 Moopantakath, Imchen, Anju, Busi, Dyavaiah, Martínez-Espinosa and Kumavath. This is an open-access article distributed under the terms of the Creative Commons Attribution License (CC BY). The use, distribution or reproduction in other forums is permitted, provided the original author(s) and the copyright owner(s) are credited and that the original publication in this journal is cited, in accordance with accepted academic practice. No use, distribution or reproduction is permitted which does not comply with these terms.
*Correspondence: Ranjith Kumavath, rnkumavath@gmail.com, rnkumavath@pondiuni.ac.in; Rosa María Martínez-Espinosa, rosa.martinez@ua.es
†These authors have contributed equally to this work