- 1Department of Veterinary and Animal Sciences, Faculty of Health and Medical Sciences, University of Copenhagen, Frederiksberg, Denmark
- 2Department of Food Science, Faculty of Science, University of Copenhagen, Frederiksberg, Denmark
Introduction: The association between the porcine pre-weaning gut microbiota composition and diversity, and subsequent post-weaning diarrhea (PWD) susceptibility is currently being studied. In this longitudinal study, we examined the association between pre-weaning fecal microbiome composition and diversity, and PWD development in a Danish sow herd.
Methods: Forty-five pigs were followed from birth until 7 days after weaning (post-natal day (PND) 33). At PND 33, the pigs were categorized as PWD cases or healthy controls based on fecal consistency. We compared their fecal microbiomes at PND 8, late lactation (PND 27) and 7 days post weaning (PND 33) using 16S rRNA V3 region high-throughput sequencing. At PND 27 and 33, we also weighed the pigs, assessed fecal shedding of hemolytic Escherichia coli by culture and characterized hemolytic isolates by ETEC virulence factors with PCR and by whole genome sequencing.
Results: A total of 25 out of 45 pigs developed PWD and one Enterotoxigenic E. coli strain with F18:LT:EAST1 virotype was isolated from most pigs. At PND 33, we found differences in beta diversity between PWD and healthy pigs (R2 = 0.027, p = 0.009) and that body weight was associated with both alpha and beta diversity. Pre-weaning fecal microbiome diversity did not differ between PWD and healthy pigs and we found no significant, differentially abundant bacteria between them.
Conclusion: In the production herd under study, pre-weaning fecal microbiome diversity and composition were not useful indicators of PWD susceptibility.
Introduction
The gut microbiota (GM) has an essential role in gut health (Wang et al., 2016). The composition of the microbial community is driven by host genetics (McKnite et al., 2012), diet (Frese et al., 2015), environmental conditions (Schmidt et al., 2011), and potentially physiological development (Leite et al., 2021). The rapid transition of diet in pig production, from milk as the primary nutrient source to solid feed at weaning, causes fast shifts in the GM composition and its enzymatic functional capacities (Frese et al., 2015). Taken together with the early time of weaning in pig production at three to four weeks of age (Jensen and Recén, 1989), and the stress associated with changes of environment, the weaning period is a vast challenge that can lead to gut microbiota dysbiosis; a state of gut microbial imbalance (Gresse et al., 2017) that may favor opportunistic pathogens. Consequently, post-weaning diarrhea (PWD) is very common in pig production and constitutes a serious problem in terms of animal welfare, financial costs and antibiotic use, where metaphylatic treatments are often used to control the disease (Rhouma et al., 2017). Post-weaning diarrhea is a multifactorial condition and enterotoxigenic Escherichia coli (ETEC) is the primary pathogen isolated from outbreaks (Dubreuil et al., 2016). Enterotoxigenic E. coli cause secretory diarrhea and are distinguished by their fimbriae type and the types of enterotoxins they produce. The fimbriae types most frequently associated with ETEC-related PWD are F18 and F4 (Frydendahl, 2002).
Recently, there has been increasing attention to the role of the GM in relation to PWD. Research shows that the early life environment affects microbiome composition and stimulates the maturation of the immune system (Mulder et al., 2009). Dysbiosis caused by unfavorable environmental conditions, such as limited or compromised exposure to microbes in the natural environment (Mulder et al., 2009; Schmidt et al., 2011), or by antibiotics early in life (Fouhse et al., 2019) may have long lasting consequences to host immune response (Mulder et al., 2009; Fouhse et al., 2019). This may consequently affect vulnerability to later infections as shown in experimentally infected mice exposed to early-life antibiotics (Roubaud-Baudron et al., 2019). Dou and colleagues found that pigs with PWD, under experimental facility conditions in France, differed in fecal microbiome diversity indices and composition from healthy pigs as early as 1 week after birth (Dou et al., 2017). Healthy pigs showed less evenness and higher abundance of taxa belonging to the families Lactobacillaceae and Ruminocaceae and other commensals. In a recent Czech herd study (Karasova et al., 2021), researchers investigated the role of the fecal microbiota in late lactation (3 days before weaning) in pigs with and without PWD. Healthy and PWD affected pigs differed in fecal microbiota composition, 3 days before weaning, although no extensive differences were found based on principal coordinate analysis. These findings indicate that certain GM communities might be associated with subsequent PWD development. However, more research is needed to elucidate if cues in the pre-weaning fecal microbiome such as diversity indices and abundance of certain taxa can reveal risk of PWD development. This may especially be relevant in a herd setting where management factors and antibiotic use can influence the GM.
In this study, we used 16S rRNA V3 high-throughput sequencing to investigate the fecal microbiome at early preweaning (post-natal day (PND) 8), at late lactation (PND 27) and at 7 days after weaning (PND 33). We determined diversity indices and microbiome composition and investigated their association with PWD development in pigs.
Materials and methods
Ethical statement
The study was ethically assessed and approved by the Animal Ethics Institutional Review Board at the Department of Veterinary and Animal Sciences, University of Copenhagen. Approval number: 2022-02-PNH-006A.
Animals
A total of 45 female, Duroc x Landrace x Yorkshire pigs from 9 sows (5 pigs per sow) were included in the study. The herd of the study was part of the Danish SPF (Specific Pathogen Free) system, declared free from Actinobacillus pleuropneumoniae serotype 2, toxigenic Pasteurella multocida, Brachyspira hyodysenteriae and porcine reproductive and respiratory syndrome virus.
Female pigs, born on a single day, were weighed at birth. No crossfostering was performed in the pre-weaning period. Sow parity ranged from 2 to 5. The piglets were fed with milk formula through milk dispensers as a supplement to sow milk and were offered creep feed pre-weaning from PND 16. Pigs were weaned at PND 27 and received a standard weaner diet without added antimicrobials or medicinal zinc. All pigs had the same treatment history and received a single dose of amoxicillin trihydrate at post-natal day (PND) 1, Toltrazuril (anticoccidial) at PND 4, and metaphylatic lincomycin hydrochloride and spectinomycin sulfate (Linco-Spectin® Vet) treatment via drinking water during the entire study period after weaning. All 45 pigs were transported to and housed together at the nursery in a single pen, without additional pigs.
Experimental design
The study was an observational case–control study with pig as the study unit. The study population was randomized in blocks by litter where five female pigs (birthweight higher than 1 kg) from each of 9 litters were selected out of available female pigs of the litter. Rectal swabs for fecal microbiome analysis were collected longitudinally from the pigs at PND 8, at late lactation (PND 27) and at 7 days after weaning (PND 33). In addition, at PND 27 and 33, fecal samples were collected rectally from the pigs for assessment of diarrhea and shedding of hemolytic E. coli and ETEC. Pigs were grouped based on fecal consistency at PND 33 into “PWD-cases” or “healthy controls” to compare their fecal microbiomes at the three time points.
Fecal scoring and fecal dry matter content analysis
Feces were assessed using a visual fecal consistency score (1–4) (Pedersen and Toft, 2011) and diarrhea was defined as a fecal score greater than 2. Fecal dry matter content was determined by weighing feces before and after drying to constant weight, at 75°C for 18 h. Dried feces were kept in desiccators between weighings.
Bacteriological culturing of fecal samples
Feces collected at PND 27 and 33 were streaked on blood agar (BA, 5% calf blood in blood agar base, Oxoid CM0055, ThermoFischer) in three dilution zones. The BA plates were incubated at 37°C overnight and semiquantitative assessment of hemolytic E. coli growth (%- hemolytic E. coli growth out of total growth, 0–100%) was performed. One haemolytic isolate per pig per timepoint was subjected to multiplex PCR to evaluate presence of the ETEC virulence factors F4, F18, STb, STa and LT with primers and protocol previously described (Zhang et al., 2007).
Whole genome sequencing of Escherichia coli F18, LT isolates
Five randomly chosen E. coli F18, LT isolates were subjected to whole genome sequencing (WGS) for further characterization and to investigate if one or more strains were present. Extraction of DNA was performed on Maxwell® RSC instrument with Maxwell RSC culture cell’s DNA kit (Promega, Madison, USA) and WGS was performed at coverage of 30X, using 2×250 basepair V2 reagent kit with NGS-MiSeq (Illumina, University of Copenhagen, Denmark) platform, by service of Veterinary Clinical Microbiology at the Department of Veterinary and Animal Sciences, University of Copenhagen, Denmark. Raw sequencing reads were analyzed for virulence genes, serotypes and antibiotic resistance genes with the bioinformatics tools: VirulenceFinder (Version 2.0) (Joensen et al., 2014; Clausen et al., 2018; Tetzschner et al., 2020), SerotypeFinder (Version 2.0) (Joensen et al., 2015), and ResFinder (Version 4.1) (Zankari et al., 2017; Clausen et al., 2018; Bortolaia et al., 2020), hosted by the Center for Genomic Epidemiology (CGE) (www.genomicepidemiology.org).
16S rRNA V3 region high-throughput sequencing
Rectal swabs (Eswabs with Liquid Amies Medium, 490 CE.A, Copan Diagnostics Inc., Brescia, Italy) were stored at −80°C before DNA extraction. One rectal swab taken at PND 33 from a pig without PWD was missing before DNA extraction. Extraction of genomic DNA was carried out using Bead-Beat Micro AX Gravity kit (A&A Biotechnology, Gdynia, Poland) following the manufacturer’s instructions. DNA concentration was measured using Qubit® dsDNA HS Assay Kit (Life Technologies, CA, USA) with Varioskan Flash Multimode Reader (Thermo Fischer Scientific, MA, USA). Extracted DNA was diluted 1:10 with sterile water before PCR amplification of the V3 region of the 16S rRNA gene. The following primers were used: nxt_338_F: 5′-CCTACGGGWGGCAGCAG-3′ and nxt_518_R: 5′-ATTACCGCGGCTGCTGG-3′ (Integrated DNA Technologies; Leuven, Belgium). Reaction mix for PCR1 contained 5 μL of PCRBIO buffer, 0.5 μL primer mix (10 μM of each primer), 0.25 μL PCRBIO HiFi polymerase (PCR Biosystems Ltd., London, United Kingdom), 1 μL bovine serum albumin, 1 μL formamide, 12.25 μL nuclease-free water and 5 μL genomic DNA (approximately 1 ng/μL), per sample. The following thermal cycling was used: 95°C for 2 min, then 33 cycles of 95°C for 15 s, 55°C for 15 s, 72°C for 20 s, and 1 cycle of 72°C for 4 min. PCR1 products were verified and quality checked on 1.5% agarose gels. Products from PCR1 were then purified using AMPure XP beads (Beckman Coulter Genomic, CA, USA) with Biomek 4,000 automated laboratory workstation. Afterwards, barcoding (PCR2) of purified PCR products was performed. Reaction mix for PCR2 contained 5 μL PCRBIO buffer, 0.25 μL PCRBIO HiFi polymerase, 4 μL barcode primers (2 μL P5 and 2 μL P7 primers, Nextera Index Kit), 13.75 μL nuclease-free water and 2 μL PCR product, per sample. The cycling conditions for PCR2 were: 95°C for 1 min, then 13 cycles of 95°C for 15 s, 55°C for 15 s, 72°C for 15 s, and finally, 1 cycle of 72°C for 5 min. The barcoded PCR products were subjected to cleaning and bead-based normalization with AMPure XP beads. DNA concentration was measured once more and samples were pooled in equimolar concentrations. Finally, high throughput Illumina MiSeq (Illumina, CA, USA) sequencing was performed following the manufacturer’s instructions.
Gut microbiota sequencing
Quality-control of reads, de-replicating, purging from chimeric reads and constructing zero-radius Operational Taxonomic Units (zOTU) was conducted with the UNOISE pipeline (Edgar, 2018) and taxonomically assigned with Sintax (Edgar, 2016). Taxonomical assignments were obtained using the EZtaxon for 16S rRNA gene database. Description of the workflow can be found in: https://github.com/jcame/Fastq_2_zOTUtable.
FUT1 genotyping
All pigs were genotyped for the M307 mutation in FUT1. The M307 is a good marker for ETEC F18 resistant animals (Meijerink et al., 1997). Pig genomic DNA extraction was done from rectal swabs (Eswabs with Liquid Amies Medium, 490 CE.A, Copan Diagnostics Inc., Brescia, Italy). Genomic DNA was extracted from each swab using a crude DNA extraction method. Briefly, 100 μL medium was placed in a tube with 100 μL of NaOH solution (25 mM NaOH +2 mM EDTA). The sample was then heated at 100°C for 20 min. After centrifugation, the liquid phase was transferred and neutraliZed with 100 μL of Tris solution. The TaqMan SNP Genotyping assays were designed by Thermo Fisher Scientific (Waltham, Massachusetts, USA).
One μL of the crude DNA solution was used for TaqMan genotyping according to the manufacturer’s instructions. Allele calling was performed on a Mx3000P qPCR System (Agilent, Santa Clara, California, USA).
Statistics
Statistical analysis was performed in R version 4.1.1 (R Core Team, 2022). p-values <0.05 were considered significant and < 0.10 a tendency.
Differences in fecal dry matter content and in shedding of hemolytic E. coli between diarrhea and non-diarrhea samples were evaluated with Wilcoxon rank sum test. Normal distribution of data was assessed using QQ-plots.
Association of litter and body weight with occurrence of PWD was evaluated with logistic regression.
For microbiome analysis and visualization, R packages vegan (Oksanen et al., 2022), phyloseq (McMurdie and Holmes, 2013), ANCOMBC (Mandal et al., 2015; Lin and Peddada, 2020), GUniFrac (Chen et al., 2022), lme4 (Bates et al., 2015), lmerTest (Kuznetsova et al., 2017), viridis (Garnier et al., 2021), tidyverse (Wickham et al., 2019), ggtext (Wilke and Wiernik, 2022), cowplot (Wilke, 2020), ggplot2 (Wickham, 2016), and RColorBrewer (Neuwirth, 2022) were used. Four samples were removed due to low number of reads (<10,000), resulting in n = 44, 43, 43 samples at PND 8, PND 27, and PND 33, respectively. All samples were rarefied to the same sequencing depth of 10,000 reads/sample.
Group differences in Canberra and binary Soerensen-Dice distance matrices between healthy and PWD pigs were assessed with PERMANOVA at 999 permutations. Model selection was based on forward selection using ordiR2step function of vegan. Group differences were assessed at each timepoint, with litter included in the model as strata. At PND 33, body weight at PND 33 was also included as a fixed effect in the model. Multivariate homogeneity of group dispersions were checked with betadisper function. Ordination plots were generated from the full model using capscale (distance-based redundancy analysis) function of vegan.
Significant group differences in Shannon and Simpson indices and number of observed zOTUs were evaluated with linear mixed model with litter included in the model as a random effect. At PND 33, body weight at PND 33 was also included as a fixed effect in the model.
Mean relative abundance between groups were calculated at genus level and visualized for each time point. Pearson’s correlation was calculated between relative abundance of Prevotella and body weight at PND 33.
Differential abundance analysis at each time point, was performed on amplicon counts at genus and zOTU level, using a taxon prevalence cut across samples of 10%, detecting structural zeros (using equation 1 in section 3.2 (Kaul et al., 2017)) and adjusting p-values with Holm–Bonferroni method. Data was analyzed with a univariable model (ANCOM-BC function) with PWD status as the explanatory variable and with a multivariable model (ANCOM function) where litter was added as random effect. At PND 33, body weight at PND 33 was also added to the model as fixed effect.
Results
Prevalence of diarrhea and risk factors for PWD
Just before weaning, at PND 27, only 1 out of 45 pigs had diarrhea. Four feces samples were missing at PND 27, as four pigs did not defecate at sample collection. Seven days after weaning, at PND 33, 25 out of the 45 pigs had diarrhea (Table 1). The majority of PWD samples were of watery consistency (16 out of 25 samples) indicative of severe diarrhea. Visual consistency scoring was confirmed by the significantly lower fecal dry matter content of PWD samples (n = 25) compared to non-diarrhea samples (n = 20) (Table 2, Wilcoxon test statistic (W) = 490, difference in medians = 12.33, 95% CI = 8.92;16.14, p < 0.0001).
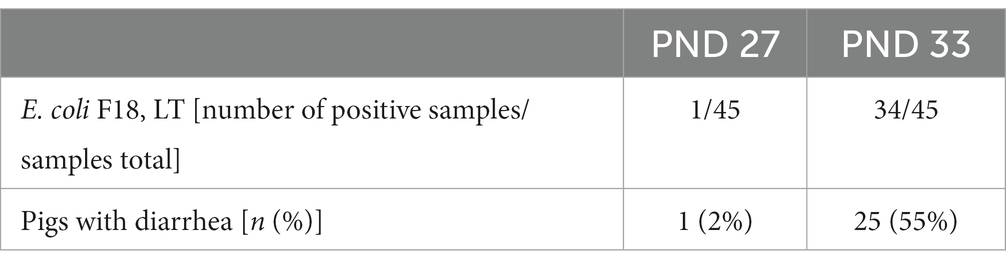
Table 1. Prevalence of diarrhea and microbiological findings at late lactation (PND 27) and 7 days post weaning (PND 33).
Neither litter, birth weight (mean ± standard deviation (SD): 1.31 ± 0.18 kg and 1.38 ± 0.23 kg in PWD and healthy pigs, respectively) or body weight at PND 33 (mean ± SD: 8.8 ± 1.56 kg and 8.0 ± 1.6 kg in PWD and healthy pigs, respectively) had a significant association with PWD status in the pigs. A higher weaning weight was associated with slightly higher odds of PWD (mean weaning weight ± SD in PWD pigs were 8.66 ± 1.54 kg and 7.55 ± 1.69 kg in healthy, respectively, odds ratio = 1.54, 95% confidence interval (CI) = 1.05;2.4, p = 0.02).
Microbiological findings
At PND 27, shedding of hemolytic E. coli was detected in a non-diarrheic sample from a single animal. Otherwise, no shedding of hemolytic E. coli was found before weaning. At PND 33, non-diarrhea samples tended to have less growth of hemolytic E. coli than the post-weaning diarrhea samples (Tables 2, W = 162, difference in medians = −20.0, 95% CI = −4.0;3.7, p = 0.06). Genes encoding for F18 and LT were detected by PCR in the hemolytic E. coli isolate from PND 27 and the majority of isolates at PND 33. No genes encoding for F4 were detected. Whole genome sequencing of five randomly chosen F18, LT positive isolates revealed the same virotype, thus confirming an ETEC F18 associated outbreak caused by a single strain. The herd strain was identified as O8:H17, F18ac, LT, EAST-1, with a WGS-predicted phenotype resistant to lincomycin, but not spectinomycin.
Host genotype susceptibility to ETEC F18 using the FUT1-marker
Overall, 33 homozygous susceptible (SS), 11 heterozygous susceptible (RS) and 1 homozygous resistant (RR) pigs were identified by genotyping the FUT1-marker (Meijerink et al., 1997). The distribution of genotypes was similar between PWD cases and healthy controls (Table 3). Surprisingly, the single resistant pig was located in the PWD group which was confirmed by genotyping two independent samples from the same pig.
Microbiome analysis
Alpha diversity
In order to identify potential indicators for PWD early in pigs’ lives, we processed all fecal samples at PND 8, 27 and 33 with 16S rRNA V3 amplicon sequencing. In analysis of alpha diversity (Shannon and Simpson’s diversity index, and observed number of zOTUs), no statistically significant differences were found between the PWD group and healthy group at any timepoint (Figure 1). At PND 33, in the post weaning period, we found that the co-variable body weight was positively associated with increased alpha diversity. We did not find the same association between body weight and alpha diversity at PND 27 in the late lactation period.
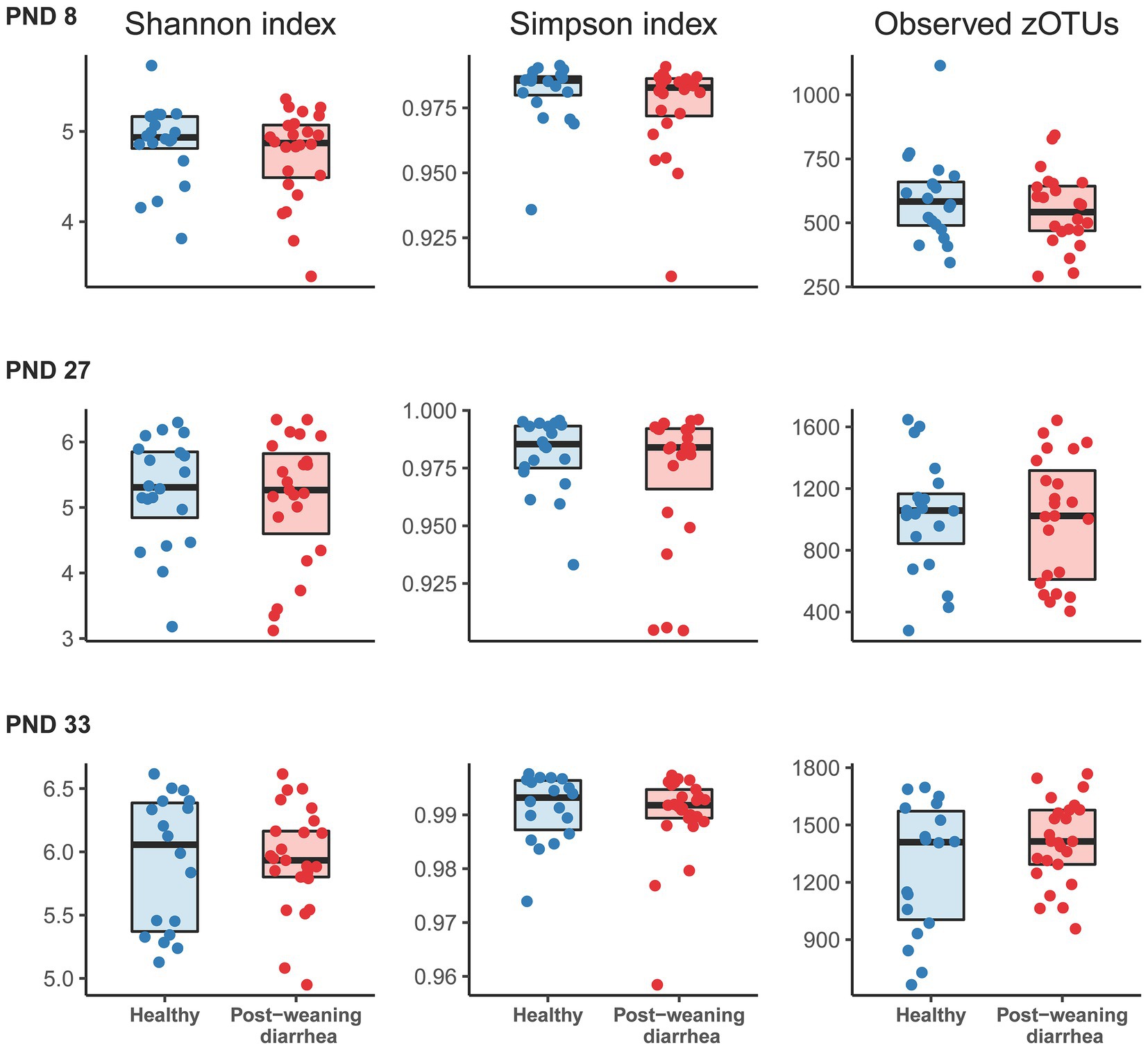
Figure 1. Comparison of alpha diversity indices based on post-weaning diarrhea (PWD) status. Groups were compared at post-natal day (PND) 8 (early lactation, healthy: n = 20 pigs, PWD: n = 24 pigs), at PND 27 (late lactation, healthy: n = 20 pigs, PWD: n = 23 pigs), and PND 33 (7 days after weaning, healthy: n = 18 pigs, PWD: n = 25 pigs). Shannon and Simpson indices and number of observed zOTUs were based on a rarefied zOTU table generated from 16S rRNA V3 high throughput amplicon sequencing. Graphs show boxplots with medians marked with bold line. Statistical differences between groups were assessed with linear mixed models. No significant group differences were found for PWD status at any time point. At PND 33, the covariable body weight at PND 33 was positively associated with increased Shannon diversity (effect size: 0.13, 95% CI: [0.068; 0.208], p = 0.0002), Simpson’s diversity (effect size: 0.001, 95% CI: [−0.00012; 0.002], p = 0.07), and number of observed zOTUs (effect size: 86.33, 95% CI: [46.27; 126.80], p = 0.00009).
Beta diversity
The analysis of beta diversity pre-weaning revealed no statistically significant differences at PND 8 (early lactation) nor at PND 27 (late lactation) based on PWD future status (Figure 2; Supplementary material 1). However at PND 33 (7 days after weaning), at the time of PWD occurrence, the fecal microbiotas of PWD pigs and healthy pigs had minor, but significant differences in their overall bacterial communities. In addition, we found that the co-variable body weight at PND 33 was also associated with differences in beta diversity at PND 33. In contrast, body weight was not associated with significant differences in beta diversity at PND 27 in the late lactation period.
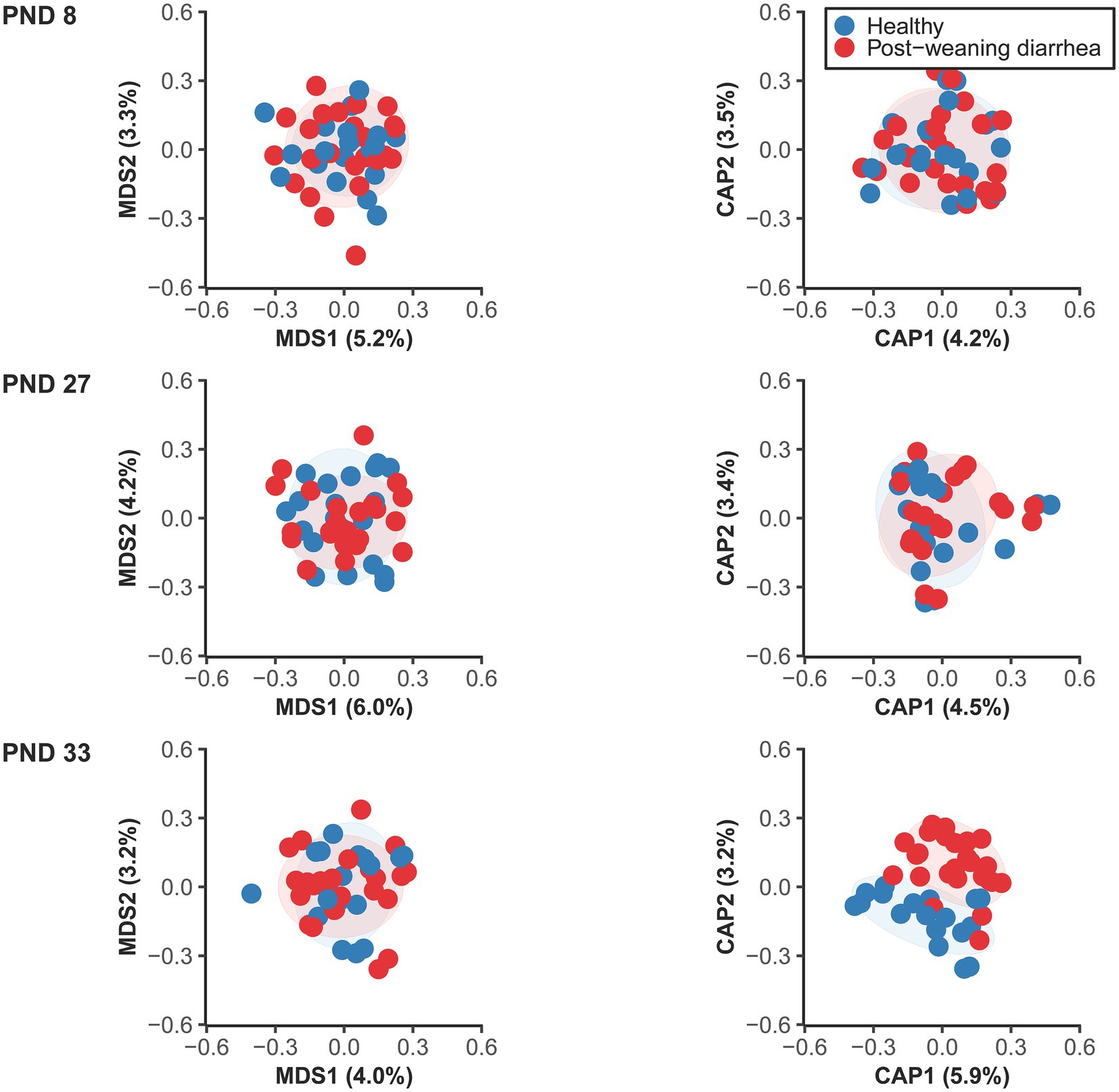
Figure 2. Unconstrained (left) and constrained ordination plots (right) of Canberra distance matrix of the fecal microbiome between healthy and post-weaning diarrhea (PWD) affected, at PND 8 (healthy: n = 20 pigs, PWD: n = 24 pigs), PND 27 (healthy: n = 20 pigs, PWD: n = 23 pigs), and PND 33 (healthy: n = 18 pigs, PWD: n = 25 pigs). Plots were created based on the rarefied zOTU table generated by 16S rRNA high throughput sequencing in the V3 region. Ellipses show 80% confidential areas assuming multivariate t-distribution. PERMANOVA was used to evaluate differences in distances at 999 permutations between the groups. Significant group difference in PWD status were found at PND 33: R2 = 0.027, p = 0.009. In addition, there was a significant effect of the covariable body weight at PND 33: R2 = 0.028, p = 0.003.
There were no significant differences in dispersions between groups (Figure 2; Supplementary material 1) which confirmed that significant PERMANOVA results were due to location effects.
Differential abundance analysis
Shift in the composition of bacterial communities were further investigated with differential abundance analysis. The most abundant bacterial genera and their mean relative abundance grouped by PWD status are illustrated in Figure 3. Four genera are of particular interest because of their dynamics in time: Lactobacillus, Escherichia/Shigella, Prevotella, Campylobacter. Lactobacillus and Escherichia/Shigella were the most dominant genera before weaning, while after weaning, the mean relative abundance of Prevotella increased in both groups (from 2.94% at PND 27 to 6.69% at PND 33 in healthy; from 3.37% at PND 27 to 9.76% at PND 33 in PWD pigs), making it the second most abundant genus post weaning. Interestingly, despite the ongoing ETEC associated PWD outbreak, the mean relative abundance of Escherichia/Shigella decreased at PND 33 both in healthy (from 9.45% at PND 27 to 2.82% at PND 33) and PWD pigs (from 18.44% at PND 27 to 7.77% at PND 33).
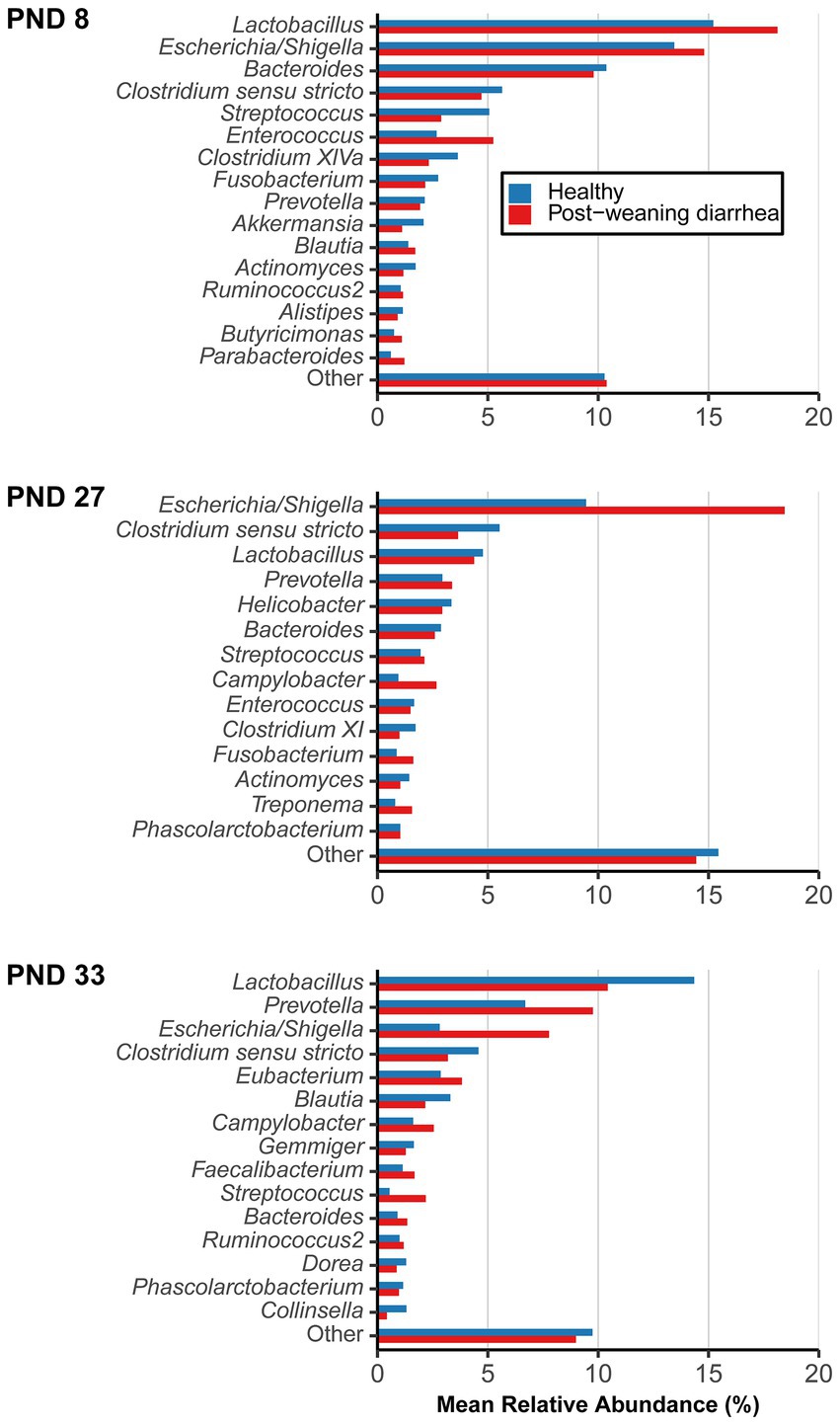
Figure 3. Mean relative abundance of most abundant genera, grouped by post-weaning diarrhea (PWD) status. Samples were collected longitudinally and the groups were compared at post-natal day (PND) 8 (early lactation, healthy: n = 20 pigs, PWD: n = 24 pigs), at PND 27 (late lactation, healthy: n = 20 pigs, PWD: n = 23 pigs), and PND 33 (7 days after weaning, healthy: n = 18 pigs, PWD: n = 25 pigs) which was the time of active PWD where pigs were divided into PWD cases and healthy controls for comparison. Genera representing <1% mean relative abundance were pooled into “Other.” Accumulated mean relative abundance of unassigned taxa (not shown) at genus level represented 20.00% (healthy) and 19.24% (PWD) at PND 8, 45.21% (healthy) and 37.62% (PWD) at PND 27, and 45.03% (healthy) and 41.36% (PWD) at PND 33.
Agglomerating amplicon counts and analyzing at genus level did not reveal any differentially abundant genera based on PWD status at any individual timepoint. When we analyzed the data at a lower taxonomic level (zOTU level) we found differences in composition of microbial communities, but only as differences in presence/absence of rare taxa between groups.
At PND 8, 12 zOTUs were detected as structural zeroes (complete absence in one of the groups) (Supplementary material 2). The zOTUs did not belong to top abundant genera, except for two zOTU identified as Prevotella (mean relative abundance across taxa and samples 0.003%) that was found present only in the PWD group, and one zOTU identified as Lactobacillus animalis (mean relative abundance across taxa and samples 0.002%) that was only present in the healthy control.
At PND 27, a total of 17 zOTUs were detected as structural zeroes (Supplementary material 3). Among these were three zOTUs identified as Lactobacillus spp. (Lactobacillus sanfranciscensis and Lactobacillus rapi, mean relative abundance across taxa and samples at PND 27 of 0.013%) that were present only in healthy pigs, a genus that also had numerically higher mean relative abundance in healthy pigs (Figure 3 –PND 27).
At PND 33, a total of 99 zOTUs (98 of which were detected as structural zeroes) were differentially abundant between healthy and PWD pigs in the univariable analysis (Supplementary material 4). In the multivariable model, only the 98 zOTUs detected as structural zeroes remained differentially abundant between healthy and PWD pigs (Supplementary material 4). Four zOTUs identified as Campylobacter hyointestinalis subsp. Lawsonii (mean relative abundance across taxa and samples at PND 33 of 0.238%) were present only in PWD pigs. Campylobacter spp. was one of the top genera that had numerically higher mean relative abundance in PWD case pigs at PND 33 (Figure 3 –PND 33). Several zOTUs identified as Prevotella spp. were only detected in PWD pigs and this genus also had numerically higher mean relative abundance in the PWD affected pigs (Figure 3 –PND 33). Relative abundance of Prevotella at PND 33 increased with body weight at PND 33 (Supplementary material 5). Thus, questioning if the presence of Prevotella and numerically higher mean relative abundance in the PWD group was simply due to the higher average body weight in this group.
In summary, findings were limited to presence/absence of rare taxa between groups. Most of these differences were detected at PND 33 in the post-weaning period, and the least differences were found at PND 8 in the early lactation period.
Discussion
In this study, we investigated whether PWD-case pigs and healthy pigs had different fecal microbiomes in the pre-weaning period. Such distinct patterns could be useful as early biomarkers for PWD susceptibility and give the scientific background for preventive measures. However, under our conditions, pigs that developed ETEC F18 associated PWD did not have distinguishable fecal microbiotas from their healthy counterparts before weaning.
This contrasts to the work of Dou and colleagues, who found that PWD pigs could be distinguished from healthy pigs that had lower evenness and higher abundance of Ruminocacaceae, Prevotellaceae, Lachnospiraceae and Lactobacillaceae already at PND 7 (Dou et al., 2017). An important difference from their study was that our study was performed in a herd setting with conditions close to reality at a farm. For example, as is common in pig farms to counteract umbilical cord infection, IM amoxicillin treatment was given to all pigs at PND 1. This may have perturbed the GM and made it harder to differentiate between PWD predisposed and healthy pigs 7 days after treatment. Transient perturbations of the GM has been observed in pigs receiving oral amoxicillin during the first 2 weeks of life (Fouhse et al., 2019). Immediate changes in the GM after IM amoxicillin has not been documented in pigs, but it was found to reduce bacterial community diversity in the colon 5 weeks after administration (Janczyk et al., 2007). However, in a recent herd study where pigs received creep feed with medicinal zinc pre-weaning and in-feed antibiotics (sulfamethoxazole and trimethoprim) after weaning, researchers still found several taxa in the fecal microbiome that were differentially abundant between their PWD-cases and healthy controls in the late pre-weaning period (Karasova et al., 2021). This suggests that it is possible to distinguish between PWD predisposed and healthy pigs regardless of previous and ongoing antimicrobial treatment. However, we found no obvious microbiome differences that could be used to identify PWD susceptible and resilient pigs in the pre-weaning period in our study. Although Escherchia/Shigella and Lactobacillus appeared more abundant based on the visualization of mean relative abundance at PND 27, we did not find these genera differentially abundant between future PWD cases and healthy controls when analyzing amplicon counts. At PND 27, a few low abundant zOTUs identified as Lactobacillus spp. were only present in healthy controls, but no significant differences in abundance were found in zOTUs related to this genus. Thereby, questioning if these structural zeros had any role the pre-weaning fecal microbiome of the healthy controls at PND 27. A possible explanation for the discrepancy between studies could also be that our study design and statistical analysis were more robust than previous studies.
In the present study, the sample size of pigs undergoing microbiome analysis was higher than in the two previous studies. In the study by Dou and colleagues, just 5 healthy and 5 diarrhoeic pigs were selected for bacterial 16S sequencing and compared. Karasova and colleagues, assessed slightly fewer pigs than us with 17 healthy and 17 pigs with PWD at each time point. The statistical analysis conducted in our study was also more conservative than previous studies. For example, we assessed confounding variables in our statistical analysis and accounted for the random effect of litter. Litter mates likely have bacterial compositions more similar to each other as their microbiota has been shaped in the same pen environment and they have the same mother (Grönlund et al., 2011). For the differential abundance analysis, we used ANCOM-BC and ANCOM which are designed for analysis of microbiome data and may be more reliable in determining differentially abundant taxa (Nearing et al., 2022). Finally, an advantage of our study was that we supported our 16S sequencing data with clinical microbiology and found that an ETEC F18ac strain was associated with PWD occurrence in the study. This meant that we could assess FUT1 genotype of the pigs to get an idea of host susceptibility to ETEC F18. We found that the distribution of FUT1 susceptible pigs were similar between groups and could therefore exclude the possibility that healthy pigs were merely resistant toward ETEC F18. We found a single pig with ETEC F18 diarrhea that was FUT1 homozygous resistant and we can only speculate on reasons for this finding. In theory, viruses or other factors could also have been present at the same time as ETEC F18 and be involved in occurrence of the diarrhea, but we did not investigate for presence of viruses in the study. Even more speculative, it could be that the marker is not the true causative mutation for ETEC F18 susceptibility, although this statement contradicts with the existing literature (Meijerink et al., 2000). This assertion would call for additional studies and cannot be concluded from this study. Dou and colleagues also genotyped their pigs to rule out that differences in host susceptibility to “Enterobacteriaceae-associated diarrhea” were involved in prevalence of PWD. However, they only used MUC13 genotyping, which is merely a marker for ETEC F4ac susceptibility (Ren et al., 2012), and did not investigate if ETEC F4ac strain(s) were actually present or associated with PWD occurrence in their study.
In relation to the types of bacteria that were most abundant at the different time points our results were quite comparable with previous literature. At PND 8, we found the most abundant bacteria were Lactobacillus spp., Prevotella spp., Enterococcus spp., Bacteroides spp. which is comparable to the OTUs that contributed most to group discrimination at PND 7 described by Dou and colleagues (Dou et al., 2017). At PND 27 (late lactation), we found family Enterobacteriaceae, Lachnospiraceae, Ruminococcaeceae, and Lactobacillaeceae (data not shown at family level, but described here for comparison) as the most abundant families, which were among the most abundant 3 days before weaning in the study by Karasova and colleagues (Karasova et al., 2021). We also observed a decrease of family Enterobacteriaceae and an increase in Prevotellaceae 7 days after weaning, similar to the observations by Karasova et al. at 4 days after weaning and to what has been described in literature (Frese et al., 2015; Mach et al., 2015).
As in previous studies, we did not collect fecal samples from the pigs every day in the 2 week period after weaning. Diarrhea occurring within this 2 week period is referred to as post-weaning diarrhea (Rhouma et al., 2017). Instead, we defined the pigs as PWD cases and healthy controls based on diarrhea occurrence at 7 days after weaning. This was the time where the farmer experienced problems with PWD in his herd and it was therefore decided to be the optimal sampling time. Diarrhea could also have occurred before or after our sampling, but it was not practically feasible at the herd to collect samples during the entire post weaning period and the same limitation apply to previous studies. Further, occurrence of diarrhea after weaning is not limited only to the first 2 weeks. However, we chose to focus on the period just after weaning, which is the period where classic, ETEC-associated post-weaning diarrhea occurs and where farmers experience most problems with diarrhea.
In the present study, the pigs with higher weaning weight had slightly higher odds of developing PWD. A possible explanation for this could be that the heavy weaners overate after weaning, which may result in increased risk for PWD (Hampson and Smith, 1986). We speculate that a higher solid feed intake of larger pigs results in more plant based substrate available and thereby promote and shape a gut microbiota with higher diversity of plant degrading bacteria (Tomova et al., 2019). This could also explain why we saw that increased body weight was associated with differences in beta diversity and a higher alpha diversity at PND 33. However, we did not measure feed intake after weaning which could have provided further insight in this. Another possible explanation for the higher odds of diarrhea in the heavier weaners could be that these pigs fought more to establish their position in the new hierarchy. This could result in additional stress and altered food and water intake (Coutellier et al., 2007) for the larger animals. It may be relevant for future similar studies to weigh the pigs at the time they are divided into disease cases and healthy controls to adjust for this potential confounder on microbiome diversity and composition.
In conclusion, the pre-weaning fecal microbiome did not contain biomarkers that could be used to identify PWD susceptible pigs in our herd. Instead, differences in the fecal microbiomes between PWD case pigs and healthy controls were first observed at 7 days after weaning. The lacking differences in the pre-weaning fecal microbiomes may be a result of similar poor gut microbiotas in both groups due to early life antibiotic treatments or be herd-specific. Alternatively, our findings may imply that the association between the pre-weaning fecal microbiome and PWD susceptibility has previously been overestimated perhaps due to small sample size and crude statistical analysis. More research is needed to assess if modulating the gut microbiota toward higher diversity and certain bacterial compositions in early-life pigs is a relevant strategy and investment for counteracting PWD.
Data availability statement
The datasets generated/analyzed for this study can be found in its supplementary information files (Supplementary material 2–4 and 6–9). Supplementary material referred to in the article can be found in the article/Supplementary material. The data presented in the study are deposited in online repositories, with the following accession numbers: Whole genome sequences of the ETEC isolates as SRA data can be found at NCBI (BioProject: PRJNA905088) 16S amplicon sequence data as SRA data are available at NCBI (BioProject: PRJNA916113) SNPs can be found in the dbSNP data base: FUT1 (dbSNP:rs335979375).
Ethics statement
The animal study was reviewed and approved by Animal Ethics Institutional Review Board at the Department of Veterinary and Animal Sciences, University of Copenhagen. Approval number: 2022-02-PNH-006A. Written informed consent was obtained from the owners for the participation of their animals in this study.
Author contributions
MR designed the study, wrote the protocol, conducted the study, collected the data, did the statistical analysis, interpreted the data, and wrote the manuscript drafts. JN assisted in designing the study. MG, LP, and JN revised the protocol. MG and LP assisted and provided scientific input in microbiological assessment. JC-M provided scientific input for 16S rRNA sequencing, prepared the zOTU table and provided advice in statistical analysis of the microbiome data. CJ did FUT1 genotyping and assisted in interpretation of the genotyping results. All authors helped revising the manuscript drafts and approved the submitted version.
Funding
This work was supported by the Innovation fund Denmark (IFD) (File number: 7076-00038B). The funding body did not play a role in the design, analysis, and reporting of the study.
Acknowledgments
The authors thank Rasmus Jelle Syhler for assistance with animal procedures and Gitte Petersen for assistance with multiplex PCR. The authors thanks to Tina Bahrt Neergaard Mahler and Amanda Bastian Andersen for technical assistance on the FUT1 genotyping. The authors thanks to Denitsa Vladimirova Stefanova for technical assistance in DNA extraction and library preparation for 16S rRNA sequencing. The authors thank Tatjana Kristensen for technical assistance in whole genome sequencing. The authors also thank to Leonardo Victor de Knegt for statistical discussions on structural zeroes and random effects.
Conflict of interest
The authors declare that the research was conducted in the absence of any commercial or financial relationships that could be construed as a potential conflict of interest.
Publisher’s note
All claims expressed in this article are solely those of the authors and do not necessarily represent those of their affiliated organizations, or those of the publisher, the editors and the reviewers. Any product that may be evaluated in this article, or claim that may be made by its manufacturer, is not guaranteed or endorsed by the publisher.
Supplementary material
The Supplementary material for this article can be found online at: https://www.frontiersin.org/articles/10.3389/fmicb.2023.1108197/full#supplementary-material
SUPPLEMENTARY MATERIAL 1 | Unconstrained (left) and constrained ordination plots (right) of binary Soerensens dice distance matrix of the fecal microbiome between healthy and post weaning diarrhea (PWD) affected, at PND 8 (healthy: n = 20 pigs, PWD: n = 24 pigs), PND 27 (healthy: n = 20 pigs, PWD: n = 23 pigs), and PND 33 (healthy: n = 18 pigs, PWD: n = 25 pigs). Plots were created based on the rarefied zOTU table generated by Illumina sequencing in the V3 region. Ellipses show 80 % confidential areas assuming multivariate t-distribution. PERMANOVA was used to evaluate differences in distances at 999 permutations between the groups. Significant group difference in PWD status were found at PND 33: R2 = 0.03, p = 0.012. Additionally, an effect of body weight at PND 33 was found: R2 = 0.038, p = 0.002.
SUPPLEMENTARY MATERIAL 2 | Results from differential abundance analysis at post-natal day 8.
SUPPLEMENTARY MATERIAL 3 | Results from differential abundance analysis at post-natal day 27.
SUPPLEMENTARY MATERIAL 4 | Results from differential abundance analysis at post-natal day 33.
SUPPLEMENTARY MATERIAL 5 | Pearson’s correlation showed a positive correlation between relative abundance of genus Prevotella and body weight at PND 33: r = 0.32, 95 % confidence interval: [0.032;0.573], p = 0.03. n = 43 pigs.
SUPPLEMENTARY MATERIAL 6 | Meta data for microbiome analysis.
SUPPLEMENTARY MATERIAL 7 | zOTU table for microbiome analysis.
SUPPLEMENTARY MATERIAL 8 | Taxonomy for microbiome analysis.
SUPPLEMENTARY MATERIAL 9 | Data sheet for analysis of clinical data and microbiology.
References
Bates, D., Mächler, M., Bolker, B., and Walker, S. (2015). Fitting linear mixed-effects models using lme4. J. Stat. Softw. 67, 1–48. doi: 10.18637/jss.v067.i01
Bortolaia, V., Kaas, R. S., Ruppe, E., Roberts, M. C., Schwarz, S., Cattoir, V., et al. (2020). ResFinder 4.0 for predictions of phenotypes from genotypes. J. Antimicrob. Chemother. 75, 3491–3500. doi: 10.1093/JAC/DKAA345
Chen, J., Zhang, X., and Yang, L. (2022). GUniFrac: generalized UniFrac distances. R package version Available at: https://CRAN.R-project.org/package=GUniFrac
Clausen, P. T. L. C., Aarestrup, F. M., and Lund, O. (2018). Rapid and precise alignment of raw reads against redundant databases with KMA. BMC Bioinform. 19, 307–308. doi: 10.1186/s12859-018-2336-6
Coutellier, L., Arnould, C., Boissy, A., Orgeur, P., Prunier, A., Veissier, I., et al. (2007). Pig’s responses to repeated social regrouping and relocation during the growing-finishing period. Appl. Anim. Behav. Sci. 105, 102–114. doi: 10.1016/j.applanim.2006.05.007
Dou, S., Gadonna-Widehem, P., Rome, V., Hamoudi, D., Rhazi, L., Lakhal, L., et al. (2017). Characterisation of early-life fecal microbiota in susceptible and healthy pigs to post-weaning diarrhoea. PLoS One 12:e0169851. doi: 10.1371/journal.pone.0169851
Dubreuil, J. D., Isaacson, R. E., and Schifferli, D. M. (2016). Animal enterotoxigenic Escherichia coli. EcoSal Plus 7:1128. doi: 10.1128/ecosalplus.ESP-0006-2016
Edgar, R. C. (2016). SINTAX: a simple non-Bayesian taxonomy classifier for 16S and ITS sequences. Preprint from bioRxiv. 074161. doi: 10.1101/074161.
Edgar, R. C. (2018). Updating the 97% identity threshold for 16S ribosomal RNA OTUs. Bioinformatics 34, 2371–2375. doi: 10.1093/bioinformatics/bty113
Fouhse, J. M., Yang, K., More-Bayona, J., Gao, Y., Goruk, S., Plastow, G., et al. (2019). Neonatal exposure to amoxicillin alters long-term immune response despite transient effects on gut-microbiota in piglets. Front. Immunol. 10:2059. doi: 10.3389/FIMMU.2019.02059/BIBTEX
Frese, S. A., Parker, K., Calvert, C. C., and Mills, D. A. (2015). Diet shapes the gut microbiome of pigs during nursing and weaning. Microbiome 3:28. doi: 10.1186/s40168-015-0091-8
Frydendahl, K. (2002). Prevalence of serogroups and virulence genes in Escherichia coli associated with postweaning diarrhoea and edema disease in pigs and a comparison of diagnostic approaches. Vet. Microbiol. 85, 169–182. doi: 10.1016/S0378-1135(01)00504-1
Garnier, S., Ross, N., Rudis, R., Camargo, A.P., Sciaini, M., and Scherer, C. (2021). Rvision – colorblind-friendly color maps for R. R package version 0.6.2.
Gresse, R., Forano, E., van de Wiele, T., Blanquet-Diot, S., Fleury, M. A., and Chaucheyras-Durand, F. (2017). Gut microbiota dysbiosis in postweaning piglets: understanding the keys to health. Trends Microbiol. 25, 851–873. doi: 10.1016/j.tim.2017.05.004
Grönlund, M.-M., Grześkowiak, Ł., Isolauri, E., and Salminen, S. (2011). Gut microbes influence of mother’s intestinal microbiota on gut colonization in the infant. Gut Microbes 2, 227–233. doi: 10.4161/gmic.2.4.16799
Hampson, D. J., and Smith, W. C. (1986). Influence of creep feeding and dietary intake after weaning on malabsorption and occurrence of diarrhoea in the newly weaned pig. Res. Vet. Sci. 41, 63–69. doi: 10.1016/S0034-5288(18)30573-3
Janczyk, P., Pieper, R., Souffrant, W. B., Bimczok, D., Rothkötter, H.-J., and Smidt, H. (2007). Parenteral long-acting amoxicillin reduces intestinal bacterial community diversity in piglets even 5 weeks after the administration. ISME J. 1, 180–183. doi: 10.1038/ismej.2007.29
Jensen, P., and Recén, B. (1989). When to wean — observations from free-ranging domestic pigs. Appl. Anim. Behav. Sci. 23, 49–60. doi: 10.1016/0168-1591(89)90006-3
Joensen, K. G., Scheutz, F., Lund, O., Hasman, H., Kaas, R. S., Nielsen, E. M., et al. (2014). Real-time whole-genome sequencing for routine typing, surveillance, and outbreak detection of verotoxigenic Escherichia coli. J. Clin. Microbiol. 52, 1501–1510. doi: 10.1128/JCM.03617-13
Joensen, K. G., Tetzschner, A. M. M., Iguchi, A., Aarestrup, F. M., and Scheutz, F. (2015). Rapid and easy in silico serotyping of Escherichia coli isolates by use of whole-genome sequencing data. J. Clin. Microbiol. 53, 2410–2426. doi: 10.1128/JCM.00008-15
Karasova, D., Crhanova, M., Babak, V., Jerabek, M., Brzobohaty, L., Matesova, Z., et al. (2021). Development of piglet gut microbiota at the time of weaning influences development of postweaning diarrhea – a field study. Res. Vet. Sci. 135, 59–65. doi: 10.1016/j.rvsc.2020.12.022
Kaul, A., Mandal, S., Davidov, O., and Peddada, S. D. (2017). Analysis of microbiome data in the presence of excess zeros. Front. Microbiol. 8:2114. doi: 10.3389/FMICB.2017.02114/BIBTEX
Kuznetsova, A., Brockhoff, P. B., and Christensen, R. H. B. (2017). lmerTest package: tests in linear mixed effects models. J. Stat. Softw. 82, 1–26. doi: 10.18637/jss.v082.i13
Leite, G., Pimentel, M., Barlow, G. M., Chang, C., Hosseini, A., Wang, J., et al. (2021). Age and the aging process significantly alter the small bowel microbiome. Cell Rep. 36:109765. doi: 10.1016/j.celrep.2021.109765
Lin, H., and Peddada, S. D. (2020). Analysis of compositions of microbiomes with bias correction. Nat. Commun. 11, 3514–3511. doi: 10.1038/s41467-020-17041-7
Mach, N., Berri, M., Estellé, J., Levenez, F., Lemonnier, G., Denis, C., et al. (2015). Early-life establishment of the swine gut microbiome and impact on host phenotypes. Environ. Microbiol. Rep. 7, 554–569. doi: 10.1111/1758-2229.12285
Mandal, S., Van Treuren, W., White, R. A., Eggesbo, M., Knight, R., and Peddada, S. D. (2015). Analysis of composition of microbiomes: a novel method for studying microbial composition. Microb. Ecol. Health Dis. 11:27663. doi: 10.1038/s41467-020-17041-7
McKnite, A. M., Perez-Munoz, M. E., Lu, L., Williams, E. G., Brewer, S., Andreux, P. A., et al. (2012). Murine gut microbiota is defined by host genetics and modulates variation of metabolic traits. PLoS One 7:e39191. doi: 10.1371/JOURNAL.PONE.0039191
McMurdie, P. J., and Holmes, S. (2013). phyloseq: an R package for reproducible interactive analysis and graphics of microbiome census data. PLoS One 8:e61217. doi: 10.1371/journal.pone.0061217
Meijerink, E., Fries, R., Vögeli, P., Masabanda, J., Wigger, G., Stricker, C., et al. (1997). Two (1,2) fucosyltransferase genes on porcine chromosome 6q11 are closely linked to the blood group inhibitor (S) and Escherichia coli F18 receptor (ECF18R) loci. Mamm. Genome 8, 736–741. doi: 10.1007/s003359900556
Meijerink, E., Neuenschwander, S., Fries, R., Dinter, A., Bertschinger, H. U., Stranzinger, G., et al. (2000). A DNA polymorphism influencing alpha(1,2)fucosyltransferase activity of the pig FUT1 enzyme determines susceptibility of small intestinal epithelium to Escherichia coli F18 adhesion. Immunogenetics 52, 129–136. doi: 10.1007/s002510000263
Mulder, I. E., Schmidt, B., Stokes, C. R., Lewis, M., Bailey, M., Aminov, R. I., et al. (2009). Environmentally-acquired bacteria influence microbial diversity and natural innate immune responses at gut surfaces. BMC Biol. 7, 1–20. doi: 10.1186/1741-7007-7-79
Nearing, J. T., Douglas, G. M., Hayes, M. G., MacDonald, J., Desai, D. K., Allward, N., et al. (2022). Microbiome differential abundance methods produce different results across 38 datasets. Nat. Commun. 13, 342–316. doi: 10.1038/s41467-022-28034-z
Neuwirth, E. (2022). RColorBrewer: ColorBrewer palettes. R package version 1.1-3, Available at: https://CRAN.R-project.org/package=RColorBrewer (Accessed February 13, 2023).
Oksanen, J., Simpson, G., Blanchet, F., Kindt, R., Legendre, P., Minchin, P., et al. (2022). Vegan: community ecology package. R package version 2.6-2. Available at: https://CRAN.R-project.org/package=vegan (Accessed February 13, 2023).
Pedersen, K. S., and Toft, N. (2011). Intra- and inter-observer agreement when using a descriptive classification scale for clinical assessment of faecal consistency in growing pigs. Prev. Vet. Med. 98, 288–291. doi: 10.1016/j.prevetmed.2010.11.016
R Core Team (2022). R: A language and Environment for Statistical Computing. R Foundation for Statistical Computing, Vienna, Austria.
Ren, J., Yan, X., Ai, H., Zhang, Z., Huang, X., Ouyang, J., et al. (2012). Susceptibility towards enterotoxigenic Escherichia coli F4ac diarrhea is governed by the MUC13 gene in pigs. PLoS One 7:e44573. doi: 10.1371/JOURNAL.PONE.0044573
Rhouma, M., Fairbrother, J. M., Beaudry, F., and Letellier, A. (2017). Post weaning diarrhea in pigs: risk factors and non-colistin-based control strategies. Acta Vet. Scand. 59:31. doi: 10.1186/s13028-017-0299-7
Roubaud-Baudron, C., Ruiz, V. E., Swan, A. M. Jr., Vallance, B. A., Ozkul, C., Pei, Z., et al. (2019). Long-term effects of early-life antibiotic exposure on resistance to subsequent bacterial infection. MBio 10, e02820–e02819. doi: 10.1128/mBio.02820-19
Schmidt, B., Mulder, I. E., Musk, C. C., Aminov, R. I., Lewis, M., Stokes, C. R., et al. (2011). Establishment of normal gut microbiota is compromised under excessive hygiene conditions. PLoS One 6:e28284. doi: 10.1371/JOURNAL.PONE.0028284
Tetzschner, A. M. M., Johnson, J. R., Johnston, B. D., Lund, O., and Scheutz, F. (2020). In Silico genotyping of Escherichia coli isolates for extraintestinal virulence genes by use of whole-genome sequencing data. J. Clin. Microbiol. 58:e01269-20. doi: 10.1128/JCM.01269-20
Tomova, A., Bukovsky, I., Rembert, E., Yonas, W., Alwarith, J., Barnard, N. D., et al. (2019). The effects of vegetarian and vegan diets on gut microbiota. Front. Nutr. 6:47. doi: 10.3389/FNUT.2019.00047
Wang, M., Monaco, M. H., and Donovan, S. M. (2016). Impact of early gut microbiota on immune and metabolic development and function. Semin. Fetal Neonatal Med. 21, 380–387. doi: 10.1016/J.SINY.2016.04.004
Wickham, H., Averick, M., Bryan, J., Chang, W., McGowan, L. D., François, R., et al. (2019). Welcome to the tidyverse. J. Open Source Softw 4:1686. doi: 10.21105/joss.01686
Wilke, C. (2020). cowplot: streamlined plot theme and plot annotations for ‘ggplot2.’ R package version 0.1.1. doi: 10.5281/zenodo.2533860
Wilke, C., and Wiernik, B. (2022). Ggtext: improved text rendering support for ‘ggplot2.’ R package version 0.1.2. Available at: https://CRAN.R-project.org/package=ggtext (Accessed February 13, 2023).
Zankari, E., Allesøe, R., Joensen, K. G., Cavaco, L. M., Lund, O., and Aarestrup, F. M. (2017). PointFinder: a novel web tool for WGS-based detection of antimicrobial resistance associated with chromosomal point mutations in bacterial pathogens. J. Antimicrob. Chemother. 72, 2764–2768. doi: 10.1093/JAC/DKX217
Keywords: gastrointestinal microbiome, enterotoxigenic Escherichia coli, diarrhea, piglet, post-weaning, F18 fimbriae, high-throughput sequencing, genetic marker
Citation: Rydal MP, Gambino M, Castro-Mejia JL, Poulsen LL, Jørgensen CB and Nielsen JP (2023) Post-weaning diarrhea in pigs from a single Danish production herd was not associated with the pre-weaning fecal microbiota composition and diversity. Front. Microbiol. 14:1108197. doi: 10.3389/fmicb.2023.1108197
Edited by:
Zhenyu Zhang, University of Wisconsin-Madison, United StatesReviewed by:
Susana María Martín-Orúe, Autonomous University of Barcelona, SpainPhilippe Fravalo, Conservatoire National des Arts et Métiers (CNAM), France
John Pluske, The University of Melbourne, Australia
Elizabeth Maga, University of California, Davis, United States
Copyright © 2023 Rydal, Gambino, Castro-Mejia, Poulsen, Jørgensen and Nielsen. This is an open-access article distributed under the terms of the Creative Commons Attribution License (CC BY). The use, distribution or reproduction in other forums is permitted, provided the original author(s) and the copyright owner(s) are credited and that the original publication in this journal is cited, in accordance with accepted academic practice. No use, distribution or reproduction is permitted which does not comply with these terms.
*Correspondence: Martin Peter Rydal, martin.rydal@sund.ku.dk