- 1Department of Gastroenterology and Hepatology, Tianjin Medical University General Hospital, Tianjin, China
- 2Tianjin Institute of Digestive Disease, Tianjin Medical University General Hospital, Tianjin, China
Lactobacillus reuteri (L. reuteri), a type of Lactobacillus spp., is a gut symbiont that can colonize many mammals. Since it was first isolated in 1962, a multitude of research has been conducted to investigate its function and unique role in different diseases as an essential probiotic. Among these, the basic functions, beneficial effects, and underlying mechanisms of L. reuteri have been noticed and understood profoundly in intestinal diseases. The origins of L. reuteri strains are diverse, with humans, rats, and piglets being the most common. With numerous L. reuteri strains playing significant roles in different intestinal diseases, DSM 17938 is the most widely used in humans, especially in children. The mechanisms by which L. reuteri improves intestinal disorders include protecting the gut barrier, suppressing inflammation and the immune response, regulating the gut microbiota and its metabolism, and inhibiting oxidative stress. While a growing body of studies focused on L. reuteri, there are still many unknowns concerning its curative effects, clinical safety, and precise mechanisms. In this review, we initially interpreted the basic functions of L. reuteri and its related metabolites. Then, we comprehensively summarized its functions in different intestinal diseases, including inflammatory bowel disease, colorectal cancer, infection-associated bowel diseases, and pediatric intestinal disorders. We also highlighted some important molecules in relation to the underlying mechanisms. In conclusion, L. reuteri has the potential to exert a beneficial impact on intestinal diseases, which should be further explored to obtain better clinical application and therapeutic effects.
Introduction
According to the World Health Organization, probiotics are defined as “live microorganisms which, when administered in adequate amounts, confer a health benefit on the host” (Mu et al., 2018). These probiotics, that have already been proven to exert a beneficial impact, include Saccharomyces boulardii, Lactobacillus spp., Bifidobacterium spp., Propionibacterium spp., Streptococcus spp., Bacillus spp., Enterococcus spp., and some specific strains of Escherichia colietc, in which Lactobacillus spp. is the most widely used in human nutrition (Kechagia et al., 2013; Markowiak and Slizewska, 2017).
Recently, numerous bodies of research demonstrated that probiotics are beneficial for various diseases, such as intestinal disorders, respiratory tract infections, vaginal diseases, and so on (Markowiak and Slizewska, 2017; Nami et al., 2018). With the development of the food and drug industries and the innovation of technology, an increasing number of emerging probiotic strains were developed and applied to different fields, including natural food preservatives, nutraceuticals, and so on (Nami et al., 2015, 2018). Some novel technologies can also enhance probiotic products' quality and sensory characteristics, which can contribute to the extensive application of probiotics (Kiani et al., 2021a,b).
Safety is also an essential issue during the process of investigating probiotics. An ocean of evidence indicated that the use of probiotics can cause some risks regarding systemic infections, deleterious metabolic activities, excessive immune stimulation in susceptible individuals, gastrointestinal side effects, and so on (Doron and Snydman, 2015). Some probiotic microorganisms can even transfer resistance genes to protect against antibiotics, which may be responsible for the development of the antibiotic resistance crisis (Daniali et al., 2020). Taken together, the application of probiotics is a double-edged sword. Before probiotics are used, we still need to carry out enough clinical trials and animal experiments to assess their benefits and harms.
Lactobacillus spp., which can be found in various food products, is one of the most widely used probiotics, and includes Lactobacillus acidophilus, Lactobacillus rhamnosus, Lactobacillus bulgaricus, Lactobacillus casei, and Lactobacillus reuteri (L. reuteri) (Giraffa et al., 2010). Lactobacillus reuteri is a gut symbiont mainly colonized in the intestines of humans, rodents, pigs, and chickens (Oh et al., 2010; Walter et al., 2011; Rattanaprasert et al., 2019). Since first isolated in 1962, there have been a great number of studies conducted on L. reuteri to explore its functions and concrete mechanisms in different diseases, which cover gastrointestinal diseases, hypercholesterolemia, skin infection, allergic asthma, periodontitis, hand, foot, and mouth disease (HFMD), and so on (Prince et al., 2012; Ang et al., 2016; Giudice et al., 2016; Mu et al., 2018; Tachi et al., 2018; Theodoro et al., 2019; Wang et al., 2022). Among these, there is an increasingly prevalent trend that the investigations of L. reuteri in terms of intestinal diseases are becoming far and away the best area, which mainly concentrates on inflammatory bowel disease (IBD), colorectal cancer (CRC), children's functional bowel diseases, and so on.
Intestinal diseases, especially IBD, have become an increasing burden on the global healthcare system and society. The prevalence of IBD is expected to increase to 790 per 100,000 in 2025 (Morales et al., 2017). Of note, there will be over 1.5 million cases of IBD in China by 2025 (Kaplan, 2015). The increased use of biological therapies and the aging population will bring new challenges and complexities to public healthcare and society's economy (Kaplan, 2015). Moreover, IBD is one of the leading causes of CRC, which is the third most common cancer globally (Weitz et al., 2005; Keller et al., 2019). Although the incidence of CRC has decreased overall, it has been estimated that the incidence rates for colon and rectal cancers may increase by 90.0 and 124.2%, respectively, for patients between the ages of 20 and 34 by 2030 (Bailey et al., 2015). This phenomenon may be attributed to the fact that the pediatric overall prevalence of IBD has increased sharply (Ye et al., 2020). IBD, including ulcerative colitis (UC), and Crohn's disease (CD), is a type of chronic relapsing-remitting disease characterized by intestinal inflammation (Guo X. et al., 2021). Conventional treatment approaches include mesalazine, glucocorticoids, immunosuppressors, and so on. However, considering multiple investigations on gut microbiota and IBD (David et al., 2014; Jost et al., 2014; Thaiss et al., 2014; Ananthakrishnan, 2015), probiotics are expected to become an effective treatment for this disease. Notably, there is an abundance of studies on the fact that L. reuteri exhibits the following beneficial capacities: anti-inflammation, immune regulation, gut micro-ecology balance, gut barrier protection, metabolic control, and so on (Mu et al., 2018).
In this study, we comprehensively reviewed the literature concerning L. reuteri and its metabolites in the pathogenesis of several common intestinal diseases, which are illustrated in a separate section for a clearer understanding. Some basic introductions and future perspectives are also discussed in this review (Tables 1–3).
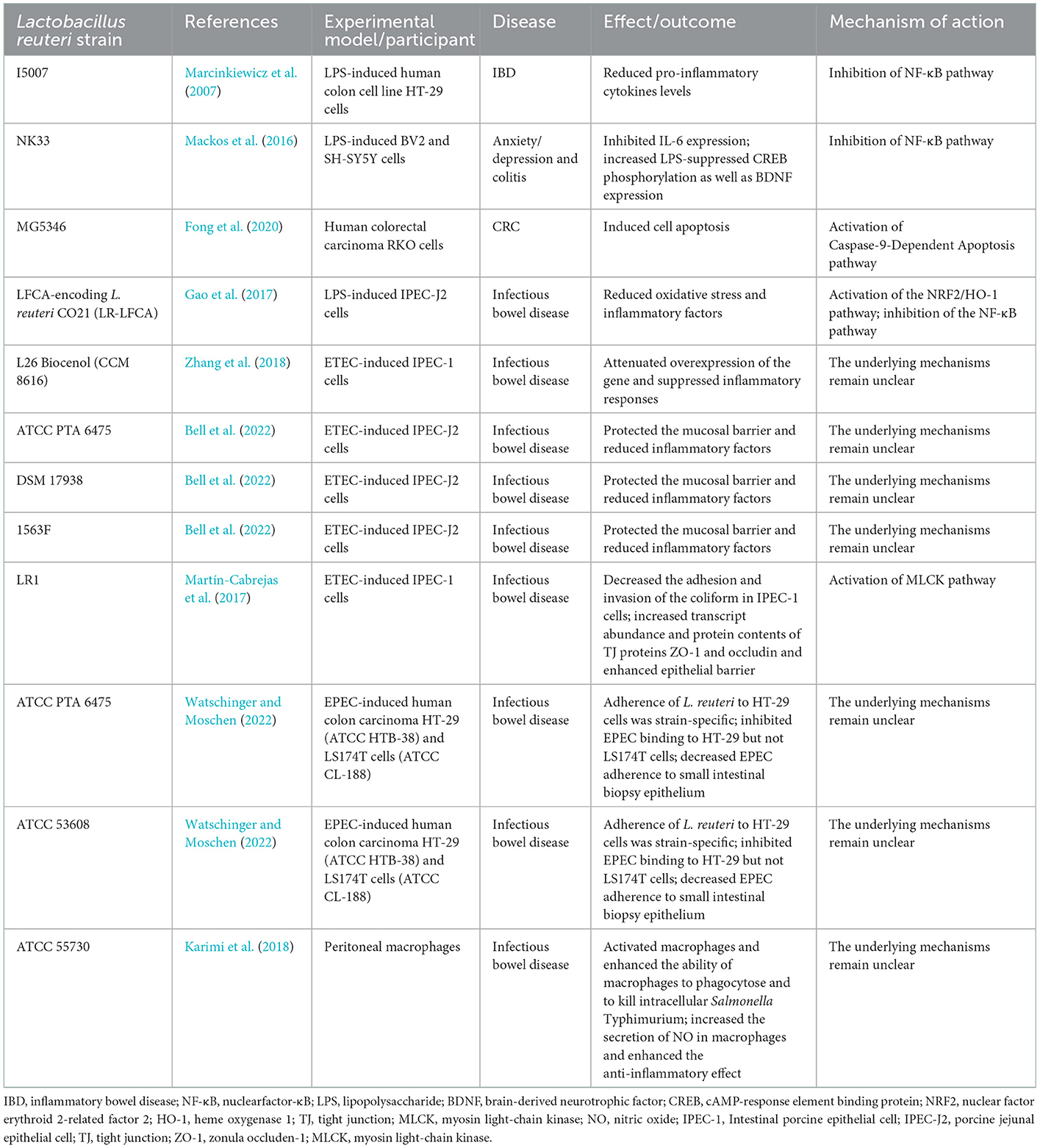
Table 1. Therapeutic efficacy and potential mechanisms of Lactobacillus reuteri strains in various intestinal diseases in cell models.
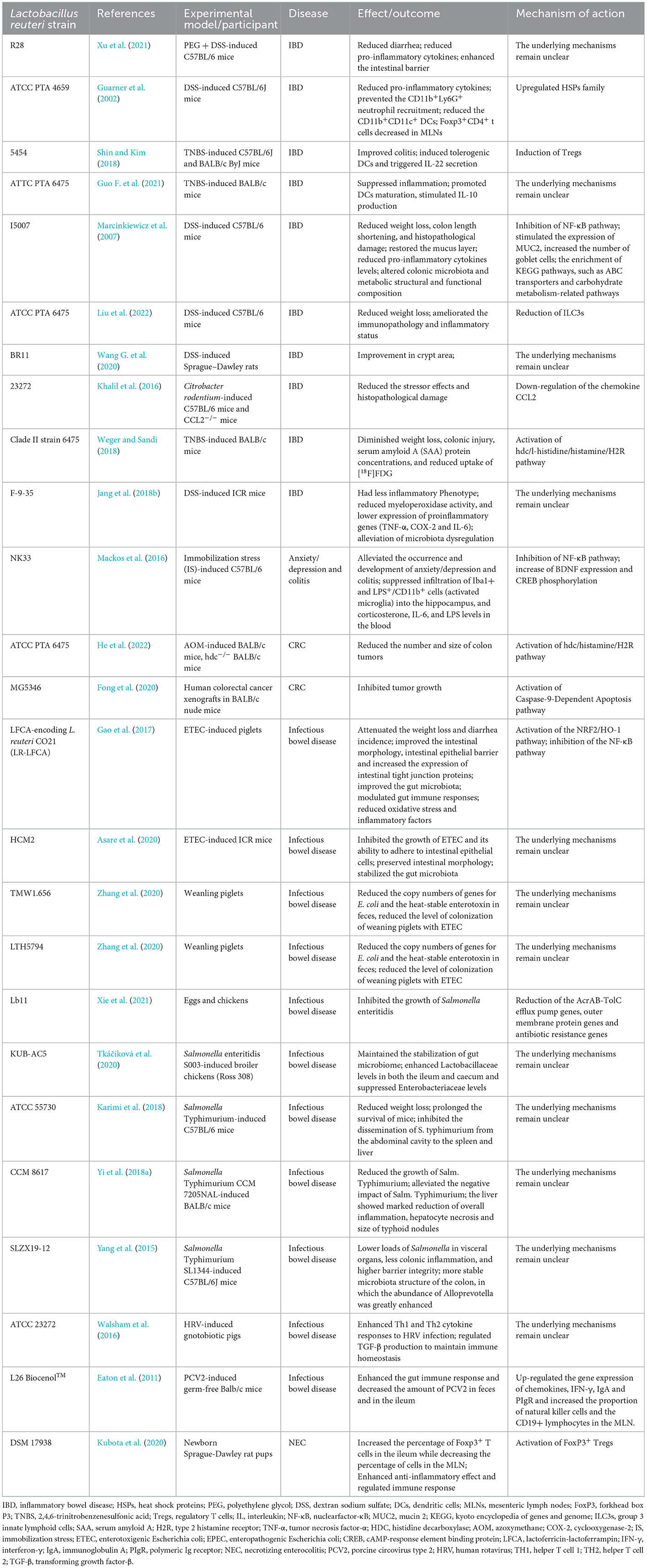
Table 2. Therapeutic efficacy and potential mechanisms of Lactobacillus reuteri strains in various intestinal diseases in animal models.
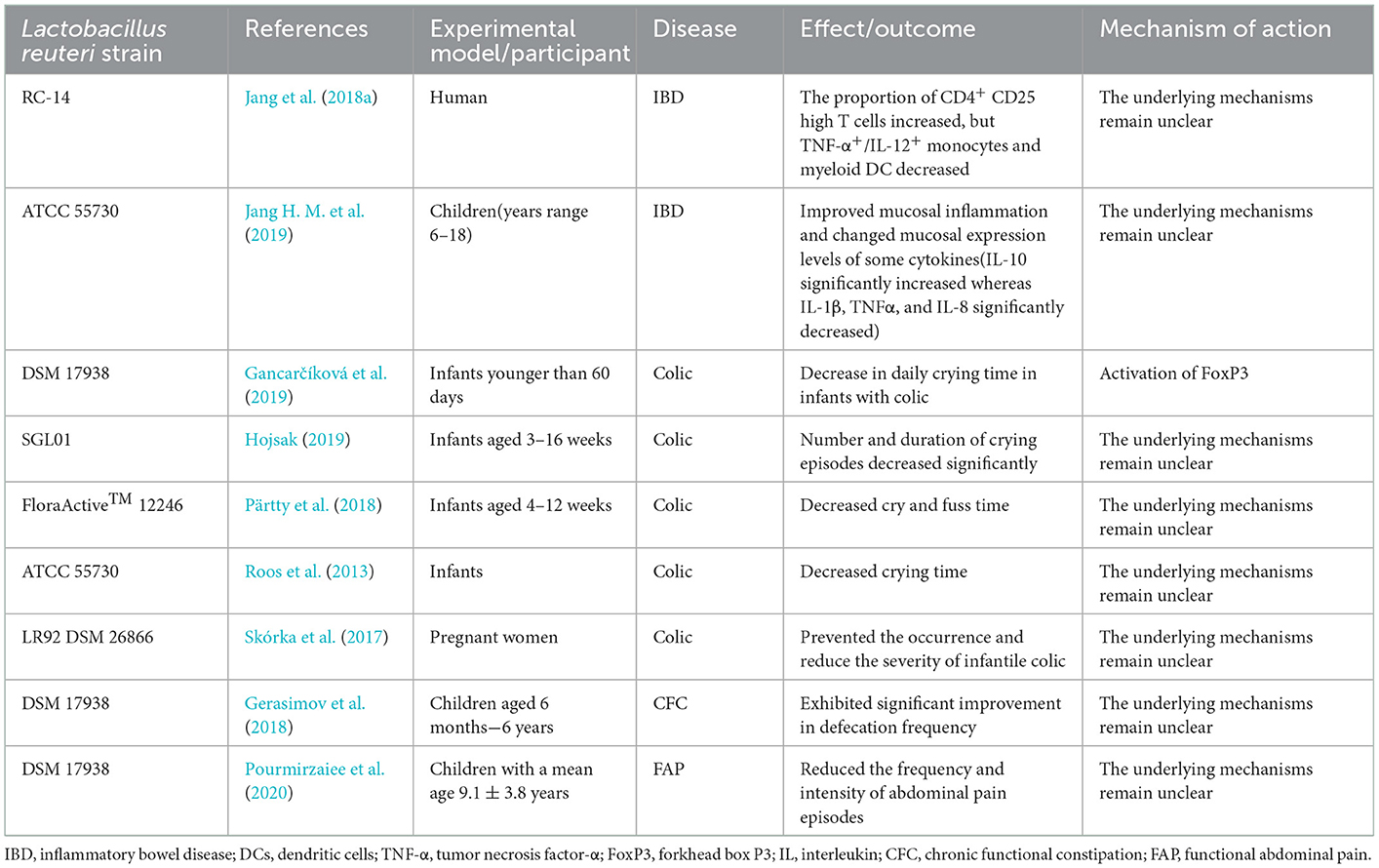
Table 3. Therapeutic efficacy and potential mechanisms of Lactobacillus reuteri strains in various intestinal diseases in humans.
The basic function of L. reuteri and its metabolites
According to incomplete statistics, L. reuteri consists of dozens of strains that originated from different samples. In addition, each stain and its unique metabolite may play a distinct role in various intestinal diseases. It is well documented that L. reuteri plays multifaceted roles in regulating immune responses, modulating gut microbiota, boosting beneficial metabolites, protecting against oxidative stress, maintaining intestinal barrier (IEB) function and intestinal morphology, and so on (Yi et al., 2018b; Liu et al., 2019; Garg et al., 2020; Singh et al., 2021). In this study, we will list some representative strains to introduce concrete pathogenesis.
It is well established that histamine is beneficial to the intestine, whose synthesis and secretion require l-histidine decarboxylase and a l-histidine/histamine exchanger (Hemarajata et al., 2013; Spinler et al., 2014). The current study identified chloride channel (ClC)-family proton/chloride antiporters as a modulator in the process of histamine production (Hall et al., 2019). Lactobacillus reuteri 6475 is special among gut microbes due to it containing a complete chromosomal histidine decarboxylase (hdc) gene cluster (genes hdcA, hdcB, and hdcP) and thus having the genetic capacity to convert histidine to histamine (Spinler et al., 2014). Lactobacillus reuteri-derived histamine can suppress gut inflammation by activating type 2 histamine receptors (H2R) and restricting pro-inflammatory H1R (Schreiber et al., 2009; Preidis et al., 2012). One of the mechanisms can be attributed to the function of a soluble bacterial enzyme named diacylglycerol kinase (Dgk), secreted by the L. reuteri strain, which can diminish Protein Kinase C (PKC) phosphorylation and suppress the H1R signaling pathway in the intestinal epithelium (Ganesh et al., 2018). Lactobacillus reuteri 6475 was also related to folate metabolism, which was mediated by dihydrofolate synthase/folylpolyglutamate synthase type 2 (folC2). Notably, the folC2 mutant can yield diminished hdc gene cluster expression and thus reduced histamine production, hinting at a link between folate and histadine/histamine metabolism (Thomas et al., 2016). Tryptophan (Trp) metabolism is also essential for gut immune homeostasis. The Trp metabolites from L. reuteri are known as aryl hydrocarbon receptor (AhR) ligand—indole-3-aldehydes, which can contribute to activating the AhR-IL-22 axis and maintaining intestinal homeostasis (Zelante et al., 2013). Another experiment also disclosed that indole-activated AhR could reprogram intraepithelial CD4+ T cells into immunoregulatory T cells to perform regulatory functions (Cervantes-Barragan et al., 2017). Özçam et al. (2019) creatively identified a novel pathway by which L. reuteri activates AhR, which is independent of Trp metabolism, known as polyketide synthase (PKS) gene clusters in L. reuteri 2010 and R2lc.
As is well-known, the adhesive ability is significant for bacterial function in the intestines of the host. In vitro experiments showed that L. reuteri has the potential to enhance adhesion in HT-29 cells (Dudík et al., 2020). Given this, Gao et al. (2016) assessed the modulatory effects of two strains of L. reuteri—ZJ617 and ZJ615, with different adhesive abilities in in vivo experiments. Finally, the authors indicated that both of them can exert anti-inflammatory and anti-oxidative effects, plus metabolism regulation, including glucose and its derivatives, galactose, amino acid metabolism, biosynthesis of antibiotics, and mineral absorption (Gao et al., 2016). Furthermore, the glyceraldehyde-3-phosphate dehydrogenase (GAPDH) protein of L. reuteri ZJ617 has been proven to work as an essential adhesion component in binding to the intestinal epithelial cells (Yang et al., 2020). In a harsh context, L. reuteri SH23 still retained its adhesive ability with the help of the Mub protein (Xu et al., 2021).
Maintaining the functions of the intestinal epithelium is a key point in protecting against bowel diseases. It was demonstrated that L. reuteri D8 has the ability to restore the epithelial damage caused by TNF by activating the Wnt/β-catenin pathway, thus stimulating the proliferation of the intestine, increasing the number of Paneth cells and increasing the expression of antimicrobial peptides (Wu et al., 2020). Lactobacillus reuteri 22 also was capable of promoting intestinal stem cell differentiation into goblet cells with increased mucin 2 (Muc-2) expression to ensure the functionality of the intestinal mucosal barrier (Xie et al., 2019). microRNAs (miRNAs) functioned as an agent during the anti-inflammatory course of L. reuteri I5007. It was able to maintain intestinal epithelial function by changing the miRNA expression of piglets, especially the PI3K-Akt and MAPK pathways, modulated by different signaling pathways (Wang Q. et al., 2020).
Association between L. reuteri and inflammatory bowel disease
With the acceleration of industrialization and changes in diet, IBD has become an emerging global disease, the incidence of which has risen considerably over the past several decades, both in the Western world and in developing countries (Kaplan, 2015). It is common knowledge that the gut microbiota's roles in the development and course of IBD are significant and enlightening. Given this, probiotics have become a hot topic both in the fundamental research and clinical practice of IBD (Martyniak et al., 2021). Research on L. reuteri is thus increasingly important.
Research on L. reuteri in fundamental fields of IBD
Fundamental study of the relationship between IBD and L. reuteri can be traced back to 2002. In that study, researchers initially found that a colonized L. reuteri strain can prevent the development of colitis in genetically susceptible mice (Guarner et al., 2002). One common feature of IBD is the disruption of the intestinal barrier and, subsequently, an uncontrollable inflammatory signal cascade (Shin and Kim, 2018; Guo F. et al., 2021). Consequently, a collection of studies focused on these two aspects. With the help of the 5(6)-carboxyfluorescein diacetate N-succinimidyl ester (cFDA-SE) labeling technique, Wang et al. (2021) found that endogenous L. reuteri R28, isolated from mouse feces, can, significantly ameliorate diarrhea caused by polyethylene glycol (PEG) through its superior colonization in the intestinal environment, regulate the expression of pro-inflammatory factors in mice with colitis induced by PEG + dextran sulfate sodium (DSS), and enhance the intestinal barrier. Marcinkiewicz et al. (2007) used a chronic active colitis animal model to explore the function of Lactobacillus strains. Among these, L. reuteri was also found to perform anti-inflammatory activities. Macrophages play a key role in the establishment of chronic intestinal inflammation observed in IBD (Heinsbroek and Gordon, 2009). It is well-documented that macrophages have two phenotypes: pro-inflammatory M1-like and anti-inflammatory M2-like. Evidence suggests that GroEL purified from L. reuteri can promote macrophage switching to M2-like polarization from the M1-like phenotype to present its anti-inflammatory properties through the Toll-like receptor (TLR) 4 and the non-canonical pathway (Dias et al., 2021). The previous study by Liu et al. (2021) demonstrated that L. reuteri can strengthen the intestinal barrier by regulating the expression level of tight junction (TJ) protein and thus protect against colitis in mice. Further, the authors found that L. reuteri ATCC PTA 4659 plays an essential role in the anti-inflammatory effect and the related immune reactions by reducing the number of CD11b+CD11c+ dendritic cells (DCs) and regulating the function of mesenteric lymph nodes (MLNs) (Liu et al., 2022). Similarly, L. reuteri 5454 and ATTC PTA 6475 have anti-inflammatory and anti-infectious capacities in 2,4,6-trinitrobenzene sulfonic acid (TNBS)-induced acute colitis in mice by promoting DC maturation, stimulating IL-10 production, and inducing the differentiation of Treg cells and Th17 cells (Hrdý et al., 2020; Engevik et al., 2021).
In addition to having anti-inflammatory effects, L. reuteri can also modulate gut microbiota and metabolic disorders in colitis in mice. Wang G. et al. (2020) first isolated L. reuteri I5007 from healthy weanling piglets and subsequently examined the effects of L. reuteri I5007 in suppressing colonic inflammation, improving colonic microbiota composition, and regulating the metabolic pathways through in vivo and in vitro models (Hou et al., 2014). In in vivo experiments, pretreatment with L. reuteri I5007 for 1 week can effectively decelerate DSS-induced weight loss, minimize the reduction in colon length, restore the function of goblet cells, and reduce the production of cytokines. Moreover, it was also beneficial to microbiota and metabolite composition. In vitro, the pretreatment of this strain was able to reduce the expression level of IL-1β and TNF-α in HT-29 cells challenged with lipopolysaccharides (LPS) for 4 h. In addition, the authors also revealed that L. reuteri treatment improves the DSS-disrupted gut microbial ecology, especially in the colon.
Inflammatory bowel disease is known to be a chronic inflammatory-immune disease, stimulating the exploration of immune-related mechanisms regulated by probiotics, in which L. reuteri can play an important role. It is currently widely known that immune checkpoint blockade (ICB) immunotherapy has become a promising cancer treatment (Postow et al., 2015; Khalil et al., 2016). However, it can also have some serious side effects, of which ICB-associated colitis is one of the most common complications (Michot et al., 2016). In the animal experiment of Wang et al. (2019) the authors finally concluded that direct administration of L. reuteri ATCC PTA 6475 can facilitate the immunopathology of ICB colitis and, inspiringly, does not exert an impact on the antitumor immunity of ICB by means of significantly decreasing the numbers of mucosal group 3 innate lymphoid cells (ILC3s) and the expression of IL17 and IL23. Nevertheless, the authors did not elaborate on the further mechanism for how L. reuteri lowered the numbers of ILC3s.
Some studies suggested that probiotic therapy can attenuate oxidative stress in rats, which is one of the main factors aggravating intestinal injury in IBD (Damiani et al., 2007; Sengül, 2011). In the experiment of Haydn et al., the authors concluded that neither wild-type BR11 nor a CyuC-deficient strain of L. reuteri could prevent the development of experimental colitis in rats. Hence, the authors stated that L. reuteri BR11 has the ability to reduce the severity of experimental IBD, owing to its unique antioxidant properties and cysteine/cystine-transport system (Turner et al., 1999; Atkins et al., 2012). However, in this study, researchers only explored the relationship between the cystine-uptake system and L. reuteri BR11. The further mechanism still requires investigation. Lactobacillus reuteri 23272 can also attenuate the effects of stressor exposure on pathogen-induced colitis by downregulating the chemokine CCL2, which was proven to be indispensable in Citrobacter rodentium-induced colitis (Mackos et al., 2016).
Anxiety disorder is a common disorder and can progress to depression, which has become a global disease (Baxter et al., 2014; Bandelow and Michaelis, 2015; Weger and Sandi, 2018). A systematic review concluded that patients with IBD have an approximately 20% prevalence rate of anxiety and a 15% prevalence rate of depression (Neuendorf et al., 2016). An ocean of research demonstrated that probiotics have the ability to reduce depression, accompanied by a series of mechanisms, in which L. reuteri was found to exert an anti-depressive impact on mice (Davis et al., 2016; Jang et al., 2018a,b). Based on the above, Jang H. M. et al. (2019) investigated the preventive and curative effects of L. reuteri NK33, isolated from healthy human feces, on immobilization stress (IS)-induced anxiety/depression and colitis in mice. The findings can be divided into two major parts: (1) L. reuteri NK33 exhibited an anti-inflammatory effect by inhibiting the expression of IL-6 and the activation NF-κB pathways in LPS-treated BV-2 cells. Further, L. reuteri NK33 could improve intestinal inflammation by restricting the expression of pro-inflammatory cytokines and the infiltration of inflammatory cells and enhancing the abundance of gut microbiota, such as Bacteroidetes, Firmicutes, and Actinobacteria in mice. (2) The treatment with L. reuteri NK33 can activate microglial cell infiltration into the hippocampus and induce hippocampal brain-derived neurotrophic factor (BDNF) expression and cAMP-response element binding protein (CREB) phosphorylation in IS-exposed mice as well as LPS-stimulated SH-SY5Y cells by suppressing the activation of the NF-κB pathway and HPA axis, thus suggesting that NK33 alleviated the suppression of NF-κB-mediated BDNF expression in the hippocampus, with the regulation of LPS infiltration into the brain, leading to the attenuation of anxiety and depression. At the same time, this research also uncovered the synergy between L. reuteri NK33 and Bifidobacterium adolescentis NK98.
Some vital molecules also play indispensable roles in treating IBD using L. reuteri, which deserve expounding. Heat shock proteins (HSPs), a type of highly conserved molecular chaperone, work as gatekeepers for intracellular proteins to maintain cell homeostasis (Liu et al., 2014a; Gupta et al., 2017). HSPs, activated by TJ protein, are vital in protecting the gut epithelium against oxidative stress and inflammation, regulating the immune response, and modulating bacterial functions (Liu et al., 2014a, 2022). The research of Liu H-Y. et al. also found that pretreatment with L. reuteri ATCC PTA 4659 can enhance the expression of two inducible HSPs, i.e., HSP7 and HSP25, in the distal colon of mice, at mRNA and/or protein levels, by increasing the mean fluorescence intensity (MFI) of HSP70 and HSP25 in both surface mucosa and the crypt as well as expanding their distribution when compared with the control animals. Furthermore, in the colon, the crypt HSP25 expression was negatively correlated with the bacterial load and the Ki67+ cell number (Liu et al., 2022).
Nevertheless, this study only revealed the expression of HSPs after treatment with L. reuteri without elucidating concrete mechanisms. Histamine, the vital molecular in histidine metabolism, played an essential role in the TNBS-induced mouse colitis model. Gao et al. (2017) demonstrated that hdc+ L. reuteri clade II strain 6475, isolated from breast milk, attenuates colonic inflammation through the activation of the histidine decarboxylase (hdc) gene and the histamine H2 receptor (H2R) and supplementation of dietary L-histidine (Gao et al., 2015). Regarding this topic, Hemarajata et al. (2013) reported that the L. reuteri-specific immunoregulatory (rsiR) gene, which originated from gene expression profiles of L. reuteri ATCC PTA 6475, is essential for TNF suppression and hdc gene expression. Further, the TNBS mouse model lacking the rsiR gene fails to exhibit anti-inflammatory effects (Hemarajata et al., 2013). MiR-142a-3p, a type of microRNAs (miRNAs), was found to alleviate colitis by promoting the growth of L. reuteri and its metabolite, further affecting the expression of inflammatory genes in intestinal epithelial cells (He et al., 2022) (Figure 1).
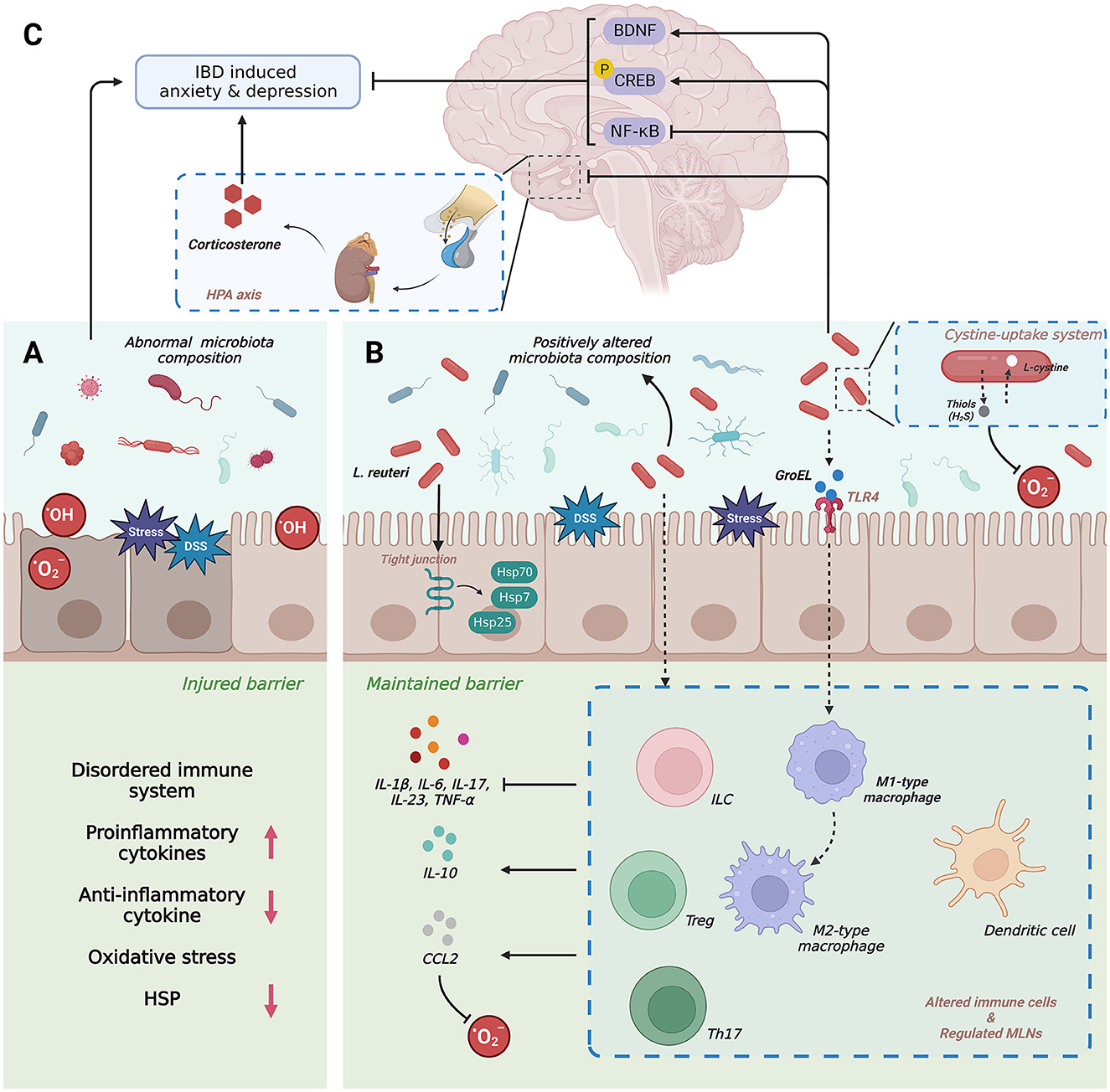
Figure 1. (A–C) Schematic diagram depicting the pathogenic role and therapeutic potential of Lactobacillus reuteri in IBD. IBD, inflammatory bowel disease; BDNF, brain-derived neurotrophic factor; CREB, cAMP-response element binding protein; DSS, dextran sodium sulphate; HSPs, heat shock proteins; NF-κB, nuclearfactor-κB; IL, interlukin; TNF-α, tumor necrosis factor-κ; CCL, C-C motif chemokine 2; Th, T helper cell; TLR, Toll-like receptors; ILC, innate lymphoid cells; Treg, regulatory T cell; MLN, mesenteric lymph node.
The clinical applications of L. reuteri in IBD
Diet therapy has always been a focus in the treatment of IBD. In the study of Kim et al. (2020) the conclusion indicated that mango intake significantly reduced biomarkers of inflammation and modulated the intestinal microbiota, which significantly increased the abundance of L. reuteri (Kim et al., 2020). The experiment by Sun et al. (2018) also supported this idea. The authors found that the space flight–induced mutant L. reuteri F-9-35 has excellent potential for the prevention of UC as a dietary supplement compared with that of the wild type and milk alone (Sun et al., 2018). With the maturity of the probiotics industry, probiotics have also been added to the daily diet. For instance, one prospective study used L. reuteri RC-14–supplemented yogurt as atherapy and finally confirmed its anti-inflammatory effect on patients with IBD (Lorea Baroja et al., 2007). As for children with active distal UC, a randomized clinical trial showed the effectiveness of L. reuteri ATCC 55730 in improving mucosal inflammation and reducing the expression level of some iconic cytokines (Oliva et al., 2012).
Nevertheless, the number of clinical research studies on L. reuteri in IBD is less than that of fundamental studies. We attribute this phenomenon to the speculation that L. reuteri is composed of multiple stains. Each stain may have different functions that must be fully investigated in fundamental experiments.
Colorectal cancer
Colorectal cancer (CRC) ranks as the third most common type of cancer and the fourth leading cause of cancer-related deaths globally (Weitz et al., 2005). It is well-known that several risk factors have been associated with the occurrence and development of CRC, such as inflammatory bowel disease (IBD), age, and genetic, and environmental factors (Keller et al., 2019; Thanikachalam and Khan, 2019). Probiotic therapy has become a hotspot treatment for CRC (Ambalam et al., 2016; Fong et al., 2020). Researchers demonstrated that L. reuteri ATCC PTA 6475 had the ability to reduce the number and size of colon tumors, with the mechanism that administration of hdc+ L. reuteri led to hdc gene expression and histamine production in the gut to suppress chronic intestinal inflammation and colorectal tumorigenesis (Gao et al., 2017). In addition, the interaction between Sirt3 and L. reuteri was proven to be crucial in gut tumorigenesis (Zhang et al., 2018). The research conducted by Bell et al. (2022) acquired some convincing results. In vitro, the authors found fecal metabolites from wild-type mice and normal humans. Both can inhibit the proliferation of CRC cell lines but not repress the noncancerous cell line NCM460. Metabolomics finally identified reuterin as the most inhibitory compound. According to a previous study, we learned that reuterin was an antimicrobial produced by L. reuteri—an intermediate in glycerol metabolism to 1,3-Propanediol (Martín-Cabrejas et al., 2017; Asare et al., 2020; Zhang et al., 2020). In addition, reuterin at a dose of 25 μM could inhibit the growth of CRC cell lines (HCT116, SW480, RKO, and DLD1), but a higher concentration of reuterin (100 μM) had no effect on normal colon epithelial cells. In vivo, the authors concluded that L. reuteri was reduced in tumors compared with normal tissues.
Moreover, the authors confirmed this result by using public datasets and patient tissue samples. Based on metabolomics and gene-enriched analysis, it was found that L. reuteri growth was suppressed by the homocysteine degradative metabolites hydrogen sulfide and cystathionine. Lactobacillus reuteri growth could not be altered by supplementing with the antioxidant glutathione ethyl ester, which indicated that the oxidative stress pathway was not specific to L. reuteri. In a bid to explore the concrete mechanism, the authors treated an intestinal cell line with 100 μM reuterin for 24 h. With the help of metabolomics, transcriptomics, and proteomics, the authors observed upregulation of the nuclear factor erythroid 2–related factor 2 (NRF2), which played an essential role in the oxidative stress response. The most enriched pathway focuses on glutathione and glutamate metabolism. Quantification of oxidized L-glutathione confirmed the role reuterin played in oxidative stress. With the subsequent observation that acetylcysteine (NAC) inhibited the induction of NRF2-dependent oxidative stress genes, it was strongly confirmed that reuterin directly controls the redox balance of a cell in a glutathione (GSH)-dependent manner. Creatively, researchers found that sodium sulfide protected cells against reuterin-induced growth inhibition, along with the result that reuterin selectively bound to cysteine residues in numerous biological replicates, indicating the significantly different cysteine proteomics profile of reuterin. The NAD pathway was also involved in the oxidative stress process. Using RNA-sequencing analysis, the puromycin incorporation assay (SUnSET), and a cell inhibition experiment, the authors finally identified the inhibiting ribosomal assembly as an essential cytotoxic pathway of reuterin, in which YEATS2 target genes were found to be downregulated after treatment with reuterin.
Taken together, reuterin was capable of repressing colorectal cancer growth in vivo. This excellent study opened new avenues for researchers. Christina Watschinger and Alexander R. Moschen expressed their distinct opinions on this comprehensive research (Watschinger and Moschen, 2022). The authors further desired to determine how reuterin's selectivity is mediated and the mechanism by which reuterin accumulates in tumor cells outside the gut. All of these outstanding findings can contribute to enhancing the transformation from basic research to clinical application. Along with the experiments on living bacteria and their secrets, Kim et al. (2022) creatively reported the joint function of heat-killed L. reuteri MG5346 and L. casei MG4584 in human CRC. The authors ultimately demonstrated that both of these strains could play an antitumor role through the caspase-9-dependent apoptosis pathway (Figure 2).
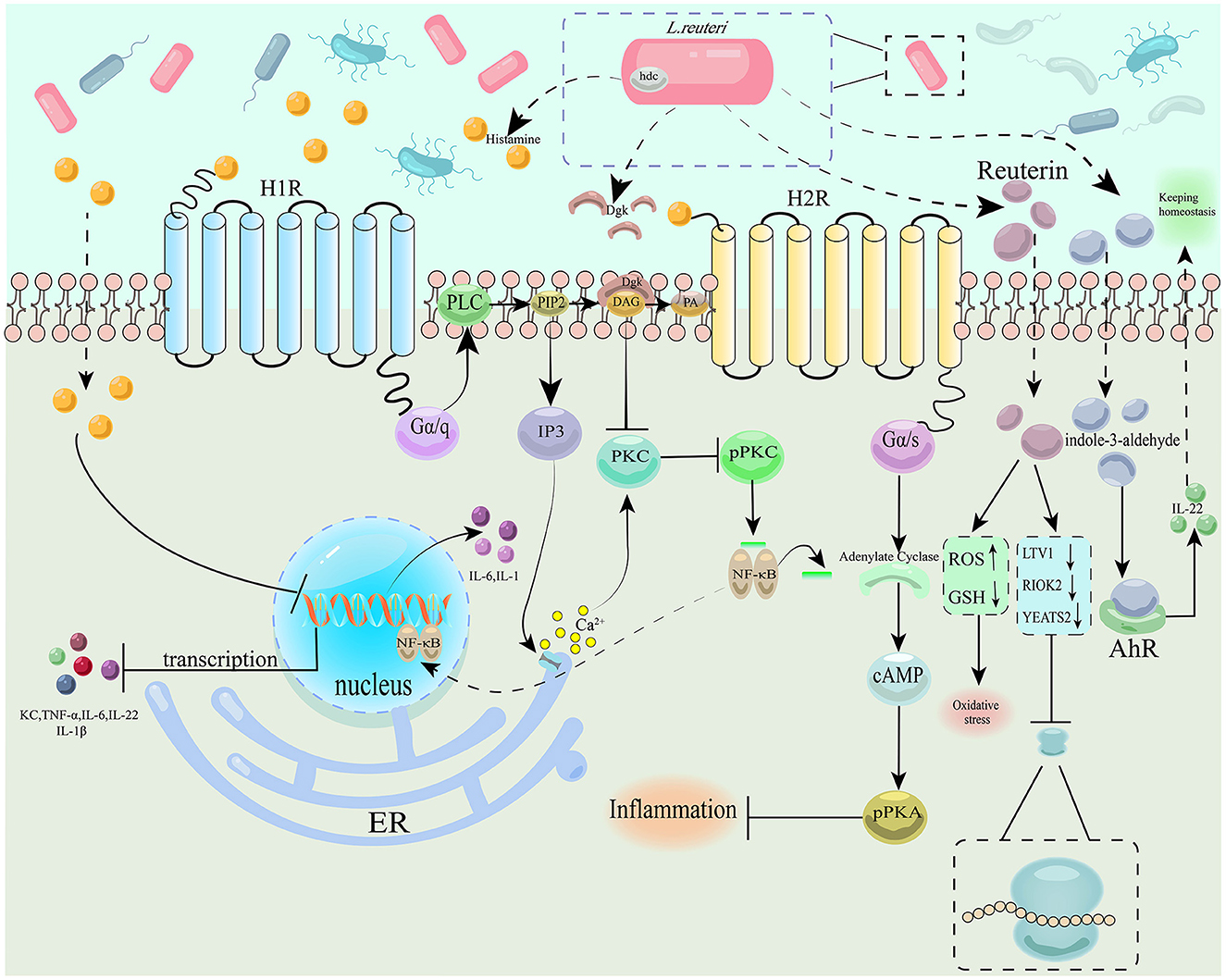
Figure 2. Schematic diagram depicting the pathogenic role and therapeutic potential of Lactobacillus reuteri in CRC. H1R, type 1 histamine receptor; H2R, type 2 histamine receptor; hdc, histidine decarboxylase; PLC, phospholipase C; PIP2, phosphatidylinositol 2; Dgk, diacylglycerol kinase; DAG, diacylglycerol; PA, phosphatidic acid; IP3, inositol triphosphate; PKC, protein kinase C; pPKC, phosphorylated protein kinase C; ER, endoplasmic reticulum; AhR, aryl hydrocarbon receptor; PKA, protein kinase A; pPKA, phosphorylated protein kinase A; KC, keratinocyte chemoattractant; ROS, reactive oxygen species; GSH, glutathione; LTV1, LTV1 ribosome biogenesis factor; RIOK2, right open reading frame kinase 2; YEATS2, YEATS domain-containing protein 2.
Infection-associated intestinal diseases
Enterotoxigenic Escherichia coli (ETEC) is a leading cause of infectious diarrhea in humans and animals. In the study of Xie et al., the authors creatively developed a new type of L. reuteri—a bovine lactoferricin-lactoferrampin (LFCA)-encoding L. reuteri CO21 (LR-LFCA) and finally demonstrated that LR-LFCA can function in the following three aspects in a newborn ETEC-infected piglet intestine model: (1) it could enhance gut immune responses by improving intestinal barrier function and gut microbiota composition, (2) it was able to protect the gut from oxidative stress by activating the NRF2/HO-1 pathway, and (3) it had the ability to inhibit the NF-κB pathway to perform its anti-inflammatory effect (Xie et al., 2021). Further, Tkáčiková et al. (2020) found that the pretreatment of L. reuteri L26 Biocenol (CCM 8616)-derived bacterial exopolysaccharides (EPSs) can attenuate the overexpression of the genes induced by ETEC infection to suppress inflammatory responses. Human-derived L. reuteri strains (ATCC PTA 6475, DSM 17938, and 1563F), a rat strain (R2LC), and piglet-derived L. reuteri (HCM2 and LR1) were also able to reduce the detrimental effect of ETEC (Karimi et al., 2018; Wang et al., 2018; Yi et al., 2018a). Reuteran and Levan, two metabolites produced by L. reuteri TMW1.656 and L. reuteri LTH5794, respectively, can also reduce the colonization of weanling piglets by ETEC (Yang et al., 2015). As for enteropathogenic E. coli (EPEC), there was evidence suggesting that L. reuteri ATCC PTA 6475 and ATCC 53608 significantly inhibited EPEC by targeting either the epithelium or the mucus layer, depending on the strain's specialty (Walsham et al., 2016). Notably, L. reuteri ATCC PTA 6475 was also effective in suppressing enterohemorrhagic E. coli (EHEC) (Eaton et al., 2011). With respect to Salmonella infections, it was demonstrated that L. reuteri Lb11, isolated from the chicken intestinal tract, can effectively prevent the formation of an efflux pump, inhibiting the production of multidrug-resistant Salmonella enteritidis in eggs (Hai and Huang, 2021). Lactobacillus reuteri KUB-AC5 can also protect against Salmonella infection in chickens (Nakphaichit et al., 2019). Jiang P. et al. (2019) revealed that L. reuteri ATCC 55730 can prevent mice from acquiring Salmonella Typhimurium by activating macrophages to produce nitric oxide. A combination of a phage cocktail and L. reuteri was able to ameliorate mouse colitis caused by S. Typhimurium by improving the intestinal barrier and colonic pathological damage, in which the metabolites of L. reuteri-acetate and reuterin played important roles (Eaton et al., 2011). Remarkably, glycerol supplementation had the ability to enhance L. reuteri ATCC PTA 6475 's protective effect against S. Typhimurium colonization (De Weirdt et al., 2012). Lactobacillus reuteri CCM 8617 and SLZX19-12 were also shown to exert vital impacts on S. Typhimurium (Gancarčíková et al., 2019; Wu et al., 2022). In regard to virus infection, some investigations have already indicated that L. reuteri ATCC 23272 functions as a significant modulator in gnotobiotic pigs infected with human rotavirus, and L. reuteri L26 Biocenol™ plays an essential role in protecting against porcine circovirus type 2 (Azevedo et al., 2012; Karaffová et al., 2017).
Pediatric intestinal diseases
Functional intestinal disorders
At present, the majority of the research for L. reuteri on functional intestinal disorders focuses on clinical research conducted on children and infants. Infantile colic, functional constipation (FC), functional abdominal pain (FAP), and irritable bowel syndrome (IBS) are the most common functional gastrointestinal disorders in children (Hojsak, 2019). In these fields, probiotics have proven to be promising therapeutic options (Pärtty et al., 2018). In 2013, a randomized DBPC trial showed no difference in microbiota between colicky infants with or without treatment using L. reuteri DSM 17938 (Roos et al., 2013). A systematic review also summarized the effects of L. reuteri ATCC 55730 and L. reuteri DSM 17938, concluding that none affected infantile colic relief (Skórka et al., 2017). However, several studies indicated that treatment with L. reuteri DSM 17938 can relieve infantile colic (Savino et al., 2018a,b, 2019; Turco et al., 2021). A combination containing heat-killed L. reuteri SGL01 and Bifidobacterium brevis SGB01 had better curative effects in infantile colic than ordinary dietary supplements (Vandenplas et al., 2017). Lactobacillus reuteri (FloraActive™) 12246, L. reuteri (American Type Culture Collection Strain 55730), and LR92 DSM 26866 all came into play in infantile colic (Savino et al., 2007; Gerasimov et al., 2018; Pourmirzaiee et al., 2020). Over 90% of child-associated constipation can be classified as FC, and some meaningful studies have been conducted in this area (Tambucci et al., 2018). Some reviews summarized the effectiveness of L. reuteri DSM 17938 in infants and children, with the conclusion that it is not recommended to use L. reuteri DSM 17938 routinely in the management of infants with constipation (Urbańska and Szajewska, 2014; Wegh et al., 2018). Similarly, some randomized controlled trials also found that L. reuteri DSM 17938 is not beneficial for the treatment of FC in children (Jadrešin et al., 2018; Wegner et al., 2018). Conversely, Kubota et al. (2020) noted a remarkable improvement in the defecation frequency with L. reuteri DSM 17938 and Magnesium Oxide in FC. Current research on FAP in children focuses on L. reuteri DSM 17938. Authors have found that L. reuteri DSM 17938 effectively alleviates pain and restores normal activities in children with FAP (Weizman et al., 2016; Maragkoudaki et al., 2017; Jadrešin et al., 2020; Trivić et al., 2021). With respect to IBS, the corresponding studies were marginal, and their findings all indicated that L. reuteri DSM 17938 is unable to improve the symptoms of IBS (Niv et al., 2005; Jadrešin et al., 2020). Another functional disorder in children, diarrhea, can also be relieved by L. reuteri DSM 17938 (Gutierrez-Castrellon et al., 2014). From the above interpretations, we can see that L. reuteri does not = have effective therapeutic results. Our point of view can be divided into two aspects: (1) this can be attributed to the limitation of sample size because nearly all studies' sample size was < 100 cases and (2) current trials are mostly centered on L. reuteri DSM 17938 and perhaps other strains would have some effects we still do not know. Thus, more trials need to be carried out in these areas.
Necrotizing enterocolitis
Necrotizing enterocolitis (NEC) is a common intestinal disease that occurs in premature infants and is the leading cause of short bowel syndrome in neonates (Neu and Walker, 2011). It is well documented that L. reuteri has become an effective treatment for this disease. Lactobacillus reuteri DSM 17938 can improve survival and reduce the incidence and severity of NEC by modulating the immune response and the induction and migration of Foxp3+ regulatory T cells (Tregs) (Liu et al., 2013). Further, this research team found that the anti-inflammatory effect of L. reuteri DSM 17938 on NEC relied on differential modulation of effector memory T cells and Foxp3+Tregs (Liu et al., 2014b). In 2018, the authors also discovered that TLR2 could play a part in alleviating NEC by means of activating DC (Hoang et al., 2018). Based on previous research results, the authors conducted their experiment on newborn mice by feeding experimental animals L. reuteri DSM 17938, concluding that oral administration of this probiotic can increase levels of tryptophan metabolites and purine nucleoside adenosine and can be beneficial to general health (Liu et al., 2019). Probiotic persistence is a major topic in probiotic therapy. Given this, Olson et al. (2018) have fully used biofilm's function to enhance the persistence of L. reuteri in the protection against NEC. Similarly, Al-Hadidi et al. (2021) also developed a new formulation of enterally delivered probiotics to improve probiotic survival through biofilm formation. Shelby et al. (2022) showed that, compared with the planktonic state of L. reuteri, its biofilm state significantly decreased the incidence of NEC through antibacterial and anti-inflammatory effects.
Conclusions and future perspectives
A growing number of studies showed that intestinal diseases can cause mounting healthcare bills and economic burdens. It is well-documented that gut microbiota plays an increasingly essential role in the treatment and prognosis of gut diseases. Lactobacillus reuteri is a common and well-studied microbe. Extensive investigations have been conducted in this area. However, we still have numerous unanswered questions. As a gut symbiont, L. reuteri can be colonized in the intestine of humans, rodents, pigs, and chickens and can perform multiple actions, including regulating immune responses, modulating gut microbiota, boosting beneficial metabolites, protecting against oxidative stress, maintaining intestinal barrier (IEB) function and intestinal morphology, and so on (Yi et al., 2018b; Liu et al., 2019; Garg et al., 2020; Singh et al., 2021). In this review, we first elucidated the basic function of L. reuteri and its related metabolites. Next, we systematically interpreted its function in different intestinal diseases, such as inflammatory bowel disease, colorectal cancer, infection-associated bowel disease, and pediatric intestinal disorders. We also emphasized some vital molecules in association with the underlying mechanisms. Cumulatively, L. reuteri is potentially beneficial to intestinal diseases, which should be further investigated in a bid to obtain better clinical application and therapeutic effects.
Although an increasing number of research studies on L. reuteri are well-studied by current researchers, there are still some key issues that are in doubt. First, there is a substantial gap between basic research and clinical applications based on the present literature data, probably owing to the unspecific mechanisms and doubtful safety of this microbe. Safety is an important issue for the wide application of probiotics. Conducting standardized safety assessments and finding effective methods to control the side effects of probiotics may be the future research direction. In addition, the paradoxical results of clinical research also restrict the development of L. reuteri, which may be attributed to the fact that L. reuteri has many distinct strains, and each strain may have its own unique function, for better or worse.
We need to fully evaluate the clinical effect of each strain and the mechanism underlying it. These issues may be addressed with the improvement of industrialized probiotics and experimental techniques. At last, the development of multiple omics analyses, especially metabonomics, allows us to investigate the functions of L. reuteri's metabolites, which may help us thoroughly investigate this field. Based on this, researchers can develop metabolites-targeted probiotic products, contributing to the refinement management of the probiotic industry.
Author contributions
ZY, JC, and YL: writing—original draft and visualization. QM, HL, QY, WS, and XR: conceptualization. XC: conceptualization and writing—review, editing, and supervision. All authors contributed to the article and approved the submitted version.
Funding
This study was supported by the National Key R&D Program of China (No. 2019YFB1311505) and the Science and Technology Program of Tianjin (No. 21JCQNJC00990).
Conflict of interest
The authors declare that the research was conducted in the absence of any commercial or financial relationships that could be construed as a potential conflict of interest.
Publisher's note
All claims expressed in this article are solely those of the authors and do not necessarily represent those of their affiliated organizations, or those of the publisher, the editors and the reviewers. Any product that may be evaluated in this article, or claim that may be made by its manufacturer, is not guaranteed or endorsed by the publisher.
References
Al-Hadidi, A., Navarro, J., Goodman, S. D., Bailey, M. T., and Besner, G. E. (2021). Lactobacillus reuteri in its biofilm state improves protection from experimental necrotizing enterocolitis. Nutrients 13, 918. doi: 10.3390/nu13030918
Ambalam, P., Raman, M., Purama, R. K., and Doble, M. (2016). Probiotics, prebiotics and colorectal cancer prevention. Best Pract. Res. Clin. Gastroenterol. 30, 119–131. doi: 10.1016/j.bpg.2016.02.009
Ananthakrishnan, A. N. (2015). Epidemiology and risk factors for IBD. Nat. Rev. Gastroenterol. Hepatol. 12, 205–217. doi: 10.1038/nrgastro.2015.34
Ang, L. Y., Too, H. K., Tan, E. L., Chow, T. K., Shek, L. P., Tham, E. H., et al. (2016). Antiviral activity of Lactobacillus reuteri protectis against coxsackievirus A and enterovirus 71 infection in human skeletal muscle and colon cell lines. Virol. J. 13, 111. doi: 10.1186/s12985-016-0567-6
Asare, P. T., Zurfluh, K., Greppi, A., Lynch, D., Schwab, C., Stephan, R., et al. (2020). Reuterin demonstrates potent antimicrobial activity against a broad panel of human and poultry meat Campylobacter spp. isolates. Microorganisms 8, 78. doi: 10.3390/microorganisms8010078
Atkins, H. L., Geier, M. S., Prisciandaro, L. D., Pattanaik, A. K., Forder, R. E. A., Turner, M. S., et al. (2012). Effects of a Lactobacillus reuteri BR11 mutant deficient in the cystine-transport system in a rat model of inflammatory bowel disease. Dig. Dis. Sci. 57, 713–719. doi: 10.1007/s10620-011-1943-0
Azevedo, M. S. P., Zhang, W., Wen, K., Gonzalez, A. M., Saif, L. J., Yousef, A. E., et al. (2012). Lactobacillus acidophilus and Lactobacillus reuteri modulate cytokine responses in gnotobiotic pigs infected with human rotavirus. Benef. Microbes. 3, 33–42. doi: 10.3920/BM2011.0041
Bailey, C. E., Hu, C.-Y., You, Y. N., Bednarski, B. K., Rodriguez-Bigas, M. A., Skibber, J. M., et al. (2015). Increasing disparities in the age-related incidences of colon and rectal cancers in the United States, 1975-2010. JAMA Surg. 150, 17–22. doi: 10.1001/jamasurg.2014.1756
Bandelow, B., and Michaelis, S. (2015). Epidemiology of anxiety disorders in the 21st century. Dialogues Clin. Neurosci. 17, 327–335. doi: 10.31887/DCNS.2015.17.3/bbandelow
Baxter, A. J., Scott, K. M., Ferrari, A. J., Norman, R. E., Vos, T., Whiteford, H. A., et al. (2014). Challenging the myth of an “epidemic” of common mental disorders: trends in the global prevalence of anxiety and depression between 1990 and 2010. Depress. Anxiety 31, 506–516. doi: 10.1002/da.22230
Bell, H. N., Rebernick, R. J., Goyert, J., Singhal, R., Kuljanin, M., Kerk, S. A., et al. (2022). Reuterin in the healthy gut microbiome suppresses colorectal cancer growth through altering redox balance. Cancer Cell 40, 185–200.e6. doi: 10.1016/j.ccell.2021.12.001
Cervantes-Barragan, L., Chai, J. N., Tianero, M. D., Di Luccia, B., Ahern, P. P., Merriman, J., et al. (2017). Lactobacillus reuteri induces gut intraepithelial CD4(+)CD8αα(+) T cells. Science 357, 806–810. doi: 10.1126/science.aah5825
Damiani, C. R., Benetton, C. A. F., Stoffel, C., Bardini, K. C., Cardoso, V. H., Di Giunta, G., et al. (2007). Oxidative stress and metabolism in animal model of colitis induced by dextran sulfate sodium. J. Gastroenterol. Hepatol. 22, 1846–1851. doi: 10.1111/j.1440-1746.2007.04890.x
Daniali, M., Nikfar, S., and Abdollahi, M. (2020). Antibiotic resistance propagation through probiotics. Expert Opin. Drug Metab. Toxicol. 16, 1207–1215. doi: 10.1080/17425255.2020.1825682
David, L. A., Maurice, C. F., Carmody, R. N., Gootenberg, D. B., Button, J. E., Wolfe, B. E., et al. (2014). Diet rapidly and reproducibly alters the human gut microbiome. Nature 505, 559–563. doi: 10.1038/nature12820
Davis, D. J., Doerr, H. M., Grzelak, A. K., Busi, S. B., Jasarevic, E., Ericsson, A. C., et al. (2016). Lactobacillus plantarum attenuates anxiety-related behavior and protects against stress-induced dysbiosis in adult zebrafish. Sci. Rep. 6, 33726. doi: 10.1038/srep33726
De Weirdt, R., Crabbé, A., Roos, S., Vollenweider, S., Lacroix, C., van Pijkeren, J. P., et al. (2012). Glycerol supplementation enhances L. reuteri's protective effect against S. Typhimurium colonization in a 3-D model of colonic epithelium. PLoS ONE 7, e37116. doi: 10.1371/journal.pone.0037116
Dias, A. M. M., Douhard, R., Hermetet, F., Regimbeau, M., Lopez, T. E., Gonzalez, D., et al. (2021). Lactobacillus stress protein GroEL prevents colonic inflammation. J. Gastroenterol. 56, 442–455. doi: 10.1007/s00535-021-01774-3
Doron, S., and Snydman, D. R. (2015). Risk and safety of probiotics. Clin. Infect. Dis. 60(Suppl 2), S129–S134. doi: 10.1093/cid/civ085
Dudík, B., Kinová Sepová, H., Bilka, F., Pašková, L., and Bilková, A. (2020). Mucin pre-cultivated Lactobacillus reuteri E shows enhanced adhesion and increases mucin expression in HT-29 cells. Antonie Van Leeuwenhoek 113, 1191–1200. doi: 10.1007/s10482-020-01426-1
Eaton, K. A., Honkala, A., Auchtung, T. A., and Britton, R. A. (2011). Probiotic Lactobacillus reuteri ameliorates disease due to enterohemorrhagic Escherichia coli in germfree mice. Infect. Immun. 79, 185–191. doi: 10.1128/IAI.00880-10
Engevik, M. A., Ruan, W., Esparza, M., Fultz, R., Shi, Z., Engevik, K. A., et al. (2021). Immunomodulation of dendritic cells by Lactobacillus reuteri surface components and metabolites. Physiol. Rep. 9, e14719. doi: 10.14814/phy2.14719
Fong, W., Li, Q., and Yu, J. (2020). Gut microbiota modulation: a novel strategy for prevention and treatment of colorectal cancer. Oncogene 39, 4925–4943. doi: 10.1038/s41388-020-1341-1
Gancarčíková, S., Nemcová, R., Popper, M., Hrčková, G., Sciranková, L., Madar, M., et al. (2019). The influence of feed-supplementation with probiotic strain Lactobacillus reuteri CCM 8617 and alginite on intestinal microenvironment of SPF mice infected with Salmonella Typhimurium CCM 7205. Probiotics Antimicrob. Proteins 11, 493–508. doi: 10.1007/s12602-018-9413-z
Ganesh, B. P., Hall, A., Ayyaswamy, S., Nelson, J. W., Fultz, R., Major, A., et al. (2018). Diacylglycerol kinase synthesized by commensal Lactobacillus reuteri diminishes protein kinase C phosphorylation and histamine-mediated signaling in the mammalian intestinal epithelium. Mucosal Immunol. 11, 380–393. doi: 10.1038/mi.2017.58
Gao, C., Ganesh, B. P., Shi, Z., Shah, R. R., Fultz, R., Major, A., et al. (2017). Gut microbe-mediated suppression of inflammation-associated colon carcinogenesis by luminal histamine production. Am. J. Pathol. 187, 2323–2336. doi: 10.1016/j.ajpath.2017.06.011
Gao, C., Major, A., Rendon, D., Lugo, M., Jackson, V., Shi, Z., et al. (2015). Histamine H2 receptor-mediated suppression of intestinal inflammation by probiotic Lactobacillus reuteri. MBio 6, e01358-15. doi: 10.1128/mBio.01358-15
Gao, K., Liu, L., Dou, X., Wang, C., Liu, J., Zhang, W., et al. (2016). Doses Lactobacillus reuteri depend on adhesive ability to modulate the intestinal immune response and metabolism in mice challenged with lipopolysaccharide. Sci. Rep. 6, 28332. doi: 10.1038/srep28332
Garg, S., Singh, T. P., and Malik, R. K. (2020). In vivo implications of potential probiotic Lactobacillus reuteri LR6 on the gut and immunological parameters as an adjuvant against protein energy malnutrition. Probiotics Antimicrob. Proteins. 12, 517–534. doi: 10.1007/s12602-019-09563-4
Gerasimov, S., Gantzel, J., Dementieva, N., Schevchenko, O., Tsitsura, O., Guta, N., et al. (2018). Role of Lactobacillus rhamnosus (FloraActive™) 19070-2 and Lactobacillus reuteri (FloraActive™) 12246 in infant colic: a randomized dietary study. Nutrients 10, 1975. doi: 10.3390/nu10121975
Giraffa, G., Chanishvili, N., and Widyastuti, Y. (2010). Importance of lactobacilli in food and feed biotechnology. Res. Microbiol. 161, 480–487. doi: 10.1016/j.resmic.2010.03.001
Giudice, M. M. D., Maiello, N., Allegorico, A., Iavarazzo, L., Capasso, M., Capristo, C., et al. (2016). Lactobacillus reuteri DSM 17938 plus vitamin D as ancillary treatment in allergic children with asthma. Ann. Allergy Asthma Immunol. 117, 710–712. doi: 10.1016/j.anai.2016.09.004
Guarner, F., Casellas, F., Borruel, N., Antolín, M., Videla, S., Vilaseca, J., et al. (2002). Role of microecology in chronic inflammatory bowel diseases. Eur. J. Clin. Nutr. 56, S34–S38. doi: 10.1038/sj.ejcn.1601662
Guo, F., Cai, D., Li, Y., Gu, H., Qu, H., Zong, Q., et al. (2021). How early-life gut microbiota alteration sets trajectories for health and inflammatory bowel disease. Front Nutr. 8, 690073. doi: 10.3389/fnut.2021.690073
Guo, X., Huang, C., Xu, J., Xu, H., Liu, L., Zhao, H., et al. (2021). Gut microbiota is a potential biomarker in inflammatory bowel disease. Front Nutr. 8, 818902. doi: 10.3389/fnut.2021.818902
Gupta, A., Chauhan, N. R., Chowdhury, D., Singh, A., Meena, R. C., Chakrabarti, A., et al. (2017). Heat stress modulated gastrointestinal barrier dysfunction: role of tight junctions and heat shock proteins. Scand. J. Gastroenterol. 52, 1315–1319. doi: 10.1080/00365521.2017.1377285
Gutierrez-Castrellon, P., Lopez-Velazquez, G., Diaz-Garcia, L., Jimenez-Gutierrez, C., Mancilla-Ramirez, J., Estevez-Jimenez, J., et al. (2014). Diarrhea in preschool children and Lactobacillus reuteri: a randomized controlled trial. Pediatrics 133, e904–e949. doi: 10.1542/peds.2013-0652
Hai, D., and Huang, X. (2021). Protective effect of Lactobacillus reuteri Lb11 from chicken intestinal tract against Salmonella enteritidis SE05 in vitro. Antonie Van Leeuwenhoek 114, 1745–1757. doi: 10.1007/s10482-021-01625-4
Hall, A. E., Engevik, M. A., Oezguen, N., Haag, A., and Versalovic, J. (2019). ClC transporter activity modulates histidine catabolism in Lactobacillus reuteri by altering intracellular pH and membrane potential. Microb. Cell Fact. 18, 212. doi: 10.1186/s12934-019-1264-0
He, L., Zhou, X., Liu, Y., Zhou, L., and Li, F. (2022). Fecal miR-142a-3p from dextran sulfate sodium-challenge recovered mice prevents colitis by promoting the growth of Lactobacillus reuteri. Mol. Ther. 30, 388–399. doi: 10.1016/j.ymthe.2021.08.025
Heinsbroek, S. E., and Gordon, S. (2009). The role of macrophages in inflammatory bowel diseases. Expert Rev. Mol. Med. 11, e14. doi: 10.1017/S1462399409001069
Hemarajata, P., Gao, C., Pflughoeft, K. J., Thomas, C. M., Saulnier, D. M., Spinler, J. K., et al. (2013). Lactobacillus reuteri-specific immunoregulatory gene rsiR modulates histamine production and immunomodulation by Lactobacillus reuteri. J. Bacteriol. 195, 5567–5576. doi: 10.1128/JB.00261-13
Hoang, T. K., He, B., Wang, T., Tran, D. Q., Rhoads, J. M., Liu, Y., et al. (2018). Protective effect of Lactobacillus reuteri DSM 17938 against experimental necrotizing enterocolitis is mediated by Toll-like receptor 2. Am. J. Physiol. Gastrointest. Liver Physiol. 315, G231–G240. doi: 10.1152/ajpgi.00084.2017
Hojsak, I. (2019). Probiotics in functional gastrointestinal disorders. Adv. Exp. Med. Biol. 1125, 121–137. doi: 10.1007/5584_2018_321
Hou, C., Wang, Q., Zeng, X., Yang, F., Zhang, J., Liu, H., et al. (2014). Complete genome sequence of Lactobacillus reuteri I5007, a probiotic strain isolated from healthy piglet. J. Biotechnol. 179, 63–64. doi: 10.1016/j.jbiotec.2014.03.019
Hrdý, J., Alard, J., Couturier-Maillard, A., Boulard, O., Boutillier, D., Delacre, M., et al. (2020). Lactobacillus reuteri 5454 and Bifidobacterium animalis ssp. lactis 5764 improve colitis while differentially impacting dendritic cells maturation and antimicrobial responses. Sci. Rep. 10, 5345. doi: 10.1038/s41598-020-62161-1
Jadrešin, O., Sila, S., Trivić, I., Mišak, Z., Hojsak, I., Kolaček, S., et al. (2018). Lack of benefit of Lactobacillus reuteri DSM 17938 as an addition to the treatment of functional constipation. J. Pediatr. Gastroenterol. Nutr. 67, 763–766. doi: 10.1097/MPG.0000000000002134
Jadrešin, O., Sila, S., Trivić, I., Mišak, Z., Kolaček, S., Hojsak, I., et al. (2020). Lactobacillus reuteri DSM 17938 is effective in the treatment of functional abdominal pain in children: results of the double-blind randomized study. Clin. Nutr. 39, 3645–3651. doi: 10.1016/j.clnu.2020.04.019
Jang, H. M., Jang, S. E., Han, M. J., and Kim, D. H. (2018a). Anxiolytic-like effect of Bifidobacterium adolescentis IM38 in mice with or without immobilisation stress. Benef. Microbes 9, 123–132. doi: 10.3920/BM2016.0226
Jang, H. M., Lee, H. J., Jang, S. E., Han, M. J., and Kim, D. H. (2018b). Evidence for interplay among antibacterial-induced gut microbiota disturbance, neuro-inflammation, and anxiety in mice. Mucosal Immunol. 11, 1386–1397. doi: 10.1038/s41385-018-0042-3
Jang, H. M., Lee, K. E., and Kim, D. H. (2019). The preventive and curative effects of Lactobacillus reuteri NK33 and Bifidobacterium adolescentis NK98 on immobilization stress-induced anxiety/depression and colitis in mice. Nutrients 11, 819. doi: 10.3390/nu11040819
Jiang, P., Yang, W., Jin, Y., Huang, H., Shi, C., Jiang, Y., et al. (2019). Lactobacillus reuteri protects mice against Salmonella Typhimurium challenge by activating macrophages to produce nitric oxide. Microb. Pathog. 137, 103754. doi: 10.1016/j.micpath.2019.103754
Jost, T., Lacroix, C., Braegger, C. P., Rochat, F., and Chassard, C. (2014). Vertical mother-neonate transfer of maternal gut bacteria via breastfeeding. Environ. Microbiol. 16, 2891–2904. doi: 10.1111/1462-2920.12238
Kaplan, G. G. (2015). The global burden of IBD: from 2015 to 2025. Nat. Rev. Gastroenterol. Hepatol. 12, 720–727. doi: 10.1038/nrgastro.2015.150
Karaffová, V., Csank, T., Mudronová, D., Király, J., Revajová, V., Gancarčíková, S., et al. (2017). Influence of Lactobacillus reuteri L26 Biocenol™ on immune response against porcine circovirus type 2 infection in germ-free mice. Benef. Microbes 8, 367–378. doi: 10.3920/BM2016.0114
Karimi, S., Jonsson, H., Lundh, T., and Roos, S. (2018). Lactobacillus reuteri strains protect epithelial barrier integrity of IPEC-J2 monolayers from the detrimental effect of enterotoxigenic Escherichia coli. Physiol. Rep. 6, e13514. doi: 10.14814/phy2.13514
Kechagia, M., Basoulis, D., Konstantopoulou, S., Dimitriadi, D., Gyftopoulou, K., Skarmoutsou, N., et al. (2013). Health benefits of probiotics: a review. ISRN Nutr. 2013, 481651. doi: 10.5402/2013/481651
Keller, D. S., Windsor, A., Cohen, R., and Chand, M. (2019). Colorectal cancer in inflammatory bowel disease: review of the evidence. Tech. Coloproctol. 23, 3–13. doi: 10.1007/s10151-019-1926-2
Khalil, D. N., Smith, E. L., Brentjens, R. J., and Wolchok, J. D. (2016). The future of cancer treatment: immunomodulation, CARs and combination immunotherapy. Nat. Rev. Clin. Oncol. 13, 273–290. doi: 10.1038/nrclinonc.2016.25
Kiani, A., Nami, Y., Hedayati, S., Elieh Ali Komi, D., Goudarzi, F., Haghshenas, B., et al. (2021b). Application of tarkhineh fermented product to produce potato chips with strong probiotic properties, high shelf-life, and desirable sensory characteristics. Front. Microbiol. 12, 657579. doi: 10.3389/fmicb.2021.657579
Kiani, A., Nami, Y., Hedayati, S., Jaymand, M., Samadian, H., Haghshenas, B., et al. (2021a). Tarkhineh as a new microencapsulation matrix improves the quality and sensory characteristics of probiotic Lactococcus lactis KUMS-T18 enriched potato chips. Sci. Rep. 11, 12599. doi: 10.1038/s41598-021-92095-1
Kim, H., Venancio, V. P., Fang, C., Dupont, A. W., Talcott, S. T., Mertens-Talcott, S. U., et al. (2020). Mango (Mangifera indica L.) polyphenols reduce IL-8, GRO, and GM-SCF plasma levels and increase Lactobacillus species in a pilot study in patients with inflammatory bowel disease. Nutr. Res. 75, 85–94. doi: 10.1016/j.nutres.2020.01.002
Kim, S. J., Kang, C. H., Kim, G. H., and Cho, H. (2022). Anti-tumor effects of heat-killed L. reuteri MG5346 and L. casei MG4584 against human colorectal carcinoma through Caspase-9-dependent apoptosis in xenograft model. Microorganisms 10, 533. doi: 10.3390/microorganisms10030533
Kubota, M., Ito, K., Tomimoto, K., Kanazaki, M., Tsukiyama, K., Kubota, A., et al. (2020). Lactobacillus reuteri DSM 17938 and magnesium oxide in children with functional chronic constipation: a double-blind and randomized clinical trial. Nutrients 12, 225. doi: 10.3390/nu12010225
Liu, H., Dicksved, J., Lundh, T., and Lindberg, J. E. (2014a). Heat shock proteins: intestinal gatekeepers that are influenced by dietary components and the gut microbiota. Pathogens 3, 187–210. doi: 10.3390/pathogens3010187
Liu, H.-Y., Gu, F., Zhu, C., Yuan, L., Zhu, C., Zhu, M., et al. (2022). Epithelial heat shock proteins mediate the protective effects of limosi Lactobacillus reuteri in dextran sulfate sodium-induced colitis. Front. Immunol. 13, 865982. doi: 10.3389/fimmu.2022.865982
Liu, H. Y., Giraud, A., Seignez, C., Ahl, D., Guo, F., Sedin, F., et al. (2021). Distinct B cell subsets in Peyer's patches convey probiotic effects by limosi Lactobacillus reuteri. Microbiome 9, 198. doi: 10.1186/s40168-021-01128-4
Liu, Y., Fatheree, N. Y., Dingle, B. M., Tran, D. Q., and Rhoads, J. M. (2013). Lactobacillus reuteri DSM 17938 changes the frequency of Foxp3+ regulatory T cells in the intestine and mesenteric lymph node in experimental necrotizing enterocolitis. PLoS ONE 8, e56547. doi: 10.1371/journal.pone.0056547
Liu, Y., Tian, X., He, B., Hoang, T. K., Taylor, C. M., Blanchard, E., et al. (2019). Lactobacillus reuteri DSM 17938 feeding of healthy newborn mice regulates immune responses while modulating gut microbiota and boosting beneficial metabolites. Am. J. Physiol. Gastrointest. Liver Physiol. 317, G824–G838. doi: 10.1152/ajpgi.00107.2019
Liu, Y., Tran, D. Q., Fatheree, N. Y., and Marc Rhoads, J. (2014b). Lactobacillus reuteri DSM 17938 differentially modulates effector memory T cells and Foxp3+ regulatory T cells in a mouse model of necrotizing enterocolitis. Am. J. Physiol. Gastrointest. Liver Physiol. 307, G177–G186. doi: 10.1152/ajpgi.00038.2014
Lorea Baroja, M., Kirjavainen, P. V., Hekmat, S., and Reid, G. (2007). Anti-inflammatory effects of probiotic yogurt in inflammatory bowel disease patients. Clin. Exp. Immunol. 149, 470–479. doi: 10.1111/j.1365-2249.2007.03434.x
Mackos, A. R., Galley, J. D., Eubank, T. D., Easterling, R. S., Parry, N. M., Fox, J. G., et al. (2016). Social stress-enhanced severity of Citrobacter rodentium-induced colitis is CCL2-dependent and attenuated by probiotic Lactobacillus reuteri. Mucosal Immunol. 9, 515–526. doi: 10.1038/mi.2015.81
Maragkoudaki, M., Chouliaras, G., Orel, R., Horvath, A., Szajewska, H., Papadopoulou, A., et al. (2017). Lactobacillus reuteri DSM 17938 and a placebo both significantly reduced symptoms in children with functional abdominal pain. Acta Paediatr. 106, 1857–1862. doi: 10.1111/apa.13992
Marcinkiewicz, J., Ciszek, M., Bobek, M., Strus, M., Heczko, P. B., Kurnyta, M., et al. (2007). Differential inflammatory mediator response in vitro from murine macrophages to lactobacilli and pathogenic intestinal bacteria. Int. J. Exp. Pathol. 88, 155–164. doi: 10.1111/j.1365-2613.2007.00530.x
Markowiak, P., and Slizewska, K. (2017). Effects of probiotics, prebiotics, and synbiotics on human health. Nutrients 9, 21. doi: 10.3390/nu9091021
Martín-Cabrejas, I., Langa, S., Gaya, P., Rodríguez, E., Landete, J. M., Medina, M., et al. (2017). Optimization of reuterin production in cheese by Lactobacillus reuteri. J. Food Sci. Technol. 54, 1346–1349. doi: 10.1007/s13197-017-2563-2
Martyniak, A., Medyńska-Przeczek, A., Wedrychowicz, A., Skoczeń, S., and Tomasik, P. J. (2021). Prebiotics, probiotics, synbiotics, paraprobiotics and postbiotic compounds in IBD. Biomolecules 11, 1903. doi: 10.3390/biom11121903
Michot, J. M., Bigenwald, C., Champiat, S., Collins, M., Carbonnel, F., Postel-Vinay, S., et al. (2016). Immune-related adverse events with immune checkpoint blockade: a comprehensive review. Eur. J. Cancer 54, 139–148. doi: 10.1016/j.ejca.2015.11.016
Morales, P. E., Bucarey, J. L., and Espinosa, A. (2017). Muscle lipid metabolism: role of lipid droplets and perilipins. J. Diabetes Res. 2017, 1789395. doi: 10.1155/2017/1789395
Mu, Q., Tavella, V. J., and Luo, X. M. (2018). Role of Lactobacillus reuteri in human health and diseases. Front. Microbiol. 9, 757. doi: 10.3389/fmicb.2018.00757
Nakphaichit, M., Sobanbua, S., Siemuang, S., Vongsangnak, W., Nakayama, J., Nitisinprasert, S., et al. (2019). Protective effect of Lactobacillus reuteri KUB-AC5 against Salmonella enteritidis challenge in chickens. Benef. Microbes 10, 43–54. doi: 10.3920/BM2018.0034
Nami, Y., Haghshenas, B., Haghshenas, M., and Yari Khosroushahi, A. (2015). Antimicrobial activity and the presence of virulence factors and bacteriocin structural genes in Enterococcus faecium CM33 isolated from ewe colostrum. Front. Microbiol. 6, 782. doi: 10.3389/fmicb.2015.00782
Nami, Y., Haghshenas, B., and Yari Khosroushahi, A. (2018). Molecular identification and probiotic potential characterization of lactic acid bacteria isolated from human vaginal microbiota. Adv Pharm Bull. 8, 683–695. doi: 10.15171/apb.2018.077
Neu, J., and Walker, W. A. (2011). Necrotizing enterocolitis. N. Engl. J. Med. 364, 255–264. doi: 10.1056/NEJMra1005408
Neuendorf, R., Harding, A., Stello, N., Hanes, D., and Wahbeh, H. (2016). Depression and anxiety in patients with inflammatory bowel disease: a systematic review. J. Psychosom. Res. 87, 70–80. doi: 10.1016/j.jpsychores.2016.06.001
Niv, E., Naftali, T., Hallak, R., and Vaisman, N. (2005). The efficacy of Lactobacillus reuteri ATCC 55730 in the treatment of patients with irritable bowel syndrome–a double blind, placebo-controlled, randomized study. Clin. Nutr. 24, 925–931. doi: 10.1016/j.clnu.2005.06.001
Oh, P. L., Benson, A. K., Peterson, D. A., Patil, P. B., Moriyama, E. N., Roos, S., et al. (2010). Diversification of the gut symbiont Lactobacillus reuteri as a result of host-driven evolution. ISME J. 4, 377–387. doi: 10.1038/ismej.2009.123
Oliva, S., Di Nardo, G., Ferrari, F., Mallardo, S., Rossi, P., Patrizi, G., et al. (2012). Randomised clinical trial: the effectiveness of Lactobacillus reuteri ATCC 55730 rectal enema in children with active distal ulcerative colitis. Aliment. Pharmacol. Ther. 35, 327–334. doi: 10.1111/j.1365-2036.2011.04939.x
Olson, J. K., Navarro, J. B., Allen, J. M., McCulloh, C. J., Mashburn-Warren, L., Wang, Y., et al. (2018). An enhanced Lactobacillus reuteri biofilm formulation that increases protection against experimental necrotizing enterocolitis. Am. J. Physiol. Gastrointest. Liver Physiol. 315, G408–G419. doi: 10.1152/ajpgi.00078.2018
Özçam, M., Tocmo, R., Oh, J.-H., Afrazi, A., Mezrich, J. D., Roos, S., et al. (2019). Gut symbionts Lactobacillus reuteri R2lc and 2010 encode a polyketide synthase cluster that activates the mammalian aryl hydrocarbon receptor. Appl. Environ. Microbiol. 85, e01661-18. doi: 10.1128/AEM.01661-18
Pärtty, A., Rautava, S., and Kalliomäki, M. (2018). Probiotics on pediatric functional gastrointestinal disorders. Nutrients 10, 1836. doi: 10.3390/nu10121836
Postow, M. A., Chesney, J., Pavlick, A. C., Robert, C., Grossmann, K., McDermott, D., et al. (2015). Nivolumab and ipilimumab versus ipilimumab in untreated melanoma. N. Engl. J. Med. 372, 2006–2017. doi: 10.1056/NEJMoa1414428
Pourmirzaiee, M. A., Famouri, F., Moazeni, W., Hassanzadeh, A., and Hajihashemi, M. (2020). The efficacy of the prenatal administration of Lactobacillus reuteri LR92 DSM 26866 on the prevention of infantile colic: a randomized control trial. Eur. J. Pediatr. 179, 1619–1626. doi: 10.1007/s00431-020-03641-4
Preidis, G. A., Saulnier, D. M., Blutt, S. E., Mistretta, T.-A., Riehle, K. P., Major, A. M., et al. (2012). Host response to probiotics determined by nutritional status of rotavirus-infected neonatal mice. J. Pediatr. Gastroenterol. Nutr. 55, 299–307. doi: 10.1097/MPG.0b013e31824d2548
Prince, T., McBain, A. J., and O'Neill, C. A. (2012). Lactobacillus reuteri protects epidermal keratinocytes from Staphylococcus aureus-induced cell death by competitive exclusion. Appl. Environ. Microbiol. 78, 5119–5126. doi: 10.1128/AEM.00595-12
Rattanaprasert, M., van Pijkeren, J.-P., Ramer-Tait, A. E., Quintero, M., Kok, C. R., Walter, J., et al. (2019). Genes involved in galactooligosaccharide metabolism in Lactobacillus reuteri and their ecological role in the gastrointestinal tract. Appl. Environ. Microbiol. 85, e01788-19. doi: 10.1128/AEM.01788-19
Roos, S., Dicksved, J., Tarasco, V., Locatelli, E., Ricceri, F., Grandin, U., et al. (2013). 454 pyrosequencing analysis on faecal samples from a randomized DBPC trial of colicky infants treated with Lactobacillus reuteri DSM 17938. PLoS ONE. 8, e56710. doi: 10.1371/journal.pone.0056710
Savino, F., Galliano, I., Garro, M., Savino, A., Daprà, V., Montanari, P., et al. (2018a). Regulatory T cells and Toll-like receptor 2 and 4 mRNA expression in infants with colic treated with Lactobacillus reuteri DSM17938. Benef. Microbes 9, 917–925. doi: 10.3920/BM2017.0194
Savino, F., Galliano, I., Savino, A., Daprà, V., Montanari, P., Calvi, C., et al. (2019). Lactobacillus reuteri DSM 17938 probiotics may increase CC-chemokine receptor 7 expression in infants treated with for colic. Front Pediatr. 7, 292. doi: 10.3389/fped.2019.00292
Savino, F., Garro, M., Montanari, P., Galliano, I., and Bergallo, M. (2018b). Crying time and RORγ/FOXP3 expression in Lactobacillus reuteri DSM17938-treated infants with colic: a randomized trial. J Pediatr. 192, 171–177.e1. doi: 10.1016/j.jpeds.2017.08.062
Savino, F., Pelle, E., Palumeri, E., Oggero, R., and Miniero, R. (2007). Lactobacillus reuteri (American Type Culture Collection Strain 55730) simethicone in the treatment of infantile colic: a prospective randomized study. Pediatrics 119, e124–e130. doi: 10.1542/peds.2006-1222
Schreiber, O., Petersson, J., Phillipson, M., Perry, M., Roos, S., Holm, L., et al. (2009). Lactobacillus reuteri prevents colitis by reducing P-selectin-associated leukocyte- and platelet-endothelial cell interactions. Am. J. Physiol. Gastrointest. Liver Physiol. 296, G534–G542. doi: 10.1152/ajpgi.90470.2008
Sengül, N. (2011). Işik S, Aslim B, Uçar G, Demirbag AE. The effect of exopolysaccharide-producing probiotic strains on gut oxidative damage in experimental colitis. Dig. Dis. Sci. 56, 707–714. doi: 10.1007/s10620-010-1362-7
Shelby, R. D., Mar, P., Janzow, G. E., Mashburn-Warren, L., Tengberg, N., Navarro, J. B., et al. (2022). Antibacterial and anti-inflammatory effects of Lactobacillus reuteri in its biofilm state contribute to its beneficial effects in a rat model of experimental necrotizing enterocolitis. J. Pediatr. Surg. 57, 1382–1390. doi: 10.1016/j.jpedsurg.2021.09.001
Shin, W., and Kim, H. J. (2018). Intestinal barrier dysfunction orchestrates the onset of inflammatory host-microbiome cross-talk in a human gut inflammation-on-a-chip. Proc. Natl. Acad. Sci. USA. 115, E10539–E10547. doi: 10.1073/pnas.1810819115
Singh, T. P., Tehri, N., Kaur, G., and Malik, R. K. (2021). Cell surface and extracellular proteins of potentially probiotic Lactobacillus reuteri as an effective mediator to regulate intestinal epithelial barrier function. Arch. Microbiol. 203, 3219–3228. doi: 10.1007/s00203-021-02318-2
Skórka, A., Pieścik-Lech, M., Kołodziej, M., and Szajewska, H. (2017). To add or not to add probiotics to infant formulae? An updated systematic review. Benef. Microbes 8, 717–725. doi: 10.3920/BM2016.0233
Spinler, J. K., Sontakke, A., Hollister, E. B., Venable, S. F., Oh, P. L., Balderas, M. A., et al. (2014). From prediction to function using evolutionary genomics: human-specific ecotypes of Lactobacillus reuteri have diverse probiotic functions. Genome Biol. Evol. 6, 1772–1789. doi: 10.1093/gbe/evu137
Sun, M.-C., Zhang, F.-C., Yin, X., Cheng, B.-J., Zhao, C.-H., Wang, Y.-L., et al. (2018). Lactobacillus reuteri F-9-35 Prevents DSS-induced colitis by inhibiting proinflammatory gene expression and restoring the gut microbiota in mice. J. Food Sci. 83, 2645–2652. doi: 10.1111/1750-3841.14326
Tachi, Y., Kozuka, A., Hirai, T., Ishizu, Y., Honda, T., Kuzuya, T., et al. (2018). Impact of myosteatosis on skeletal muscle volume loss in patients with chronic liver disease. J Gastroenterol Hepatol. doi: 10.1111/jgh.14133
Tambucci, R., Quitadamo, P., Thapar, N., Zenzeri, L., Caldaro, T., Staiano, A., et al. (2018). Diagnostic tests in pediatric constipation. J. Pediatr. Gastroenterol. Nutr. 66, e89–e98. doi: 10.1097/MPG.0000000000001874
Thaiss, C. A., Zeevi, D., Levy, M., Zilberman-Schapira, G., Suez, J., Tengeler, A. C., et al. (2014). Transkingdom control of microbiota diurnal oscillations promotes metabolic homeostasis. Cell 159, 514–529. doi: 10.1016/j.cell.2014.09.048
Thanikachalam, K., and Khan, G. (2019). Colorectal cancer and nutrition. Nutrients 11, 164. doi: 10.3390/nu11010164
Theodoro, L. H., Cláudio, M. M., Nuernberg, M. A. A., Miessi, D. M. J., Batista, J. A., Duque, C., et al. (2019). Effects of Lactobacillus reuteri as an adjunct to the treatment of periodontitis in smokers: randomised clinical trial. Benef. Microbes 10, 375–384. doi: 10.3920/BM2018.0150
Thomas, C. M., Saulnier, D. M., Spinler, J. K., Hemarajata, P., Gao, C., Jones, S. E., et al. (2016). FolC2-mediated folate metabolism contributes to suppression of inflammation by probiotic Lactobacillus reuteri. Microbiol. Open 5, 802–818. doi: 10.1002/mbo3.371
Tkáčiková, L., Mochnáčová, E., Tyagi, P., Kiššová, Z., and Bhide, M. (2020). Comprehensive mapping of the cell response to E. coli infection in porcine intestinal epithelial cells pretreated with exopolysaccharide derived from Lactobacillus reuteri. Vet. Res. 51, 49. doi: 10.1186/s13567-020-00773-1
Trivić, I., Niseteo, T., Jadrešin, O., and Hojsak, I. (2021). Use of probiotics in the treatment of functional abdominal pain in children-systematic review and meta-analysis. Eur. J. Pediatr. 180, 339–351. doi: 10.1007/s00431-020-03809-y
Turco, R., Russo, M., Bruzzese, D., and Staiano, A. (2021). Efficacy of a partially hydrolysed formula, with reduced lactose content and with Lactobacillus reuteri DSM 17938 in infant colic: a double blind, randomised clinical trial. Clin. Nutr. 40, 412–419. doi: 10.1016/j.clnu.2020.05.048
Turner, M. S., Woodberry, T., Hafner, L. M., and Giffard, P. M. (1999). The bspA locus of Lactobacillus fermentum BR11 encodes an L-cystine uptake system. J. Bacteriol. 181, 2192–2198. doi: 10.1128/JB.181.7.2192-2198.1999
Urbańska, M., and Szajewska, H. (2014). The efficacy of Lactobacillus reuteri DSM 17938 in infants and children: a review of the current evidence. Eur. J. Pediatr. 173, 1327–1337. doi: 10.1007/s00431-014-2328-0
Vandenplas, Y., Bacarea, A., Marusteri, M., Bacarea, V., Constantin, M., Manolache, M., et al. (2017). Efficacy and safety of APT198K for the treatment of infantile colic: a pilot study. J. Comp. Eff. Res. 6, 137–144. doi: 10.2217/cer-2016-0059
Walsham, A. D. S., MacKenzie, D. A., Cook, V., Wemyss-Holden, S., Hews, C. L., Juge, N., et al. (2016). Lactobacillus reuteri inhibition of enteropathogenic Escherichia coli adherence to human intestinal epithelium. Front. Microbiol. 7, 244. doi: 10.3389/fmicb.2016.00244
Walter, J., Britton, R. A., and Roos, S. (2011). Host-microbial symbiosis in the vertebrate gastrointestinal tract and the Lactobacillus reuteri paradigm. Proc. Natl. Acad. Sci. USA. 108(Suppl 1), 4645–4652. doi: 10.1073/pnas.1000099107
Wang, G., Chen, Y., Fei, S., Xie, C., Xia, Y., Colonisation, A. i. L., et al. (2021). with endogenous Lactobacillus reuteri R28 and exogenous Lactobacillus plantarum AR17-1 and the effects on intestinal inflammation in mice. Food Funct. 12, 2481–2488. doi: 10.1039/D0FO02624G
Wang, G., Huang, S., Cai, S., Yu, H., Wang, Y., Zeng, X., et al. (2020). Lactobacillus reuteri ameliorates intestinal inflammation and modulates gut microbiota and metabolic disorders in dextran sulfate sodium-induced colitis in mice. Nutrients 12, 2298. doi: 10.3390/nu12082298
Wang, Q., He, Y., Li, X., Zhang, T., Liang, M., Wang, G., et al. (2022). Lactobacillus reuteri CCFM8631 alleviates hypercholesterolaemia caused by the paigen atherogenic diet by regulating the gut microbiota. Nutrients 14, 1272. doi: 10.3390/nu14061272
Wang, Q., Sun, Q., Wang, J., Qiu, X., Qi, R., Huang, J., et al. (2020). Identification of differentially expressed miRNAs after Lactobacillus reuteri treatment in the ileum mucosa of piglets. Genes Genomics 42, 1327–1338. doi: 10.1007/s13258-020-00998-6
Wang, T., Teng, K., Liu, G., Liu, Y., Zhang, J., Zhang, X., et al. (2018). Lactobacillus reuteri HCM2 protects mice against enterotoxigenic Escherichia coli through modulation of gut microbiota. Sci. Rep. 8, 17485. doi: 10.1038/s41598-018-35702-y
Wang, T., Zheng, N., Luo, Q., Jiang, L., He, B., Yuan, X., et al. (2019). Probiotics Lactobacillus reuteri abrogates immune checkpoint blockade-associated colitis by inhibiting group 3 innate lymphoid cells. Front. Immunol. 10, 1235. doi: 10.3389/fimmu.2019.01235
Watschinger, C., and Moschen, A. R. (2022). Lactobacillus reuteri-an old acquaintance takes on a new task in colorectal tumor surveillance. Cancer Cell 40, 125–127. doi: 10.1016/j.ccell.2022.01.014
Weger, M., and Sandi, C. (2018). High anxiety trait: a vulnerable phenotype for stress-induced depression. Neurosci. Biobehav. Rev. 87, 27–37. doi: 10.1016/j.neubiorev.2018.01.012
Wegh, C., Benninga, M. A., and Tabbers, M. M. (2018). Effectiveness of probiotics in children with functional abdominal pain disorders and functional constipation: a systematic review. J. Clin. Gastroenterol. 52(Suppl 1), S10–S26. doi: 10.1097/MCG.0000000000001054
Wegner, A., Banaszkiewicz, A., Kierkus, J., Landowski, P., Korlatowicz-Bilar, A., Wiecek, S., et al. (2018). The effectiveness of Lactobacillus reuteri DSM 17938 as an adjunct to macrogol in the treatment of functional constipation in children. A randomized, double-blind, placebo-controlled, multicentre trial. Clin. Res. Hepatol. Gastroenterol. 42, 494–500. doi: 10.1016/j.clinre.2018.03.008
Weitz, J., Koch, M., Debus, J., Höhler, T., Galle, P. R., Büchler, M. W., et al. (2005). Colorectal cancer. Lancet 365, 153–165. doi: 10.1016/S0140-6736(05)17706-X
Weizman, Z., Abu-Abed, J., and Binsztok, M. (2016). Lactobacillus reuteri DSM 17938 for the management of functional abdominal pain in childhood: a randomized, double-blind, placebo-controlled trial. J Pediatr. 174, 160–164.e1. doi: 10.1016/j.jpeds.2016.04.003
Wu, H., Xie, S., Miao, J., Li, Y., Wang, Z., Wang, M., et al. (2020). Lactobacillus reuteri maintains intestinal epithelial regeneration and repairs damaged intestinal mucosa. Gut Microbes 11, 997–1014. doi: 10.1080/19490976.2020.1734423
Wu, J., Lin, Z., Wang, X., Zhao, Y., Zhao, J., Liu, H., et al. (2022). Limosi Lactobacillus reuteri SLZX19-12 protects the colon from infection by enhancing stability of the gut microbiota and barrier integrity and reducing inflammation. Microbiol. Spectr. 10, e0212421. doi: 10.1128/spectrum.02124-21
Xie, S., Zhao, S., Jiang, L., Lu, L., Yang, Q., Yu, Q., et al. (2019). Lactobacillus reuteri stimulates intestinal epithelial proliferation and induces differentiation into goblet cells in young chickens. J. Agric. Food Chem. 67, 13758–13766. doi: 10.1021/acs.jafc.9b06256
Xie, W., Song, L., Wang, X., Xu, Y., Liu, Z., Zhao, D., et al. (2021). A bovine lactoferricin-lactoferrampin-encoding Lactobacillus reuteri CO21 regulates the intestinal mucosal immunity and enhances the protection of piglets against enterotoxigenic Escherichia coli K88 challenge. Gut Microbes 13, 1956281. doi: 10.1080/19490976.2021.1956281
Xu, H., Wu, L., Pan, D., Zeng, X., Cai, Z., Guo, Y., et al. (2021). Adhesion characteristics and dual transcriptomic and proteomic analysis of Lactobacillus reuteri SH23 upon gastrointestinal fluid stress. J. Proteome Res. 20, 2447–2457. doi: 10.1021/acs.jproteome.0c00933
Yang, J., Wang, C., Liu, L., and Zhang, M. (2020). Lactobacillus reuteri KT260178 supplementation reduced morbidity of piglets through its targeted colonization, improvement of cecal microbiota profile, and immune functions. Probiotics Antimicrob. Proteins 12, 194–203. doi: 10.1007/s12602-019-9514-3
Yang, Y., Galle, S., Le, M. H., Zijlstra, R. T., and Gänzle, M. G. (2015). Feed fermentation with reuteran- and levan-producing Lactobacillus reuteri reduces colonization of weanling pigs by enterotoxigenic Escherichia coli. Appl. Environ. Microbiol. 81, 5743–5752. doi: 10.1128/AEM.01525-15
Ye, Y., Manne, S., Treem, W. R., and Bennett, D. (2020). Prevalence of inflammatory bowel disease in pediatric and adult populations: recent estimates from large national databases in the United States, 2007-2016. Inflamm. Bowel Dis. 26, 619–625. doi: 10.1093/ibd/izz182
Yi, H., Wang, L., Xiong, Y., Wang, Z., Qiu, Y., Wen, X., et al. (2018a). Lactobacillus reuteri LR1 improved expression of genes of tight junction proteins via the MLCK pathway in IPEC-1 cells during infection with enterotoxigenic Escherichia coli K88. Mediators Inflamm. 2018, 6434910. doi: 10.1155/2018/6434910
Yi, H., Wang, L., Xiong, Y., Wen, X., Wang, Z., Yang, X., et al. (2018b). Effects of Lactobacillus reuteri LR1 on the growth performance, intestinal morphology, and intestinal barrier function in weaned pigs. J. Anim. Sci. 96, 2342–2351. doi: 10.1093/jas/sky129
Zelante, T., Iannitti, R. G., Cunha, C., De Luca, A., Giovannini, G., Pieraccini, G., et al. (2013). Tryptophan catabolites from microbiota engage aryl hydrocarbon receptor and balance mucosal reactivity via interleukin-22. Immunity 39, 372–385. doi: 10.1016/j.immuni.2013.08.003
Zhang, Y., Wang, X.-L., Zhou, M., Kang, C., Lang, H.-D., Chen, M.-T., et al. (2018). Crosstalk between gut microbiota and Sirtuin-3 in colonic inflammation and tumorigenesis. Exp. Mol. Med. 50, 1–11. doi: 10.1038/s12276-017-0002-0
Keywords: Lactobacillus reuteri, intestinal diseases, gut microbiota, inflammatory bowel disease, colorectal cancer
Citation: Yu Z, Chen J, Liu Y, Meng Q, Liu H, Yao Q, Song W, Ren X and Chen X (2023) The role of potential probiotic strains Lactobacillus reuteri in various intestinal diseases: New roles for an old player. Front. Microbiol. 14:1095555. doi: 10.3389/fmicb.2023.1095555
Received: 14 November 2022; Accepted: 03 January 2023;
Published: 02 February 2023.
Edited by:
Rebeca Martín, INRAE Centre Jouy-en-Josas, FranceReviewed by:
Babak Haghshenas, Kermanshah University of Medical Sciences, IranEugenia Bezirtzoglou, Democritus University of Thrace, Greece
Ali Chaari, Weill Cornell Medicine-Qatar, Qatar
Copyright © 2023 Yu, Chen, Liu, Meng, Liu, Yao, Song, Ren and Chen. This is an open-access article distributed under the terms of the Creative Commons Attribution License (CC BY). The use, distribution or reproduction in other forums is permitted, provided the original author(s) and the copyright owner(s) are credited and that the original publication in this journal is cited, in accordance with accepted academic practice. No use, distribution or reproduction is permitted which does not comply with these terms.
*Correspondence: Xin Chen, eGNoZW4wMyYjeDAwMDQwO3RtdS5lZHUuY24=
†These authors have contributed equally to this work
‡ORCID: Zihan Yu orcid.org/0000-0002-2696-2297
Xin Chen orcid.org/0000-0003-3024-9053