- Department of Orthodontics, School of Stomatology, Capital Medical University, Beijing, China
White spot lesions (WSLs) are common enamel infectious diseases in fixed orthodontic treatment, which might attribute to the dysbiosis of oral microbiome. However, the correlation of Candida albicans with oral bacteriome in WSLs still remains unrevealed. This study investigated the carriage of C. albicans and how it shaped the bacterial community in disease or healthy supragingival plaque, to explore the potential role of interkingdom interaction in orthodontic WSLs. In this study, 31 patients with WSLs (WSLs) and 23 healthy patients (Health) undergoing fixed orthodontic treatment were enrolled. The supragingival microbiota in both groups were determined using 16S rRNA gene sequencing. Colonization and abundance of C. albicans in the plaque were determined via culture-dependent and -independent methods. Among WSLs patients, the correlation of C. albicans and bacteriome was analyzed under QIIME2-based bioinformatics and Spearman’s correlation coefficient. The raw reads were deposited into the NCBI Sequence Read Archive (SRA) database (Accession Number: SRP404186). Significant differences in microbial diversity as well as composition were observed between WSLs and Health groups. Leptotrichia remarkably enriched in the WSLs group, while Neisseria and Cardiobacterium significantly enriched in the Health group. In addition, 45% of WSLs patients were C. albicans carriers but none in patients without WSLs. Among all WSLs patients, beta diversity and microbial composition were distinguished between C. albicans carriers and non-carriers. In C. albicans carriers, Corynebacterium matruchotii and Streptococcus mutans significantly enriched whereas Saccharibacteria_TM7_G-1 significantly depleted. The abundance of C. albicans was positively associated with bacteria such as Streptococcus mutans, while the negative correlation was detected between C. albicans and several bacteria such as Cardiobacterium hominis and Streptococcus sanguinis. Our study elucidated the distinguished supragingival plaque microbiome between orthodontic patients with and without WSLs. C. albicans frequently existed and enriched in orthodontic derived WSLs. The carriage of C. albicans shape plaque bacterial community in demineralized lesions and might play roles in WSLs pathogenesis.
1. Introduction
White spot lesions (WSLs) are milky white opaque areas around brackets on the labial surface of teeth in fixed orthodontics. These lesions are caused by subsurface porosity from enamel demineralization, which has a greater risk of progressing to severe cavities than sound enamel (Enaia et al., 2011). As a frequently diagnosed side-effect, WSLs have a considerably high incidence in orthodontic treatment, even reported to occur in up to 60.9% of patients (Zheng et al., 2016). It is generally believed that lesions will recover by natural remineralization after bracket removal. However, mineral re-uptake on the surface layer through saliva has little improvement on the aesthetic and structural properties of enamel. Therefore, WSLs seriously jeopardize the dental hard tissue and negatively affect patient satisfaction (Travess et al., 2004).
Multiple factors attribute to the development of WSLs, such as microorganisms, diet, and local micro-environment. Among them, microorganisms play the most important role (Tang et al., 2022). With the placement of orthodontic appliances, biofilms can easily accumulate around brackets, particularly in patients with poor oral hygiene (Badea et al., 2019). An imbalanced bacterial community can be derived from a high-carbohydrate diet, thus leading to the enrichment of acidogenic and aciduric bacteria (Valm, 2019; Da Costa Rosa et al., 2021). Cariogenic bacteria like Streptococcus mutans (S. mutans) and Leptotrichia wadei could be isolated and cultivated from the enamel lesions of WSLs patients in previous studies (Andrucioli et al., 2017; KarabekİRoĞLu et al., 2017; Lee et al., 2021).
In recent years, the profile of oral microbiome and its change in fixed orthodontics have attracted more attention due to the advancement of high-throughput techniques (Sadeq et al., 2015; Sun et al., 2018). Through microbiological analysis of saliva and supragingival plaque samples, previous studies proposed that the oral microbiota was changed during the process of orthodontic treatment, and the plaque accumulation was boosted by the orthodontic appliance (Guo et al., 2021). With the progress of treatment, some caries-related bacteria increased, but the genus generally associated with a healthy status, such as Rothia, was decreased (Koopman et al., 2015). However, most studies mainly observed the microbiome change from the start of orthodontic treatment but rarely mentioned how the microbiome changed from healthy to the occurrence of typical WSLs. The difference in microbiome characteristics in supragingival plaque from orthodontic patients with or without WSLs has not been well reported. Hence, exploring the supragingival microbiome between healthy and disease status may attribute to a better understanding of the pathogenesis of WSLs.
In addition to bacteria, fungi were much less abundant but a non-negligible kingdom in the oral microbiome, which have been widely reported in multiple oral diseases (Dağistan et al., 2009; Peleg et al., 2010; Xu et al., 2013). Candida albicans (C. albicans) could be isolated in dental caries diseases such as early childhood caries (ECC) and root caries (Valm, 2019; Chen Y. et al., 2021). In these caries lesions, C. albicans had active interkingdom interactions with bacteria like S. mutans, which synergistically contribute to the development of dental caries (Valm, 2019; Ev et al., 2020). It was reported that C. albicans had a positive correlation with S. mutans in ECC-related biofilm (Bachtiar and Bachtiar, 2018). S. mutans-secreted GTFB binds to the mannan layer of C. albicans to promote extracellular matrix formation and their co-existence within biofilms in children with ECC (Hwang et al., 2017). Previous studies have investigated the correlation between C. albicans and oral bacteriome in ECC, results also showed that C. albicans had the strongest positive correlation with S. mutans (Yang et al., 2022). Besides S. mutans, the presence of oral C. albicans was associated with a highly acidogenic and acid-tolerant bacterial community in ECC, with an increased abundance of the genera such as Streptococcus, Lactobacillus, and Scardovia (Xiao et al., 2018). In addition, C. albicans was positively correlated with several caries-associated species, such as Actinomyces sp. ICM58, Actinomyces sp. oral taxon 172, and Scardovia wiggsiae (Baraniya et al., 2020). However, to date there was no study reporting whether C. albicans would be enriched in orthodontic WSLs. How C. albicans shape bacterial communities and interact with bacteriome in the WSLs disease sites was also unrevealed.
Therefore, both C. albicans and bacterial communities were investigated in this study. Supragingival bacteriomes were distinguished in orthodontic on-going patients with or without WSLs. In addition, the prevalence of C. albicans and its association with oral bacteria was studied. Three null hypotheses were proposed: (1) there were no significant differences in supragingival plaque microbiome between orthodontic patients with and without WSLs; (2) C. albicans would not enrich in patients with WSLs; and (3) The existence of C. albicans could not shape the bacterial composition and correlation in local supragingival plaque community.
2. Materials and methods
2.1. Patient recruitment
This study was approved by the Ethical Committee of Beijing Stomatological Hospital (No. CMUSH-IRB-KJ-PJ-2022-11). The patients provided their written informed consent to participate in this study. A total of 54 patients (aged 14.25 ± 1.92 years) who underwent fixed orthodontic treatment within 6–12 months were recruited from the department of orthodontics, Beijing stomatological hospital. Patients who had WSLs in anterior teeth or premolars area evaluated by averaged enamel decalcification index (EDI) >0.12 (Robertson et al., 2011) were allocated to the “WSLs group” (n = 31). Otherwise, patients without detectable WSLs were assigned to the “Health group” (n = 23). All included subjects were periodontal healthy, with no attachment loss, probing depth (PD) ≤3 mm, and gingival index (GI) ≤1. Clinical parameters were recorded by the same well-trained dentist. Subjects were excluded from this study if they had untreated caries in the whole dentition, enamel hypoplasia, tetracycline-stained teeth, dental fluorosis, or other oral and systemic diseases. Subjects who used antibiotics or any other medications that might affect the oral microbiota in the past 3 months were also excluded.
2.2. Sample collection
Participants refrained from any type of oral hygiene for 12 h, as well as diet intake for at least 2 h before sample collection. The supragingival plaqueswere amplified from the extracted from the labial surfaces of all anterior teeth and premolars around brackets were collected by scraping them with a sterilized periodontal curette. Samples in the WSLs group were collected from the enamel surfaces with WSLs, while samples grouped as Health were collected from the sound enamel sites. Scraped plaque sample was resuspended into a 1.5 mL microcentrifuge tube containing 900 μL sterilized TE buffer (10 mM Tris–HCl, pH 8.0, 1 mM EDTA) and then immediately transferred to the lab on ice. Each sample was vortexed for 20 s and mixed thoroughly by pipetting up and down 10 times, then divided into 3 equal aliquots for 300 μL per tube. Among the 3 aliquots, one aliquot of the sample was centrifuged and resuspended in 1 mL sterilized PBS supplemented with 20% glycerol for further Candida culture and identification. The other two aliquots were used for further DNA extraction. All three tubes were stored at −80°C before use.
2.3. DNA purification, 16S rRNA sequencing, and processing
For each sample, one aliquot tube resuspended in TE buffer was processed for DNA extraction and bacterial 16S rRNA sequencing. After thawing at room temperature, samples were firstly mechanically disrupted by 0.1 mm glass beads for 2 cycles, 30 s/cycle (Fastprep-24 5G, MP Biomedical, CA, USA). Total genomic DNA was then extracted using a commercial bacterial genome DNA extraction kit following the manufacturer’s instruction (FastDNA® Spin Kit for Soil, MP Biomedicals, CA, USA). The quality and quantity of eluted DNA were measured by a spectrophotometer (NanoDrop 2000, Thermo Fisher Scientific, MA, USA), then stored at –80°C for further use. Bacterial 16S rRNA gene fragments (V1-V3) were amplified from the extracted DNA using specific primers 27F (5′-AGAGTTTGATCCTGGCTCAG-3′) and 533R (5′-TTACCGCGGCTGCTGGCAC-3′). The sample DNA library was finally obtained and constructed by 2 × 300 bp paired-end (PE) sequencing on the Illumina MiSeq platform using PE300 chemical at Majorbio Bio-Pharm Technology Co. Ltd. (Majorbio, Shanghai, China). The raw reads were deposited into the NCBI Sequence Read Archive (SRA) database (accession number: SRP404186).
After demultiplexing the adapter and primer from the raw data, the sequences were merged with FLASH (v1.2.11) and quality filtered with fastp (0.19.6) (Magoc and Salzberg, 2011; Chen et al., 2018). To obtain high-quality data and improve the accuracy of subsequent bioinformation analysis, sequences were de-noised using DADA2 plugin in the QIIME2 pipeline with recommended parameters, which obtained single nucleotide resolution based on error profiles within samples (Callahan et al., 2016; Bolyen et al., 2019). High-resolution taxonomic assignment of ASVs yield by DADA2 was performed using the Naive bayes consensus taxonomy classifier implemented in QIIME2 and established by alignment to the HOMD database (v15.2) (Chen et al., 2010).
2.4. Detection of C. albicans
Colonization of C. albicans was checked from all samples in both WSLs and Health groups. Samples stored in PBS with 20% glycerol were plated on CHROMagar Candida selective agar plates (Becton Dickinson & Co., Franklin Lakes, NJ, USA), and incubated at 37°C for 72 h (Pusateri et al., 2009; Ali et al., 2017). Colonies cultured by CHROMagar plate were preliminarily identified according to the color of the colony (i.e., C. albicans showed green colonies). Then, colonies were collected with sterilized inoculation loops and transferred to new tubes for further DNA extraction and rDNA ITS sequence analyses (Thiyahuddin et al., 2019).
After mechanical disruption with 0.5 mm glass beads, an Epicenter MasterPure DNA purification kit (Lucigen Corporation, Middleton, WI, USA) was used to purify the total genomic DNA of each sample. Identification of yeast species was carried out by PCR amplification and rDNA ITS sequence analyses. The PCR reactions were performed using ABI GeneAmp PCR System 9700 (Eppendorf Mastercycler gradient, HH, DE). A total of 2 × Taq PCR Mastermix (KT201, Tiangen Biotechnologies, Beijing, China) was used in PCR amplification, and ITS4/ITS5 primers were used (Supplementary Table 1; White et al., 1990). Each 50 μL PCR reaction system contained 17 μL sterile water, 25 μL 2 × Taq PCR Mastermix, 4 μL template DNA, and 2 μL each of forward and reverse ITS primers. PCR was performed as the following program: 95°C for 5 min; followed by 35 cycles of the following steps: denaturation at 95°C for 30 s; annealing at 55°C for 30 s; extension at 72°C for 1 min; and final extension step at 72°C for 10 min. PCR products were sent to the Beijing genomics institution for the identification of yeast species. After the sequences obtained by sequencing were assembled and corrected, blast software was used to compare the sequences in the GenBank database, and ITS sequences of related strains with high similarity were selected. The existence of C. albicans in the sample was double confirmed by: (1) the presence of colonies on CHROMagar plate; and (2) confirmation by ITS sequencing.
2.5. Quantification of C. albicans
To quantify the abundance of C. albicans in the samples, a droplet digital polymerase chain reaction (ddPCR) was performed. The total genomic DNA of the other aliquot of the supragingival plaque sample was extracted and purified as mentioned in section “2.4 Detection of C. albicans.” The SAP gene (Supplementary Table 1) of C. albicans was amplified and the ddPCR reactions were performed by the QX200 Droplet Digital PCR system (BioRad, Hercules, CA, USA). Each 20 μL ddPCR reaction system contained 8.6 μL sterile water, 10 μL 2 × ddPCR Supermix for EvaGreen, 1 μL template DNA, and 0.2 μL each of forward and reverse SAP primers. The mixture and droplet generation oil was added into DG8 Cartridges, and droplets were generated through the QX200 Droplet Generator (BioRad, Hercules, CA, USA). Next, the droplets were transferred to a 96-well PCR plate and sealed with PCR Plate Sealer (PX1, BioRad, Hercules, CA, USA). ddPCR was performed as the following program: 95°C for 10 min, followed by 40 cycles of 95°C for 30 s and 59.5°C for 80 s, and 1 cycle of 98°C for 10 min. Then, the PCR plate was put into a Droplet Reader to detect fluorescence (QX200, BioRad, Hercules, CA, USA). Finally, Quantalife Software 1.7.4 were used to analyze the data.
2.6. Statistical analyses
For microbiome sequencing data, the non-parametric Wilcoxon rank-sum test was used to identify ASVs in QIIME2. Principal Coordinate Analysis (PCoA) was applied to compare beta diversity between groups and the statistical significance was evaluated by the ADONIS test. Chao1, ACE, Simpson and Shannon indices were used to compare alpha diversity. Differences in taxonomic composition, and community function were evaluated using the Wilcoxon rank-sum test. Metastasis analysis was employed to identify bacteria with significant differences between groups at different classification levels based on false discovery rate (FDR). Indicated taxa enriched in different groups were determined by linear discriminant analysis (LDA) effect size (LEfSe). The threshold for distinguished logarithmic LDA score was set to 3.5.
Pearson’s chi-squared test was used to compare the detection rate of C. albicans between WSLs and the Health group. The Kruskal-Wallis test was used to compare the absolute abundance of C. albicans in different groups. Spearman’s correlation coefficient was applied to examine the correlations of the abundance of C. albicans, bacterial taxa, and EDI. A value of two-tailed P < 0.05 was considered statistically significant.
3. Results
3.1. The supragingival microbiome between WSLs and health were significantly different
In this research, patients with typical WSLs or without demineralization were recruited to study their microbial differences under fixed orthodontic treatment (Table 1). We first compared the characteristics of the supragingival microbiome between both groups via amplicon sequencing. A total of 3,779,993 raw reads were obtained from the 54 samples. By quality filtration, 2,728,802 optimized sequences were got, and the mean sequence length was 483 bp. After de-noised using the DADA2 plugin in the QIIME2 pipeline with recommended parameters, finally, a total of 407,413 sequences were obtained. Taxonomically classified ASVs were associated with 11 phyla, 20 classes, 28 orders, 42 families, 81 genera, and 297 species from all samples. Among all annotated genera, 63 genera were uniformly shared by both groups. Twelve genera were uniquely detected in the Health group while 6 genera were in the WSLs group (Supplementary Figure 1A).
To determine whether differences existed between patients with or without WSLs, alpha and beta diversity in both groups were analyzed (Figure 1). Simpson and Shannon indices showed that significantly higher community diversity in the Health group was found (P < 0.05). No significant difference was detected in richness by Chao 1 and ACE indices. In addition, we analyzed the overall bacterial community composition using PCoA plots. Results showed a significant difference in beta diversity (P < 0.05), revealing that most samples obtained from WSLs remarkably clustered away from those obtained from healthy teeth.
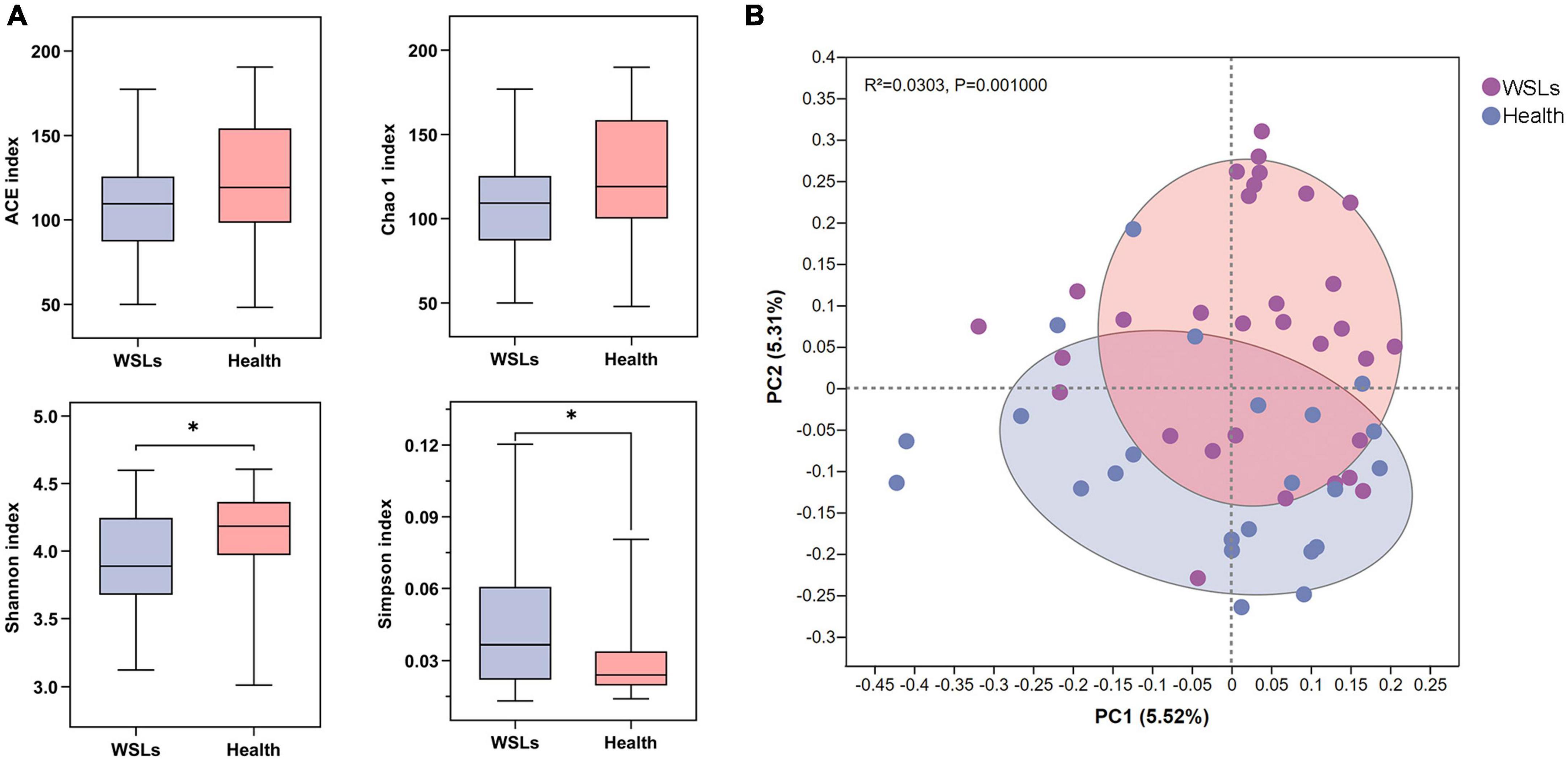
Figure 1. Comparisons of microbial diversities between WSLs and health group. (A) Alpha diversity was evaluated by indices of ACE, Chao1, Shannon, and Simpson. Simpson and Shannon indices showed significantly higher diversity in the microbial community of health group (*P < 0.05). (B) Beta diversity was calculated via the comparison of principal coordinates analysis (PCoA) of both groups. Each dot represents one sample. The communities in WSLs group tended to cluster apart from the communities in health group (P < 0.05).
To further identify the distinct microbial composition between WSLs and healthy niches, relative abundance in phylum, genera, and species taxonomic levels was focused. In all phenotypes, the dominant phyla were Firmicutes, Bacteroidetes, Actinobacteria, Saccharibacteria_TM7, Fusobacteria, and Proteobacteria (Supplementary Figure 2A). The core genera (relative abundance >1.0%) in both groups were plotted in Figure 2A. Among them, the most dominant 10 genera were Saccharibacteria_TM7_G-1, Streptococcus, Corynebacterium, Leptotrichia, Capnocytophaga, Actinomyces, Prevotella, Veillonella, Selenomonas, and Porphyromonas. Compared with the Health group, Leptotrichia and Prevotella were relatively elevated in the WSLs group. Leptotrichia also showed significance in the WSLs group based on the analysis of the Wilcoxon rank-sum test and LDA score (P < 0.05). Besides, compared with the WSLs group, Neisseria, Cardiobacterium, Bacteroidales G-2, Pseudopropionibacterium, and Lautropia were significantly enriched in the Health group (P < 0.05) (Figure 2B).
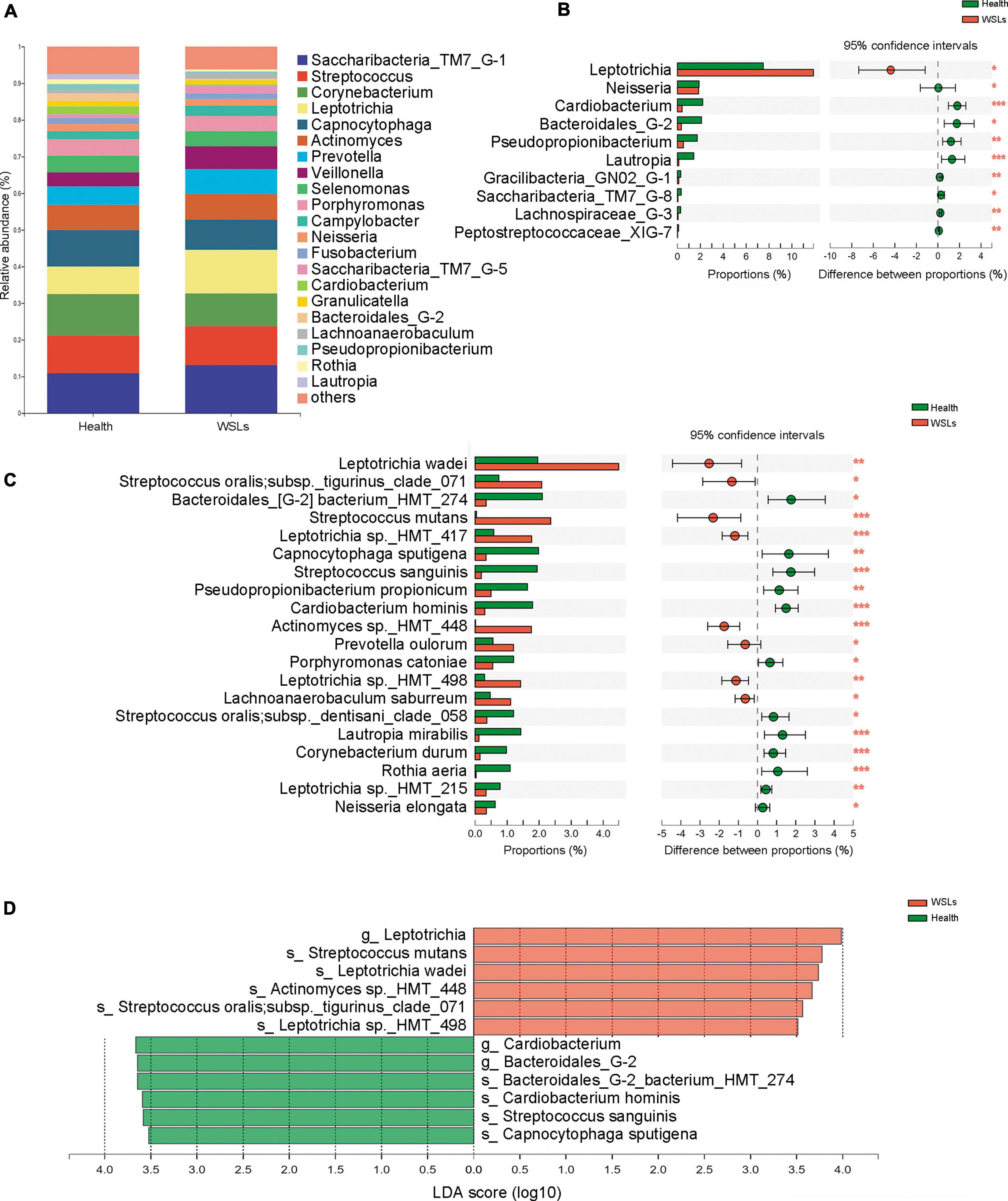
Figure 2. Comparison of microbial composition between WSLs and health group. (A) Relative abundances of the core genera (relative abundance >1.0%) in WSLs and health groups. “Others” represented all the genera less than 1%. (B) The most significantly different 10 genera in proportion between WSLs and health group were plotted (ranked by relative abundance). Leptotrichia was dominant in WSLs lesions while the others enriched in health group. (C) The top 20 species showed significant differences between WSLs and health group (ranked by relative abundance). (D) LEfSe analysis was performed between both groups at genus and species levels. Significantly differentiated distributed genera and species were displayed as the LDA score >3.5 (*P < 0.05, **P < 0.01, ***P < 0.001).
At the species level, some Streptococcus spp. displayed prominently difference in abundance (Figures 2C, D), despite no statistical significance being examined in the genera Streptococcus between WSLs and Health. As the member of the mitis group (MGS), Streptococcus sanguinis was abundant in the Health group (P < 0.05). On the contrary, S. mutans, considered a contributor to the etiology of dental caries, was more abundant in the WSLs group (P < 0.05) (Simón-Soro et al., 2014). Moreover, Leptotrichia wadei, Leptotrichia sp._HMT_417, Actinomyces sp._HMT_448, Prevotella oulorum, and Leptotrichia sp._HMT_498 were also enriched in the WSLs group (P < 0.05). In addition, Bacteroidales_G-2 bacterium_HMT_274, Capnocytophaga sputigena, Pseudopropionibacterium propionicum, Cardiobacterium hominis, Porphyromonas catoniae, Lautropia mirabilis, Corynebacterium durum, Rothia aeria, Leptotrichia sp._HMT_215, and Neisseria elongata had a richer abundance in Health group (P < 0.05).
3.2. C. albicans colonization and load was remarkably higher in WSLs group
The colonization of C. albicans in the plaque was first examined by sample inoculation in CHROMagar, and further molecular identification of colonies that emerged on CHROMagar was carried out by ITS sequencing. Interestingly, no colony emerged on CHROMagar in the Health group, while 14 samples showed green colonies among 31 WSLs samples (Supplementary Figure 3A). In further species identification by PCR and ITS sequencing, colonies from 14 samples were all C. albicans. Accordingly, as shown in Figure 3A, the detection rate of C. albicans in WSLs was significantly higher than that in the Health group (χ2 = 14.586, P < 0.01). This result indicated that the colonization of C. albicans was more likely to occur in demineralization lesions rather than in healthy niches.
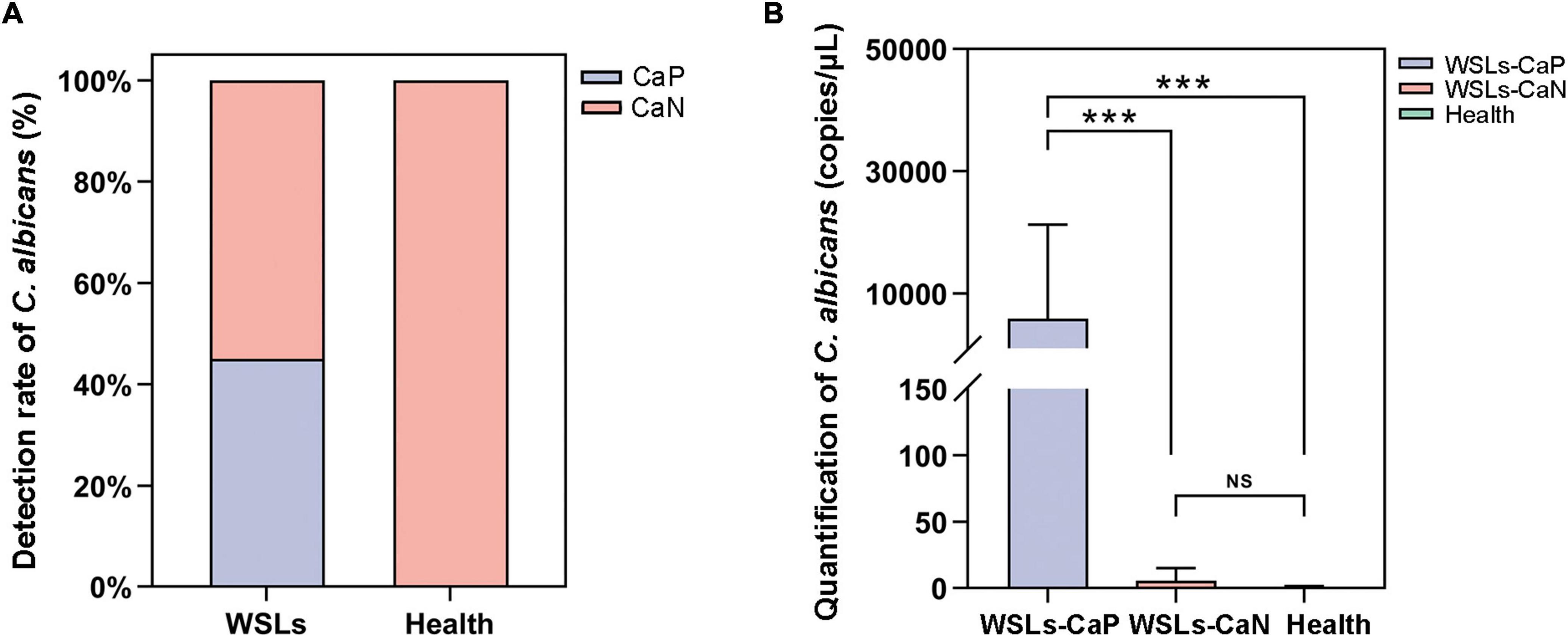
Figure 3. Candida albicans infection in the supragingival plaque. (A) The positive rate of C. albicans in WSLs and health groups. Positive result of C. albicans infection was determined by inoculation once the colonies emerged on CHROMAgar. Among all WSLs patients, 45% were C. albicans positive, but none in the health group. CaP: C. albicans positive; CaN: C. albicans negative. (B) Quantification of C. albicans in supragingival plaque among WSLs-CaP, WSLs-CaN, and health groups. C. albicans (copies/uL) in WSL-CaP group was remarkably higher than WSL-CaN and health groups (NS: no significance, ***P < 0.001).
Next, samples from the WSLs group were further divided into two subgroups, namely the C. albicans cultivation positive subgroup (WSLs-CaP) and the C. albicans cultivation negative subgroup (WSLs-CaN). The absolute abundance of C. albicans was determined by ddPCR analysis. All three groups were performed with ddPCR although most samples didn’t have any colony come up. As shown in Figure 3B, the abundance of C. albicans in the WSLs-CaP group was significantly higher than in WSLs-CaN as well as Health group (P < 0.01), which verified those samples could be inoculated with C. albicans colonies indeed had much higher load than colony-free samples. In addition, no significant difference was found between WSLs-CaN as well as Health groups (P > 0.05).
3.3. Enrichment of C. albicans affects the composition of supragingival microbiome in WSLs
We next proposed whether the enrichment of C. albicans could affect the bacterial composition in the plaque. Following the results above, analysis was performed between WSLs-CaP and WSLs-CaN subgroups in this section. Among all annotated genera, 57 genera were uniformly shared by both groups. One genus was uniquely detected in the WSLs-CaP group while 11 genera were in the WSLs-CaN group (Supplementary Figure 1B). As shown in Figure 4, there was no significant difference in alpha diversity. For beta diversity, the PCoA of the two principal components accounted for 36.77 and 14.42% of the total variation, respectively, suggesting the separation between the two subgroups was significant (R2 = 0.0966, P < 0.05).
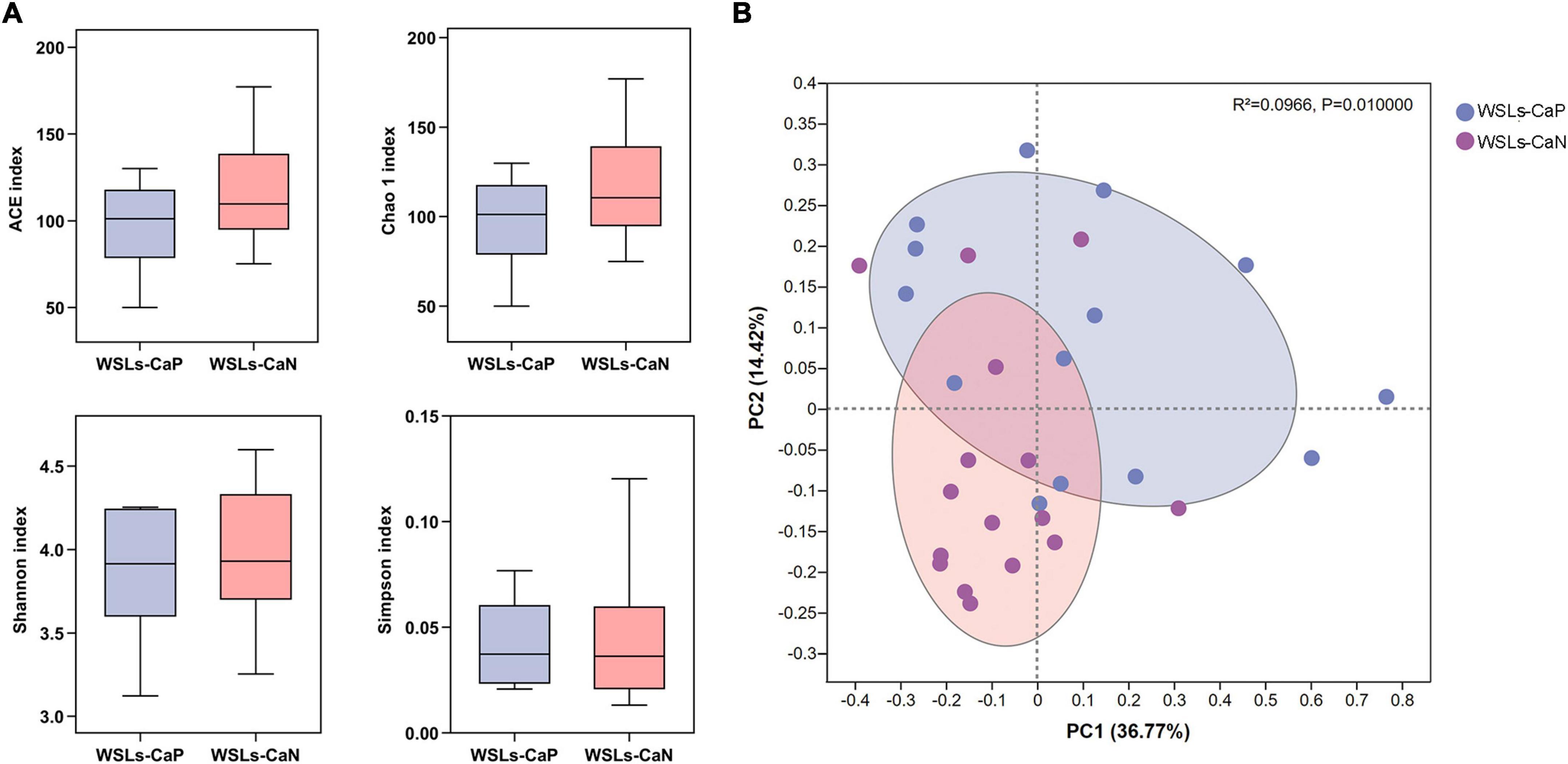
Figure 4. Comparisons of microbial diversities between WSLs-CaP and WSLs-CaN groups. (A) Alpha diversity was evaluated by indices of ACE, Chao1, Shannon, and Simpson. No significance was detected in all indices. (B) Beta diversity was calculated via the comparison of principal coordinates analysis (PCoA). Each dot represents one sample. The communities in WSLs-CaP group tended to cluster apart from the communities in WSLs-CaN group (P < 0.05).
In all phenotypes, the dominant phyla were also Firmicutes, Bacteroidetes, Actinobacteria, Saccharibacteria_TM7, Fusobacteria, and Proteobacteria (Supplementary Figure 2B). The relative abundance of core genera (relative abundance >1.0%) were compared to illustrate whether C. albicans affect the microbial composition (Figure 5A). Compared to the WSLs-CaN subgroup, Corynebacterium and Rothia were the predominant genera in the WSLs-CaP subgroup (P < 0.05). Corynebacterium matruchotii and Streptococcus mutans had much higher abundance in the WSLs-CaP subgroup (P < 0.05). On the contrary, Saccharibacteria_TM7_G-1 significantly enriched in the WSLs-CaN group (P < 0.05) with its two species Saccharibacteria_TM7_G-1 bacterium_HMT_346 and Saccharibacteria_TM7_G-1 bacterium_HMT_349 showing the same trend (P < 0.05) (Figures 5B–D). This interesting results suggested the existence of C. albicans might shape the bacterial community in WSLs disease condition.
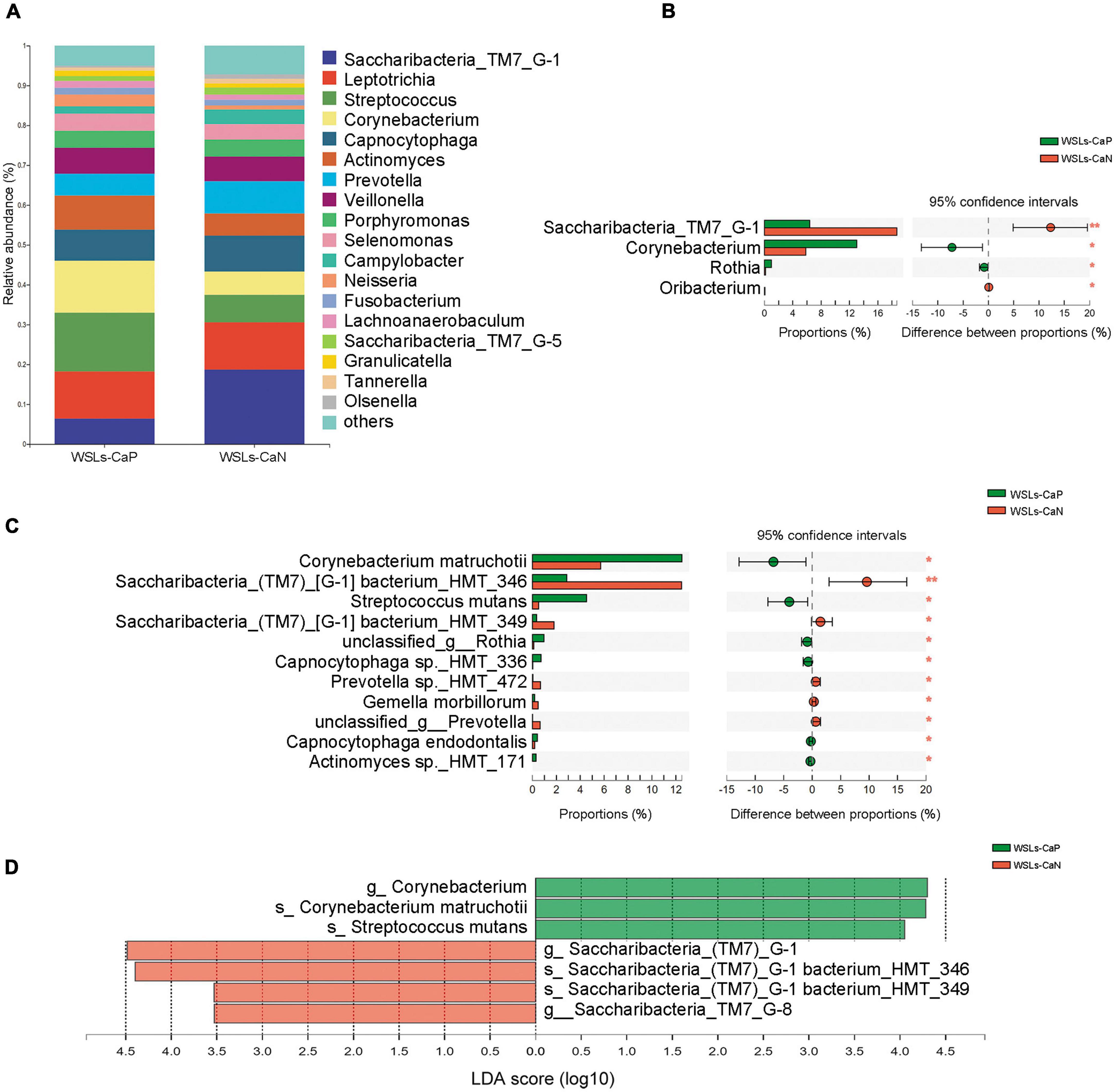
Figure 5. Comparison of microbial composition between Candida albicans carriers and non-carriers in WSLs patients. (A) Relative abundances of the core genera (relative abundance >1.0%) in WSLs-CaP and WSLs-CaN groups. “Others” represented all the genera less than 1%. (B) Significantly differentiated genera between WSLs-CaP and WSLs-CaN groups. Saccharibacteria_TM7_G-1 was much enriched in plaque without C. albicans infection, while Corynebacterium, Rothia and Oribacterium were enriched in C. albicans positive group. (C) Significantly different species examined between WSLs-CaP and WSLs-CaN group were displayed. In C. albicans carriers, Corynebacterium matruchotii and Streptococcus mutans significantly enriched, whereas several species such as Saccharibacteria_TM7_G-1 bacterium_HMT_346 and Saccharibacteria_TM7_G-1 bacterium_HMT_349 significantly enriched in C. albicans non-carriers. (D) LEfSe analysis was performed between WSLs-CaP and WSLs-CaN groups at the genus and species levels. Significantly differentiated distributed genera and species were displayed as the LDA score >3.5 (*P < 0.05, **P < 0.01).
3.4. Correlation between C. albicans, bacterial and EDI
Although the existence of C. albicans may change the bacterial community in dental plaque, the correlation between the abundance of C. albicans and bacterial changes was not clear. We thus performed Spearman’s rank correlation analysis to specifically examine the correlation between the abundance of C. albicans and bacterial taxa (Figure 6). S. mutans, which is the main contributor to dental caries, was positively correlated with C. albicans. In addition, bacteria showed significantly higher abundance in patients with WSLs, such as Leptotrichia wadei, Actinomyces sp._HMT_448, and Lachnoanaerobaculum saburreum, were also exhibited a positive correlation with C. albicans.
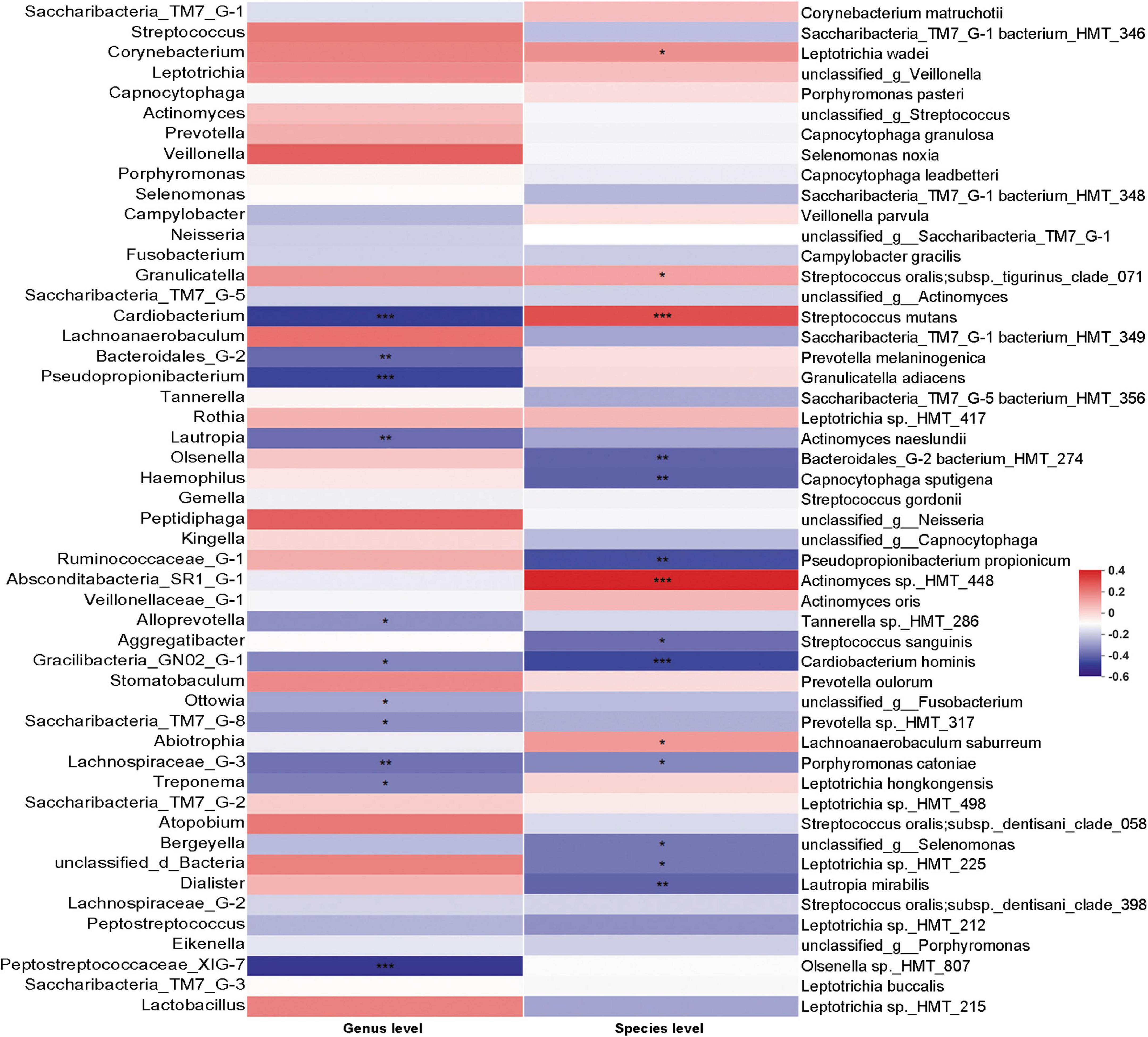
Figure 6. Correlation analysis between Candida albicans and bacteria based on Spearman correlation coefficient. The correlation between the quantification of C. albicans and the top 50 abundant bacteria genera and species in the rank of relative abundance was evaluated. At genus level, bacteria such as Saccharibacteria_TM7_G-8 negatively associated with C. albicans. At species level, Streptococcus mutans had positive association with the load of C. albicans. Several species such as Streptococcus sanguinis and Cardiobacterium hominis had depleting trend in C. albicans enriched samples. Blocks in red showed positive correlation, while blocks in blue showed negative correlation. Color depth implicated the strength of correlation (*P < 0.05, **P < 0.01, ***P < 0.001).
Interestingly, on the contrary, S. sanguinis, which can release H2O2 thereby inhibiting the growth of S. mutans and being prone to be detected on the healthy teeth surface, were negatively correlated with C. albicans (Kreth et al., 2009). In addition, some other bacteria showed significantly higher abundance in the Health group, such as Cardiobacterium, Bacteroidales_G-2, Pseudopropionibacterium, Lautropia, Gracilibacteria_GN02_G-1, Saccharibacteria_TM7_G-8, Lachnospiraceae_G-3, and Peptostreptococcaceae_XIG-7 exhibited a negative correlation with C. albicans. At the species level, Bacteroidales_G-2 bacterium_HMT_274, Capnocytophaga sputigena, Pseudopropionibacterium propionicum, Cardiobacterium hominis, Porphyromonas catoniae, Leptotrichia sp._HMT_225, and Lautropia mirabilis exhibited a negative correlation with C. albicans as well.
To address whether the C. albicans abundance was correlated with the severity of EDI, we investigated a Spearman’s rank correlation between EDI and C. albicans abundance (Supplementary Figure 3B). The result showed that no significant correlation between EDI and C. albicans abundance in the WSLs group (P = 0.09). In other words, although C. albicans was significantly enriched in the WSLs group, it did not show a significant correlation with the severity of WSLs.
4. Discussion
White spot lesions in fixed orthodontics are demineralization opacities that occur on the enamel surface and induce irreversible effects on dental hard tissue. The process of demineralization is closely related to plaque accumulation from which the microbial structure and metabolism might change accordingly. To deepen the understanding of microbiological pathogenesis in the development of WSLs, this study firstly investigated the differences of the supragingival microbiome in orthodontic patients with or without visible WSLs. Our results demonstrated that great changes could be observed between healthy and disease status, and all the three null-hypotheses were rejected. Compared to patients with healthy enamel, the WSLs group had significantly lower alpha diversity and differentiated clustering features in the community, which was consistent with previous studies (Hurley et al., 2019; Schoilew et al., 2019). This might be due to the cariogenic microenvironment that acidogenic bacteria produced acidic metabolites and then decreased the local pH (Corralo et al., 2021). Inhibition of acid-non-resistant bacteria and the overgrowth of aciduric bacteria led to lower diversity in the plaque community.
In terms of the difference in microbial composition, our results undoubtedly showed that the abundance of S. mutans had a significant difference between both groups. This demonstrated the cohorts we selected were comparative and that the samples we collected were typical (Thompson and Pikis, 2012; Qudeimat et al., 2021). Leptotrichia was one of the predominant genera in the plaque from the WSLs niche, which was consistent with the previous report (Yun et al., 2019). Leptotrichia could actively metabolize carbohydrates with higher membrane transport capacity (Eribe and Olsen, 2017; Chen Y. et al., 2021). This plaque-enriched genus was also reported to be able to produce extracellular polysaccharides (EPS) through carbohydrate anabolism, as well as multiple cariogenic organic acids as its end product through catabolism. With such a higher abundance in supragingival plaque, Leptotrichia was very likely associated with the development of enamel demineralization (Eribe and Olsen, 2017). Moreover, two of its main species Leptotrichia wadei and Leptotrichia sp._HMT_498 were also reported with increasing abundance in adolescents with active dental caries (Eriksson et al., 2017). Leptotrichia wadei, with significantly higher abundance in our patients with demineralization, was also regarded as one of the predictors for dental caries (Hurley et al., 2019; Chen J. et al., 2021). This implies that its richness in orthodontic patients might be a warning indicator of WSLs. Besides, we found Actinomyces sp._HMT_448 enriched in the WSLs group, despite the abundance of Actinomyces spp. having almost no difference between both groups. Actinomyces sp._HMT_448 was reported with vigorous metabolism of carbohydrates, and producing lactic acid at lower pH level (Eriksson et al., 2017; Corralo et al., 2021). Therefore, the accumulation of this species also needs to be paid attention to in orthodontic WSLs.
Some genera such as Neisseria, Cardiobacterium, Bacteroidales G-2, Pseudopropionibacterium, and Lautropia were found enriched on healthy sites but depleted in WSLs. These results were consistent with the previous studies (Glogauer et al., 2015; Chen Y. et al., 2021), which indicated these bacteria might be associated with the stability of a healthy microbiome. Some of these healthy associated members are enriched may be due to their intrinsic metabolic characteristics. Lautropia had a higher level of signal transduction, xenobiotic biodegradation, and metabolism, which was conducive to dental health (Al-Kamel et al., 2019; Chen Y. et al., 2021). Neisseria could degrade lactate to acetate and exhibit higher arginine metabolism, thus neutralizing the acidification of the dental plaque (Rosier et al., 2020). Patients without dental caries also reportedly had a higher abundance of Lautropia and Neisseria in their dental plaques, their predominant abundance could be regarded as beneficial to oral health (Rosier et al., 2020). Besides, Pseudopropionibacterium propionicum could actively metabolize amino acid, which might be conducive to the neutralization of acid in dental plaque (Chen Y. et al., 2021). In addition, although result showed no significant differences in the abundance of Corynebacterium in two groups, it was relatively elevated in the Health group (Figure 2A). Previous study proposed that Corynebacterium could raise the pH of dental plaque by utilizing organic acids produced by other microbes (Qudeimat et al., 2021). Also, it can shape symbiotic supragingival biofilm communities by having anfractuous interactions with health-associated bacteria (Treerat et al., 2020). Moreover, we observed completely opposite trends in the abundance distribution of S. mutans and S. sanguinis in both groups. As a member of the Mitis Group Streptococcus, S. sanguinis, enriched in the Health group, can release H2O2 thereby inhibiting the growth of S. mutans (Kreth et al., 2009).
Candida carriage in patients undergoing orthodontic treatment has raised the attention of orthodontists (Zheng et al., 2016; Alhamadi et al., 2017). However, to date, there was no study yet reporting the association between C. albicans and orthodontic-derived early caries. In addition to oral bacteria, we studied whether C. albicans were dissimilarly allocated in both groups. Interestingly, all colonies emerged on CHROMagar only from the WSLs group, suggesting patients with WSLs preferably had C. albicans infection. We further confirmed a much higher load of C. albicans carriage in WSLs sites by ddPCR quantification. As a technique with high sensitivity and accuracy, ddPCR can realize the absolute quantification of nucleic acids (Hindson et al., 2011). ddPCR divides reagents into tens of thousands of nanoliter or picoliter partitions by a microfluidic chip, and each droplet contains 0 or 1 DNA template. After polymerase chain reaction amplification and the detection of fluorescence, the target nucleic acids were calculated according to the number of positive droplets (Pinheiro et al., 2012). Furthermore, each droplet is an independent closed reaction environment, which can reduce the possibility of contamination between droplets (Wang et al., 2018). On the other hand, one of the disadvantages is that the operation of ddPCR is relatively complex. The ddPCR results also proved that C. albicans would be more possible to occur and accumulate in orthodontic patients who had enamel demineralization. Furthermore, we found that the enrichment of C. albicans could affect the bacterial composition in the supragingival dental plaque. Analysis between Candida carriers and non-carriers among WSLs patients showed the difference in beta-diversity was significant (P < 0.05), revealing that the microbial community obtained from Candida carriers remarkably clustered away from non-carriers.
The existence of Candida could affect the bacteriome composition, which has been reported in different habitats (Du et al., 2021). Intriguingly, in our study S. mutans was more predominant in C. albicans carriers rather than non-carriers among all WSLs patients. This result supported these two interkingdom species having close interactions and positive correlations. Their co-existence has been widely reported in caries disease models like ECC and root caries (Xiao et al., 2016). Moreover, their cariogenic co-pathogenesis has also been extensively investigated. Coaggregation of C. albicans and S. mutans was very important for their co-localization and biofilm formation on tooth surfaces (Metwalli et al., 2013; Xu et al., 2014). C. albicans could grow better in C. albicans-S. mutans dual-species biofilm than its mono-species biofilm under the stimulation of S. mutans (Arzmi et al., 2016). When pioneer colonizers (e.g., S. mutans) initially adhered to the teeth, the activation of glucosyltransferases (GTFs) increased, especially the gtfB gene. Among GTFs, GTF-I was encoded by the gtfB gene and used sucrose as a substrate to synthesize water-insoluble glucan, which could participate in sucrose-dependent adhesion between bacteria and enamel surface (Bowen and Koo, 2011; Gregoire et al., 2011). These further became the binding sites for subsequent colonizers like C. albicans (Gregoire et al., 2011). Conversely, the Candida-derived mannan, and β-glucan also provided binding sites for GTFB, and the structure of EPS could be affected by β-1,3-glucans as well (Falsetta et al., 2014; Xu et al., 2014). Our results showed this interkingdom interaction might also exist in orthodontic derived initial enamel caries, that is particularly noteworthy in fixed orthodontic treatment. However, their potential role in the development of orthodontic WSLs still required long-term observation of microbial change to confirm.
In this study, other bacteria such as C. hominis, S. sanguinis, and L. mirabilis showed negative correlation with C. albicans. Among them, C. albicans showed lower virulence in interaction with S. sanguinis (Do Rosário Palma et al., 2019). The adhesion ability and Young’s modulus of C. albicans also decreased after being antagonized by S. sanguinis bacteriocin (Ma et al., 2017). The intracellular protein of S. sanguinis has a significant inhibitory effect on C. albicans and its biofilm. In addition, the growth curve and morphology of C. albicans changed as well, leading to discoid depressions on the surface of fungal spores and mycelia (Ma et al., 2014). Besides S. sanguinis, however, other bacteria which were negatively related to C. albicans in this study were rarely reported in previous research, so further studies are needed.
Besides, the result showed that there was no significant correlation between the abundance of C. albicans and the severity of EDI in the WSLs group. It was known that obvious WSLs can occur within 6 months of orthodontic treatment (Lucchese and Gherlone, 2012). Patients undergoing fixed orthodontic treatment within 6 to 12 months were recruited in this study (Tufekci et al., 2011). However, with the prolonged treatment time, such as at the 24 months of treatment, the occurrence of WSLs would further increase (Khalaf, 2014). Previous studies had shown that compared with the healthy teeth in caries-free children, the dental plaque on the healthy teeth surface of caries-active children was more similar to that with enamel caries, and had a higher risk of dental caries (Richards et al., 2017). Therefore, it was speculated that the change of microorganisms was earlier than the occurrence and aggravation of WSLs. In addition, the sample size will also affect the correlation results. One of the limitations of this study was that the sample size was relatively small, it is necessary to further increase the sample size in future research.
5. Conclusion
In conclusion, the present study revealed significant differences in the supragingival plaque microbiome between orthodontic patients with and without WSLs. C. albicans was more frequently detected and enriched in the plaque with WSLs rather than in the healthy site. The existence of C. albicans could shape the bacterial composition in the supragingival plaque community. C. albicans have a certain interkingdom association with bacteriomes. This study further enhanced the understanding of WSLs from the perspective of the oral microbiome, which is conducive to the exploration of potential diagnostic approaches and interventions in orthodontic-induced WSLs.
Data availability statement
The datasets presented in this study can be found in online repositories. The names of the repository/repositories and accession number(s) can be found below: https://www.ncbi.nlm.nih.gov/, SRP404186.
Ethics statement
This study was approved by the Ethical Committee of Beijing Stomatological Hospital (No. CMUSH-IRB-KJ-PJ-2022-11). Written informed consent to participate in this study was provided by the participants’ legal guardian/next of kin.
Author contributions
HY and YM performed the experiments, data analyses, and manuscript writing. XX contributed to the data analysis. HW analyzed the data and revised the manuscript. XL and DF helped complete the experiments. YM designed the study, proposed ideas, provided discussions, and advised on manuscript revise. YB conceived the experiments, supervised the experiment progress, and revised the manuscript. All authors contributed to the article and approved the submitted version.
Funding
This study was supported by the “Capital’s Funds for Health Improvement and Research CFH 2020-4-2144 (YM),” “Natural Science Foundation of China NSFC 82001078 (YM),” and “Innovation Research Team Project of Beijing Stomatological Hospital, Capital Medical University, NO. CXTD202203.”
Acknowledgments
We are very grateful to Prof. Dongming Li and her group, from Peking University Third Hospital, who provided theoretical and operational guidance on experiments related to Candida albicans. And, we are also very appreciative of the Institute of Microbiology, Chinese Academy of for providing Candida albicans standard strains.
Conflict of interest
The authors declare that the research was conducted in the absence of any commercial or financial relationships that could be construed as a potential conflict of interest.
Publisher’s note
All claims expressed in this article are solely those of the authors and do not necessarily represent those of their affiliated organizations, or those of the publisher, the editors and the reviewers. Any product that may be evaluated in this article, or claim that may be made by its manufacturer, is not guaranteed or endorsed by the publisher.
Supplementary material
The Supplementary Material for this article can be found online at: https://www.frontiersin.org/articles/10.3389/fmicb.2023.1084850/full#supplementary-material
References
Alhamadi, W., Al-Saigh, R. J., Al-Dabagh, N. N., and Al-Humadi, H. W. (2017). Oral Candida in patients with fixed orthodontic appliance: In vitro combination therapy. Biomed. Res. Int. 2017:1802875. doi: 10.1155/2017/1802875
Ali, R. H., Luma, T. A., and Ali, S. M. (2017). Molecular and genotypes identification of C. albicans isolated from children with diarrea in diyala province Iraq. Int. J. Biotechnol. Res. 7, 1–10.
Al-Kamel, A., Baraniya, D., Al-Hajj, W. A., Halboub, E., Abdulrab, S., Chen, T., et al. (2019). Subgingival microbiome of experimental gingivitis: Shifts associated with the use of chlorhexidine and N-acetyl cysteine mouthwashes. J. Oral. Microbiol. 11:1608141. doi: 10.1080/20002297.2019.1608141
Andrucioli, M. C. D., Faria, G., Nelson-Filho, P., Romano, F. L., and Matsumoto, M. A. N. (2017). Influence of resin-modified glass ionomer and topical fluoride on levels of Streptococcus mutans in saliva and biofilm adjacent to metallic brackets. J. Appl. Oral. Sci. 25, 196–202. doi: 10.1590/1678-77572016-0231
Arzmi, M. H., Alnuaimi, A. D., Dashper, S., Cirillo, N., Reynolds, E. C., and McCullough, M. (2016). Polymicrobial biofilm formation by Candida albicans, Actinomyces naeslundii, and Streptococcus mutans is Candida albicans strain and medium dependent. Med. Mycol. 54, 856–864. doi: 10.1093/mmy/myw042
Bachtiar, E. W., and Bachtiar, B. M. (2018). Relationship between Candida albicans and Streptococcus mutans in early childhood caries, evaluated by quantitative PCR. F1000Res 7, 1645–1660. doi: 10.12688/f1000research.16275.2
Badea, M. E., Mesaroş, A., Şuhani, R. D., and Cosma, L. L. (2019). Current treatment modalities of orthodontically induced white spot lesions and their outcome-a literature review. Med. Pharm. Rep. 92, 25–30. doi: 10.15386/cjmed-1090
Baraniya, D., Chen, T., Nahar, A., Alakwaa, F., Hill, J., Tellez, M., et al. (2020). Supragingival mycobiome and inter-kingdom interactions in dental caries. J. Oral. Microbiol. 12:1729305. doi: 10.1080/20002297.2020.1729305
Bolyen, E., Rideout, J. R., Dillon, M. R., Bokulich, N. A., Abnet, C. C., Al-Ghalith, G. A., et al. (2019). Reproducible, interactive, scalable and extensible microbiome data science using QIIME 2. Nat. Biotechnol. 37, 852–857. doi: 10.1038/s41587-019-0209-9
Bowen, W. H., and Koo, H. (2011). Biology of Streptococcus mutans-derived glucosyltransferases: Role in extracellular matrix formation of cariogenic biofilms. Caries Res. 45, 69–86. doi: 10.1159/000324598
Callahan, B. J., McMurdie, P. J., Rosen, M. J., Han, A. W., Johnson, A. J. A., and Holmes, S. P. (2016). DADA2: High-resolution sample inference from Illumina amplicon data. Nat. Methods 13, 581–583. doi: 10.1038/nmeth.3869
Chen, J., Kong, L., Peng, X., Chen, Y., Ren, B., Li, M., et al. (2021). Core microbiota promotes the development of dental caries. Appl. Sci. 11:3638. doi: 10.3390/app11083638
Chen, S., Zhou, Y., Chen, Y., and Gu, J. (2018). fastp: An ultra-fast all-in-one FASTQ preprocessor. Bioinformatics 34, i884–i890. doi: 10.1093/bioinformatics/bty560
Chen, T., Yu, W. H., Izard, J., Baranova, O. V., Lakshmanan, A., and Dewhirst, F. E. (2010). The human oral microbiome database: A web accessible resource for investigating oral microbe taxonomic and genomic information. Database 2010:baq013. doi: 10.1093/database/baq013
Chen, Y., Dou, G., Wang, D., Yang, J., Zhang, Y., Garnett, J. A., et al. (2021). Comparative microbial profiles of caries and black extrinsic tooth stain in primary dentition. Caries Res. 55, 310–321. doi: 10.1159/000517006
Corralo, D. J., Ev, L. D., Damé-Teixeira, N., Maltz, M., Arthur, R. A., Do, T., et al. (2021). Functionally active microbiome in supragingival biofilms in health and caries. Caries Res. 55, 603–616. doi: 10.1159/000518963
Da Costa Rosa, T., De Almeida Neves, A., Azcarate-Peril, M. A., Divaris, K., Wu, D., Cho, H., et al. (2021). The bacterial microbiome and metabolome in caries progression and arrest. J. Oral. Microbiol. 13:1886748. doi: 10.1080/20002297.2021.1886748
Dağistan, S., Aktas, A. E., Caglayan, F., Ayyildiz, A., and Bilge, M. (2009). Differential diagnosis of denture-induced stomatitis, Candida, and their variations in patients using complete denture: A clinical and mycological study. Mycoses 52, 266–271. doi: 10.1111/j.1439-0507.2008.01592.x
Do Rosário Palma, A. L., Domingues, N., De Barros, P. P., Brito, G. N. B., and Jorge, A. O. C. (2019). Influence of Streptococcus mitis and Streptococcus sanguinis on virulence of Candida albicans: In vitro and in vivo studies. Folia. Microbiol. 64, 215–222. doi: 10.1007/s12223-018-0645-9
Du, Q., Ren, B., He, J., Peng, X., Guo, Q., Zheng, L., et al. (2021). Candida albicans promotes tooth decay by inducing oral microbial. ISME. J. 15, 894–908. doi: 10.1038/s41396-020-00823-8
Enaia, M., Bock, N., and Ruf, S. (2011). White-spot lesions during multibracket appliance treatment: A challenge for clinical excellence. Am. J. Orthod. Dentofacial. Orthop. 140, e17–e24. doi: 10.1016/j.ajodo.2010.12.016
Eribe, E. R. K., and Olsen, I. (2017). Leptotrichia species in human infections II. J. Oral. Microbiol. 9:1368848. doi: 10.1080/20002297.2017.1368848
Eriksson, L., Lif Holgerson, P., and Johansson, I. (2017). Saliva and tooth biofilm bacterial microbiota in adolescents in a low caries community. Sci. Rep. 7:5861. doi: 10.1038/s41598-017-06221-z
Ev, L. D., DamÉ-Teixeira, N., Do, T., Maltz, M., and Parolo, C. C. F. (2020). The role of Candida albicans in root caries biofilms: An RNA-seq analysis. J. Appl. Oral. Sci. 28:e20190578. doi: 10.1590/1678-7757-2019-0578
Falsetta, M. L., Klein, M. I., Colonne, P. M., Scott-Anne, K., Gregoire, S., Pai, C.-H., et al. (2014). Symbiotic relationship between Streptococcus mutans and Candida albicans synergizes virulence of plaque biofilms in vivo. Infect. Immun. 82, 1968–1981. doi: 10.1128/iai.00087-14
Glogauer, M., Chen, L., Qin, B., Du, M., Zhong, H., Xu, Q., et al. (2015). Extensive description and comparison of human supragingival microbiome in root caries and health. PLoS One 10:e0117064. doi: 10.1371/journal.pone.0117064
Gregoire, S., Xiao, J., Silva, B. B., Gonzalez, I., Agidi, P. S., Klein, M. I., et al. (2011). Role of glucosyltransferase B in interactions of Candida albicans with Streptococcus mutans and with an experimental pellicle on hydroxyapatite surfaces. Appl. Environ. Microbiol. 77, 6357–6367. doi: 10.1128/aem.05203-11
Guo, R., Zheng, Y., Zhang, L., Shi, J., and Li, W. (2021). Salivary microbiome and periodontal status of patients with periodontitis during the initial stage of orthodontic treatment. Am. J. Orthod. Dentofacial. Orthop. 159, 644–652.
Hindson, B. J., Ness, K. D., Masquelier, D. A., Belgrader, P., Heredia, N. J., Makarewicz, A. J., et al. (2011). High-throughput droplet digital PCR system for absolute quantitation of DNA copy number. Anal. Chem. 83, 8604–8610. doi: 10.1021/ac202028g
Hurley, E., Barrett, M. P. J., Kinirons, M., Whelton, H., Ryan, C. A., Stanton, C., et al. (2019). Comparison of the salivary and dentinal microbiome of children with severe-early childhood caries to the salivary microbiome of caries-free children. BMC Oral Health 19:13. doi: 10.1186/s12903-018-0693-1
Hwang, G., Liu, Y., Kim, D., Li, Y., Krysan, D. J., and Koo, H. (2017). Candida albicans mannans mediate Streptococcus mutans exoenzyme GtfB binding to modulate cross-kingdom biofilm development in vivo. PLoS. Pathog. 13:e1006407. doi: 10.1371/journal.ppat.1006407
KarabekİRoĞLu, S., ÜNlÜ, N., KÜÇÜKyilmaz, E., ŞEner, S., Botsali, M. S., and MalkoÇ, S. (2017). Treatment of post-orthodontic white spot lesions with CPP-ACP paste: A three year follow up study. Dent. Mater. J. 36, 791–797. doi: 10.4012/dmj.2016-228
Khalaf, K. (2014). Factors affecting the formation, severity and location of white spot lesions during orthodontic treatment with fixed appliances. J. Oral. Maxillofac. Res. 5:e4. doi: 10.5037/jomr.2014.5104
Koopman, J. E., Van der Kaaij, N. C., Buijs, M. J., Elyassi, Y., Van der Veen, M. H., Crielaard, W., et al. (2015). The effect of fixed orthodontic appliances and fluoride mouthwash on the oral microbiome of adolescents - a randomized controlled clinical trial. PLoS One 10:e0137318. doi: 10.1371/journal.pone.0137318
Kreth, J., Vu, H., Zhang, Y., and Herzberg, M. C. (2009). Characterization of hydrogen peroxide-induced DNA release by Streptococcus sanguinis and Streptococcus gordonii. J. Bacteriol. 191, 6281–6291. doi: 10.1128/jb.00906-09
Lee, E., Park, S., Um, S., Kim, S., Lee, J., Jang, J., et al. (2021). Microbiome of saliva and plaque in children according to age and dental caries experience. Diagnostics 11:1324. doi: 10.3390/diagnostics11081324
Lucchese, A., and Gherlone, E. (2012). Prevalence of white-spot lesions before and during orthodontic treatment with fixed appliances. Eur. J. Orthod. 35, 664–668. doi: 10.1093/ejo/cjs070
Ma, S., Ge, W., Yan, Y., Huang, X., Ma, L., Li, C., et al. (2017). Effects of Streptococcus sanguinis Bacteriocin on deformation, adhesion ability, and young’s modulus of Candida albicans. Biomed. Res. Int. 2017:5291486. doi: 10.1155/2017/5291486
Ma, S., Li, H., Yan, C., Wang, D., Li, H., Xia, X., et al. (2014). Antagonistic effect of protein extracts from Streptococcus sanguinis on pathogenic bacteria and fungi of the oral cavity. Exp. Ther. Med. 7, 1486–1494. doi: 10.3892/etm.2014.1618
Magoc, T., and Salzberg, S. L. (2011). FLASH: Fast length adjustment of short reads to improve genome assemblies. Bioinformatics 27, 2957–2963. doi: 10.1093/bioinformatics/btr507
Metwalli, K. H., Khan, S. A., Krom, B. P., and Jabra-Rizk, M. A. (2013). Streptococcus mutans, Candida albicans, and the human mouth: A sticky situation. PLoS Pathog. 9:e1003616. doi: 10.1371/journal.ppat.1003616
Peleg, A. Y., Hogan, D. A., and Mylonakis, E. (2010). Medically important bacterial–fungal interactions. Nat. Rev. Microbiol. 8, 340–349. doi: 10.1038/nrmicro2313
Pinheiro, L. B., Coleman, V. A., Hindson, C. M., Herrmann, J., Hindson, B. J., Bhat, S., et al. (2012). Evaluation of a droplet digital polymerase chain reaction format for DNA copy number quantification. Anal. Chem. 84, 1003–1011. doi: 10.1021/ac202578x
Pusateri, C. R., Monaco, E. A., and Edgerton, M. (2009). Sensitivity of Candida albicans biofilm cells grown on denture acrylic to antifungal proteins and chlorhexidine. Arch. Oral. Biol. 54, 588–594. doi: 10.1016/j.archoralbio.2009.01.016
Qudeimat, M. A., Alyahya, A., Karched, M., Behbehani, J., and Salako, N. O. (2021). Dental plaque microbiota profiles of children with caries-free and caries-active dentition. J. Dent. 104:103539. doi: 10.1016/j.jdent.2020.103539
Richards, V. P., Alvarez, A. J., Luce, A. R., Bedenbaugh, M., Mitchell, M. L., Burne, R. A., et al. (2017). Microbiomes of site-specific dental plaques from children with different caries status. Infect. Immun. 85, e106–e117. doi: 10.1128/iai.00106-17
Robertson, M. A., Kau, C. H., English, J. D., Lee, R. P., Powers, J., and Nguyen, J. T. (2011). MI paste plus to prevent demineralization in orthodontic patients: A prospective randomized controlled trial. Am. J. Orthod.Dentofac. Orthop. 140, 660–668. doi: 10.1016/j.ajodo.2010.10.025
Rosier, B. T., Buetas, E., Moya-Gonzalvez, E. M., Artacho, A., and Mira, A. (2020). Nitrate as a potential prebiotic for the oral microbiome. Sci. Rep. 10:12895. doi: 10.1038/s41598-020-69931-x
Sadeq, A., Risk, J. M., Pender, N., Higham, S. M., and Valappil, S. P. (2015). Evaluation of the co-existence of the red fluorescent plaque bacteria P. gingivalis with S. gordonii and S. mutans in white spot lesion formation during orthodontic treatment. Photodiagnosis. Photodyn. Ther. 12, 232–237. doi: 10.1016/j.pdpdt.2015.03.001
Schoilew, K., Ueffing, H., Dalpke, A., Wolff, B., Frese, C., Wolff, D., et al. (2019). Bacterial biofilm composition in healthy subjects with and without caries experience. J. Oral. Microbiol. 11:1633194. doi: 10.1080/20002297.2019.1633194
Simón-Soro, A., Guillen-Navarro, M., and Mira, A. (2014). Metatranscriptomics reveals overall active bacterial composition in caries lesions. J. Oral. Microbiol. 6:25443. doi: 10.3402/jom.v6.25443
Sun, F., Ahmed, A., Wang, L., Dong, M., and Niu, W. (2018). Comparison of oral microbiota in orthodontic patients and healthy individuals. Microb. Pathog. 123, 473–477. doi: 10.1016/j.micpath.2018.08.011
Tang, Z., Xu, W., Zhou, Z., Qiao, Y., Zheng, S., and Rong, W. (2022). Taxonomic and functional alterations in the salivary microbiota of children with and without severe early childhood caries (S-ECC) at the age of 3. PeerJ. 10:13529. doi: 10.7717/peerj.13529
Thiyahuddin, N. M., Lamping, E., Rich, A. M., and Cannon, R. D. (2019). Yeast species in the oral cavities of older people: A comparison between people living in their own homes and those in rest homes. J. Fungi. 5:30. doi: 10.3390/jof5020030
Thompson, J., and Pikis, A. (2012). Metabolism of sugars by genetically diverse species of oral Leptotrichia. Mol. Oral. Microbiol. 27, 34–44. doi: 10.1111/j.2041-1014.2011.00627.x
Travess, H., Roberts-Harry, D., and Sandy, J. (2004). Orthodontics. part 6: Risks in orthodontic treatment. Br. Dent. J. 196, 71–77. doi: 10.1038/sj.bdj.4810891
Treerat, P., Redanz, U., Redanz, S., Giacaman, R. A., Merritt, J., and Kreth, J. (2020). Synergism between Corynebacterium and Streptococcus sanguinis reveals new interactions between oral commensals. ISME. J. 14, 1154–1169. doi: 10.1038/s41396-020-0598-2
Tufekci, E., Dixon, J. S., Gunsolley, J. C., and Lindauer, S. J. (2011). Prevalence of white spot lesions during orthodontic treatment with fixed appliances. Angle. Orthod. 81, 206–210. doi: 10.2319/051710-262.1
Valm, A. M. (2019). The structure of dental plaque microbial communities in the transition from health to dental caries and periodontal disease. J. Mol. Biol. 431, 2957–2969. doi: 10.1016/j.jmb.2019.05.016
Wang, F., Zhu, L., Liu, B., Zhu, X., Wang, N., Deng, T., et al. (2018). Noninvasive and accurate detection of hereditary hearing loss mutations with buccal swab based on droplet digital PCR. Anal. Chem. 90, 8919–8926. doi: 10.1021/acs.analchem.8b01096
White, T. J., Bruns, T., Lee, S., and Taylor, J. (1990). “Amplification and direct sequencing of fungal ribosomal rna genes for phylogenetics,” in PCR Protocols. A Guide to Methods and Applications, eds M.A. Innis, D. H. Gelfand, J. J. Sninsky, and T. J. White (Cambridge, MA: Academic Press), 315–322.
Xiao, J., Grier, A., Faustoferri, R. C., Alzoubi, S., Gill, A. L., Feng, C., et al. (2018). Association between oral Candida and Bacteriome in children with severe ECC. J. Dent. Res. 97, 1468–1476. doi: 10.1177/0022034518790941
Xiao, J., Moon, Y., Li, L., Rustchenko, E., Wakabayashi, H., Zhao, X., et al. (2016). Candida albicans carriage in children with severe early childhood caries (S-ECC) and maternal relatedness. PLoS One 11:e0164242. doi: 10.1371/journal.pone.0164242
Xu, H., Jenkinson, H. F., and Dongari-Bagtzoglou, A. (2014). Innocent until proven guilty: Mechanisms and roles of Streptococcus–Candidainteractions in oral health and disease. Mol. Oral. Microbiol. 29, 99–116. doi: 10.1111/omi.12049
Xu, H., Sobue, T., Thompson, A., Xie, Z., Poon, K., Ricker, A., et al. (2013). Streptococcal co-infection augments Candida pathogenicity by amplifying the mucosal inflammatory response. Cell Microbiol. 16, 214–231. doi: 10.1111/cmi.12216
Yang, F., Dinis, M., Haghighi, F., He, X., Shi, W., and Chaichanasakul Tran, N. (2022). Oral colonization of Candida albicans and Streptococcus mutans in children with or without fixed orthodontic appliances: A pilot study. J. Dent. Sci. 17, 451–458. doi: 10.1016/j.jds.2021.07.026
Yun, C., Zhiyan, L., Chong, Z., Jing, L., Xin, Z., and Derui, Z. (2019). Illumina-based sequencing analysis of pathogenic microorganisms in dental caries patients of different chinese ethnic groups. J. Int. Med. Res. 47, 5037–5047. doi: 10.1177/0300060519866939
Keywords: microbial community, white spot lesion, fixed orthodontic treatment, Candida albicans, 16S rRNA gene sequencing
Citation: Yang H, Ma Y, Xie X, Wang H, Li X, Fang D and Bai Y (2023) Candida albicans enriched in orthodontic derived white spot lesions and shaped focal supragingival bacteriome. Front. Microbiol. 14:1084850. doi: 10.3389/fmicb.2023.1084850
Received: 31 October 2022; Accepted: 09 January 2023;
Published: 24 January 2023.
Edited by:
Santosh Pandit, Chalmers University of Technology, SwedenReviewed by:
Dongyeop Kim, Jeonbuk National University, Republic of KoreaZhenting Xiang, University of Pennsylvania, United States
Copyright © 2023 Yang, Ma, Xie, Wang, Li, Fang and Bai. This is an open-access article distributed under the terms of the Creative Commons Attribution License (CC BY). The use, distribution or reproduction in other forums is permitted, provided the original author(s) and the copyright owner(s) are credited and that the original publication in this journal is cited, in accordance with accepted academic practice. No use, distribution or reproduction is permitted which does not comply with these terms.
*Correspondence: Yansong Ma, bWF5YW5zb25nMDkxMUAxMjYuY29t; Yuxing Bai,
Ynl1eGluZ0BjY211LmVkdS5jbg==
†These authors have contributed equally to this work and share first authorship