- 1Clinical Microbiology Research Center, Ilam University of Medical Sciences, Ilam, Iran
- 2Department of Microbiology and Immunology, School of Medicine, Kashan University of Medical Sciences, Kashan, Iran
- 3Department of Microbiology, School of Medicine, Ilam University of Medical Sciences, Ilam, Iran
- 4Department of Pediatrics, School of Medicine, Imam Khomeini Hospital, Ilam University of Medical Sciences, Ilam, Iran
- 5Department of Laboratory Sciences, School of Paramedical Sciences, Sabzevar University of Medical Sciences, Sabzevar, Iran
- 6Cellular and Molecular Research Center, Sabzevar University of Medical Sciences, Sabzevar, Iran
Acinetobacter baumannii (A. baumannii) is now considered a highly resistant pathogen to various types of antibiotics. Therefore, tracking the source of its prevalence and continuous control is crucial. This study aimed to determine antibiotic resistance and perform various molecular typing methods on clinical isolates of A. baumannii isolated from hospitalized burn patients in Shahid Motahari Burn Hospital, Tehran, Iran. Hospital isolates were confirmed by phenotypic and molecular methods. Then the sensitivity to different antibiotics was determined using the minimum inhibitory concentration (MIC) method. In order to perform molecular typing, three-locus dual assay multiplex polymerase chain reaction (PCR), multiple-locus variable-number tandem repeat analysis (MLVA), and multilocus sequence typing (MLST) methods were used. Among the 60 isolates collected, the frequencies of multidrug-resistant (MDR) and extensively drug-resistant (XDR) isolates were 90 and 10%, respectively. The most effective antibiotics were colistin with 100% and tigecycline with 83.33% sensitivity. Isolates were 100% resistant to piperacillin/tazobactam and cephalosporins, and 68.3% were resistant to carbapenem. The results of multiplex PCR showed five groups that international clone I (IC I) and IC II were the most common. The MLVA method identified 34 MLVA types (MTs), 5 clusters, and 25 singletons. Multilocus sequence typing results for tigecycline-resistant isolates showed seven different sequence types (STs). Increasing antibiotic resistance in A. baumannii isolates requires careful management to control and prevent the occurrence of the pre-antibiotic era. The results of this study confirm that the population structure of A. baumannii isolates has a high diversity. More extensive studies are needed in Iran to better understand the epidemiology of A. baumannii.
Introduction
Non-fermenting gram-negative bacteria bacterium, Acinetobacter baumannii, is a nosocomial pathogen in hospitals (Gholami et al., 2017; Sato et al., 2021). It can be responsible for a vast range of nosocomial severe infections such as ventilator-associated pneumonia (VAP), bloodstream infections, skin, and soft tissue infections, wound infections, urinary tract infections (UTI), and meningitis (Ayoub Moubareck and Hammoudi, 2020). Owing to high resistance to commonly used antibiotics, more than 80% of Acinetobacter species are regarded as multidrug-resistant (MDR). In addition, because of the inability to treat the infections caused by this bacterium, it has also increased the rate of mortality in patients, imposing a tremendous burden on health care (Khoshnood et al., 2017; Shi et al., 2020). Lately, a dramatic rise has been seen in the number of extensively drug-resistant (XDR) and pandrug-resistant (PDR) A. baumannii isolates as well (Mirnejad et al., 2018; Teerawattanapong et al., 2018).
Due to its considerable threat to public health, the World Health Organization (WHO) has introduced A. baumannii as a crucial priority pathogen toward which the production of new antibiotics is essential (Jiang et al., 2021). Carbapenem antibiotics, such as meropenem and imipenem, are the most effective antibacterial agents for A. baumannii isolates. The rapid spread of carbapenem-resistant MDR A. baumannii (CRAB) has led to the use of other antibiotics. For treatment of clinical MDR bacterial infections, the third-generation tetracycline antibiotic, tigecycline, is used as one of the final-resort antibiotics (Chen et al., 2020). Protein synthesis is adversely affected by hindering aminoacyl-tRNA entry into the aminoacyl (A) site of the 30S ribosome in prokaryotic translation (Ahmad et al., 2019). Tigecycline is prescribed to treat severe infections such as infectious diseases caused by MDR A. baumannii isolates (Owrang et al., 2018).
Nevertheless, resistance to tigecycline and colistin in A. baumannii has increased and is very concerning (Dortet et al., 2018; Hua et al., 2021). Percentages of tigecycline non-susceptibility rates against A. baumannii in Asia have been reported to be 14.2 to 57.6% (Xu et al., 2020). Follow-up research on the susceptibility profile of these two antibiotics is necessary for public health worldwide. The epidemiology analysis of A. baumannii is needed to comprehend its genetic variation and raise awareness about treating and controlling this bacterial infection (Bian et al., 2021). Several effective typing methods have been employed to determine the genetic diversity, strains’ relatedness, and the epidemiology of A. baumannii. One of these typing methods consists of two multiplex polymerase chain reactions (PCRs) to amplify alleles of the gene encoding outer membrane protein A (ompA), the gene encoding part of a pilus assembly system required for biofilm formation (csuE), and the gene encoding the intrinsic carbapenemase gene of A. baumannii (blaOXA-51-like) that can be utilized to recognize international clones (ICs) I, II, and III (Turton et al., 2007). This method performs a preparatory typing approach to rapidly investigate the hospital epidemiology (Giannouli et al., 2009). Other typing methods, multiple-locus variable-number tandem repeat analysis (MLVA), and multilocus sequence typing (MLST), can be mentioned as well (Farshadzadeh et al., 2015). An interesting source of genetic polymorphism is provided by tandemly repeated sequences, known as variable number of tandem repeats (VNTRs), whose number of repetitions varies at different rates depending on the different loci and even alleles. Molecular typing based on the analysis of repeat copy number at multiple VNTR loci, known as MLVA, is a genotyping method that is being used for strain comparison, and can also provide insights into population structure (Dahyot et al., 2018). This method has some benefits such as high resolution, data portability, and intra-laboratory reproducibility (Lindstedt et al., 2013). Multilocus sequence typing described as a nucleotide sequence-based method providing an allelic profile or a sequence type (ST) for each isolate, is now extremely considered a supplementary tool for global epidemiological research that offers possibilities for the identification of epidemics and virulence of A. baumannii clones and the monitoring of their spread at both the national and international levels (Khuntayaporn et al., 2021). Both approaches (MLVA and MLST) allow for identification of clonal lineages and the investigation of genetic diversity of the clinical A. baumannii clones (Hauck et al., 2012). The main aim of the current study was to evaluate the antibiotic resistance and molecular typing of clinical isolates of A. baumannii collected from hospitalized burn patients in Shahid Motahari Burn Hospital, Tehran, Iran.
Materials and methods
Bacterial isolates and identification
This cross-sectional study received ethical approval from the local ethics committee of Ilam University of Medical Sciences (IR.MEDILAM.REC.1400.135). All patients provided written informed consent. From the beginning of October 2020 to the end of July 2021, 60 non-duplicate A. baumannii isolates were obtained from 111 various clinical specimens (catheter, pleural fluid, tracheal aspirates, blood, cerebrospinal fluid (CSF), and urine) taken from hospitalized patients in Shahid Motahari burn hospital in Tehran, Iran. Isolates were streaked on blood agar (blood agar base by Merck Chemicals, Darmstadt, supplemented with 5% sheep blood) and MacConkey agar (Merck, Darmstadt, Germany) and incubated aerobically at 37°C for 24 h. Primary identification A. baumannii isolates was based on the colony morphology and Gram staining reaction. Standard biochemical tests such as citrate, oxidase, catalase, triple sugar iron agar, methyl red, Voges Prausker, urease test, oxidation and fermentation of sugars, and indole production were used to identify the A. baumannii isolates (Forbes, 2007). Identification of A. baumannii was confirmed by PCR detection of the blaOXA-51-like gene according to Turton et al. (Turton et al., 2006) and gyrB gene based on the method of Higgins et al. (Higgins et al., 2007). The A. baumannii ATCC 19606 was used as a positive control.
Susceptibility testing
Minimum inhibitory concentrations (MICs) were determined using the VITEK 2 system (bioMérieux, Marcy l’Etoile, France) for the following seven antimicrobial categories: β-lactam/β-lactamase inhibitors combinations (Piperacillin/tazobactam PIP/TAZ), extended-spectrum cephalosporins (ceftazidime (CAZ), cefepime (CEP)), carbapenems (imipenem (IMI), meropenem (MER), Lipopeptide (colistin, COL), aminoglycosides (gentamicin(GEN), tobramycin (TOB), tetracyclines (tigecycline (TGC), tetracycline (TET), and fluoroquinolones (ciprofloxacin (CIP). The results were evaluated according to the Clinical and Laboratory Standards Institute (CLSI) criteria (Wayne, 2020), except for tigecycline, in which case the results were evaluated according to the criteria suggested by Jones et al. (2007); MIC ≥8 μg/ml, as the resistant breakpoint. Multidrug resistance profile as defined by the isolate being non-susceptible to at least one agent in ≥3 antimicrobial categories. Isolates of A. baumannii with resistance to at least one agent in all but two or fewer antimicrobial categories were considered XDR (Magiorakos et al., 2012). Reference strains Escherichia coli ATCC 25922 and Pseudomonas aeruginosa ATCC 27853 were used as quality controls.
Molecular typing methods
Multiplex PCR
The sequence group (SG) or IC type of the isolates were determined by using two multiplex PCR assays designed to selectively amplify ompA, csuE and blaOXA-51-like genes (Turton et al., 2007). The PCR tests were carried out in a final volume of 25-μL containing 3 μl of extracted DNA, 10 pmol of each primer and 1.5 U of Taq DNA polymerase in 1 × PCR buffer containing 1.5 mM MgCl2 (Qiagen, Crawley, United Kingdom) and 200 μM each dNTP. PCR mixtures were subjected to the following thermal cycling: 3 min at 94°C, followed by 30 cycles with denaturation at 94°C for 45 s, annealing at 46–65°C for 45 s, extension at 72°C for 1 min, and a final extension at 72°C for 5 min (Turton et al., 2007). Identification of a strain as a member of Group 1 (G1) or Group 2 (G2) required the amplification of all fragments in the corresponding multiplex PCR and an absence of any amplification by the other multiplex PCR. Group 3 (G3) isolates were defined by the amplification of only the ompA fragment in the Group 2 PCR and the amplification of only the csuE and blaOXA-51-like fragments in the Group 1 PCR (For other groups, see Supplementary File 1). According to the new combination of amplified products, and isolates pertained to the novel variant of the PCR-based group. Isolates were grouped in G1 (IC II), G2 (IC I), and G3 (IC III). Other isolates that did not belong to these types were reported as the IC variants.
Multiple loci VNTR analysis
Isolates were genotyped using the MLVA-8 scheme method developed by Pourcel et al. (2011). The MLVA-8 scheme profiles in each isolate were identified by the number of repeats estimated at each VNTR locus. The bacterial genomic DNA was extracted using the boiling method (Gomaa et al., 2017) and its concentration was assessed using a NanoDrop ND-1000 spectrophotometer (NanoDrop Technologies, Wilmington, DE, USA). Oligonucleotide primers targeting the flanking regions of the S-repeat VNTRs (Abaum3468, Abaum0845, Abaum0826, and Abaum2396) and L-repeat VNTRs (Abaum-3,530, Abaum3002, Abaum2240, and Abaum1988) were used for the amplification of A. baumannii genomic DNA. Small (S)-repeat VNTRs were the loci with a repeat unit size of up to 9 bp, whereas L (Large)-repeat VNTR loci were the loci with a repeat unit size of above 9 bp. In the following, PCRs were performed in a 25 μl final volume containing 20 pM of each primer, 200 μM of each deoxynucleoside triphosphate (dNTPs), 1.5 mM MgCl2, 3 ng genomic DNA, ×1′ reaction buffer, and1Uof Taq DNA polymerase. Amplification cycles included an initial 94°C denaturation for 5 min, followed by 36 cycles of 94°C for 30, 30 s of annealing at 50°C for Abaum0826 and Abaum0845 and at 55°C for the remainder loci, elongation at 72°C for 30 s, and a final elongation at 72°C for 10 min. Then, 5 μl of each PCR amplicon solution were analyzed on 3% agarose gel (SinaClon BioScience, Iran) by electrophoresis in 1× Tris–borate-EDTA (TBE) buffer. Size markers included a 50-bp DNA standards (Thermo Scientific) and 100-bp (SinaClon Bioscience), for S- and L-VNTRs, respectively. The size of the amplicon was determined using GeneTools v.3.08 automatic image analysis software (Syngene, Cambridge, United Kingdom). The number of repeats in VNTR alleles for isolates was estimated by subtracting the flanking region size from the amplicon size and then dividing by the repeat unit length. Primers, PCR conditions and characteristics of VNTRs used in this study are shown in Supplementary File 2. A cut-off value of 90% similarity was applied to define clusters, and MLVA type (MT) was determined using a 100% similarity cut-off, as previously described (Nhu et al., 2014).
Multilocus sequence typing
Seven housekeeping genes (gltA, gyrB, gdhB, recA, cpn60, gpi, and rpoD) were amplified and sequenced to determine the genotype. DNA sequence variations and STs were analyzed using the MLST database for A. baumannii.1 MLST was performed using the Oxford scheme as previously described (Bartual et al., 2005). This typing method was carried out for isolates with resistance to tigecycline (MICs ≥8 μg/ml).
Statistical analysis
Statistical analysis was performed using the “IBM SPSS statistics 22” software (IBM analytics; United States). Statistical significance of variables determined by chi-square and Fisher’s exact tests. The results are presented as descriptive statistics in terms of relative frequency. A p-value of ≤0.05 was considered statistically significant.
Results
Studied population and bacterial isolates
A. baumannii isolates were identified by various tests that included: Gram-negative coccobacilli, oxidase negative, catalase positive, non-fermentative (oxidative), urease negative, non-fastidious, gas negative, citrate positive, H2S negative, indole negative, methyl red positive, and Voges-Proskauer negative. In the present study, 60 non-duplicative A. baumannii isolates were collected from 23 (38.3%) females and 37 (61.6%) males with the mean age of 41.4 ± 9 (range 4–75) years, and with the maximum number of cases in the age group of 41 to 60 years (n = 28). These isolates were obtained from different clinical specimens, including catheter (23, 38.3%) pleural fluid (7, 11.6%), tracheal aspirates (18, 30%), blood (6, 10%), CSF (3, 5%), and urine (3, 5%). All the 60 isolates originated from the following wards: (5, 8.3%) from infectious diseases, as well as (2, 3.3%) from pediatrics, (11, 18.3%) from surgery, (3, 5%) from urology, (4, 6.6%) from general and (35, 58.3%) from intensive care unit (ICU).
Antibiotic susceptibility testing
The results showed that colistin and tigecycline with the sensitivity rates of 100% (MICs range from 0.125 to 1 μg/ml) and 83.33% (MICs range from 0.25 to 4 μg/ml) were the most active antibiotics, respectively, while PIP/TAZ and cephalosporins with 100% resistance had no effect on A. baumannii isolates (Table 1). The MIC range of tigecycline in all resistant A. baumannii isolates were from 8 to 64 μg/ml. Besides, resistance to other antibiotics was ≥65% (ciprofloxacin = 86.66%, imipenem = 65%, meropenem = 68.33%, gentamicin = 86.66%, tobramycin: 68.33% and tetracycline: 70%). According to antibiotic susceptibility testing, among 60 A. baumannii isolates screened, 54 (90%) and 6 (10%) were MDR and XDR, respectively. Also, 41 isolates (68.33%) were CRAB with the MICs range from 8 to ≥256 μg/ml.
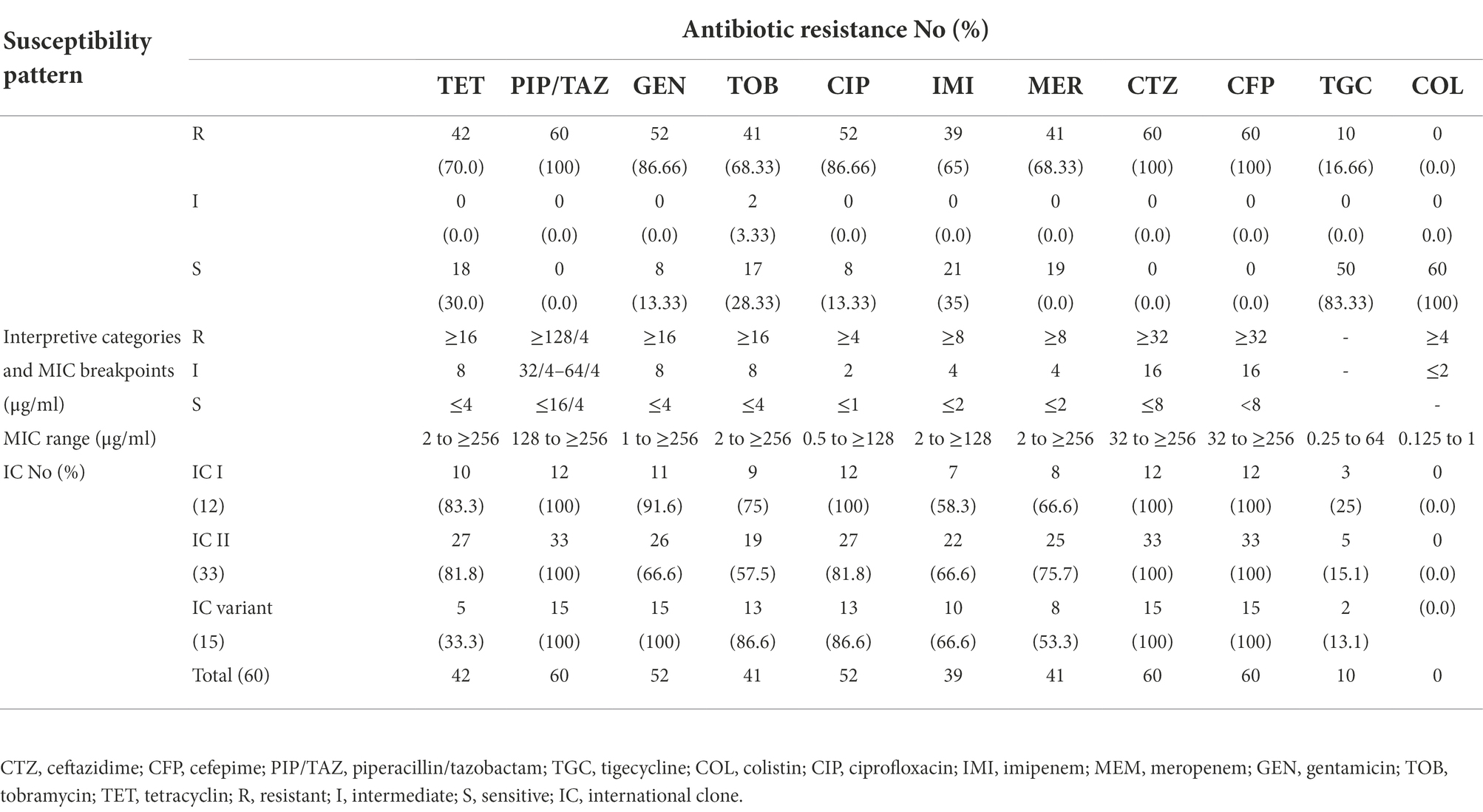
Table 1. Antibiotic susceptibility pattern of 60 A. baumannii isolates using MIC determination against 11 different antibiotics.
Molecular typing
International clone type analysis indicated five PCR-based groups (G1, G2, G9, G10, and G16) among A. baumannii isolates. Twelve (20%) and 33 (55%) isolates belonged to G2 (IC I) and G1 (IC II), respectively. Group 3 was not found in any of the isolates. As for other isolates, they belonged to 3 IC variants PCR-based groups, including 2 (3.3%), 5 (8.3%), and 8 (13.3%), and to G9, G10, and G16, respectively. The frequency of antibiotic resistance in three epidemic lineages is shown in Table 1. More than 55% of the isolates belonged to IC I and IC II were resistant to all the antibiotics, except for colistin and tigecycline. Overall, by the MLVA typing method, 60 A. baumannii isolates were grouped into 34 distinct MTs with 5 clusters and 25 singleton genotypes. The most isolates were in cluster 1(n = 19) and cluster 4 (n = 12), respectively. The most MTs were in cluster 4 (n = 7 MTs; Figure 1). In this study, the VNTR loci MLVA-AB_2,396 (Number of different repeats, n = 5), MLVA-AB_3,002 (n = 4) and MLVA-AB_0845 (n = 4) showed a high level of diversity (Figure 2). While most MTs had a single member, the three main MTs were MT30 (n = 4), MT6 (n = 4), MT4 (n = 6), and MT3 (n = 9), which together comprised over 35% of all isolates. MLST analysis for isolates with resistance to tigecycline revealed a total of seven different STs. Among these isolates, four isolates belonged to clonal complex 92 (CC 92; Ab 43, Ab 6, Ab 20, and Ab 52, corresponding to ST118). These isolates had a MIC range: 8–64 μg/ml and belonged to IC G1, G2, G10, and G16.
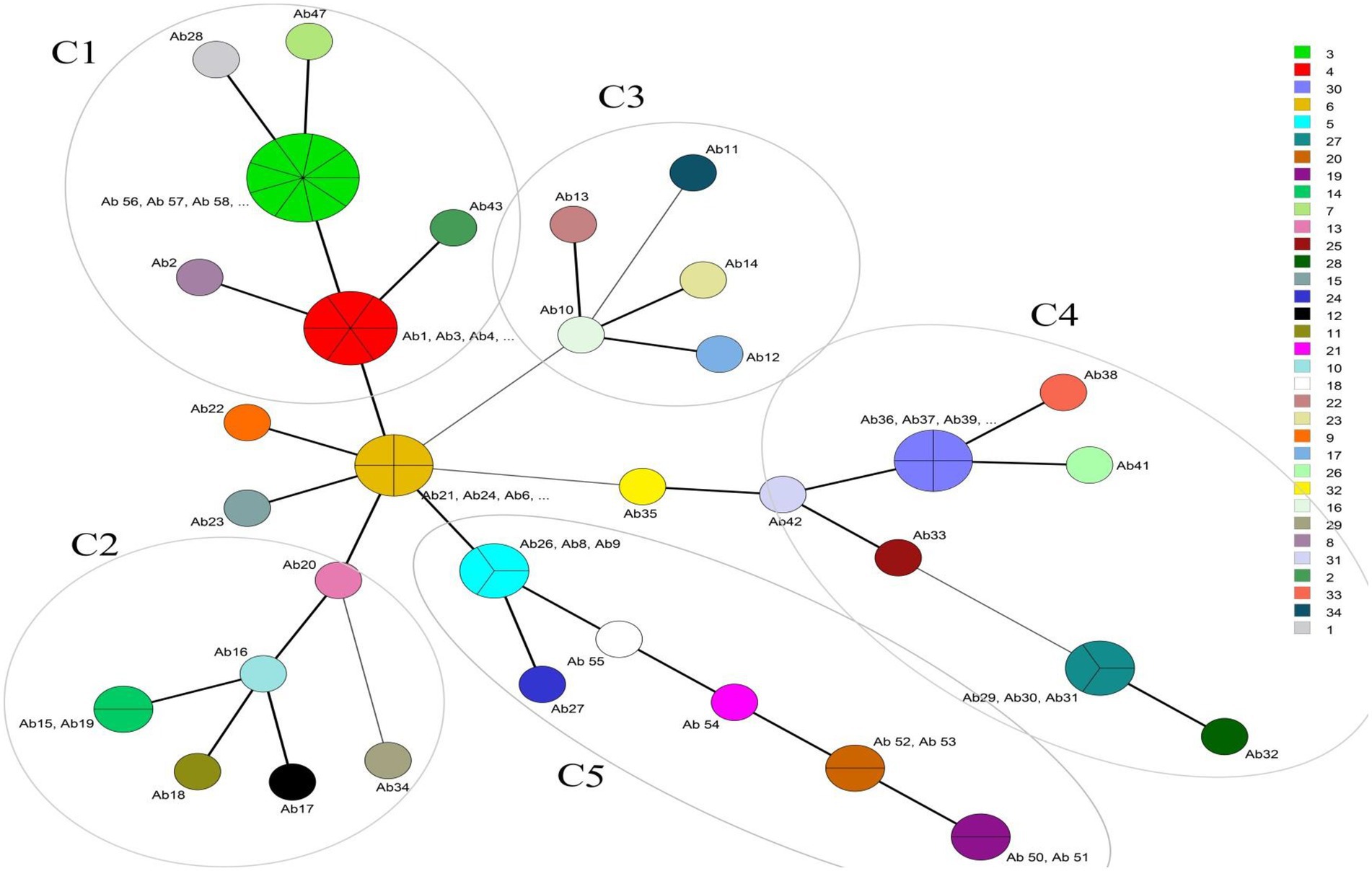
Figure 1. Minimum spanning tree of 60 A. baumannii isolates detected for MLVA types (MTs). The right column shows different MTs. Each circle represents isolate number (Ab). The size of each circle indicates the number of isolates within these MTs. Strains that fall into the same MT type are shown with the same color. For example Ab1, Ab3, Ab4, Ab5, Ab44, and Ab45 are located in MT4 because they have the same VNTR numbers (6 7 5 5 9 12 8 12). The different clusters (C1-C5) are annotated. The most isolates were in cluster 1(C1, n = 19) and cluster 4 (C4, n = 12), respectively. The highest diversity of MTs is in cluster 4. Thick and short lines connecting two types denote types differing in a single locus; thin, longer lines connect double-locus variants; and dashed lines indicate the most likely connection between two types differing in more than two loci.
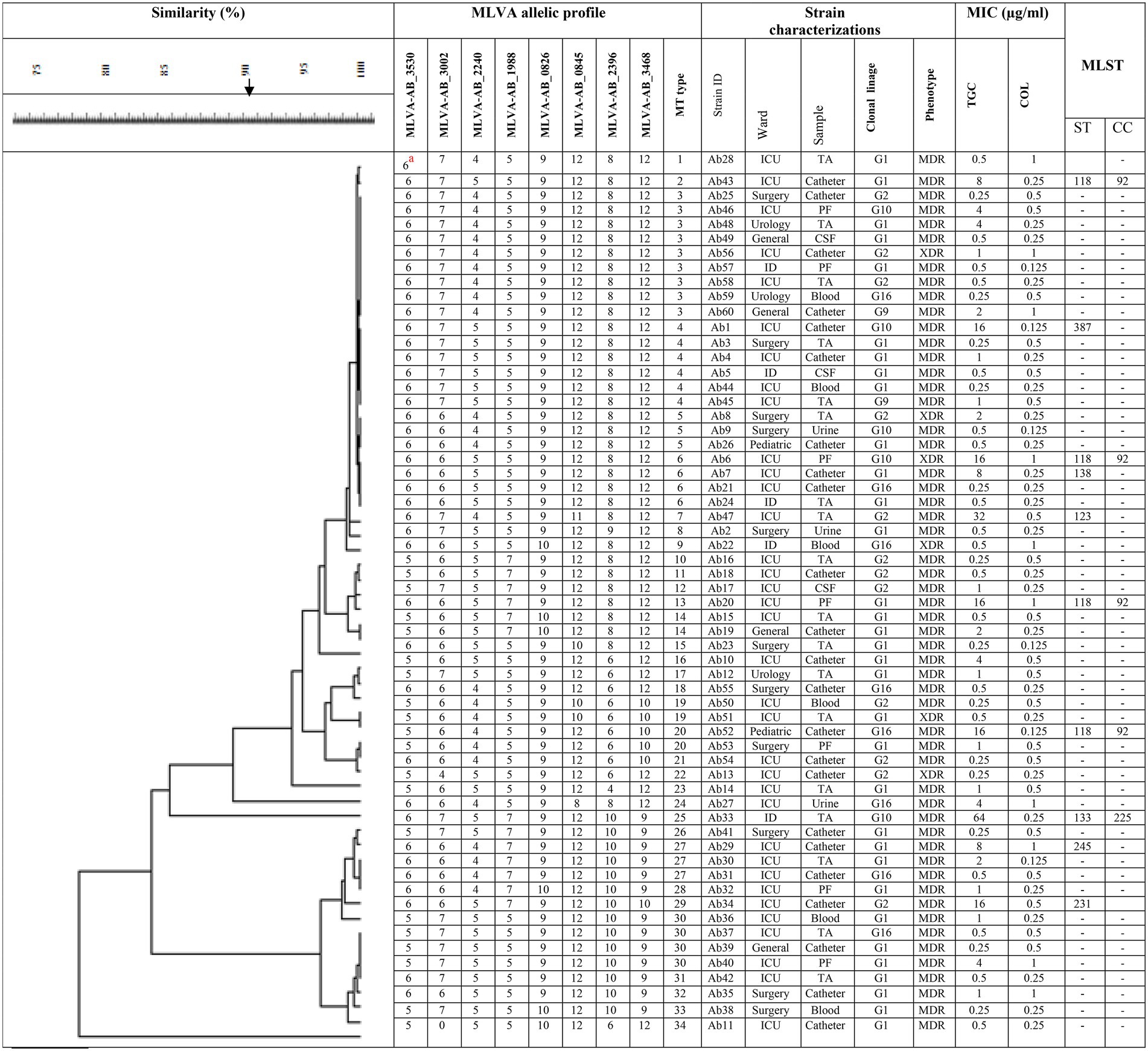
Figure 2. Dendogram shows the genetic diversity of 60 A. baumannii isolates by MLVA and MLST. Ab 1, A. baumannii number 1; TA, tracheal aspirates; PF, pleural fluid; IC,: International clonal linage; MDR, multidrug resistant; XDR, extensively drug-resistant; MIC, minimum inhibitory concentration; TGC, tigecycline; COL, colistin; CC, clonal complex; ST, sequence type. aThe number of repeats in VNTR alleles for each isolate.
Discussion
A. baumannii has become a main hospital pathogen, due to MDR strains and it is now considered one of the most important bacteria that causes hospital-acquired infections (HAIs), with mortality rate from 8 to 35% according to type of infection and strain as well as increase in health care expenditures and hospital stays (Antunes et al., 2014; Cornejo-Juárez et al., 2020). It is noteworthy that A. baumannii infection increases the mortality rate in the ICU (Playford et al., 2007; Heidary et al., 2017). A. baumannii is classified as an ESKAPE hospital pathogen due to its resistance to colistin and tigecycline. Since A. baumannii is an immediate threat to human health, a new antibiotic design is needed (Whiteway et al., 2022). The reason for antibiotic resistance is the acquisition of resistance genes through integrons from the environment or intra-species (Liu et al., 2014). Antibiotic resistance, high environmental stability, and failure to identify harmful toxins in the host and its genome indicate the potential for pathogenicity of A. baumannii based on “persist and resist” (Whiteway et al., 2022). If a patient is suspected of having antibiotic resistance, appropriate bacterial susceptibility testing should be performed, especially for patients admitted to the ICU. Imipenem susceptibility testing is necessary if the patient has previously used carbapenems and glycopeptides. In order to reduce the emergence and spread of PDR, continuous monitoring of A. baumannii resistance should be performed (Xie et al., 2018). In this study, multiplex PCR, MLVA, and MLST were performed to evaluate the clonality of A. baumannii. Also, the effectiveness of different antibiotics on hospital isolates was investigated. The results indicate that none of the strains were sensitive to the piperacillin/tazobactam and cephalosporins. There are different results for piperacillin/tazobactam resistance from around the world. Resistance is reported at 94% in the Poland (Grochowalska et al., 2017), and the sensitivity was 33.3% in India (Tewari et al., 2018).
Bacteria use different strategies to fight antibiotics (Robatjazi et al., 2021) such as the efflux pump that plays an important role in the emergence of multiple resistance to antibiotics especially in A. baumannii (Basatian-Tashkan et al., 2020). At present, MDR and XDR Acinetobacter have high frequency all over the world (Tafreshi et al., 2019). For example in a study that conducted in Iran, the frequency of MDR and XDR of A. baumannii was reported to be 94.2 and 71.2%, respectively (Maspi et al., 2016). In our study, the frequencies of MDR and XDR isolates were 90 and 10%, respectively. The difference between the results is probably due to geographical differences, the lack of a standard pattern in the definition of MDR and XDR (Magiorakos et al., 2012) and the different origin of the isolates. Over the past decade, carbapenem has been effective against A. baumannii and used as an alternative treatment for MDR infections (Ying et al., 2015). However, due to the high consumption of carbapenem, its resistance has increased (Ying et al., 2015). There are reports from all over the world of high resistance to carbapenems (Rynga et al., 2015; Nowak et al., 2017). The frequency of carbapenem-resistant isolates in the present study was 68.3%. In a systematic review meta-analysis that examined studies published in Iran between 1995 and 2017 on carbapenem-resistant isolates, the pooled frequency of carbapenem resistance was reported to be 85.1%. Among Iran’s neighbors, such as Afghanistan, Turkey, Pakistan, and Iraq, there was a high rate of carbapenem resistance in A. baumannii (Nasiri et al., 2020). The difference observed in the results of our study and the aforementioned study can be due to various reasons. The difference in the number of patients in the two studies can be one of these reasons. Another reason can be attributed to the fact that although all the studies included in the systematic review are from Iran, they are not from all parts and cities of Iran and perhaps for this reason, different percentages of the prevalence are seen in the two studies. In a report by El-Mahdy et al., 100% of A. baumannii isolates were resistant to cephalosporins (El-Mahdy et al., 2017). The results of the study by Safari and her colleagues in 2015 in Iran showed that the resistance to ceftazidime and cefotaxime was 95 and 100%, respectively (Safari et al., 2015). According to the results obtained from the present study, the similarity in the high resistance of A. baumannii to cephalosporin is determined in both studies.
Tigecycline is a new Glycycline that works very well against A. baumannii. Tigecycline was licensed first in the United States and later in Canada and the Middle East (Karmostaji et al., 2014). With its bacteriostatic activity, tigecycline is considered as the last line of treatment for MDR isolates (Zhang et al., 2022). Colistin and tigecycline are the main choices for treating CRAB, but there are concerns about their toxicity and effectiveness (Ying et al., 2015). In cases where colistin and imipenem-resistant A. baumannii are reported, tigecycline was used as a treatment option for the patient (Cai et al., 2012; Sarada et al., 2014). In 2006, all strains isolated in Iran were susceptible to tigecycline, but between 2011 and 2014, resistance to this antibiotic increased (Taheri et al., 2022). In a study conducted by Mohammadi Bardbari et al. in Iran, all clinical and environmental isolates of A. baumannii were sensitive to colistin and tigecycline (Mohammadi Bardbari et al., 2020). Rahimi et al. in Iran examined 80 isolates, 100% sensitive to colistin, and 6% resistant to tigecycline (Rahimi et al., 2018). The results of the present study confirm that tigecycline and colistin are still the most effective antibiotics, which is consistent with previously published reports (Salehi et al., 2018; Tewari et al., 2018; Boral et al., 2019). With the exception of a few studies that have reported high resistance of A. baumannii to tigecycline, the highest range of resistance to tigecycline in Iran has been reported between 2 and 38.4% (Razavi Nikoo et al., 2017). In a study by Morfin-Otero et al. on A. baumannii strains from around the world, a slight increase in MIC90 for tigecycline was shown to confirm the effectiveness of tigecycline remained more stable than that of other microbial agents between 2004 and 2009 (Morfin-Otero and Dowzicky, 2012). In a similar study by Mendes et al. on global A. baumannii spp., >90% of all A. baumannii strains and 95% of MDR A. baumannii were inhibited by tigecycline at ≤2 mg/L (Mendes et al., 2010).
In this study, 83.33% of all A. baumannii strains were inhibited at ≤2 μg/ml of tigecycline. According to the results obtained from the above studies and our study, it is considered that tigecycline can still be used as an effective antibiotic in the treatment of A. baumannii infections, but to prevent the occurrence of antibiotic resistance, the use of this antibiotic should be controlled and done carefully. Since A. baumannii has genomic flexibility and heterogeneous resistance, this facilitates the emergence of resistance to tigecycline and colistin.
The global population structure of A. baumannii is changing, so molecular typing is important. So far, different G variants have been responsible for infections acquired from the hospital in different areas, of which 5–24% of these variants have been resistant to tigecycline (Taheri et al., 2022). In previous studies in Iran, G1 (IC II) was the most dominant clonal lineage of A. baumannii (Hojabri et al., 2014; Taheri et al., 2022), which is consistent with our study. In the study of Taheri et al. (2022), conducted in Iran, it was found that the G variants rate had an increasing trend (4–43%) between 2006 and 2015. Also, the resistance to tigecycline from 2013 to 2015 had an increasing trend of 22–45% among the isolates of G variants. The results also showed an increase in XDR among these lineages. Therefore, the prevalence of antibiotic-resistant phenotypes could be a reason for the increase in G variants over time. The increase in tigecycline and XDR resistance in the G variants indicates that some of their components are shared (Taheri et al., 2022).
IC II is the most scattered lineage ever identified in at least 34 countries. IC I and IC II are also called global clones (GC; Gaiarsa et al., 2019). Initially, three high-resistance ICs were identified (IC1,2 and 3), which are responsible for the MLST IP/UO (Institute Pasteur/University of Oxford) scheme to CCs 1/109, 2/118, and 3/187 (Martins et al., 2013). In the present study, the results of multiplex PCR showed five PCR-based or IC lineage groups (G1, G2, G9, G10, and G16), among which IC II and IC I were the most common. In the study conducted by Farshadzadeh et al., 8 IC types (G1, G2, G6, G9, G10, and G15–G17) were reported, the most common of which were IC II and IC I, respectively. They also found that IC variants had the highest resistance to tigecycline (Farshadzadeh et al., 2015). In a study conducted by Bahador et al. in Iran, IC-V was the most common, followed by IC II (Bahador et al., 2018), while in the study of Hojabri et al., IC II was the most common (Hojabri et al., 2014).
Also, in another study conducted in 2012 by Bahador et al. in Iran, IC II (58%) and IC I (29%) were the most common lineages (Bahador et al., 2015). Similarly, in a study conducted in Japan, the prevalence of IC II was higher than in non-IC II lineages (Matsui et al., 2018). A. baumannii belongs to IC I and IC II in connection with the high prevalence of hospitalization and virulence than others. Some of the surface features of these two lineages are different from each other, which may be a reason for the difference in their prevalence. For example, isolates belonging to IC II are immobile, do not form a pellicle, and have distinct polysaccharide capsules compared to IC I. IC I also has a very high hydrophobic property (Skerniškytė et al., 2019).
The basis of MLVA is determining the number of tandem repeat that are often located in the noncoding regions of the genome (Jenke et al., 2011). Multiple-locus variable-number tandem repeat analysis results indicate that isolates that are in the same G-type may be in different MLVAs or vice versa (Taheri et al., 2022). In studies in Iran (Najar Peerayeh and Karmostaji, 2015) and France (Hauck et al., 2012), due to partial deletion of locus, Abaum3468, Abaum3002, Abaum3530, and Abaum0826 had an amplification failure. In our study, the number of MTs was 34 different types, but in a similar study in Iran, 32 MTs were reported (Bahador et al., 2018). In the study of Azimi et al., there were five different molecular typing algorithms based on the size of VNTR (Azimi et al., 2016). In the present study, MLVA-AB-2396, MLVA-AB-3002, and MLVA-AB-0845 had the highest diversity, while in other studies conducted in Iran (Najar-Peerayeh and Karmostaji, 2019) and Spain (Villalón et al., 2015), MLVA-AB_0845 had the highest diversity. In another study conducted by Farshadzadeh and his colleagues in Iran, none of the CRAB A. baumannii isolates contained MLVA-AB_0845; instead, in their study, MLVA-AB_2,396 and MLVA-AB_3468 had the highest diversity (Farshadzadeh et al., 2015). In a study conducted by Karimi et al. in Iran, A. baumannii isolates were isolated from human and animal samples, and their typing pattern was determined using MLVA. Their research showed that 0845, 0826, and 3,406 VNTRs loci were present in all animal isolates and 3,406 VNTR loci were present in all human isolates (Karimi et al., 2021).
In a study conducted in Lithuania, it was found that there is no relationship between the specific years in which the study was conducted and the clustering of the isolates. On the other hand, it was found that most varieties belonged to Abaum_0845 and Abaum_0826 (Kirtikliene et al., 2021). A study conducted by Peerayeh et al. showed that different strains are circulating in two Iranian hospitals and the population of XDR A. baumannii in Iranian hospitals is genetically diverse, which necessitates preventive measures and strict control (Najar Peerayeh and Karmostaji, 2015). Multilocus sequence typing is a convenient way to describe bacterial populations and has the ability to cluster isolates into large clones (Villalón et al., 2015; Wareth et al., 2021). Therefore, with the reproducibility of this technique, the isolates can be compared globally with each other, and each can be placed directly in its CC (Rafei et al., 2014). In the present study, tigecycline-resistant isolates were placed in 7 different STs, 4 of which belong to CC92. In the study of Farshadzadeh and his colleagues, various STs from Iran were identified, among which ST118 was dominant (Farshadzadeh et al., 2015).
The results of various studies conducted in Iran have introduced CC92 as a common clone (Hojabri et al., 2014; Farshadzadeh et al., 2015) and has a global superiority (Ying et al., 2015). In a study conducted in China by Chang et al., CC92 was reported to be the most common CC resistant to carbapenem (Chang et al., 2015). Another study in China identified five different STs, and 85.2% of all clinical isolates belonged to CC92 (Ying et al., 2015). In a study conducted by Saffari et al. in Iran between 2014 and 2015, three main STs (ST848, ST451, and ST195) were identified, all of which belonged to CC92. None of the identified STs in Saffari’s study previously existed in Iran. However, the critical point is that ST195 was identified in the Arab countries of the Persian Gulf, so it seems that the emergence of this strain in Iran has occurred due to the transfer of microbial resistance from neighboring countries (Saffari et al., 2017). In another study conducted by Abhari et al. in Iran in 2017, the highest prevalence of clones belonged to ST10, followed by ST2, ST3, and ST513. ST10 was first identified in China and is highly virulent and associated with prevalence. The results of the above studies show that the prevalence of clones varies in each hospital and the geographical area (Abhari et al., 2019). There are several reasons for the prevalence of CC92, including high compatibility and survival, and resistance to antibiotic stress (Chang et al., 2015).
Conclusion
Antibiotic resistance is an important issue of public health that threatens to undermine decades of medical progress. A. baumannii, one of the causes of nosocomial infections, is frequently resistant to carbapenem antibiotics, usually leaving colistin and tigecycline as the last-resort antibiotics. However, increasing tigecycline resistance and colistin’s nephrotoxicity severely restrict the use of these antibiotics. Finally, the results obtained from this study showed that the frequency of IC2 was much higher than other ICs, and considering the important role of this lineage in the occurrence of antibiotic resistance. Continuous investigation and tracking of different lineages to find resistance patterns is recommended. The diverse A. baumannii lineages in the current study highlight the need for designing an adequate surveillance program in each country based on the main circulating clone to select the appropriate therapy. Governments, researchers, and pharmaceutical companies should also be encouraged to design new antibiotics, given the growing resistance in A. baumannii and other pathogenic bacteria. On the other hand, controlling and monitoring the use of antibiotics in the treatment of A. baumannii infections plays an important role in controlling the prevalence of resistance.
Data availability statement
The original contributions presented in the study are included in the article/Supplementary material, further inquiries can be directed to the corresponding authors.
Ethics statement
The studies involving human participants were reviewed and approved by IR.MEDILAM.REC.1400.135. Written informed consent to participate in this study was provided by the participants’ legal guardian/next of kin.
Author contributions
AM and SKh contributed to the study conception and design. Data collection and analysis were performed by SKh, BK, VK, SKa, NO, and MHas. The first draft of the manuscript was written by SKh, AM, MHad, and MK and all authors commented on previous versions of the manuscript. All authors contributed to the article and approved the submitted version.
Acknowledgments
We would like to thank the Clinical Microbiology Research Center, Ilam University of Medical Sciences, for their cooperation. Our appreciation goes to the Vice Chancellor for Research Affairs, Ilam University of Medical Sciences, Ilam, Iran, for their executive and financial support.
Conflict of interest
The authors declare that the research was conducted in the absence of any commercial or financial relationships that could be construed as a potential conflict of interest.
Publisher’s note
All claims expressed in this article are solely those of the authors and do not necessarily represent those of their affiliated organizations, or those of the publisher, the editors and the reviewers. Any product that may be evaluated in this article, or claim that may be made by its manufacturer, is not guaranteed or endorsed by the publisher.
Supplementary material
The Supplementary material for this article can be found online at: https://www.frontiersin.org/articles/10.3389/fmicb.2022.994303/full#supplementary-material
Footnotes
References
Abhari, S. S., Badmasti, F., Modiri, L., Aslani, M. M., and Asmar, M. (2019). Circulation of imipenem-resistant Acinetobacter baumannii ST10, ST2 and ST3 in a university teaching hospital from Tehran, Iran. J. Med. Microbiol. 68, 860–865. doi: 10.1099/jmm.0.000987
Ahmad, S., Ranaghan, K. E., and Azam, S. S. (2019). Combating tigecycline resistant Acinetobacter baumannii: a leap forward towards multi-epitope based vaccine discovery. Eur. J. Pharm. Sci. 132, 1–17. doi: 10.1016/j.ejps.2019.02.023
Antunes, L., Visca, P., and Towner, K. J. (2014). Acinetobacter baumannii: evolution of a global pathogen. Pathog. Dis. 71, 292–301. doi: 10.1111/2049-632X.12125
Ayoub Moubareck, C., and Hammoudi, H. D. (2020). Insights into Acinetobacter baumannii: a review of microbiological, virulence, and resistance traits in a threatening nosocomial pathogen. Antibiotics. 9:119. doi: 10.3390/antibiotics9030119
Azimi, L., Talebi, M., Khodaei, F., Najafi, M., and Lari, A. R. (2016). Comparison of multiple-locus variable-number tandem-repeat analysis with pulsed-field gel electrophoresis typing of carbapenemases producing Acinetobacter baumannii isolated from burn patients. Burns 42, 441–445. doi: 10.1016/j.burns.2015.08.024
Bahador, A., Farshadzadeh, Z., Raoofian, R., Mokhtaran, M., Pourakbari, B., Pourhajibagher, M., et al. (2018). Association of virulence gene expression with colistin-resistance in Acinetobacter baumannii: analysis of genotype, antimicrobial susceptibility, and biofilm formation. Ann. Clin. Microbiol. Antimicrob. 17:24. doi: 10.1186/s12941-018-0277-6
Bahador, A., Raoofian, R., Farshadzadeh, Z., Beitollahi, L., Khaledi, A., Rahimi, S., et al. (2015). The prevalence of ISAba1 and ISAba4 in Acinetobacter baumannii species of different international clone lineages among patients with burning in Tehran, Iran. Jundishapur J. Microbiol. 8:e1716. doi: 10.5812/jjm.17167v
Bartual, S. G., Seifert, H., Hippler, C., Luzon, M. A. D., Wisplinghoff, H., and Rodríguez-Valera, F. (2005). Development of a multilocus sequence typing scheme for characterization of clinical isolates of Acinetobacter baumannii. J. Clin. Microbiol. 43, 4382–4390. doi: 10.1128/JCM.43.9.4382-4390.2005
Basatian-Tashkan, B., Niakan, M., Khaledi, M., Afkhami, H., Sameni, F., Bakhti, S., et al. (2020). Antibiotic resistance assessment of Acinetobacter baumannii isolates from Tehran hospitals due to the presence of efflux pumps encoding genes (adeA and adeS genes) by molecular method. BMC Res. Notes 13:543. doi: 10.1186/s13104-020-05387-6
Bian, X., Liu, X., Zhang, X., Li, X., Zhang, J., Zheng, H., et al. (2021). Epidemiological and genomic characteristics of Acinetobacter baumannii from different infection sites using comparative genomics. BMC Genomics 22, 1–13. doi: 10.1186/s12864-021-07842-5
Boral, B., Unaldi, Ö., Ergin, A., Durmaz, R., and Eser, Ö. K. (2019). A prospective multicenter study on the evaluation of antimicrobial resistance and molecular epidemiology of multidrug-resistant Acinetobacter baumannii infections in intensive care units with clinical and environmental features. Ann. Clin. Microbiol. Antimicrob. 18:19. doi: 10.1186/s12941-019-0319-8
Cai, Y., Chai, D., Wang, R., Liang, B., and Bai, N. (2012). Colistin resistance of Acinetobacter baumannii: clinical reports, mechanisms and antimicrobial strategies. J. Antimicrob. Chemother. 67, 1607–1615. doi: 10.1093/jac/dks084
Chang, Y., Luan, G., Xu, Y., Wang, Y., Shen, M., Zhang, C., et al. (2015). Characterization of carbapenem-resistant Acinetobacter baumannii isolates in a Chinese teaching hospital. Front. Microbiol. 6:910. doi: 10.3389/fmicb.2015.00910
Chen, C., Cui, C.-Y., Yu, J.-J., He, Q., Wu, X.-T., He, Y.-Z., et al. (2020). Genetic diversity and characteristics of high-level tigecycline resistance Tet (X) in Acinetobacter species. Genome Med. 12, 1–13. doi: 10.1186/s13073-020-00807-5
Cornejo-Juárez, P., Cevallos, M. A., Castro-Jaimes, S., Castillo-Ramírez, S., Velázquez-Acosta, C., Martínez-Oliva, D., et al. (2020). High mortality in an outbreak of multidrug resistant Acinetobacter baumannii infection introduced to an oncological hospital by a patient transferred from a general hospital. PLoS One 15:e0234684. doi: 10.1371/journal.pone.0234684
Dahyot, S., Lebeurre, J., Argemi, X., François, P., Lemée, L., Prévost, G., et al. (2018). Multiple-locus variable number tandem repeat analysis (MLVA) and tandem repeat sequence typing (TRST), helpful tools for subtyping staphylococcus lugdunensis. Sci. Rep. 8, 1–11. doi: 10.1038/s41598-018-30144-y
Dortet, L., Potron, A., Bonnin, R. A., Plesiat, P., Naas, T., Filloux, A., et al. (2018). Rapid detection of colistin resistance in Acinetobacter baumannii using MALDI-TOF-based lipidomics on intact bacteria. Sci. Rep. 8, 1–5. doi: 10.1038/s41598-018-35041-y
El-Mahdy, T. S., Al-Agamy, M. H., Al-Qahtani, A. A., and Shibl, A. M. (2017). Detection of Bla OXA-23-like and Bla NDM-1 in Acinetobacter baumannii from the eastern region, Saudi Arabia. Microbial. Drug Res. 23, 115–121. doi: 10.1089/mdr.2015.0304
Farshadzadeh, Z., Hashemi, F. B., Rahimi, S., Pourakbari, B., Esmaeili, D., Haghighi, M. A., et al. (2015). Wide distribution of carbapenem resistant Acinetobacter baumannii in burns patients in Iran. Front. Microbiol. 6:1146. doi: 10.3389/fmicb.2015.01146
Gaiarsa, S., Batisti Biffignandi, G., Esposito, E. P., Castelli, M., Jolley, K. A., Brisse, S., et al. (2019). Comparative analysis of the two Acinetobacter baumannii multilocus sequence typing (MLST) schemes. Front. Microbiol. 10:930. doi: 10.3389/fmicb.2019.00930
Giannouli, M., Tomasone, F., Agodi, A., Vahaboglu, H., Daoud, Z., Triassi, M., et al. (2009). Molecular epidemiology of carbapenem-resistant Acinetobacter baumannii strains in intensive care units of multiple Mediterranean hospitals. J. Antimicrob. Chemother. 63, 828–830. doi: 10.1093/jac/dkp032
Gholami, M., Haghshenas, M., Moshiri, M., Razavi, S., Pournajaf, A., Irajian, G., et al. (2020). Frequency of 16S rRNA methylase and aminoglycoside-modifying enzyme genes among clinical isolates of Acinetobacter baumannii in Iran. Iranian J. Pathol. 12:329. doi: 10.30699/ijp.2017.27989
Gomaa, F. A. M., Helal, Z. H., and Khan, M. I. (2017). High prevalence of Bla NDM-1, Bla VIM, qacE, and qacEΔ1 genes and their association with decreased susceptibility to antibiotics and common hospital biocides in clinical isolates of Acinetobacter baumannii. Microorganisms 5:18. doi: 10.3390/microorganisms5020018
Grochowalska, A., Kozioł-Montewka, M., and Sobieszczańska, A. (2017). Analysis of Acinetobacter baumannii resistance patterns in patients with chronic obstructive pulmonary disease (COPD) in terms of choice of effective empiric antibiotic therapy. Ann. Agric. Environ. Med. 24, 307–311. doi: 10.26444/aaem/74710
Hauck, Y., Soler, C., Jault, P., Mérens, A., Gérome, P., Nab, C. M., et al. (2012). Diversity of Acinetobacter baumannii in four French military hospitals, as assessed by multiple locus variable number of tandem repeats analysis. PLoS One 7:e44597. doi: 10.1371/journal.pone.0044597
Heidary, M., Salimi Chirani, A., Khoshnood, S., Eslami, G., Atyabi, S. M., Nazem, H., et al. (2017). Molecular detection of aminoglycoside-modifying enzyme genes in Acinetobacter baumannii clinical isolates. Acta microbiologica et immunologica Hungarica 64, 143–50. doi: 10.1556/030.63.2016.022
Higgins, P., Wisplinghoff, H., Krut, O., and Seifert, H. (2007). A PCR-based method to differentiate between Acinetobacter baumannii and Acinetobacter genomic species 13TU. Clin. Microbiol. Infect. 13, 1199–1201. doi: 10.1111/j.1469-0691.2007.01819.x
Hojabri, Z., Pajand, O., Bonura, C., Aleo, A., Giammanco, A., and Mammina, C. (2014). Molecular epidemiology of Acinetobacter baumannii in Iran: endemic and epidemic spread of multiresistant isolates. J. Antimicrob. Chemother. 69, 2383–2387. doi: 10.1093/jac/dku045
Hua, X., He, J., Wang, J., Zhang, L., Zhang, L., Xu, Q., et al. (2021). Novel tigecycline resistance mechanisms in Acinetobacter baumannii mediated by mutations in adeS, rpoB and rrf. Emerg. Microbes Infect. 10, 1404–1417. doi: 10.1080/22221751.2021.1948804
Jenke, C., Lindstedt, B. A., Harmsen, D., Karch, H., Brandal, L. T., and Mellmann, A. (2011). Comparison of multilocus variable-number tandem-repeat analysis and multilocus sequence typing for differentiation of hemolytic-uremic syndrome-associated Escherichia coli (HUSEC) collection strains. J. Clin. Microbiol. 49, 3644–3646. doi: 10.1128/JCM.05035-11
Jiang, M., Chen, X., Liu, S., Zhang, Z., Li, N., Dong, C., et al. (2021). Epidemiological analysis of multidrug-resistant Acinetobacter baumannii isolates in a tertiary hospital over a 12-year period in China. Front. Public Health 9:707435. doi: 10.3389/fpubh.2021.707435
Jones, R. N., Ferraro, M. J., Reller, L. B., Schreckenberger, P. C., Swenson, J. M., and Sader, H. S. (2007). Multicenter studies of tigecycline disk diffusion susceptibility results for Acinetobacter spp. J. Clin. Microbiol. 45, 227–230. doi: 10.1128/JCM.01588-06
Karimi, S., Momtaz, H., and Fazel, N. (2021). Multiple locus variable number tandem repeat analysis of Acinetobacter baumannii strains isolated from human clinical and animal meat samples. Rev. Med. Microbiol. 32, 237–245. doi: 10.1097/MRM.0000000000000225
Karmostaji, A., Javadpour, S., Davoodian, P., and Moradi, N. (2014). In vitro activity of tigecycline and colistin against clinical isolates of Acinetobacter baumannii in hospitals in Tehran and Bandar-Abbas, Iran. Elect. Phy. 6:919. doi: 10.14661/2014.919-924
Khoshnood, S., Eslami, G., Hashemi, A., Bahramian, A., Heidary, M., Yousefi, N., et al. (2017). Distribution of aminoglycoside resistance genes among Acinetobacter baumannii strains isolated from burn patients in Tehran, Iran. Arch. Pediatr. Infect. Dis. 31:5. doi: 10.5812/pedinfect.57263
Khuntayaporn, P., Kanathum, P., Houngsaitong, J., Montakantikul, P., Thirapanmethee, K., and Chomnawang, M. T. (2021). Predominance of international clone 2 multidrug-resistant Acinetobacter baumannii clinical isolates in Thailand: a nationwide study. Ann. Clin. Microbiol. Antimicrob. 20, 1–11. doi: 10.1186/s12941-021-00424-z
Kirtikliene, T., Mierauskaitė, A., Razmienė, I., and Kuisiene, N. (2021). Multidrug-resistant Acinetobacter baumannii genetic characterization and spread in Lithuania in 2014, 2016, and 2018. Life 11:151. doi: 10.3390/life11020151
Lindstedt, B.-A., Torpdahl, M., Vergnaud, G., Le Hello, S., Weill, F., Tietze, E., et al. (2013). Use of multilocus variable-number tandem repeat analysis (MLVA) in eight European countries, 2012. Eur. Secur. 18:20385. doi: 10.2807/ese.18.04.20385-en
Liu, C.-C., Tang, C. Y., Chang, K.-C., Kuo, H.-Y., and Liou, M.-L. (2014). A comparative study of class 1 integrons in Acinetobacter baumannii. Gene 544, 75–82. doi: 10.1016/j.gene.2014.04.047
Magiorakos, A.-P., Srinivasan, A., Carey, R. B., Carmeli, Y., Falagas, M., Giske, C., et al. (2012). Multidrug-resistant, extensively drug-resistant and pandrug-resistant bacteria: an international expert proposal for interim standard definitions for acquired resistance. Clin. Microbiol. Infect. 18, 268–281. doi: 10.1111/j.1469-0691.2011.03570.x
Martins, N., Dalla-Costa, L., Uehara, A. A., Riley, L. W., and Moreira, B. M. (2013). Emergence of Acinetobacter baumannii international clone II in Brazil: reflection of a global expansion. Infect. Genet. Evol. 20, 378–380. doi: 10.1016/j.meegid.2013.09.028
Maspi, H., Hosseini, H. M., Amin, M., and Fooladi, A. A. I. (2016). High prevalence of extensively drug-resistant and metallo beta-lactamase-producing clinical Acinetobacter baumannii in Iran. Microb. Pathog. 98, 155–159. doi: 10.1016/j.micpath.2016.07.011
Matsui, M., Suzuki, M., Suzuki, M., Yatsuyanagi, J., Watahiki, M., Hiraki, Y., et al. (2018). Distribution and molecular characterization of Acinetobacter baumannii international clone II lineage in Japan. Antimicrob. Agents Chemother. 62, e02190–e02117. doi: 10.1128/AAC.02190-17
Mendes, R. E., Farrell, D. J., Sader, H. S., and Jones, R. N. (2010). Comprehensive assessment of tigecycline activity tested against a worldwide collection of Acinetobacter spp. (2005–2009). Diagn. Microbiol. Infect. Dis. 68, 307–311. doi: 10.1016/j.diagmicrobio.2010.07.003
Mirnejad, R., Heidary, M., Bahramian, A., Goudarzi, M., and Pournajaf, A. (2018). Evaluation of Polymyxin B susceptibility profile and detection of drug resistance genes among Acinetobacter baumannii Clinical Isolates in Tehran, Iran during 2015–2016. Mediterr. J. Hematol. Infect. Dis. 10. doi: 10.4084/mjhid.2018.044
Mohammadi Bardbari, A., Mohajeri, P., Arabestani, M. R., Karami, M., Keramat, F., Asadollahi, S., et al. (2020). Molecular typing of multi-drug resistant Acinetobacter baumannii isolates from clinical and environmental specimens in three Iranian hospitals by pulsed field gel electrophoresis. BMC Microbiol. 20, 1–7. doi: 10.1186/s12866-020-01792-w
Morfin-Otero, R., and Dowzicky, M. J. (2012). Changes in MIC within a global collection of Acinetobacter baumannii collected as part of the Tigecycline evaluation and surveillance trial, 2004 to 2009. Clin. Ther. 34, 101–112. doi: 10.1016/j.clinthera.2011.11.028
Najar Peerayeh, S., and Karmostaji, A. (2015). Molecular identification of resistance determinants, Integrons and genetic relatedness of extensively drug resistant Acinetobacter baumannii isolated from hospitals in Tehran, Iran. Jundishapur J. Microbiol. 8:e27021. doi: 10.5812/jjm.27021v2
Najar-Peerayeh, S, and Karmostaji, A. Evaluation of multilocus variable-number tandem-repeat (MLVA-8Orsay) for typing of Carbapenem-resistant Acinetobacter baumannii isolated from patients in Tehran, Iran Arch. Clin. Infect. Dis. 2019. (in press). doi: 10.5812/archcid.64402.
Nasiri, M. J., Zamani, S., Fardsanei, F., Arshadi, M., Bigverdi, R., Hajikhani, B., et al. (2020). Prevalence and mechanisms of carbapenem resistance in Acinetobacter baumannii: a comprehensive systematic review of cross-sectional studies from Iran. Microb. Drug Resist. 26, 270–283. doi: 10.1089/mdr.2018.0435
Nhu, N. T. K., Lan, N. P. H., Campbell, J. I., Parry, C. M., Thompson, C., Tuyen, H. T., et al. (2014). Emergence of carbapenem-resistant Acinetobacter baumannii as the major cause of ventilator-associated pneumonia in intensive care unit patients at an infectious disease hospital in southern Vietnam. J. Med. Microbiol. 63, 1386–1394. doi: 10.1099/jmm.0.076646-0
Nowak, J., Zander, E., Stefanik, D., Higgins, P. G., Roca, I., Vila, J., et al. (2017). High incidence of pandrug-resistant Acinetobacter baumannii isolates collected from patients with ventilator-associated pneumonia in Greece, Italy and Spain as part of the MagicBullet clinical trial. J. Antimicrob. Chemother. 72, 3277–3282. doi: 10.1093/jac/dkx322
Owrang, M., Karimi, A., Azimi, L., Motaghi Nezhad, R., and Fallah, F. (2018). Relative gene expression of RND-type efflux pumps in tigecycline resistant Acinetobacter baumannii isolated from training hospitals in Tehran, Iran. Int. J. Pediatr. 6, 8669–8674. doi: 10.22038/IJP.2018.33108.2922
Playford, E., Craig, J., and Iredell, J. (2007). Carbapenem-resistant Acinetobacter baumannii in intensive care unit patients: risk factors for acquisition, infection and their consequences. J. Hosp. Infect. 65, 204–211. doi: 10.1016/j.jhin.2006.11.010
Pourcel, C., Minandri, F., Hauck, Y., d'Arezzo, S., Imperi, F., Vergnaud, G., et al. (2011). Identification of variable-number tandem-repeat (VNTR) sequences in Acinetobacter baumannii and interlaboratory validation of an optimized multiple-locus VNTR analysis typing scheme. J. Clin. Microbiol. 49, 539–548. doi: 10.1128/JCM.02003-10
Rafei, R., Dabboussi, F., Hamze, M., Eveillard, M., Lemarié, C., Gaultier, M.-P., et al. (2014). Molecular analysis of Acinetobacter baumannii strains isolated in Lebanon using four different typing methods. PLoS One 9:e115969. doi: 10.1371/journal.pone.0115969
Rahimi, S., Farshadzadeh, Z., Taheri, B., Mohammadi, M., Haghighi, M.-A., and Bahador, A. (2018). The relationship between antibiotic resistance phenotypes and biofilm formation capacity in clinical isolates of Acinetobacter baumannii. Jundishapur. J. Microbiol. 11:e74315. doi: 10.5812/jjm.74315
Razavi Nikoo, H., Ardebili, A., and Mardaneh, J. (2017). Systematic review of antimicrobial resistance of clinical Acinetobacter baumannii isolates in Iran: an update. Microb. Drug Resist. 23, 744–756. doi: 10.1089/mdr.2016.0118
Robatjazi, S., Nikkhahi, F., Niazadeh, M., Amin Marashi, S. M., Peymani, A., Javadi, A., et al. (2021). Phenotypic identification and genotypic characterization of plasmid-mediated AmpC β-lactamase-producing Escherichia coli and Klebsiella pneumoniae isolates in Iran. Curr. Microbiol. 78, 2317–2323. doi: 10.1007/s00284-021-02479-9
Rynga, D., Shariff, M., and Deb, M. (2015). Phenotypic and molecular characterization of clinical isolates of Acinetobacter baumannii isolated from Delhi, India. Ann. Clin. Microbiol. Antimicrob. 14, 1–8. doi: 10.1186/s12941-015-0101-5
Safari, M., Nejad, A. S. M., Bahador, A., Jafari, R., and Alikhani, M. Y. (2015). Prevalence of ESBL and MBL encoding genes in Acinetobacter baumannii strains isolated from patients of intensive care units (ICU). Saudi J. Biol. Sci. 22, 424–429. doi: 10.1016/j.sjbs.2015.01.004
Saffari, F., Monsen, T., Karmostaji, A., Azimabad, F. B., and Widerström, M. (2017). Significant spread of extensively drug-resistant Acinetobacter baumannii genotypes of clonal complex 92 among intensive care unit patients in a university hospital in southern Iran. J. Med. Microbiol. 66, 1656–1662. doi: 10.1099/jmm.0.000619
Salehi, B., Goudarzi, H., Nikmanesh, B., Houri, H., Alavi-Moghaddam, M., and Ghalavand, Z. (2018). Emergence and characterization of nosocomial multidrug-resistant and extensively drug-resistant Acinetobacter baumannii isolates in Tehran, Iran. J. Infect. Chemother. 24, 515–523. doi: 10.1016/j.jiac.2018.02.009
Sarada, V., Rao, R., Mani, R., and Ramana, K. (2014). Colistin, polymyxin B and tigecycline suspectibility to metallo betalactamase producing Acinetobacter baumannii isolated from tertiary health care hospital. Am. J. Microbiol. Res. 2, 60–62. doi: 10.12691/ajmr-2-2-3
Sato, Y., Ubagai, T., Tansho-Nagakawa, S., Yoshino, Y., and Ono, Y. (2021). Effects of colistin and tigecycline on multidrug-resistant Acinetobacter baumannii biofilms: advantages and disadvantages of their combination. Sci. Rep. 11, 1–11. doi: 10.1038/s41598-021-90732-3
Shi, Y., Hua, X., Xu, Q., Yang, Y., Zhang, L., He, J., et al. (2020). Mechanism of eravacycline resistance in Acinetobacter baumannii mediated by a deletion mutation in the sensor kinase adeS, leading to elevated expression of the efflux pump AdeABC. Infect. Genet. Evol. 80:104185. doi: 10.1016/j.meegid.2020.104185
Skerniškytė, J., Krasauskas, R., Péchoux, C., Kulakauskas, S., Armalytė, J., and Sužiedėlienė, E. (2019). Surface-related features and virulence among Acinetobacter baumannii clinical isolates belonging to international clones I and II. Front. Microbiol. 9:3116. doi: 10.3389/fmicb.2018.03116
Tafreshi, N., Babaeekhou, L., and Ghane, M. (2019). Antibiotic resistance pattern of Acinetobacter baumannii from burns patients: increase in prevalence of bla (OXA-24-like) and bla (OXA-58-like) genes. Iran J. Microbiol. 11, 502–509. doi: 10.18502/ijm.v11i6.2222
Taheri, B, Bahador, A, and Assarehzadegan-dezfuli, A. The role of AdeABC, AdeFGH and AdeIJK efflux pumps in reduced susceptibility to Tigecycline: Emergence of an outbreak associated with an international clone variant of Acinetobacter baumannii isolated from burn patients in Iran. 2022.
Teerawattanapong, N., Panich, P., Kulpokin, D., Ranong, S. N., Kongpakwattana, K., Saksinanon, A., et al. (2018). A systematic review of the burden of multidrug-resistant healthcare-associated infections among intensive care unit patients in Southeast Asia: the rise of multidrug-resistant Acinetobacter baumannii. Infect. Control Hosp. Epidemiol. 39, 525–533. doi: 10.1017/ice.2018.58
Tewari, R., Chopra, D., Wazahat, R., Dhingra, S., and Dudeja, M. (2018). Antimicrobial susceptibility patterns of an emerging multidrug resistant nosocomial pathogen: Acinetobacter baumannii. Malays. J. Med. Sci. 25, 129–134. doi: 10.21315/mjms2018.25.3.13
Turton, J., Gabriel, S., Valderrey, C., Kaufrnann, M., and Pitt, T. (2007). Use of sequence-based typing and multiplex PCR to identify clonal lineages of outbreak strains of Acinetobacter baumannii. Clin. Microbiol. Infect. 13, 807–815. doi: 10.1111/j.1469-0691.2007.01759.x
Turton, J. F., Woodford, N., Glover, J., Yarde, S., Kaufmann, M. E., and Pitt, T. L. (2006). Identification of Acinetobacter baumannii by detection of the bla OXA-51-like carbapenemase gene intrinsic to this species. J. Clin. Microbiol. 44, 2974–2976. doi: 10.1128/JCM.01021-06
Villalón, P., Valdezate, S., Cabezas, T., Ortega, M., Garrido, N., Vindel, A., et al. (2015). Endemic and epidemic Acinetobacter baumannii clones: a twelve-year study in a tertiary care hospital. BMC Microbiol. 15, 1–9. doi: 10.1186/s12866-015-0383-y
Wareth, G., Linde, J., Hammer, P., Splettstoesser, W. D., Pletz, M. W., Neubauer, H., et al. (2021). Molecular characterization of German Acinetobacter baumannii isolates and multilocus sequence typing (MLST) analysis based on WGS reveals novel STs. Pathogens 10:690. doi: 10.3390/pathogens10060690
Wayne, P. (2020). “The Clinical & Laboratory Standards Institute (CLSI),” in Performance standards for antimicrobial susceptibility testing. 30th Edn. CLSI supplement EP06. Wayne, PA: CLSI.
Whiteway, C., Breine, A., Philippe, C., and Van der Henst, C. (2022). Acinetobacter baumannii. Trends Microbiol. 30, 199–200. doi: 10.1016/j.tim.2021.11.008
Xie, R., Zhang, X. D., Zhao, Q., Peng, B., and Zheng, J. (2018). Analysis of global prevalence of antibiotic resistance in Acinetobacter baumannii infections disclosed a faster increase in OECD countries. Emerg. Microbes Infect. 7, 1–10. doi: 10.1038/s41426-018-0038-9
Xu, Q., Hua, X., He, J., Zhang, D., Chen, Q., Zhang, L., et al. (2020). The distribution of mutations and hotspots in transcription regulators of resistance-nodulation-cell division efflux pumps in tigecycline non-susceptible Acinetobacter baumannii in China. Int. J. Med. Microbiol. 310:151464. doi: 10.1016/j.ijmm.2020.151464
Ying, C., Li, Y., Wang, Y., Zheng, B., and Yang, C. (2015). Investigation of the molecular epidemiology of Acinetobacter baumannii isolated from patients and environmental contamination. J. Antibiot. 68, 562–567. doi: 10.1038/ja.2015.30
Keywords: Acinetobacter baumannii, extensively drug-resistant, multidrug-resistant, multilocus sequence typing, tigecycline
Citation: Maleki A, Kaviar VH, Koupaei M, Haddadi MH, Kalani BS, Valadbeigi H, Karamolahi S, Omidi N, Hashemian M, Sadeghifard N, Mohamadi J, Heidary M and Khoshnood S (2022) Molecular typing and antibiotic resistance patterns among clinical isolates of Acinetobacter baumannii recovered from burn patients in Tehran, Iran. Front. Microbiol. 13:994303. doi: 10.3389/fmicb.2022.994303
Edited by:
Benjamin Andrew Evans, University of East Anglia, United KingdomReviewed by:
Amir Sasan Mozaffari Nejad, Jiroft University of Medical Sciences, IranLina Maarouf, Alexandria University, Egypt
Copyright © 2022 Maleki, Kaviar, Koupaei, Haddadi, Kalani, Valadbeige, Karamolahi, Omidi, Hashemian, Sadeghifard, Mohamadi, Heidary and Khoshnood. This is an open-access article distributed under the terms of the Creative Commons Attribution License (CC BY). The use, distribution or reproduction in other forums is permitted, provided the original author(s) and the copyright owner(s) are credited and that the original publication in this journal is cited, in accordance with accepted academic practice. No use, distribution or reproduction is permitted which does not comply with these terms.
*Correspondence: Saeed Khoshnood, Saeed.khoshnood22@gmail.com; Mohsen Heidary, mohsenheidary40@gmail.com