- 1Department of Biology, University of Nairobi, Nairobi, Kenya
- 2Elakistos Biosciences, Nairobi, Kenya
The importance of Xenorhabdus and Photorhabdus symbionts to their respective Steinernema and Heterorhabditis nematode hosts is that they not only contribute to their entomopathogenicity but also to their fecundity through the production of small molecules. Thus, this mini-review gives a brief introductory overview of these nematophilic bacteria. Specifically, their type species, nematode hosts, and geographic region of isolations are tabulated. The use of nucleotide sequence-based techniques for their species delineation and how pangenomes can improve this are highlighted. Using the Steinernema–Xenorhabdus association as an example, the bacterium-nematode lifecycle is visualized with an emphasis on the role of bacterial biomolecules. Those currently in drug development are discussed, and two potential antimalarial lead compounds are highlighted. Thus, this mini-review tabulates forty-eight significant nematophilic bacteria and visualizes the ecological importance of their biomolecules. It further discusses three of these biomolecules that are currently in drug development. Through it, one is introduced to Xenorhabdus and Photorhabdus bacteria, their natural production of biomolecules in the nematode-bacterium lifecycle, and how these molecules are useful in developing novel therapies.
Introduction
Nematophilic “nematode loving” bacteria are prokaryotes that are symbiotically associated with members of phylum Nematoda. Three such genera are Xenorhabdus, Photorhabdus, and Serratia, which are symbionts of Steinernematidae, Heterorhabditidae, and Rhabditidae members, respectively. Each of these three families of the order Rhabditida contain entomopathogenic species—all members of Steinernema and Heterorhabditis, all members of the Insectivora—group of Oscheius, and Caenorhabditis briggsae. However, it is their Xenorhabdus, Photorhabdus, or Serratia symbionts that contribute in large part to this trait through both septicemia and toxemia (Bowen et al., 1998; Ffrench-Constant and Waterfield, 2005; Abebe et al., 2011; Clarke, 2020). Hence, Xenorhabdus, Photorhabdus, and a few Serratia strains are also termed entomopathogenic bacteria. Whereas Serratia symbionts form associations with Oscheius and Caenorhabditis hosts, Xenorhabdus and Photorhabdus are more genus-specific associating only with Steinernema and Heterorhabditis hosts, respectively (Table 1).
Apart from Xenorhabdus and Serratia, other entomopathogenic bacterial symbionts such as Pseudomonas sp. (Ogier et al., 2020) and Alcaligenes sp. (Shan et al., 2019) associate with Steinernema and Oscheius nematodes, respectively.
This classification of Serratia as nematophilic bacteria has caveats. Not all species in the Serratia genus are nematode symbionts (Grimont and Grimont, 2006), and only a few Oscheius-Serratia/Caenorhabditis-Serratia associations are known (Table 1). Moreover, for some of these associations, Serratia were only facultative symbionts—Serratia sp. strain SCBI and Serratia marcescens from C. briggsae and Oscheius carolinensis, respectively (Abebe et al., 2011; Torres-Barragan et al., 2011). Conversely, except one, all characterized Xenorhabdus and Photorhabdus species are natural nematode intestinal symbionts (Table 1). Thus, this mini-review focused on Xenorhabdus and Photorhabdus as nematophilic bacteria.
Xenorhabdus and Photorhabdus bacteria
Xenorhabdus and Photorhabdus are both gram-negative, rod-shaped, peritrichously flagellated, facultative anaerobes of the family Morganellaceae, order Enterobacterales, and class Gammaproteobacteria (Adeolu et al., 2016). They are uniquely characterized by not only having primary and secondary variants but also an endosymbiosis with entomopathogenic nematodes (EPNs). Other distinguishing traits include Photorhabdus as the only terrestrial bioluminescent bacterium genus and Xenorhabdus as the only member of Enterobacterales that does not produce catalase (Boemare and Akhurst, 2006). Despite this taxonomic relatedness, the similar ecological niche of the two is more due to convergent evolution (Chaston et al., 2011). Twenty-seven Xenorhabdus species that were isolated from twenty-seven steinernematids have been described to date (Table 1). However, 100 Steinernema species have been characterized (Bhat et al., 2020) highlighting that at most—because of species with more than one nematode host (Table 1)—63 novel Xenorhabdus species could be added to the genus from these respective under-investigated yet described steinernematids. This prediction can be mathematically supported by determining whether the Xenorhabdus pangenome is open (Medini et al., 2020).
Nucleotide sequence-based techniques are not only the gold standard for prokaryotic species delineation (Chun et al., 2018) but are also useful for either identification of new isolates or emendation of already described taxon. For example, Xenorhabdus sp. strain BMMCB was described as an Xenorhabdus. griffiniae species (Mothupi et al., 2015), but we (Awori et al., 2017) demonstrated that its nucleotide identities values for the recombinase A (recA), phosphoserine transferase (serC), and small subunit ribosomal ribonucleic acid (rRNA) (SSU) genes, with those of the type species, were below the accepted threshold for conspecific strains—97% for protein-coding genes (Tailliez et al., 2010) and 98.7% for SSU gene (Kim et al., 2014).
Two powerful nucleotide sequence-based techniques are average nucleotide identities (ANI) and digital DNA–DNA hybridization (dDDH), which both delineate species by calculating how related two genomes are. The thresholds for conspecific strains are >95% (Richter and Rosselló-Móra, 2009) and >70% (Auch et al., 2010) for ANI and dDDH, respectively. Both were used to reclassify Photorhabdus species (Machado et al., 2018). However, strains S8-52, S9-53, and S10-54 identified as Photorhabdus kleinii had ANI values of 96.7% with the Photorhabdus bodei type strain and Photorhabdus temperata Meg1 had ANI values of 96.3% with the Photorhabdus thracensis type strain demonstrating the difficulty in delineating species of Photorhabdus (Fischer-Le Saux et al., 1998; Tailliez et al., 2010; Machado et al., 2021b) even with these nucleotide-based thresholds (Bobay, 2020). Thus, the use of pangenome analysis for species delineations—as was done in the Prochlorococcus genus (Moldovan and Gelfand, 2018)—is recommended for Photorhabdus systematics when sufficient genome sequences—at least five per species (Medini et al., 2020)—are available.
The nematode-bacterium lifecycle and bacterial biomolecules
The nematode-bacterium lifecycle begins with soil-dwelling infective third larval stage juvenile nematode (IJ3) preying on an insect (Figure 1). Anatomically, IJ3 are third larval stage juvenile nematodes (J3) with a retained second larval stage cuticle that seals both mouth and anus rendering the nematodes into a non-feeding, developmentally arrested, and perennation-like stage (Poinar and Leutenegger, 1968). Steinernematids IJ3 infect an insect only through natural openings, whereas heterorhabditis can additionally gain entry by piercing into the hemocoel using a bursa (Bedding and Molyneux, 1982). Once within, the IJ3s undergo “recovery” (Clarke, 2020) whereby they shed their second larval stage cuticle and release into the hemocoel, their gut bacterial symbionts. For Steinernema, Xenorhabdus would have been previously localized in a receptacle (Stilwell et al., 2018) at the anterior gut whereas, in Heterorhabditis, Photorhabdus would have previously lined the entire gut (Waterfield et al., 2009). Detection of L-proline concentrations >4.8 mM in insect hemolymph triggers an upregulated bacterial secretion of specialized metabolites of various ecological functions (Crawford et al., 2010).
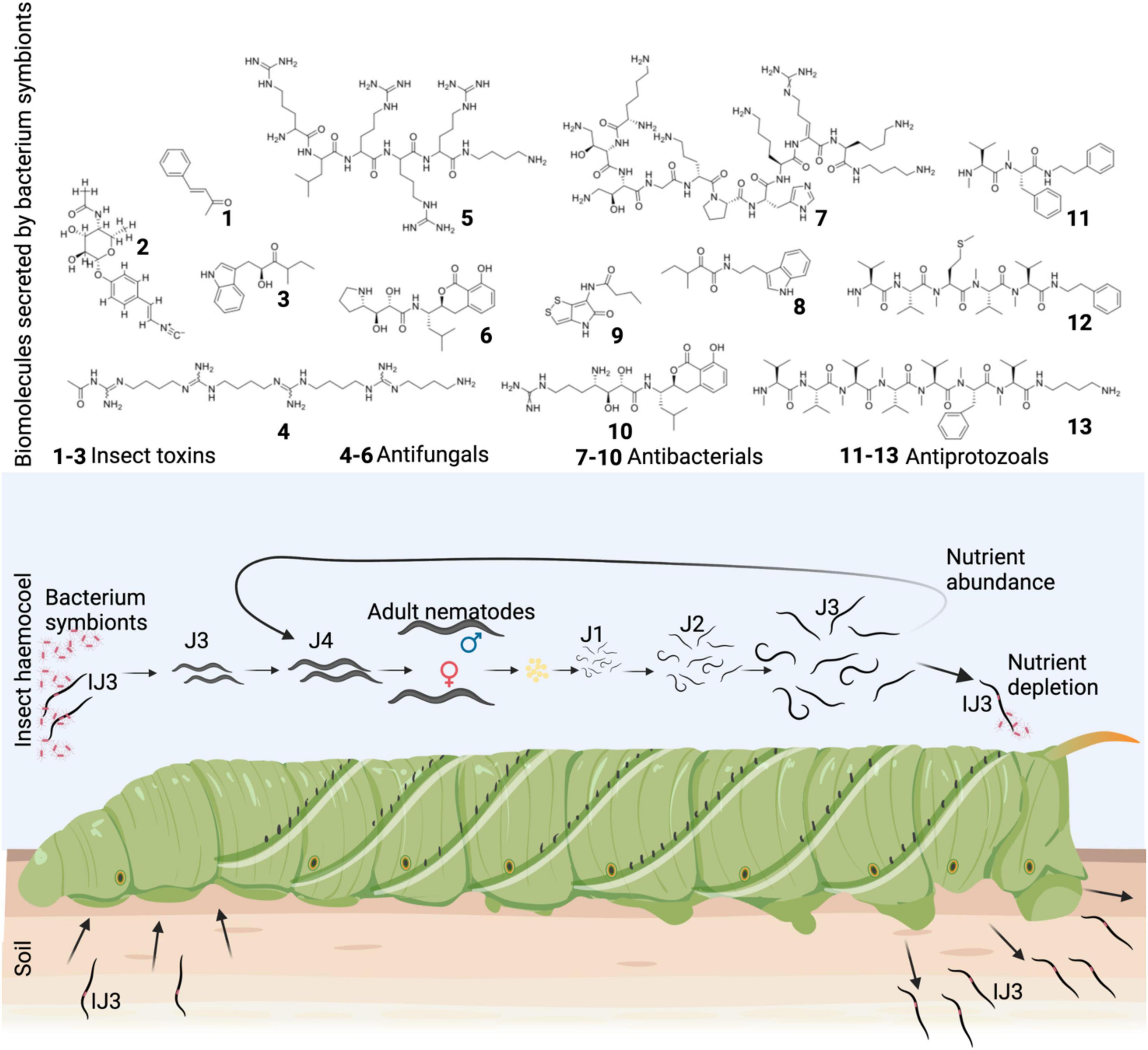
Figure 1. Xenorhabdus-Steinernema lifecycle and selected biomolecules that contribute toward nematode fecundity. Free-living infective third-stage juvenile (IJ3) nematodes seek out insects and gain entry through natural openings such as spiracles, and once within the hemocoel, nematodes exit their non-feeding state and release Xenorhabdus gut symbionts. The bacteria secrete a range of biomolecules (1–13) that increase the fecundity of the nematodes. Nematodes go through complete lifecycles thus increasing their numbers and upon depletion of nutrients each J3 re-associates with a few Xenorhabdus bacteria and exit the insect cadaver as an IJ3. J1, J2, J3, and J4 = first, second, third, and fourth larval stage juvenile nematodes, respectively. Benzylacetone (1), rhabduscin (2), xenocyloin (3), cabanillasin (4), biocornutin (5), xenocoumacin 2 (6), odilorhabdin (7), nematophin (8), xenorhabdin (9), xenocoumacin 1 (10), xenortide (11), rhabdopeptide (12), and rhabdopeptide/xenortide-like peptides (13) were created in biorender.com.
Despite the following grouping of biomolecules from both Xenorhabdus and Photorhabdus according to the similarity of ecological function, their biosynthesis is species-specific. The first grouping is insecticidal toxins, and these can be divided into insect immune suppressors via inhibition of phenoloxidase pathway:-1,2-benzene dicarboxylic acid (PA) (Ullah et al., 2014), benzylideneacetone (BZA) (Song et al., 2011), rhabduscin (Crawford et al., 2012; Eugenia Nuñez-Valdez et al., 2019), and 1,3-dihydroxy-2-(isopropyl)-5-(2-phenylethenyl)benzene (Eleftherianos et al., 2007); hemocyte pore-forming complexes: Xenorhabdus particulate toxins (Xpt) (Sheets et al., 2011), toxin complex toxins (Tc) (Blackburn et al., 1998), and Xenorhabdus α-xenorhabdolysin toxins (Xax) (Vigneux et al., 2007); apoptosis inducers: make caterpillar floppy toxins (Mcf) (Daborn et al., 2002; Dowling et al., 2004) and PaTox toxins (Jank et al., 2016); and those with yet unknown modes of action: PirAB (Yang et al., 2017) and xenocyloin (Proschak et al., 2014). Another ecological function of secreted metabolites is bioconversion by enzymes such as lipases, proteases, amylases, and proteases—their respective genes are enriched in Xenorhabdus and Photorhabdus genomes (Chaston et al., 2011)—creating a rich nutrient pool. To defend this from colonization by microbial competitors, a broad spectrum of antimicrobials is produced. These include antifungals: biocornutin (Böszörményi et al., 2009), cabanillasin (Houard et al., 2013), EP-19, GP-20 (Xiao et al., 2012), and xenocoumacin (Yang et al., 2011); antibacterials: darobactin (Imai et al., 2019), xenematide (Lang et al., 2008), photoditritide (Zhao et al., 2019), xenobactin (Grundmann et al., 2013), odilorhabdins (Pantel et al., 2018), xenorhabdin (McInerney et al., 1991), and PAX peptides (Gualtieri et al., 2009); antiprotozoals: phototemtide (Zhao et al., 2020), szentiamide (Ohlendorf et al., 2011), chaiyaphumins (Grundmann et al., 2014), rhabdopeptide/xenortide-like peptides (RXP) (Zhao L. et al., 2018), xenortide (Reimer et al., 2014), xenoamicin (Zhou et al., 2013), and ambactin (Schimming et al., 2014); and cytotoxic agents: fabclavines (Wenski et al., 2020) and phenylethylamine (PEA) derivatives (Proschak et al., 2011).
Recovered IJ3s leverage this nutrient-filled, enclosed environment to molt to fourth larval stage juvenile nematodes (J4) and then adults (Figure 1) that lay eggs after mating in the case of all steinernematids except Steinernema hermaphroditum—these species lay eggs without mating due to their hermaphroditic nature. This is like the androdioecious heterorhabditis whose adult females are also self-fertilized. Uniquely, Heterorhabditis adult females lay eggs into their uterus which hatch and develop into first larval stage juvenile nematodes (J1) through endotokia matricida (Clarke, 2020). Newly hatched EPNs molt from J1 through to J4 and then adults, which mate and lay eggs thus beginning another lifecycle. This continues until nutrients are depleted (Figure 1). Notably, infected cadavers are themselves protected from consumption by non-microbial competitors such as ants by the bacterial production of scavenger deterring factors (Zhou et al., 2002; Gulcu et al., 2012).
Upon nutrient depletion, J3 nematodes commence transformation to IJ3s by reassociating with bacterial symbionts (Figure 1)—this can be as few as one per nematode in the case of Xenorhabdus reassociations (Stilwell et al., 2018). Moreover, a highly species-specific reassociation occurs in Xenorhabdus-Steinernema complexes, and in Xenorhabdus nematophila, this is attributed to the NilC protein (Cowles and Goodrich-Blair, 2004). By retaining the second larval stage cuticle, J3s complete their transformation to IJ3s that then emigrate the cadaver in search of new insect prey. Notably, all seven macrocyclic antimicrobial non-ribosomal peptides (NRPs) with known toxicities—chaiyaphumins, photoditritide, szentiamide, xenobactin, phototemtide, xenoamicin, and PAX lipopeptides—were lowly toxic to mammalian cells—the lowest half-maximal inhibitory concentration (IC50) was 52 μM (Gualtieri et al., 2009; Ohlendorf et al., 2011; Grundmann et al., 2013, 2014; Zhou et al., 2013; Zhao et al., 2019, 2020). The bacteria possibly evolved to synthesize these compounds to inhibit diverse soil microorganisms while remaining lowly toxic to animal nematode hosts (Racine and Gualtieri, 2019). Biotechnologically, their low toxicity, natural derivatization, and macrocyclic structure (Dathe et al., 2004; Rodríguez et al., 2021) make them suitable for antibiotic drug development.
Xenorhabdus/Photorhabdus molecules in drug development
Many Xenorhabdus/Photorhabdus molecules have the potential to be developed into approved drugs (Challinor and Bode, 2015; Dreyer et al., 2018; Racine and Gualtieri, 2019; Booysen and Dicks, 2020). For example, Photorhabdus luminescens biosynthesized 3,5-dihydroxy-4-isopropylstilbene (Hu et al., 1997)—this is the active pharmaceutical ingredient in the drugs benvitimod and tapinarof (Zhang et al., 2022), which are approved for market in China and the USA, respectively, for the treatment of psoriasis and topical dermatitis (Lebwohl et al., 2021). NOS-502, an antibiotic lead compound currently in pre-clinical development, is a synthetic derivative of the odilorhabdins (Figure 1). These are cationic antimicrobial NRPs biosynthesized by X. nematophila that inhibit protein synthesis via unique sites on the 30S ribosome (Pantel et al., 2018). NOS-502 not only had a good in vivo safety profile but also inhibited beta-lactam resistant strains of both Escherichia coli and Klebsiella pneumoniae at minimum inhibitory concentrations (MICs) of 1.85 and 0.93 μM, respectively (Zhao M. et al., 2018). Another lead compound in pre-clinical development is darobactin A which is produced by Photorhabdus khanii (Lewis, 2020). It too was lowly toxic in murine models and inhibited beta-lactam resistant strains of both E. coli and K. pneumoniae at an MIC of 2.1 μM (Imai et al., 2019).
The development of novel antimalarial drugs is of current global health importance due to increasing resistance to artemisinin-based therapies in malaria-endemic regions such as East Africa (Asua et al., 2021), because of mutations in the Plasmodium falciparum K13 gene (Amaratunga et al., 2019). Two potential antimalarial lead compounds for pre-clinical development are the NRPs chaiyaphumin A from Xenorhabdus sp. PB61.4 (Grundmann et al., 2014) and rhabdopeptide/xenortide-like peptide (RXP) 6 from Xenorhabdus innexi (Zhao L. et al., 2018). This is because RXP 6 and chaiyaphumin A were inhibitory to P. falciparum at IC50 of 0.091 and 0.61 μM, respectively. Moreover, they had respective selectivity indexes of 63 and 151. Biochemically, the bioactivity of chaiyaphumin A was affected by the fatty acid acylated to its N terminal as the natural swapping of phenylacetic acid for n-butyrate created a derivative with an IC50 of 15.4 μM and selectivity index of 10. Thus, a probable route for creating chaiyaphumin derivatives with enhanced pharmacological properties is by swapping the Cstarter domain of its non-ribosomal peptide synthetase (NRPS) via NRPS re-engineering (Beck et al., 2020).
Although antibody–drug conjugates are promising anticancer therapies, their intrinsic high cost of development makes the price of approved drugs—such as enfortumab vedotin for the treatment of urothelial carcinoma—currently cost-ineffective (Wu et al., 2022). A possible solution is replacing the antibody component with a modified Photorhabdus Tc toxin, to translocate—within a cocoon-like structure (Roderer et al., 2019)—and deliver cytotoxic compounds into targeted cancer cells (Nǵanǵa et al., 2019). However, the concept that the Photorhabdus TcA subunit can selectively bind to a cancer cell needs to be first proven.
Conclusion
Twenty-seven Xenorhabdus, twenty-one Photorhabdus species, and four Serratia strains were identified as isolated from EPNs. Sixty-three novel species of Xenorhabdus could be discovered from corresponding characterized but under-investigated steinernematids. Due to the low phylogenetic diversity in the genus, the use of pangenome analyses for species delineation in Photorhabdus is recommended when enough genomes per species are available. The lifecycle of the nematode-bacterium complex is marked by the secretion of diverse bioactive bacterial molecules in the presence of juvenile nematodes, necessitating high selectivity. Thus, many of these molecules are applicable in biotechnology and a few are currently in drug development pipelines. This highlights the practical importance of discovering more nematophilic bacteria: They are a source of novel therapeutics.
Author contributions
RA did the research, wrote the manuscript, and approved the submitted version.
Funding
This research was funded by the Kenya National Research Fund: NRF 1st CALL/MULTIDISCIPLINARY RESEARCH/127, “Drug Development of Antibiotics: Xenorhabdus bacteria from Kenya”.
Conflict of interest
RA is the proprietor of Elakistos Biosciences. This did not influence this research.
Publisher’s note
All claims expressed in this article are solely those of the authors and do not necessarily represent those of their affiliated organizations, or those of the publisher, the editors and the reviewers. Any product that may be evaluated in this article, or claim that may be made by its manufacturer, is not guaranteed or endorsed by the publisher.
References
Abebe, E., Abebe-Akele, F., Morrison, J., Cooper, V., and Thomas, W. K. (2011). An insect pathogenic symbiosis between a Caenorhabditis and Serratia. Virulence 2, 158–161. doi: 10.4161/viru.2.2.15337
Abebe, E., Jumba, M., Bonner, K., Gray, V., Morris, K., and Thomas, W. K. (2010). An entomopathogenic Caenorhabditis briggsae. J. Exp. Biol. 213, 3223–3229. doi: 10.1242/jeb.043109
Adeolu, M., Alnajar, S., Naushad, S., and Gupta, R. S. (2016). Genome-based phylogeny and taxonomy of the ‘Enterobacteriales’: Proposal for Enterobacterales ord. nov. divided into the families Enterobacteriaceae, Erwiniaceae fam. nov., Pectobacteriaceae fam. nov., Yersiniaceae fam. nov., Hafniaceae fam. nov., Morganellaceae fam. nov., and Budviciaceae fam. nov. Int. J. Syst. Evol. Microbiol. 66, 5575–5599. doi: 10.1099/ijsem.0.001485
Aguera de Doucet, M. M. (1986). A new species of Neoaplectana Steiner, 1929 (Nematoda: Steinernematidae) from Cordoba, Argentina. Rev. Nématol. 9, 317–323.
Akhurst, R. J. (1983). Taxonomic Study of Xenorhabdus, a genus of bacteria symbiotically associated with insect pathogenic nematodes. Int. J. Syst. Evol. Microbiol. 33, 38–45.
Akhurst, R. J. (1987). Use of starch gel electrophoresis in the taxonomy of the genus Heterorhabditis (Nematoda: Heterorhabditidae). Nematologica 33, 1–9.
Akhurst, R. J., and Boemare, N. E. (1988). A numerical taxonomic study of the genus Xenorhabdus (Enterobacteriaceae) and proposed elevation of the subspecies of X. nematophilus to species. Microbiology 134, 1835–1845. doi: 10.1099/00221287-134-7-1835
Akhurst, R. J., Boemare, N. E., Janssen, P. H., Peel, M. M., Alfredson, D. A., and Beard, C. E. (2004). Taxonomy of Australian clinical isolates of the genus Photorhabdus and proposal of Photorhabdus asymbiotica subsp. asymbiotica subsp. nov. and P. asymbiotica subsp. australis subsp. nov. Int. J. Syst. Evol. Microbiol. 54, 1301–1310. doi: 10.1099/ijs.0.03005-0
Amaratunga, C., Andrianaranjaka, V. H., Ashley, E., Bethell, D., Björkman, A., Bonnington, C. A., et al. (2019). Association of mutations in the Plasmodium falciparum Kelch13 gene (Pf3D7_1343700) with parasite clearance rates after artemisinin-based treatments—a WWARN individual patient data meta-analysis. BMC Med. 17:1. doi: 10.1186/s12916-018-1207-3
An, R., and Grewal, P. S. (2011). Photorhabdus luminescens subsp. kleinii subsp. nov.(Enterobacteriales: Enterobacteriaceae). Curr. Microbiol. 62, 539–543.
Artyukhovsky, A. K. (1997). Redescription of Steinernema arenarium (Artyukhovsky, 1967) topotypes from Central Russia and a proposal for S. anomalae (Kozodoi, 1984) as a junior synonym. Russ. J. Nematol. 5, 31–38.
Asua, V., Conrad, M. D., Aydemir, O., Duvalsaint, M., Legac, J., Duarte, E., et al. (2021). Changing prevalence of potential mediators of aminoquinoline, antifolate, and artemisinin resistance across uganda. J. Infect. Dis. 223, 985–994. doi: 10.1093/infdis/jiaa687
Auch, A. F., von Jan, M., Klenk, H.-P., and Göker, M. (2010). Digital DNA-DNA hybridization for microbial species delineation by means of genome-to-genome sequence comparison. Stand. Genomic Sci. 2, 117–134. doi: 10.4056/sigs.531120
Awori, R. M., Njenga Ng’ang’a, P., Nyongesa, L. N., Amugune, N. O., and Masiga, D. (2017). Mursamacin: A novel class of antibiotics from soil-dwelling roundworms of Central Kenya that inhibits methicillin-resistant Staphylococcus aureus [version 2; peer review: 2 approved, 1 approved with reservations]. F1000Research 5:2431. doi: 10.12688/f1000research.9652.2
Beck, C., Garzón, J. F. G., and Weber, T. (2020). Recent advances in re-engineering modular PKS and NRPS assembly lines. Biotechnol. Bioprocess Eng. 25, 886–894. doi: 10.1007/s12257-020-0265-5
Bedding, R. A., and Molyneux, A. S. (1982). Penetration of insect cuticle by infective juveniles of Heterorhabditis Spp. (Heterorhabditidae: Nematoda). Nematologica 28, 354–359. doi: 10.1163/187529282X00402
Bhat, A. H., Chaubey, A. K., and Askary, T. H. (2020). Global distribution of entomopathogenic nematodes, Steinernema and Heterorhabditis. Egypt. J. Biol. Pest Control 30:31. doi: 10.1186/s41938-020-0212-y
Blackburn, M., Golubeva, E., Bowen, D., and Ffrench-Constant, R. H. (1998). A novel insecticidal toxin from Photorhabdus luminescens, toxin complex a (Tca), and its histopathological effects on the midgut of Manduca sexta. Appl. Environ. Microbiol. 64, 3036–3041. doi: 10.1128/AEM.64.8.3036-3041.1998
Bobay, L.-M. (2020). “The prokaryotic species concept and challenges,” in The pangenome: Diversity, dynamics and evolution of genomes, eds H. Tettelin and D. Medini (Cham: Springer), 21–49. doi: 10.1007/978-3-030-38281-0_2
Bode, E., Brachmann, A. O., Kegler, C., Simsek, R., Dauth, C., Zhou, Q., et al. (2015). Simple “on-demand” production of bioactive natural products. Chembiochem 16, 1115–1119. doi: 10.1002/cbic.201500094
Boemare, N. E., Akhurst, R. J., and Mourant, R. G. (1993). DNA relatedness between Xenorhabdus spp. (Enterobacteriaceae), symbiotic bacteria of entomopathogenic nematodes, and a proposal to transfer Xenorhabdus luminescens to a new genus, Photorhabdus gen. nov. Int. J. Syst. Bacteriol. 43, 249–255.
Boemare, N., and Akhurst, R. (2006). “The genera Photorhabdus and Xenorhabdus,” in The prokaryotes: A handbook on the biology of bacteria: Proteobacteria: Gamma subclass, Vol. 6, eds M. Dworkin, S. Falkow, E. Rosenberg, K.-H. Schleifer, and E. Stackebrandt (New York, NY: Springer New York), 451–494. doi: 10.1007/0-387-30746-X_16
Booysen, E., and Dicks, L. M. T. (2020). Does the future of antibiotics lie in secondary metabolites produced by Xenorhabdus spp.? A review. Probiotics Antimicrob. Proteins 12, 1310–1320. doi: 10.1007/s12602-020-09688-x
Böszörményi, E., Érsek, T., Fodor, A., Fodor, A. M., Földes, L. S., Hevesi, M., et al. (2009). Isolation and activity of Xenorhabdus antimicrobial compounds against the plant pathogens Erwinia amylovora and Phytophthora nicotianae. J. Appl. Microbiol. 107, 746–759. doi: 10.1111/j.1365-2672.2009.04249.x
Bowen, D., Rocheleau, T. A., Blackburn, M., Andreev, O., Golubeva, E., Bhartia, R., et al. (1998). Insecticidal toxins from the bacterium Photorhabdus luminescens. Science 280, 2129–2132. doi: 10.1126/science.280.5372.2129
Cabanillas, H. E., Poinar, G. O. Jr., and Raulston, J. R. (1994). Steinernema riobravis n. sp. (Rhabditida: Steinernematidae) from Texas. Fundam. Appl. Nematol. 17, 123–131.
Castaneda-Alvarez, C., Prodan, S., Zamorano, A., San-Blas, E., and Aballay, E. (2021). Xenorhabdus lircayensis sp. nov., the symbiotic bacterium associated with the entomopathogenic nematode Steinernema unicornum. Int. J. Syst. Evol. Microbiol. 71:005151. doi: 10.1099/ijsem.0.005151
Challinor, V. L., and Bode, H. B. (2015). Bioactive natural products from novel microbial sources. Ann. N. Y. Acad. Sci. 1354, 82–97. doi: 10.1111/nyas.12954
Chaston, J. M., Suen, G., Tucker, S. L., Andersen, A. W., Bhasin, A., Bode, E., et al. (2011). The entomopathogenic bacterial endosymbionts Xenorhabdus and Photorhabdus: Convergent lifestyles from divergent genomes. PLoS One 6:e27909. doi: 10.1371/journal.pone.0027909
Chun, J., Oren, A., Ventosa, A., Christensen, H., Arahal, D. R., da Costa, M. S., et al. (2018). Proposed minimal standards for the use of genome data for the taxonomy of prokaryotes. Int. J. Syst. Evol. Microbiol. 68, 461–466.
Clarke, D. J. (2020). Photorhabdus: A tale of contrasting interactions. Microbiology 166, 335–348. doi: 10.1099/mic.0.000907
Cowles, C. E., and Goodrich-Blair, H. (2004). Characterization of a lipoprotein, NilC, required by Xenorhabdus nematophila for mutualism with its nematode host. Mol. Microbiol. 54, 464–477. doi: 10.1111/j.1365-2958.2004.04271.x
Crawford, J. M., Kontnik, R., and Clardy, J. (2010). Regulating alternative lifestyles in entomopathogenic bacteria. Curr. Biol. 20, 69–74. doi: 10.1016/j.cub.2009.10.059
Crawford, J. M., Portmann, C., Zhang, X., Roeffaers, M. B. J., and Clardy, J. (2012). Small molecule perimeter defense in entomopathogenic bacteria. Proc. Natl. Acad. Sci. U.S.A. 109, 10821–10826. doi: 10.1073/pnas.1201160109
Daborn, P. J., Waterfield, N., Silva, C. P., Au, C. P. Y., Sharma, S., and Ffrench-Constant, R. H. (2002). A single Photorhabdus gene, makes caterpillars floppy (mcf), allows Escherichia coli to persist within and kill insects. Proc. Natl. Acad. Sci. U.S.A. 99, 10742–10747. doi: 10.1073/pnas.102068099
Dathe, M., Nikolenko, H., Klose, J., and Bienert, M. (2004). Cyclization increases the antimicrobial activity and selectivity of arginine- and tryptophan-containing hexapeptides. Biochemistry 43, 9140–9150. doi: 10.1021/bi035948v
Dowling, A. J., Daborn, P. J., Waterfield, N. R., Wang, P., Streuli, C. H., and Ffrench-Constant, R. H. (2004). The insecticidal toxin Makes caterpillars floppy (Mcf) promotes apoptosis in mammalian cells. Cell. Microbiol. 6, 345–353. doi: 10.1046/j.1462-5822.2003.00357.x
Dreyer, J., Malan, A. P., and Dicks, L. M. T. (2018). Bacteria of the genus Xenorhabdus, a novel source of bioactive compounds. Front. Microbiol. 9:3177. doi: 10.3389/fmicb.2018.03177
Edgington, S., Buddie, A. G., Tymo, L., Hunt, D. J., Nguyen, K. B., France, A. I., et al. (2009). Steinernema australe n. sp. (Panagrolaimomorpha: Steinernematidae), a new entomopathogenic nematode from Isla Magdalena, Chile. Nematology 11, 699–717. doi: 10.1163/156854108X399326
Eleftherianos, I., Boundy, S., Joyce, S. A., Aslam, S., Marshall, J. W., Cox, R. J., et al. (2007). An antibiotic produced by an insect-pathogenic bacterium suppresses host defenses through phenoloxidase inhibition. Proc. Natl. Acad. Sci. U.S.A. 104, 2419–2424. doi: 10.1073/pnas.0610525104
Eugenia Nuñez-Valdez, M., Lanois, A., Pagès, S., Duvic, B., and Gaudriault, S. (2019). Inhibition of Spodoptera frugiperda phenoloxidase activity by the products of the Xenorhabdus rhabduscin gene cluster. PLoS One 14:e0212809. doi: 10.1371/journal.pone.0212809
Farmer, J. J., Jorgensen, J. H., Grimont, P. A., Akhurst, R. J., Poinar, G. O., Ageron, E., et al. (1989). Xenorhabdus luminescens (DNA hybridization group 5) from human clinical specimens. J. Clin. Microbiol. 27, 1594–1600. doi: 10.1128/jcm.27.7.1594-1600.1989
Ferreira, T., van Reenen, C. A., Endo, A., Spröer, C., Malan, A. P., and Dicks, L. M. T. (2013). Description of Xenorhabdus khoisanae sp. nov., the symbiont of the entomopathogenic nematode Steinernema khoisanae. Int. J. Syst. Evol. Microbiol. 63, 3220–3224. doi: 10.1099/ijs.0.049049-0
Ferreira, T., van Reenen, C. A., Endo, A., Tailliez, P., Pagès, S., Spröer, C., et al. (2014). Photorhabdus heterorhabditis sp. nov., a symbiont of the entomopathogenic nematode Heterorhabditis zealandica. Int. J. Syst. Evol. Microbiol. 64, 1540–1545. doi: 10.1099/ijs.0.059840-0
Ffrench-Constant, R., and Waterfield, N. (2005). “An ABC guide to the bacterial toxin complexes,” in Advances in applied microbiology, eds A. I. Laskin, J. W. Bennett, G. M. Gadd, and S. Sariaslani (San Diego, CA: Academic Press), 169–183. doi: 10.1016/S0065-2164(05)58005-5
Fischer-Le Saux, M., Mauléon, H., Constant, P., Brunel, B., and Boemare, N. (1998). PCR-ribotyping of Xenorhabdus and Photorhabdus isolates from the Caribbean region in relation to the taxonomy and geographic distribution of their nematode hosts. Appl. Environ. Microbiol. 64, 4246–4254. doi: 10.1128/AEM.64.11.4246-4254.1998
Fischer-Le Saux, M., Viallard, V., Brunel, B., Normand, P., and Boemare, N. E. (1999). Polyphasic classification of the genus Photorhabdus and proposal of new taxa: P. luminescens subsp. luminescens subsp. nov., P. luminescens subsp. akhurstii subsp. nov., P. luminescens subsp. laumondii subsp. nov., P. temperata sp. nov., P. temperata subsp. temperata subsp. nov. and P. asymbiotica sp. nov. Int. J. Syst. Bacteriol. 49, 1645–1656.
Ganguly, S., Kumar, S., and Rathour, K. S. (2010). Availability of biopesticidal nematodes to suit different agro-climatic region of india, for commercialisation. Indian J. Nematol. 40, 261–264.
Glaeser, S. P., Tobias, N. J., Thanwisai, A., Chantratita, N., Bode, H. B., and Kämpfer, P. (2017). Photorhabdus luminescens subsp. namnaonensis subsp. nov., isolated from Heterorhabditis baujardi nematodes. Int. J. Syst. Evol. Microbiol. 67, 1046–1051.
Grewal, P. S., Grewal, S. K., Malik, V. S., and Klein, M. G. (2002). Differences in susceptibility of introduced and native white grub species to entomopathogenic nematodes from various geographic localities. Biol. Control 24, 230–237. doi: 10.1016/S1049-9644(02)00025-7
Grimont, F., and Grimont, P. A. D. (2006). “The genus Serratia,” in The prokaryotes: A handbook on the biology of bacteria: Proteobacteria: gamma subclass, Vol. 6, eds M. Dworkin, S. Falkow, E. Rosenberg, K.-H. Schleifer, and E. Stackebrandt (New York, NY: Springer New York), 219–244. doi: 10.1007/0-387-30746-X_11
Grundmann, F., Kaiser, M., Kurz, M., Schiell, M., Batzer, A., and Bode, H. B. (2013). Structure determination of the bioactive depsipeptide xenobactin from Xenorhabdus sp. PB30.3. RSC Adv. 3, 22072–22077. doi: 10.1039/C3RA44721A
Grundmann, F., Kaiser, M., Schiell, M., Batzer, A., Kurz, M., Thanwisai, A., et al. (2014). Antiparasitic chaiyaphumines from entomopathogenic Xenorhabdus sp. PB61.4. J. Nat. Prod. 77, 779–783. doi: 10.1021/np4007525
Gualtieri, M., Aumelas, A., and Thaler, J.-O. (2009). Identification of a new antimicrobial lysine-rich cyclolipopeptide family from Xenorhabdus nematophila. J. Antibiot. 62, 295–302. doi: 10.1038/ja.2009.31
Gulcu, B., Hazir, S., and Kaya, H. K. (2012). Scavenger deterrent factor (SDF) from symbiotic bacteria of entomopathogenic nematodes. J. Invertebr. Pathol. 110, 326–333. doi: 10.1016/j.jip.2012.03.014
Hazir, S., Keskin, N., Stock, S. P., Kaya, H. K., and Özcan, S. (2003). Diversity and distribution of entomopathogenic nematodes (Rhabditida: Steinernematidae and Heterorhabditidae) in Turkey. Biodiv. Conserv. 12, 375–386.
Houard, J., Aumelas, A., Noël, T., Pages, S., Givaudan, A., Fitton-Ouhabi, V., et al. (2013). Cabanillasin, a new antifungal metabolite, produced by entomopathogenic Xenorhabdus cabanillasii JM26. J. Antibiot. (Tokyo) 66, 617–620. doi: 10.1038/ja.2013.58
Hu, K., Li, J., and Webster, J. M. (1997). Quantitative analysis of a bacteria-derived antibiotic in nematode-infected insects using HPLC-UV and TLC-UV methods. J. Chromatogr. B Biomed. Sci. Appl. 703, 177–183. doi: 10.1016/s0378-4347(97)00398-8
Hussein, M. A., and El-Souud, A. B. A. (2006). Isolation and characterization of two heterorhabditid and one steinernematid nematodes from Egypt. Int. J. Nematol. 16:7.
Imai, Y., Meyer, K. J., Iinishi, A., Favre-Godal, Q., Green, R., Manuse, S., et al. (2019). A new antibiotic selectively kills Gram-negative pathogens. Nature 576, 459–464. doi: 10.1038/s41586-019-1791-1
Jank, T., Lang, A. E., and Aktories, K. (2016). Rho-modifying bacterial protein toxins from Photorhabdus species. Toxicon 116, 17–22. doi: 10.1016/j.toxicon.2015.05.017
Kämpfer, P., Tobias, N. J., Ke, L. P., Bode, H. B., and Glaeser, S. P. (2017). Xenorhabdus thuongxuanensis sp. nov. and Xenorhabdus eapokensis sp. nov., isolated from Steinernema species. Int. J. Syst. Evol. Microbiol. 67, 1107–1114. doi: 10.1099/ijsem.0.001770
Kegler, C., and Bode, H. B. (2020). Artificial splitting of a non-ribosomal peptide synthetase by inserting natural docking domains. Angew. Chem. Int. Ed. 59, 13463–13467. doi: 10.1002/anie.201915989
Khan, A., Brooks, W. M., and Hirschmann, H. (1976). Chromonema heliothidis n. gen., n. sp. (Steinernematidae, Nematoda), a parasite of Heliothis zea (Noctuidae, Lepidoptera), and other insects. J. Nematol. 8, 159–168.
Kim, M., Oh, H.-S., Park, S.-C., and Chun, J. (2014). Towards a taxonomic coherence between average nucleotide identity and 16S rRNA gene sequence similarity for species demarcation of prokaryotes. Int. J. Syst. Evol. Microbiol. 64, 346–351. doi: 10.1099/ijs.0.059774-0
Kronenwerth, M., Bozhüyük, K. A. J., Kahnt, A. S., Steinhilber, D., Gaudriault, S., Kaiser, M., et al. (2014). Characterisation of taxlllaids A–G; natural products from Xenorhabdus indica. Chem. Eur. J. 20, 17478–17487. doi: 10.1002/chem.201403979
Kuwata, R., Qiu, L., Wang, W., Harada, Y., Yoshida, M., Kondo, E., et al. (2013). Xenorhabdus ishibashii sp. nov., isolated from the entomopathogenic nematode Steinernema aciari. Int. J. Syst. Evol. Microbiol. 63, 1690–1695. doi: 10.1099/ijs.0.041145-0
Lang, G., Kalvelage, T., Peters, A., Wiese, J., and Imhoff, J. F. (2008). Linear and cyclic peptides from the entomopathogenic bacterium Xenorhabdus nematophilus. J. Nat. Prod. 71, 1074–1077. doi: 10.1021/np800053n
Lebwohl, M. G., Stein Gold, L., Strober, B., Papp, K. A., Armstrong, A. W., Bagel, J., et al. (2021). Phase 3 trials of tapinarof cream for plaque psoriasis. N. Engl. J. Med. 385, 2219–2229. doi: 10.1056/NEJMoa2103629
Lengyel, K., Lang, E., Fodor, A., Szállás, E., Schumann, P., and Stackebrandt, E. (2005). Description of four novel species of Xenorhabdus, family Enterobacteriaceae: Xenorhabdus budapestensis sp. nov., Xenorhabdus ehlersii sp. nov., Xenorhabdus innexi sp. nov., and Xenorhabdus szentirmaii sp. nov. Syst. Appl. Microbiol. 28, 115–122. doi: 10.1016/j.syapm.2004.10.004
Lephoto Tiisetso, E., Featherston, J., and Gray Vincent, M. (2015). Draft whole-genome sequence of Serratia sp. strain TEL, associated with Oscheius sp. TEL-2014 (Nematoda: Rhabditidae) isolated from a Grassland in South Africa. Genome Announc. 3:e00747-15. doi: 10.1128/genomeA.00747-15
Lewis, K. (2020). The science of antibiotic discovery. Cell 181, 29–45. doi: 10.1016/j.cell.2020.02.056
Machado, R. A. R., Muller, A., Ghazal, S. M., Thanwisai, A., Pagès, S., Bode, H. B., et al. (2021a). Photorhabdus heterorhabditis subsp. aluminescens subsp. nov., Photorhabdus heterorhabditis subsp. heterorhabditis subsp. nov., Photorhabdus australis subsp. thailandensis subsp. nov., Photorhabdus australis subsp. australis subsp. nov., and Photorhabdus aegyptia sp. nov. isolated from Heterorhabditis entomopathogenic nematodes. Int. J. Syst. Evol. Microbiol. 71:4610. doi: 10.1099/ijsem.0.004610
Machado, R. A. R., Somvanshi, V. S., Muller, A., Kushwah, J., and Bhat, C. G. (2021b). Photorhabdus hindustanensis sp. nov., Photorhabdus akhurstii subsp. akhurstii subsp. nov., and Photorhabdus akhurstii subsp. bharatensis subsp. nov., isolated from Heterorhabditis entomopathogenic nematodes. Int. J. Syst. Evol. Microbiol. 71, 4998–5006. doi: 10.1099/ijsem.0.004998
Machado, R. A. R., Wüthrich, D., Kuhnert, P., Arce, C. C. M., Thönen, L., Ruiz, C., et al. (2018). Whole-genome-based revisit of Photorhabdus phylogeny: Proposal for the elevation of most Photorhabdus subspecies to the species level and description of one novel species Photorhabdus bodei sp. nov., and one novel subspecies Photorhabdus laumondii subsp. clarkei subsp. nov. Int. J. Syst. Evol. Microbiol. 68, 2664–2681. doi: 10.1099/ijsem.0.002820
Malan, A. P., Knoetze, R., and Moore, S. D. (2011). Isolation and identification of entomopathogenic nematodes from citrus orchards in South Africa and their biocontrol potential against false codling moth. J. Invertebr. Pathol. 108, 115–125. doi: 10.1016/j.jip.2011.07.006
Malan, A. P., Nguyen, K. B., and Addison, M. F. (2006). Entomopathogenic nematodes (Steinernematidae and Heterorhabditidae) from the southwestern parts of South Africa. Afr. Plant Prot. 12, 65–69.
McInerney, B., Taylor, W., Lacey, M., Akhurst, R., and Gregson, R. (1991). Biologically active metabolites from Xenorhabdus spp., Part 2. Benzopyran-1-one derivatives with gastroprotective activity. J. Nat. Prod. 54, 785–795. doi: 10.1021/np50075a006
Medini, D., Donati, C., Rappuoli, R., and Tettelin, H. (2020). “The pangenome: A data-driven discovery in biology,” in The Pangenome: diversity, dynamics and evolution of genomes, eds H. Tettelin and D. Medini (Cham: Springer International Publishing), 3–20. doi: 10.1007/978-3-030-38281-0_1
Moldovan, M. A., and Gelfand, M. S. (2018). Pangenomic definition of prokaryotic species and the phylogenetic structure of Prochlorococcus spp. Front. Microbiol. 9:428. doi: 10.3389/fmicb.2018.00428
Mothupi, B. (2016). Genomics of entomopathogenic bacterial endosymbiont species associated with desiccation tolerant entomopathogenic nematode. Available online at: https://wiredspace.wits.ac.za/xmlui/bitstream/handle/10539/21282/Boipelo%20Mothupi_MSc_FINAL.pdf?isAllowed=y&sequence=1 (accessed July 13, 2022).
Mothupi, B., Featherston, J., and Gray, V. (2015). Draft whole-genome sequence and annotation of Xenorhabdus griffiniae strain BMMCB associated with the South African entomopathogenic nematode Steinernema khoisanae Strain BMMCB. Genome Announc. 3:e00785-15. doi: 10.1128/genomeA.00785-15
Nǵanǵa, P. N., Ebner, J. K., Plessner, M., Aktories, K., and Schmidt, G. (2019). Engineering Photorhabdus luminescens toxin complex (PTC) into a recombinant injection nanomachine. Life Sci. Alliance 2:e201900485. doi: 10.26508/lsa.201900485
Nguyen, K. B., and Smart, G. C. (1990). Steinernema scapterisci n. sp. (Rhabditida: Steinernematidae). J. Nematol. 22, 187–199.
Nishimura, Y., Hagiwara, A., Suzuki, T., and Yamanaka, S. (1994). Xenorhabdus japonicus sp. nov. associated with the nematode Steinernema kushidai. World J. Microbiol. Biotechnol. 10, 207–210. doi: 10.1007/BF00360889
Ogier, J.-C., Pagès, S., Frayssinet, M., and Gaudriault, S. (2020). Entomopathogenic nematode-associated microbiota: From monoxenic paradigm to pathobiome. Microbiome 8:25. doi: 10.1186/s40168-020-00800-5
Ohlendorf, B., Simon, S., Wiese, J., and Imhoff, J. F. (2011). Szentiamide, an N-formylated cyclic depsipeptide from Xenorhabdus szentirmaii DSM 16338T. Nat. Prod. Commun. 6, 1247–1250.
Pantel, L., Florin, T., Dobosz-Bartoszek, M., Racine, E., Sarciaux, M., Serri, M., et al. (2018). Odilorhabdins, antibacterial agents that cause miscoding by binding at a new ribosomal site. Molecular Cell 70, 83–94.e7. doi: 10.1016/j.molcel.2018.03.001
Park, D., Ciezki, K., Van Der Hoeven, R., Singh, S., Reimer, D., Bode, H. B., et al. (2009). Genetic analysis of xenocoumacin antibiotic production in the mutualistic bacterium Xenorhabdus nematophila. Mol. Microbiol. 73, 938–949. doi: 10.1111/j.1365-2958.2009.06817.x
Peel, M. M., Alfredson, D. A., Gerrard, J. G., Davis, J. M., Robson, J. M., McDougall, R. J., et al. (1999). Isolation, identification, and molecular characterization of strains of Photorhabdus luminescens from infected humans in Australia. J. Clin. Microbiol. 37, 3647–3653. doi: 10.1128/JCM.37.11.3647-3653.1999
Phan, K. L., Nguyen, N. C., and Moens, M. (2001). Steinernema sangi sp n. (Rabditida: Steinernematidae) from Vietnam. Russ. J. Nematol. 9, 1–7.
Phan, K. L., Spiridonov, S. E., Subbotin, S. A., and Moens, M. (2006). Four new species of Steinernema Travassos, 1928 with short infective juveniles from Vietnam. Russ. J. Nematol. 14:11.
Plichta, K. L., Joyce, S. A., Clarke, D., Waterfield, N., and Stock, S. P. (2009). Heterorhabditis gerrardi n. sp. (Nematoda: Heterorhabditidae): The hidden host of Photorhabdus asymbiotica (Enterobacteriaceae: Gamma-Proteobacteria). J. Helminthol. 83, 309–320. doi: 10.1017/S0022149X09222942
Poinar, G. O. (1978). Generation polymorphism in Neoaplectana glaseri steiner (Steinernematidae: Nematoda), redescribed from strigoderma arboricola (Fab.) Scarabaeidae: Coleoptera) in North Carolina. Nematologica 24, 105–114. doi: 10.1163/187529278X00137
Poinar, G. O. Jr., and Leutenegger, R. (1968). Anatomy of the infective and normal third-stage juveniles of Neoaplectana carpocapsae Weiser (Steinernematidae: Nematoda). J. Parasitol. 54, 340–350.
Poinar, G. O., Hansen, E. L., Yarwood, E. A., and Weiser, J. (1972). Clarification of the status of the DD-136 strain of Neoaplectana carpocapsae weiser. Nematologica 18, 288–290. doi: 10.1163/187529272X00539
Proschak, A., Schultz, K., Herrmann, J., Dowling, A. J., Brachmann, A. O., Ffrench-Constant, R., et al. (2011). Cytotoxic fatty acid amides from Xenorhabdus. ChemBioChem 12, 2011–2015. doi: 10.1002/cbic.201100223
Proschak, A., Zhou, Q., Schöner, T., Thanwisai, A., Kresovic, D., Dowling, A., et al. (2014). Biosynthesis of the insecticidal xenocyloins in Xenorhabdus bovienii. Chembiochem 15, 369–372. doi: 10.1002/cbic.201300694
Qiu, L., Fang, Y., Zhou, Y., Pang, Y. I., and Nguyen, K. B. (2004). Steinernema guangdongense sp. n. (Nematoda: Steinernematidae), a new entomopathogenic nematode from southern China with a note on S. serratum (nomen nudum). Zootaxa 704, 1–20.
Qiu, L., Yan, X., Zhou, Y., Nguyen, K. B., and Pang, Y. (2005). Steinernema aciari sp. n. (Nematoda: Steinernematidae), a new entomopathogenic nematode from Guangdong, China. J. Invertebr. Pathol. 88, 58–69. doi: 10.1016/j.jip.2004.09.004
Racine, E., and Gualtieri, M. (2019). From worms to drug candidate: The story of Odilorhabdins, a new class of antimicrobial agents. Front. Microbiol. 10:2893. doi: 10.3389/fmicb.2019.02893
Reimer, D., Cowles, K. N., Proschak, A., Nollmann, F. I., Dowling, A. J., Kaiser, M., et al. (2013). Rhabdopeptides as insect-specific virulence factors from entomopathogenic bacteria. ChemBioChem 14, 1991–1997. doi: 10.1002/cbic.201300205
Reimer, D., Nollmann, F. I., Schultz, K., Kaiser, M., and Bode, H. B. (2014). Xenortide biosynthesis by entomopathogenic Xenorhabdus nematophila. J. Nat. Prod. 77, 1976–1980. doi: 10.1021/np500390b
Richter, M., and Rosselló-Móra, R. (2009). Shifting the genomic gold standard for the prokaryotic species definition. Proc. Natl. Acad. Sci. U.S.A. 106, 19126–19131. doi: 10.1073/pnas.0906412106
Roderer, D., Schubert, E., Sitsel, O., and Raunser, S. (2019). Towards the application of Tc toxins as a universal protein translocation system. Nat. Commun. 10:5263. doi: 10.1038/s41467-019-13253-8
Rodríguez, A. A., Otero-González, A., Ghattas, M., and Ständker, L. (2021). Discovery, optimization, and clinical application of natural antimicrobial peptides. Biomedicines 9:1381. doi: 10.3390/biomedicines9101381
Román, J., and Figueroa, W. (1994). Steinernema puertoricensis n. sp. (Rhabditida: Steinernematidae), a new entomopathogenic nematode from Puerto Rico. J. Agric. Univ. Puerto Rico 78, 167–175.
Schimming, O., Fleischhacker, F., Nollmann, F. I., and Bode, H. B. (2014). Yeast homologous recombination cloning leading to the novel peptides ambactin and xenolindicin. ChemBioChem 15, 1290–1294. doi: 10.1002/cbic.201402065
Serepa-Dlamini, M. H., and Gray, V. M. (2018). A new species of entomopathogenic nematode Oscheius safricana n. sp. (Nematoda: Rhabditidae) from South Africa. Arch. Phytopathol. Plant Prot. 51, 309–321.
Shan, S., Wang, W., Song, C., Wang, M., Sun, B., Li, Y., et al. (2019). The symbiotic bacteria Alcaligenes faecalis of the entomopathogenic nematodes Oscheius spp. exhibit potential biocontrol of plant- and entomopathogenic fungi. Microb. Biotechnol. 12, 459–471. doi: 10.1111/1751-7915.13365
Sheets, J. J., Hey, T. D., Fencil, K. J., Burton, S. L., Ni, W., Lang, A. E., et al. (2011). Insecticidal toxin complex proteins from Xenorhabdus nematophilus: Structure and pore formation. J. Biol. Chem. 286, 22742–22749. doi: 10.1074/jbc.m111.227009
Shi, Y.-M., Hirschmann, M., Shi, Y.-N., Ahmed, S., Abebew, D., Tobias, N. J., et al. (2022). Global analysis of biosynthetic gene clusters reveals conserved and unique natural products in entomopathogenic nematode-symbiotic bacteria. Nat. Chem. 14, 701–712. doi: 10.1038/s41557-022-00923-2
Somvanshi, V. S., Lang, E., Ganguly, S., Swiderski, J., Saxena, A. K., and Stackebrandt, E. (2006). A novel species of Xenorhabdus, family Enterobacteriaceae: Xenorhabdus indica sp. nov., symbiotically associated with entomopathogenic nematode Steinernema thermophilum Ganguly and Singh, 2000. Syst. Appl. Microbiol. 29, 519–525. doi: 10.1016/j.syapm.2006.01.004
Song, C. J., Seo, S., Shrestha, S., and Kim, Y. (2011). Bacterial metabolites of an entomopathogenic bacterium, Xenorhabdus nematophila, inhibit a catalytic activity of phenoloxidase of the diamondback moth, Plutella xylostella. J. Microbiol. Biotechnol. 21, 317–322.
Stilwell, M. D., Cao, M., Goodrich-Blair, H., and Weibel, D. B. (2018). Studying the symbiotic bacterium Xenorhabdus nematophila in individual, living Steinernema carpocapsae nematodes using microfluidic systems. mSphere 3:e00530-17. doi: 10.1128/msphere.00530-17
Stock, S. P. (1998). Steinernema siamkayai n. sp. (Rhabditida: Steinernematidae), an entomopathogenic nematode from Thailand. Syst. Parasitol. 41, 105–113. doi: 10.1023/A:1006087017195
Stock, S. P., and Koppenhöfer, A. (2003). Steinernema scarabaei n. sp. (Rhabditida: Steinernematidae), a natural pathogen of scarab beetle larvae (Coleoptera: Scarabaeidae) from New Jersey, USA. Nematology 5, 191–204. doi: 10.1163/156854103767139680
Stock, S. P., Griffin, C. T., and Chaerani, R. (2004). Morphological and molecular characterisation of Steinernema hermaphroditum n. sp. (Nematoda: Steinernematidae), an entomopathogenic nematode from Indonesia, and its phylogenetic relationships with other members of the genus. Nematology 6, 401–412.
Sudershan, G., and Singh, L. K. (2000). Steinernema thermophilum sp. n. (Rhabditida: Steinernematidae) from India. Int. J. Nematol. 10, 183–191.
Tailliez, P., Laroui, C., Ginibre, N., Paule, A., Pagès, S., and Boemare, N. (2010). Phylogeny of Photorhabdus and Xenorhabdus based on universally conserved protein-coding sequences and implications for the taxonomy of these two genera. Proposal of new taxa: X. vietnamensis sp. nov., P. luminescens subsp. caribbeanensis subsp. nov., P. luminescens subsp. hainanensis subsp. nov., P. temperata subsp. khanii subsp. nov., P. temperata subsp. tasmaniensis subsp. nov., and the reclassification of P. luminescens subsp. thracensis as P. temperata subsp. thracensis comb. nov. Int. J. Syst. Evol. Microbiol. 60, 1921–1937. doi: 10.1099/ijs.0.014308-0
Tailliez, P., Pagès, S., Edgington, S., Tymo, L. M., and Buddie, A. G. (2012). Description of Xenorhabdus magdalenensis sp. nov., the symbiotic bacterium associated with Steinernema australe. Int. J. Syst. Evol. Microbiol. 62, 1761–1765. doi: 10.1099/ijs.0.034322-0
Tailliez, P., Pagès, S., Ginibre, N., and Boemare, N. (2006). New insight into diversity in the genus Xenorhabdus, including the description of ten novel species. Int. J. Syst. Evol. Microbiol. 56, 2805–2818. doi: 10.1099/ijs.0.64287-0
Tallosi, B., Peters, A., and Ehlers, R.-U. (1995). Steinernema bicornutum sp. n. (Rhabditida: Steinernematidae) from Vojvodina, Yugoslavia. Russ. J. Nematol. 3, 71–80.
Thomas, G. M., and Poinar, G. O. (1979). Xenorhabdus gen. nov., a genus of entomopathogenic, nematophilic bacteria of the family enterobacteriaceae. Int. J. Syst. Evol. Microbiol. 29, 352–360.
Tobias, N. J., Wolff, H., Djahanschiri, B., Grundmann, F., Kronenwerth, M., Shi, Y.-M., et al. (2017). Natural product diversity associated with the nematode symbionts Photorhabdus and Xenorhabdus. Nat. Microbiol. 2, 1676–1685. doi: 10.1038/s41564-017-0039-9
Torres-Barragan, A., Suazo, A., Buhler, W. G., and Cardoza, Y. J. (2011). Studies on the entomopathogenicity and bacterial associates of the nematode Oscheius carolinensis. Biol. Control 59, 123–129. doi: 10.1016/j.biocontrol.2011.05.020
Tóth, T., and Lakatos, T. (2008). Photorhabdus temperata subsp. cinerea subsp. nov., isolated from Heterorhabditis nematodes. Int. J. Syst. Evol. Microbiol. 58, 2579–2581. doi: 10.1099/ijs.0.2008/000273-0
Ullah, I., Khan, A. L., Ali, L., Khan, A. R., Waqas, M., Lee, I.-J., et al. (2014). An insecticidal compound produced by an insect-pathogenic bacterium suppresses host defenses through phenoloxidase inhibition. Molecules 19, 20913–20928. doi: 10.3390/molecules191220913
Vigneux, F., Zumbihl, R., Jubelin, G., Ribeiro, C., Poncet, J., Baghdiguian, S., et al. (2007). The xaxAB genes encoding a new apoptotic toxin from the insect pathogen Xenorhabdus nematophila are present in plant and human pathogens*. J. Biol. Chem. 282, 9571–9580. doi: 10.1074/jbc.M604301200
Waterfield, N. R., Ciche, T., and Clarke, D. (2009). Photorhabdus and a host of hosts. Annu. Rev. Microbiol. 63, 557–574. doi: 10.1146/annurev.micro.091208.073507
Waturu, C. N., Hunt, D. J., and Reid, A. P. (1997). Steinernema karii sp. n. (Nematoda: Steinernematidae), a new entomopathogenic nematode from Kenya. Int. J. Nematol. 7, 68–75.
Wenski, S. L., Cimen, H., Berghaus, N., Fuchs, S. W., Hazir, S., and Bode, H. B. (2020). Fabclavine diversity in Xenorhabdus bacteria. Beilstein J. Org. Chem. 16, 956–965. doi: 10.3762/bjoc.16.84
Wu, Q., Qin, Y., Liao, W., Zhang, M., Yang, Y., Zhang, P., et al. (2022). Cost-effectiveness of enfortumab vedotin in previously treated advanced urothelial carcinoma. Ther. Adv. Med. Oncol. 14:17588359211068732. doi: 10.1177/17588359211068733
Xiao, Y., Meng, F., Qiu, D., and Yang, X. (2012). Two novel antimicrobial peptides purified from the symbiotic bacteria Xenorhabdus budapestensis NMC-10. Peptides 35, 253–260. doi: 10.1016/j.peptides.2012.03.027
Yang, Q., Zhang, J., Li, T., Liu, S., Song, P., Nangong, Z., et al. (2017). PirAB protein from Xenorhabdus nematophila HB310 exhibits a binary toxin with insecticidal activity and cytotoxicity in Galleria mellonella. J. Invertebr. Pathol. 148, 43–50. doi: 10.1016/j.jip.2017.04.007
Yang, X., Qiu, D., Yang, H., Liu, Z., Zeng, H., and Yuan, J. (2011). Antifungal activity of xenocoumacin 1 from Xenorhabdus nematophilus var. pekingensis against Phytophthora infestans. World J. Microbiol. Biotechnol. 27, 523–528. doi: 10.1007/s11274-010-0485-5
Ye, W., Torres-Barragan, A., and Cardoza, Y. J. (2010). Oscheius carolinensis n. sp. (Nematoda: Rhabditidae), a potential entomopathogenic nematode from vermicompost. Nematology 12, 121–135.
Zhang, C.-X., Yang, S.-Y., Xu, M.-X., Sun, J., Liu, H., Liu, J.-R., et al. (2009). Serratia nematodiphila sp. nov., associated symbiotically with the entomopathogenic nematode Heterorhabditidoides chongmingensis (Rhabditida: Rhabditidae). Int. J. Syst. Evol. Microbiol. 59, 1603–1608. doi: 10.1099/ijs.0.65718-0
Zhang, J., Cai, L., and Zheng, M. (2022). A novel topical treatment for plaque psoriasis: Benvitimod/tapinarof. J. Am. Acad. Dermatol. 86, e137–e138. doi: 10.1016/j.jaad.2021.10.053
Zhao, L., Awori, R. M., Kaiser, M., Groß, J., Opatz, T., and Bode, H. B. (2019). Structure, biosynthesis, and bioactivity of photoditritide from Photorhabdus temperata Meg1. J. Nat. Prod. 82, 3499–3503. doi: 10.1021/acs.jnatprod.9b00932
Zhao, L., Kaiser, M., and Bode, H. B. (2018). Rhabdopeptide/Xenortide-like peptides from Xenorhabdus innexi with terminal amines showing potent antiprotozoal activity. Org. Lett. 20, 5116–5120. doi: 10.1021/acs.orglett.8b01975
Zhao, L., Vo, T. D., Kaiser, M., and Bode, H. B. (2020). Phototemtide A, a cyclic lipopeptide heterologously expressed from Photorhabdus temperata Meg1, shows selective antiprotozoal activity. Chembiochem 21, 1288–1292. doi: 10.1002/cbic.201900665
Zhao, M., Lepak, A. J., Marchillo, K., VanHecker, J., and Andes, D. R. (2018). In Vivo pharmacodynamic characterization of a Novel Odilorhabdin Antibiotic, NOSO-502, against Escherichia coli and Klebsiella pneumoniae in a murine thigh infection model. Antimicrob. Agents Chemother. 62:e01067-18. doi: 10.1128/AAC.01067-18
Zhou, G., Yang, H., Wang, F., Bao, H., Wang, G., Hou, X., et al. (2017). Oscheius microvilli n. sp. (Nematoda: Rhabditidae): A facultatively pathogenic nematode from Chongming Island, China. J. Nematol. 49, 33–41. doi: 10.21307/jofnem-2017-044
Zhou, Q., Grundmann, F., Kaiser, M., Schiell, M., Gaudriault, S., Batzer, A., et al. (2013). Structure and biosynthesis of xenoamicins from entomopathogenic Xenorhabdus. Chem. Eur. J. 19, 16772–16779. doi: 10.1002/chem.201302481
Keywords: nematophilic bacteria, Xenorhabdus bacteria, Photorhabdus bacteria, entomopathogenic nematode (EPN), natural product (NP), pangenomics, drug development
Citation: Awori RM (2022) Nematophilic bacteria associated with entomopathogenic nematodes and drug development of their biomolecules. Front. Microbiol. 13:993688. doi: 10.3389/fmicb.2022.993688
Received: 14 July 2022; Accepted: 24 August 2022;
Published: 15 September 2022.
Edited by:
Claudia S. L. Vicente, University of Évora, PortugalReviewed by:
Kazuki Sato, RIKEN Yokohama, JapanCopyright © 2022 Awori. This is an open-access article distributed under the terms of the Creative Commons Attribution License (CC BY). The use, distribution or reproduction in other forums is permitted, provided the original author(s) and the copyright owner(s) are credited and that the original publication in this journal is cited, in accordance with accepted academic practice. No use, distribution or reproduction is permitted which does not comply with these terms.
*Correspondence: Ryan Musumba Awori, cnlhbi1tdXN1bWJhLmF3b3JpQGVsYWtpc3Rvc2Jpb3NjaWVuY2VzLmNvbQ==