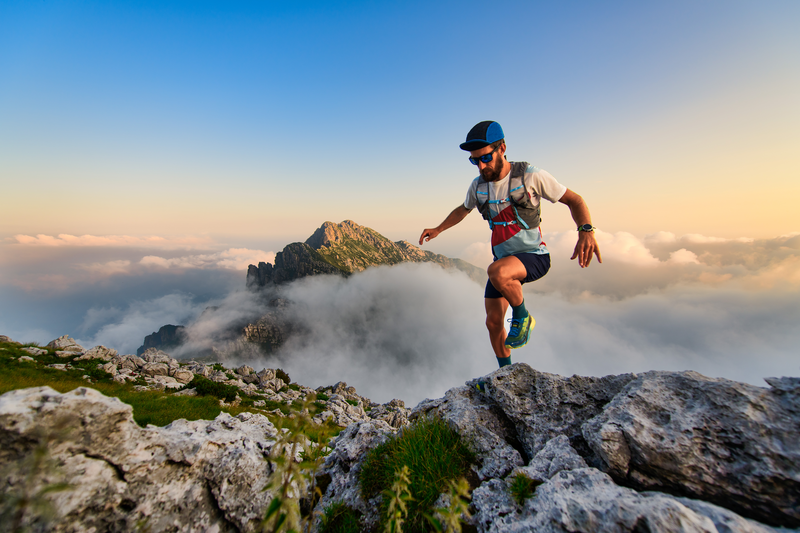
95% of researchers rate our articles as excellent or good
Learn more about the work of our research integrity team to safeguard the quality of each article we publish.
Find out more
ORIGINAL RESEARCH article
Front. Microbiol. , 09 September 2022
Sec. Microbe and Virus Interactions with Plants
Volume 13 - 2022 | https://doi.org/10.3389/fmicb.2022.993147
This article is part of the Research Topic Rhizosphere conversation among the Plant-Plant Microbiome-Soil under Consecutive Monoculture Regimes View all 8 articles
Acid rain not only has serious harm to the environment, but also has the same threat to plants, but the invasive plant Alternanthera philoxeroides still grows well compared to the native plant Alternanthera sessilis under acid rain stress. However, the underlying mechanism of resistance to the acid rain environment in invasive Alternanthera philoxeroides remains unclear. In the current study, we comparatively analyzed the plant physiological characteristics, soil physicochemical properties, and rhizosphere microbial communities of invasive A. philoxeroides and native A. sessilis under different pH condition. The simulated acid rain had a significant inhibitory effect on the morphological and physiological traits of A. philoxeroides and A. sessilis and reduced the soil nutrient content. However, A. philoxeroides was more tolerant of acid rain. Compared with CK, simulated acid rain treatment at pH 2.5 significantly increased the Chao1, ACE, and Shannon indexes of A. philoxeroides microorganisms. Under simulated acid rain treatment at pH 2.5, the fungal flora Chao1, ACE and Shannon index were significantly higher than those of CK by 14.5%, 12.4%, and 30.4%, respectively. The dominant bacterial phyla of soil bacteria were Proteobacteria, Actinobacteria, Bacteroidota, Actinobacteria, Firmicutes, Myxococcota, Chloroflexi, Patescibacteria, Gemmatimonadota, Verrucomicrobiota, and Armatimonadota. The dominant fungi were Ascomycota, Basidiomycota, Rozellomycota, and Olpidiomycota. The bacterial and fungal diversity and structure of A. philoxeroides and A. sessilis showed the greatest difference between the pH 2.5 treatment and CK. Redundancy analysis showed that electrical conductivity (EC) and total phosphorus (TP) were the main factors changing the bacterial communities, and available phosphorus (AP), organic matter (OM), EC, and pH were the main factors changing the fungal communities. This study contributes to the microbial community structure of the invasive plant A. philoxeroides and provides a theoretical basis for studying the invasion mechanism of invasive plants under acid rain.
Acid rain has been a global environmental issue since 1980 and has had an adverse impact on human health and ecosystem functions (Liu et al., 2019; Shu et al., 2019). At present, China’s acid rain area accounts for about 40% of the land area (Lv et al., 2014), and it has become the third largest acid rain pollution area after Europe and North America (Ramlall et al., 2015). The impact of acid rain on terrestrial ecosystems has attracted extensive attention (Wei et al., 2020; Zhang et al., 2020). Plants are an important part of terrestrial ecosystems and are the direct and largest victims of acid rain pollution (Ramlall et al., 2015). When plants are exposed to acid rain stress, the wax on the surface of plant leaves is eroded, accumulating harmful cations, reducing the chlorophyll content and photosynthetic rate, resulting in leaf aging, affecting plant biomass, inhibiting plant growth, and causing death (Zhang et al., 2020). In addition, acid rain has significant effects on community structure and diversity of rhizosphere microorganisms (Shafer, 1992; Wang et al., 2014). At present, research on the effect of acid rain on plants mainly focuses on the damage mechanism of acid rain on crops and economic crops and the resistance mechanism in plants (Du et al., 2017; Zheng et al., 2022), while studies on the response mechanism of alien invasive plants to acid rain are relatively limited.
Biological invasion is a common environmental problem faced by countries all over the world, and is considered the second largest threat to biodiversity worldwide, forming a mono-dominant population by competing with local species, resulting in the reduction in local biodiversity (Wang et al., 2021a). Alternanthera philoxeroides is a malignant invasive weed native to South America. It is a perennial plant of the genus Alternanthera Forsk in Amaranthaceae. It propagates vegetatively by stem nodes (Pan et al., 2006). Because it can grow on land and water and has a strong reproductive ability and phenotypic plasticity, it has spread all over the Yangtze River region and southern provinces in China. It has had a huge impact on China’s ecosystems and social economy (Wang et al., 2021b). Alternanthera sessilis is a native species of the invasive plant A. philoxeroides in China. It occupies the same niche as A. philoxeroides and can coexist in the same plant communities (Sun et al., 2010). Field investigations have found that A. philoxeroides under acid rain stress showed a good growth trend (Guo et al., 2012), and comparison of the different performances of invasive species and related species facing environmental interference is an effective method to reveal species invasion (Wang et al., 2009). However, the underlying mechanism of resistance to acid rain in invasive A. philoxeroides remains unclear.
Soil is the largest recipient of acid rain in terrestrial ecosystem (Wei et al., 2017). Acid rain can change soil physicochemical properties and affect soil ecosystems (Wu et al., 2016b). Additionally, soil microorganisms are an important part of soil ecosystems (Falkowski et al., 2008), maintaining ecosystem stability and functional diversity by decomposing soil organic matter and participating in the circulation and flow of carbon, nitrogen, phosphorus, and sulfur in the ecosystem (Averill and Classen, 2014; Whitaker et al., 2014). When the ecosystem is disturbed by natural or human factors, microbial composition, structure, and function change accordingly (Chu et al., 2016). Most studies show that soil acidification caused by acid rain changes soil physicochemical properties (Mohsen and Elaheh, 2012; Liu et al., 2020), reduces soil nutrients, decreases soil microbial biomass, and adversely affects microbial community structure (Xu et al., 2015; Shu et al., 2021), thereby affecting plant growth and development (El-Tarabily et al., 2007). In some short-term experimental studies, the activity of soil microbial communities’ diversity and richness has a positive response to acid rain (Liu et al., 2017; Li et al., 2020). However, most of these studies are about the response of farmland and forest soil microbial community structure and function under acid rain conditions, while there is little information about the response of invasive plant soil microbial communities under acid rain conditions.
In the current study, we focused on the invasive plant A. philoxeroides and the native plant A. sessilis. We investigated the effects of acid rain at different pH values (2.5, 4.5, and 7.0) on the phenotypic characteristics, physiological, and ecological characteristics, soil physicochemical properties, and rhizosphere microbial communities of invasive A. philoxeroides and native A. sessilis. The ecological adaptability and tolerance of A. philoxeroides and A. sessilis under acid rain stress were analyzed to understand the invasion mechanism of A. philoxeroides when acid rain occurs and to provide theoretical support for research on invasive plants.
The experiment was carried out in a greenhouse at Anhui Agricultural University, China (31°51′50′N, 117°14′48′E). Both A. philoxeroides and A. sessilis were collected from the terrestrial environment around Nangang (31°52′27′N, 116°43′34′E), Shushan District, Hefei City, Anhui Province, China. We selected A. philoxeroides and A. sessilis plants with good growth and similar stem diameters as cut seedlings and cut them short and evenly with one node (1.5 cm on both ends of the node). They were then cultivated in a warm room. When the seedlings grew to 2–4 cm, we selected seedlings with similar growth for the pot experiment.
According to acid rain monitoring in China, the simulated acid rain was configured into two acidic gradients with pH values of 2.5 and 4.5 according to the volume ratio of H2SO4:HNO3 (5:1; Liu et al., 2018). The seedlings of the two selected plants were transplanted into pots (19 cm × 13 cm × 5 cm) with 350 g nutrient soil. The contents of nitrogen, phosphorus, and potassium in soil were 11.5, 2.7, and 8.3 g/kg, respectively. Four seedlings were planted in each pot, and six replicates were set for each treatment. The experiment was carried out in a greenhouse (EAV = 500 lux, illumination for 12 h, Rh = 75%) in September 2020. Acid rain or deionized water (control, CK) was sprayed on the plants every 2 days. Each application was carried out using a 2-L sprayer 20 cm above the plant top leaves, with 200 ml of each solution (calculated by annual precipitation in Hefei). During the experiment, the pot positions were randomly changed every day to reduce the effects of environmental differences, such as light and temperature, on plant growth. The experiment lasted 40 days.
After the experiment, plant height was measured using a ruler. The plant was placed in an envelope and dried in an oven at 65°C until a constant weight. The plant dry weight was measured using an electronic balance with an accuracy of 0.001 g. The chlorophyll content in plant leaves was determined using the acetone method (Dolatabadian et al., 2013). The photosynthesis index (net photosynthetic rate—Pn; stomatal conductance—Gs; intercellular carbon dioxide concentration—Ci; and transpiration rate—Tr) was measured using a portable photosynthesis system (6400XT, LI-COR, Lincoln, NE, United States).
The soil pH was determined using the potentiometric method, and the electrical conductivity (EC) was measured using a conductivity meter (Meng et al., 2019). The organic matter (OM) was determined using the external heating potassium dichromate volumetric method(Wu et al., 2022). Total nitrogen (TN) was determined using the semi-micro Kjeldahl method, and total phosphorus (TP) and total potassium (TK) were measured using the NaOH melting method. Ammonium nitrogen (AN) was determined using Indigo colorimetry. The Olsen method was used to determine the available phosphorus (AP) content (Ren et al., 2021).
At the end of the experiment, fresh rhizosphere soil of two species under pH 2.5, pH 4.5 and CK was collected by root shaking method in sterile centrifuge tubes and recorded as AP1, AP2, AP3, AS1, AS2, and AS3, respectively. Genomic DNA was extracted from soil samples using an OMEGA soil DNA extraction kit (MP Biomedicals, United States). The DNA extract was examined on 1% agarose gel, and NanoDrop 2000 (Thermo Scientific, Wilmington, NC, United States) was used to determine the DNA concentration and purity. The V3–V4 region of the bacterial 16 s RNA gene using primers (338F: 5′-ACTCCTACGGGAGGCAGCAG-3′; 806R: 5′-GGACTACHVGGGTWTCTAAT-3′) was amplified by ABI GeneAmp® 9,700(ABI, CA, United States). The fungal ITS region was amplified using the following primers (ITS1F:5′-CTTGGTCATTTAGAGGAAGTAA-3′; ITS2R:5′-GCTGCGTTCTTCATCGATGC-3′). The PCR amplification of 16 s RNA gene was performed as follows: initial denaturation at 95°C for 3 min, followed by 27 cycles of denaturing at 95°C for 30 s, annealing at 55°C for 30 s and extension at 72°C for 45 s, and single extension at 72°C for 10 min, and end at 10°C. Similarly, the PCR amplification of fungal ITS region was only 35 cycles, and its parameters were consistent with the PCR amplification program of 16 s RNA. The PCR reaction mixture contained Takara rTaq DNA polymerase in a 20 μl reaction system with 2 μl of 10× Fastpfu buffer, 2 μl of 2.5 mM dNTPs, 0.8 μl forward primer (5 μM), 0.8 μl reverse primer (5 μM), 0.2 μl rTaq polymer, 0.2 μl BSA, 10 ng template DNA, and supplemented with ddH2O to 20 μl. Each sample was replicated three times. The PCR products of the same sample were mixed and detected using 2% agarose gel electrophoresis. The PCR product was recovered using the AxyPrepDNA gel Recovery Kit (AXYGEN company), and the PCR product was detected and quantified using the QuantiFluor™—ST blue fluorescence quantitative system (Promega, United States). Sequencing was carried out on the Illumina MiSeq PE300 platform.
The sequencing file was transferred from FASTP0.19.6 software filtering (Chen et al., 2018) to FLASH1.2.7 (Mago and Salzberg, 2011) to screen and merge the sequences, using the following steps: (1) The bases were filtered with a mass value of less than 20 at the tail of reads. A 50 bp window was set; if the average mass value in the window was less than 20, the back-end bases were cut from the window. The reads were filtered with a mass value of less than 50 bp after quality control, and reads containing N bases were removed. (2) According to the overlapping relationship between PE reads, paired reads were spliced into a sequence, and the minimum overlap length was 10 bp. (3) The maximum allowable mismatch ratio of the overlapping regions of splicing sequences was 0.2, and the non-conforming sequences were screened. (4) The samples were distinguished according to the barcodes and primers at the beginning and end of the sequences, and the sequence direction was adjusted.
UPARSE (version 7.0.1090) was used for operational taxonomic unit (OTU) clustering of non-repeat sequences (excluding single sequences) according to a 97% similarity to identify and delete chimeric sequences (Stakenrandt and Goebel, 1994; Edgar, 2013). The ɑ-diversity (Shannon, Simpson, CHAO1, ACE) of the microbial communities was calculated using QIIME1.9.1 software. The β-diversity and difference OTUs were calculated by the vegan and edgeR packages in R language (Version 3.5.5) software. The correlation analysis between microbial diversity, relative abundance, and soil physicochemical properties was performed using Pearson and Spearman correlations. All experimental data were analyzed using Excel and IBM SPSS Statistics.
The plant height and biomass of A. philoxeroides and A. sessilis decreased with a decrease in pH value and reached a minimum at pH 2.5. Compared with CK, plant height of A. philoxeroides and A. sessilis decreased by 4.8% and 25.5%, and biomass of A. philoxeroides and A. sessilis decreased by 22.2% and 29.2%, respectively (Figure 1). With a decrease in pH value, the contents of chlorophyll a, chlorophyll b, and total chlorophyll decreased. Compared with CK, chlorophyll of A. philoxeroides and A. sessilis decreased by 14.0% and 40.6%, chlorophyll b of A. philoxeroides and A. sessilis decreased by 3.8% and 31.3%, and total chlorophyll of A. philoxeroides and A. sessilis decreased by 11.8% and 39.1%, respectively. Only the intercellular carbon dioxide concentration of A. philoxeroides increased and then decreased with the decrease in pH value, and its other parameters and all gas exchange parameters of A. sessilis showed a decreasing trend. Compared with CK, the net photosynthetic rate (Pn) decreased by 44.3% and 54.1%, the stomatal conductance (Gs) decreased by 60.5% and 60.7%, the intercellular carbon dioxide concentration (Ci) decreased by 7.0% and 3.4%, and the transpiration rate (Tr) decreased by 47.9% and 59.6%, respectively (Table 1).
Figure 1. Effects of simulated acid rain on morphological and physiological indexes(A—height; B—biomass; C—chlorophyll a; D—chlorophyll b; E—chlorophyll.) of Alternanthera philoxeroides and Alternanthera sessilis. Different letters in the same species indicate significant differences among the different treatments (p < 0.05).
Table 1. Effects of simulated acid rain on plant gas exchange parameters of Alternanthera philoxeroides and Alternanthera sessilis.
The soil pH of A. philoxeroides decreased with the decrease in pH value of simulated acid rain (Table 2). The original soil pH value under CK was 6.93, while the soil pH value under the simulated acid rain treatment (pH 2.5) was 6.30, resulting in a decrease of 9.1%. The change trend of organic matter was the same as that of soil pH, reaching the lowest at pH 2.5, which was 7.6% lower than that of CK. The electrical conductivity showed an increasing trend (Table 2) and was 188.2% higher under the simulated acid rain treatment (pH 2.5) than under CK (p < 0.05). The total nitrogen and available phosphorus changed with the increase in pH value of simulated acid rain (Table 2), and were 2.5% and 21.0% higher, respectively, under the simulated acid rain treatment at pH 4.5, and 2.5% and 10.4% lower, respectively, under the simulated acid rain at pH 2.5 than under CK (p < 0.05). Total phosphorus, total potassium, and ammonium nitrogen in A. philoxeroides soil were not significantly affected by acid rain (Tables 2; p > 0.05).
Table 2. Effects of simulated acid rain on soil physicochemical properties of Alternanthera philoxeroides and Alternanthera sessilis.
In A. sessilis, the soil pH, organic matter, total phosphorus, total potassium, available phosphorus, and ammonium nitrogen showed a downward trend with a decrease in the pH of acid rain (Table 2). Under the simulated acid rain treatment at pH 2.5, these parameters were significantly lower than CK (p < 0.05), and the maximum decreases were 11.3%, 12.5%, 27.8%, 12.0%, 26.2%, and 29.7%, respectively. The electrical conductivity showed an increasing trend (Table 2) and was 108.2% higher under the simulated acid rain treatment at pH 2.5 than under CK (p < 0.05). There was no significant difference in soil total nitrogen between the acid rain treatments and CK (Tables 2; p > 0.05). Acid rain reduces the content of soil nutrients. Under the same treatment (pH 2.5 and pH 4.5), the decline in soil pH, OM, TP, TK, AN, and AP under A. philoxeroides was less than that of A. sessilis, and the influence of A. philoxeroides soil nutrients under acid rain was less than that of A. sessilis.
With the decrease in simulated acid rain pH, the bacterial flora richness index (Chao1 and ACE) and Shannon index of A. philoxeroides increased and were 6.8%, 8.8%, and 5.5% higher, respectively, under simulated acid rain at pH 2.5 than under CK (p < 0.05). The Simpson index showed a downward trend and was 30.2% lower under the simulated acid rain treatment at pH 2.5 than under CK (p < 0.05). The richness index of fungal flora changed with the increase in pH value of simulated acid rain, and was significantly higher under the simulated acid rain at pH 2.5 than under CK (18.0% and 17.0%; p < 0.05). The Shannon index showed an increasing trend, which was significantly higher than under the simulated acid rain at pH 2.5 than under CK (14.8%; p < 0.05). The Simpson index showed a downward trend and was 27.9% lower under the simulated acid rain at pH 2.5 than under CK (p < 0.05).
The bacterial flora richness index and diversity index (Simpson and Shannon) of A. sessilis were not significantly affected by acid rain (p > 0.05). The fungal flora richness index (Chao1 and ACE) and Shannon index changed with the increase in pH value of simulated acid rain. Under the simulated acid rain treatment at pH 2.5, they were significantly higher than those of CK by 14.5%, 12.4%, and 30.4%, respectively (p < 0.05). The Simpson index increased first and then decreased, and was significantly lower under simulated acid rain at pH 2.5 than under CK (37.3%; p < 0.05). Under acid rain stress, the change in the bacterial richness and diversity of A. philoxeroides was greater than that of A. sessilis. The change in fungal richness was greater than that of A. sessilis, and the change in diversity was less than that of A. sessilis (Table 3).
To intuitively reflect the differences in microbial communities’ composition and structure between A. philoxeroides and A. sessilis under different acid rain treatments, principal coordinate analysis was carried out using the Bray–Curtis distance algorithm (Figure 2). The cumulative contribution rate of the first two principal components (PC1 and PC2) was 43.72% (Figure 2A), indicating that these two principal components were the main contributors to the difference in bacterial ommunity composition between A. philoxeroides and A. sessilis under simulated acid rain. Under each treatment, the soil bacteria of A. philoxeroides were clustered in the positive semi-axis of PC2, and the soil bacteria of A. sessilis were clustered in the negative semi-axis of PC2. Furthermore, the soil bacteria of A. philoxeroides and A. sessilis were all in the negative semi-axis of PC1 under pH 2.5 treatment. The ANOSIM and ADONIS methods were used to analyze the similarity of soil fungal communities under different acid rain treatments. Simulated acid rain changed the bacterial community structures of A. philoxeroides and A. sessilis. The cumulative contribution rate of the first two principal components to the difference in the composition and structure of soil fungal communities between A. philoxeroides and A. sessilis was 45.92% (Figure 2B). Under the pH 2.5 treatment, the soil fungi of A. philoxeroides aggregated in the negative semi-axis of PC1, while the soil fungi of A. philoxeroides aggregated in the positive semi-axis of PC1 under the pH 4.5 treatment and CK. Under pH 2.5 treatment, the soil fungi of A. sessilis aggregated in the negative semi-axis of PC2, while the soil fungi of A. sessilis aggregated in the positive semi-axis of PC2 under the pH 4.5 treatment and CK. Correlation analysis showed that simulated acid rain changed the fungal community structure of A. philoxeroides and A. sessilis.
Figure 2. Effects of simulated acid rain on the community structure of soil bacteria (A) and fungi (B). AP1, AP2, and AP3 indicate that Alternanthera philoxeroides was treated with simulated acid rain at pH 2.5, 4.5, and 7.0, and AS1, AS2, and AS3 indicate that A. sessilis was treated with simulated acid rain at 2.5, 4.5, and 7.0.
Under simulated acid rain treatment, the dominant bacteria (relative abundance > 1%) in the soil shared by A. philoxeroides and A. sessilis were Proteobacteria, Actinobacteriota, Bacteroidota, Acidobacteriota, Firmicutes, Myxococcota, Chloroflexi, Patescibacteria, Gemmatimonadota, Verrucomicrobiota, and Armtimonadota (Figure 3A). Among them, the proportion of Proteobacteria, Actinobacteriota, Bacteroidota, Acidobacteriota, and Firmicutes was as high as 80%. Under CK, there was no significant difference in the relative abundance of bacterial communities between A. philoxeroides and A. sessilis in the five phyla (Figure 4A, P > 0.05). With the simulated acid rain treatment, the relative abundance of Bacteroidota in A. sessilis soil was significantly higher than that in the other treatments only under the treatment at pH 4.5 (p < 0.05). Therefore, different degrees of simulated acid rain had little effect on the composition of bacterial communities at the soil phyla level in A. philoxeroides and A. sessilis.
Figure 3. Effects of simulated acid rain on the composition and relative abundance of soil bacterial (A) and fungal (B) communities.
Figure 4. Difference analysis of dominant bacteria groups at the level of soil bacterial (A) and fungal (B) communities under simulated acid rain. *p < 0.05, **p < 0.01, ***p < 0.005.
The dominant fungi in the A. philoxeroides and A. sessilis soil were Ascomycota, Basidiomycota, Rozellomycota, and Olpidiomycota (Figure 3B). There were significant differences in the relative abundance of the dominant fungal flora of A. philoxeroides and A. sessilis under different simulated acid rain treatments (Figure 4B). With the simulated acid rain treatment, the relative abundance of Ascomycota in both plants increased first and then decreased. Under the pH 2.5 treatment, the relative abundance of Ascomycota in A. philoxeroides was significantly higher than that in A. sessilis (12.3%, p < 0.05), and the relative abundance of Basidiomycota was significantly inhibited by acid rain (p < 0.05). The relative abundance of Basidiomycota in A. philoxeroides decreased by 23.8%–49.0%, and that in A. sessilis decreased by 63.6%–83.3%. The relative abundance of Rozellomycota in A. philoxeroides under the pH 2.5 treatment was 1.8 times higher than that of CK. The relative abundance of Rozellomycota in A. sessilis under acid rain treatment (pH2.5 and pH4.5) was 20.4 times and 5.4 times higher, respectively, than that of CK.
Through the functional prediction of the soil microbial communities of A. philoxeroides and A. sessilis under simulated acid rain treatment, the functional prediction of the soil bacteria of A. philoxeroides and A. sessilis under acid rain treatment was relatively similar (Figure 5A). Those with relatively large functional abundance were as follows: S (Function unknown), E (Amino acid transport and metabolism), R (General function prediction only), K (Transcription), C (Energy production and conversion), M (Cell wall/membrane/envelope biogenesis), T (Signal transduction mechanisms), G (Carbohydrate transport and metabolism), and P (Inorganic ion transport and metabolism). Acid rain did not change the function of the soil bacteria of A. philoxeroides and A. sessilis.
Figure 5. Function prediction of simulated acid rain on soil bacteria (A) and fungi (B) of Alternanthera philoxeroides and Alternanthera sessilis.
The soil fungal function of A. philoxeroides and A. sessilis changed under acid rain treatment (Figure 5B). With the decrease in pH of simulated acid rain, the abundance of undefined saprotrophs and plant pathogens of A. philoxeroides increased first and then decreased, but both were higher than that in CK, with maximum increases of 10.0% and 20.7%, respectively. The abundance of plant saprotroph–wood saprotroph and ericoid mycorrhizal decreased, with maximum decreases of 59.4% and 39.5%, respectively. The abundance of undefined saprotrophs and plant pathogens of A. sessilis increased first and then decreased, with decreases of 10.6% and 36.6%, respectively. The relative abundance of plant pathogens of A. sessilis was highest under the simulated acid rain treatment at pH 4.5. Plant saprotroph–wood saprotroph decreased first and then increased, with a maximum decrease of 84.5%. Ericoid mycorrhizal decreased first and then increased, with an increase of 113.8% and a decrease of 14.9%.
Correlation analysis between environmental factors and microbial diversity showed that (Table 4) bacterial Chao1 and ACE indexes responded significantly to soil total nitrogen, total potassium, and available phosphorus (p < 0.05), and the bacterial Shannon index responded significantly to total potassium (p < 0.05). The fungal Chao1 index responded significantly to electrical conductivity, total nitrogen, total potassium, organic matter, and available phosphorus (p < 0.05). The fungal ACE index responded significantly to total nitrogen, total potassium, organic matter, and available phosphorus (p < 0.05). The fungal Shannon index responded significantly to pH, electrical conductivity, total nitrogen, total phosphorus, total potassium, organic matter, and available phosphorus (p < 0.05). The Simpson index responded significantly to pH, electrical conductivity, total nitrogen, total potassium, organic matter, and available phosphorus (p < 0.05).
Bacterial RDA analysis showed that the cumulative explained variation of the first axis and the second axis was 31.74% (Figure 6A), and EC and TP were the main factors that changed bacterial communities. Correlation analysis showed that (Table 5) soil EC was significantly positively correlated with Actinobacteriota (p < 0.05), and TP was significantly positively correlated with Acidobacteriota (p < 0.05). Fungal RDA showed that the cumulative explanatory variation of the first axis and the second axis was 53.8% (Figure 6B), in which AP, OM, EC, and pH were the main factors that changed fungal communities. Correlation analysis showed that AP was significantly positively correlated with Ascomycota (p < 0.05) and significantly negatively correlated with Rozellomycota, Olpidiomycota, and Chytridiomycota (p < 0.05). OM was significantly negatively correlated with Rozellomycota and Olpidiomycota (p < 0.05), and EC showed a significant positive correlation with Rozellomycota and Olpidiomycota (p < 0.05). There was a significant negative correlation between pH and Rozellomycota and Olpidiomycota (p < 0.05).
Figure 6. Redundancy analysis of the response of soil bacterial (A) and fungal (B) communities to environmental factors.
There are differences in the response of plants to acid rain at different pH values (Wang and Zhou, 2010; Yi et al., 2014). When the pH value is reduced to the tolerance range of plants, acid rain damages plant and inhibits their growth (Ju et al., 2017), which is consistent with the results of our study, showing that the plant height and total biomass of A. philoxeroides and A. sessilis decreased with the decrease in the pH of acid rain. Under the same simulated acid rain concentration, the damage to A. philoxeroides was less than that of A. sessilis, indicating that the invasive plant A. philoxeroides had stronger acid rain tolerance than the native plant A. sessilis. The chlorophyll content is greatly affected by acid rain, and the stability of chlorophyll b is stronger when it is damaged by acid rain (Ma et al., 2021), which is consistent with the results of our study. Acid rain inhibited the chlorophyll content of A. philoxeroides and A. sessilis. The decrease in chlorophyll content is an important index for judging leaf senescence. The chlorophyll content of A. philoxeroides under the pH 2.5 treatment was significantly lower than that of CK, indicating that leaf senescence accelerated. However, the leaves of A. sessilis had begun senescence under the pH 4.5 treatment, which may be due to the morphological structure, cell pH value, chloroplast membrane, or stomatal stability of A. philoxeroides leaves (Hu et al., 2021). Acid rain caused less damage to the chlorophyll of A. philoxeroides, thus reducing the degradation of chlorophyll by acid rain. In contrast, chlorophyll b has the function of absorbing and converting light energy, and A. philoxeroides can maintain the stability of the photosynthetic system to resist adversity damage by regulating chlorophyll b content (Rudiger, 2002). Photosynthesis is the basis of plant production, and the response to acid rain stress is very sensitive (Zhang et al., 2021). Some studies have found that acid rain can inhibit photosynthesis in plants, and the degree of inhibition increases with a decrease in pH (Qiu et al., 2002), which is consistent with the results of our study. The Pn, Gs, and Tr of A. philoxeroides and A. sessilis decreased with the decrease in pH value. The Pn of A. philoxeroides and A. sessilis decreased significantly under the pH 4.5 treatment, which was consistent with the decreasing trend of Ci. Therefore, the decrease in the photosynthetic rate was due to stomatal factors (Gao et al., 2021). Leaves of A. philoxeroides and A. sessilis adapt to acid rain stress by regulating stomata, reducing transpiration, and reducing the entry of acid rain. The invasive plant A. philoxeroides had a stronger adaptability to acid rain than the native plant A. sessilis.
A previous study indicated that plant diseases are associated with variations in the composition and structure of the rhizosphere microbial community (Wu et al., 2019, 2021). Rhizosphere microorganisms are the most active life forms in the soil (Heijden et al., 2008). Through diversity analysis of soil microbial community structures, acid rain increased the abundance and diversity of soil microbial communities in A. philoxeroides and A. sessilis. This is in contrast to previous studies that found that soil microbial diversity and richness indexes first increased and then decreased with the decrease in pH of acid rain (Wang et al., 2014), which may be the “fertilization effect” caused by short-term acid rain input, and the activation of effective nutrients in soil can resist the negative effects of acid rain and stimulate the reproduction of soil microorganisms (Liu et al., 2020). Soil microbial diversity is the result of the joint influence of many factors, and soil physicochemical properties are the key influencing factors (Kourtev et al., 2002). With the application of acid rain, the soil pH, organic matter, total nitrogen, total phosphorus, total potassium, ammonium nitrogen, and available phosphorus of A. philoxeroides and A. sessilis decreased, and these nutrients were significantly negatively correlated with Chao1, ACE, and Shannon indexes of soil bacteria and fungi and significantly positively correlated with the Simpson index, further indicating that acid rain stress led to the loss of soil nutrients to improve the microbial diversity of A. philoxeroides and A. sessilis. The higher the microbial diversity, the stronger the stability and stress resistance of the ecosystem (Zhou et al., 2002). In this study, the increase in microbial flora richness and diversity of A. philoxeroides under acid rain treatment was greater than that of A. sessilis, indicating that A. philoxeroides obtained higher soil microbial diversity and formed functional redundancy under acid rain stress. Functional redundancy ensures that the soil stability and resistance of A. philoxeroides are stronger than that of A. sessilis under acid rain stress (Chaer et al., 2009).
Different plants can form adaptive microbial communities in the surrounding soil, and the structure of soil microbial communities changes under acid rain stress (Wang et al., 2020). Consistent with the results of this study, there were significant differences in the fungal and bacterial community structures of A. philoxeroides and A. sessilis between CK and the simulated acid rain treatment at pH 2.5. High-throughput sequencing showed that the dominant bacterial groups of A. philoxeroides and A. sessilis under acid rain treatment were Proteobacteria, Actinobacteriota, Bacteroidota, and Acidobacteriota, and the dominant fungal groups were Ascomycota, Basidiomycota, which was consistent with a previous analysis of microbial communities in A. philoxeroides and A. sessilis (Yang et al., 2019). Acid rain did not significantly change the community structure of soil bacterial phyla between A. philoxeroides and A. sessilis, and the relative proportion of dominant fungal phyla was significantly different. This may be because the community structure and composition of soil fungi can quickly respond to environmental changes (Wan et al., 2015). Ascomycota tend to be more abundant in stress environments, and their relative abundance increases with the application of nitrogen fertilizer (He et al., 2016). This study also found a significant positive correlation between Ascomycota and total soil nitrogen. The content of soil total nitrogen of A. philoxeroides and A. sessilis increased first and then decreased with the decrease in the pH of acid rain, and the relative abundance of Ascomycota also changed accordingly. The decrease in organic matter in soil reduces the richness of available substrate abundance of Basidiomycota and inhibits their growth, which is consistent with the present study. Basidiomycota were positively correlated with organic matter. Ascomycota and Basidiomycota are mostly saprophytic bacteria that are important decomposers in soil (Ma et al., 2013). They can decompose refractory organic matter, such as lignin and keratin, in the soil and promote nutrient circulation and energy flow (Beimforde et al., 2014). Under acid rain stress, the decline in saprophytic microbial communities of A. philoxeroides was less than that of A. sessilis, which was consistent with the function of soil bacteria in A. philoxeroides and A. sessilis, as it was not affected by acid rain, while the decline of saprophytic vegetative fungi in the soil of A. philoxeroides was less than that of A. sessilis.
Previous studies have shown that the root exudates of plants alter the composition and diversity of the rhizosphere microbiome, mediating the abundance of specific pathogens and beneficial microbes in the rhizosphere soil and causing plant diseases (Wu et al., 2016a, 2017, 2019). In this study, fewer plant pathogens were observed in the rhizosphere soil of A. philoxeroides than in A. sessilis. This might be because invasive A. philoxeroides inhibits the growth of pathogens by some specific root exudate. The process and mechanism of this phenomenon are worth further study. The application of acid rain to reduce phosphorus content can lead to the transformation of soil bacterial microbial communities to fungal microbial communities (Lauber et al., 2008). In this study, TP was positively correlated with Acidobacteriota, negatively correlated with Rozellomycota, AP was positively correlated with Bacteroidota, and negatively correlated with Rozellomycota and Olpidiomycota. This is because bacteria have higher specific surface area requirements for phospholipids, and the decrease in soil phosphorus content inhibits bacterial growth (Nottingham et al., 2018). Although acid rain can reduce soil nutrient content, fungi have a strong adaptability to the environment and the ability to obtain nutrients under acidic conditions (DeForest and Scott, 2010).
In the current study, we provided evidence that simulated acid rain had a significant inhibitory effect on the morphological and physiological traits of invasive A. philoxeroides and native A. sessilis, reduced the soil nutrient content, and changed the microbial community structure of the two species. Soil microorganisms of A. philoxeroides maintained higher diversity by changing species composition, recruiting fewer plant pathogens, and showing better acid rain adaptability and resistance than A. sessilis. This study provides a new perspective for a better understanding of the plant invasion process.
The datasets presented in this study can be found in online repositories. The names of the repository/repositories and accession number(s) can be found at: NCBI—PRJNA863323.
YLiu and ZZ conceived the study and helped to revise the manuscript. MH, ZH, HC, and YLi collected the samples and analyzed the data. All authors contributed to the article and approved the submitted version.
This work was funded by the National Science Foundation of China (grant no. 31772235) and State Key Laboratory of Vegetation and Environmental Change (grant no. LVEC-2022kf01).
The authors declare that the research was conducted in the absence of any commercial or financial relationships that could be construed as a potential conflict of interest.
All claims expressed in this article are solely those of the authors and do not necessarily represent those of their affiliated organizations, or those of the publisher, the editors and the reviewers. Any product that may be evaluated in this article, or claim that may be made by its manufacturer, is not guaranteed or endorsed by the publisher.
Averill, C., and Classen, A. (2014). Divergence in plant and microbial allocation strategies explains continental patterns in microbial allocation and biogeochemical fluxes. Ecol. Lett. 17, 1202–1210. doi: 10.1111/ele.12324
Beimforde, C., Feldberg, K., Nylinder, S., Rikkinen, J., Tuovila, H., Drfelt, H., et al. (2014). Estimating the Phanerozoic history of the Ascomycota lineages: combining fossil and molecular data. Mol. Phylogenet. Evol. 78, 386–398. doi: 10.1016/j.ympev.2014.04.024
Chaer, G., Fernandes, M., Myrold, D., and Bottomley, P. (2009). Comparative resistance and resilience of soil microbial communities and enzyme activities in adjacent native forest and agricultural soils. Microb. Ecol. 58, 414–424. doi: 10.1007/s00248-009-9508-x
Chen, S., Zhou, Y., Chen, Y., and Jia, G. (2018). Fastp: an ultra-fast all-in-one FASTQ preprocessor. Bioinformatics 34, i884–i890. doi: 10.1093/bioinformatics/bty560
Chu, H. Y., Sun, H. B., Tripathi, B. M., Adams, J. M., Huang, R., Zhang, Y. J., et al. (2016). Bacterial community dissimilarity between the surface and subsurface soils equals horizontal differences over several kilometers in the western Tibetan plateau. Environ. Microbiol. 18, 1523–1533. doi: 10.1111/1462-2920.13236
DeForest, J. L., and Scott, L. G. (2010). Available organic soil phosphorus has an important influence on microbial community composition. Soil Sci. Soc. Am. J. 74, 2059–2066. doi: 10.2136/sssaj2009.0426
Dolatabadian, A., Sanavy, S. A. M. M., Gholamhoseini, M., Joghan, A. K., Majdi, M., and Kashkooli, A. B. (2013). The role of calcium in improving photosynthesis and related physiological and biochemical attributes of spring wheat subjected to simulated acid rain. Physiol. Mol. Biol. Plants 19, 189–198. doi: 10.1007/s12298-013-0165-7
Du, E. Z., Dong, D., Zeng, X. T., Sun, Z. Z., Jiang, X. F., and de Vries, W. (2017). Direct effect of acid rain on leaf chlorophyll content of terrestrial plants in China. Sci. Total Environ. 605-606, 764–769. doi: 10.1016/j.scitotenv.2017.06.044
Edgar, R. C. (2013). UPARSE: highly accurate OTU sequences from microbial amplicon reads. Nat. Methods 10, 996–998. doi: 10.1038/nmeth.2604
El-Tarabily, K. A., Nassar, A. H., and Sivasithamparam, K. (2007). Promotion of growth of bean (Phaseolus vulgaris L.) in a calcareous soil by a phosphate-solubilizing, rhizosphere-competent isolate of Micromonospora endolithica. Appl. Soil Ecol. 39, 161–171. doi: 10.1016/j.apsoil.2007.12.005
Falkowski, P. G., Fenchel, T., and Delong, E. F. (2008). The microbial engines that drive Earth's biogeochemical cycles. Science 320, 1034–1039. doi: 10.1126/science.1153213
Gao, Y. F., Rong, L. P., Zhao, D. H., Zhang, J. Q., and Chen, J. S. (2021). Effects of simulated acid rain on the photosynthetic physiology of Acer ginnala seedlings. Can. J. For. Res. 51, 18–24. doi: 10.1139/cjfr-2020-0091
Guo, W., Li, J. M., and Hu, Z. H. (2012). Effects of clonal integration on growth of Alternanthera philoxeroides under simulated acid rain and herbivory. Acta Ecol. Sin. 32, 151–158. doi: 10.5846/stxb201011031575
He, D., Xiang, X. J., He, J. S., Wang, C., Cao, G. M., Adams, J., et al. (2016). Composition of the soil fungal community is more sensitive to phosphorus than nitrogen addition in the alpine meadow on the Qinghai-Tibetan plateau. Biol. Fertil. Soils 52, 1059–1072. doi: 10.1007/s00374-016-1142-4
Heijden, M., BaRdgett, R. D., and Straalen, N. (2008). The unseen majority: soil microbes as drivers of plant diversity and productivity in terrestrial ecosystems. Ecol. Lett. 11, 296–310. doi: 10.1111/j.1461-0248.2007.01139.x
Hu, H., Hua, W., Shen, A. L., Zhou, H. K., Sheng, L., Lou, W. D., et al. (2021). Photosynthetic rate and chlorophyll fluorescence of barley exposed to simulated acid rain. Environ. Sci. Pollut. Res. 28, 42776–42786. doi: 10.1007/s11356-021-13807-8
Ju, S. M., Wang, L. P., Yin, N. N., Li, D., Wang, Y. K., and Zhang, C. Y. (2017). Silicon alleviates simulated acid rain stress of Oryza sativa L. seedlings by adjusting physiology activity and mineral nutrients. Protoplasma 254, 2071–2081. doi: 10.1007/s00709-017-1099-7
Kourtev, P. S., Ehrenfeld, J. G., and HaGgblom, M. (2002). Exotic plant species alter the microbial community structure and function in the soil. Ecology 83, 3152–3166. doi: 10.1890/0012-9658(2002)083[3152:EPSATM]2.0.CO;2
Lauber, C., Strickland, M. S., Bradford, M. A., and Fierer, N. (2008). The influence of soil properties on the structure of bacterial and fungal communities across land-use types. Soil Biol. Biochem. 40, 2407–2415. doi: 10.1016/j.soilbio.2008.05.021
Li, Y. B., Liang, S. W., Du, X. F., Kou, X. C., Lv, X. T., and Li, Q. (2020). Mowing did not mitigate the negative effects of nitrogen deposition on soil nematode community in a temperate steppe. Soil Ecol. Lett. 3, 1–9. doi: 10.1007/s42832-020-0048-0
Liu, M., Huang, X., Song, Y., Tang, J., and Zhu, T. (2019). Ammonia emission control in China would mitigate haze pollution and nitrogen deposition, but worsen acid rain. Proc. Natl. Acad. Sci. 116, 7760–7765. doi: 10.1073/pnas.1814880116
Liu, Z. Q., Li, D. F., Zhang, J. E., Saleem, M., Zhang, Y., Ma, R., et al. (2020). Effect of simulated acid rain on soil CO2, CH4 and N2O emissions and microbial communities in an agricultural soil. Geoderma 366:114222. doi: 10.1016/j.geoderma.2020.114222
Liu, X., Zhang, B., Zhao, W., Wang, L., Xie, D., Huo, W., et al. (2017). Comparative effects of sulfuric and nitric acid rain on litter decomposition and soil microbial community in subtropical plantation of Yangtze River delta region. Sci. Total Environ. 601-602, 669–678. doi: 10.1016/j.scitotenv.2017.05.151
Liu, X., Zhao, W. R., Meng, M. J., Fu, Z. Y., Xu, L. H., Zha, Y., et al. (2018). Comparative effects of simulated acid rain of different ratios of SO42− to NO3− on fine root in subtropical plantation of China. Sci. Total Environ. 618, 336–346. doi: 10.1016/j.scitotenv.2017.11.073
Lv, Y. N., Wang, C. Y., Jia, Y. Y., Wang, W. W., Ma, X., Du, J. J., et al. (2014). Effects of sulfuric, nitric, and mixed acid rain on litter decomposition, soil microbial biomass, and enzyme activities in subtropical forests of China. Appl. Soil Ecol. 79, 1–9. doi: 10.1016/j.apsoil.2013.12.002
Ma, S. L., Liu, X., Jia, Z. H., Meng, M. J., Li, C., Ren, Q., et al. (2021). Response of Quercus acutissima foliage to different types of simulated acid rain. Atmos. Pollut. Res. 12:101112. doi: 10.1016/j.apr.2021.101112
Ma, A. Z., Zhuang, X. L., Wu, J., Mei, C. M. M., Lv, D., Liu, C. Z., et al. (2013). Ascomycota members dominate fungal communities during straw residue decomposition in arable soil. PLoS One 8:e66146. doi: 10.1371/journal.pone.0066146
Mago, T., and Salzberg, S. L. (2011). FLASH: fast length adjustment of short reads to improve genome assemblies. Bioinformatics 27, 2957–2963. doi: 10.1093/bioinformatics/btr507
Meng, F. B., Yang, X. D., Duan, L. C., Naidu, R., Nuruzzaman, M., and Semple, K. T. (2019). Influence of pH, electrical conductivity and ageing on the extractability of benzo[a]pyrene in two contrasting soils. Sci. Total Environ. 690, 647–653. doi: 10.1016/j.scitotenv.2019.06.445
Mohsen, J., and Elaheh, N. (2012). The impact of acid rain on phosphorus leaching from a sandy loam calcareous soil of western Iran. Environ. Earth Sci. 66, 311–317. doi: 10.1007/s12665-011-1240-4
Nottingham, A. T., Hicks, L. C., Ccahuana, A. J. Q., Salinas, N., Baath, E., and Meir, P. (2018). Nutrient limitations to bacterial and fungal growth during cellulose decomposition in tropical forest soils. Biol. Fertil. Soils 54, 219–228. doi: 10.1007/s00374-017-1247-4
Pan, X. Y., Geng, Y. P., Zhang, W. J., Li, B., and Chen, J. K. (2006). Cover shift and morphological plasticity of invasive Alternanthera philoxeroides along ariparian zone in South China. J. Plant Ecol. 30, 835–843. doi: 10.17521/cjpe.2006.0106
Qiu, D. L., Liu, X. H., and Guo, S. Z. (2002). Effects of simulated acid rain and chlorophyll a fluorescence parameters in leaves of longan. Acta Phytoecol. Sin. 26, 441–446. doi: 10.1006/jfls.2001.0409
Ramlall, C., Varghese, B., Ramdhani, S., Pammenter, N. W., Bhatt, A., Berjak, P., et al. (2015). Effects of simulated acid rain on germination, seedling growth and oxidative metabolism of recalcitrant-seeded Trichilia dregeana grown in its natural seed bank. Physiol. Plant. 153, 149–160. doi: 10.1111/ppl.12230
Ren, J. H., Liu, X. L., Yang, W. P., Yang, X. X., Li, W. G., Xia, Q., et al. (2021). Rhizosphere soil properties, microbial community, and enzyme activities: short-term responses to partial substitution of chemical fertilizer with organic manure. J. Environ. Manage. 299:113650. doi: 10.1016/j.jenvman.2021.113650
Rudiger, W. (2002). Biosynthesis of chlorophyll b and the chlorophyll cycle. Photosynth. Res. 74, 187–193. doi: 10.1023/A:1020959610952
Shafer, S. R. (1992). Responses of microbial populations in the rhizosphere to deposition of simulated acidic rain onto foliage and/or soil. Environ. Pollut. 76, 267–278. doi: 10.1016/0269-7491(92)90146-2
Shu, X., Zhang, K. R., Zhang, Q. F., and Wang, W. B. (2019). Ecophysiological responses of Jatropha curcas L. seedlings to simulated acid rain under different soil types. Ecotoxicol. Environ. Saf. 185:109705. doi: 10.1016/j.ecoenv.2019.109705
Shu, X., Zhang, K. R., Zhang, Q. F., and Wang, W. B. (2021). Tolerant mechanism of Jatropha curcas L. roots to acid rain in soils with different acid-buffering capacities. Acta Physiol. Plant. 43, 1–11. doi: 10.1007/s11738-021-03329-8
Stakenrandt, E., and Goebel, B. (1994). Taxonomic note: a place for DNA-DNA reassociation and 16S rRNA sequence analysis in the present species definition in bacteriology. Int. J. Syst. Bacteriol. 44, 846–849.
Sun, Y., Ding, J. Q., and Frye, M. (2010). Effects of resource availability on tolerance of herbivory in the invasive Alternanthera philoxeroides and the native Alternanthera sessilis. Weed Res. 50, 527–536. doi: 10.1111/j.1365-3180.2010.00822.x
Wan, X. H., Huang, Z. Q., He, Z. M., Yu, Z. P., Wang, M. H., Davis, M. R., et al. (2015). Soil C:N ratio is the major determinant of soil microbial community structure in subtropical coniferous and broadleaf forest plantations. Plant and Soil 387, 103–116. doi: 10.1007/s11104-014-2277-4
Wang, L., Chen, Z., Shang, H., Wang, J., and Zhang, P. Y. (2014). Impact of simulated acid rain on soil microbial community function in Masson pine seedlings. Electron. J. Biotechnol. 17, 199–203. doi: 10.1016/j.ejbt.2014.07.008
Wang, L. C., Liu, Y., Zhu, X. M., Zhang, Z., and Huang, X. Q. (2021a). Identify potential allelochemicals from Humulus scandens (Lour.) Merr. Root extracts that induce allelopathy on Alternanthera philoxeroides (Mart.) Griseb. Sci. Rep. 11, 7068–7076. doi: 10.1038/s41598-021-86656-7
Wang, N., Pan, X. C., Bai, S. B., and Zhnag, T. (2020). Effects of acid rain on root morphology and distribution pattern in the buffer zone of broad-leaved forest invaded by Moso bamboo. Acta Ecol. Sin. 40, 4670–4678. doi: 10.5846/stxb201909031826
Wang, Y., Xiong, Y. T., Wang, Y., and Li, Q. J. (2021b). Long period exposure to serious cadmium pollution benefits an invasive plant (Alternanthera philoxeroides) competing with its native congener (Alternanthera sessilis). Sci. Total Environ. 786:147456. doi: 10.1016/j.scitotenv.2021.147456
Wang, K., Yang, J., and Chen, J. K. (2009). The applications of congeneric comparisons in plant invasion ecology. Biodivers. Sci. 17, 353–361. doi: 10.3724/SP.J.1003.2009.09055
Wang, L. H., and Zhou, Q. (2010). Responses of rice seed germination to acid rain stress. Seed Sci. Technol. 38, 26–35. doi: 10.15258/sst.2010.38.1.03
Wei, H., Liu, W., Zhang, J. E., and Qin, Z. (2017). Effects of simulated acid rain on soil fauna community composition and their ecological niches. Environ. Pollut. 220, 460–468. doi: 10.1016/j.envpol.2016.09.088
Wei, H., Ma, R., Zhang, J. E., Zhou, L. Y., Liu, Z. Q., Fan, Z. Y., et al. (2020). Quality dependence of litter decomposition and its carbon, nitrogen and phosphorus release under simulated acid rain treatments. Environ. Sci. Pollut. Res. 27, 19858–19868. doi: 10.1007/s11356-020-08423-x
Whitaker, J., Ostle, N., Nottingham, A. T., Ccahuana, A., Salinas, N., Bardgett, R. D., et al. (2014). Microbial community composition explains soil respiration responses to changing carbon inputs along an Andes-to-Amazon elevation gradient. J. Ecol. 102, 1058–1071. doi: 10.1111/1365-2745.12247
Wu, J. P., Liang, G. H., Hui, D. F., Deng, Q., Xiong, X., Qiu, Q. Y., et al. (2016b). Prolonged acid rain facilitates soil organic carbon accumulation in a mature forest in southern China. Sci. Total Environ. 544, 94–102. doi: 10.1016/j.scitotenv.2015.11.025
Wu, G., Ling, J., Xu, Y. P., Zhao, D. Q., Liu, Z. X., Wen, Y., et al. (2022). Effects of soil warming and straw return on soil organic matter and greenhouse gas fluxes in winter wheat seasons in the North China plain. J. Clean. Prod. 356:131810. doi: 10.1016/j.jclepro.2022.131810
Wu, H. M., Qin, X. J., Wang, J. Y., Wu, L. K., Chen, J., Fan, J. K., et al. (2019). Rhizosphere responses to environmental conditions in Radix pseudostellariae under continuous monoculture regimes. Agric. Ecosyst. Environ. 270, 19–31. doi: 10.1016/j.agee.2018.10.014
Wu, H. M., Wu, H. M., Qin, X. J., Lin, M. H., Zhao, Y. L., Rensing, C., et al. (2021). Replanting disease alters the faunal community composition and diversity in the rhizosphere soil of radix pseudostellariae. Agric. Ecosyst. Environ. 310:107304. doi: 10.1016/j.agee.2021.107304
Wu, H. M., Wu, L. K., Wang, J. Y., Zhu, Q., Sheng, L., Xu, J. H., et al. (2016a). Mixed phenolic acids mediated proliferation of pathogens Talaromyces helicus and Kosakonia sacchari in continuously monocultured radix pseudostellariae rhizosphere soil. Front. Microbiol. 7:335. doi: 10.3389/fmicb.2016.00335
Wu, H. M., Wu, L. K., Zhu, Q., Wang, J. Y., Qin, X. J., Xu, J. H., et al. (2017). The role of organic acids on microbial deterioration in the radix pseudostellariae rhizosphere under continuous monoculture regimes. Sci. Rep. 7:3497. doi: 10.1038/s41598-017-03793-8
Xu, H. Q., Zhang, J. E., OuYang, Y., Lin, L., Quan, G. M., Zhao, B. L., et al. (2015). Effects of simulated acid rain on microbial characteristics in a lateritic red soil. Environ. Sci. Pollut. Res. 22, 18260–18266. doi: 10.1007/s11356-015-5066-6
Yang, B. F., Zhang, X., Zagorchev, L., Li, J. M., Frey, B., and Li, M. H. (2019). Parasitism changes rhizospheric soil microbial communities of invasive Alternanthera philoxeroides, benefitting the growth of neighboring plants. Appl. Soil Ecol. 143, 1–9. doi: 10.1016/j.apsoil.2019.05.025
Yi, L. T., Liu, M. H., Yu, S. Q., Yu, F., and Yin, X. M. (2014). Effects of simulated acid rain stress on chlorophyll fluorescence characteristics and growth in leaves of Lithocarpus glaber and Schima superba seedlings. Asian J. Chem. 26, 4619–4622. doi: 10.14233/ajchem.2014.16141
Zhang, Y. Y., Tian, C., Yu, T., Dayananda, B., Fu, B., Senaratne, S. L., et al. (2021). Differential effects of acid rain on photosynthetic performance and pigment composition of the critically endangered Acer amplum subsp. catalpifolium. Glob. Ecol. Conserv. 30:e01773. doi: 10.1016/j.gecco.2021.e01773
Zhang, C. Y., Yi, X. Q., Gao, X. Z., Wang, M. H., Shao, C. Y., Lv, Z. D., et al. (2020). Physiological and biochemical responses of tea seedlings (Camellia sinensis) to simulated acid rain conditions. Ecotoxicol. Environ. Saf. 192:110315. doi: 10.1016/j.ecoenv.2020.110315
Zheng, Y. F., Wang, Y. Q., Zheng, Y. L., and Li, Y. F. (2022). Effects of simulated acid rain on soil enzyme activity and related chemical indexes in woodlands. Forests 13:860. doi: 10.3390/f13060860
Keywords: simulated acid rain, invasive species, native species, rhizosphere microorganisms, microbial communities
Citation: He M, Hua Z, Chen H, Liu Y, Li Y and Zhang Z (2022) Effects of simulated acid rain on rhizosphere microorganisms of invasive Alternanthera philoxeroides and native Alternanthera sessilis. Front. Microbiol. 13:993147. doi: 10.3389/fmicb.2022.993147
Received: 13 July 2022; Accepted: 15 August 2022;
Published: 09 September 2022.
Edited by:
Changxun Fang, Fujian Agriculture and Forestry University, ChinaReviewed by:
Fengjuan Zhang, Hebei University, ChinaCopyright © 2022 He, Hua, Chen, Liu, Li and Zhang. This is an open-access article distributed under the terms of the Creative Commons Attribution License (CC BY). The use, distribution or reproduction in other forums is permitted, provided the original author(s) and the copyright owner(s) are credited and that the original publication in this journal is cited, in accordance with accepted academic practice. No use, distribution or reproduction is permitted which does not comply with these terms.
*Correspondence: Zhen Zhang, eGp6aGFuZ3poZW5AMTYzLmNvbQ==
Disclaimer: All claims expressed in this article are solely those of the authors and do not necessarily represent those of their affiliated organizations, or those of the publisher, the editors and the reviewers. Any product that may be evaluated in this article or claim that may be made by its manufacturer is not guaranteed or endorsed by the publisher.
Research integrity at Frontiers
Learn more about the work of our research integrity team to safeguard the quality of each article we publish.