- 1College of Life Science, Northwest Normal University, Lanzhou, China
- 2Bioactive Products Engineering Research Center for Gansu Distinctive Plants, Northwest Normal University, Lanzhou, China
- 3New Rural Development Research Institute, Northwest Normal University, Lanzhou, China
Volatile organic compounds (VOCs) produced by antagonistic microorganisms have good biocontrol prospects against postharvest diseases. Infection caused by Alternaria iridiaustralis and 10 other significant fungal diseases can be successfully inhibited by VOCs produced by an identified and screened endophytic strain L1 (Bacillus velezensis). This study revealed the in vivo and in vitro biocontrol effects of VOCs released by B. velezensis L1 on A. iridiaustralis, a pathogenic fungus responsible for rot of wolfberry fruit. The inhibition rates of VOCs of B. velezensis L1 on the mycelial growth of A. iridiaustralis in vitro were 92.86 and 90.30%, respectively, when the initial inoculum concentration on the plate was 1 × 109 colony forming unit (CFU)/ml. Spore germination and sporulation were 66.89 and 87.96%, respectively. VOCs considerably decreased the wolfberry’s disease index and decay incidence in vivo. Scanning electron microscopy revealed that the morphological and structural characteristics of A. iridiaustralis could be altered by VOCs. Ten VOCs were identified through headspace-gas chromatography-ion mobility spectrometry. Pure chemical tests revealed that 2.3-butanedione had the strongest antifungal effects, totally inhibiting A. iridiaustralis in wolfberry fruit at a 60 μl/L concentration. The theory underpinning the potential application of VOCs from B. velezensis is provided herein. This is also the first study to document the antifungal capabilities of the B. velezensis strain on postharvest wolfberry fruit.
Introduction
Wolfberry (Lycium barbarum L) is gradually becoming one of the most popular functional fruits worldwide as it is rich in nutrients and phytochemicals (Wenli et al., 2021). Wolfberry has anticancer, antioxidant, antiaging, blood sugar control, and immune-enhancing properties. Therefore, this fruit is widely used in food and medicine fields (Zhou et al., 2017; Yajun et al., 2019). However, wolfberry fruit is highly perishable because of its susceptibility to various postharvest pathogenic diseases. Fungal diseases are the main cause of postharvest losses of wolfberry fruits. Particularly, Alternaria sp. are the most common postharvest pathogenic fungi of wolfberry fruits and can significantly reduce the fruit value and shelf life, causing severe economic losses (Yuan et al., 2012; Wei et al., 2017).
Regarding postharvest diseases of fruits, traditional physical methods can delay senescence but cannot completely prevent rot, which ultimately reduces fruit quality (Alijani et al., 2019). On the other hand, chemical fungicides have a substantial inhibitory effect on pathogenic fungi, but their long-term and large-scale use leads to deposition of residues in the environment and resistance of pathogenic fungi (Mari et al., 2016). Therefore, finding new, safe, efficient, and environmentally friendly methods for controlling these diseases is of great significance.
Increasing number of researchers have recently focused on biological control agents (BCAs; Do Kim et al., 2020). According to research, many bacteria can produce volatile organic compounds (VOCs) with antifungal function, which have shown strong inhibitory effects on fungal diseases (Di Francesco et al., 2020a). Gotor-Vila et al. (2017) reported that VOCs produced by Bacillus amyloliquefaciens CPA-8 significantly inhibited the mycelial growth of postharvest pathogens and reduced the rot of cherry fruits. Arrarte et al. (2017) revealed that VOCs released by Candida sake can control decay of apples caused by postharvest pathogens. Similarly, Oro et al. (2018) found that VOCs from Wickerhamomyces anomalus, Metschnikowia pulcherrima, and Saccharomyces cerevisiae can inhibit pathogenic fungi that cause rot in strawberries and control postharvest diseases. In this way, VOCs released by BCAs provide a new solution for the prevention and treatment of postharvest diseases.
VOCs have the characteristics of strong permeability and high diffusion efficiency, and are easy degradation, can greatly reduce residues. In a relatively closed environment, a full range of biological fumigation can be performed on items to achieve better results (Qin et al., 2017; Toffano et al., 2017). Therefore, compared with chemical fungicides, the mode of action of VOCs produced by BCAs is safer and more efficient. B. velezensis has been widely studied because of its highly effective inhibitory effect on pathogenic microorganisms. Li et al. (2020) reported that VOCs from B. velezensis CT32 could inhibit strawberry vascular wilt pathogens Verticillium dahliae and Fusarium oxysporum. Gao et al. (2017) indicated that VOCs emitted by B. velezensis ZSY-1 could inhibit A. solani and Botrytis cinerea. Calvo et al. (2020) showed that VOCs produced by B. velezensis strains BUZ-14, I3 and I5 could inhibit postharvest fungal pathogens of fruits, thereby reducing the disease severity in grapes and apricots. B. velezensis is considered a promising BCA against postharvest fungal diseases.
Currently, research on postharvest preservation of wolfberry fresh fruit fruits is lacking. Therefore, this study evaluated the in vivo and in vitro effects of VOCs produced by B. velezensis L1 and individual pure substance against postharvest pathogenic fungi A. iridiaustralis in wolfberry fruit.
Materials and methods
Fruit and plants
The wolfberry fruit was purchased from Ningxia, Gansu. Fully ripe fruits of the same size and without any mechanical damage were sterilized with 1% sodium hypochlorite, rinsed three times with sterile distilled water (SDW), and air-dried naturally on an ultra-clean bench.
Lonicera maackii (Rupr.) Maxim is from Lanzhou Botanical Garden, Gansu, China. Picked stems and leaves were stored in airtight bags and kept at 4°C for later use.
Culture media and microorganisms
Tianqi Gene Biotechnology Co., Ltd. provided Luria–Bertani medium (LB) and potato dextrose agar medium (PDA; Lanzhuo, China). Agar was purchased from Niuniu Biochemical Co., Ltd. (Lanzhuo, China).
Wolfberry fungal pathogen A. iridiaustralis LB7 (MN 944921) was supplied by the College of Life Sciences, Northwest Normal University, China’s Plant-Microbe Interactions Research Lab. This pathogen has been demonstrated to significantly worsen postharvest wolfberry deterioration in our previous study (Ling et al., 2021). A. iridiaustralis was inoculated on PDA at 28°C for 7 days, the fungal spores were resuspended in SDW, and the concentration of the spore suspension was adjusted to 1 × 105 spores/mL with a hemocytometer.
Pathogenic fungi Phytophthora capsici (ACCC 37132), Colletotrichum capsici (ACCC 36946), F. oxysporum (ACCC 39326), B. cinerea (ACCC 37347), Rhizoctonia solani (ACCC 38870), F. graminearum (ACCC 39334) were obtained from Agricultural Culture Collection of China, Beijing. F. annulatum (MT 434004), Talaromyces tumuli (MT 434003), Colletotrichum fioriniae (MN 944922), and F. arcuatisporum (MN 944920) were isolated from disease plants and all pathogenic strains were stored in the laboratory (Ling et al., 2021).
Isolation, screening and identification of a highly antifungal bacterial endophyte
Using our previous technique, we isolated and characterized endophytic bacteria from L. maackii (Rupr.) Maxim (Ling et al., 2019). Briefly, the fresh stems and leaves stored in the refrigerator were removed, rinsed under running water, air-dried naturally, sterilized, and rinsed three times with sterile water. Next, the stems and leaves were cut using a sterile scalpel and cultured in solid LB medium plates at 37°C. Each colony was transferred to new medium until a pure culture of the strain is obtained. Using the two sealed base plate approach, the strain with the greatest capacity to suppress the pathogenic fungus was selected for further investigation. In general, 80 μl of bacterial suspension was evenly distributed on LB solid medium. Subsequently, a 6 mm-diameter fungal disk was cut and placed in the center of a sterile PDA petri dish. The two plates were sealed to prevent the loss of VOCs. The bacteriostatic ability of endophytic bacteria against pathogenic fungi was evaluated on the basis of the diameter of the fungus.
The bacterial DNA template obtained by screening was extracted using of the bacterial genome extraction kit (Huada Gene Co., Ltd., Wuhan, China). The 16S rDNA fragment was amplified through PCR by using universal primers 27F (5′AGAGTTTGATCCTGGCTCAG3′) and 1429R (5′GGTTACCTTGTTACGACTT3′). The cycle parameters were as follows: predenaturation at 96°C for 5 min, followed by 30 cycles of denaturation at 96°C for 30 s, annealing at 62°C for 30 s, and a final extension at 72°C for 30 s. The extension was performed at 72°C for 10 min at the end of the cycle. PCR products were detected through 1% agarose gel electrophoresis and sequenced. The sequencing results of 16S rDNA were input into the nucleic acid database alignment system of the National Center for Biological Information (NCBI) website, and nucleic acid sequence alignment analysis was performed using the Blast program. A Phylogenetic tree was constructed using the neighbor-joining method with MEGA-X software to analyze the phylogenetic relationship.
In vitro inhibitory activity of VOCs from Bacillus Velezensis L1
Inhibitory activity of VOCs with different inoculation concentrations of Bacillus Velezensis L1
A dual culture method was used to evaluate the inhibitory effect of antagonistic bacteria produced VOCs on A. iridiaustralis mycelial growth and conidial germination (Ye et al., 2020; Di Francesco et al., 2020b). SDW was used to inoculate the control plates with the same amounts of bacteria as the test plates, which was 80 μl of bacterial suspension at a concentration of 106–109 colony forming units (CFU)/ml. The plate cover was then changed out by a PDA plate that had been injected with a 6 mm-diameter disk or 20 μl of A. iridiaustralis spore suspension. Both plates were immediately sealed with parafilm, and cultured for 7 days at 28°C. Each experiment was conducted three times.
Effect of VOCs from Bacillus velezensis L1 on the spore germination and sporulation capacity of Alternaria iridiaustralis
With a few minor adjustments, the preceding approach was used to measure spore germination and sporulation capacity (Bu et al., 2021; Wang et al., 2021b). LB plates were inoculated with strain L1 and PDA plates were covered with a fungal spore suspension (1 × 105 spores/mL), as indicated earlier. After 12 h incubation at 28°C, spore germination could be visualized under a light microscope. The sporulation capacity experiment was conducted according to instructions provided in Section 2.4.1. In general, a 6 mm fungal plug was removed from the cultivated PDA medium and vortexed in SDW. A hemocytometer was used to count the spores.
Effect of VOC prefumigation on mycelium growth
The LB plate coated with the 80 μl bacterial suspension and the PDA plate containing a 6 mm-diameter disk were snap-sealed. After 4 or 5 days of fumigation in the incubator, 6 mm-diameter disks were cut from the PDA plate by using a punching bear, transferred to a new PDA plate, and incubated at 28°C for 7 days. Then, mycelial growth of pathogenic fungi were measured.
Scanning electron microscopy analysis
The effect of VOCs produced by B. velezensis L1 on pathogenic fungi was observed using scanning electron microscopy (SEM). SEM analysis was performed as described previously (Wonglom et al., 2020).
In vivo biocontrol of Alternaria iridiaustralis on wolfberry fruit by VOCs from Bacillus velezensis L1
Inhibitory effects of VOCs on Alternaria iridiaustralis in vivo
The biocontrol effects of different initial amounts of VOCs in vivo were investigated using different numbers of plates. LB plates were treated with 80 μl bacterial suspension (1 × 108 CFU/ml), and these uncovered plates were then placed at the bottom of sterile glass containers in numbers of 2, 4, 6, and 8, respectively. Then, 5 μl of A. iridiaustralis spore suspension was inoculated into wolfberry fruits. After inoculation, the fruits were placed in sterile petri dishes and placed on top of glass containers. The container was sealed and incubated at room temperature for 7 days. The decay incidence and disease index of the wolfberry fruits were measured using previous methods (Xu et al., 2016).
Effect of VOCs on wolfberry fruit postharvest natural decay
Untreated wolfberry fruits were treated with different numbers of LB plates, and after fumigation for 7 days at room temperature, the decay incidence and disease index were measured.
Identification of VOCs
The method used for identifying VOC components of B. velezensis L1 is consistent with that described in our previous study (Ling et al., 2021). Then, 10 μl of the bacterial suspension of strain L1 was inoculated into a 20-mL headspace vial containing 5 ml of solid LB medium. The solid LB medium without the strain was used as a blank control, and each sample was repeated three times. After incubating the vials for 3 days in a 37°C incubator, the headspace-gas chromatography-ion mobility spectrometry (HS-GC-IMS) assay was performed. VOCs were collected and sent to the G.A.S. Department of Shandong HaiNeng Science Instrument Co., Ltd. (Shandong, China) for measurement using the HS-GC-IMS instrument (FlavourSpec®). The sample was incubated at 40°C for 15 min, and VOCs from the 500 μL headspace were injected into the testing instrument using a heated syringe (85°C). GC equipped with the MXT-5 column (15 m, ID: 0.53 mm df: 1 μm) was used for chromatographic separation at 60°C. Pure nitrogen (99.999% purity) was used as the carrier gas. The flow of drift gas (nitrogen) of the IMS was set to 150 ml/min, and a 9.8 cm drift tube was operated at a constant voltage at 45°C. The program was as follows: 2 ml/min for 2 min, 10 ml/min for 10 min, and 100 ml/min for 20 min. Data were acquired and processed using instrumental analysis software such as VOCal and Reporter, Gallery plot, Dynamic PCA, and GC × IMS Library. The GC-IMS Library Search software uses the National Institute of Standards and Technology database and IMS database for qualitative analysis of the components.
In vitro responses of Alternaria iridiaustralis to a single component of VOC
The pathogenic fungus A. iridiaustralis was grown on PDA medium at 28°C for 7 days. Using a punching bear, a 6 mm-diameter disks were removed from the PDA plate’s edge and placed one by one in the center of each fresh petri plate. A sterile filter paper (diameter: 6 mm) was placed in the center of the petri dish lid. Then, equal volumes of each individual pure VOC (Table 1) were added to increase the air concentration from 20 μl/L to 100 μl/L. An identical volume of SDW was used for the control. A Vernier caliper was used to measure the plug diameter before the petri dishes were sealed and grown for 4 days at 28°C.
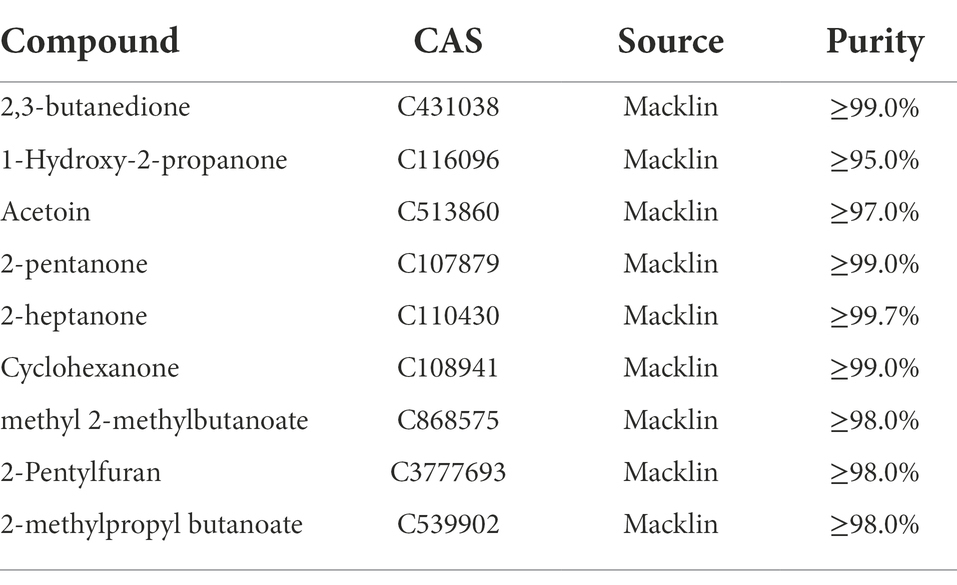
Table 1. Pure components comprising the VOCs of Bacillus velezensis L1, these substances were purchased for further experiments.
In vivo control of Alternaria iridiaustralis on wolfberry fruit by pure synthetic components of VOCs
A single VOC component was chosen for additional testing in vivo based on the outcomes of in vitro testing. Fruits were infected and placed in a sterile petri plate. Then, the decay incidence and disease index of wolfberry fruit was determined with various quantities of pure components.
Broad antagonistic activity of strain L1
We determined whether strain L1 has broad-spectrum antifungal activity against other significant fungal infections. Twelve pathogens were used in our test, namely P. capsici, C. capsici, F. oxysporum, B. cinerea, R. solani, F. graminearum, F. annulatum, T. tumuli, C. fioriniae and F. arcuatisporum. The following formula was used to compute the percentage of mycelial growth inhibition:
Inhibition rate (%) = [(dc − dt)/dc] × 100
Where the terms dc (cm) represent the average colony diameters of the control and treatment groups, respectively.
Statistical analysis
SPSS 20.0 software was used to perform statistical analyses. Followed by the Duncan’s test, p < 0.05 was set to indicate a statistically significant difference. Drawn with the Origin 9.0 software, by measuring three independent replicates, all data were reported as the mean ± standard error.
Results
Identification of strain L1 using morphological and molecular markers
An endophyte (strain L1) was obtained from L. maackii (Rupr.) Maxim (Figure 1A). The strain L1 exhibited phenotypic similarity to the Bacillus spp. with respect to the biochemical, morphological, Gram staining, and cultural characteristics. Nucleotide blast on NCBI revealed that strain L1 shared extremely high similarity with Bacillus velezensis species (99%). On the basis of the strain L1 16S rRNA’s sequence and blast alignment results, MEGA-X was employed to construct a phylogenetic neighbor connection tree that reflected the evolutionary relationship for L1 (Figure 1B). Combining all the resulting analyses, the isolate was identified to be B. velezensis (GenBank Accession No. ON340771).
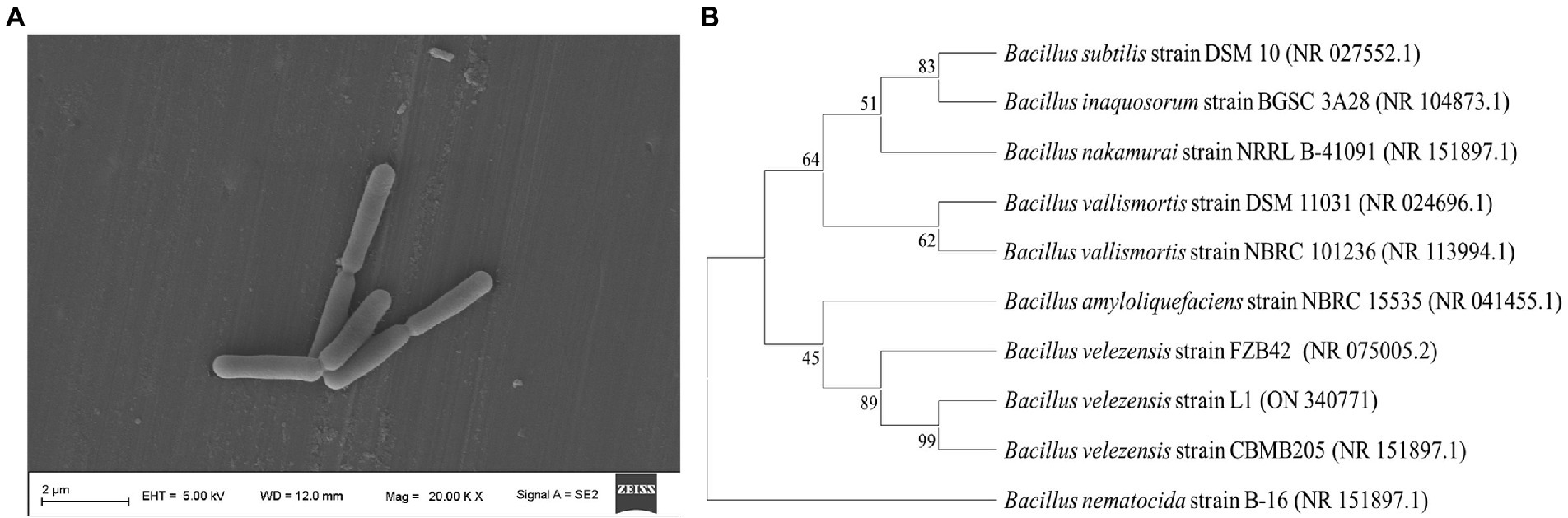
Figure 1. (A) Morphological characteristics of B. velezensis L1 detected by a scanning electron microscope. (B) Neighbor-joining tree of the B. velezensis L1 and its closely related species with the MEGA-X and 16S ribosomal RNA-sequencing data. The numbers at the nodes support the values derived from the MEGA-X bootstrap analysis.
In vitro antifungal activity of VOCs
Figure 2 presents the findings related to the antifungal activity of VOCs produced by B. velezensis L1. Mycelial development, spore germination, and sporulation ability of A. iridiaustralis were significantly (p < 0.05) inhibited by VOCs produced by strain L1. An increased inoculum concentration of B. velezensis L1 improved VOC-induced inhibition. Furthermore, increasing the concentration also enhanced the inhibitory effect of VOCs on pathogenic fungi. The mycelial growth of plug and spore suspensions was inhibited by the bacterial suspension at 1 × 109 CFU/ml by approximately 92.86 and 90.30%, respectively (Figures 2A,B); the spore germination and sporulation processes were inhibited by 33.11 and 12.04%, respectively (Figures 2C,D). A. iridiaustralis fumigated with VOCs grew more slowly than usual, as observed in (Figure 2E). The hyphal morphology of pathogenic fungi are dramatically altered by VOCs, as observed through SEM. The treatment group’s hyphae were twisted, flattened, enlarged, and lost their linearity compared with the control group (Figure 2F).
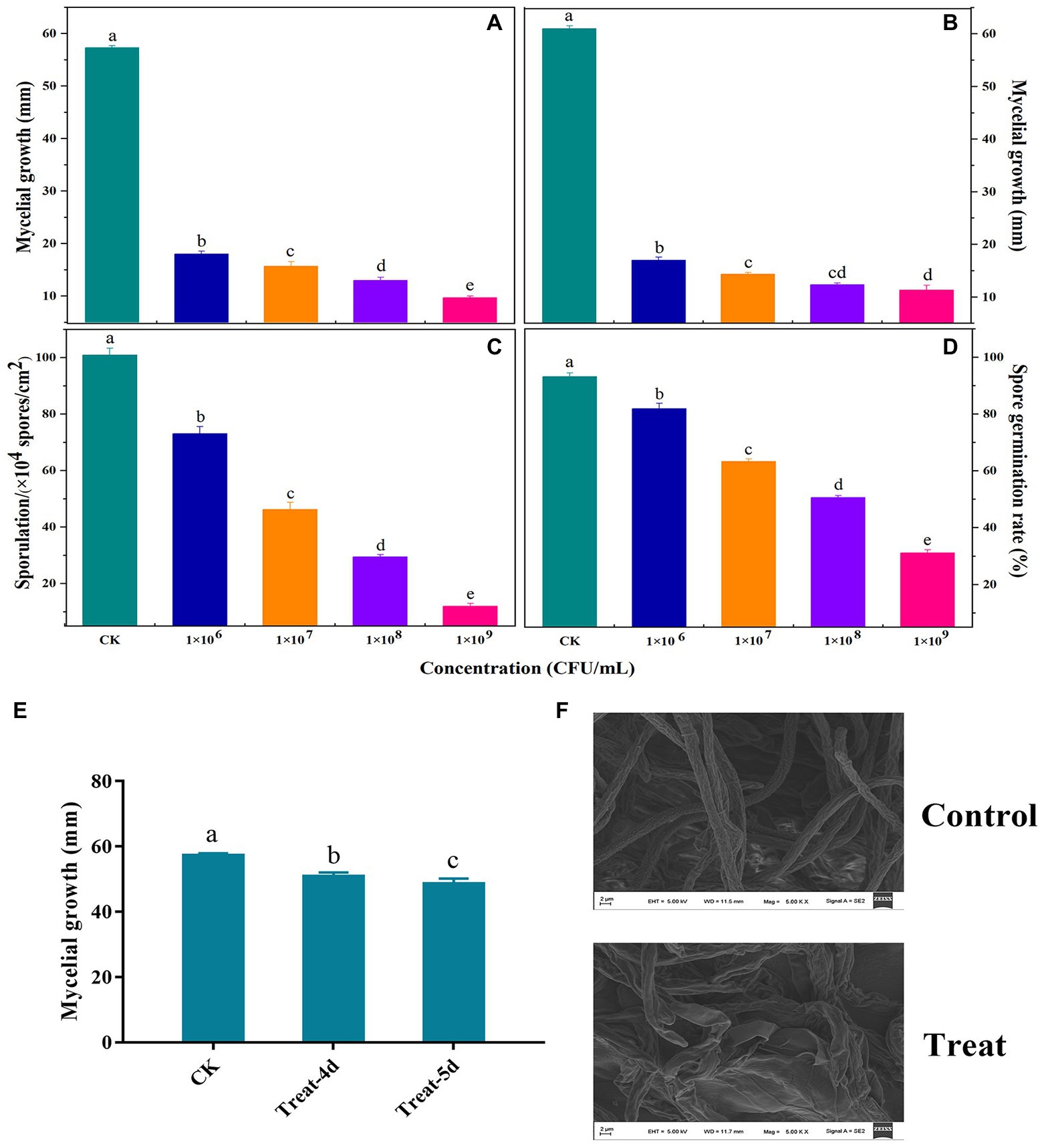
Figure 2. Antifungal activity of volatile organic compounds (VOCs) released by B. velezensis L1 against A. iridiaustralis in vitro. LB plates were coated with 80 μl of bacterial suspension at the concentration of 106, 107, 108, 109 colony forming units (CFU)/ml and then incubated at 37°C for 2 days. Their lids were subsequently replaced with PDA plates containing a 6 mm-diameter fungal disk or 20 μl of spore suspension of A. iridiaustralis. These plates were incubated at 28°C for 7 days. (A) Mycelial growth inhibition of a fungal disk of A. iridiaustralis on PDA plates exposed to VOCs at different concentrations. Different letters in represent differences (p < 0.05) among groups. (B) Mycelial growth inhibition 20 μl of spore suspension of A. iridiaustralis on PDA plates exposed to VOCs. Effect of VOCs produced by B. velezensis L1 suspension at different concentrations on sporulation (C) and spore germination (D) of A. iridiaustralis. Data are presented as means ± SE with three replicates. (E) Mycelial of A. iridiaustralis was fumigated with VOCs for 4 and 5 days, then a 6-mm-diameter fungal disk was inoculated on fresh PDA plates, after incubated at 28°C for 7 days, the diameter of the mycelium was measured. (F) Scanning electronic micrographs of A. iridiaustralis in the absence and presence of the VOCs produced by B. velezensis L1 strain.
In vivo biocontrol effects of VOCs
To investigate the impact of VOC concentration on the effectiveness of biocontrol ability of VOC in vivo, various numbers of LB plates containing viable cells were used. VOCs considerably impeded the growth and spread of A. iridiaustralis (Figure 3). No mycelial was observed growth on the wolfberry fruits after exposure to the 8 LB plates. The decay incidence and disease index of the treatment group were 4.45 and 3.33%, respectively, which were considerably lower than those of the control group (100 and 50.67%, respectively). In general, biological control effects were better at higher VOC concentrations.
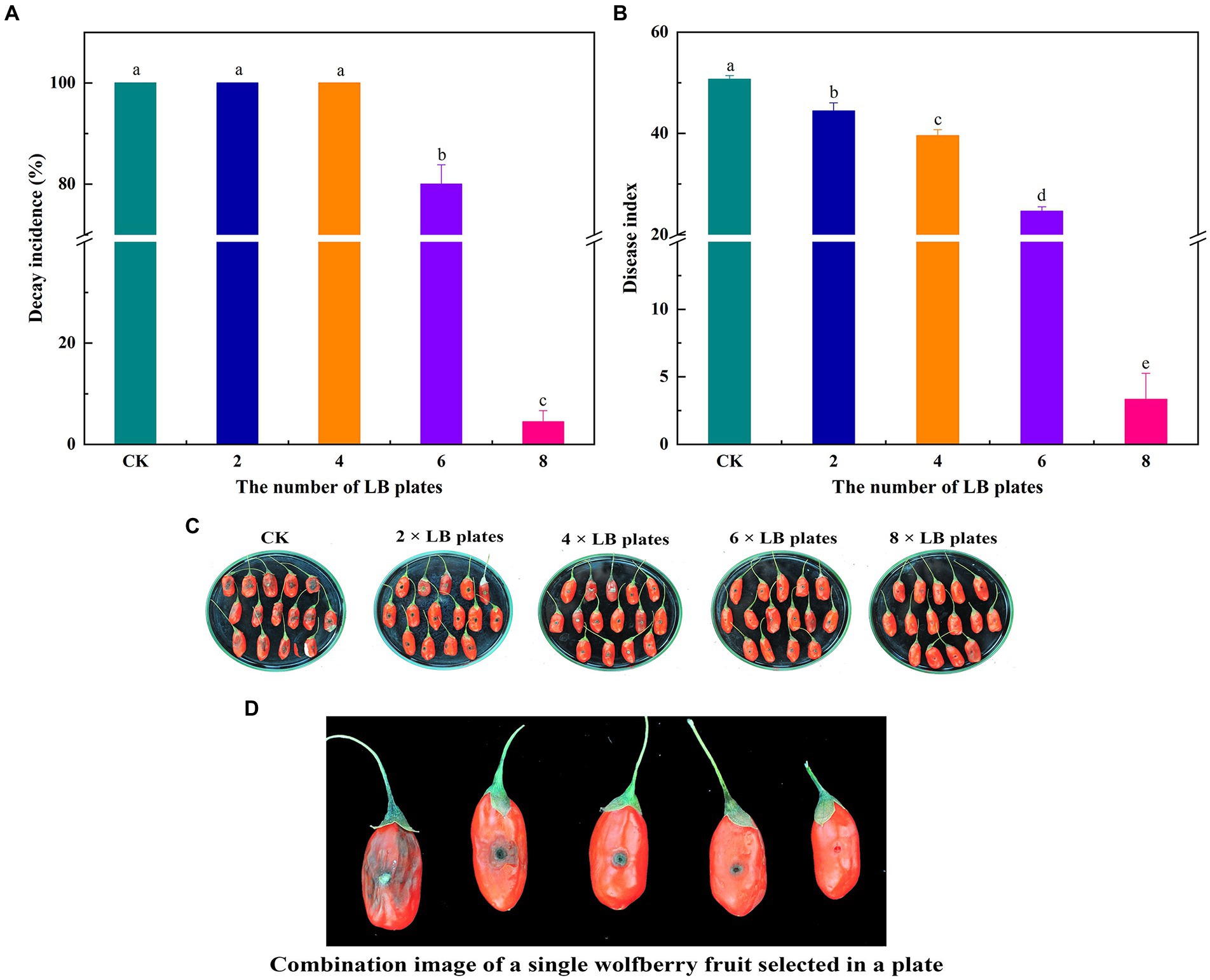
Figure 3. Fruits were fumigated with 2, 4, 6 and 8 LB plates, respectively, and the decay incidence (A) and disease index (B) were calculated according to the disease severity of the fruit infected with A. iridiaustralis. Biocontrol of wolfberry rot by biofumigation with different numbers of LB plates (C). Combination image of a single wolfberry fruit selected in a plate (D). Columns with different lower-case letters within the same panel are significantly different at p < 0.05 level according to Duncan’s multiple range test.
Effect of VOCs on postharvest natural decay
Figure 4 demonstrates the outcomes of the impact of VOCs on the fruit’s natural decay after harvest. Under natural circumstances, the VOCs generated by B. velezensis L1 had a larger inhibitory effect on the decay incidence of postharvest wolfberry as the number of LB plates increased. The best inhibitory effect was observed when 8 LB plates were used, the wolfberry fruit was intact, and the lowest disease index was 3.33.
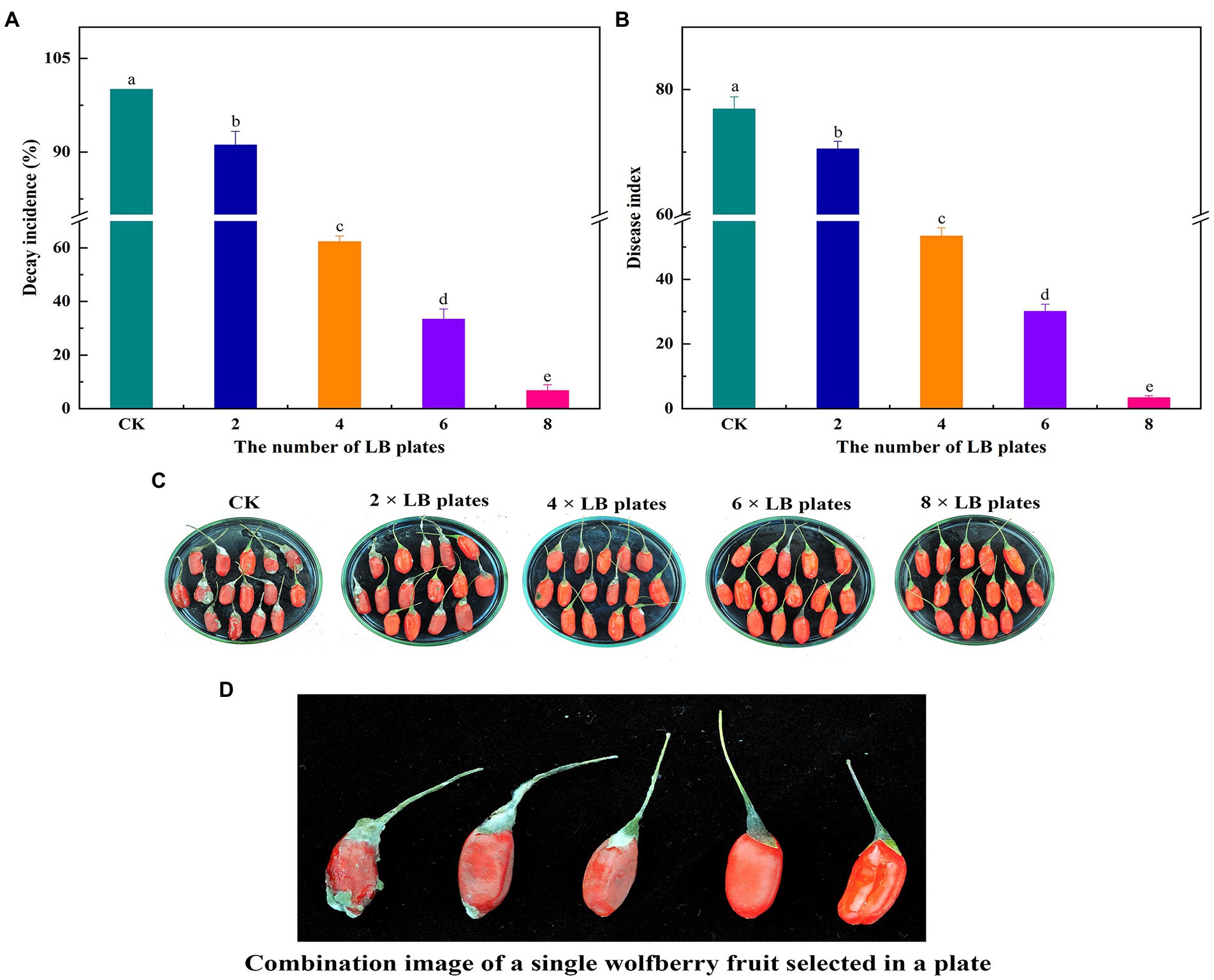
Figure 4. 2, 4, 6 and 8 LB plates were used to fumigate untreated wolfberry fruit. The container were sealed and followed by incubation at room temperature for 7 days, the decay incidence (A) and disease index (B) were measured. Biocontrol of VOCs produced by B. velezensis L1 on postharvest natural decay of wolfberry fruit (C) and combination image of a single wolfberry fruit selected in a plate (D). Columns with different lower-case letters within the same panel are significantly different at p < 0.05 level according to Duncan’s multiple range test.
HS-GC-IMS identification of VOCs
From Figure 5, the whole VOC information of each sample as well as the variations in VOCs across samples can be observed when each row represents all signal peaks selected in a single sample and each column represents the signal peaks of the same VOC in numerous samples. The chemical’s concentration is qualitatively represented by the hues of the peaks; the higher the red color, the higher the concentration. Table 2 lists a collection of specific details. Strain L1 produced the following recognized VOCs: 2-butanone, 2,3-butanedione, 1-hydroxy-2-propanone, 2-pentanone, acetoin, 2-heptanone, cyclohexanone, methyl 2-methylbutanoate, 2-pentylfuran, and 2-methylpropyl butanoate.
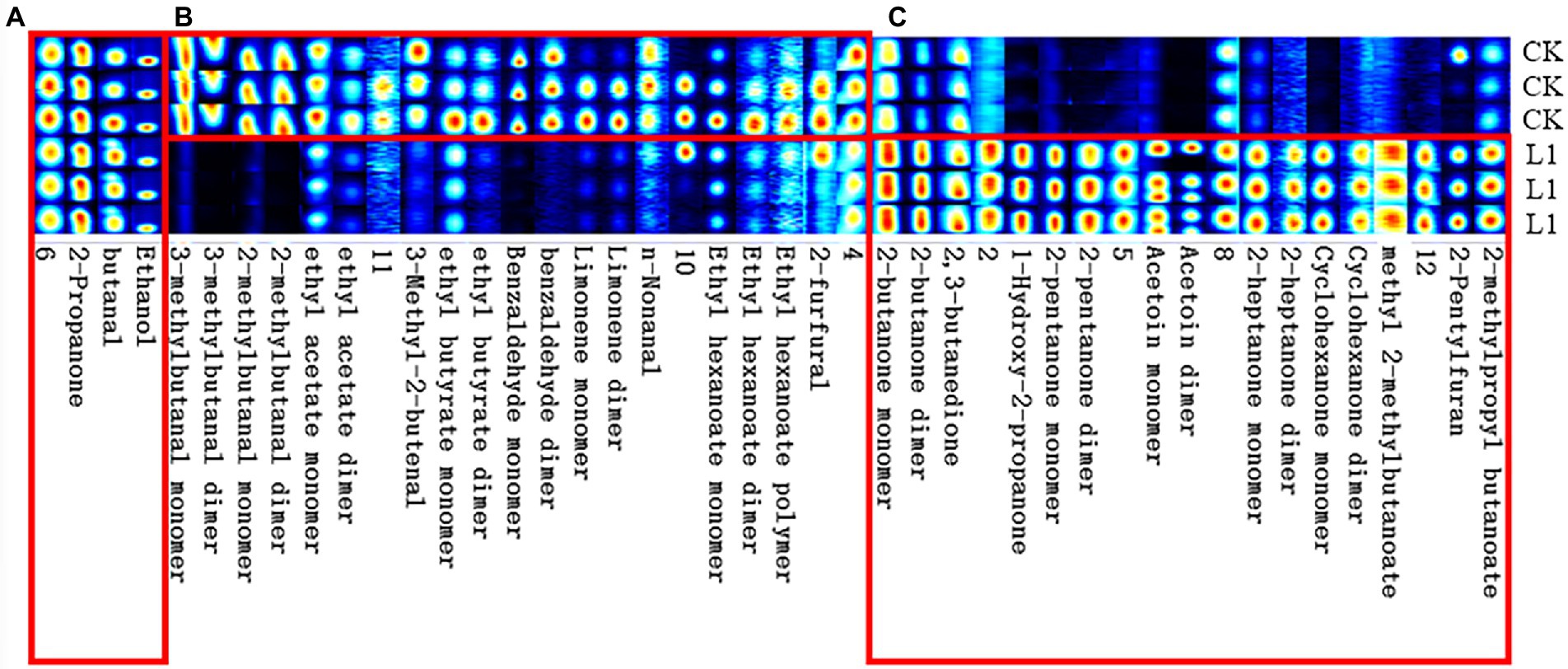
Figure 5. The Gallery plot of selected VOCs in the gas-phase ion mobility spectrum. Each row in the figure represents that all the signal peaks were selected in one sample, and each column represented the signal peaks of the same VOCs in different samples. Substances in area (A) were both identified in CK and B. velezensis L1. Substances in area (B) were identified in CK. Substances in area (C) were identified in B. velezensis L1.
Alternaria iridiaustralis is inhibited by a single known VOC
The effects of nine components on the growth of A. iridiaustralis mycelium were determined (Table 3). Among the nine tested pure substances, only 2,3-butanedione (40 μl/L) showed a strong effect, completely inhibiting the growth of the A. iridiaustralis mycelium. The remaining substances, including 1-hydroxy-2-propanone, 2-pentanone, acetoin, 2-heptanone, cyclohexanone, methyl 2-methylbutanoate, 2-pentylfuran and 2-methylpropyl butanoate, showed very weak or no antifungal activity on PDA plates.
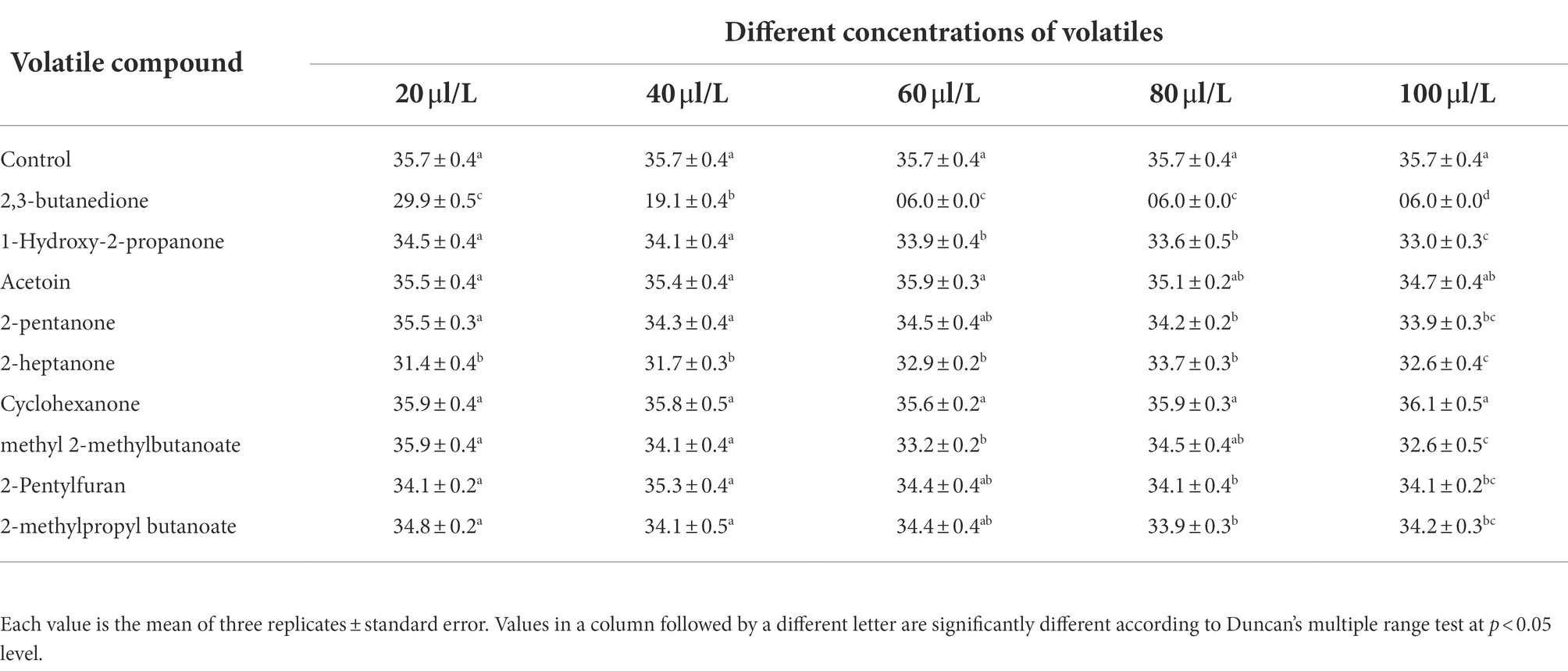
Table 3. Effects different concentrations of tested compounds on the mycelial growth of A. iridiaustralis after an incubation at 28°C for 4 d.
Effects of pure VOC chemicals against Alternaria iridiaustralis on wolfberry
As shown in Table 4, 2,3-butanedione was found to be an effective BCA. At 60 μl/L, it completely inhibited the growth of A. iridiaustralis, and no obvious lesions were found on the wolfberry fruits. The decay incidence and disease index decreased significantly.
Broad spectrum antifungal activity of volatiles from Bacillus velezensis L1
Ten important pathogenic fungi including P. capsici, C. capsici, F. oxysporum, B. cinerea, R. solani, F. graminearum, F. annulatum, T. tumuli, C. fioriniae and F. arcuatisporum, were used to test the broad antifungal activity of B. velezensis L1. In the strain L1 treatment, the mycelial growth of the pathogenic fungi was greatly inhibited (Figure 6). The inhibition rate of L1 strain against the nine pathogenic strains was >80%. These results suggest that active volatiles produced by B. velezensis L1 have a broad antifungal activity against different genera of pathogenic fungi.
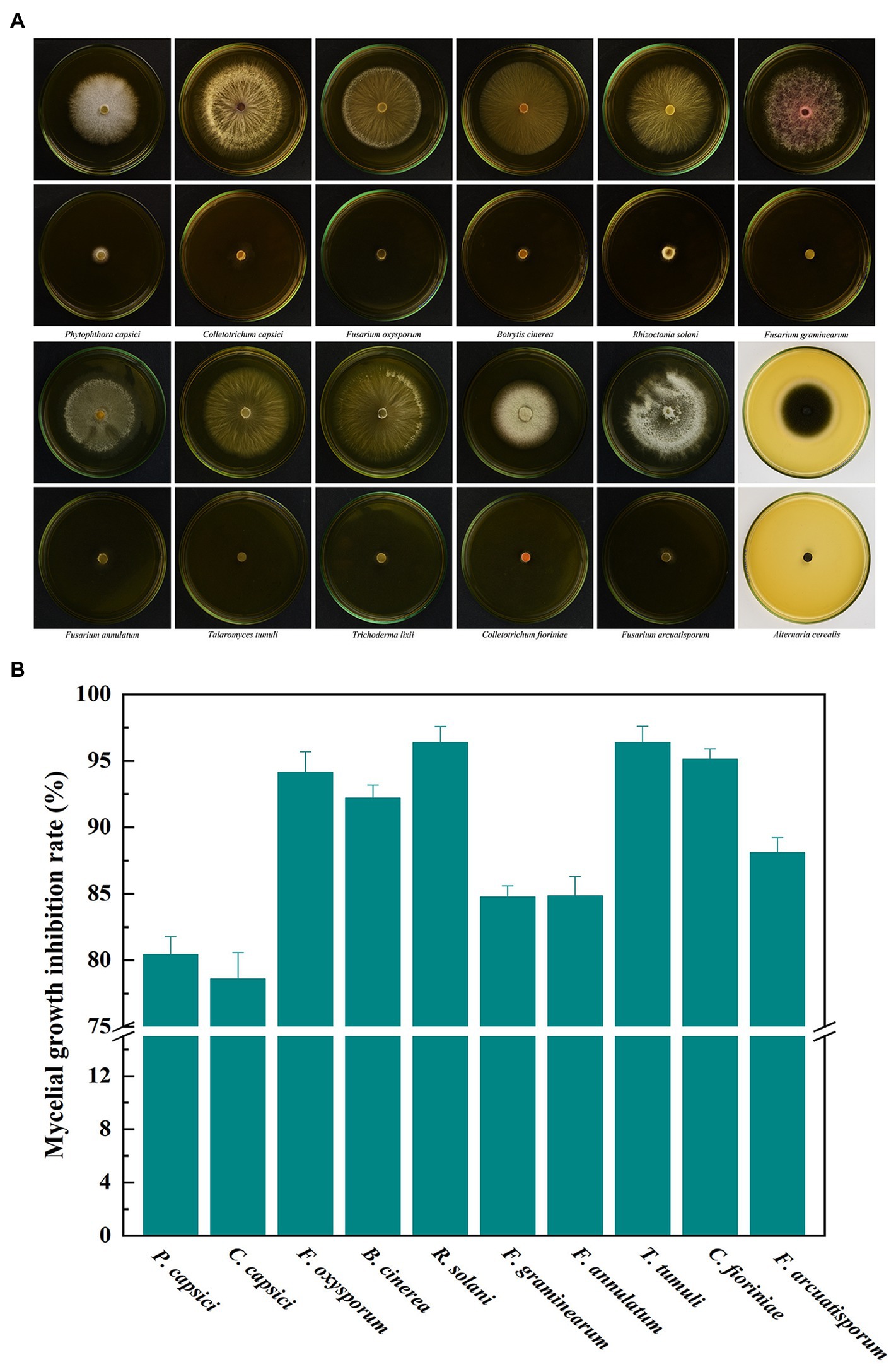
Figure 6. (A) Broad antifungal activity of B. velezensis L1 against ten fungal pathogens. (B) Corresponding histograms for statistical data on mycelial growth inhibition rate. Different fungal strains including P. capsici, C. capsici, F. oxysporum, B. cinerea, R. solani, F. graminearum, F. annulatum, T. tumuli, C. fioriniae and F. arcuatisporum were used in the tests.
Discussion
Postharvest regulation of fruits and vegetables can result in an incredibly high value (Lemos Junior et al., 2020). Recent VOCs control research has produced positive results and is regarded as a useful alternative strategy for reducing fungal infection (Raza et al., 2016; Syed-Ab-Rahman et al., 2019). In this study, we isolated and characterized a strain of B. velezensis L1 that suppresses A. iridiaustralis growth in wolfberry during the postharvest process and displayed the broad-spectrum antifungal activity of VOCs obtained from B. velezensis L1. Therefore, B. velezensis L1 is anticipated to be employed as an antagonistic microbe. To the best of our knowledge, this is the first known study exhibiting the antagonistic activity of B. velezensis VOCs against A. iridiaustralis on postharvest wolfberry.
In vitro antifungal experiments revealed that VOCs produced by B. velezensis L1 strongly inhibited the growth of A. iridiaustralis mycelium, spore germination, and sporulation. VOCs generated by B. velezensis C16 significantly suppress A. solani mycelium development and spore germination (Zhang et al., 2021). According to Jiang et al. (2018), VOCs from the B. velezensis strain may prevent sporulation of B. cinerea in peppers. This agrees with the findings obtained for B. velezensis L1. Additionally, the regrowth of A. iridiaustralis was reduced by the VOCs from B. velezensis L1. The effects of VOCs on B. cinerea were comparable to those of Pseudomonas fluorescens ZX (Zhong et al., 2021). This might be because VOCs disrupt the pathogenic fungi’s natural morphological structure and interfere with their normal proliferation. However, the damage appears to be lost with a reduction in VOC concentration, possibly because of the robust recovery and reproductive capacity of the fungi. According to SEM analysis, the VOCs from B. velezensis L1 can severely damage the morphology of A. iridiaustralis. Numerous studies have demonstrated that VOCs can harm pathogenic the walls and membrane systems of pathogenic fungi, impairing their ability to perform essential tasks (Zhao et al., 2019; Wang et al., 2021b). This might be one of the key ways through which VOCs prevent harmful the growth of fungi.
The effectiveness of VOCs in vivo was further investigated in light of the superior inhibitory capacity of B. velezensis L1 in vitro. The findings demonstrated that VOCs from B. velezensis L1 can greatly reduce disease severity in wolfberry. Although many descriptive studies have investigated the effectiveness of VOCs (Archana et al., 2021; Wang et al., 2021a), more research is focused on the screening of strains and the identification of volatiles produced, with most authors not choosing to explore the relationship between their concentrations and diseases, which may be due to difficulties associated with testing. Calvo et al. (2020) reported that the B. velezensis strain inhibits mycelial growth and sporulation and is not pathogenic to humans after use. However, the VOCs concentration was not considered in their study. Myo et al. (2019) found that B. velezensis NKG-2 adversely affected the growth of six pathogenic fungi, but they too did not consider the VOCs concentration. The effect of VOC concentrations on the prevention, occurrence, and severity of diseases is of great value for the postharvest preservation of fruits and vegetables (Sharifi and Ryu, 2016). These concentrations were influenced by different numbers of LB plates. With an increase in concentration, the disease status of wolfberry fruits inoculated with the pathogenic fungus A. iridiaustralis changed significantly. Wang et al. (2021c) also confirmed that a certain concentration of VOCs produced by P. fluorescens ZX can significantly control gray mold in citrus and inhibit decay development. When VOCs reach a certain concentration, the growth of pathogenic fungi in fruits is completely inhibited, resulting in the best control effect. This suggests that it is entirely feasible to utilize VOCs produced by antagonistic microorganisms as potential BCAs. More importantly, VOCs also have an excellent control effect on fruits that rot naturally after harvest, inhibited all pathogenic fungi that caused the disease, which greatly prolongs the storage time of wolfberry. Studies have confirmed that VOCs produced by some bacteria, yeast and fungi can induce resistance against pathogens in fruits and vegetables (Zheng et al., 2019). This may be related to another antifungal mechanism of VOCs. Zhou et al. (2019) reported that the VOCs of strain CF-3 could reduce the enzymatic activity involved in fruit decomposition, activate the antioxidant enzymes to prevent cell damage, and elevate the disease-resistant enzyme activity to prevent the invasion of pathogenic fungi, thereby inducing resistance to fungi. Lee et al. (2019) showed that VOCs released by microorganisms induce self-resistance in the host to avoid the pathogen-induced harm. For the control of postharvest natural decay, strain L1 can be used as a new preservative with significant effect, which is of great value in commercial production.
In general, antagonistic microorganisms can produce VOCs such as ketones, alcohols, aldehydes, esters, and acidic and aromatic compounds with antifungal activity, and different strains released VOCs are specific (Raza et al., 2015; Kai, 2020). In addition, VOCs can be altered by changes in culture conditions. For example, P. fluorescens ZX produced different VOCs when incubated on NB or NA (Wang et al., 2020). B. amyloliquefaciens PP19, Exiguobacterium acetylicum SI17, B. pumilus PI26 were reported to vary greatly in their VOCs with incubation time and between strains (L. Zheng et al., 2019). The FlavourSpec® Flavor Analyzer, which uses HS-GC-IMS technology, is mainly used for the research of food flavor (Wang et al., 2019; Yuan et al., 2019). Because of the high sensitivity and high resolution, the HS-GC-IMS technique is effective in identifying VOCs released by different strains. In this study, based on the complexity and diversity of VOCs, volatile gas chemicals emitted by B. velezensis L1 were identified using HS-GC-IMS, and 10 main components were found. The components identified varied from the volatile components discovered in other studies, which could be due to variations in the growth environment of the hostile bacteria (Wallace et al., 2017). On the other hand, the results obtained may vary because of the use of different types of equipment and materials in experiments to identify VOC components.
Nine pure compounds were commercially available and selected for further analysis. Among the components identified, 2,3-butanedione had the strongest inhibitory effect on pathogenic fungi both in vitro and in vivo. 2,3-Butanedione could reduce the rot disease index of wolfberry fruit. Compared with the complex VOCs components, pure compounds have clear structural characteristics and are easy to produce and synthesize and may have practical applications in future. Calvo et al. (2020) showed that 20 μl/L of 2,3-butanedione could completely control gray mold in grapes and reduce blue rot in mandarins to 60%. Gergolet Diaz et al. (2021) showed that 2,3-butanedione had the strongest inhibitory effect on the mycelial growth of F. verticillioides. 2,3-Butanedione is a naturally occurring, safe and edible volatile alpha-diketone often used in food additives and flavors because of its good flavor. Pathogenic fungi are very sensitive to even very low concentrations of VOC, and therefore, this VOC is considered a promising compound for postharvest preservation (Zheng et al., 2020). 2-Pentanone, 2-heptanone, and acetoin are the most common and abundant VOCs in many strains (Wu et al., 2015; Lazazzara et al., 2021). As observed in our study, they have little impact on the hyphal development of pathogenic fungi. However, VOCs are usually complex in composition, and their inhibitory effects often do not depend on a single active ingredient (Wang et al., 2021c). Few studies have investigated the optimal application and activity of the combination of VOC. Thus, whether the main components in these VOCs are synergistically involved in the antifungal effect deserves further investigation. 1-Hydroxy-2-propanone, cyclohexanone, methyl 2-methylbutanoate, 2-pentylfuran and 2-methylpropyl butan were found to be produced by the B. velezensis strain for the first time. However, they showed no antifungal activity against A. iridiaustralis in the tests. This could be related to the concentration chosen, some antifungal compounds showed weak activity or were ineffective at low concentrations (Vicentini et al., 2007). However, it is worth considering that high concentrations of antifungal substances can harm the human body, which is not in line with our original intention.
In addition, tests on pathogenic fungi that cause severe diseases in crops, fruits, vegetables and other foods have found that VOCs from B. velezensis L1 also significantly inhibit its growth. This provides more possibilities for the application of B. velezensis L1 and its VOCs as preservatives for controlling these diseases during storage. For different pathogenic fungi, the inhibitory effect of VOCs is also different. At present, the antifungal mechanisms discovered by VOCs mainly include inhibition of mycelial growth, damage to cell walls, regulation of enzyme activities, and induction of host resistance (Zhong et al., 2021). It is still important to explore the interactions and connections between VOCs, pathogenic fungi and hosts.
This work is the first to investigate the inhibitory effect of B. velezensis L1 on postharvest disease of wolfberry. In experiments, B. velezensis L1 and its volatiles (2,3-butanedione) can effectively reduce the decay caused by pathogenic fungi and prolong storage time. These results provide favorable evidence for the biocontrol activity of strain L1 against A. iridiaustralis and other important fungal pathogens, and also provides insights to develop storage systems for fresh fruits and vegetables after harvest.
Data availability statement
The original contributions presented in the study are included in the article/supplementary material, further inquiries can be directed to the corresponding author.
Author contributions
All authors contributed to the study conception and design. Material preparation, data collection, and analysis were performed by HL, CY, and LL. The first draft of the manuscript was written by HL. All authors contributed to the article and approved the submitted version.
Funding
The Lanzhou Science and Technology Plan Project 2018-1-104 is sincerely acknowledged by the authors; Special Fund Project for Guiding Science and Technology Innovation and Development in Gansu Province 2019ZX-05. Higher Education Industry Support Plan of Gansu Province (2020C-21).
Conflict of interest
The authors declare that the research was conducted in the absence of any commercial or financial relationships that could be construed as a potential conflict of interest.
Publisher’s note
All claims expressed in this article are solely those of the authors and do not necessarily represent those of their affiliated organizations, or those of the publisher, the editors and the reviewers. Any product that may be evaluated in this article, or claim that may be made by its manufacturer, is not guaranteed or endorsed by the publisher.
References
Alijani, Z., Amini, J., Ashengroph, M., and Bahramnejad, B. (2019). Antifungal activity of volatile compounds produced by Staphylococcus sciuri strain MarR44 and its potential for the biocontrol of Colletotrichum nymphaeae, causal agent strawberry anthracnose. Int. J. Food Microbiol. 307:108276. doi: 10.1016/j.ijfoodmicro.2019.108276
Archana, T. J., Gogoi, R., Kaur, C., Varghese, E., Sharma, R. R., Srivastav, M., et al. (2021). Bacterial volatile mediated suppression of postharvest anthracnose and quality enhancement in mango. Postharvest Biol. Technol. 177:111525. doi: 10.1016/j.postharvbio.2021.111525
Arrarte, E., Garmendia, G., Rossini, C., Wisniewski, M., and Vero, S. (2017). Volatile organic compounds produced by Antarctic strains of Candida sake play a role in the control of postharvest pathogens of apples. Biol. Control 109, 14–20. doi: 10.1016/j.biocontrol.2017.03.002
Bu, S., Munir, S., He, P., Li, Y., Wu, Y., Li, X., et al. (2021). Bacillus subtilis L1-21 as a biocontrol agent for postharvest gray mold of tomato caused by Botrytis cinerea. Biol. Control 157:104568. doi: 10.1016/j.biocontrol.2021.104568
Calvo, H., Mendiara, I., Arias, E., Gracia, A. P., Blanco, D., and Venturini, M. E. (2020). Antifungal activity of the volatile organic compounds produced by Bacillus velezensis strains against postharvest fungal pathogens. Postharvest Biol. Technol. 166:111208. doi: 10.1016/j.postharvbio.2020.111208
Di Francesco, A., Di Foggia, M., and Baraldi, E. (2020a). Aureobasidium pullulans volatile organic compounds as alternative postharvest method to control brown rot of stone fruits. Food Microbiol. 87:103395. doi: 10.1016/j.fm.2019.103395
Di Francesco, A., Di Foggia, M., and Baraldi, E. (2020b). Aureobasidium pullulans volatile organic compounds as alternative postharvest method to control brown rot of stone fruits. Food Microbiol. 87:103395. doi: 10.1016/j.fm.2019.103395
Do Kim, J., Kang, J. E., and Kim, B. S. (2020). Postharvest disease control efficacy of the polyene macrolide lucensomycin produced by Streptomyces plumbeus strain CA5 against gray mold on grapes. Postharvest Biol. Technol. 162:111115. doi: 10.1016/j.postharvbio.2019.111115
Gao, Z., Zhang, B., Liu, H., Han, J., and Zhang, Y. (2017). Identification of endophytic Bacillus velezensis ZSY-1 strain and antifungal activity of its volatile compounds against Alternaria solani and Botrytis cinerea. Biol. Control 105, 27–39. doi: 10.1016/j.biocontrol.2016.11.007
Gergolet Diaz, D. G., Pizzolitto, R. P., Vázquez, C., Usseglio, V. L., Zunino, M. P., Dambolena, J. S., et al. (2021). Effects of the volatile organic compounds produced by enterococcus spp. strains isolated from maize grain silos on Fusarium verticillioides growth and fumonisin B1 production. J. Stored Prod. Res. 93:101825. doi: 10.1016/j.jspr.2021.101825
Gotor-Vila, A., Teixidó, N., Di Francesco, A., Usall, J., Ugolini, L., Torres, R., et al. (2017). Antifungal effect of volatile organic compounds produced by Bacillus amyloliquefaciens CPA-8 against fruit pathogen decays of cherry. Food Microbiol. 64, 219–225. doi: 10.1016/j.fm.2017.01.006
Jiang, C. H., Liao, M. J., Wang, H. K., Zheng, M. Z., Xu, J. J., and Guo, J. H. (2018). Bacillus velezensis, a potential and efficient biocontrol agent in control of pepper gray mold caused by Botrytis cinerea. Biol. Control 126, 147–157. doi: 10.1016/j.biocontrol.2018.07.017
Kai, M. (2020). Diversity and distribution of volatile secondary metabolites throughout Bacillus subtilis isolates. Front. Microbiol. 11:559. doi: 10.3389/fmicb.2020.00559
Lazazzara, V., Vicelli, B., Bueschl, C., Parich, A., Pertot, I., Schuhmacher, R., et al. (2021). Trichoderma spp. volatile organic compounds protect grapevine plants by activating defense-related processes against downy mildew. Physiol. Plant. 172, 1950–1965. doi: 10.1111/ppl.13406
Lee, S., Behringer, G., Hung, R., and Bennett, J. (2019). Effects of fungal volatile organic compounds on Arabidopsis thaliana growth and gene expression. Fungal Ecol. 37, 1–9. doi: 10.1016/j.funeco.2018.08.004
Lemos Junior, W. J. F., Binati, R. L., Felis, G. E., Slaghenaufi, D., Ugliano, M., and Torriani, S. (2020). Volatile organic compounds from Starmerella bacillaris to control gray mold on apples and modulate cider aroma profile. Food Microbiol. 89:103446. doi: 10.1016/j.fm.2020.103446
Li, X., Wang, X., Shi, X., Wang, B., Li, M., Wang, Q., et al. (2020). Antifungal Effect of Volatile Organic Compounds from Bacillus velezensis CT32 against Verticillium dahliae and Fusarium oxysporum. Processes 8:1674. doi: 10.3390/pr8121674
Ling, L., Li, Z., Jiao, Z., Zhang, X., Ma, W., Feng, J., et al. (2019). Identification of novel endophytic yeast strains from tangerine Peel. Curr. Microbiol. 76, 1066–1072. doi: 10.1007/s00284-019-01721-9
Ling, L., Zhao, Y., Tu, Y., Yang, C., Ma, W., Feng, S., et al. (2021). The inhibitory effect of volatile organic compounds produced by Bacillus subtilis CL2 on pathogenic fungi of wolfberry. J. Basic Microbiol. 61, 110–121. doi: 10.1002/jobm.202000522
Mari, M., Bautista-Baños, S., and Sivakumar, D. (2016). Decay control in the postharvest system: role of microbial and plant volatile organic compounds. Postharvest Biol. Technol. 122, 70–81. doi: 10.1016/j.postharvbio.2016.04.014
Myo, E. M., Liu, B., Ma, J., Shi, L., Jiang, M., Zhang, K., et al. (2019). Evaluation of Bacillus velezensis NKG-2 for bio-control activities against fungal diseases and potential plant growth promotion. Biol. Control 134, 23–31. doi: 10.1016/j.biocontrol.2019.03.017
Oro, L., Feliziani, E., Ciani, M., Romanazzi, G., and Comitini, F. (2018). Volatile organic compounds from Wickerhamomyces anomalus, Metschnikowia pulcherrima and Saccharomyces cerevisiae inhibit growth of decay causing fungi and control postharvest diseases of strawberries. Int. J. Food Microbiol. 265, 18–22. doi: 10.1016/j.ijfoodmicro.2017.10.027
Qin, X., Xiao, H., Cheng, X., Zhou, H., and Si, L. (2017). Hanseniaspora uvarum prolongs shelf life of strawberry via volatile production. Food Microbiol. 63, 205–212. doi: 10.1016/j.fm.2016.11.005
Raza, W., Ling, N., Liu, D., Wei, Z., Huang, Q., and Shen, Q. (2016). Volatile organic compounds produced by Pseudomonas fluorescens WR-1 restrict the growth and virulence traits of Ralstonia solanacearum. Microbiol. Res. 192, 103–113. doi: 10.1016/j.micres.2016.05.014
Raza, W., Yuan, J., Ling, N., Huang, Q., and Shen, Q. (2015). Production of volatile organic compounds by an antagonistic strain Paenibacillus polymyxa WR-2 in the presence of root exudates and organic fertilizer and their antifungal activity against Fusarium oxysporum f. sp. niveum. Biol. Control 80, 89–95. doi: 10.1016/j.biocontrol.2014.09.004
Sharifi, R., and Ryu, C. M. (2016). Are bacterial volatile compounds poisonous odors to a fungal pathogen Botrytis cinerea, alarm signals to arabidopsis seedlings for eliciting induced resistance, or both? Front. Microbiol. 7:196. doi: 10.3389/fmicb.2016.00196
Syed-Ab-Rahman, S. F., Carvalhais, L. C., Chua, E. T., Chung, F. Y., Moyle, P. M., Eltanahy, E. G., et al. (2019). Soil bacterial diffusible and volatile organic compounds inhibit Phytophthora capsici and promote plant growth. Sci. Total Environ. 692, 267–280. doi: 10.1016/j.scitotenv.2019.07.061
Toffano, L., Fialho, M. B., and Pascholati, S. F. (2017). Potential of fumigation of orange fruits with volatile organic compounds produced by Saccharomyces cerevisiae to control citrus black spot disease at postharvest. Biol. Control 108, 77–82. doi: 10.1016/j.biocontrol.2017.02.009
Vicentini, C. B., Romagnoli, C., Andreotti, E., and Mares, D. (2007). Synthetic pyrazole derivatives as growth inhibitors of some phytopathogenic fungi. J. Agric. Food Chem. 55, 10331–10338. doi: 10.1021/jf072077d
Wallace, R. L., Hirkala, D. L., and Nelson, L. M. (2017). Postharvest biological control of blue mold of apple by Pseudomonas fluorescens during commercial storage and potential modes of action. Postharvest Biol. Technol. 133, 1–11. doi: 10.1016/j.postharvbio.2017.07.003
Wang, Z., Mei, X., Du, M., Chen, K., Jiang, M., Wang, K., et al. (2020). Potential modes of action of Pseudomonas fluorescens ZX during biocontrol of blue mold decay on postharvest citrus. J. Sci. Food Agric. 100, 744–754. doi: 10.1002/jsfa.10079
Wang, F., Xiao, J., Zhang, Y., Li, R., Liu, L., and Deng, J. (2021a). Biocontrol ability and action mechanism of Bacillus halotolerans against Botrytis cinerea causing grey mould in postharvest strawberry fruit. Postharvest Biol. Technol. 174:111456. doi: 10.1016/j.postharvbio.2020.111456
Wang, X., Yang, S., He, J., Chen, L., Zhang, J., Jin, Y., et al. (2019). A green triple-locked strategy based on volatile-compound imaging, chemometrics, and markers to discriminate winter honey and sapium honey using headspace gas chromatography-ion mobility spectrometry. Food Res. Int. 119, 960–967. doi: 10.1016/j.foodres.2019.01.004
Wang, Z., Zhong, T., Chen, K., Du, M., Chen, G., Chen, X., et al. (2021b). Antifungal activity of volatile organic compounds produced by Pseudomonas fluorescens ZX and potential biocontrol of blue mold decay on postharvest citrus. Food Control 120:107499. doi: 10.1016/j.foodcont.2020.107499
Wang, Z., Zhong, T., Chen, X., Yang, B., Du, M., Wang, K., et al. (2021c). Potential of volatile organic compounds emitted by pseudomonas fluorescens ZX as biological fumigants to control citrus green mold decay at postharvest. J. Agric. Food Chem. 69, 2087–2098. doi: 10.1021/acs.jafc.0c07375
Wei, D., Wang, Y., Jiang, D., Feng, X., Li, J., and Wang, M. (2017). Survey of Alternaria toxins and other mycotoxins in dried fruits in China. Toxins 9, 1–12. doi: 10.3390/toxins9070200
Wenli, S., Shahrajabian, M. H., and Qi, C. (2021). Health benefits of wolfberry (Gou Qi Zi, Fructus barbarum L.) on the basis of ancient Chinese herbalism and Western modern medicine. Avicenna J Phytomed. 11, 109–119. doi: 10.22038/AJP.2020.17147
Wonglom, P., Shin-Ichi, I., and Sunpapao, A. (2020). Volatile organic compounds emitted from endophytic fungus Trichoderma asperellum T1 mediate antifungal activity, defense response and promote plant growth in lettuce (Lactuca sativa). Fungal Ecol. 43:100867. doi: 10.1016/j.funeco.2019.100867
Wu, Y., Yuan, J., Yaoyao, E., Raza, W., Shen, Q., and Huang, Q. (2015). Effects of volatile organic compounds from Streptomyces albulus NJZJSA2 on growth of two fungal pathogens. J. Basic Microbiol. 55, 1104–1117. doi: 10.1002/jobm.201400906
Xu, F., Wang, S., Xu, J., Liu, S., and Li, G. (2016). Effects of combined aqueous chlorine dioxide and UV-C on shelf-life quality of blueberries. Postharvest Biol. Technol. 117, 125–131. doi: 10.1016/j.postharvbio.2016.01.012
Yajun, W., Xiaojie, L., Sujuan, G., Yuekun, L., Bo, Z., Yue, Y., et al. (2019). Evaluation of nutrients and related environmental factors for wolfberry (Lycium barbarum) fruits grown in the different areas of China. Biochem. Syst. Ecol. 86:103916. doi: 10.1016/j.bse.2019.103916
Ye, X., Chen, Y., Ma, S., Yuan, T., Wu, Y., Li, Y., et al. (2020). Biocidal effects of volatile organic compounds produced by the myxobacterium Corrallococcus sp. EGB against fungal phytopathogens. Food Microbiol. 91:103502. doi: 10.1016/j.fm.2020.103502
Yuan, Z. Y., Qu, H. Y., Xie, M. Z., Zeng, G., Huang, H. Y., Ren, F., et al. (2019). Direct authentication of three Chinese materia medica species of the Lilii Bulbus family in terms of volatile components by headspace-gas chromatography-ion mobility spectrometry. Anal. Methods 11, 530–536. doi: 10.1039/c8ay02338g
Yuan, H. J., Yang, A. M., and Wu, G. F. (2012). Etiology of fruit rot of barbary wolfberry (Lycium barbarum L.) in the drying process with insolation method in green house. Adv. Mater. Res. 554-556, 1530–1533. doi: 10.4028/www.scientific.net/AMR.554-556.1530
Zhang, D., Yu, S., Zhao, D., Zhang, J., Pan, Y., Yang, Y., et al. (2021). Inhibitory effects of non-volatiles lipopeptides and volatiles ketones metabolites secreted by Bacillus velezensis C16 against Alternaria solani. Biol. Control 152:104421. doi: 10.1016/j.biocontrol.2020.104421
Zhao, P., Li, P., Wu, S., Zhou, M., Zhi, R., and Gao, H. (2019). Volatile organic compounds (VOCs) from Bacillus subtilis CF-3 reduce anthracnose and elicit active defense responses in harvested litchi fruits. AMB Express 9:119. doi: 10.1186/s13568-019-0841-2
Zheng, Y., Fei, Y., Yang, Y., Jin, Z., Yu, B., and Li, L. (2020). A potential flavor culture: lactobacillus harbinensis M1 improves the organoleptic quality of fermented soymilk by high production of 2,3-butanedione and acetoin. Food Microbiol. 91:103540. doi: 10.1016/j.fm.2020.103540
Zheng, L., Situ, J. J., Zhu, Q. F., Xi, P. G., Zheng, Y., Liu, H. X., et al. (2019). Identification of volatile organic compounds for the biocontrol of postharvest litchi fruit pathogen Peronophythora litchii. Postharvest Biol. Technol. 155, 37–46. doi: 10.1016/j.postharvbio.2019.05.009
Zhong, T., Wang, Z., Zhang, M., Wei, X., Kan, J., Zalán, Z., et al. (2021). Volatile organic compounds produced by Pseudomonas fluorescens ZX as potential biological fumigants against gray mold on postharvest grapes. Biol. Control 163:104754. doi: 10.1016/j.biocontrol.2021.104754
Zhou, M., Li, P., Wu, S., Zhao, P., and Gao, H. (2019). Bacillus subtilis CF-3 volatile organic compounds inhibit Monilinia fructicola growth in peach fruit. Front. Microbiol. 10:1804. doi: 10.3389/fmicb.2019.01804
Keywords: volatile organic compounds, Bacillus velezensis L1, Alternaria iridiaustralis, wolfberry disease, biological control
Citation: Ling L, Luo H, Yang C, Wang Y, Cheng W, Pang M and Jiang K (2022) Volatile organic compounds produced by Bacillus velezensis L1 as a potential biocontrol agent against postharvest diseases of wolfberry. Front. Microbiol. 13:987844. doi: 10.3389/fmicb.2022.987844
Edited by:
Khamis Youssef, Agricultural Research Center, EgyptReviewed by:
Elsherbiny A. Elsherbiny, Mansoura University, EgyptSheng Qin, Jiangsu Normal University, China
Copyright © 2022 Ling, Luo, Yang, Wang, Cheng, Pang and Jiang. This is an open-access article distributed under the terms of the Creative Commons Attribution License (CC BY). The use, distribution or reproduction in other forums is permitted, provided the original author(s) and the copyright owner(s) are credited and that the original publication in this journal is cited, in accordance with accepted academic practice. No use, distribution or reproduction is permitted which does not comply with these terms.
*Correspondence: Lijun Ling, 13919343210@163.com