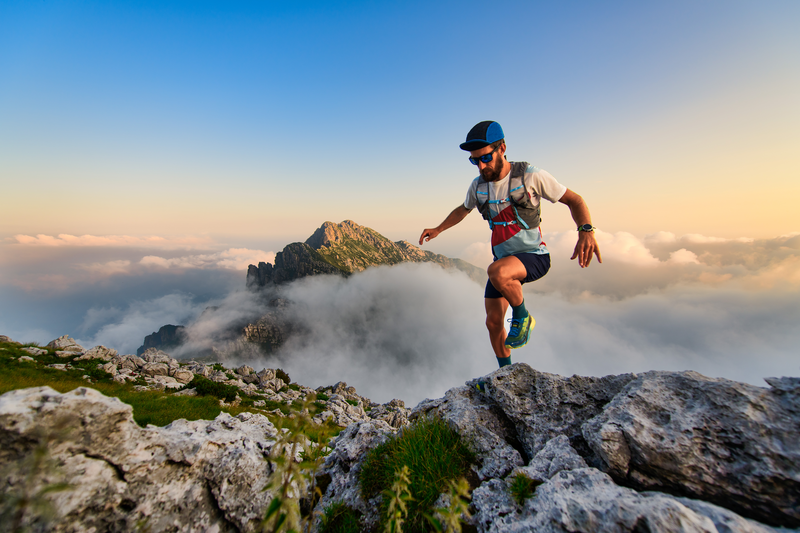
94% of researchers rate our articles as excellent or good
Learn more about the work of our research integrity team to safeguard the quality of each article we publish.
Find out more
ORIGINAL RESEARCH article
Front. Microbiol. , 06 January 2023
Sec. Antimicrobials, Resistance and Chemotherapy
Volume 13 - 2022 | https://doi.org/10.3389/fmicb.2022.987164
This article is part of the Research Topic Mechanisms of Biofilm Development and Antibiofilm Strategies View all 16 articles
Background: Tannins have demonstrated antibacterial and antibiofilm activity, but there are still unknown aspects on how the chemical properties of tannins affect their biological properties. We are interested in understanding how to modulate the antibiofilm activity of tannins and in delineating the relationship between chemical determinants and antibiofilm activity.
Materials and methods: The effect of five different naturally acquired tannins and their chemical derivatives on biofilm formation and planktonic growth of Salmonella Typhimurium, Pseudomonas aeruginosa, Escherichia coli and Staphylococcus aureus was determined in the Calgary biofilm device.
Results: Most of the unmodified tannins exhibited specific antibiofilm activity against the assayed bacteria. The chemical modifications were found to alter the antibiofilm activity level and spectrum of the tannins. A positive charge introduced by derivatization with higher amounts of ammonium groups shifted the anti-biofilm spectrum toward Gram-negative bacteria, and derivatization with lower amounts of ammonium groups and acidifying derivatization shifted the spectrum toward Gram-positive bacteria. Furthermore, the quantity of phenolic OH-groups per molecule was found to have a weak impact on the anti-biofilm activity of the tannins.
Conclusion: We were able to modulate the antibiofilm activity of several tannins by specific chemical modifications, providing a first approach for fine tuning of their activity and antibacterial spectrum.
Plant-derived tannins have been used from ancient times in leather industry because of their ability of making leather last over time, and the name “tannin” comes from the use of these chemicals for “tanning” leather (Falcão and Araújo, 2011; Pizzi, 2019). Accordingly, it was hypothesized that the resistance of leather to microbial decomposition could be explained by this use of tannin-rich compounds in leather curation and several studies have pointed to multiple additional pharmacological properties of tannins, including anti-inflammatory and anti-cancer effects, which are contributing to a renewed interest in these products as a source of new bio-based pharmaceuticals (Widsten et al., 2014; Vu et al., 2017; Pizzi, 2019; Farha et al., 2020). Tannins have been shown to inhibit bacterial growth of different Gram-positive and Gram-negative bacteria (Taguri et al., 2004; Ekambaram et al., 2016; Slobodníková et al., 2016; Chandra et al., 2017; Vu et al., 2017), and are shown to be able to disperse biofilms (Trentin et al., 2013). On some occasions this antibiofilm activity is specific and independent from the ability to inhibit bacterial growth (Ulrey et al., 2014). Common examples of tannins with antibacterial activity are tannic acid (Chung et al., 1998), ellagic acid (De et al., 2018) and epigallocatechin gallate (Akiyama, 2001).
From a molecular point of view, tannins can be divided in two main groups: condensed and hydrolysable tannins. Hydrolysable tannins are esters of gallic acid with a core sugar, often glucose or quinic acid. Tannic acid is the most prominent representative of the family of hydrolysable tannins comprising a glucose center (Koleckar et al., 2008; Ekambaram et al., 2016). Condensed tannins are oligomeric and polymeric proanthocyanidins, consisting of flavan-3-ol units, linked by carbon–carbon bonds not susceptible to hydrolytic cleavage (Koleckar et al., 2008; Versari et al., 2013). The scaffold of the subclass of tannins called complex tannins is very similar to those found in condensed and hydrolysable tannins, where a flavan-3-ol unit is linked to gallic acid in a monomeric or polymeric system (Falcão and Araújo, 2011). However, there is no reference in literature that this kind of differentiation has any effect on the level and kind of antimicrobial activity of the tannins.
To date, there is no clear understanding on the factors that explain the antimicrobial activity of tannins. It has been suggested that the observable activity could be explained by the presence of free phenolic hydroxyl groups which can affect, for example, enzymatic activity via covalent or non-covalent linking (Scalbert, 1991). In this respect, it has been seen that phenolic compounds can have antimicrobial effects against Pseudomonas aeruginosa and Staphylococcus aureus (Jagani et al., 2009; Lahiri et al., 2019). This ultimately means that the typical phenolic character of the tannins could play an important role for the antimicrobial activity (Mori et al., 1987; Baba and Malik, 2015). Other mechanisms of action for the antimicrobial activity of tannins have also been described, in particular for tannic acid, like disruption of peptidoglycan formation (Dong et al., 2018), iron chelation (Chung et al., 1998), membrane disruption (Kim et al., 2010), efflux pump inhibition (Tintino et al., 2016) and fatty acid synthesis (Wu et al., 2010).
It has also been shown in previous literature that tannins have the ability to reduce biofilm formation (Jagani et al., 2009; Klug et al., 2017; Dettweiler et al., 2019; Lahiri et al., 2019). Biofilms are conglomerates of bacteria, usually at an interphase (solid-air, solid–liquid, liquid-air), that are surrounded by a protective mesh of extracellular polymeric substances (EPS). This enhances the ability of the bacteria to survive dehydration, disinfectants and antibiotics (Rabin et al., 2015; Roy et al., 2018). The mechanisms of protection include reduced penetration of antimicrobial compounds, reduced bacterial metabolism, induction of efflux pumps and more frequent horizontal gene transfer (Rabin et al., 2015).
Regarding the antibiofilm activity of tannins, it has been described that some of them have a biofilm-specific mechanism of action, such as inhibition of quorum sensing (QS) in P. aeruginosa by the tannin-rich fractions of Terminalia catappa (Taganna et al., 2011) and T. chebulata (Sarabhai et al., 2013), and induction of transglucosylase activity in S. aureus by tannic acid (Payne et al., 2013). This type of biofilm-specific behavior is actually desired, because the lack of direct growth inhibition decreases the selective pressure toward resistance phenotypes (Allen et al., 2010; Imperi et al., 2019; Dieltjens et al., 2020).
Because of this reduced potential of resistance development, in the current study we evaluated selected chemical variants of tannins for their ability to inhibit biofilm formation without inhibiting planktonic growth. A diverse range of five commercially obtained tannins, three condensed tannins and two hydrolysable tannins, were included. The tannins were selected because they have been structurally thoroughly characterized in prior works and they are representative of the known molecular diversity of tannins. Also, we investigated how different chemical modifications change the activity level and spectrum of the tannins. Understanding the effect of structural features on activity allows to enhance or finetune activity. The functional groups chosen for derivatization were selected for known capacity (i) to interfere with membranes, i.e., ammonium groups; and (ii) to alter modes of interactions with surfaces, i.e., carboxylic acids and poly(ethylene glycol). The objective was to study the consequences of such chemical derivatizations at the molecular level and at the level of intermolecular interaction possibilities and obtain hints regarding matches and mismatches between tannin-type and tannin functionalization with respect to tuning anti-biofilm activities. The scarcity of structure–activity relationship (SAR) research in the field of tannins and bioactive phenolic compounds from plant sources as antimicrobial compounds highlights the value of this work (Fang et al., 2016; Bouarab-Chibane et al., 2019).
Five commercially available tannin extracts, comprising three condensed and two hydrolysable tannins were used. The three condensed tannins were Omnivin 20R (monomeric (epi) catechin, Vv-20), Omnivin WG (procyanidins (62%)/profisetidins (34%), Vv) and Mimosa ATO ME (prorobinetidins (33%)/profisetidins (67%), Am), and the two hydrolysable tannins were Tanal 01 (tannic acid, Ta-01) and Tanal 04 (galloylquinic acid, Ta-04). The chemical structures of these tannins were elucidated in detail as reported elsewhere (Zhen et al., 2021a). Figure 1A gives an overview of the structural features.
Figure 1. (A) Chemical structure of commercially available condensed and hydrolysable tannins used in this study (40) and (B) their chemically derivatized structures as described elsewhere (41). Exemplary structural aspects are shown; synthetic route leads to generation of both primary and secondary aliphatic alcohols within the total C3 linker moiety connecting the functional to the tannin (41). (i) C3NMe3Cl-eq – hydroxy-N,N,N-trimethylpropanyl-3-aminium chloride; (ii) C3COOH-eq – hydroxypropyl-1-carboxylic acid; and (iii) PEG500-eq – oligomeric ethylene glycol polyether (PEG500-eq).
The five selected tannins were chemically modified by derivatizing them via their phenolic functionalities, as reported in detail elsewhere (Zhen et al., 2021b), with different levels of specific functional motifs: (i) hydroxy-N,N,N-trimethylpropanyl-3-aminium chloride (C3NMe3Cl-eq), (ii) hydroxypropyl-1-carboxylic acid (C3COOH-eq), and (iii) oligomeric ethylene glycol polyether (PEG500-eq), whereby “eq.” in the compounds listed in Figure 1B indicates the equivalents of the functional motif that were used for the chemical modification (Zhen et al., 2021b). These chemical functionalizations gave several properties to the tannins: (i) C3NMe3Cl-eq added positive charges to the tannin molecule, (ii) C3COOH-eq, as a weak acid, potentially added negative charges to the molecule, and (iii) PEG500-eq polymerized the tannin molecules. During the various functionalizations, control tannins were re-isolated from blank reactions. Tannins labeled as “Blank-W” are tannins isolated from blank reactions performed in water and tannins labeled as “Blank-D” are tannins isolated from blank reaction preformed in dimethylformamide.
Because of the low solubility of the tannins in aqueous media, the dry compounds were first suspended in dimethyl sulfoxide (DMSO) at a stock concentration of 60 g/L and from there diluted to the desired concentration in the following experiments. A 1% v/v concentration of DMSO was never exceeded in order to prevent potential effects of DMSO on bacterial growth or biofilm formation.
All the tests were done in aerobic conditions in growth media with a pH of ~7.4 and a salinity range of 0.025–0.5% w/v.
The antibiofilm activities of the tannins were determined using 96-well plates with a cover lid with pegs for growing the bacterial biofilms in presence of several concentration of the assayed tannins. These assays, divided in a 1st exploratory screening (Section “Antibiofilm screening”) and a 2nd validation experiment (Section “Validation anti-biofilm screening: Experimental design and statistical analysis”), are explained in detail in the following section.
Biofilms of Salmonella enterica var. Typhimurium ATCC14028, Pseudomonas aeruginosa PA14, Escherichia coli TG1 and Staphylococcus aureus SH1000 were grown in the Calgary biofilm device via a protocol that was previously described (Janssens et al., 2008; Steenackers et al., 2011). The bacteria were grown overnight (ON) in LB broth at 37°C. These ON cultures were then diluted 1/100 in diluted (1/20) Tryptic Soy Broth (TSB, Thermo Fisher Scientific) for Gram-negative bacteria and in undiluted TSB for S. aureus. 100 μl of growth medium or a solution of the tannins in growth medium was added to the wells of a 96-well Calgary device (Nunc nos. 269789 and 445497, Thermo Fisher Scientific). The diluted ON cultures were then added to the wells to obtain a starting inoculum of 106 cfu/ml in a final volume of 200 μl of growth medium per well. Also, both for the exploratory screening test and validation of anti-biofilm activity, one row of the plate was filled with inoculum with growth media without tannin and another row was filled with media without bacteria. To account for potential effects of the compounds themselves, the same tannin-concentration was added to separate control 96-well Calgary biofilm plates without the inoculation of the bacteria. Afterwards, all plates were incubated in a wet chamber for the appropriate time and temperature: 48 h at 37°C for S. aureus, 24 h at 25°C for the Gram-negatives.
Biofilm formation and planktonic growth were determined by crystal violet staining of the pegs and OD600 measurements of the base plate of the device, respectively. Specifically, after incubation the covers of the plates (which contain the pegs) were removed and washed once with PBS. The pegs were then stained with 200 μl per well of 0.1% v/v of crystal violet (CV, VWR International) for 30 min. After staining, the excess of CV was washed once with 200 μl per well of distilled water and let dry for 30 min. Finally, the CV was recovered in a new 96-well plate with 200 μl per well of 30% v/v glacial acetic and the optical density at 570 nm (OD570) measured in a plate reader. The optical density at 600 nm (OD600) of the bacteria in the base plates was measured to determine the growth of the planktonic cells.
In a preliminary experiment, the tannins were tested in two-fold dilution series ranging from 600 to 9.38 mg/L in 3 independent replicates. The obtained raw OD570 (for the biofilm formation) and OD600 (for the planktonic growth) were corrected by the OD of the tannins incubated in absence of bacterial inoculum and then normalized using the average OD of the bacterial inoculum incubated in absence of tannin, thus being converted to percentage of biofilm formation (OD570) and percentage of bacterial growth (OD600).
The BIC50 and IC50 (the compound concentration required to inhibit the biofilm formation and the bacterial growth by 50%, respectively) were calculated by applying a log [tannin] vs. percentage of biofilm formation or percentage of planktonic growth non-linear regression (four-parameters) using the statistical package GraphPad 8.0.
Based on the information from the preliminary experiment, a definitive antibiofilm experiment in the Calgary biofilm device was set up with eight independent repeats. We first defined the factors under study, i.e., the parameters that potentially have an influence on the formation of biofilm. The considered parameters are: (i) original unmodified tannin: Vv-20, Vv, Am, Ta-01, Ta-04; (ii) concentration of tannin (mg/L): 9.38, 79.69, 150; (iii) chemical modifier: C3COOH, PEG, C3NMe3Cl, Blank-D (blank reaction with dimethylformamide), Blank-W (blank reaction with water), unmodified; and (iv) concentration of applied chemical modifier: low and high (low: 0.05 and 0.1 eq of chemical substitution; high: 0.25 and 0.5 eq of chemical substitution). In order to minimize effects of plate-to-plate variation, we applied an optimal randomized experimental design with the statistical package JMP 15.0 to reduce experimental noise and confounding factors. Different to the preliminary experiment, only three concentrations were assayed, which were chosen to best capture the antibiofilm activity of the tannins: 9.38, 79.69, and 150 mg/L. The remaining part of the protocol of this experiment is identical to that of the preliminary experiment described above.
To determine the effect of the chemical derivatizations on the antibiofilm and antibacterial effect of the unmodified tannins on each of the assayed bacteria, an ANOVA test with Tukey post-hoc test comparing the unmodified tannins with their respective derivatized tannins was done based on the obtained biofilm formation and planktonic growth levels.
To determine the effect of phenolic hydroxyl (OH) content on the antimicrobial and antibiofilm effect of the different tannins, a simple linear regression between the phenolic OH content of the tannins and the level of biofilm formation (or planktonic growth) was performed. To better calculate this correlation, we used the different tannin assayed concentrations and the obtained mmol of phenolic OH per gram of material to calculate the mmol of phenolic OH present in the system for each tannin at each assayed concentration, and we correlated this value with the respective percentage of biofilm inhibition and planktonic growth inhibition. The phenolic OH content of the tannins was determined via 31PNMR as described elsewhere (Zhen et al., 2021a).
A diverse range of five commercially obtained tannins, and five types of derivatives, were selected and screened for anti-biofilm activity. The condensed tannins were previously identified as mixtures of (epi) catechins and fisetinidols (Zhen et al., 2021a) and consisted of (i) the essentially monomeric Vv-20, (ii) the low oligomeric Vv and (iii) the higher oligomeric Am. The hydrolysable tannins consisted of two large tannins: (i) the “typical” tannic acid Ta-01 and (ii) the galloquinic acid derivative Ta-04. All tannins were previously functionalized with a positive charge introducing ammonium salt hydroxy-N,N,N-trimethylpropanyl-3-aminium chloride (C3NMe3Cl-0.1 and C3NMe3Cl-0.5), an acidifying hydroxypropyl-1-carboxylic acid (C3COOH-0.1 and C3COOH-0.1-0.5) motive and a polymerizing oligomeric ethylene glycol polyether (PEG500-0.05; Figure 1). The preventive activity of both the natural and derivatized tannins against the biofilm formation and planktonic growth of the Gram-negative species S. Typhimurium, P. aeruginosa, E. coli and the Gram-positive species S. aureus was evaluated by means of the Calgary biofilm device. In a first set of exploratory experiments, two-fold serial dilutions (from 600 till 4.96 mg/L) of the tannins were evaluated in order to obtain a first glance on activity spectrum and active concentration range. Activities against all four bacterial species were observed, with BIC50 values ranging from 4.69 to 545.8 mg/L and IC50 values ranging from 37.5 to 459.2 mg/L (Supplementary Table S1). As such this experiment allowed to determine the test concentrations for future validation experiments: 9.38 mg/L was the lowest assayed concentration in the preliminary screening and the most active tannins exhibited BIC50 equal to or below that value; 150 mg/L was the concentration at which almost all tannins with antibiofilm effect were active; and 79.69 mg/L is the average of those two concentrations. Such validation experiments were required because the exploratory experiments only had three independent repeats and this did not provide sufficient statistical power to distinguish the levels of activity of the different tannins and delineate the relationship between the chemistry and the antibiofilm level of the tannins. Furthermore, there was a statistically significant plate-to-plate variation between the controls of each plate (see Supplementary Figures S1, S2).
To allow a multivariate analysis considering tannin scaffold, derivatization and concentration as well as bacterial target species, the previous antibiofilm and antimicrobial experiments were repeated in one experiment with eight repeats per condition, but only focusing on the three tannin concentrations that could capture best the antibiofilm effect of the tannins: 9.38, 79.69, and 150 mg/L. In order to minimize previously observed effects of plate-to-plate variation, these experiments were designed in a randomized way, i.e., all the tannins were distributed through all the plates in a random fashion. This allowed to decrease the random error and the possibility of confounding factors, a necessary step for doing a complex statistical analysis that allows to link the different chemical characteristics. In what follows we will first focus on the unmodified tannins, after which we will elaborate on the effect of chemical derivatization.
To determine the percentual inhibition of biofilm formation and planktonic growth of the assayed tannins against the selected bacteria, the bacterial growth and biofilm development in absence of tannin was taken as reference. As can be seen in Supplementary Figure S3, the assayed bacteria exhibited differences in their biofilm formation and bacterial growth, Staphylococcus aureus being the bacterium that reached the highest planktonic population density and formed most biofilm. On the contrary, the assayed Gram-negative bacteria showed less biofilm and less planktonic growth in comparison with S. aureus.
In Figure 2 it can be seen that the commercially available natural tannins showed different antibiofilm activities against the four assayed bacterial species. All unmodified tannins can be considered to have “broad spectrum activity,” since all of them exhibited statistically significant antibiofilm activity against Gram-positive and Gram-negative bacteria at least at the concentration of 150 mg/L, according to an ANOVA test with Tukey post-hoc analysis. Also, most of the assayed tannins exhibited a concentration-dependent activity, depending on the assayed tannin and the tested bacteria.
Figure 2. Biofilm formation and planktonic growth of the assayed bacteria (expressed as percentage in comparison to control) in the presence of 9.38, 79.69, and 150 mg/L of unmodified tannins. The letters in the biofilm formation graphs indicate groups of tannin-concentration combinations whose effects are significantly different from the control but not significantly different to each other; the bars with no letter are those tannin-concentration combinations which are not significantly different from the control. Asterixis in the planktonic growth graphs indicate a significant difference from control, * = p < 0.05; ** = p < 0.01. The statistical differences were determined via ANOVA test with Tukey post-hoc analysis, with a p-value of 0.05.
However, there were clear differences in the degree of antibiofilm activity of each tannin. Starting from the condensed tannins, the monomeric Vv-20 exhibited significant antibiofilm activity against all the assayed bacteria at all the assayed concentrations in a clear dose-dependent way, a dose of 79.69 mg/L being sufficient for inhibiting biofilm formation more than 50% against the four assayed bacteria. Also, Vv-20 was the most effective tannin against S. Typhimurium, with more than 75% of biofilm inhibition at 79.69 and 150 mg/L, and against E. coli, with more than 80% biofilm inhibition at 79.69 mg/L. Regarding the low oligomeric Vv, the antibiofilm activity was preferential against P. aeruginosa and S. aureus, for which it exhibited potent dose-dependent antibiofilm activity. This compound was able to inhibit more than 50% of biofilm formation by S. aureus both at 79.69 and 150 mg/L, and is the unmodified tannin with highest effect against P. aeruginosa, displaying antibiofilm activity of more than 90% at 79.69 mg/L and more than 95% at 150 mg/L. On the contrary, Vv was less effective against S. Typhimurium and E. coli and was able to inhibit <30% of biofilm formation of both bacterial species, regardless of the assayed concentration. Contrary to the previous two unmodified condensed tannins, the high oligomeric Am had preferential antibiofilm activity against S. Typhimurium and S. aureus, but also had significant antibiofilm activity against P. aeruginosa. Moreover, Am is the unmodified tannin with the highest antibiofilm activity against S. aureus, with more than 85% of antibiofilm activity at 79.69 and 150 mg/L, also inhibiting more than 50% of biofilm formation of S. Typhimurium both at 79.69 and 150 mg/L, and inhibiting P. aeruginosa biofilm formation in more than 30% at 79.69 mg/L and more than 50% at 150 mg/L. On the contrary, Am had unnoticeable inhibitory activity against E. coli biofilms at any of the assayed concentrations.
With respect to the hydrolysable tannins, tannic acid, Ta-01 exhibited preferential activity against P. aeruginosa and S. Typhimurium. The biofilm inhibitory activity of Ta-01 ranged from 40% at 9.38 mg/L to 60% at 150 mg/L against S. Typhimurium, and from 15% at 9.38 mg/L to more than 90% at 150 mg/L against P. aeruginosa. On the contrary, the galloquinic acid derivative, Ta-04 exhibited preferential activity against S. aureus. While the antibiofilm activity of Ta-04 against S. Typhimurium and E. coli was dose dependent (reaching a maximum of 50% biofilm inhibition against both bacteria at a concentration of 150 mg/L), the antibiofilm activity of Ta-04 against S. aureus was not dose dependent, with more than 70% biofilm inhibition at the three assayed concentrations.
Importantly, the unmodified tannins were in general not found to have antibacterial activity against the planktonic bacteria, except for the low oligomeric condensed Vv against S. Typhimurium and the hydrolysable tannic acid Ta-01 against E. coli at the highest concentration of compound (Figure 2). In previous reports, it was found that hydrolysable tannins similar to galloylquinic acid, hence similar to Ta-04, exhibit broad spectrum antibiofilm activity (Widsten et al., 2014; Ekambaram et al., 2016; Puljula et al., 2020). Those same reports, however, also suggest that hydrolysable tannins have antibacterial effects against planktonic Gram positive and Gram bacteria, which was not observed in our experiment. On the other hand, tannic acid (Lee et al., 2013) and 1,2,3,4,6-penta-O-galloyl-β-D-glucopyranose (Lin et al., 2011), both hydrolysable tannins, have been reported to inhibit biofilm formation of S. aureus without inhibiting planktonic growth, which is consistent with the results observed for tannic acid Ta-01. In our assay, also galloylquinic acid Ta-04 showed such activity. This selective activity against biofilms offers opportunities for potential applications, such as the titanium-tannin composite coating for implants developed by Shukla and Bhathena (2015), that allows sustained release of the tannin.
The aim of the modifications was to partially functionalize via ether linkages the phenolic OH-groups of each tannin molecule and to add tannin-alien functionalities at various levels to test the possibility to modulate the native activity of tannins toward biofilms and planktonic bacteria.
Figure 3 shows the effect of derivatization on the antibiofilm activity. The differences in antibiofilm activity between the different modifications were assessed via the Tukey test for multiple comparisons, by comparing if there were differences in the maximum activity (i.e., the antibiofilm activity at the highest assayed concentration). If there were no differences in the maximum activity, the activity at lower concentrations was also evaluated, thus allowing to determine if a derivatization was able to obtain the same effect as the unmodified tannin, but at a lower concentration.
Figure 3. Effect of natural and chemically modified tannins on biofilm formation (expressed as percentage compared with positive control) on several bacterial species at 9.38, 79.69, and 150 mg/L of tannin. The colors indicate the percentage of biofilm formation in presence of several concentrations of the assayed tannins compared to the untreated control. The asterisks indicate significant differences with the unmodified tannin, following ANOVA test with Tukey post-hoc analysis. *: p ≤ 0.05; **: p ≤ 0.01; ***: p ≤ 0.001; ****: p ≤ 0.0001.
As a general conclusion, it could be established that most of the chemical derivatizations, but especially positive charge introducing C3NMe3Cl-0.1 reduce the antibiofilm activity of the tannins, while some of them can shift the activity spectrum toward preferential activity against Gram-positive or Gram-negative bacteria. More importantly, it must be noted that, in general, the chemical derivatizations increased the activity against planktonic bacteria and decreased the activity against bacterial biofilms of the unmodified tannins, thus decreasing the biofilm specificity of the assayed tannins (see Supplementary Figures S4–S7 for a direct comparison between growth inhibitory effect and biofilm inhibitory capability of the assayed tannins against each bacterial species). This effect will be discussed in more detail in Section “Biofilm selectivity of the antibiofilm effect of the assayed tannins.”
Two derivatizations generally shifted the antibiofilm spectrum toward the Gram-negative group of bacteria: polymerizing PEG500-0.05 and positive charge-introducing C3NMe3Cl-0.5. Contrarily, derivatization with acidifying C3COOH-0.1 and C3COOH-0.5 in general decreased the antibiofilm activity against Gram-negative bacteria, while it retained activity against S. aureus for larger tannins Am, Ta-01 and Ta-04, and increased activity against S. aureus for monomeric and low oligomeric Vv-20 and Vv. The other derivatizations in general decreased the activity against S. aureus. However, this effect of derivatizations on the spectrum and potency was highly dependent on the specific tannin submitted to the derivatization.
In more detail, it can be seen that the blank reaction (both in water -Blank-W- and in dimethylformamide -Blank-D-) already modified the antibiofilm effect of the assayed tannins, a phenomenon that could be attributed to removal of impurities that affect the antibiofilm effect of the tannins. It can be seen that Blank-W conditions increased the maximum antibiofilm effect of Vv against Salmonella Typhimurium, but decreased the maximum antibiofilm effect of Vv-20 and Vv against P. aeruginosa and decreases the antibiofilm effect of Vv-20 against E. coli at 79.69 mg/L without affecting its maximum antibiofilm effect. It can also be seen that Blank-D conditions only affected the antibiofilm activity of Ta-04, by reducing its antibiofilm effect against Gram-negative bacteria.
Regarding actual substitutions, it can be seen that the effect of a derivatization with positive charge introducing ammonium groups, i.e., C3NMe3Cl, was different depending on the equivalents of chemical substitution.
On the one hand, C3NMe3Cl-0.5, the high equivalent derivatization, decreased the maximum antibiofilm effect of condensed tannins against S. aureus but did not affect the anti-staphylococcal effect of hydrolysable tannins. However, C3NMe3Cl-0.5 derivatization had a tannin-dependent effect against Gram-negative bacteria. It did not affect the antibiofilm effect of monomeric Vv-20 against any Gram-negative bacteria but increased the maximum antibiofilm effect of low oligomeric Vv against Salmonella Typhimurium while decreasing the maximum antibiofilm effect of Vv against P. aeruginosa. It increased the effect of high oligomeric Am against all the Gram-negative bacteria at 79.69 mg/L without significantly changing the maximum effect compared to the unmodified tannin. It increased the maximum effect of tannic acid Ta-01 against E. coli and Salmonella Typhimurium but decreased the effect against P. aeruginosa at 79.69 mg/L and it significantly decreased the maximum antibiofilm effect of galloquinic acid derivative Ta-04 against Salmonella Typhimurium. On the other hand, C3NMe3Cl-0.1, the low equivalent derivatization, reduced the maximum effect of all tannins (with the exception of the galloquinic acid derivative Ta-04, which was not affected) against Gram-negative bacteria without affecting the antibiofilm effect against S. aureus.
Contrary to C3NMe3Cl, there were no big differences between different levels of derivatizations with acidifying motif CH3COOH, i.e., CH3COOH-0.1 and CH3COOH-0.5, in terms of their impact on the activities compared to the unmodified tannins. For S. aureus, neither derivatization level affected the antibiofilm effect exerted by larger tannins Am, Ta-01, and Ta-04, comparable to the C3NMe3Cl-0.1 derivatization, while CH3COOH-0.1 increased the maximum effect of low oligomeric Vv. CH3COOH-0.5 had a similar effect on essentially monomeric Vv-20. For the Gram-negative bacteria, both derivatizations equally decreased the maximum antibiofilm effect of condensed tannins Vv-20 and Am, as well as for hydrolysable tannin Ta-04 against S. Typhimurium, but only CH3COOH-0.1 significantly decreased the maximum antibiofilm effect against S. Typhimurium of Vv. While both derivatization levels drastically decreased the maximum antibiofilm effect of condensed tannins against P. aeruginosa, only CH3COOH-0.1 decreased the maximum antibiofilm effect of tannic acid Ta-01. Neither derivatization level significantly affected the antibiofilm effect of hydrolysable Ta-04. This effect was similar regarding E. coli because neither derivatization level affected the maximum antibiofilm effect of hydrolysable tannins, while CH3COOH-0.1 decreased the maximum antibiofilm effect of all condensed tannins. CH3COOH-0.5 only significantly decreased the maximum antibiofilm effect of Vv-20 against E. coli.
Regarding derivatization with polymerizing PEG500, it can be seen that PEG500-0.05 in general did not affect the antibiofilm effect of the assayed tannins. The only exceptions are the increase in the maximum antibiofilm effect of low oligomeric Vv against Salmonella Typhimurium and the decrease in the antibiofilm effect of monomeric Vv-20 against S. aureus at 79.69 mg/L without changing the maximum antibiofilm effect. It has to be taken in account that, due to technical issues, it was not possible to analyze the effect of polymerizing PEG500-0.05 derivatization on Ta-04. Polymerizing PEG500-0.05 derivatization proofed difficult to analyze in terms of loading for Ta-04 (Zhen et al., 2021b).
No literature data are yet available that would describe the effects of chemical modifications of tannins on their antibiofilm, or more generally antibacterial activity. With the aim of elucidating a hypothesis about the reason behind the effect of the derivatizations on our assayed tannins in comparison to the parent tannins and the blanks, we decided to look into other non-tannin organic compounds.
One of these examples targets the effect of the high equivalent derivatization C3NMe3Cl-0.5 in shifting the anti-biofilm spectrum toward Gram-negative bacteria, which may be associated with addition of positive charges in the form of ammonium groups to the tannin molecules. This allows for comparison of our results with data obtained by Dalcin et al. (2017), who discovered that nanoencapsulation of dihydromyricetin within the polycationic polymer Eudragit RS 100® not only increased its antibiofilm activity against P. aeruginosa, but that the polymer itself had antibiofilm activity. This finding goes in accordance with a previous publication of Campanac et al. (2002) which states that cationic quaternary ammonium compounds were more effective against P. aeruginosa than S. aureus biofilm. Also, Gao et al. (2019) showed a decreased biofilm formation in E. coli and S. aureus using positively charged nanoaggregates based on zwitterionic pillar-[5] arene, requiring a 10 times lower concentration of nanoaggregate to decrease biofilm formation in E. coli compared to S. aureus. These results suggest that equipping the tannins with positive charge-introducing ammonium groups may give preferential action against Gram-negative bacteria. However, tannins should be derivatized with enough positive charge-introducing ammonium groups to obtain this shift toward inhibition of Gram-negative bacteria since the low equivalent derivatization C3NMe3Cl-0.1 appeared to lower the activity against Gram-negative bacteria.
Regarding the effect of acidifying CH3COOH derivatizations, there is a precedent of the effect of several substitutions on the antibiofilm effect of anthraquinones against methicillin-resistant S. aureus (MRSA; Song et al., 2021). This study shows that a carboxyl group at position 2 of the anthraquinone molecule increases both the antibiofilm and the antimicrobial activity, which is partially in agreement with our data that shows that CH3COOH derivatizations increase the antibiofilm activity of low oligomeric Vv-20 and essentially monomeric Vv without affecting the bacterial growth. Relatedly, Warraich et al. (2020) found that the acidic D-amino acids D-aspartic acid and D-glutamic acid were effective in dispersing and inhibiting biofilm formation in S. aureus, and they attributed this effect to the negative charges introduced by carboxyl groups of the molecules under growth conditions.
Finally, regarding polymerizing PEG derivatizations, there are several studies about the potential of PEG cross-linked hydrogels for wound healing because of their antimicrobial, pro-angiogenesis and pro-epithelization capabilities (Chen et al., 2019), but there is no indication regarding the effect of an introduction of a PEG-motif on the antibiofilm capability of an organic compound.
In the last years, there has been increasing research on non-lethal antimicrobial targets against several bacterial species, from virulence factors to biofilm formation, including inhibition of regulatory mechanisms such as quorum sensing and production of public goods (Defoirdt et al., 2013; Defoirdt, 2016; Maura et al., 2016; Totsika, 2016). The rationale behind this research is the assumption that if bacterial viability is not affected, the selective pressure will be lower and thus the risk for emergence of antimicrobial resistance will be lower too (Kalia et al., 2014; Dieltjens et al., 2020; Hemmati et al., 2020). However, there is still discussion about the effectiveness of this “resistant-proof” approach (García-Contreras et al., 2016).
The heatmap of Figure 4 shows the anti-planktonic activity of the tannins at the assayed concentrations. We defined that the antibiofilm activity of a particular tannin was biofilm specific in case the tannin did not exhibit significant anti-planktonic effect at that concentration (Dos Santos Goncalves et al., 2014; Vijayakumar and Thirunanasambandham, 2021). Most of the tannins with antibiofilm activity did not exhibit anti-planktonic effects, indicating that they are biofilm specific. This is particularly true for unmodified tannins, of which only Vv and Ta-01 exhibited anti-planktonic activity against Salmonella Typhimurium and E. coli, respectively. Also, unmodified tannins re-isolated from blank reactions, i.e., Blank-W or Blank-D, with the exception of Blank-D against Salmonella Typhimurium, did not reduce the planktonic growth of the assayed bacteria.
Figure 4. Effect of natural and chemically modified tannins on planktonic growth (expressed as percentage compared with control) on several bacterial species at 9.38, 79.69, and 150 mg/L of tannin. The crosses (+) for PEG500-0.05 derivatization on Vv-20 and Vv indicate values below zero, which is an effect of potential overcorrection of the raw values by the negative control. The colors indicate the percentage of planktonic growth in presence of several concentrations of the assayed tannins compared to the untreated control. The asterisks indicate significant differences with the unmodified tannin, following ANOVA test with Tukey post-hoc analysis. *: p ≤ 0.05, **: p ≤ 0.01; ***: p ≤ 0.001; ****: p ≤ 0.0001.
Regarding the effect of derivatizations on the biofilm specificity of the tannins, this was both derivatization and species dependent. Derivatization with positive charge introducing C3NMe3Cl-0.1 not only generally decreased the antibiofilm activity of the assayed tannins, but also increased the anti-planktonic activity specifically against Gram-negative bacteria. Antibiofilm specificity of tannins derivatized with C3NMe3Cl-0.5 was highly dependent on both the assayed bacteria and the derivatized tannin: the antibiofilm effect was non-specific when the derivatization was applied to Vv-20, but it was biofilm-specific for Vv, Am and Ta-01. Also, tannins derivatized with C3NMe3Cl-0.5 most strongly affected the growth of P. aeruginosa except for Am-C3NMe3Cl-0.5, which did not affect the growth of P. aeruginosa at any concentration. Only a derivatization of Ta-04, and here especially with C3NMe3Cl-0.5, led to a dose-dependent anti-planktonic effect, thus exhibiting non-specific antibiofilm activity at higher concentrations. With respect to a functionalization with the acidifying element CH3COOH at various concentrations, it can be stated that these in this study did not significantly change the anti-planktonic behavior of the derivatized Vv, Am and Ta-01 against the assayed bacteria, but increased the antibacterial effect of Vv-20 and Ta-04 against planktonic bacteria. However, one notable exception are the tannins that were modified with crosslinking PEG500-0.05, whose antibiofilm activity against Gram negative bacteria was highly correlated with the ability to inhibit the planktonic growth (Figure 4).
As a general summary, tannin derivatization did not affect biofilm specificity against S. aureus but affected the biofilm specificity against Gram-negative bacteria in a tannin-specific and derivatization specific manner. More importantly, there was no correlation in the assayed tannins between the degree of inhibition of planktonic growth and the degree of antibiofilm effect, a situation that goes in accordance with some previous reports (Janecki and Kolodziej, 2010; Payne et al., 2013; Hricovíniová et al., 2021).
One of the potential consequences of the chemical derivatization of the tannins are changes in the content of free phenolic hydroxyl groups present in the chemical structure of the tannins, since functionalization occurs at these hydroxyl groups. This is potentially important, because in previous literature it has been described that the biological activity of polyphenols could be mediated by their phenolic hydroxyl groups (Ultee et al., 2002; Ito et al., 2005; Ben Arfa et al., 2006; Veldhuizen et al., 2006; Alves et al., 2013; d’Avila Farias et al., 2014; Salar et al., 2017). Particularly regarding hydrolysable tannins, Taguri linked the degree of antibacterial activity to the presence of galloyl groups (Taguri et al., 2004). Because of this, we aimed to determine if there was a significant impact of the derivatizations on the phenolic hydroxyl (OH) content of the tannins, and if the phenolic OH content affected the antibiofilm.
In a first approach, we studied the impact of derivatizations on the phenolic OH content of the tannins. As can be seen in Table 1, derivatizations with C3NMe3Cl-0.1 decreased the phenolic OH content of the unmodified tannin. This is important, since derivatization with C3NMe3Cl-0.1 significantly decreased the antibiofilm effect of tannins, mostly against Gram negative bacteria. In a similar trend, derivatizations with CH3COOH tended to decrease the phenolic content of condensed tannins: derivatization with CH3COOH decreased the antibiofilm effect against Gram negative bacteria of condensed tannins, but, interestingly, not of still larger hydrolysable tannins.
Table 1. Phenolic OH content (in mmol phenolic OH/g materials) of unmodified and derivatized tannins.
In a second approach, we hence studied potential correlations between the phenolic OH content and the antibiofilm activity and we observed a weak correlation between the phenolic OH content and the antibiofilm activity against Gram negative bacteria and, to a lesser extent, against S. aureus (see Supplementary Figure S8). These data, combined with the previously mentioned effect of the different derivatizations on the phenolic OH content of the tannins, suggest that the phenolic OH content of the tannins is more important for the antibiofilm effect against Gram negative bacteria than against S. aureus. However, we did not observe a correlation between the antibacterial activity against planktonic bacteria and the phenolic OH content of the assayed tannins (see Supplementary Figure S9), which is in accordance with the study Kim et al. (2020), who did not find a significant correlation between the total phenolic content of several plant extracts from Chinese traditional medicine and the antimicrobial activity against S. aureus. However, this contradicts a previous study from Vattem et al. (2004), who found a linear correlation between the phenolic content of cranberry pomace and the antimicrobial activity against Listeria monocytogenes, Vibrio parahaemolyticus and E. coli.
It is important to note that the previous studies are focused on the antibacterial effect against planktonic bacteria, since there are no previous studies pointing to the effect of the hydroxyl or phenolic content on the capability of tannins to inhibit biofilms. However, research on other classes of polyphenolic compounds showed that the quantity and the position of the hydroxyl groups affects the capabilities of polyphenolic compounds to disperse biofilm, which could explain the results obtained in this research with the assayed tannins. In more detail, the research of Cho et al. on the capability of flavonoids to disperse S. aureus biofilms showed that there is a direct correlation between the quantity of hydroxyl substitutions of the flavonoid molecules and their antibiofilm capability (Cho et al., 2015), and the research of Kim et al. with phorbaketals against S. aureus biofilms indicated that the position and quantity of hydroxyls substitutions in the molecule affects their antibiofilm capacity (Kim et al., 2021), which is also consistent with the results of Arshia et al. (2017).
Our work provides a clear understanding on which chemical modifications can be made to enhance the activity level or change the activity spectrum of natural tannins, and which chemical modifications are inconvenient for increasing their antibiofilm activity. This is one of the few studies that uses a systematic and statistical analysis to correlate specific chemical characteristics of modified tannins with their antibiofilm activity (Ge et al., 2003; Peeters et al., 2016; Kemegne et al., 2017; Bouarab-Chibane et al., 2019; De Paiva et al., 2019; Jia et al., 2019).
From our work, it can be concluded that tannins not only have good activity against biofilms of different bacterial species, but that this antibiofilm activity is in most cases also biofilm specific. More important, we could identify that modifying the tannins with C3NMe3Cl-0.5 and PEG500-0.05 can increase the antibiofilm activity against Gram-negative bacteria, although this often coincides with a decrease in activity against Gram-positive bacteria. Modifying the tannins with C3NMe3Cl-0.1, CH3COOH-0.1 and CH3COOH-0.5 generally decreases the effect against Gram-negatives, without affecting the activity against S. aureus. We can thus modulate the spectrum and the antibiofilm potency of tannins by the applied chemical modifications.
We could identify a weak correlation between the antibiofilm effect and the content of phenolic hydroxyl groups for Gram-negative bacteria and, to a lesser extent, for S. aureus. However, exploring the mode of action against other bacteria is a necessary and interesting avenue to explore further based on the initial insights generated in this work, pointing to a more complex interplay between functionalization, type of tannin in the sense of exposed galloyl units, and tannin size. A continued exploration of the possible mechanisms of actions of these compounds and the possible modifications that can be made to enhance their effect is necessary to better optimize the antibiofilm potential of the tannins.
The original contributions presented in the study are included in the article/Supplementary material, further inquiries can be directed to the corresponding author.
XV performed the experimental biological work, data analysis, and article writing. LZ performed the chemical manipulations and provided the chemical analysis of the assayed tannins. JA developed the experimental design and contributed to the statistical analysis. TV contributed to the article writing and data analysis. HL was part of the coordination of the research project, and contributed to the results analysis and the writing of the text. CC contributed to the research project funding and coordination. HS contributed to project funding, project coordination, article writing, and data analysis. All authors contributed to the article and approved the submitted version.
This work was supported by H2020-MSCA-ITN-2016-BIOCLEAN project (grant agreement no. 722871). HL would like to additionally thank the MIUR for the Grant “Dipartimento di Eccellenza 2018-2022” to the Department of Earth and Environmental Sciences of the University of Milano-Bicocca. CC acknowledges the Ca’Foscari FPI 2019 funding.
We would like to thank A.S. Ajinomoto OmniChem N.V. in Belgium for generously providing samples of commercialized tannins. We especially like to thank David De Coster for his technical support during the experimental work.
The authors declare that the research was conducted in the absence of any commercial or financial relationships that could be construed as a potential conflict of interest.
All claims expressed in this article are solely those of the authors and do not necessarily represent those of their affiliated organizations, or those of the publisher, the editors and the reviewers. Any product that may be evaluated in this article, or claim that may be made by its manufacturer, is not guaranteed or endorsed by the publisher.
The Supplementary material for this article can be found online at: https://www.frontiersin.org/articles/10.3389/fmicb.2022.987164/full#supplementary-material
Akiyama, H. (2001). Antibacterial action of several tannins against Staphylococcus aureus. J. Antimicrob. Chemother. 48, 487–491. doi: 10.1093/jac/48.4.487
Allen, H. K., Donato, J., Wang, H. H., Cloud-Hansen, K. A., Davies, J., and Handelsman, J. (2010). Call of the wild: antibiotic resistance genes in natural environments. Nat. Rev. Microbiol. 8, 251–259. doi: 10.1038/nrmicro2312
Alves, M. J., Ferreira, I. C. F. R., Froufe, H. J. C., Abreu, R. M. V., Martins, A., and Pintado, M. (2013). Antimicrobial activity of phenolic compounds identified in wild mushrooms, SAR analysis and docking studies. J. Appl. Microbiol. 115, 346–357. doi: 10.1111/jam.12196
Arshia, K. A. K., Khan, K. M., Ahmed, A., Taha, M., and Perveen, S. (2017). Antibiofilm potential of synthetic 2-amino-5-chlorobenzophenone Schiff bases and its confirmation through fluorescence microscopy. Microb. Pathog. 110, 497–506. doi: 10.1016/j.micpath.2017.07.040
Baba, S. A., and Malik, S. A. (2015). Determination of total phenolic and flavonoid content, antimicrobial and antioxidant activity of a root extract of Arisaema jacquemontii Blume. J. Taibah Univ. Sci. 9, 449–454. doi: 10.1016/j.jtusci.2014.11.001
Ben Arfa, A., Combes, S., Preziosi-Belloy, L., Gontard, N., and Chalier, P. (2006). Antimicrobial activity of carvacrol related to its chemical structure. Lett. Appl. Microbiol. 43, 149–154. doi: 10.1111/j.1472-765X.2006.01938.x
Bouarab-Chibane, L., Forquet, V., Lantéri, P., Clément, Y., Léonard-Akkari, L., Oulahal, N., et al. (2019). Antibacterial properties of polyphenols: characterization and QSAR (quantitative structure–activity relationship) models. Front. Microbiol. 10:829. doi: 10.3389/fmicb.2019.00829
Campanac, C., Pineau, L., Payard, A., Baziard-Mouysset, G., and Roques, C. (2002). Interactions between biocide cationic agents and bacterial biofilms. Antimicrob. Agents Chemother. 46, 1469–1474. doi: 10.1128/AAC.46.5.1469-1474.2002
Chandra, H., Bishnoi, P., Yadav, A., Patni, B., Mishra, A., and Nautiyal, A. (2017). Antimicrobial resistance and the alternative resources with special emphasis on plant-based antimicrobials—a review. Plan. Theory 6:16. doi: 10.3390/plants6020016
Chen, H., Cheng, R., Zhao, X., Zhang, Y., Tam, A., Yan, Y., et al. (2019). An injectable self-healing coordinative hydrogel with antibacterial and angiogenic properties for diabetic skin wound repair. NPG Asia Mater 11, 3. doi: 10.1038/s41427-018-0103-9
Cho, H. S., Lee, J.-H., Cho, M. H., and Lee, J. (2015). Red wines and flavonoids diminish Staphylococcus aureus virulence with anti-biofilm and anti-hemolytic activities. Biofouling 31, 1–11. doi: 10.1080/08927014.2014.991319
Chung, K.-T., Lu, Z., and Chou, M. (1998). Mechanism of inhibition of tannic acid and related compounds on the growth of intestinal bacteria. Food Chem. Toxicol. 36, 1053–1060. doi: 10.1016/S0278-6915(98)00086-6
d’Avila Farias, M., Oliveira, P. S., Dutra, F. S. P., Fernandes, T. J., De Pereira, C. M. P., De Oliveira, S. Q., et al. (2014). Eugenol derivatives as potential anti-oxidants: is phenolic hydroxyl necessary to obtain an effect? J. Pharm. Pharmacol. 66, 733–746. doi: 10.1111/jphp.12197
Dalcin, A. J. F., Santos, C. G., Gündel, S. S., Roggia, I., Raffin, R. P., Ourique, A. F., et al. (2017). Anti biofilm effect of dihydromyricetin-loaded nanocapsules on urinary catheter infected by Pseudomonas aeruginosa. Colloids Surf. B Biointerfaces 156, 282–291. doi: 10.1016/j.colsurfb.2017.05.029
De Paiva, R. K. C., Da Silva, J. F., Moreira, H. A., Pinto, O. G., Camargo, L. T. F. M., Naves, P. L. F., et al. (2019). Synthesis, antimicrobial activity and structure-activity relationship of some 5-Arylidene-thiazolidine-2, 4-dione derivatives. J. Braz. Chem. Soc. 30, 164–172. doi: 10.21577/0103-5053.20180167
De, R., Sarkar, A., Ghosh, P., Ganguly, M., Karmakar, B. C., Saha, D. R., et al. (2018). Antimicrobial activity of ellagic acid against helicobacter pylori isolates from India and during infections in mice. J. Antimicrob. Chemother. 73, 1595–1603. doi: 10.1093/jac/dky079
Defoirdt, T. (2016). Specific Antivirulence activity, a new concept for reliable screening of virulence inhibitors. Trends Biotechnol. 34, 527–529. doi: 10.1016/j.tibtech.2016.01.009
Defoirdt, T., Brackman, G., and Coenye, T. (2013). Quorum sensing inhibitors: how strong is the evidence? Trends Microbiol. 21, 619–624. doi: 10.1016/j.tim.2013.09.006
Dettweiler, M., Lyles, J. T., Nelson, K., Dale, B., Reddinger, R. M., Zurawski, D. V., et al. (2019). American civil war plant medicines inhibit growth, biofilm formation, and quorum sensing by multidrug-resistant bacteria. Sci. Rep. 9:7692. doi: 10.1038/s41598-019-44242-y
Dieltjens, L., Appermans, K., Lissens, M., Lories, B., Kim, W., Van der Eycken, E. V., et al. (2020). Inhibiting bacterial cooperation is an evolutionarily robust anti-biofilm strategy. Nat. Commun. 11:107. doi: 10.1038/s41467-019-13660-x
Dong, G., Liu, H., Yu, X., Zhang, X., Lu, H., Zhou, T., et al. (2018). Antimicrobial and anti-biofilm activity of tannic acid against Staphylococcus aureus. Nat. Prod. Res. 32, 2225–2228. doi: 10.1080/14786419.2017.1366485
Dos Santos Goncalves, M., Delattre, C., Balestrino, D., Charbonnel, N., Elboutachfaiti, R., Wadouachi, A., et al. (2014). Anti-biofilm activity: a function of Klebsiella pneumoniae capsular polysaccharide. PLoS One 9:e99995. doi: 10.1371/journal.pone.0099995
Ekambaram, S. P., Perumal, S. S., and Balakrishnan, A. (2016). Scope of hydrolysable tannins as possible antimicrobial agent. Phyther. Res. 30, 1035–1045. doi: 10.1002/ptr.5616
Falcão, L., and Araújo, M. E. M. (2011). Tannins characterisation in new and historic vegetable tanned leathers fibres by spot tests. J. Cult. Herit. 12, 149–156. doi: 10.1016/j.culher.2010.10.005
Fang, Y., Lu, Y., Zang, X., Wu, T., Qi, X., Pan, S., et al. (2016). 3D-QSAR and docking studies of flavonoids as potent Escherichia coli inhibitors. Sci. Rep. 6:23634. doi: 10.1038/srep23634
Farha, A. K., Yang, Q.-Q., Kim, G., Li, H.-B., Zhu, F., Liu, H.-Y., et al. (2020). Tannins as an alternative to antibiotics. Food Biosci. 38:100751. doi: 10.1016/j.fbio.2020.100751
Gao, L., Li, M., Ehrmann, S., Tu, Z., and Haag, R. (2019). Positively charged nanoaggregates based on Zwitterionic pillar[5]arene that combat planktonic bacteria and disrupt biofilms. Angew. Chemie – Int. Ed. 58, 3645–3649. doi: 10.1002/anie.201810314
García-Contreras, R., Maeda, T., and Wood, T. K. (2016). Can resistance against quorum-sensing interference be selected? ISME J. 10, 4–10. doi: 10.1038/ismej.2015.84
Ge, J., Shi, X., Cai, M., Wu, R., and Wang, M. (2003). A novel biodegradable antimicrobial PU foam from wattle tannin. J. Appl. Polym. Sci. 90, 2756–2763. doi: 10.1002/app.12928
Hemmati, F., Salehi, R., Ghotaslou, R., Samadi Kafil, H., Hasani, A., Gholizadeh, P., et al. (2020). Quorum quenching: a potential target for antipseudomonal therapy. Infect. Drug Resist. 13, 2989–3005. doi: 10.2147/IDR.S263196
Hricovíniová, Z., Mascaretti, Š., Hricovíniová, J., Čížek, A., and Jampílek, J. (2021). New unnatural Gallotannins: a way toward green antioxidants, antimicrobials and antibiofilm agents. Antioxidants 10:1288. doi: 10.3390/antiox10081288
Imperi, F., Fiscarelli, E. V., Visaggio, D., Leoni, L., and Visca, P. (2019). Activity and impact on resistance development of two antivirulence fluoropyrimidine drugs in Pseudomonas aeruginosa. Front. Cell. Infect. Microbiol. 9, 1–11. doi: 10.3389/fcimb.2019.00049
Ito, M., Murakami, K., and Yoshino, M. (2005). Antioxidant action of eugenol compounds: role of metal ion in the inhibition of lipid peroxidation. Food Chem. Toxicol. 43, 461–466. doi: 10.1016/j.fct.2004.11.019
Jagani, S., Chelikani, R., and Kim, D. S. (2009). Effects of phenol and natural phenolic compounds on biofilm formation by Pseudomonas aeruginosa. Biofouling 25, 321–324. doi: 10.1080/08927010802660854
Janecki, A., and Kolodziej, H. (2010). Anti-adhesive activities of Flavan-3-ols and Proanthocyanidins in the interaction of group A-streptococci and human epithelial cells. Molecules 15, 7139–7152. doi: 10.3390/molecules15107139
Janssens, J. C. A., Steenackers, H., Robijns, S., Gellens, E., Levin, J., Zhao, H., et al. (2008). Brominated furanones inhibit biofilm formation by salmonella enterica serovar typhimurium. Appl. Environ. Microbiol. 74, 6639–6648. doi: 10.1128/AEM.01262-08
Jia, B., Ma, Y. M., Liu, B., Chen, P., Hu, Y., and Zhang, R. (2019). Synthesis, antimicrobial activity, structure-activity relationship, and molecular docking studies of indole Diketopiperazine alkaloids. Front. Chem. 7, 1–13. doi: 10.3389/fchem.2019.00837
Kalia, V. C., Wood, T. K., and Kumar, P. (2014). Evolution of resistance to quorum-sensing inhibitors. Microb. Ecol. 68, 13–23. doi: 10.1007/s00248-013-0316-y
Kemegne, G. A., Mkounga, P., Essia Ngang, J. J., Sado Kamdem, S. L., and Nkengfack, A. E. (2017). Antimicrobial structure activity relationship of five anthraquinones of emodine type isolated from Vismia laurentii. BMC Microbiol. 17, 41–48. doi: 10.1186/s12866-017-0954-1
Kim, G., Gan, R.-Y., Zhang, D., Farha, A. K., Habimana, O., Mavumengwana, V., et al. (2020). Large-scale screening of 239 traditional Chinese medicinal plant extracts for their antibacterial activities against multidrug-resistant Staphylococcus aureus and cytotoxic activities. Pathogens 9:185. doi: 10.3390/pathogens9030185
Kim, J.-K., Kim, N., and Lim, Y.-H. (2010). Evaluation of the antibacterial activity of Rhapontigenin produced from Rhapontin by biotransformation against Propionibacterium acnes. J. Microbiol. Biotechnol. 20, 82–87. doi: 10.4014/jmb.0907.07022
Kim, Y.-G., Lee, J.-H., Lee, S., Lee, Y.-K., Hwang, B. S., and Lee, J. (2021). Antibiofilm activity of Phorbaketals from the marine sponge Phorbas sp. against Staphylococcus aureus. Mar. Drugs 19:301. doi: 10.3390/md19060301
Klug, T. V., Novello, J., Laranja, D. C., Aguirre, T. A. S., de Oliveira Rios, A., Tondo, E. C., et al. (2017). Effect of tannin extracts on biofilms and attachment of Escherichia coli on lettuce leaves. Food Bioprocess Technol. 10, 275–283. doi: 10.1007/s11947-016-1812-0
Koleckar, V., Kubikova, K., Rehakova, Z., Kuca, K., Jun, D., Jahodar, L., et al. (2008). Condensed and hydrolysable tannins as antioxidants influencing the health. Mini-Reviews Med. Chem. 8, 436–447. doi: 10.2174/138955708784223486
Lahiri, D., Dash, S., Dutta, R., and Nag, M. (2019). Elucidating the effect of anti-biofilm activity of bioactive compounds extracted from plants. J. Biosci. 44:52. doi: 10.1007/s12038-019-9868-4
Lee, J.-H., Park, J.-H., Cho, H. S., Joo, S. W., Cho, M. H., and Lee, J. (2013). Anti-biofilm activities of quercetin and tannic acid against Staphylococcus aureus. Biofouling 29, 491–499. doi: 10.1080/08927014.2013.788692
Lin, M. H., Chang, F. R., Hua, M. Y., Wu, Y. C., and Liu, S. T. (2011). Inhibitory effects of 1,2,3,4,6-penta-O-galloyl-β-D-glucopyranose on biofilm formation by Staphylococcus aureus. Antimicrob. Agents Chemother. 55, 1021–1027. doi: 10.1128/AAC.00843-10
Maura, D., Ballok, A. E., and Rahme, L. G. (2016). Considerations and caveats in anti-virulence drug development. Curr. Opin. Microbiol. 33, 41–46. doi: 10.1016/j.mib.2016.06.001
Mori, A., Nishino, C., Enoki, N., and Tawata, S. (1987). Antibacterial activity and mode of action of plant flavonoids against Proteus vulgaris and Staphylococcus aureus. Phytochemistry 26, 2231–2234. doi: 10.1016/S0031-9422(00)84689-0
Payne, D. E., Martin, N. R., Parzych, K. R., Rickard, A. H., Underwood, A., and Boles, B. R. (2013). Tannic acid inhibits Staphylococcus aureus surface colonization in an IsaA-dependent manner. Infect. Immun. 81, 496–504. doi: 10.1128/IAI.00877-12
Peeters, E., Hooyberghs, G., Robijns, S., Waldrant, K., De Weerdt, A., Delattin, N., et al. (2016). Modulation of the substitution pattern of 5-Aryl-2-Aminoimidazoles allows fine-tuning of their Antibiofilm activity Spectrum and toxicity. Antimicrob. Agents Chemother. 60, 6483–6497. doi: 10.1128/AAC.00035-16
Pizzi, A. (2019). Tannins: prospectives and actual industrial applications. Biomol. Ther. 9:344. doi: 10.3390/biom9080344
Puljula, E., Walton, G., Woodward, M. J., and Karonen, M. (2020). Antimicrobial activities of Ellagitannins against Clostridiales perfringens, Escherichia coli, Lactobacillus plantarum and Staphylococcus aureus. Molecules 25:3714. doi: 10.3390/molecules25163714
Rabin, N., Zheng, Y., Opoku-Temeng, C., Du, Y., Bonsu, E., and Sintim, H. O. (2015). Biofilm formation mechanisms and targets for developing antibiofilm agents. Future Med. Chem. 7, 493–512. doi: 10.4155/fmc.15.6
Roy, R., Tiwari, M., Donelli, G., and Tiwari, V. (2018). Strategies for combating bacterial biofilms: a focus on anti-biofilm agents and their mechanisms of action. Virulence 9, 522–554. doi: 10.1080/21505594.2017.1313372
Salar, R. K., Purewal, S. S., and Sandhu, K. S. (2017). Relationships between DNA damage protection activity, total phenolic content, condensed tannin content and antioxidant potential among Indian barley cultivars. Biocatal. Agric. Biotechnol. 11, 201–206. doi: 10.1016/j.bcab.2017.07.006
Sarabhai, S., Sharma, P., and Capalash, N. (2013). Ellagic acid derivatives from Terminalia chebula Retz. Downregulate the expression of quorum sensing genes to attenuate Pseudomonas aeruginosa PAO1 virulence. PLoS One 8:e53441. doi: 10.1371/journal.pone.0053441
Scalbert, A. (1991). Antimicrobial properties of tannins. Phytochemistry 30, 3875–3883. doi: 10.1016/0031-9422(91)83426-L
Shukla, V., and Bhathena, Z. (2015). Sustained release of a purified tannin component of Terminalia chebula from a titanium implant surface prevents biofilm formation by Staphylococcus aureus. Appl. Biochem. Biotechnol. 175, 3542–3556. doi: 10.1007/s12010-015-1525-2
Slobodníková, L., Fialová, S., Rendeková, K., Kováč, J., and Mučaji, P. (2016). Antibiofilm activity of plant polyphenols. Molecules 21:1717. doi: 10.3390/molecules21121717
Song, Z.-M., Zhang, J.-L., Zhou, K., Yue, L.-M., Zhang, Y., Wang, C.-Y., et al. (2021). Anthraquinones as potential Antibiofilm agents against methicillin-resistant Staphylococcus aureus. Front. Microbiol. 12, 1–16. doi: 10.3389/fmicb.2021.709826
Steenackers, H. P. L., Ermolatev, D. S., Savaliya, B., Weerdt, A. D., Coster, D. D., Shah, A., et al. (2011). Structure–activity relationship of 2-hydroxy-2-aryl-2,3-dihydro-imidazo[1,2-a]pyrimidinium salts and 2N-substituted 4(5)-aryl-2-amino-1H-imidazoles as inhibitors of biofilm formation by Salmonella typhimurium and Pseudomonas aeruginosa. Bioorg. Med. Chem. 19, 3462–3473. doi: 10.1016/j.bmc.2011.04.026
Taganna, J. C., Quanico, J. P., Perono, R. M. G., Amor, E. C., and Rivera, W. L. (2011). Tannin-rich fraction from Terminalia catappa inhibits quorum sensing (QS) in Chromobacterium violaceum and the QS-controlled biofilm maturation and LasA staphylolytic activity in Pseudomonas aeruginosa. J. Ethnopharmacol. 134, 865–871. doi: 10.1016/j.jep.2011.01.028
Taguri, T., Tanaka, T., and Kouno, I. (2004). Antimicrobial activity of 10 different plant polyphenols against bacteria causing food-borne disease. Biol. Pharm. Bull. 27, 1965–1969. doi: 10.1248/bpb.27.1965
Tintino, S. R., Oliveira-Tintino, C. D. M., Campina, F. F., Silva, R. L. P., Costa, M. D. S., Menezes, I. R. A., et al. (2016). Evaluation of the tannic acid inhibitory effect against the NorA efflux pump of Staphylococcus aureus. Microb. Pathog. 97, 9–13. doi: 10.1016/j.micpath.2016.04.003
Totsika, M. (2016). Benefits and challenges of Antivirulence antimicrobials at the Dawn of the post-antibiotic era. Drug Deliv. Lett. 6, 30–37. doi: 10.2174/2210303106666160506120057
Trentin, D. S., Silva, D. B., Amaral, M. W., Zimmer, K. R., Silva, M. V., Lopes, N. P., et al. (2013). Tannins possessing bacteriostatic effect impair Pseudomonas aeruginosa adhesion and biofilm formation. PLoS One 8:e66257. doi: 10.1371/journal.pone.0066257
Ulrey, R. K., Barksdale, S. M., Zhou, W., and van Hoek, M. L. (2014). Cranberry proanthocyanidins have anti-biofilm properties against Pseudomonas aeruginosa. BMC Complement. Altern. Med. 14:499. doi: 10.1186/1472-6882-14-499
Ultee, A., Bennik, M. H. J., and Moezelaar, R. (2002). The phenolic hydroxyl Group of Carvacrol is Essential for action against the food-borne pathogen Bacillus cereus. Appl. Environ. Microbiol. 68, 1561–1568. doi: 10.1128/AEM.68.4.1561-1568.2002
Vattem, D. A., Lin, Y.-T., Labbe, R. G., and Shetty, K. (2004). Antimicrobial activity against select food-borne pathogens by phenolic antioxidants enriched in cranberry pomace by solid-state bioprocessing using the food grade fungus Rhizopus oligosporus. Process Biochem. 39, 1939–1946. doi: 10.1016/j.procbio.2003.09.032
Veldhuizen, E. J. A., Tjeerdsma-van Bokhoven, J. L. M., Zweijtzer, C., Burt, S. A., and Haagsman, H. P. (2006). Structural requirements for the antimicrobial activity of Carvacrol. J. Agric. Food Chem. 54, 1874–1879. doi: 10.1021/jf052564y
Versari, A., du Toit, W., and Parpinello, G. P. (2013). Oenological tannins: a review. Aust. J. Grape Wine Res. 19, 1–10. doi: 10.1111/ajgw.12002
Vijayakumar, K., and Thirunanasambandham, R. (2021). 5-Hydroxymethylfurfural inhibits Acinetobacter baumannii biofilms: an in vitro study. Arch. Microbiol. 203, 673–682. doi: 10.1007/s00203-020-02061-0
Vu, T. T., Kim, H., Tran, V. K., Vu, H. D., Hoang, T. X., Han, J. W., et al. (2017). Antibacterial activity of tannins isolated from Sapium baccatum extract and use for control of tomato bacterial wilt. PLoS One 12:e0181499. doi: 10.1371/journal.pone.0181499
Warraich, A. A., Mohammed, A. R., Perrie, Y., Hussain, M., Gibson, H., and Rahman, A. (2020). Evaluation of anti-biofilm activity of acidic amino acids and synergy with ciprofloxacin on Staphylococcus aureus biofilms. Sci. Rep. 10:9021. doi: 10.1038/s41598-020-66082-x
Widsten, P., Cruz, C. D., Fletcher, G. C., Pajak, M. A., and McGhie, T. K. (2014). Tannins and extracts of fruit byproducts: antibacterial activity against foodborne bacteria and antioxidant capacity. J. Agric. Food Chem. 62, 11146–11156. doi: 10.1021/jf503819t
Wu, D., Wu, X.-D., You, X.-F., Ma, X.-F., and Tian, W.-X. (2010). Inhibitory effects on bacterial growth and b-ketoacyl-ACP reductase by different species of maple leaf extracts and tannic acid. Phyther. Res. 24, S35–S41. doi: 10.1002/ptr.2873
Zhen, L., Lange, H., and Crestini, C. (2021a). An analytical toolbox for fast and straightforward structural characterisation of commercially available tannins. Molecules 26:2532. doi: 10.3390/molecules26092532
Keywords: tannins, antibiofilm activity, structure–activity relationships, Salmonella Typhimurium, Escherichia coli, Pseudomonas aeruginosa, Staphylococcus aureus
Citation: Villanueva X, Zhen L, Ares JN, Vackier T, Lange H, Crestini C and Steenackers HP (2023) Effect of chemical modifications of tannins on their antimicrobial and antibiofilm effect against Gram-negative and Gram-positive bacteria. Front. Microbiol. 13:987164. doi: 10.3389/fmicb.2022.987164
Received: 05 July 2022; Accepted: 18 November 2022;
Published: 06 January 2023.
Edited by:
Huancai Lin, Sun Yat-sen University, ChinaReviewed by:
Jintae Lee, Yeungnam University, South KoreaCopyright © 2023 Villanueva, Zhen, Ares, Vackier, Lange, Crestini and Steenackers. This is an open-access article distributed under the terms of the Creative Commons Attribution License (CC BY). The use, distribution or reproduction in other forums is permitted, provided the original author(s) and the copyright owner(s) are credited and that the original publication in this journal is cited, in accordance with accepted academic practice. No use, distribution or reproduction is permitted which does not comply with these terms.
*Correspondence: Hans P. Steenackers, aGFucy5zdGVlbmFja2Vyc0BrdWxldXZlbi5iZQ==
Disclaimer: All claims expressed in this article are solely those of the authors and do not necessarily represent those of their affiliated organizations, or those of the publisher, the editors and the reviewers. Any product that may be evaluated in this article or claim that may be made by its manufacturer is not guaranteed or endorsed by the publisher.
Research integrity at Frontiers
Learn more about the work of our research integrity team to safeguard the quality of each article we publish.