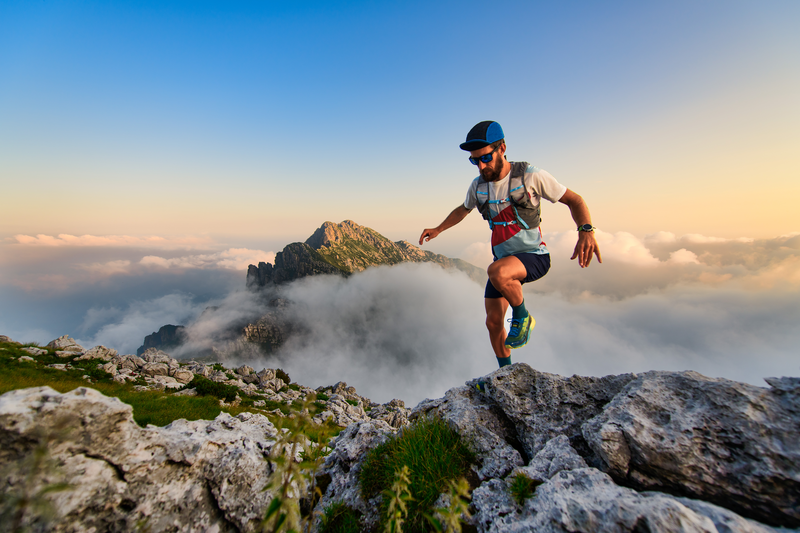
94% of researchers rate our articles as excellent or good
Learn more about the work of our research integrity team to safeguard the quality of each article we publish.
Find out more
ORIGINAL RESEARCH article
Front. Microbiol. , 22 August 2022
Sec. Food Microbiology
Volume 13 - 2022 | https://doi.org/10.3389/fmicb.2022.982152
Alginate oligosaccharides (AOS), natural polymers from brown seaweeds (such as Laminaria japonica, Undaria pinnatifida, and Sargassum fusiforme), have been reported to possess many beneficial advantages for health. In the current study, after 9 weeks of dietary supplementation, AOS 10 mg/kg group (AOS 10) group increased boar sperm motility from 87.8% to 93.5%, p < 0.05. Moreover, AOS10 increased the relative abundances of Bifidobacterium, Coprococcus, Butyricicoccus (1.3–2.3-fold; p < 0.05) to increase the beneficial blood and sperm metabolites (1.2–1.6-fold; p < 0.05), and important sperm proteins such as gelsolin, Zn-alpha2 glycoprotein, Cation Channel Sperm-Associated Protein, outer dense fiber of sperm tails, etc. (1.5–2.2-fold; p < 0.05). AOS had a long-term beneficial advantage on boar semen quality by the increase in semen volume (175 vs. 160 ml/ejaculation, p < 0.05). AOS may be used as dietary additives for improving semen quality.
Alginate oligosaccharides (AOS), natural biodegradable polymers derived from the degradation of alginate (brown seaweed), are made up of α-L-guluronate (G) and β-D-mannuronate (M) linked by 1, 4-glycoside bonds (Hu et al., 2019). AOS have many biological advantages with the great characteristics: non-immunogenicity, non-toxicity, and biodegradability (Ueno et al., 2012; Moriya et al., 2013; Ruvinov and Cohen, 2016; Pritchard et al., 2017; Han et al., 2019; Hu et al., 2019). AOS can act as anti-inflammation (Moriya et al., 2013), anti-apoptosis (Tusi et al., 2011), anti-proliferation (Tajima et al., 1999), antioxidant activities (Tusi et al., 2011; Ueno et al., 2012; Guo et al., 2016), and even anti-cancer reagent (Yang et al., 2017). Recently, dietary AOS improved intestinal cell development and intestinal morphology and barrier function, and stimulating weaned pig growth (Wan et al., 2017, 2018a,b; Zhao et al., 2020a). Thus, AOS have been approved as a safe biopolymer by the U.S. Food and Drug Administration (reference no. 21CFR184.1724) to be applied in pharmaceutical, cosmeceutical, and nutraceutical fields (Park et al., 2016; Hu et al., 2019).
Reproductive biotechnologies, especially artificial insemination (AI), play a vital role in the genetic improvement of pigs and other farm animals (Singh et al., 2021). Moreover, AI not only makes significant contributions to the development of swine production worldwide, it also raises the importance of the reproductive efficiency of boars in pig herds (Tsakmakidis et al., 2010). Semen quality is used as a proxy measure of boar fertility owing to the close correlation between sperm quality with boar fertility, and it creates a desired effect on piglet production in terms of the reproductive performance of sows (Dong et al., 2016). In summary, good semen quality is fundamental for successful AI (Tsakmakidis et al., 2010; Singh et al., 2021).
The production and quality of semen not only depend on intrinsic factors such as breed (Wolf, 2009) and age (Huang et al., 2010), but also on environmental extrinsic factors, for example, temperature, photoperiod, and nutrition (Ciereszko et al., 2000; Yeste et al., 2010; Dong et al., 2016). Boar age and semen quality are factors that are considered in boar culling (Tsakmakidis et al., 2012). Reports show that a maximum semen volume and sperm concentration can be obtained from boars of ≤3.5 years of age (Smital, 2009; Tsakmakidis et al., 2012). Hormonal and cellular changes take place in males with aging, which alters sperm quality and fertilization capacity (Araujo and Wittert, 2011). Aging also induces a decrease in testosterone levels, which is involved with intrinsic and extrinsic factors associated with Leydig cells (Midzak et al., 2009; Araujo and Wittert, 2011; Tsakmakidis et al., 2012).
Nutrition influences boar libido, sperm output, semen quality, and fertility (sow pregnancy rate and litter sizes; Dong et al., 2016; Liu et al., 2017a). It is known that protein levels in the diet affect boar semen quality; both low protein or excessive protein can decrease sperm quality (Louis et al., 1994; Dong et al., 2016). Individual amino acids have potential impacts on semen quality (Ren et al., 2015) as follows: dietary lysine (1.03%) improves boar semen quality compared to 0.86% (Dong et al., 2016); supplementation of threonine benefits ram and boar sperm quality (Wilson et al., 2004); tryptophan significantly improves ram sperm motility (Pichardo et al., 2011; Dong et al., 2016). Furthermore, polyunsaturated fatty acids (PUFAs) have been shown to benefit sperm motility and fertility in human and animal studies (Murphy et al., 2017; Liu et al., 2017a). The major commercial source of omega-3 fatty acids [specifically docosahexaenoic acid (DHA)] are fish oils, and the most abundant source for linolenic acid is flaxseed (~53%). Chestnut polysaccharides have been discovered to improve the spermatogenesis and semen quality recently (Yu et al., 2020; Sun et al., 2022). With the great advantages, the purpose of this investigation was to explore AOS benefit boar semen quality and the underlying mechanisms.
AOS (>98%) was from Qingdao BZ Oligo Biotech Co. Eosin, insulin, EGF, cysteine, pyruvate, kanamycin, paraformaldehyde, and Triton X-100 were from Sigma-Aldrich. BSA, goat serum, and TCM-199 medium were from Life Technologies Ltd. Polyvinylidene fluoride (PVDF) membrane was from Merck. E.Z.N.A.® Stool DNA Kit was from Omega Bio-tek Inc. The antibodies were purchased from different companies listed in Supplementary Table S1.
All animal procedures were approved by the Animal Care and Use Committee of the Institute of Animal Sciences of Chinese Academy of Agricultural Sciences (IAS2021-67). Twenty boars (~24 months of age; male) were used in this investigation at the artificial insemination center of Yangxiang Joint Stock Company (Guangxi, China; Guo et al., 2020). Boar feeding conditions have been previously reported (Supplementary Table S2; Wu et al., 2019a). Our preliminary study has found that 10 mg/kg was good concentration for improving boar semen quality. There were two treatments: (1) Control group (CON), 10 boars fed with a basal diet, and (2) AOS 10 mg/kg group (AOS 10), 10 boars fed with a basal diet plus 10 mg/kg body weight of AOS. Semen samples were collected after 9-week feeding (Figure 1A) by gloved-hand techniques. After collection, four semen parameters were assessed: semen volume, sperm concentration, sperm motility, and abnormal sperm rate, according to the reported methods (Wu et al., 2019b; Guo et al., 2020). Blood samples were harvested by venipuncture from the hindlimb vein of boars during ejaculations. Each blood sample was then centrifuged at 3000× g for 10 min at 4°C to obtain a plasma sample and subsequently stored at −80°C until analysis. Each boar’s rectum was massaged to stimulate defecation, and then, fresh feces were collected and stored at −80°C for subsequent microbiota analysis (Guo et al., 2020). The long-term effects of AOS on boar semen quality were determined. After AOS supplementation, all the boars were fed with basal diet (without AOS supplementation) for another 8 weeks. The semen was collected every 5 days and the semen quality was analyzed (Figure 1A).
Figure 1. The impacts of AOS on boar sperm quality. (A) Study scheme. (B) Spermatozoa motility determined by CASA. Y-axis = % of total cells, X-axis = treatment group (mg/kg body weight). n = 10. (C) Semen volume. Y-axis = volume (ml), X-axis = treatment concentration (mg/kg body weight). n = 10. (D) Sperm concentration. Y-axis = sperm concentration (10^8/ml), X-axis = treatment group (mg/kg body weight). n = 10. (E) In vitro fertilization rate. Y-axis = % of total oocytes, X-axis = treatment group (mg/kg body weight). n = 10.
Spermatozoa motility and concentration were determined by the computer-assisted sperm assay (CASA) method according to World Health Organization guidelines (WHO, 2010; Zhao et al., 2016; Zhang et al., 2018, 2019). Boar spermatozoa were incubated at 37.5°C for 30 min then samples were placed in a pre-warmed counting chamber (MICROPTIC S.L., Barcelona, Spain). The Microptic Sperm class analyzer (CASA system) was used in this investigation. It was equipped with a 20-fold objective, a camera adaptor (Eclipse E200, Nikon, Japan), and a camera (acA780-75gc, Basler, Germany); it was operated by an SCA sperm class analyzer (MICROPTIC S.L.).
Boar sperm was stained with Eosin Y (1%; Zhao et al., 2016; Zhang et al., 2018, 2019). Spermatozoa abnormalities were then viewed using a bright-field microscopy (AH3-RFCA, Olympus, Tokyo, Japan) and were classified into head or tail morphological abnormalities: two heads, two tails, blunt hooks, and short tails. The examinations were repeated three times, and 500 spermatozoa per animal were scored.
The procedure for the preparation of porcine oocytes has been reported previously (Redel et al., 2019; Zhou et al., 2020). Porcine ovaries were obtained from a slaughterhouse. Follicular fluid from 3 to 6 mm antral follicles was aspirated with an 18-gauge syringe. Cumulus oocyte complexes (COCs) with uniform cytoplasm and several layers of cumulus cells were selected and rinsed three times in washing medium [TCM-199 medium supplemented with 10% porcine follicular fluid (pFF), 5 μg/ml insulin, 10 ng/ml EGF, 0.6 mM cysteine, 0.2 mM pyruvate, and 25 μg/ml kanamycin]. Approximately 70 COCs per well were cultured under mineral oil in 4-well plates containing TCM-199 medium supplemented with 10% porcine follicular fluid (pFF), 5 μg/ml insulin, 10 ng/ml EGF, 0.6 mM cysteine, 0.2 mM pyruvate, 25 μg/ml kanamycin, and 5 IU/ml of each of eCG and hCG. The oocytes were matured for 44 h at 38.5°C, 5% CO2 in a humidified incubator.
The in vitro fertilization (IVF) medium Tyrode’s albumin lactate pyruvate (TALP) 29 was previously equilibrated for ~3 h at 38.5°C, with 5% CO2 in air and a humidified incubator until it reached a final pH of 7.4. In vitro mature (IVM) oocytes were mechanically denuded with an automatic pipette, washed in TALP medium, and transferred in groups of 50 oocytes to a 4-well plate (Nunc, Roskilde, Denmark) containing 500 μl TALP medium per well. Sperm suspensions were added to the IVF wells at a final concentration of 25 ◊ 103 cells/ml. After a 6-h coculture, the putative zygotes were fixed with 0.5% glutaraldehyde in phosphate-buffered saline (PBS), stained with 1% (w/v) Hoechst 33342 in PBS, and examined under an epifluorescence microscope. The parameters analyzed were the percentage of oocytes penetrated by one or more spermatozoa (Pen, %; Zapata-Carmona et al., 2020).
Total genomic DNA of boar feces was isolated using an E.Z.N.A.® Stool DNA Kit (Omega Bio-tek Inc., United States) following the manufacturer’s instructions. DNA quantity and quality were analyzed using NanoDrop 2000 (Thermo Scientific, United States) and 1% agarose gel (Zhang et al., 2020).
The V3–V4 region of the 16S rRNA gene was amplified using the primers 338F (5′-ACTCCTACGGGAGGCAGCAG-3′) and 806R (5′-GGACTACHVGGGTWTCTAAT-3′) with Barcode. The PCR reactions (total 30 μl) included 15 μl Phusion® High-Fidelity PCR Master Mix (New England Biolabs), 0.2 mM primers, and 10 ng DNA. The thermal cycle was carried out with an initial denaturation at 98°C, followed by 30 cycles of 98°C for 10 s, 50°C for 30 s, 72°C for 30 s, and a final extension at 72°C for 5 min. PCR products were purified using an AxyPrep DNA Gel Extraction Kit (Axygen Biosciences, United States). The sequencing libraries were constructed with NEB Next® UltraTM DNA Library Prep Kit for Illumina (NEB, United States) following the manufacturer’s instructions, and index codes were added. Then, the library was sequenced on the Illumina MiSeq 2,500 platform (Illumina, United States) and 300 bp paired-end reads were generated at the Novo gene. The paired-end reads were merged using FLASH (V1.2.71; Magoč and Salzberg, 2011). The quality of the tags was controlled in QIIME (V1.7.02); meanwhile, all chimeras were removed (Zhang et al., 2020). The “Core Set” of the Greengenes database3 (DeSantis et al., 2006) was used for classification, and sequences with >97% similarity were assigned to the same operational taxonomic units (OTUs).
OTU abundance information was normalized using a standard of sequence number corresponding to the sample with the least sequences. The alpha diversity indices were calculated with QIIME (Version 1.7.0; Caporaso et al., 2010). Partial least squares discrimination analysis (PLS-DA) was performed using R software (v2.15.3).
Plasma and sperm metabolites were determined by LC–MS/MS (Zhang et al., 2020). Boar plasma and sperm were collected and maintained at −80°C. The protein was removed from the samples before LC–MS/MS analysis with ACQUITY UPLC and AB Sciex Triple TOF 5600 (LC/MS) as reported previously (Zhang et al., 2020).
The conditions for HPLC were: ACQUITY UPLC BEH C18 column (100 mm × 2.1 mm, 1.7 μm), solvent A [aqueous solution with 0.1% (v/v) formic acid], and solvent B [acetonitrile with 0.1% (v/v) formic acid] with a gradient program: 0–2 min, 5%–20% B; 2–4 min, 20%–25% B; 4–9 min, 25%–60% B; 9–17 min, 60%–100% B; 17–19 min, 100% B; 19–19.1 min, 100%–5% B; and 19.1–20.1 min, 5% B. The flow rate was set at 0.4 ml/min and 5 μl was injected. ESI was used in the mass spectrometry program. Progenesis QI v.2.3 (Nonlinear Dynamics, Newcastle, United Kingdom) was used to normalize the peaks. Human Metabolome Database (HMDB), LIPID MAPS (v. 2.3), and METLIN software were used to qualify the data. Furthermore, the data were analyzed with SIMCA software (v. 14.0, Umetrics, Umeå, Sweden) and the KEGG database1 was used for pathway enrichment analysis.
The methods for IHF of boar sperm have been reported in our previous articles. Boar spermatozoa were fixed in 4% paraformaldehyde for 1 h, then the cells were spread onto poly-L-lysine coated microscope slides and air-dried. After three washings with PBS (5 min each), spermatozoa were incubated with 2% (vol/vol) Triton X-100 in PBS for 1 h at RT. Then, after three washes with PBS, the cells were blocked with 1% (wt/vol) BSA and 1% goat serum in PBS for 30 min at RT, followed by incubation with primary antibodies (1:100; Supplementary Table S2) diluted in blocking solution overnight at 4°C. The following morning, after three washes with PBS Tween 20 (0.5%) the slides were incubated with Alexa Fluor 546 goat anti-rabbit IgG (1,200) for 30 min in darkness at RT. The negative control samples were incubated with a secondary antibody and without a primary antibody. Slides were washed with PBS Tween-20 three times and then incubated with DAPI (4.6-diamidino-2-phenylindole hydrochloride, 100 ng/ml) as a nuclear stain for 5 min. After a brief wash with ddH2O, the slides were covered with an anti-fading mounting medium (Vector, Burlingame, United States). Fluorescence images were obtained with a Leica Laser Scanning Confocal Microscope (LEICA TCS SP5 II, Germany; Zhao et al., 2016; Zhang et al., 2020).
The procedure for Western blotting analysis of boar sperm proteins is reported in our previous publications (Zhao et al., 2016; Zhang et al., 2020). Briefly, sperm cells were lysed in RIPA buffer containing the protease inhibitor cocktail from Sangon Biotech, Ltd. (Shanghai, China). Protein concentration was determined using a BCA kit (Beyotime Institute of Biotechnology, Shanghai, China). Actin was used as a loading control. The primary antibodies (Abs) are listed in Supplementary Table S2. Secondary donkey anti-goat Ab (Cat no.: A0181) was purchased from Beyotime Institute of Biotechnology (Shanghai, P.R. China), and goat anti-rabbit (Cat no.: A24531) Abs were bought from Novex® by Life Technologies (United States). Fifty micrograms of total protein per sample were loaded onto 10% SDS polyacrylamide electrophoresis gels. The gels were transferred to a polyvinylidene fluoride (PVDF) membrane at 300 mA for 2.5 h at 4°C. Then, the membranes were blocked with 5% BSA for 1 h at RT, followed by three washes with 0.1% Tween-20 in TBS (TBST). The membranes were incubated with primary Abs diluted at 1:500 in TBST with 1% BSA overnight at 4°C. After three washes with TBST, the blots were incubated with the HRP-labeled secondary goat anti-rabbit or donkey anti-goat Abs, respectively, for 1 h at RT. After three washes, the blots were imaged.
All data were expressed as mean ± standard deviation (SD). Data were determined by SPSS statistical software (IBM Co., NY) with one-way analysis of variance (ANOVA), followed by LSD multiple comparison test. Graphs were created using GraphPad Prism 5.20 (GraphPad Software Inc., La Jolla, CA, United States). All groups were compared with each other for every parameter. p < 0.05 was considered to be significant.
The microbiota raw sequencing data generated in this study has been uploaded to the NCBI SRA database with the accession number GSE178723.
After 9 weeks of feeding (Figure 1A), the AOS10 group showed significantly increased sperm motility from 87.8% to 93.5% (Figure 1B; p < 0.05). The semen volume per boar per day (204 ml/ejaculation vs. 176 ml/ejaculation; p = 0.612) and the concentration (5.6 × 10^8/ml vs. 5.0 × 10^8/ml; p = 0.137) of sperm also showed an increasing trend in AOS10 over CON while the difference was not significant (Figures 1C,D). Moreover, the in vitro fertilization rate was also higher in AOS10 (58.6%) than in CON (52.1%; Figure 1E) while it was not significant.
To understand the mechanisms underlying the AOS improvement of semen quality, we explored the protein levels of important genes for sperm quality. AOS10 increased the protein levels of gelsolin (1.61-fold), p-AKT (phosphorylated protein kinase B; 1.48-fold), protein kinase A (PKA; 1.95-fold), and Zn-alpha2 glycoprotein (ZAG; 2.05-fold) according to IHF detection (Figures 2A,B; *p < 0.05). The data for these protein levels were confirmed by western blotting analysis. Moreover, the levels of the other four sperm proteins such as cation channel sperm-associated protein (CatSper; 2.12-fold), outer dense fiber of sperm tails 2 (ODF2; 1.42-fold), p-ERK1 (phosphorylated extracellular signal-regulated kinase; 1.51-fold 1), and Phosphoinositide-3-Kinase (PI3K; 1.47-fold) were also elevated in AOS10 over CON (Figures 2C,D; *p < 0.05).
Figure 2. The influence of AOS on the protein expression in boar sperm. (A) Sperm protein levels of important genes for semen quality as detected by immunofluorescence staining. N > 6. (B) Quantitative data for immunofluorescence staining. Y-axis = fold change to CON, X-axis = treatment group (mg/kg body weight). *p < 0.05. (C) Sperm protein levels of important genes for semen quality as detected by Western blotting. N > 3. (D) Quantitative data for western blotting analysis. Y-axis = fold change to CON, X-axis = treatment group (mg/kg body weight). *p < 0.05.
There were 1,031 metabolites detected in the boar sperm samples (Supplementary Figure S1; Supplementary Table S3). The OPLS-DA analysis showed that the AOS10 and CON groups were well separated (Supplementary Figure S1A). Twenty-eight metabolites were significantly different in AOS10 compared to CON (Figure 3A; Supplementary Tables S4, S5). Nine of the significantly increased metabolites including β-leucine, D-glutamic acid, γ-glutamylthreonine, L-lysine, L-norleucine, L-proline, methionine sulfoxide, O-acetylserine, and tyrosyl-glutamate are shown in Figure 3B (1.2–1.6folds; p < 0.05). Some of the significantly decreased metabolites (such as 2-hydroxylfelbamate, 14,15-diHETrE, heptadecanoic acid, and methyl hexadecenoic acid) are shown in Figure 3C (5%–10%; p < 0.05). These 28 metabolites were well correlated with sperm motility, sperm concentration, and sperm volume (Figure 3D), with the increased metabolites in AOS10 being positively correlated with these sperm parameters, while the decreased metabolites in AOS10 were negatively correlated. At the same time, the metabolites were well correlated with each other (Supplementary Figure S1D).
Figure 3. The impacts of AOS on boar sperm metabolome. (A) Heatmap of changed sperm metabolites. The sperm metabolites were determined by LC–MS/MS. (B) List of AOS increased sperm metabolites. Y-axis = relative amount, X-axis = treatment group (mg/kg body weight). (C) List of AOS decreased sperm metabolites. Y-axis = relative amount, X-axis = treatment group (mg/kg body weight). (D) Correlation of sperm metabolites and sperm concentration, volume, and motility.
There were 1,087 metabolites detected in boar plasma samples (Supplementary Figure S2; Supplementary Table S6). The OPLS-DA analysis showed that the AOS10 and CON groups were well separated (Supplementary Figure S2A). Eighteen metabolites were significantly different in AOS10 compared with CON (Figure 4A; Supplementary Tables S7, S8). Functional enrichment of these metabolites showed that they were involved in amino acid metabolism and retinol metabolism (Figure 4B). Some of the significantly changed metabolites are shown in Figure 4C. 11-cis-retinol, betaine, 5-hydroxy-indoleacetaldehyde, N1-methyl-2-pyridone-5-carboxamide, and quinolinic acid were increased from 1.5–2.4-fold (p < 0.05), while iminoaspartic acid was decreased (45%, p < 0.05). At the same time, these metabolites were well correlated with each other (Supplementary Figure S2C). Moreover, the significantly changed blood metabolites and the significantly changed sperm metabolites were well correlated (Figure 4D; Supplementary Table S9).
Figure 4. The effects of AOS on boar blood metabolome. (A) Heatmap of changed blood metabolites. The blood metabolites were determined by LC–MS/MS. (B) KEGG enriched pathways of changed blood metabolites. (C) List of AOS changed blood metabolites. Y-axis = relative abundance, X-axis = treatment group (mg/kg body weight). (D) Correlation of blood metabolites and sperm metabolites.
AOS10 affected the gut microbiota composition (Figure 5; Supplementary Figure S3). At the phylum level, compared to CON, AOS10 increased the relative abundances of Bacteroidetes (1.21fold; p > 0.05), decreased the levels of Firmicutes (Figure 5B; p > 0.05), and increased the ratio of Bacteroidetes/Firmicutes (Supplementary Figure S3E) in boar fecal samples. Moreover, at the class level, the microbiota and sperm motility were well correlated and Bacteroidetes was positively correlated with sperm motility; meanwhile, Firmicutes was negatively correlated with sperm motility (Figure 5C). At the genus level, AOS10 increased the relative abundances of several beneficial bacteria: Bifidobacterium (1.51-fold), Coprococcus (2.33-fold), and Butyricicoccus (1.33-fold). (Figure 5D; p > 0.05). Moreover, Coprococcus was positively correlated with sperm motility (Figure 5E), while Intestinimonas and Lactobacillus were positively correlated with both sperm motility and concentration (Figure 5E).
Figure 5. The influence of AOS on boar gut microbiota. (A) Differences of bacterial abundance at the genus level. Y-axis = relative abundance, X-axis = treatment group (mg/kg body weight). (B) Differences of bacterial abundance at the class level. (C) Correlation of bacterial abundance at the class level with sperm motility and concentration. (D) Representative differences of bacterial abundance at the genus level. Y-axis = relative abundance, X-axis = treatment group (mg/kg body weight). (E) Correlation of bacterial abundance at the genus level with sperm motility and concentration.
Furthermore, sperm metabolites and fecal microbiota were well correlated (Figure 6A; Supplementary Table S10). Sperm metabolites increased in the AOS10 group were positively correlated with the beneficial microbiota Bifidobacterium, Coprococcus, and Butyricicoccus (Figure 6A). Similarly, blood metabolites and fecal microbiota were well correlated (Figure 6B; Supplementary Table S11), and the blood metabolites increased in AOS10 were positively correlated with the beneficial microbiota Bifidobacterium, Coprococcus, and Lactobacillus (Figure 6B).
Figure 6. (A) Correlation of sperm metabolites with fecal microbiota. (B) Correlation of blood metabolites with fecal microbiota.
AOS had a long-term beneficial improvement on boar semen quality by the increase in the semen volume (175 vs. 160 ml/ ejaculation, p < 0.05) and sperm motility, while the decrease in the percentage of abnormal sperm after another 2 months on basal diet (without AOS addition; Figures 7A–C). The sperm concentration was in an increased trend in AOS10 group compared to CON (Figure 7D; p = 0.185).
Figure 7. Long-term effects of AOS on boar semen quality. After AOS supplementation, boars were fed with basal diet for 8 weeks. Semen quality was determined every 5 days for 2 months (no AOS supplementation). (A) Semen volume. Y-axis = volume (ml), X-axis = treatment group (mg/kg body weight). (B) Abnormal sperm. Y-axis = % of total cells, X-axis = treatment group (mg/kg body weight). (C) Sperm motility. Y-axis = % of total cells, X-axis = treatment group (mg/kg body weight). (D) Sperm concentration. Y-axis = sperm concentration (10^8/ml), X-axis = treatment group (mg/kg body weight). n = 10, *p < 0.05.
AOS have been used in many different perspectives as anti-inflammation (Moriya et al., 2013), anti-apoptosis (Tusi et al., 2011), anti-proliferation (Tajima et al., 1999), and even anti-cancer chemicals (Wan et al., 2017) because of the highly desired natural properties (non-immuno-genicity, biodegradability, and non-toxicity). AOS decrease the production of nitric oxide, prostaglandin E2, and pro-inflammatory cytokines, and block the expression of toll-like receptor 4 and nuclear factor (NF)-κB to prevent neuroinflammation or neurodegenerative diseases (such as Alzheimer’s disease; Zhou et al., 2015). Wan and coauthors reported that AOS improved antioxidant levels and increased piglet growth rates (Wan et al., 2017, 2018a,b). AOS benefited intestinal epithelial cell growth and differentiation to improve livestock growth rates (Zhao et al., 2020a). Moreover, AOS improved busulfan disrupted spermatogenesis in mice (Zhao et al., 2020b). In the current investigation, we found that AOS 10 mg/kg could benefit boar sperm motility. The difference between AOS10 and CON was small, which may be because the boars used in this investigation were at an optimal age for sperm production; therefore, the room for improvement in these parameters was limited. However, altogether, the data suggested that AOS has the potential to improve boar semen quality and fertility. Moreover, AOS increased the levels of important proteins in boar sperm such as gelsolin, ODF2, PKA, AKT, etc. All these proteins play important roles in sperm function or fertility (Lee, 2012; Liu et al., 2012; Finkelstein et al., 2013; Zhao et al., 2016; Sun et al., 2017). The data here indicated that AOS10 improved boar sperm quality. The data indicated that AOS could be used as a dietary additive for boars to improve their semen quality.
Gut microbiota play many physiological roles not limited to metabolic-related disorders such as obesity and diabetes (Bouter et al., 2017; Liu et al., 2017b), but also including nervous system and reproductive system-related diseases or conditions (Dai et al., 2015). Previously, we found that AOS benefited gut microbiota by the increase in the “beneficial” bacteria while the decrease in “harmful” bacteria in murine small intestines to rescue cell development (Zhang et al., 2020). Gut microbiota metabolize nutrients in the intestine and regulate intestinal metabolites to influence the blood metabolome (Tajima et al., 1999; Tusi et al., 2011). In turn, while traveling through body organs, blood metabolites can influence their development or induce disorders (Dai et al., 2015). It is known that metabolic regulation is essential for spermatogenesis (Cheng et al., 2010; Rato et al., 2010, 2012), and cholesterol and lipid homeostasis play a vital role in male fecundity (Cross, 1998; Ergün et al., 2007; Maqdasy et al., 2013; Lu et al., 2016; Kim et al., 2017). Sertoli cells act as nurse cells, providing the nutrients and energy for the process of spermatogenesis. Many other components such as hormones and endogenous or exogenous factors have a synergistic role in the homeostasis of metabolism in the testis and the progression of spermatogenesis (Rato et al., 2012). It has been shown that abnormal lipid metabolism in the reproductive system or blood contributes to human male infertility (Ergün et al., 2007; Lu et al., 2016; Kim et al., 2017). AOS could improve busulfan-damaged homeostasis of lipid metabolism in murine blood (Zhang et al., 2020). In the current investigation, the relative abundances of beneficial bacteria, such as Lactobacillus, were increased (1.35-fold), and the ratio of Bacteroidetes/Firmicutes was elevated by AOS. At the same time, AOS benefited boar sperm and blood metabolites by increasing levels of proline, lysine, retinol, betaine, etc. All the data indicated that AOS can boost boar semen quality through improving the gut microbiota, blood, and testicular metabolites.
The very interesting finding in the current study was that AOS benefited gut microbiota can continuingly improve boar semen quality after AOS treatment followed by 8 weeks of basal diet (without AOS supplementation). Because AOS cannot be directly absorbed into blood to reach the organs to affect their functions (Liu et al., 2019), the beneficial effects of AOS on sperm metabolites and semen quality may be due to the benefited gut microbiota. Otherwise, the effects cannot last for so long time. The data in the current investigation and our previous reports confirm that AOS benefit gut microbiota in mice and boars, and the benefited gut microbiota to increase semen quality.
In summary, the beneficial impact of AOS on boar semen quality possibly through the positive changes in the gut microbiota and plasma/sperm metabolism was revealed. These improvements will large litter sizes and increase the economical supply of porcine meat for global consumption.
The datasets presented in this study can be found in online repositories. The names of the repository/repositories and accession number(s) can be found in the article/Supplementary material.
Ethical review and approval was not required for the animal study because we did not kill animals in the study, if necessary we will provide later. Written informed consent was obtained from the owners for the participation of their animals in this study.
HH, YZo, BX, RZ, YJ, LC, HS, JT, BZ, and CG performed the experiments and analyzed the data. LC, HZ, and YZa designed and supervised the study and wrote the manuscript. All authors contributed to the article and approved the submitted version.
This study was supported by the National Natural Science Foundation of China (32070536 and 31772408 to YZa; 31672428 to HZ).
We thank the investigators and staff of the Beijing Genomics Institute (BGI) and Shanghai LUMING Biotechnology CO., LCD for technical support.
HS and JT were employed by YangXiang Joint Stock Company. BZ and CG were employed by Qingdao BZ Oligo Biotech Co., Ltd.
The remaining authors declare that the research was conducted in the absence of any commercial or financial relationships that could be construed as a potential conflict of interest.
All claims expressed in this article are solely those of the authors and do not necessarily represent those of their affiliated organizations, or those of the publisher, the editors and the reviewers. Any product that may be evaluated in this article, or claim that may be made by its manufacturer, is not guaranteed or endorsed by the publisher.
The Supplementary material for this article can be found online at: https://www.frontiersin.org/articles/10.3389/fmicb.2022.982152/full#supplementary-material
SUPPLEMENTARY FIGURE S1 | Sperm metabolite data. (A) PCA of sperm metabolites. (B) Quality control of sperm metabolite data. (C) Enriched pathways of changed sperm metabolites. (D) Correlation of sperm metabolite with each other.
SUPPLEMENTARY FIGURE S2 | Blood metabolite data. (A) PCA of blood metabolites. (B) Quality control of blood metabolite data. (C) Correlation of blood metabolite with each other.
SUPPLEMENTARY FIGURE S3 | Fecal microbiota data. (A) Quality control of fecal microbiota data. (B) The alpha index of the fecal microbiota. (C) PCA of blood metabolites. (D) LDA distribution and Cladogram. Linear discriminate analysis effect size (LEfSe) was performed to determine the difference in abundance; the threshold of LDA score was 4.0. (E) The ratio of Bacteroidetes/Firmicutes.
Araujo, A. B., and Wittert, G. A. (2011). Endocrinology of the aging male. Best Pract. Res. Clin. Endocrinol. Metab. 25, 303–319. doi: 10.1016/j.beem.2010.11.004
Bouter, K. E., van Raalte, D. H., Groen, A. K., and Nieuwdorp, M. (2017). Role of the gut microbiome in the pathogenesis of obesity and obesity-related metabolic dysfunction. Gastroenterology 152, 1671–1678. doi: 10.1053/j.gastro.2016.12.048
Caporaso, J. G., Kuczynski, J., Stombaugh, J., Bittinger, K., Bushman, F. D., Costello, E. K., et al. (2010). QIIME allows analysis of high-throughput community sequencing data. Nat. Methods 7, 335–336. doi: 10.1038/nmeth.f.303
Cheng, C. Y., Wong, E. W., Yan, H. H., and Mruk, D. D. (2010). Regulation of spermatogenesis in the microenvironment of the seminiferous epithelium: new insights and advances. Mol. Cell. Endocrinol. 315, 49–56. doi: 10.1016/j.mce.2009.08.004
Ciereszko, A., Ottobre, J. S., and Glogowski, J. (2000). Effects of season and breed on sperm acrosin activity and semen quality of boars. Anim. Reprod. Sci. 64, 89–96. doi: 10.1016/S0378-4320(00)00194-9
Cross, N. L. (1998). Role of cholesterol in sperm capacitation. Biol. Reprod. 59, 7–11. doi: 10.1095/biolreprod59.1.7
Dai, Z., Wu, Z., Hang, S., Zhu, W., and Wu, G. (2015). Amino acid metabolism in intestinal bacteria and its potential implications for mammalian reproduction. Mol. Hum. Reprod. 21, 389–409. doi: 10.1093/molehr/gav003
DeSantis, T. Z., Hugenholtz, P., Larsen, N., Rojas, M., Brodie, E. L., Keller, K., et al. (2006). Greengenes, a chimera-checked 16S rRNA gene database and workbench compatible with ARB. Appl. Environ. Microbiol. 72, 5069–5072. doi: 10.1128/AEM.03006-05
Dong, H., Wu, D., Xu, S., Li, Q., Fang, Z., Che, L., et al. (2016). Effect of dietary supplementation with amino acids on boar sperm quality and fertility. Anim. Reprod. Sci. 172, 182–189. doi: 10.1016/j.anireprosci.2016.08.003
Ergün, A., Köse, S. K., Aydos, K., Ata, A., and Avci, A. (2007). Correlation of seminal parameters with serum lipid profile and sex hormones. Arch. Androl. 53, 21–23. doi: 10.1080/01485010600888961
Finkelstein, M., Megnagi, B., Ickowicz, D., and Breitbart, H. (2013). Regulation of sperm motility by PIP2(4,5) and actin polymerization. Dev. Biol. 381, 62–72. doi: 10.1016/j.ydbio.2013.06.014
Guo, J. J., Ma, L. L., Shi, H. T., Zhu, J. B., Wu, J., Ding, Z. W., et al. (2016). Alginate oligosaccharide prevents acute doxorubicin cardiotoxicity by suppressing oxidative stress and endoplasmic reticulum-mediated apoptosis. Mar. Drugs 14:231. doi: 10.3390/md14120231
Guo, L., Wu, Y., Wang, C., Wei, H., Tan, J., Sun, H., et al. (2020). Gut microbiological disorders reduce semen utilization rate in Duroc boars. Front. Microbiol. 11:581926. doi: 10.3389/fmicb.2020.581926
Han, Z. L., Yang, M., Fu, X. D., Chen, M., Su, Q., Zhao, Y. H., et al. (2019). Evaluation of prebiotic potential of three marine algae oligosaccharides from enzymatic hydrolysis. Mar. Drugs 17:E173. doi: 10.3390/md17030173
Hu, Y., Feng, Z., Feng, W., Hu, T., Guan, H., and Mao, Y. (2019). AOS ameliorates monocrotaline-induced pulmonary hypertension by restraining the activation of P-selectin/p38MAPK/NF-κB pathway in rats. Biomed. Pharmacother. 109, 1319–1326. doi: 10.1016/j.biopha.2018.10.109
Huang, Y. H., Lo, L. L., Liu, S. H., and Yang, T. S. (2010). Age-related changes in semen quality characteristics and expectations of reproductive longevity in Duroc boars. Anim. Sci. J. 81, 432–437. doi: 10.1111/j.1740-0929.2010.00753.x
Kim, N., Nakamura, H., Masaki, H., Kumasawa, K., Hirano, K. I., and Kimura, T. (2017). Effect of lipid metabolism on male fertility. Biochem. Biophys. Res. Commun. 485, 686–692. doi: 10.1016/j.bbrc.2017.02.103
Lee, K. H. (2012). Ectopic expression of Cenexin1 S796A mutant in ODF2(+/−) knockout background causes a sperm tail development defect. Dev. Reprod. 16, 363–370. doi: 10.12717/DR.2012.16.4.363
Liu, R., Hong, J., Xu, X., Feng, Q., Zhang, D., Gu, Y., et al. (2017b). Gut microbiome and serum metabolome alterations in obesity and after weight-loss intervention. Nat. Med. 23, 859–868. doi: 10.1038/nm.4358
Liu, Y., Qu, F., Cao, X., Chen, G., Guo, Q., Ying, X., et al. (2012). Con A-binding protein Zn-alpha2-glycoprotein on human sperm membrane is related to acrosome reaction and sperm fertility. Int. J. Androl. 35, 145–157. doi: 10.1111/j.1365-2605.2011.01195.x
Liu, J., Yang, S., Li, X., Yan, Q., Reaney, M. J. T., and Jiang, Z. (2019). Alginate oligosaccharides: production, biological activities, and potential applications. Compr. Rev. Food Sci. Food Saf. 18, 1859–1881. doi: 10.1111/1541-4337.12494
Liu, Q., Zhou, Y., Duan, R., Wei, H., Peng, J., and Jiang, S. (2017a). Dietary n-6:n-3 ratio and vitamin E improve motility characteristics in association with membrane properties of boar spermatozoa. Asian J. Androl. 19, 223–229. doi: 10.4103/1008-682X.170446
Louis, G. F., Lewis, A. J., Weldon, W. C., Miller, P. S., Kittok, R. J., and Stroup, W. W. (1994). The effect of protein intake on boar libido, semen characteristics, and plasma hormone concentrations. J. Anim. Sci. 72, 2038–2050. doi: 10.2527/1994.7282038x
Lu, J. C., Jing, J., Yao, Q., Fan, K., Wang, G. H., Feng, R. X., et al. (2016). Relationship between lipids levels of serum and seminal plasma and semen parameters in 631 Chinese subfertile men. PLoS One 11:e0146304. doi: 10.1371/journal.pone.0146304
Magoč, T., and Salzberg, S. L. (2011). FLASH: fast length adjustment of short reads to improve genome assemblies. Bioinformatics 27, 2957–2963. doi: 10.1093/bioinformatics/btr507
Maqdasy, S., Baptissart, M., Vega, A., Baron, S., Lobaccaro, J. M., and Volle, D. H. (2013). Cholesterol and male fertility: what about orphans and adopted? Mol. Cell. Endocrinol. 368, 30–46. doi: 10.1016/j.mce.2012.06.011
Midzak, A. S., Chen, H., Papadopoulos, V., and Zirkin, B. R. (2009). Leydig cell aging and the mechanisms of reduced testosterone synthesis. Mol. Cell. Endocrinol. 299, 23–31. doi: 10.1016/j.mce.2008.07.016
Moriya, C., Shida, Y., Yamane, Y., Miyamoto, Y., Kimura, M., Huse, N., et al. (2013). Subcutaneous administration of sodium alginate oligosaccharides prevents salt-induced hypertension in Dahl salt-sensitive rats. Clin. Exp. Hypertens. 35, 607–613. doi: 10.3109/10641963.2013.776568
Murphy, E. M., Stanton, C., Brien, C. O., Murphy, C., Holden, S., Murphy, R. P., et al. (2017). The effect of dietary supplementation of algae rich in docosahexaenoic acid on boar fertility. Theriogenology 90, 78–87. doi: 10.1016/j.theriogenology.2016.11.008
Park, H. J., Ahn, J. M., Park, R. M., Lee, S. H., Sekhon, S. S., Kim, S. Y., et al. (2016). Effects of alginate oligosaccharide mixture on the bioavailability of lysozyme as an antimicrobial agent. J. Nanosci. Nanotechnol. 16, 1445–1449. doi: 10.1166/jnn.2016.10757
Pichardo, A. I., Tlachi-López, J. L., Jiménez-Trejo, F., Fuentes-Farías, A. L., Báez-SaldaN, A., Molina-Cerón, M. L., et al. (2011). Increased serotonin concentration and tryptophan hydroxylase activity in reproductive organs of copulator males: a case of adaptive plasticity. Adv. Biosci. Biotechnol. 02, 75–84. doi: 10.4236/abb.2011.22012
Pritchard, M. F., Powell, L. C., Jack, A. A., Powell, K., Beck, K., Florance, H., et al. (2017). A low-molecular-weight alginate oligosaccharide disrupts pseudomonal microcolony formation and enhances antibiotic effectiveness. Antimicrob. Agents Chemother. 61, e00762–e00717. doi: 10.1128/AAC.00762-17
Rato, L., Alves, M. G., Socorro, S., Duarte, A. I., Cavaco, J. E., and Oliveira, P. F. (2012). Metabolic regulation is important for spermatogenesis. Nat. Rev. Urol. 9, 330–338. doi: 10.1038/nrurol.2012.77
Rato, L., Socorro, S., Cavaco, J., and Oliveira, P. F. (2010). Tubular fluid secretion in the seminiferous epithelium: ion transporters and aquaporins in Sertoli cells. J. Membr. Biol. 236, 215–224. doi: 10.1007/s00232-010-9294-x
Redel, B. K., Spate, L. D., and Prather, R. S. (2019). In vitro maturation, fertilization, and culture of pig oocytes and embryos. Methods Mol. Biol. 2006, 93–103. doi: 10.1007/978-1-4939-9566-0_6
Ren, B., Cheng, X., Wu, D., Xu, S. Y., Che, L. Q., Fang, Z. F., et al. (2015). Effect of different amino acid patterns on semen quality of boars fed with low-protein diets. Anim. Reprod. Sci. 161, 96–103. doi: 10.1016/j.anireprosci.2015.08.010
Ruvinov, E., and Cohen, S. (2016). Alginate biomaterial for the treatment of myocardial infarction: progress, translational strategies, and clinical outlook: from ocean algae to patient bedside. Adv. Drug Deliv. Rev. 96, 54–76. doi: 10.1016/j.addr.2015.04.021
Singh, M., Mollier, R. T., Sharma, R., Kadirvel, G., Doley, S., Sanjukta, R. K., et al. (2021). Dietary flaxseed oil improve boar semen quality, antioxidant status and in-vivo fertility in humid sub-tropical region of north East India. Theriogenology 159, 123–131. doi: 10.1016/j.theriogenology.2020.10.023
Smital, J. (2009). Eff ects infl uencing boar semen. Anim. Reprod. Sci. 110, 335–346. doi: 10.1016/j.anireprosci.2008.01.024
Sun, Z. Y., Yu, S., Tian, Y., Han, B. Q., Zhao, Y., Li, Y. Q., et al. (2022). Chestnut polysaccharides restore impaired spermatogenesis by adjusting gut microbiota and the intestinal structure. Food Funct. 13, 425–436. doi: 10.1039/D1FO03145G
Sun, X. H., Zhu, Y. Y., Wang, L., Liu, H. L., Ling, Y., Li, Z. L., et al. (2017). The Catsper channel and its roles in male fertility: a systematic review. Reprod. Biol. Endocrinol. 15:65. doi: 10.1186/s12958-017-0281-2
Tajima, S., Inoue, H., Kawada, A., Ishibashi, A., Takahara, H., and Hiura, N. (1999). Alginate oligosaccharides modulate cell morphology, cell proliferation and collagen expression in human skin fibroblasts in vitro. Arch. Dermatol. Res. 291, 432–436. doi: 10.1007/s004030050434
Tsakmakidis, I. A., Khalifa, T. A. A., and Boscos, C. M. (2012). Age-related changes in quality and fertility of porcine semen. Biol. Res. 45, 381–386. doi: 10.4067/S0716-97602012000400009
Tsakmakidis, I. A., Lymberopoulos, A. G., and Khalifa, T. A. (2010). Relationship between sperm quality traits and field-fertility of porcine semen. J. Vet. Sci. 11, 151–154. doi: 10.4142/jvs.2010.11.2.151
Tusi, S. K., Khalaj, L., Ashabi, G., Kiaei, M., and Khodagholi, F. (2011). Alginate oligosaccharide protects against endoplasmic reticulum- and mitochondrial-mediated apoptotic cell death and oxidative stress. Biomaterials 32, 5438–5458. doi: 10.1016/j.biomaterials.2011.04.024
Ueno, M., Tamura, Y., Toda, N., Yoshinaga, M., Terakado, S., Otsuka, K., et al. (2012). Sodium alginate oligosaccharides attenuate hypertension in spontaneously hypertensive rats fed a low-salt diet. Clin. Exp. Hypertens. 34, 305–310. doi: 10.3109/10641963.2011.577484
Wan, J., Zhang, J., Chen, D., Yu, B., and He, J. (2017). Effects of alginate oligosaccharide on the growth performance, antioxidant capacity and intestinal digestion-absorption function in weaned pigs. Anim. Feed Sci. Technol. 234, 118–127. doi: 10.1016/j.anifeedsci.2017.09.006
Wan, J., Zhang, J., Chen, D., Yu, B., Huang, Z., Mao, X., et al. (2018b). Alginate oligosaccharide enhances intestinal integrity of weaned pigs through altering intestinal inflammatory responses and antioxidant status. RSC Adv. 8, 13482–13492. doi: 10.1039/C8RA01943F
Wan, J., Zhang, J., Chen, D., Yu, B., Mao, X., Zheng, P., et al. (2018a). Alginate oligosaccharide-induced intestinal morphology, barrier function and epithelium apoptosis modifications have beneficial effects on the growth performance of weaned pigs. J. Anim. Sci. Biotechnol. 9:58. doi: 10.1186/s40104-018-0273-x
WHO (2010). WHO Laboratory Manual for the Examination and Processing of Human Semen. 5th edition. Cambridge, UK: Cambridge University Press.
Wilson, M. E., Rozeboom, K. J., and Crenshaw, T. D. (2004). Boar nutrition for optimum sperm production. Adv. Pork Prod. 15, 295–306.
Wolf, J. (2009). Genetic parameters for semen traits in AI boars estimated from data on individual ejaculates. Reprod. Domest. Anim. 44, 338–344. doi: 10.1111/j.1439-0531.2008.01083.x
Wu, Y. H., Guo, L. L., Liu, Z. H., Wei, H. K., Zhou, Y. F., Tan, J. J., et al. (2019b). Microelements in seminal and serum plasma are associated with fresh semen quality in Yorkshire boars. Theriogenology 132, 88–94. doi: 10.1016/j.theriogenology.2019.04.002
Wu, Y. H., Lai, W., Liu, Z. H., Wei, H. K., Zhou, Y. F., Tan, J. J., et al. (2019a). Serum and seminal plasma element concentrations in relation to semen quality in Duroc boars. Biol. Trace Elem. Res. 189, 85–94. doi: 10.1007/s12011-018-1459-y
Yang, Y., Ma, Z., Yang, G., Wan, J., Li, G., Du, L., et al. (2017). Alginate oligosaccharide indirectly affects toll-like receptor signaling via the inhibition of microrna-29b in aneurysm patients after endovascular aortic repair. Drug Des. Devel. Ther. 11, 2565–2579. doi: 10.2147/DDDT.S140206
Yeste, M., Sancho, S., Briz, M., Pinart, E., Bussalleu, E., and Bonet, S. (2010). A diet supplemented with L-carnitine improves the sperm quality of Pietrain but not of Duroc and large white boars when photoperiod and temperature increase. Theriogenology 73, 577–586. doi: 10.1016/j.theriogenology.2009.10.013
Yu, S., Zhao, Y., Zhang, F. L., Li, Y. Q., Shen, W., and Sun, Z. Y. (2020). Chestnut polysaccharides benefit spermatogenesis through improvement in the expression of important genes. Aging 12, 11431–11445. doi: 10.18632/aging.103205
Zapata-Carmona, H., Soriano-Úbeda, C., París-Oller, E., and Matás, C. (2020). Periovulatory oviductal fluid decreases sperm protein kinase A activity, tyrosine phosphorylation, and in vitro fertilization in pig. Andrology 8, 756–768. doi: 10.1111/andr.12751
Zhang, P., Liu, J., Xiong, B., Zhang, C., Kang, B., Gao, Y., et al. (2020). Microbiota from alginate oligosaccharide dosed mice successfully mitigated small intestinal mucositis. Microbiome 8:112. doi: 10.1186/s40168-020-00886-x
Zhang, W., Zhao, Y., Zhang, P., Hao, Y., Yu, S., Min, L., et al. (2018). Decrease in male mouse fertility by hydrogen sulfide and/or ammonia can be inheritable. Chemosphere 194, 147–157. doi: 10.1016/j.chemosphere.2017.11.164
Zhang, P., Zhao, Y., Zhang, H., Liu, J., Feng, Y., Yin, S., et al. (2019). Low dose Chlorothalonil impairs mouse spermatogenesis through the intertwining of estrogen receptor pathways with histone and DNA methylation. Chemosphere 230, 384–395. doi: 10.1016/j.chemosphere.2019.05.029
Zhao, Y., Feng, Y., Liu, M., Chen, L., Meng, Q., Tang, X., et al. (2020a). Single-cell RNA sequencing analysis reveals alginate oligosaccharides preventing chemotherapy-induced mucositis. Mucosal Immunol. 13, 437–448. doi: 10.1038/s41385-019-0248-z
Zhao, Y., Zhang, P., Ge, W., Feng, Y., Li, L., Sun, Z., et al. (2020b). Alginate oligosaccharides improve germ cell development and testicular microenvironment to rescue busulfan disrupted spermatogenesis. Theranostics 10, 3308–3324. doi: 10.7150/thno.43189
Zhao, Y., Zhang, W., Liu, X., Zhang, P., Hao, Y., Li, L., et al. (2016). Hydrogen sulfide and/or ammonia reduces spermatozoa motility through AMPK/AKT related pathways. Sci. Rep. 6:37884. doi: 10.1038/srep37884
Zhou, J., Ji, T., He, H., Yin, S., Liu, X., Zhang, X., et al. (2020). Induction of autophagy promotes porcine parthenogenetic embryo development under low oxygen conditions. Reprod. Fertil. Dev. 32, 657–666. doi: 10.1071/RD19322
Zhou, R., Shi, X. Y., Gao, Y., Cai, N., Jiang, Z. D., and Xu, X. (2015). Anti-inflammatory activity of guluronate oligosaccharides obtained by oxidative degradation from alginate in lipopolysaccharide-activated murine macrophage RAW 264.7 cells. J. Agric. Food Chem. 63, 160–168. doi: 10.1021/jf503548a
Keywords: boar, alginate oligosaccharides, sperm motility, sperm concentration, in vitro fertilization, metabolism, gut microbiota
Citation: Han H, Zhou Y, Xiong B, Zhong R, Jiang Y, Sun H, Tan J, Zhang B, Guan C, Schroyen M, Chen L, Zhao Y and Zhang H (2022) Alginate oligosaccharides increase boar semen quality by affecting gut microbiota and metabolites in blood and sperm. Front. Microbiol. 13:982152. doi: 10.3389/fmicb.2022.982152
Received: 30 June 2022; Accepted: 08 August 2022;
Published: 22 August 2022.
Edited by:
Man Kit Cheung, The Chinese University of Hong Kong, ChinaReviewed by:
Astghik Zaven Pepoyan, Armenian National Agrarian University, ArmeniaCopyright © 2022 Han, Zhou, Xiong, Zhong, Jiang, Sun, Tan, Zhang, Guan, Schroyen, Chen, Zhao and Zhang. This is an open-access article distributed under the terms of the Creative Commons Attribution License (CC BY). The use, distribution or reproduction in other forums is permitted, provided the original author(s) and the copyright owner(s) are credited and that the original publication in this journal is cited, in accordance with accepted academic practice. No use, distribution or reproduction is permitted which does not comply with these terms.
*Correspondence: Liang Chen, Y2hlbmxpYW5nMDFAY2Fhcy5jbg==; Yong Zhao, eXpoYW84MThAaG90bWFpbC5jb20=; WW9uZy5aaGFvQG11cmRvY2guZWR1LmF1
†These authors have contributed equally to this work
Disclaimer: All claims expressed in this article are solely those of the authors and do not necessarily represent those of their affiliated organizations, or those of the publisher, the editors and the reviewers. Any product that may be evaluated in this article or claim that may be made by its manufacturer is not guaranteed or endorsed by the publisher.
Research integrity at Frontiers
Learn more about the work of our research integrity team to safeguard the quality of each article we publish.