- Key Laboratory of Animal Immunology of the Ministry of Agriculture, Henan Provincial Key Laboratory of Animal Immunology, Henan Academy of Agricultural Sciences, Zhengzhou, China
Porcine reproductive and respiratory syndrome (PRRS), caused by PRRS virus (PRRSV), is a highly contagious disease that brings tremendous economic losses to the global swine industry. As an intracellular obligate pathogen, PRRSV infects specific host cells to complete its replication cycle. PRRSV attachment to and entry into host cells are the first steps to initiate the replication cycle and involve multiple host cellular factors. In this review, we recapitulated recent advances on host cellular factors involved in PRRSV attachment and entry, and reappraised their functions in these two stages, which will deepen the understanding of PRRSV infection and provide insights to develop promising antiviral strategies against the virus.
Introduction
As intracellular obligate pathogens, viruses infect host cells to complete their replication cycles, including attachment, entry, replication, synthesis, assembly, and release (Jones et al., 2021). Attachment and entry are the first and essential steps for viruses to establish infection, which are ideal antiviral targets (Lu et al., 2021; Tompa et al., 2021). Therefore, an in-depth investigation of viral attachment and entry will provide novel insights to develop potent antiviral strategies.
Porcine reproductive and respiratory syndrome (PRRS) is a highly contagious swine disease characterized by reproductive failures in sows of late-term gestation and respiratory diseases in pigs of all ages (Done and Paton, 1995; Rossow, 1998). Since its emergence in the late 1980s, PRRS keeps burdening the global swine industry with an annual economic loss of up to $664 million in the United States (Holtkamp et al., 2013). PRRS virus (PRRSV), as the causative agent, is an enveloped single-stranded positive-sense RNA virus and belongs to the order Nidovirales, family Arteriviridae, and genus Betaarterivirus.1
Infection by PRRSV shows a strongly restricted tropism for target cells, including porcine alveolar macrophages (PAMs) in vivo (Duan et al., 1997), and African green monkey kidney epithelial cell line MA-104 and its derivative MARC-145 in vitro (Kim et al., 1993). Multiple host cellular factors have been reported to be involved in PRRSV attachment to and entry into these target cells (Shi et al., 2015; Zhang and Yoo, 2015), such as heparan sulfate (HS) (Delputte et al., 2002), sialoadhesin (Sn/CD169) (Vanderheijden et al., 2003), vimentin (Kim et al., 2006), CD163 (Calvert et al., 2007), CD151 (Shanmukhappa et al., 2007), dendritic cell-specific intercellular adhesion molecule-3-grabbing non-integrin (DC-SIGN/CD209) (Huang et al., 2009).
HS, Sn, and CD163 have been intensively studied during PRRSV infection. According to a previous model (Van Breedam et al., 2010a), PRRSV firstly bound to HS on the target cell surface (Jusa et al., 1997) and subsequently interacted with Sn to be internalized via low pH-dependent clathrin-mediated endocytosis (Kreutz and Ackermann, 1996; Nauwynck et al., 1999; Delputte et al., 2005). During internalization, PRRSV was associated with CD163, which was considered to cooperate with Sn in facilitating viral internalization (Van Gorp et al., 2008) as well as to mediate viral membrane fusion and uncoating (Yu et al., 2019).
However, this model is challenged based on recent studies of PRRSV infection. For example, Sn knockout pigs are susceptible to PRRSV, demonstrating that it is not required for PRRSV attachment and internalization (Prather et al., 2013). In addition to attachment and entry, HS and vimentin are shown to participate in other stages of PRRSV replication cycle (Song et al., 2016; Guo et al., 2017a; Chang et al., 2018; Zheng et al., 2021). Moreover, several novel host cellular factors are identified to be involved in PRRSV attachment and entry, such as non-muscle myosin heavy chain 9 (MYH9) (Gao et al., 2016), syndecan-4 (Wang et al., 2016a), epidermal growth factor receptor (EGFR) (Wang et al., 2016b), T-cell immunoglobulin and mucin domain (TIM)-1/4 (Wei et al., 2020), and heat shock protein member 8 (HSPA8) (Wang et al., 2022).
In this review, we summarized recent advances on host cellular factors involved in PRRSV attachment and entry, and re-evaluated their roles in PRRSV infection in the hope of supporting ideas for developing antiviral strategies against the virus (Table 1).
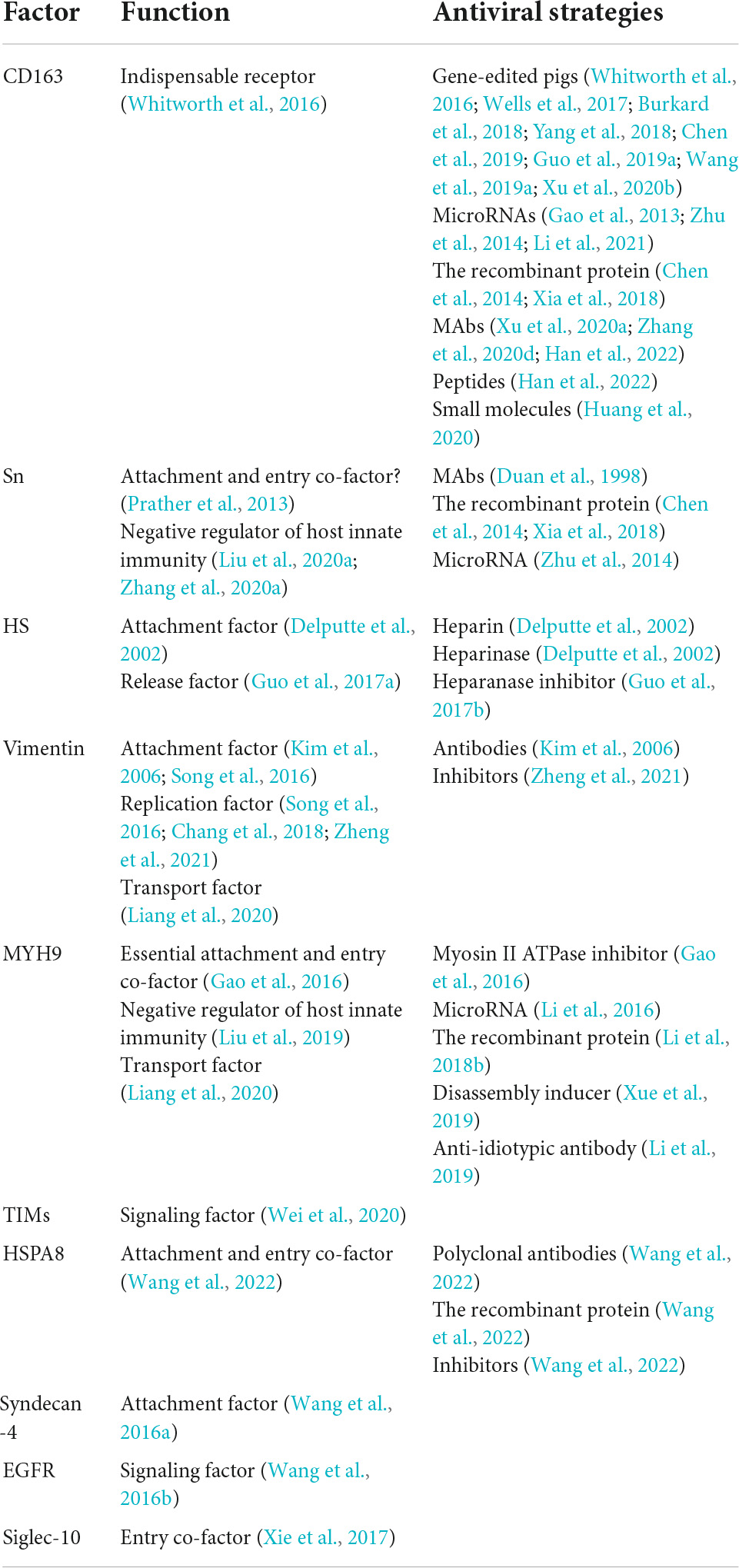
Table 1. Functional reappraisal of and antiviral strategies against host cellular factors involved in PRRSV attachment and entry.
Previously identified host cellular factors
CD163
CD163 is a member of class I scavenger receptors (SRs) (Law et al., 1993; Zani et al., 2015). It is a type I membrane glycoprotein consisting of nine SR cysteine-rich (SRCR) domains (SRCR1-9) and two proline-serine-threonine (PST)-rich motifs (PST I and II) in its large extracellular region, a single transmembrane domain and a short cytoplasmic tail (Van Gorp et al., 2010a). CD163 plays critical roles under physiological and pathological conditions, such as hemoglobin-haptoglobin SR (Kristiansen et al., 2001), erythroblast adhesion receptor (Fabriek et al., 2007), and TWEAK SR (Bover et al., 2007; Moreno et al., 2009; Akahori et al., 2015). CD163 functions as an innate immune sensor for bacteria (Fabriek et al., 2009) and an anti-inflammatory receptor for HMGB1-haptoglobin complexes (Yang et al., 2016). Moreover, it is a receptor for simian hemorrhagic fever virus (Caì et al., 2015) and PRRSV (Welch and Calvert, 2010).
CD163 expression renders various non-permissive cells susceptible to PRRSV infection (Calvert et al., 2007; Lee et al., 2010; Wang et al., 2013, 2019c; Li et al., 2017; Xu et al., 2020c). CD163 knockout pigs are fully resistant to PRRSV, confirming that it is an indispensable receptor for PRRSV (Whitworth et al., 2016; Yang et al., 2018; Xu et al., 2020b). CD163 SRCR5 domain is further demonstrated to be crucial for PRRSV infection both in vitro and in vivo (Van Gorp et al., 2010b; Burkard et al., 2017, 2018; Wells et al., 2017; Chen et al., 2019; Guo et al., 2019a; Wang et al., 2019a). Consequently, CD163 is the most suitable target for preventing and controlling PRRS.
First of all, modulation of CD163 expression is effective in restraining PRRSV infection. As described above, gene editing via CRISPR-Cas9 has been applied to breed pigs lacking functional CD163, which confers resistance to PRRSV (Prather et al., 2017; Whitworth and Prather, 2017; Tu et al., 2022). However, considering its significant physiological roles, pigs with deletion or partial deletion of CD163 SRCR5 domain are probably superior to those lacking intact CD163 (Reiner, 2016). Down-regulation of CD163 expression by microRNAs also inhibits PRRSV infection in vitro (Gao et al., 2013; Zhu et al., 2014; Li et al., 2021). With progress in efficient delivery techniques (Momin et al., 2021), microRNAs targeting CD163 can be utilized as in vivo anti-PRRSV reagents. In addition to direct knockout or knockdown, CD163 upstream regulators can be targeted to modulate its expression. For instance, a disintegrin and metalloprotease 17 (ADAM17) down-regulates CD163 expression and hinders PRRSV entry in vitro (Guo et al., 2014b; Zhu et al., 2020), and therefore ADAM17 overexpression via genetic modification methods may enhance resistance to PRRSV infection in vivo.
CD163 itself can be exploited to restrict PRRSV infection. On the one hand, the recombinant adenovirus-delivered soluble CD163 SRCR5-9 protein has been shown to suppress PRRSV infection both in vitro and in vivo (Chen et al., 2014; Xia et al., 2018). On the other hand, specific monoclonal antibodies (mAbs) targeting CD163 SRCR5, SRCR6, SRCR7, or PST I domain have been recently reported to inhibit PRRSV infection in vitro (Xu et al., 2020a; Zhang et al., 2020d; Han et al., 2022). In one study, peptides derived from the epitopes bound by the mAbs display inhibitory effect on PRRSV infection in a dose-dependent manner (Han et al., 2022). Since CD163 does not mutate as quickly as PRRSV, the recombinant CD163 protein along with anti-CD163 mAbs and peptides are promising to be developed as broad-spectrum therapeutic agents against different PRRSV isolates.
The crystal structure of the CD163 SRCR5 domain determined by us greatly facilitates precise control and prevention of PRRS from the receptor perspective (Ma et al., 2017, 2021). Site-directed mutagenesis of the CD163 SRCR5 domain will be beneficial for breeding gene-edited pigs resistant to PRRSV while maintaining CD163 biological functions (Stoian et al., 2022). Based on the crystal structure, a set of small molecule compounds targeting CD163 SRCR5 have been screened through artificial intelligence molecular screening and validated against PRRSV infection in vitro (Huang et al., 2020).
Sialoadhesin
Sn belongs to the sialic acid-binding Ig-like lectin (Siglec) family, namely Siglec-1. It is a macrophage-restricted molecule with an extracellular domain consisting of one N-terminal V-set Ig-like domain and 16 C2-set domains, a transmembrane domain, and a short cytoplasmic tail (Crocker et al., 1994; Hartnell et al., 2001). Sn was first identified as a sialic acid-dependent sheep erythrocyte receptor and subsequently shown to participate in other physiological or pathological processes (O’Neill et al., 2013).
A prepared mAb was characterized to block PRRSV infection in PAMs and afterward identified to recognize Sn (Duan et al., 1998). Sn was further found to take part in PRRSV attachment and internalization via interaction with PRRSV glycoprotein (GP) 5 dependent on its sialic acid-binding activity of V-set Ig-like domain in vitro (Vanderheijden et al., 2003; Delputte and Nauwynck, 2004; Delputte et al., 2007; An et al., 2010; Van Breedam et al., 2010b,2013; Jiang et al., 2013). However, the involvement of Sn in PRRSV attachment and entry is mired in controversy for MA-104 and MARC-145 cell lines permissive to PRRSV possess no Sn, whereas CD163 by itself is capable of mediating PRRSV infection (Kim et al., 1993; Wang et al., 2013). Sn knockout pigs further prove that Sn is dispensable for PRRSV attachment and internalization in vivo (Prather et al., 2013).
Interestingly, co-expression of Sn and CD163 in non-permissive cells significantly enhances PRRSV infection compared to the expression of CD163 alone (Van Gorp et al., 2008). Besides, additive anti-PRRSV effects are observed with simultaneous administration of recombinant Sn and CD163 proteins or Sn- and CD163-targeted microRNAs (Chen et al., 2014; Zhu et al., 2014; Xia et al., 2018). These results suggest that Sn may function differently as an indispensable receptor during PRRSV infection.
Sn has been shown to antagonize antiviral immune responses as other Siglecs (Crocker et al., 2007; Zheng et al., 2015; Akiyama et al., 2017). A recent study unravels that Sn suppresses host innate immunity by down-regulating antiviral cytokine production during PRRSV infection (Zhang et al., 2020a). We further elaborate that Sn interacts with the immune adaptor DNAX-activation protein of 12 kDa (DAP12) to attenuate PRRSV-triggered nuclear factor kappa B (NF-κB) activation and negatively regulate host antiviral innate immune (Liu et al., 2020a,b).
Despite its controversial role in PRRSV infection, Sn is still expected to be conducive to PRRSV eradication in pigs because the adenovirus-delivered recombinant Sn protein additively protects pigs from PRRSV along with CD163 (Xia et al., 2018).
Heparan sulfate
HS is a linear, unbranched, negatively charged polysaccharide attached to various cell surface or extracellular matrix proteins. It mediates cellular signaling, maintains homeostasis, and regulates cellular growth and metabolism (Ling et al., 2022). At first, HS was found bound by PRRSV matrix protein during viral attachment to PAMs (Delputte et al., 2002, 2005). Various viruses exploit HS for attachment to host cells (Agelidis and Shukla, 2020), including severe acute respiratory syndrome virus 2 (SARS-CoV-2) (Clausen et al., 2020; Zhang et al., 2020b; Chu et al., 2021).
As HS usually functions as the first attachment factor to concentrate virus particles on the target cell surface, interference with the interaction between HS and viruses is supposed to be a potential antiviral approach (Cagno et al., 2019; Cheudjeu, 2021). PRRSV treated with heparin or PAMs treated with heparinase resulted in a significant reduction in viral infection (Delputte et al., 2002). One study also shows a strong anti-SARS-CoV-2 activity by heparin (Tandon et al., 2021).
In 2017, Guo et al. (2017a) showed that HS expression on the cell surface was down-regulated by heparanase to facilitate viral release. Heparanase was up-regulated by PRRSV during the late-stage infection, where heparanase knockdown suppressed PRRSV release while its overexpression enhanced. Inhibition of heparanase by pyrithione, a zinc ionophore used as an antibacterial and antifungal agent, has been shown to block PRRSV release (Guo et al., 2017b). A recent study also determines heparanase as a potential target for SARS-CoV-2 for a heparanase inhibitor Roneparstat (in phase I clinical trial for multiple myeloma therapy) reduces viral infection (Xiang et al., 2022).
Based on these results, both HS and heparanase can be targeted to interfere with PRRSV attachment and release.
Vimentin
Vimentin is a major component of class-III intermediate filaments, which stabilizes the cytoskeleton and maintains cell integrity (Goldman et al., 1996). Vimentin was previously shown to bind to PRRSV nucleocapsid (N) protein, and anti-vimentin antibodies were found to block PRRSV infection in MARC-145 cells (Kim et al., 2006). Delivery of the recombinant simian vimentin was further indicated to render non-susceptible cell lines susceptible to PRRSV (Kim et al., 2006). Next, vimentin was identified to form a complex with PRRSV non-structural protein 2 and N protein that may be essential for viral attachment and replication (Song et al., 2016). Afterward, vimentin was revealed to bind to Annexin A2 and contribute to PRRSV multiplication (Chang et al., 2018). We have recently demonstrated that vimentin reorganizes into cage-like structures enwrapping the PRRSV replication complex during the post-entry stage and is beneficial for PRRSV replication in vitro. PRRSV replication is significantly lowered by either 3, 3′-iminodipropionitrile to inhibit vimentin dynamics and network or a specific inhibitor KN-93 targeting calcium calmodulin-dependent protein kinase II gamma responsible for vimentin rearrangement (Zheng et al., 2021). Moreover, PRRSV particles are visualized to move along vimentin during intracellular transport (Liang et al., 2020).
Increasing studies corroborate vimentin as a promising antiviral target due to its diverse functions in viral replication cycles (Ramos et al., 2020; Zhang et al., 2020c). Consequently, vimentin is appropriately targeted for developing antibodies and chemical inhibitors against PRRSV since it affects viral attachment, replication, and transport.
Newly identified host cellular factors
Non-muscle myosin heavy chain 9
MYH9, also referred to as non-muscle myosin heavy chain IIA (NMHC-IIA), is a subunit of non-muscle myosin IIA (NM-IIA) (Vicente-Manzanares et al., 2009). MYH9 participates in various cellular physiological processes, including cell shape maintenance, adhesion, migration, signal transduction, and division (Heissler and Manstein, 2013; Pecci et al., 2018).
It has been verified that MYH9 is an essential factor for PRRSV infection via interaction with PRRSV GP5 dependent on its C-terminal domain by Professor Zhou’s group (Gao et al., 2016). They demonstrate that the MYH9 C-terminal domain interacts with the CD163 SRCR1-4 domains to facilitate PRRSV internalization in permissive cells (Hou et al., 2019). They further define that the MYH9 C-terminal domain was directly bound by the PRRSV GP5 first ectodomain which induces MYH9 aggregation and polymerization required for PRRSV internalization (Xue et al., 2019). Later, the group identified MYH9 key residues involved in PRRSV internalization by a specific anti-idiotypic antibody Mab2-5G2 to the viral GP5 (Li et al., 2019). Based on these results, MYH9 is demonstrated to be essential for PRRSV attachment and entry.
On the other hand, we uncover that MYH9 recognizes sialic acids on PRRSV GP5 and interacts with DAP12 to activate downstream spleen tyrosine kinase (Syk), resulting in antagonized antiviral pro-inflammatory responses. More importantly, the MYH9-DAP12-Syk pathway plays a negative regulatory role in pro-inflammatory responses upon recognizing sialylated RNA viruses or sialic acid mimics (Liu et al., 2019).
As mentioned above, PRRSV particles also contact MYH9 during intracellular transport along with other cytoskeleton components (Liang et al., 2020).
These extensive studies on MYH9 are helpful for the development of control strategies against PRRSV. The specific myosin II ATPase inhibitor blebbistatin inhibits PRRSV infection in vitro and in vivo demonstrated by Professor Zhou’s group (Gao et al., 2016). MicroRNA let-7f-5p is reported to significantly suppress PRRSV replication by lowering MYH9 expression (Li et al., 2016). Their data also show that pre-incubation of PRRSV with the MYH9 C-terminal domain suppresses viral infection in susceptible cells in a dose-dependent manner, suggesting that it may serve as a novel anti-PRRSV agent in vivo (Li et al., 2018b). Moreover, overexpression of MYH9-specific disassembly inducer S100A4 remarkably leads to diminished MYH9 aggregation and decreased PRRSV internalization in MARC-145 cells (Xue et al., 2019). The anti-idiotypic antibody Mab2-5G2 is demonstrated to diminish PRRSV internalization in PAMs via interruption of direct interaction between MYH9 and GP5, which may act as another antiviral agent against the virus in pigs (Li et al., 2019). Our finding of MYH9 as a negative regulator of inflammation provides a molecular basis to design anti-inflammatory drugs against highly pathogenic (HP)-PRRSV (Liu et al., 2019), which causes aberrant pro-inflammatory responses, high fever, morbidity, and mortality in pigs (Qiao et al., 2011; Han et al., 2017).
T-cell immunoglobulin and mucin domain-1/4 and heat shock protein member 8
In 2020, our group demonstrated that PRRSV externalizes phosphatidylserine (PS) on the envelope as viral apoptotic mimicry, which is recognized by PS receptor TIM-1 or TIM-4 to trigger the downstream signaling pathway and macropinocytosis as an alternative entry pathway for PRRSV into MARC-145 cells and PAMs, respectively (Wei et al., 2020).
In this year, our group identified that HSPA8, a housekeeping chaperone, interacts with PRRSV GP4, and is involved in PRRSV attachment and internalization for the first time. Anti-HSPA8 polyclonal antibodies, inhibitors, and the recombinant soluble HSPA8 protein inhibit PRRSV infection in vitro (Wang et al., 2022).
Our findings enrich novel host cellular factors involved in PRRSV attachment and entry, and support them as potential antiviral targets against PRRSV infection.
Syndecan-4 and epidermal growth factor receptor
Syndecans are a family of transmembrane HS proteoglycans and are involved in human cancers, infectious diseases, obesity, wound healing, and angiogenesis. In addition, syndecans act as receptors/co-receptors for viral infections (Fears and Woods, 2006). Wang et al. (2016a) found that syndecan-4 played a critical role in PRRSV attachment and entry in MARC-145 cells. They further found that syndecan-4 interacted with EGFR during PRRSV entry.
EGFR is a member of the ErbB family of receptor tyrosine kinases and a versatile signal transducer involved in various cellular processes. EGFR has also been exploited by various viruses during different stages (Carlin, 2021; Lai and Lee, 2022). Ni et al. (2015) and Wang et al. (2016b) demonstrated that EGFR was activated to initiate its downstream signal pathways, and modulated actin fragmentation and reorganization to facilitate PRRSV entry.
Based on their findings, we speculate that syndecan-4 functions as an alternative attachment factor, whereas EGFR is a signaling factor to mobilize the cytoskeleton for PRRSV entry. As its commercial targeted drugs are available, including inhibitors and mAbs (Yamaoka et al., 2017), EGFR may be a potential therapeutic target for controlling PRRSV infection.
Sialic acid-binding Ig-like lectin-10
Xie et al. (2017) identified Siglec-10 as an alternative factor for PRRSV entry. They further stated preferential use of Sn or Siglec-10 by different PRRSV isolates (Xie et al., 2018). These studies suggest the utilization of several redundant Siglecs by PRRSV. The importance of Siglec-10 needs to be verified in vivo in the future.
Perspectives and concluding remarks
It has long been acknowledged that host cellular factors involved in attachment and entry are the major determinants for PRRSV infection (Kreutz, 1998). In particular, CD163 is a well-documented indispensable receptor for PRRSV and, therefore, the optimal antiviral target (Su et al., 2021). CD163-targeted genetic editing, microRNAs, mAbs, inhibitors, proteins, and peptides support various potent antiviral tools against PRRSV. Despite the great successes of CD163-edited pigs in resistance to PRRSV, their clinical performances need continuous monitoring in consideration of the multifaceted functions of the receptor. In addition to CD163 and Sn, other reported host cellular factors require further in vivo validation of their involvement in PRRSV attachment and entry to evaluate whether they are qualified as antiviral targets. Moreover, it cannot be ruled out that there are unrecognized host cellular factors or even co-receptors for PRRSV attachment and entry.
PRRSV attachment and entry are initiated by the interactions between the viral envelope proteins and host cellular receptors/factors (Tian et al., 2012; Veit et al., 2014). However, it remains obscure which PRRSV envelope proteins are responsible for binding to the indispensable receptor CD163 (Das et al., 2010, 2011; Du et al., 2012; Wei et al., 2012). Our study showed that PRRSV GP5 was cleaved during viral membrane fusion (Hou et al., 2020). Whether it functions as a viral fusion protein needs further demonstration. As vaccination is another effective strategy to prevent and control PRRS, addressing these conundrums will clarify vaccine antigens and accelerate vaccine development (Stoian and Rowland, 2019).
The importance of antiviral drugs is increasingly apparent owing to PRRSV persistence in pigs. There are a growing number of antiviral agents reported interfering with PRRSV attachment or/and entry, including antimicrobial peptides protegrin-1 (Guo et al., 2015), cecropin P1 (Guo et al., 2014a), cecropin D (Liu et al., 2015), lavaspidic acid AB (Yang et al., 2013), glycyrrhizin (Duan et al., 2015), tetrahydroaltersolanol C (Zhang et al., 2016), curcumin (Du et al., 2017), griffithsin (Li et al., 2018a), iota-carrageenan (Guo et al., 2019b), polyethylenimine (Wang et al., 2019b), 25-hydroxycholesterol (in vitro and in vivo) (Ke et al., 2017; Song et al., 2017, 2019; Dong et al., 2018) and rottlerin (in vitro and in vivo) (Kang et al., 2021). Chinese herbal medicines also contain antiviral molecules to block PRRSV attachment and entry (Bello-Onaghise et al., 2020). Unfortunately, the specific targets of these antiviral agents have not yet been identified. A bigger concern is that the antiviral efficacies of the majority of these antiviral agents have not been validated in vivo unless otherwise stated. However, developing anti-PRRSV drugs remains a promising therapeutic strategy. It would be resultful to develop specific antiviral drugs blocking crucial host cellular factors, e.g., CD163, involved in PRRSV attachment and entry.
In conclusion, recent advances on host cellular factors involved in PRRSV attachment and entry have laid a strong basis for developing multi-target antiviral strategies. Intensive investigation in this field is still necessary to elucidate PRRSV infection, which is beneficial for preventing and controlling PRRS.
Author contributions
RL recapitulated recent advances on host cellular factors involved in PRRSV attachment and entry and wrote the review. SQ and GZ provided guidance and modified the review. All authors contributed to the article and approved the submitted version.
Funding
This work was supported by National Natural Science Foundation of China (31972690) and Henan Academy of Agricultural Sciences (2021JQ01 and 2022ZC66).
Conflict of interest
The authors declare that the research was conducted in the absence of any commercial or financial relationships that could be construed as a potential conflict of interest.
Publisher’s note
All claims expressed in this article are solely those of the authors and do not necessarily represent those of their affiliated organizations, or those of the publisher, the editors and the reviewers. Any product that may be evaluated in this article, or claim that may be made by its manufacturer, is not guaranteed or endorsed by the publisher.
Footnotes
References
Agelidis, A., and Shukla, D. (2020). Heparanase, heparan sulfate and viral infection. Adv. Exp. Med. Biol. 1221, 759–770. doi: 10.1007/978-3-030-34521-1_32
Akahori, H., Karmali, V., Polavarapu, R., Lyle, A. N., Weiss, D., Shin, E., et al. (2015). CD163 interacts with TWEAK to regulate tissue regeneration after ischaemic injury. Nat. Commun. 6:7792. doi: 10.1038/ncomms8792
Akiyama, H., Ramirez, N. P., Gibson, G., Kline, C., Watkins, S., Ambrose, Z., et al. (2017). Interferon-inducible CD169/Siglec1 attenuates anti-HIV-1 effects of alpha interferon. J. Virol. 91:e00972–17. doi: 10.1128/jvi.00972-17
An, T. Q., Tian, Z. J., He, Y. X., Xiao, Y., Jiang, Y. F., Peng, J. M., et al. (2010). Porcine reproductive and respiratory syndrome virus attachment is mediated by the N-terminal domain of the sialoadhesin receptor. Vet. Microbiol. 143, 371–378. doi: 10.1016/j.vetmic.2009.11.006
Bello-Onaghise, G., Wang, G., Han, X., Nsabimana, E., Cui, W., Yu, F., et al. (2020). Antiviral strategies of Chinese herbal medicine against PRRSV infection. Front. Microbiol. 11:1756. doi: 10.3389/fmicb.2020.01756
Bover, L. C., Cardó-Vila, M., Kuniyasu, A., Sun, J., Rangel, R., Takeya, M., et al. (2007). A previously unrecognized protein-protein interaction between TWEAK and CD163: potential biological implications. J. Immunol. 178, 8183–8194. doi: 10.4049/jimmunol.178.12.8183
Burkard, C., Lillico, S. G., Reid, E., Jackson, B., Mileham, A. J., Ait-Ali, T., et al. (2017). Precision engineering for PRRSV resistance in pigs: macrophages from genome edited pigs lacking CD163 SRCR5 domain are fully resistant to both PRRSV genotypes while maintaining biological function. PLoS Pathog. 13:e1006206. doi: 10.1371/journal.ppat.1006206
Burkard, C., Opriessnig, T., Mileham, A. J., Stadejek, T., Ait-Ali, T., Lillico, S. G., et al. (2018). Pigs lacking the scavenger receptor cysteine-rich domain 5 of CD163 are resistant to porcine reproductive and respiratory syndrome virus 1 infection. J. Virol. 92:e415–e418. doi: 10.1128/jvi.00415-18
Cagno, V., Tseligka, E. D., Jones, S. T., and Tapparel, C. (2019). Heparan sulfate proteoglycans and viral attachment: true receptors or adaptation bias? Viruses 11:596. doi: 10.3390/v11070596
Caì, Y., Postnikova, E. N., Bernbaum, J. G., Yú, S. Q., Mazur, S., Deiuliis, N. M., et al. (2015). Simian hemorrhagic fever virus cell entry is dependent on CD163 and uses a clathrin-mediated endocytosis-like pathway. J. Virol. 89, 844–856. doi: 10.1128/jvi.02697-14
Calvert, J. G., Slade, D. E., Shields, S. L., Jolie, R., Mannan, R. M., Ankenbauer, R. G., et al. (2007). CD163 expression confers susceptibility to porcine reproductive and respiratory syndrome viruses. J. Virol. 81, 7371–7379. doi: 10.1128/jvi.00513-07
Carlin, C. R. (2021). Role of EGF Receptor Regulatory Networks in the Host Response to Viral Infections. Front. Cell. Infect. Microbiol. 11:820355. doi: 10.3389/fcimb.2021.820355
Chang, X. B., Yang, Y. Q., Gao, J. C., Zhao, K., Guo, J. C., Ye, C., et al. (2018). Annexin A2 binds to vimentin and contributes to porcine reproductive and respiratory syndrome virus multiplication. Vet. Res. 49:75. doi: 10.1186/s13567-018-0571-5
Chen, J., Wang, H., Bai, J., Liu, W., Liu, X., Yu, D., et al. (2019). Generation of pigs resistant to highly pathogenic-porcine reproductive and respiratory syndrome virus through gene editing of CD163. Int. J. Biol. Sci. 15, 481–492. doi: 10.7150/ijbs.25862
Chen, Y., Guo, R., He, S., Zhang, X., Xia, X., and Sun, H. (2014). Additive inhibition of porcine reproductive and respiratory syndrome virus infection with the soluble sialoadhesin and CD163 receptors. Virus Res. 179, 85–92. doi: 10.1016/j.virusres.2013.11.008
Cheudjeu, A. (2021). Antiviral strategies should focus on stimulating the biosynthesis of heparan sulfates, not their inhibition. Life Sci. 277:119508. doi: 10.1016/j.lfs.2021.119508
Chu, H., Hu, B., Huang, X., Chai, Y., Zhou, D., Wang, Y., et al. (2021). Host and viral determinants for efficient SARS-CoV-2 infection of the human lung. Nat. Commun. 12:134. doi: 10.1038/s41467-020-20457-w
Clausen, T. M., Sandoval, D. R., Spliid, C. B., Pihl, J., Perrett, H. R., Painter, C. D., et al. (2020). SARS-CoV-2 infection depends on cellular heparan sulfate and ACE2. Cell 183, 1043–1057.e15. doi: 10.1016/j.cell.2020.09.033
Crocker, P. R., Mucklow, S., Bouckson, V., McWilliam, A., Willis, A. C., Gordon, S., et al. (1994). Sialoadhesin, a macrophage sialic acid binding receptor for haemopoietic cells with 17 immunoglobulin-like domains. EMBO J. 13, 4490–4503.
Crocker, P. R., Paulson, J. C., and Varki, A. (2007). Siglecs and their roles in the immune system. Nat. Rev. Immunol. 7, 255–266. doi: 10.1038/nri2056
Das, P. B., Dinh, P. X., Ansari, I. H., de Lima, M., Osorio, F. A., and Pattnaik, A. K. (2010). The minor envelope glycoproteins GP2a and GP4 of porcine reproductive and respiratory syndrome virus interact with the receptor CD163. J. Virol. 84, 1731–1740. doi: 10.1128/jvi.01774-09
Das, P. B., Vu, H. L., Dinh, P. X., Cooney, J. L., Kwon, B., Osorio, F. A., et al. (2011). Glycosylation of minor envelope glycoproteins of porcine reproductive and respiratory syndrome virus in infectious virus recovery, receptor interaction, and immune response. Virology 410, 385–394. doi: 10.1016/j.virol.2010.12.002
Delputte, P. L., Costers, S., and Nauwynck, H. J. (2005). Analysis of porcine reproductive and respiratory syndrome virus attachment and internalization: distinctive roles for heparan sulphate and sialoadhesin. J. Gen. Virol. 86, 1441–1445. doi: 10.1099/vir.0.80675-0
Delputte, P. L., and Nauwynck, H. J. (2004). Porcine arterivirus infection of alveolar macrophages is mediated by sialic acid on the virus. J. Virol. 78, 8094–8101. doi: 10.1128/jvi.78.15.8094-8101.2004
Delputte, P. L., Van Breedam, W., Delrue, I., Oetke, C., Crocker, P. R., and Nauwynck, H. J. (2007). Porcine arterivirus attachment to the macrophage-specific receptor sialoadhesin is dependent on the sialic acid-binding activity of the N-terminal immunoglobulin domain of sialoadhesin. J. Virol. 81, 9546–9550. doi: 10.1128/jvi.00569-07
Delputte, P. L., Vanderheijden, N., Nauwynck, H. J., and Pensaert, M. B. (2002). Involvement of the matrix protein in attachment of porcine reproductive and respiratory syndrome virus to a heparinlike receptor on porcine alveolar macrophages. J. Virol. 76, 4312–4320. doi: 10.1128/jvi.76.9.4312-4320.2002
Done, S. H., and Paton, D. J. (1995). Porcine reproductive and respiratory syndrome: clinical disease, pathology and immunosuppression. Vet. Rec. 136, 32–35. doi: 10.1136/vr.136.2.32
Dong, H., Zhou, L., Ge, X., Guo, X., Han, J., and Yang, H. (2018). Antiviral effect of 25-hydroxycholesterol against porcine reproductive and respiratory syndrome virus in vitro. Antivir. Ther. 23, 395–404. doi: 10.3851/imp3232
Du, T., Shi, Y., Xiao, S., Li, N., Zhao, Q., Zhang, A., et al. (2017). Curcumin is a promising inhibitor of genotype 2 porcine reproductive and respiratory syndrome virus infection. BMC Vet. Res. 13:298. doi: 10.1186/s12917-017-1218-x
Du, Y., Pattnaik, A. K., Song, C., Yoo, D., and Li, G. (2012). Glycosyl-phosphatidylinositol (GPI)-anchored membrane association of the porcine reproductive and respiratory syndrome virus GP4 glycoprotein and its co-localization with CD163 in lipid rafts. Virology 424, 18–32. doi: 10.1016/j.virol.2011.12.009
Duan, E., Wang, D., Fang, L., Ma, J., Luo, J., Chen, H., et al. (2015). Suppression of porcine reproductive and respiratory syndrome virus proliferation by glycyrrhizin. Antivir. Res. 120, 122–125. doi: 10.1016/j.antiviral.2015.06.001
Duan, X., Nauwynck, H. J., Favoreel, H. W., and Pensaert, M. B. (1998). Identification of a putative receptor for porcine reproductive and respiratory syndrome virus on porcine alveolar macrophages. J. Virol. 72, 4520–4523. doi: 10.1128/jvi.72.5.4520-4523.1998
Duan, X., Nauwynck, H. J., and Pensaert, M. B. (1997). Virus quantification and identification of cellular targets in the lungs and lymphoid tissues of pigs at different time intervals after inoculation with porcine reproductive and respiratory syndrome virus (PRRSV). Vet. Microbiol. 56, 9–19. doi: 10.1016/s0378-1135(96)01347-8
Fabriek, B. O., Polfliet, M. M., Vloet, R. P., van der Schors, R. C., Ligtenberg, A. J., Weaver, L. K., et al. (2007). The macrophage CD163 surface glycoprotein is an erythroblast adhesion receptor. Blood 109, 5223–5229. doi: 10.1182/blood-2006-08-036467
Fabriek, B. O., van Bruggen, R., Deng, D. M., Ligtenberg, A. J., Nazmi, K., Schornagel, K., et al. (2009). The macrophage scavenger receptor CD163 functions as an innate immune sensor for bacteria. Blood 113, 887–892. doi: 10.1182/blood-2008-07-167064
Fears, C. Y., and Woods, A. (2006). The role of syndecans in disease and wound healing. Matrix Biol. 25, 443–456. doi: 10.1016/j.matbio.2006.07.003
Gao, J., Xiao, S., Xiao, Y., Wang, X., Zhang, C., Zhao, Q., et al. (2016). MYH9 is an essential factor for porcine reproductive and respiratory syndrome virus infection. Sci. Rep. 6:25120. doi: 10.1038/srep25120
Gao, L., Guo, X. K., Wang, L., Zhang, Q., Li, N., Chen, X. X., et al. (2013). MicroRNA 181 suppresses porcine reproductive and respiratory syndrome virus (PRRSV) infection by targeting PRRSV receptor CD163. J. Virol. 87, 8808–8812. doi: 10.1128/jvi.00718-13
Goldman, R. D., Khuon, S., Chou, Y. H., Opal, P., and Steinert, P. M. (1996). The function of intermediate filaments in cell shape and cytoskeletal integrity. J. Cell Biol. 134, 971–983. doi: 10.1083/jcb.134.4.971
Guo, C., Cong, P., He, Z., Mo, D., Zhang, W., Chen, Y., et al. (2015). Inhibitory activity and molecular mechanism of protegrin-1 against porcine reproductive and respiratory syndrome virus in vitro. Antivir. Ther. 20, 573–582. doi: 10.3851/imp2918
Guo, C., Huang, Y., Cong, P., Liu, X., Chen, Y., and He, Z. (2014a). Cecropin P1 inhibits porcine reproductive and respiratory syndrome virus by blocking attachment. BMC Microbiol. 14:273. doi: 10.1186/s12866-014-0273-8
Guo, L., Niu, J., Yu, H., Gu, W., Li, R., Luo, X., et al. (2014b). Modulation of CD163 expression by metalloprotease ADAM17 regulates porcine reproductive and respiratory syndrome virus entry. J. Virol. 88, 10448–10458. doi: 10.1128/jvi.01117-14
Guo, C., Wang, M., Zhu, Z., He, S., Liu, H., Liu, X., et al. (2019a). Highly efficient generation of pigs harboring a partial deletion of the CD163 SRCR5 domain, which are fully resistant to porcine reproductive and respiratory syndrome virus 2 infection. Front. Immunol. 10:1846. doi: 10.3389/fimmu.2019.01846
Guo, C., Zhu, Z., Yu, P., Zhang, X., Dong, W., Wang, X., et al. (2019b). Inhibitory effect of iota-carrageenan on porcine reproductive and respiratory syndrome virus in vitro. Antivir. Ther. 24, 261–270. doi: 10.3851/imp3295
Guo, C., Zhu, Z., Guo, Y., Wang, X., Yu, P., Xiao, S., et al. (2017a). Heparanase upregulation contributes to porcine reproductive and respiratory syndrome virus release. J. Virol. 91:e00625–17. doi: 10.1128/jvi.00625-17
Guo, C., Zhu, Z., Wang, X., Chen, Y., and Liu, X. (2017b). Pyrithione inhibits porcine reproductive and respiratory syndrome virus replication through interfering with NF-κB and heparanase. Vet. Microbiol. 201, 231–239. doi: 10.1016/j.vetmic.2017.01.033
Han, G., Yang, H., Xu, H., Zheng, S., Li, Y., Liu, Z., et al. (2022). Broad antiviral peptides against PRRSV based on novel linear epitopes on porcine CD163. Int. J. Biol. Macromol. 207, 635–643. doi: 10.1016/j.ijbiomac.2022.03.040
Han, J., Zhou, L., Ge, X., Guo, X., and Yang, H. (2017). Pathogenesis and control of the Chinese highly pathogenic porcine reproductive and respiratory syndrome virus. Vet. Microbiol. 209, 30–47. doi: 10.1016/j.vetmic.2017.02.020
Hartnell, A., Steel, J., Turley, H., Jones, M., Jackson, D. G., and Crocker, P. R. (2001). Characterization of human sialoadhesin, a sialic acid binding receptor expressed by resident and inflammatory macrophage populations. Blood 97, 288–296. doi: 10.1182/blood.v97.1.288
Heissler, S. M., and Manstein, D. J. (2013). Nonmuscle myosin-2: mix and match. Cell. Mol. Life Sci. 70, 1–21. doi: 10.1007/s00018-012-1002-9
Holtkamp, D. J., Kliebenstein, J. B., and Neumann, E. J. (2013). Assessment of the economic impact of porcine reproductive and respiratory syndrome virus on United States pork producers. J. Swine Health Prod. 21, 72–84. doi: 10.2460/javma.2005.227.385
Hou, G., Xue, B., Li, L., Nan, Y., Zhang, L., Li, K., et al. (2019). Direct interaction between CD163 N-terminal domain and MYH9 C-terminal domain contributes to porcine reproductive and respiratory syndrome virus internalization by permissive cells. Front. Microbiol. 10:1815. doi: 10.3389/fmicb.2019.01815
Hou, J., Li, R., Qiao, S., Chen, X. X., Xing, G., and Zhang, G. (2020). Glycoprotein 5 is cleaved by cathepsin E during porcine reproductive and respiratory syndrome virus membrane fusion. J. Virol. 94:e00097–20. doi: 10.1128/jvi.00097-20
Huang, C., Bernard, D., Zhu, J., Dash, R. C., Chu, A., Knupp, A., et al. (2020). Small molecules block the interaction between porcine reproductive and respiratory syndrome virus and CD163 receptor and the infection of pig cells. Virol. J. 17:116. doi: 10.1186/s12985-020-01361-7
Huang, Y. W., Dryman, B. A., Li, W., and Meng, X. J. (2009). Porcine DC-SIGN: molecular cloning, gene structure, tissue distribution and binding characteristics. Dev. Comp. Immunol. 33, 464–480. doi: 10.1016/j.dci.2008.09.010
Jiang, Y., Khan, F. A., Pandupuspitasari, N. S., Kadariya, I., Cheng, Z., Ren, Y., et al. (2013). Analysis of the binding sites of porcine sialoadhesin receptor with PRRSV. Int. J. Mol. Sci. 14, 23955–23979. doi: 10.3390/ijms141223955
Jones, J. E., Le Sage, V., and Lakdawala, S. S. (2021). Viral and host heterogeneity and their effects on the viral life cycle. Nat. Rev. Microbiol. 19, 272–282. doi: 10.1038/s41579-020-00449-9
Jusa, E. R., Inaba, Y., Kouno, M., and Hirose, O. (1997). Effect of heparin on infection of cells by porcine reproductive and respiratory syndrome virus. Am. J. Vet. Res. 58, 488–491.
Kang, Y. L., Oh, C., Ahn, S. H., Choi, J. C., Choi, H. Y., Lee, S. W., et al. (2021). Inhibition of endocytosis of porcine reproductive and respiratory syndrome virus by rottlerin and its potential prophylactic administration in piglets. Antivir. Res. 195:105191. doi: 10.1016/j.antiviral.2021.105191
Ke, W., Fang, L., Jing, H., Tao, R., Wang, T., Li, Y., et al. (2017). Cholesterol 25-hydroxylase inhibits porcine reproductive and respiratory syndrome virus replication through enzyme activity-dependent and –independent mechanisms. J. Virol. 91:e00827–17. doi: 10.1128/jvi.00827-17
Kim, H. S., Kwang, J., Yoon, I. J., Joo, H. S., and Frey, M. L. (1993). Enhanced replication of porcine reproductive and respiratory syndrome (PRRS) virus in a homogeneous subpopulation of MA-104 cell line. Arch. Virol. 133, 477–483. doi: 10.1007/bf01313785
Kim, J. K., Fahad, A. M., Shanmukhappa, K., and Kapil, S. (2006). Defining the cellular target(s) of porcine reproductive and respiratory syndrome virus blocking monoclonal antibody 7G10. J. Virol. 80, 689–696. doi: 10.1128/jvi.80.2.689-696.2006
Kreutz, L. C. (1998). Cellular membrane factors are the major determinants of porcine reproductive and respiratory syndrome virus tropism. Virus Res. 53, 121–128. doi: 10.1016/s0168-1702(97)00134-2
Kreutz, L. C., and Ackermann, M. R. (1996). Porcine reproductive and respiratory syndrome virus enters cells through a low pH-dependent endocytic pathway. Virus Res. 42, 137–147. doi: 10.1016/0168-1702(96)01313-5
Kristiansen, M., Graversen, J. H., Jacobsen, C., Sonne, O., Hoffman, H. J., Law, S. K., et al. (2001). Identification of the haemoglobin scavenger receptor. Nature 409, 198–201. doi: 10.1038/35051594
Lai, K. M., and Lee, W. L. (2022). The roles of epidermal growth factor receptor in viral infections. Growth Factors 40, 46–72. doi: 10.1080/08977194.2022.2063123
Law, S. K., Micklem, K. J., Shaw, J. M., Zhang, X. P., Dong, Y., Willis, A. C., et al. (1993). A new macrophage differentiation antigen which is a member of the scavenger receptor superfamily. Eur. J. Immunol. 23, 2320–2325. doi: 10.1002/eji.1830230940
Lee, Y. J., Park, C. K., Nam, E., Kim, S. H., Lee, O. S., Lee du, S., et al. (2010). Generation of a porcine alveolar macrophage cell line for the growth of porcine reproductive and respiratory syndrome virus. J. Virol. Methods 163, 410–415. doi: 10.1016/j.jviromet.2009.11.003
Li, L., Tian, X., Chen, J., Li, P., Zheng, Q., and Hou, J. (2018a). Griffithsin inhibits porcine reproductive and respiratory syndrome virus infection in vitro. Arch. Virol. 163, 3317–3325. doi: 10.1007/s00705-018-4029-x
Li, L., Xue, B., Sun, W., Gu, G., Hou, G., Zhang, L., et al. (2018b). Recombinant MYH9 protein C-terminal domain blocks porcine reproductive and respiratory syndrome virus internalization by direct interaction with viral glycoprotein 5. Antivir. Res. 156, 10–20. doi: 10.1016/j.antiviral.2018.06.001
Li, L., Wu, C., Hou, G., Xue, B., Xie, S., Zhao, Q., et al. (2017). Generation of murine macrophage-derived cell lines expressing porcine CD163 that support porcine reproductive and respiratory syndrome virus infection. BMC Biotechnol. 17:77. doi: 10.1186/s12896-017-0399-5
Li, L., Zhang, L., Hu, Q., Zhao, L., Nan, Y., Hou, G., et al. (2019). MYH9 key amino acid residues identified by the anti-idiotypic antibody to porcine reproductive and respiratory syndrome virus glycoprotein 5 involve in the virus internalization by porcine alveolar macrophages. Viruses 12:40. doi: 10.3390/v12010040
Li, N., Du, T., Yan, Y., Zhang, A., Gao, J., Hou, G., et al. (2016). MicroRNA let-7f-5p inhibits porcine reproductive and respiratory syndrome virus by targeting MYH9. Sci. Rep. 6:34332. doi: 10.1038/srep34332
Li, N., Huang, K., Chen, Y., Huang, Z., Zhang, Y., Leng, C., et al. (2021). MicroRNA ssc-miR-124a exhibits antiviral activity against porcine reproductive and respiratory syndrome virus via suppression of host genes CD163. Vet. Microbiol. 261:109216. doi: 10.1016/j.vetmic.2021.109216
Liang, Z., Li, P., Wang, C., Singh, D., and Zhang, X. (2020). Visualizing the transport of porcine reproductive and respiratory syndrome virus in live cells by quantum dots-based single virus tracking. Virol. Sin. 35, 407–416. doi: 10.1007/s12250-019-00187-0
Ling, J., Li, J., Khan, A., Lundkvist, Å, and Li, J. P. (2022). Is heparan sulfate a target for inhibition of RNA virus infection? Am. J. Physiol. Cell Physiol. 322:C605–C613. doi: 10.1152/ajpcell.00028.2022
Liu, X., Guo, C., Huang, Y., Zhang, X., and Chen, Y. (2015). Inhibition of porcine reproductive and respiratory syndrome virus by Cecropin D in vitro. Infect. Genet. Evol. 34, 7–16. doi: 10.1016/j.meegid.2015.06.021
Liu, Y., Li, R., Chen, X. X., Zhi, Y., Deng, R., Zhou, E. M., et al. (2019). Nonmuscle myosin heavy chain IIA recognizes sialic acids on sialylated RNA viruses to suppress proinflammatory responses via the DAP12-Syk pathway. mBio 10:e00574–19. doi: 10.1128/mBio.00574-19
Liu, Y., Li, R., Qiao, S., Chen, X. X., Deng, R., and Zhang, G. (2020a). Porcine sialoadhesin suppresses type I interferon production to support porcine reproductive and respiratory syndrome virus infection. Vet. Res. 51:18. doi: 10.1186/s13567-020-00743-7
Liu, Y., Li, R., Zhang, Y., Qiao, S., Chen, X. X., and Zhang, G. (2020b). Porcine reproductive and respiratory syndrome virus up-regulates sialoadhesin via IFN-STAT signaling to facilitate its infection. Microb. Pathog. 142:104112. doi: 10.1016/j.micpath.2020.104112
Lu, L., Su, S., Yang, H., and Jiang, S. (2021). Antivirals with common targets against highly pathogenic viruses. Cell 184, 1604–1620. doi: 10.1016/j.cell.2021.02.013
Ma, H., Jiang, L., Qiao, S., Zhi, Y., Chen, X. X., Yang, Y., et al. (2017). The crystal structure of the fifth scavenger receptor cysteine-rich domain of porcine CD163 reveals an important residue involved in porcine reproductive and respiratory syndrome virus infection. J. Virol. 91:e01897–16. doi: 10.1128/jvi.01897-16
Ma, H., Li, R., Jiang, L., Qiao, S., Chen, X. X., Wang, A., et al. (2021). Structural comparison of CD163 SRCR5 from different species sheds some light on its involvement in porcine reproductive and respiratory syndrome virus-2 infection in vitro. Vet. Res. 52:97. doi: 10.1186/s13567-021-00969-z
Momin, M. Y., Gaddam, R. R., Kravitz, M., Gupta, A., and Vikram, A. (2021). The challenges and opportunities in the development of microRNA therapeutics: a multidisciplinary viewpoint. Cells 10:3097. doi: 10.3390/cells10113097
Moreno, J. A., Muñoz-García, B., Martín-Ventura, J. L., Madrigal-Matute, J., Orbe, J., Páramo, J. A., et al. (2009). The CD163-expressing macrophages recognize and internalize TWEAK: potential consequences in atherosclerosis. Atherosclerosis 207, 103–110. doi: 10.1016/j.atherosclerosis.2009.04.033
Nauwynck, H. J., Duan, X., Favoreel, H. W., Van Oostveldt, P., and Pensaert, M. B. (1999). Entry of porcine reproductive and respiratory syndrome virus into porcine alveolar macrophages via receptor-mediated endocytosis. J. Gen. Virol. 80, 297–305. doi: 10.1099/0022-1317-80-2-297
Ni, B., Wen, L. B., Wang, R., Hao, H. P., Huan, C. C., Wang, X., et al. (2015). The involvement of FAK-PI3K-AKT-Rac1 pathway in porcine reproductive and respiratory syndrome virus entry. Biochem. Biophys. Res. Commun. 458, 392–398. doi: 10.1016/j.bbrc.2015.01.126
O’Neill, A. S., van den Berg, T. K., and Mullen, G. E. (2013). Sialoadhesin – a macrophage-restricted marker of immunoregulation and inflammation. Immunology 138, 198–207. doi: 10.1111/imm.12042
Pecci, A., Ma, X., Savoia, A., and Adelstein, R. S. (2018). MYH9: structure, functions and role of non-muscle myosin IIA in human disease. Gene 664, 152–167. doi: 10.1016/j.gene.2018.04.048
Prather, R. S., Rowland, R. R., Ewen, C., Trible, B., Kerrigan, M., Bawa, B., et al. (2013). An intact sialoadhesin (Sn/SIGLEC1/CD169) is not required for attachment/internalization of the porcine reproductive and respiratory syndrome virus. J. Virol. 87, 9538–9546. doi: 10.1128/jvi.00177-13
Prather, R. S., Whitworth, K. M., Schommer, S. K., and Wells, K. D. (2017). Genetic engineering alveolar macrophages for host resistance to PRRSV. Vet. Microbiol. 209, 124–129. doi: 10.1016/j.vetmic.2017.01.036
Qiao, S., Feng, L., Bao, D., Guo, J., Wan, B., Xiao, Z., et al. (2011). Porcine reproductive and respiratory syndrome virus and bacterial endotoxin act in synergy to amplify the inflammatory response of infected macrophages. Vet. Microbiol. 149, 213–220. doi: 10.1016/j.vetmic.2010.11.006
Ramos, I., Stamatakis, K., Oeste, C. L., and Pérez-Sala, D. (2020). Vimentin as a multifaceted player and potential therapeutic target in viral infections. Int. J. Mol. Sci. 21:4675. doi: 10.3390/ijms21134675
Reiner, G. (2016). Genetic resistance – an alternative for controlling PRRS? Porcine Health Manag. 2:27. doi: 10.1186/s40813-016-0045-y
Rossow, K. D. (1998). Porcine reproductive and respiratory syndrome. Vet. Pathol. 35, 1–20. doi: 10.1177/030098589803500101
Shanmukhappa, K., Kim, J. K., and Kapil, S. (2007). Role of CD151, a tetraspanin, in porcine reproductive and respiratory syndrome virus infection. Virol. J. 4:62. doi: 10.1186/1743-422x-4-62
Shi, C., Liu, Y., Ding, Y., Zhang, Y., and Zhang, J. (2015). PRRSV receptors and their roles in virus infection. Arch. Microbiol. 197, 503–512. doi: 10.1007/s00203-015-1088-1
Song, T., Fang, L., Wang, D., Zhang, R., Zeng, S., An, K., et al. (2016). Quantitative interactome reveals that porcine reproductive and respiratory syndrome virus nonstructural protein 2 forms a complex with viral nucleocapsid protein and cellular vimentin. J. Proteom. 142, 70–81. doi: 10.1016/j.jprot.2016.05.009
Song, Z., Bai, J., Nauwynck, H., Lin, L., Liu, X., Yu, J., et al. (2019). 25-Hydroxycholesterol provides antiviral protection against highly pathogenic porcine reproductive and respiratory syndrome virus in swine. Vet. Microbiol. 231, 63–70. doi: 10.1016/j.vetmic.2019.02.035
Song, Z., Zhang, Q., Liu, X., Bai, J., Zhao, Y., Wang, X., et al. (2017). Cholesterol 25-hydroxylase is an interferon-inducible factor that protects against porcine reproductive and respiratory syndrome virus infection. Vet. Microbiol. 210, 153–161. doi: 10.1016/j.vetmic.2017.09.011
Stoian, A. M. M., and Rowland, R. R. R. (2019). Challenges for porcine reproductive and respiratory syndrome (PRRS) vaccine design: reviewing virus glycoprotein interactions with CD163 and targets of virus neutralization. Vet. Sci. 6:9. doi: 10.3390/vetsci6010009
Stoian, A. M. M., Rowland, R. R. R., and Brandariz-Nuñez, A. (2022). Mutations within scavenger receptor cysteine-rich (SRCR) protein domain 5 of porcine CD163 involved in infection with porcine reproductive and respiratory syndrome virus (PRRS). J. Gen. Virol. 103:1740. doi: 10.1099/jgv.0.001740
Su, C. M., Rowland, R. R. R., and Yoo, D. (2021). Recent advances in PRRS virus receptors and the targeting of receptor-ligand for control. Vaccines 9:354. doi: 10.3390/vaccines9040354
Tandon, R., Sharp, J. S., Zhang, F., Pomin, V. H., Ashpole, N. M., Mitra, D., et al. (2021). Effective inhibition of SARS-CoV-2 entry by heparin and enoxaparin derivatives. J. Virol. 95:e01987–20. doi: 10.1128/jvi.01987-20
Tian, D., Wei, Z., Zevenhoven-Dobbe, J. C., Liu, R., Tong, G., Snijder, E. J., et al. (2012). Arterivirus minor envelope proteins are a major determinant of viral tropism in cell culture. J. Virol. 86, 3701–3712. doi: 10.1128/jvi.06836-11
Tompa, D. R., Immanuel, A., Srikanth, S., and Kadhirvel, S. (2021). Trends and strategies to combat viral infections: a review on FDA approved antiviral drugs. Int. J. Biol. Macromol. 172, 524–541. doi: 10.1016/j.ijbiomac.2021.01.076
Tu, C. F., Chuang, C. K., and Yang, T. S. (2022). The application of new breeding technology based on gene editing in pig industry – A review. Anim. Biosci. 35, 791–803. doi: 10.5713/ab.21.0390
Van Breedam, W., Delputte, P. L., Van Gorp, H., Misinzo, G., Vanderheijden, N., Duan, X., et al. (2010a). Porcine reproductive and respiratory syndrome virus entry into the porcine macrophage. J. Gen. Virol. 91, 1659–1667. doi: 10.1099/vir.0.020503-0
Van Breedam, W., Van Gorp, H., Zhang, J. Q., Crocker, P. R., Delputte, P. L., and Nauwynck, H. J. (2010b). The M/GP(5) glycoprotein complex of porcine reproductive and respiratory syndrome virus binds the sialoadhesin receptor in a sialic acid-dependent manner. PLoS Pathog. 6:e1000730. doi: 10.1371/journal.ppat.1000730
Van Breedam, W., Verbeeck, M., Christiaens, I., Van Gorp, H., and Nauwynck, H. J. (2013). Porcine, murine and human sialoadhesin (Sn/Siglec-1/CD169): portals for porcine reproductive and respiratory syndrome virus entry into target cells. J. Gen. Virol. 94, 1955–1960. doi: 10.1099/vir.0.053082-0
Van Gorp, H., Delputte, P. L., and Nauwynck, H. J. (2010a). Scavenger receptor CD163, a Jack-of-all-trades and potential target for cell-directed therapy. Mol. Immunol. 47, 1650–1660. doi: 10.1016/j.molimm.2010.02.008
Van Gorp, H., Van Breedam, W., Van Doorsselaere, J., Delputte, P. L., and Nauwynck, H. J. (2010b). Identification of the CD163 protein domains involved in infection of the porcine reproductive and respiratory syndrome virus. J. Virol. 84, 3101–3105. doi: 10.1128/jvi.02093-09
Van Gorp, H., Van Breedam, W., Delputte, P. L., and Nauwynck, H. J. (2008). Sialoadhesin and CD163 join forces during entry of the porcine reproductive and respiratory syndrome virus. J. Gen. Virol. 89, 2943–2953. doi: 10.1099/vir.0.2008/005009-0
Vanderheijden, N., Delputte, P. L., Favoreel, H. W., Vandekerckhove, J., Van Damme, J., van Woensel, P. A., et al. (2003). Involvement of sialoadhesin in entry of porcine reproductive and respiratory syndrome virus into porcine alveolar macrophages. J. Virol. 77, 8207–8215. doi: 10.1128/jvi.77.15.8207-8215.2003
Veit, M., Matczuk, A. K., Sinhadri, B. C., Krause, E., and Thaa, B. (2014). Membrane proteins of arterivirus particles: structure, topology, processing and function. Virus Res. 194, 16–36. doi: 10.1016/j.virusres.2014.09.010
Vicente-Manzanares, M., Ma, X., Adelstein, R. S., and Horwitz, A. R. (2009). Non-muscle myosin II takes centre stage in cell adhesion and migration. Nat. Rev. Mol. Cell Biol. 10, 778–790. doi: 10.1038/nrm2786
Wang, H., Shen, L., Chen, J., Liu, X., Tan, T., Hu, Y., et al. (2019a). Deletion of CD163 exon 7 confers resistance to highly pathogenic porcine reproductive and respiratory viruses on pigs. Int. J. Biol. Sci. 15, 1993–2005. doi: 10.7150/ijbs.34269
Wang, J., Li, J., Wang, N., Ji, Q., Li, M., Nan, Y., et al. (2019b). The 40 kDa linear polyethylenimine inhibits porcine reproductive and respiratory syndrome virus infection by blocking its attachment to permissive cells. Viruses 11:876. doi: 10.3390/v11090876
Wang, X., Wang, G., Wang, N., Liu, J., Cai, Y., Ren, M., et al. (2019c). A simple and efficient method for the generation of a porcine alveolar macrophage cell line for high-efficiency Porcine reproductive and respiratory syndrome virus 2 infection. J. Virol. Methods 274:113727. doi: 10.1016/j.jviromet.2019.113727
Wang, L., Li, R., Geng, R., Zhang, L., Chen, X. X., Qiao, S., et al. (2022). Heat shock protein member 8 (HSPA8) is involved in porcine reproductive and respiratory syndrome virus attachment and internalization. Microbiol. Spectr. 10:e0186021. doi: 10.1128/spectrum.01860-21
Wang, R., Wang, X., Ni, B., Huan, C. C., Wu, J. Q., Wen, L. B., et al. (2016a). Syndecan-4, a PRRSV attachment factor, mediates PRRSV entry through its interaction with EGFR. Biochem. Biophys. Res. Commun. 475, 230–237. doi: 10.1016/j.bbrc.2016.05.084
Wang, R., Wang, X., Wu, J. Q., Ni, B., Wen, L. B., Huang, L., et al. (2016b). Efficient porcine reproductive and respiratory syndrome virus entry in MARC-145 cells requires EGFR-PI3K-AKT-LIMK1-COFILIN signaling pathway. Virus Res. 225, 23–32. doi: 10.1016/j.virusres.2016.09.005
Wang, X., Wei, R., Li, Q., Liu, H., Huang, B., Gao, J., et al. (2013). PK-15 cells transfected with porcine CD163 by PiggyBac transposon system are susceptible to porcine reproductive and respiratory syndrome virus. J. Virol. Methods 193, 383–390. doi: 10.1016/j.jviromet.2013.06.035
Wei, X., Li, R., Qiao, S., Chen, X. X., Xing, G., and Zhang, G. (2020). Porcine reproductive and respiratory syndrome virus utilizes viral apoptotic mimicry as an alternative pathway to infect host cells. J. Virol. 94:e709–e720. doi: 10.1128/jvi.00709-20
Wei, Z., Tian, D., Sun, L., Lin, T., Gao, F., Liu, R., et al. (2012). Influence of N-linked glycosylation of minor proteins of porcine reproductive and respiratory syndrome virus on infectious virus recovery and receptor interaction. Virology 429, 1–11. doi: 10.1016/j.virol.2012.03.010
Welch, S. K., and Calvert, J. G. (2010). A brief review of CD163 and its role in PRRSV infection. Virus Res. 154, 98–103. doi: 10.1016/j.virusres.2010.07.018
Wells, K. D., Bardot, R., Whitworth, K. M., Trible, B. R., Fang, Y., Mileham, A., et al. (2017). Replacement of porcine CD163 scavenger receptor cysteine-rich domain 5 with a CD163-like homolog confers resistance of pigs to genotype 1 but not genotype 2 porcine reproductive and respiratory syndrome virus. J. Virol. 91:e01521–16. doi: 10.1128/jvi.01521-16
Whitworth, K. M., and Prather, R. S. (2017). Gene editing as applied to prevention of reproductive porcine reproductive and respiratory syndrome. Mol. Reprod. Dev. 84, 926–933. doi: 10.1002/mrd.22811
Whitworth, K. M., Rowland, R. R., Ewen, C. L., Trible, B. R., Kerrigan, M. A., Cino-Ozuna, A. G., et al. (2016). Gene-edited pigs are protected from porcine reproductive and respiratory syndrome virus. Nat. Biotechnol. 34, 20–22. doi: 10.1038/nbt.3434
Xia, W., Wu, Z., Guo, C., Zhu, S., Zhang, X., Xia, X., et al. (2018). Recombinant adenovirus-delivered soluble CD163 and sialoadhesin receptors protected pigs from porcine reproductive and respiratory syndrome virus infection. Vet. Microbiol. 219, 1–7. doi: 10.1016/j.vetmic.2018.04.006
Xiang, J., Lu, M., Shi, M., Cheng, X., Kwakwa, K. A., Davis, J. L., et al. (2022). Heparanase blockade as a novel dual-targeting therapy for COVID-19. J. Virol. 96:e0005722. doi: 10.1128/jvi.00057-22
Xie, J., Christiaens, I., Yang, B., Breedam, W. V., Cui, T., and Nauwynck, H. J. (2017). Molecular cloning of porcine Siglec-3, Siglec-5 and Siglec-10, and identification of Siglec-10 as an alternative receptor for porcine reproductive and respiratory syndrome virus (PRRSV). J. Gen. Virol. 98, 2030–2042. doi: 10.1099/jgv.0.000859
Xie, J., Christiaens, I., Yang, B., Trus, I., Devriendt, B., Cui, T., et al. (2018). Preferential use of Siglec-1 or Siglec-10 by type 1 and type 2 PRRSV strains to infect PK15(S1-CD163) and PK15(S10-CD163) cells. Vet. Res. 49:67. doi: 10.1186/s13567-018-0569-z
Xu, Y. L., Wu, S. P., Li, Y. G., Sun, F. X., Wang, Q. J., Zhao, Q., et al. (2020c). A porcine alveolar macrophage cell line stably expressing CD163 demonstrates virus replication and cytokine secretion characteristics similar to primary alveolar macrophages following PRRSV infection. Vet. Microbiol. 244:108690. doi: 10.1016/j.vetmic.2020.108690
Xu, H., Liu, Z., Zheng, S., Han, G., and He, F. (2020a). CD163 antibodies inhibit PRRSV infection via receptor blocking and transcription suppression. Vaccines 8:592. doi: 10.3390/vaccines8040592
Xu, K., Zhou, Y., Mu, Y., Liu, Z., Hou, S., Xiong, Y., et al. (2020b). CD163 and pAPN double-knockout pigs are resistant to PRRSV and TGEV and exhibit decreased susceptibility to PDCoV while maintaining normal production performance. Elife 9:e57132. doi: 10.7554/eLife.57132
Xue, B., Hou, G., Zhang, G., Huang, J., Li, L., Nan, Y., et al. (2019). MYH9 aggregation induced by direct interaction with PRRSV GP5 ectodomain facilitates viral internalization by permissive cells. Front. Microbiol. 10:2313. doi: 10.3389/fmicb.2019.02313
Yamaoka, T., Ohba, M., and Ohmori, T. (2017). Molecular-targeted therapies for epidermal growth factor receptor and its resistance mechanisms. Int. J. Mol. Sci. 18:2420. doi: 10.3390/ijms18112420
Yang, H., Wang, H., Levine, Y. A., Gunasekaran, M. K., Wang, Y., Addorisio, M., et al. (2016). Identification of CD163 as an antiinflammatory receptor for HMGB1-haptoglobin complexes. JCI Insight 1:e85375. doi: 10.1172/jci.insight.85375
Yang, H., Zhang, J., Zhang, X., Shi, J., Pan, Y., Zhou, R., et al. (2018). CD163 knockout pigs are fully resistant to highly pathogenic porcine reproductive and respiratory syndrome virus. Antivir. Res. 151, 63–70. doi: 10.1016/j.antiviral.2018.01.004
Yang, Q., Gao, L., Si, J., Sun, Y., Liu, J., Cao, L., et al. (2013). Inhibition of porcine reproductive and respiratory syndrome virus replication by flavaspidic acid AB. Antivir. Res. 97, 66–73. doi: 10.1016/j.antiviral.2012.11.004
Yu, P., Wei, R., Dong, W., Zhu, Z., Zhang, X., Chen, Y., et al. (2019). CD163(ΔSRCR5) MARC-145 cells resist PRRSV-2 infection via inhibiting virus uncoating, which requires the interaction of CD163 with calpain 1. Front. Microbiol. 10:3115. doi: 10.3389/fmicb.2019.03115
Zani, I. A., Stephen, S. L., Mughal, N. A., Russell, D., Homer-Vanniasinkam, S., Wheatcroft, S. B., et al. (2015). Scavenger receptor structure and function in health and disease. Cells 4, 178–201. doi: 10.3390/cells4020178
Zhang, L., Xu, R., Wei, F., Li, W., Li, X., Zhang, G., et al. (2020a). Activation of sialoadhesin down-regulates the levels of innate antiviral cytokines in porcine alveolar macrophages in vitro. Virus Res. 275:197792. doi: 10.1016/j.virusres.2019.197792
Zhang, Q., Chen, C. Z., Swaroop, M., Xu, M., Wang, L., Lee, J., et al. (2020b). Heparan sulfate assists SARS-CoV-2 in cell entry and can be targeted by approved drugs in vitro. Cell Discov. 6:80. doi: 10.1038/s41421-020-00222-5
Zhang, Y., Wen, Z., Shi, X., Liu, Y. J., Eriksson, J. E., and Jiu, Y. (2020c). The diverse roles and dynamic rearrangement of vimentin during viral infection. J. Cell Sci. 134:jcs250597. doi: 10.1242/jcs.250597
Zhang, Y., Zhang, K., Zheng, H., Liu, C., Jiang, Y., Du, N., et al. (2020d). Development of a monoclonal antibody against porcine CD163 SRCR5 domain which partially blocks infection of PRRSV. Front. Vet. Sci. 7:597843. doi: 10.3389/fvets.2020.597843
Zhang, Q., and Yoo, D. (2015). PRRS virus receptors and their role for pathogenesis. Vet. Microbiol. 177, 229–241. doi: 10.1016/j.vetmic.2015.04.002
Zhang, S. L., Wu, Y. C., Cheng, F., Guo, Z. Y., and Chen, J. F. (2016). Anti-PRRSV effect and mechanism of tetrahydroaltersolanol C in vitro. J. Asian Nat. Prod. Res. 18, 303–314. doi: 10.1080/10286020.2015.1072516
Zheng, Q., Hou, J., Zhou, Y., Yang, Y., Xie, B., and Cao, X. (2015). Siglec1 suppresses antiviral innate immune response by inducing TBK1 degradation via the ubiquitin ligase TRIM27. Cell Res. 25, 1121–1136. doi: 10.1038/cr.2015.108
Zheng, X. X., Li, R., Qiao, S., Chen, X. X., Zhang, L., Lu, Q., et al. (2021). Vimentin rearrangement by phosphorylation is beneficial for porcine reproductive and respiratory syndrome virus replication in vitro. Vet. Microbiol. 259:109133. doi: 10.1016/j.vetmic.2021.109133
Zhu, L., Song, H., Zhang, X., Xia, X., and Sun, H. (2014). Inhibition of porcine reproductive and respiratory syndrome virus infection by recombinant adenovirus- and/or exosome-delivered the artificial microRNAs targeting sialoadhesin and CD163 receptors. Virol. J. 11:225. doi: 10.1186/s12985-014-0225-9
Keywords: PRRSV, attachment, entry, host cellular factors, antiviral strategies
Citation: Li R, Qiao S and Zhang G (2022) Reappraising host cellular factors involved in attachment and entry to develop antiviral strategies against porcine reproductive and respiratory syndrome virus. Front. Microbiol. 13:975610. doi: 10.3389/fmicb.2022.975610
Received: 22 June 2022; Accepted: 08 July 2022;
Published: 26 July 2022.
Edited by:
Chunfu Zheng, University of Calgary, CanadaReviewed by:
Qin Zhao, Northwest A&F University, ChinaGaopeng Hou, Washington University in St. Louis, United States
Copyright © 2022 Li, Qiao and Zhang. This is an open-access article distributed under the terms of the Creative Commons Attribution License (CC BY). The use, distribution or reproduction in other forums is permitted, provided the original author(s) and the copyright owner(s) are credited and that the original publication in this journal is cited, in accordance with accepted academic practice. No use, distribution or reproduction is permitted which does not comply with these terms.
*Correspondence: Songlin Qiao, Y2RqNTY1QGdtYWlsLmNvbQ==; Gaiping Zhang, emhhbmdnYWlwQDEyNi5jb20=