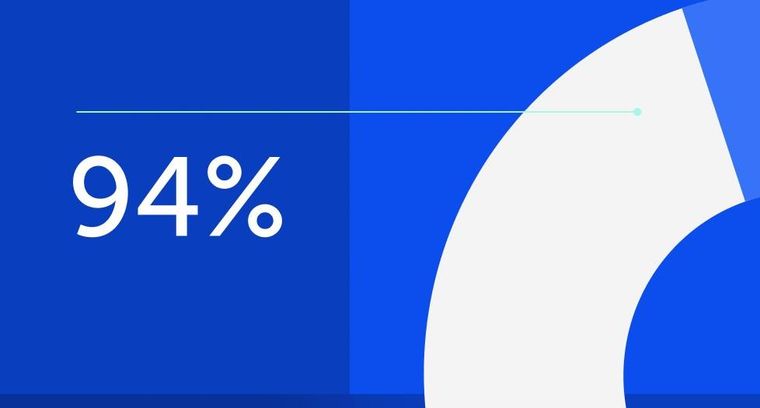
94% of researchers rate our articles as excellent or good
Learn more about the work of our research integrity team to safeguard the quality of each article we publish.
Find out more
ORIGINAL RESEARCH article
Front. Microbiol., 07 September 2022
Sec. Terrestrial Microbiology
Volume 13 - 2022 | https://doi.org/10.3389/fmicb.2022.975571
Manure application is an effective way to improve the utilization efficiency of organic resources and alleviate the adverse effects of long-term application of chemical fertilizers. However, the impact of applying manure under different nitrogen rates on soil microbial community in wheat field remains unclear. Treatments with and without chicken manure application under three nitrogen rates (N 135, 180 and 225 kg⋅hm–2) were set in wheat field. Soil organic carbon, available nutrients, and abundance, diversity, structure and co-occurrence pattern of soil microbial community at wheat maturity were investigated. Compared with no manure application, chicken manure application increased the soil organic carbon and available phosphorus, while the effects on soil mineral nitrogen and available potassium varied with different nitrogen rates. Chicken manure application significantly increased soil bacterial abundance under the nitrogen fertilization of 135 and 225 kg⋅hm–2, increased soil fungal abundance under the nitrogen fertilization of 135 kg⋅hm–2, but decreased soil fungal abundance under the nitrogen fertilization of 180 and 225 kg⋅hm–2 (P < 0.05). There was no significant difference in alpha diversity indices of soil microbial communities between treatments with and without chicken manure application under different nitrogen rates (P > 0.05). Chicken manure application and its interaction with nitrogen rate significantly changed soil bacterial and fungal community structures (P < 0.05). There were significantly different taxa of soil microbial communities between treatments with and without chicken manure application. Chicken manure application reduced the ecological network complexity of soil bacterial community and increased that of soil fungal community. In summary, the responses of soil available nutrients and microbial abundance to applying chicken manure varied with different nitrogen rates. One growing season application of chicken manure was sufficient to alter the soil microbial community structure, composition and co-occurrence pattern, whereas not significantly affected soil microbial community diversity.
For more than a century, the application of chemical nitrogen fertilizer has greatly boosted crop productivity and provided food security for about half of the population in the world (Erisman et al., 2008). Meanwhile, long-term excessive application of chemical nitrogen fertilizer has resulted in adverse consequences for crop production and environment, such as reduced fertilizer utilization efficiency (Anas et al., 2020), accelerated soil acidification (Guo et al., 2010), and increased water and air pollution (Zhang et al., 2013; Wang H. et al., 2017).
The livestock and poultry industry produces a large amount of manure with low utilization efficiency (Bai et al., 2018). However, livestock and poultry manure is an important organic resource, rich in organic matter and a variety of nutrients available for crop growth, and could be used as an effective substitution for chemical fertilizer (Du et al., 2020). Moreover, recycling manure back to cropland is also an important means to realize the organic waste reutilization and increase ecosystem service (Tang et al., 2019). Many studies have shown that application of manure (including supplemental application and partial substitution for chemical fertilizer) has multiple benefits compared to chemical fertilizer, including improving soil physical characteristics (Khaliq and Abbasi, 2015), preventing soil acidification (Wang et al., 2019), increasing soil organic carbon (Tang et al., 2019; Wang et al., 2019), increasing soil enzyme activity (Liu et al., 2021), improving soil fertility (Kobierski et al., 2017; Wei et al., 2017; Liu et al., 2021), reducing greenhouse gas emission (Tang et al., 2019), promoting crop nutrient accumulation (Xin et al., 2017; Zhang et al., 2018), improving fertilizer utilization efficiency (Xin et al., 2017; Wei et al., 2020), and increasing crop yield (Zhang et al., 2018; Du et al., 2020; Liu et al., 2021).
Soil microbial community is an important part of soil ecosystem, which is related to the regulation of nutrient biogeochemical cycling and maintenance of soil fertility. The species diversity, composition and interaction of the soil microbial community determine the resistance and stability of soil ecosystem, and are important indicators to assess soil quality (Anderson, 2003; Schloter et al., 2018). Thus, revealing the impacts of agricultural management practices on soil microbial community diversity, structure, species composition and co-occurrence pattern are vital to regulate soil ecosystem function. Among agricultural practices, fertilization (organic and chemical fertilizer) has great impact on soil microorganisms and is the primary factor affecting soil microbial community structure (Guo et al., 2020). Previous studies have shown that the application of nitrogen fertilizer and manure had significant effect on soil microbial community. Long-term nitrogen application increased the soil fungal abundance, decreased its alpha diversity, and changed its species composition (Zhou et al., 2016). Fungal community composition differed among soils under different nitrogen application rates (Zhou et al., 2016). High nitrogen rate significantly increased soil fungal abundance, while soil bacterial abundance had no significant response to different nitrogen application rates (Zhao S. et al., 2014). Compared with chemical fertilization, manure application combined with chemical fertilization could reduce the influence of environmental factors on soil microbial community (Guo et al., 2020). The application of manure increased soil microbial diversity (Legrand et al., 2018), changed the community structure and species composition of soil bacteria and fungi, had a significant impact on soil microbial ecological network and key taxa (Ji et al., 2021), and enhanced the potential soil ecosystem function (Gu et al., 2019).
The soil nutrient stoichiometry could cause changes in soil microbial community and interactions among species (Zechmeister-Boltenstern et al., 2015). The effects of nitrogen fertilization and manure application on soil microbial community in previous studies may also be related to the changes of soil resource stoichiometry. However, most studies only focused on evaluating the effects of applying chemical nitrogen or manure alone on soil microbial community, while the impact of applying manure under different nitrogen rates on soil microbial community in wheat field remains poorly understood. Consequently, this study examined the responses of chicken manure application under three nitrogen rates on soil microbial abundance, diversity, structure and ecological network. The objectives of this work were to (i) investigate the effects of chicken manure application on soil microbial abundance, diversity and structure under different nitrogen rates; and (ii) reveal the short-term effects of chicken manure application on species composition and co-occurrence pattern of soil microbial community in wheat field. We hypothesized that short-term application of chicken manure would significantly affect soil properties and microbial community, and the effect of chicken manure application would varied with different nitrogen rates, considering the change of carbon and nitrogen inputs.
The field experiment was carried out from October 2020 to June 2021 in Haojie Village, Xigang Town, Qi County, Hebi City, Henan Province, China (35°35′ N, 114°13′ E). The average annual temperature and precipitation in this region are 14.4°C and 594 mm, respectively. The soil type was fluvo-aquic soil, and the previous crop was summer corn. The initial properties of 0–20 cm soil were as follows: organic carbon 10.84 g⋅kg–1, total nitrogen 1.10 g⋅kg–1, total phosphorus 0.38 g⋅kg–1, total potassium 16.37 g⋅kg–1, pH 8.07.
In the experiment, no manure (C) and applying chicken manure (M) treatments were set under three nitrogen rates (N 135, 180 and 225 kg⋅hm–2), respectively. The experiment was set up in a randomized block design, with three replicates for each treatment. There were a total of 18 plots, and each plot was 7 m long and 5 m wide. The chicken manure for application was composted until thermal stabilization, and then air-dried. It contained 46.42% organic matter, 7.18% nitrogen (N), 1.82% phosphorus (P2O5), and 2.42% potassium (K2O). The amount of chicken manure application was 2250 kg⋅hm–2 dry weight. The application rates of phosphorus and potassium fertilizers in all plots were 120 kg⋅hm–2 P2O5 and 90 kg⋅hm–2 K2O, respectively. The 135 kg⋅hm–2 of nitrogen fertilizer and all of chicken manure, phosphate and potassium fertilizer were applied as basal fertilization, and the remaining nitrogen fertilizer was applied as topdressing during the jointing period of wheat. Urea (46% N), calcium superphosphate (12% P2O5) and potassium chloride (60% K2O) were used as chemical fertilizers. Wheat was sown on October 18, 2020. Before sowing, the basal fertilizers were evenly broadcast on the soil surface, and then the soil was rotated by tillage of 20 cm. Except for the application rate of manure and nitrogen fertilizer, the field management of each treatment was same.
Soil samples were collected at the depth of 0-20 cm after harvesting the winter wheat on June 5, 2021. Five soil cores (2.5 cm diameter) were collected randomly from each plot and mixed as soil sample for the plot. Soil samples were sieved through 5 mm to remove impurities and further homogenize. Each sample was divided into two parts and brought back to the laboratory on ice. One was stored at a −80°C for soil microbial community analysis, and the other was air-dried for soil organic carbon and available nutrient analysis.
Soil organic carbon (SOC) was determined by potassium dichromate volumetric method. Soil NO3–-N and NH4+-N were determined by continuous flow analyzer after extracting with 1 mol⋅L–1 KCl. Soil mineral nitrogen was expressed as the total concentration of NO3–-N and NH4+-N. Soil available phosphorus was extracted with 0.5 mol⋅L–1 NaHCO3 and determined by the molybdenum antimony colorimetric method. Soil available potassium was extracted with 1 mol⋅L–1 NH4OAc and determined by flame photometry.
Soil total DNA was extracted from ∼0.5 g of each soil sample using the FastDNA Spin Kit for Soil (MP Biomedicals, United States). The concentration and quality of the extracted DNA were evaluated using NanoDrop 2000 (Thermo Scientific, United States) and 1% agarose gel electrophoresis.
Real-time fluorescent quantitative polymerase chain reaction (PCR) of the bacterial 16S rRNA gene and the fungal ITS sequence was performed using the primers 515F/907R (Song et al., 2019) and ITS3F/ITS4R (Gade et al., 2013). The qPCR reagent kit was ChamQ SYBR Color qPCR Master Mix (Cat. No. Q411-02, Vazyme Biotech, China). According to the pre-experiment results, the optimal annealing temperatures for the 16S rRNA gene and ITS sequence were set at 60°C and 58°C, respectively. A total of 40 cycles of qPCR were performed. The plasmid DNA containing the target sequence with known concentration was used as standard. The standard series was prepared by a 10-fold serial dilution, which was detected by qPCR at the same time as the sample. Each sample and the standard were measured three times to obtain the average value, and the copy number of the target sequence in the sample was calculated according to the standard curve.
PCR amplification of the bacterial 16S rRNA gene and the fungal ITS sequence was performed using the primers 515F/907R (Song et al., 2019) and ITS3F/ITS4R (Gade et al., 2013). The PCR reagent kit was TransStart FastPfu DNA Polymerase (CAT. No. Ap221-02, TransGen Biotech, China). PCR conditions for the bacterial 16S rRNA gene and fungal ITS sequence were as follows: 3 min of initial denaturation at 95°C, followed by 27 (16S rRNA gene) and 35 (ITS sequence) cycles of 95°C for 30 s, 55°C for 30 s and 72°C for 45 s, and then a final extension step at 72°C for 10 min. The PCR amplification volume was 20 μL, and each sample had three replicate wells. The AxyPrep DNA Gel Extraction Kit (CAT. No. AP-GX-250, Axygen, United States) was used to obtain the target DNA fragment. Miseq PE300 high-throughput sequencing was performed after the sequencing library preparation.
The high-throughput sequencing data were analyzed using the online platform of Majorbio Cloud Platform1 (Ren et al., 2022). FLASH (Magoč and Salzberg, 2011) and fastp (Chen et al., 2018) were used for assembly, quality control and filtering of the raw data. USEARCH (Edgar, 2010) was used for OTU clustering according to 97% similarity, and the sequences were randomly resampled with the minimum sequence number. The OTU taxonomy assignment of bacteria and fungi was performed by RDP Classifier (Wang et al., 2007) based on the SILVA (Pruesse et al., 2007) and UNITE (Nilsson et al., 2019) databases, respectively. The mothur (Schloss, 2020) was used to calculate alpha diversity indices (Chao1 index and Shannon index) of soil microbial community at OTU level. Non-metric multidimensional scale analysis (NMDS) based on Abund Jaccard distance algorithm and similarity analysis (ANOSIM) were used to analyze the differences of soil microbial community structure. Mantel test was used to analyze the correlation between soil microbial community structure and soil properties. Linear discriminant analysis effect size (LEfSe) analysis (LDA > 3) (Segata et al., 2011) was used to analyze the differential taxa of soil microbial communities in treatments with and without chicken manure application. According to the Spearman correlation (| r | > 0.7, Benjamini-Hochberg adjusted P < 0.05), soil microbial ecological network analysis among genera with relative abundance of >0.1% was performed by the Cytoscape plugin CoNet (Faust and Raes, 2016). Topological parameter of the network was calculated by NetworkAnalyzer (Assenov et al., 2008). Finally, Gephi was used to visualize the network. Soil organic carbon, available nutrients, microbial abundance and alpha diversity indices among different treatments were compared under ANOVA and LSD test for multiple comparisons at P < 0.05 in SPSS.
Soil organic carbon and available nutrients under different treatments are shown in Figure 1. Compared with no manure application, manure application increased soil organic carbon, and the increase rate was the largest under 135 kg⋅hm–2 nitrogen fertilization (increased by 13.90%). With the increase of nitrogen rate, the soil mineral nitrogen was increased at wheat maturity. Manure application significantly increased soil mineral nitrogen under 135 and 180 kg⋅hm–2 nitrogen fertilization (P < 0.05), while there was no significant difference in soil mineral nitrogen between treatments with and without manure application under 225 kg⋅hm–2 nitrogen fertilization (P > 0.05). Compared with no manure application, applying manure significantly increased soil available phosphorus under different nitrogen rates (P < 0.05), the increase rates were 76.71–97.87%. The effect of manure application on soil available potassium was not significant under 135 and 180 kg⋅hm–2 nitrogen fertilization (P > 0.05), while manure application under 225 kg⋅hm–2 nitrogen rate significantly increased soil available potassium (P < 0.05).
Figure 1. Soil organic carbon and available nutrients under different nitrogen and manure treatments. M and C indicate treatments with and without chicken manure application, respectively. Error bars represent standard deviations (n = 3). Different letters above bars indicate significant difference at 0.05 level.
Quantitative PCR analysis showed that compared with 135 kg⋅hm–2 nitrogen fertilization, increasing nitrogen rate (180 and 225 kg⋅hm–2) increased the abundance of soil bacteria (Figure 2). Under 135 and 225 kg⋅hm–2 nitrogen fertilization, the application of manure significantly increased the abundance of soil bacteria (P < 0.05). Compared with 135 kg⋅hm–2 nitrogen fertilization, increasing nitrogen rate (180 and 225 kg⋅hm–2) without manure application significantly increased the abundance of soil fungi (P < 0.05), while increasing nitrogen fertilizer rate (180 and 225 kg⋅hm–2) with manure application significantly decreased the abundance of soil fungi (P < 0.05), which indicated that application of manure changed the response of soil fungal abundance to nitrogen rate. Compared with no manure application, the application of manure significantly increased the soil fungal abundance under 135 kg⋅hm–2 nitrogen fertilization (P < 0.05), but significantly decreased soil fungal abundance under 180 and 225 kg⋅hm–2 nitrogen fertilization (P < 0.05).
Figure 2. Soil microbial abundance under different nitrogen and manure treatments. M and C indicate treatments with and without chicken manure application, respectively. Error bars represent standard deviations (n = 3). Different letters above bars indicate significant difference at 0.05 level.
After the sequencing data of 16S rRNA gene were randomly resampled according to the minimum sequence number, 27952 sequences with an average length of 377 bp were obtained for each sample. After OTU taxonomy assignment, a total of 4860 OTUs were obtained from 18 samples, belonging to 828 genera, 471 families, 298 orders, 125 classes and 45 phyla. After the sequencing data of ITS sequence were randomly resampled according to the minimum sequence number, 49288 sequences with an average length of 312 bp were extracted from each sample. After OTU taxonomy assignment, a total of 1400 OTUs were obtained from 18 samples, belonging to 394 genera, 209 families and 104 orders, 53 classes and 18 phyla.
The alpha diversity indices (Chao1 richness index and Shannon diversity index) of soil microbial community in different treatments are shown in Table 1. Under the same nitrogen rate, there was no significant difference in alpha diversity indices of soil microbial communities between treatments with and without manure application (P > 0.05). In addition, there was no significant difference in alpha diversity indices of soil microbial communities among treatments with manure application under different nitrogen rates (P > 0.05). The above results indicated that short-term nitrogen rate and manure application had little impact on the alpha diversity of soil microbial community.
Table 1. Soil microbial community alpha diversity indices under different nitrogen and manure treatments.
As shown in Figure 3, non-metric multidimensional scale analysis (NMDS) was used to study the differences of soil microbial community structure under different treatments. The structure of soil bacterial and fungal community responded identically to different treatments. The soil microbial communities in treatments with and without manure application were separated obviously at NMDS1 axis, while there was no obvious separation among different nitrogen rates. The results of similarity analysis (ANOSIM) were shown in Table 2. Nitrogen rate had no significant effect on soil bacterial and fungal community structure, while manure application and its interaction with nitrogen rate significantly changed soil bacterial and fungal community structure (P < 0.05).
Figure 3. Non-metric multidimensional scaling analysis of soil microbial communities under different nitrogen and manure treatments. M and C indicate treatments with and without chicken manure application, respectively.
Table 2. Similarity analysis (ANOSIM) of soil microbial communities under different nitrogen and manure treatments.
Since the application of manure had the significant effect on soil microbial community structure, Linear discriminant analysis effect size (LEfSe) analysis was further used to recognize the differential taxa between treatments with and without manure application (LDA > 3). A total of 13 bacterial taxa and 58 fungal taxa were identified with significant differences (Supplementary Table 1). In soil bacterial community, the family Comamonadaceae was significantly enriched in no manure application treatments, while the order Solirubrobacterales and Gaiellales, the family Entotheonellaceae, and the genus Luteimonas were significantly enriched in manure application treatments. In soil fungal community, the treatments without manure application had significant enrichment of 14 genera, 8 families, 5 orders and 2 classes, and treatments with manure application had significant enrichment of 16 genera, 9 families, 3 orders and 1 class. At the genus level, Fusariella, unclassified Sympoventuriaceae, Preussia, Neosetophoma, Alternaria, Rhizophlyctis, Ochroconis, Schizothecium, unclassified Dothideomycetes, Setophoma, Monocillium, unclassified Sordariomycetes, Podospora and Staphylotrichum were significantly enriched in the treatments without manure application, while Leucothecium, Niesslia, Actinomucor, unclassified Ascodesmidaceae, Diutina, Cephaliophora, Dactylaria, Pseudaleuria, Cutaneotrichosporon, Microascus, Lophotrichus, unclassified Sporormiaceae, Neonectria, unclassified Chaetomiaceae, Hyalorbilia and Thelonectria were significantly enriched in the treatments with manure application.
As shown in Table 3, mantel test was conducted to analyze the correlation between soil microbial community structure and soil properties. Soil bacterial and fungal community structures were significantly correlated with soil available phosphorus and potassium (P < 0.05), and soil fungal community structure was also significantly correlated with soil organic carbon (P < 0.05), while the correlation between soil microbial community structure and soil mineral nitrogen was not significant (P > 0.05).
In order to decipher the potential interactions among microbial taxa, network analysis was performed with sequence data of soil microbial community. As shown in Figure 4 and Table 4, the soil bacterial ecological network of no manure application treatments was composed of 160 nodes and 967 edges, and the soil bacterial ecological network of manure application treatments was composed of 161 nodes and 710 edges. The average number of neighbors, clustering coefficient and network density of soil bacterial ecological network of manure application treatments were smaller than those of no manure application treatments, which indicated that applying manure reduced the complexity of soil bacterial ecological network. The response of soil fungal ecological network to manure application was on the contrary to that of soil bacterial community. The soil fungal ecological network of no manure application treatments was composed of 70 nodes and 186 edges, and the soil fungal ecological network of manure application treatments was composed of 74 nodes and 356 edges. Compared with no manure application, the average number of neighbors, clustering coefficient and network density of soil fungal ecological network were increased by applying manure, which indicated that applying manure increased the complexity of soil fungal ecological network. For the ecological networks of soil total microbial community, compared with no manure application, manure application decreased the number of total and mutual exclusion edges, while there was little difference of other topological parameters (Supplementary Figure 1 and Table 4). As shown in Supplementary Table 2, the percentage of bacterial-bacterial correlation was higher than those of fungal-fungal and bacterial-fungal correlations in soil total microbial networks. Compared with no manure application, manure application decreased the percentage of bacterial-bacterial correlation and increased the percentage of fungal-fungal correlation in soil total microbial network.
Figure 4. Ecological networks of soil bacterial community and fungal community under different organic manure treatments. M and C indicate treatments with and without chicken manure application, respectively. Genera are represented as nodes and correlations as edges (red: copresence, blue: mutual exclusion). The node sizes are correlated to the genus degree, and node color indicates the corresponding taxonomic assignment at phylum level.
Table 4. Topological parameters of soil microbial ecological networks under different organic manure treatments.
It is expected that soil organic carbon and available nutrients could be affected by manure application because manure itself is rich in organic carbon and available nutrients. In this study, manure application increased soil organic carbon under different nitrogen rates (Figure 1), which was consistent with the results of previous studies (Zhong et al., 2010; Wei et al., 2017; Lin et al., 2019). The increase of soil organic carbon was related to the additional carbon input. Although the increase in soil carbon input could stimulate the mineralization of soil organic carbon, the replenishment was more than priming loss of soil organic carbon with additional carbon input (Liang et al., 2018). The results of previous studies showed that the soil available phosphorus with long-term manure application was significantly higher than that without (Zhong et al., 2010; Kobierski et al., 2017; Wei et al., 2017). Ma et al. found that short-term application of manure (four rice growing seasons) significantly enriched soil total and available phosphorus (Ma et al., 2016). In this study, applying chicken manure in one wheat growing season could significantly increase the soil available phosphorus at wheat maturity, with an increase of 76.71-97.87% (Figure 1). It has been demonstrated that soil available phosphorus was significantly positively correlated with soil organic matter (Shen et al., 2014). Compared with chemical fertilization, manure application could improve the activity of soil phosphatase, which is responsible for soil phosphorus cycling and could increase available phosphorus (Li et al., 2015). The improvement of soil phosphatase activity by manure application may be another reason for the increase of soil available phosphorus in this study. Therefore, it may be feasible to rapidly increase soil available phosphorus by applying chicken manure to meet the phosphorus demand of crop production in phosphorus-restricted soil. Meanwhile, it could not be ignored that a large amount of available phosphorus residues at crop harvest may increase the risk of phosphorus leaching.
The results also showed that the responses of soil organic carbon, mineral nitrogen and available potassium to manure application were affected by different nitrogen rates (Figure 1). The increase of soil organic carbon and mineral nitrogen with manure application under low nitrogen rate was greater than that under high nitrogen rate, and the difference was not significant under high nitrogen rate. The response of soil available potassium was on the contrary that the application of manure under low nitrogen rate had no significant effect on soil available potassium, while increased significantly under high nitrogen rate. It was speculated that these results may be related to crop absorption under different treatments and soil enzyme activity under different soil nutrient stoichiometry.
This short-term experimental results showed that there was no significant difference in alpha diversity indices of soil microbial communities among treatments with manure application under different nitrogen rates (P > 0.05) (Table 1). In previous studies, the effects of manure application on soil microbial alpha diversity were inconsistent among different crop systems. In a double-cropping rice system, 3-year manure application increased soil bacterial and fungal alpha diversity compared to chemical fertilization (Tang et al., 2020). In a broccoli system, soil bacterial community richness index was significantly increased by short-term application of chicken manure, but there was no significant difference in soil bacterial Shannon diversity index between chicken manure application and control soil (Ye et al., 2022). In a winter wheat-summer maize system, there was no significant difference in Chao1 and Shannon indices of soil bacterial and fungal community between short-term manure and chemical fertilizer treatments (Wang et al., 2022). In a rice-wheat rotation system, long-term manure application had no significant effect on Shannon index of soil bacterial and fungal community, and Chao1 index of soil fungal community, while significantly increased the Chao1 index of soil bacterial community (Wang J. et al., 2017). The results of this and previous studies showed that soil microbial community was acclimated to manure application, and the reasons for the inconsistent results in different experiments may be related to the soil and manure types.
Wang et al. found that fertilization regime of wheat and rice did not change the soil bacterial community diversity, but changed the soil bacterial community structure (Wang et al., 2016). Similarly, the results showed that the application of manure had no significant effect on the diversity, but significantly changed the structure of soil microbial community (Figure 3), which indicated that soil microbial community structure was more sensitive than soil microbial community diversity to the application of manure. Our results echoed the viewpoint of previous studies what the soil bacterial and fungal community structure could be significantly affected by manure application (Gu et al., 2019; Lin et al., 2019; Ren et al., 2021). In line with the previous research (Wei et al., 2017; Luan et al., 2020), the results of NMDS and ANOSIM showed that the impact of manure application on soil microbial community structure was more significant than that of nitrogen rate, and manure application and its interaction with nitrogen rate significantly changed soil bacterial and fungal community structure (P < 0.05) (Figure 3 and Table 2). It was suggested that the mineralization of organic matter in manure is a long-term process, which is difficult to fully exert its nutritional potential on soil microbial community in a short-term period (Zhang M. et al., 2021). Chemical nitrogen fertilizers had stronger effect on soil microbial community than manure under short-term condition, as nitrogen addition could increase microbial activities by mitigating nitrogen limitation (Zhang M. et al., 2021). While in this study, nitrogen may not be the main factor limiting soil microbial growth. Mantel test results further demonstrated that soil microbial community structure was not significantly correlated with soil mineral nitrogen, but significantly correlated with soil available phosphorus and potassium (Table 3). The results were supported by Ma et al. who found that phosphorus and phosphorus-related nutrient stoichiometry drove the changes of soil microbial community composition in paddy soil (Ma et al., 2016). Additionally, livestock and poultry manure is rich in gut microbial communities (Bebber and Richards, 2022), and the microorganisms in manure may also have an impact on the structure of soil microbial community. Studies have shown that gut microorganisms could survive in manure- or compost- amended soils for a long time (Sharma and Reynnells, 2016), and diverse fungal taxa in manure were transmitted into soil by the application of manure, which account for 1.10-2.04% of the relative abundance of soil fungal community (Sun et al., 2020). Therefore, the effect of manure application on soil microbial community structure could also be attributed to the microbial community brought by manure itself.
This study used the same LDA threshold for soil bacterial and fungal communities to detect the differential taxa between treatments with and without manure application. The results showed that the number of differential taxa of fungi was more than that of bacteria (Supplementary Table 1), which indicated that the effect of manure application on composition of fungal community was greater than that of bacterial community. For soil bacterial community, manure application reduced relative abundance of the family Comamonadaceae. Certain members of the family Comamonadaceae have been reported to exhibit extensive metabolic pathways, such as degradation of cyclohexane, accumulation of polyphosphate, denitrification, etc. (Ding et al., 2019). Previous results confirmed that exogenous fertilizers significantly affected an unclassified Comamonadaceae, and the unclassified Comamonadaceae was negatively correlated with soil nitrogen mineralization (Wu et al., 2021). The results also showed that the order Gaiellales and genus Luteimonas were significantly enriched in manure application treatments. In a field experiment with Ultisols, Gaiellales was also found to be enriched by pig manure application (Lin et al., 2019). Luteimonas is a nirK-type denitrifier, which is associated with soil nitrogen loss and nitrous oxide emission (Zhong et al., 2020). For soil fungal community, manure application reduced the relative abundance of potential pathogens, such as Alternaria (Lou et al., 2013), Setophoma (Ikeda et al., 2012; Yoshida, 2022), unclassified Sordariomycetes (Maharachchikumbura et al., 2015, 2016). Among the genus-level taxa enriched by manure application, Dactylaria and Cutaneotrichosporon were potentially beneficial fungi. In previous studies, Dactylaria brochopaga of the genus Dactylaria could manage root-knot disease of wheat by trapping Meloidogyne graminicola (Kumar and Singh, 2011). Dactylaria higginsii of the genus Dactylaria could be used as fungal bioherbicide agent for purple nutsedge (Kadir and Charudattan, 2000). Cutaneotrichosporon was negatively associated with Fusarium oxysporum, and could inhibit the infection of Fusarium oxysporum (Zhu et al., 2022). Notably, manure application also enriched coprophilous fungi such as Niesslia, Cephaliophora, Microascus and Chaetomiaceae (Dischler et al., 2019; Zhang X. et al., 2021). Due to the lack of sufficient culture studies for some differential species, it was difficult to estimate the ecological impact brought about by the changes in their relative abundance. It could be confirmed that the differences in soil microbial community composition caused by manure application would further affect soil ecological function. On the other hand, the different composition of soil microbial community may also cause changes in the interactions among soil microbial taxa.
Various microbial taxa do not exist independently in ecosystem, but interact with each other to form a complex network (Zhou et al., 2010). The co-occurrence network analysis could explore the interactions among soil microbial taxa (Zhang M. et al., 2021), which play an important role in ecosystem process and function. In this study, the response of co-occurrence network to manure application was different for bacterial and fungal communities (Figure 4 and Table 4). After one growing season, applying manure decreased the average number of neighbors, clustering coefficient and network density of soil bacterial ecological network, but increased those of soil fungal ecological network, which implied that manure application decreased the complexity of soil bacterial ecological network and increased the complexity of soil fungal ecological network. The previous study has shown that the effect of manure application on soil bacterial network was related to the application rate, that is, low manure application rate could lead to complex and stable bacterial community, while high manure application rate decreased the stability of bacterial network (Liu et al., 2020). For soil fungal community, previous studies have consistently shown that manure application could increase the network edges, average degree and density (Wang et al., 2022), and enhance the network complexity (Ji et al., 2020). As the C/N ratio of fungal hyphae is higher than that of bacterial cells (Wallander et al., 2003), bacteria and fungi often exhibit different substrate preference. Manure application increased soil carbon input and the C/N ratio, which was beneficial to the growth of soil fungi (Grosso et al., 2016). Therefore, it was reasonable that manure application increased the interaction between soil fungal species. The topological data indicated that the increase of edge number in soil fungal network was mainly due to the change of positive links (Table 4). Positive correlations between microorganisms in ecological network could be explained by common preferred conditions or cooperative activities (Fuhrman, 2009). It could be speculated that the recalcitrant organic matters introduced by manure application required more microbial cooperation to be degraded, which resulted in a complex fungal ecological network (Ye et al., 2021). The more complex network indicates that there is a closer interaction among microorganisms, which would be beneficial to resist the environmental stresses (Ling et al., 2016). In this study, manure application decreased the number of mutual exclusion edges in the ecological network of soil total microbial community. The studies of ecological theory showed that competitive interactions could promote stability of ecological networks (Coyte et al., 2015). It is worth noting that interspecific interactions within microbial community are highly complex, further studies with more experimental evidence are needed to compensate for the limitations of co-occurrence network analysis only (Banerjee et al., 2018; Guseva et al., 2022; Jin et al., 2022).
In general, this study showed that application of chicken manure could alter soil properties, microbial community structure, species composition and co-occurrence pattern in a short-term period. At the same time, it should be noted that manure is also a pollution source rich in heavy metals, antibiotics and antibiotic resistance genes (Zhao Y. et al., 2014; Guo et al., 2018), and the potential environmental risks of long-term manure application under the condition of this study need to be further evaluated.
Results in this study showed that one growing season application of chicken manure significantly increased soil available phosphorus under different nitrogen rates, significantly increased soil organic carbon and mineral nitrogen under low nitrogen rate, and significantly increased soil available potassium under high nitrogen rate. The response of soil bacterial and fungal abundance to applying chicken manure varied with different nitrogen rates. Chicken manure application and nitrogen rate did not significantly affect soil microbial community alpha diversity in a short term, while applying chicken manure significantly changed soil microbial community structure and species composition. The application of chicken manure reduced the complexity of soil bacterial ecological network and increased the complexity of soil fungal ecological network.
The datasets presented in this study can be found in online repositories. The names of the repository/repositories and accession number(s) can be found below: https://www.ncbi.nlm.nih.gov/, PRJNA851220.
HJ, DZ, and XL designed the work. HJ, DZ, and YY analyzed the data and wrote the manuscript. CY, BF, and JY performed laboratory measurements. HC and YaS carried out field sampling. YuS, HW, and FQ performed the field experiment. YW and XL revised the manuscript. All authors discussed the results and approved the final manuscript.
This work was supported by the Zhongyuan Science and Technology Innovation Leading Talents Program (224200510028), the National Key Research and Development Project of China (SQ2020YFF0426480), the Zhongyuan Young Postdoctoral Innovative Talents Program (ZYYCYU202012184), the Science and Technology Innovation Team Program of Henan Academy of Agricultural Sciences (2022TD09), and the Special Fund for Henan Agriculture Research System (HARS-22-01-G5).
We thank all persons in our lab for offering the constructive suggestions on this work.
The authors declare that the research was conducted in the absence of any commercial or financial relationships that could be construed as a potential conflict of interest.
All claims expressed in this article are solely those of the authors and do not necessarily represent those of their affiliated organizations, or those of the publisher, the editors and the reviewers. Any product that may be evaluated in this article, or claim that may be made by its manufacturer, is not guaranteed or endorsed by the publisher.
The Supplementary Material for this article can be found online at: https://www.frontiersin.org/articles/10.3389/fmicb.2022.975571/full#supplementary-material
Anas, M., Liao, F., Verma, K. K., Sarwar, M. A., Mahmood, A., Chen, Z.-L., et al. (2020). Fate of nitrogen in agriculture and environment: agronomic, eco-physiological and molecular approaches to improve nitrogen use efficiency. Biol. Res. 53:47. doi: 10.1186/s40659-020-00312-4
Anderson, T. H. (2003). Microbial eco-physiological indicators to asses soil quality. Agric. Ecosyst. Environ. 98, 285–293. doi: 10.1016/s0167-8809(03)00088-4
Assenov, Y., Ramírez, F., Schelhorn, S.-E., Lengauer, T., and Albrecht, M. (2008). Computing topological parameters of biological networks. Bioinformatics 24, 282–284. doi: 10.1093/bioinformatics/btm554
Bai, Z. H., Ma, W. Q., Ma, L., Velthof, G. L., Wei, Z. B., Havlik, P., et al. (2018). China’s livestock transition: driving forces, impacts, and consequences. Sci. Adv. 4:eaar8534. doi: 10.1126/sciadv.aar8534
Banerjee, S., Schlaeppi, K., and Van Der Heijden, M. G. A. (2018). Keystone taxa as drivers of microbiome structure and functioning. Nat. Rev. Microbiol. 16, 567–576. doi: 10.1038/s41579-018-0024-1
Bebber, D. P., and Richards, V. R. (2022). A meta-analysis of the effect of organic and mineral fertilizers on soil microbial diversity. Appl. Soil Ecol. 175:104450. doi: 10.1016/j.apsoil.2022.104450
Chen, S., Zhou, Y., Chen, Y., and Gu, J. (2018). fastp: an ultra-fast all-in-one FASTQ preprocessor. Bioinformatics 34, i884–i890. doi: 10.1093/bioinformatics/bty560
Coyte, K. Z., Schluter, J., and Foster, K. R. (2015). The ecology of the microbiome: networks, competition, and stability. Science 350, 663–666. doi: 10.1126/science.aad2602
Ding, Y. P., Khan, I. U., Li, M. M., Xian, W. D., Liu, L., Zhou, E. M., et al. (2019). Calidifontimicrobium sediminis gen. nov., sp. nov., a new member of the family Comamonadaceae. Int. J. Syst. Evol. Microbiol. 69, 434–440. doi: 10.1099/ijsem.0.003167
Dischler, N. M., Xu, L. J., Li, Y., Nichols, C. B., Alspaugh, J. A., Bills, G. F., et al. (2019). Wortmannin and wortmannine analogues from an undescribed Niesslia sp. J. Nat. Product. 82, 532–538. doi: 10.1021/acs.jnatprod.8b00923
Du, Y. D., Cui, B. J., Zhang, Q., Wang, Z., Sun, J., and Niu, W. Q. (2020). Effects of manure fertilizer on crop yield and soil properties in China: a meta-analysis. Catena 193:104617. doi: 10.1016/j.catena.2020.104617
Edgar, R. C. (2010). Search and clustering orders of magnitude faster than BLAST. Bioinformatics 26, 2460–2461. doi: 10.1093/bioinformatics/btq461
Erisman, J. W., Sutton, M. A., Galloway, J., Klimont, Z., and Winiwarter, W. (2008). How a century of ammonia synthesis changed the world. Nat. Geosci. 1, 636–639. doi: 10.1038/ngeo325
Faust, K., and Raes, J. (2016). CoNet app: inference of biological association networks using Cytoscape. F1000Research 5:1519. doi: 10.12688/f1000research.9050.2
Fuhrman, J. A. (2009). Microbial community structure and its functional implications. Nature 459, 193–199. doi: 10.1038/nature08058
Gade, L., Scheel, C. M., Pham, C. D., Lindsley, M. D., Iqbal, N., Cleveland, A. A., et al. (2013). Detection of fungal DNA in human body fluids and tissues during a multistate outbreak of fungal meningitis and other infections. Eukaryot. Cell 12, 677–683. doi: 10.1128/EC.00046-13
Grosso, F., Bååth, E., and De Nicola, F. (2016). Bacterial and fungal growth on different plant litter in Mediterranean soils: effects of C/N ratio and soil pH. Appl. Soil Ecol. 108, 1–7. doi: 10.1016/j.apsoil.2016.07.020
Gu, S., Hu, Q., Cheng, Y., Bai, L., Liu, Z., Xiao, W., et al. (2019). Application of organic fertilizer improves microbial community diversity and alters microbial network structure in tea (Camellia sinensis) plantation soils. Soil Till. Res. 195:104356. doi: 10.1016/j.still.2019.104356
Guo, J. H., Liu, X. J., Zhang, Y., Shen, J. L., Han, W. X., Zhang, W. F., et al. (2010). Significant acidification in major Chinese croplands. Science 327, 1008–1010. doi: 10.1126/science.1182570
Guo, T., Lou, C., Zhai, W., Tang, X., Hashmi, M. Z., Murtaza, R., et al. (2018). Increased occurrence of heavy metals, antibiotics and resistance genes in surface soil after long-term application of manure. Sci. Total Environ. 635, 995–1003. doi: 10.1016/j.scitotenv.2018.04.194
Guo, Z., Wan, S., Hua, K., Yin, Y., Chu, H., Wang, D., et al. (2020). Fertilization regime has a greater effect on soil microbial community structure than crop rotation and growth stage in an agroecosystem. Appl. Soil Ecol. 149:103510. doi: 10.1016/j.apsoil.2020.103510
Guseva, K., Darcy, S., Simon, E., Alteio, L. V., Montesinos-Navarro, A., and Kaiser, C. (2022). From diversity to complexity: microbial networks in soils. Soil Biol. Biochem. 169:108604. doi: 10.1016/j.soilbio.2022.108604
Ikeda, K., Kuwabara, K., Urushibara, T., Soyai, P., Miki, S., and Shibata, S. (2012). Pink root rot of squash caused by Setophoma terrestris in Japan. J. Gen. Plant. Pathol. 78, 372–375. doi: 10.1007/s10327-012-0398-2
Ji, L., Ni, K., Wu, Z., Zhang, J., Yi, X., Yang, X., et al. (2020). Effect of organic substitution rates on soil quality and fungal community composition in a tea plantation with long-term fertilization. Biol. Fert. Soils 56, 633–646. doi: 10.1007/s00374-020-01439-y
Ji, L., Si, H., He, J., Fan, L., and Li, L. (2021). The shifts of maize soil microbial community and networks are related to soil properties under different organic fertilizers. Rhizosphere 19:100388. doi: 10.1016/j.rhisph.2021.100388
Jin, X., Wang, Z. L., Wu, F. Z., Li, X. G., and Zhou, X. G. (2022). Litter mixing alters microbial decomposer community to accelerate tomato root litter decomposition. Microbiol. Spectr. 10:e00186-22. doi: 10.1128/spectrum.00186-22
Kadir, J., and Charudattan, R. (2000). Dactylaria higginsii, a fungal bioherbicide agent for purple nutsedge (Cyperus rotundus). Biol. Control 17, 113–124. doi: 10.1006/bcon.1999.0791
Khaliq, A., and Abbasi, M. K. (2015). Improvements in the physical and chemical characteristics of degraded soils supplemented with organic-inorganic amendments in the Himalayan region of Kashmir, Pakistan. Catena 126, 209–219. doi: 10.1016/j.catena.2014.11.015
Kobierski, M., Bartkowiak, A., Lemanowicz, J., and Piekarczyk, M. (2017). Impact of poultry manure fertilization on chemical and biochemical properties of soils. Plant Soil Environ. 63, 558–563. doi: 10.17221/668/2017-pse
Kumar, N., and Singh, K. P. (2011). Use of Dactylaria brochopaga, a predacious fungus, for managing root-knot disease of wheat (Triticum aestivum) caused by Meloidogyne graminicola. Mycobiology 39, 113–117. doi: 10.4489/MYCO.2011.39.2.113
Legrand, F., Picot, A., Cobo-Díaz, J. F., Carof, M., Chen, W., and Le Floch, G. (2018). Effect of tillage and static abiotic soil properties on microbial diversity. Appl. Soil Ecol. 132, 135–145. doi: 10.1016/j.apsoil.2018.08.016
Li, J., Cooper, J. M., Lin, Z. A., Li, Y., Yang, X., and Zhao, B. (2015). Soil microbial community structure and function are significantly affected by long-term organic and mineral fertilization regimes in the North China Plain. Appl. Soil Ecol. 96, 75–87. doi: 10.1016/j.apsoil.2015.07.001
Liang, J., Zhou, Z., Huo, C., Shi, Z., Cole, J. R., Huang, L., et al. (2018). More replenishment than priming loss of soil organic carbon with additional carbon input. Nat. Commun. 9:3175. doi: 10.1038/s41467-018-05667-7
Lin, Y., Ye, G., Kuzyakov, Y., Liu, D., Fan, J., and Ding, W. (2019). Long-term manure application increases soil organic matter and aggregation, and alters microbial community structure and keystone taxa. Soil Biol. Biochem. 134, 187–196. doi: 10.1016/j.soilbio.2019.03.030
Ling, N., Zhu, C., Xue, C., Chen, H., Duan, Y. H., Peng, C., et al. (2016). Insight into how organic amendments can shape the soil microbiome in long-term field experiments as revealed by network analysis. Soil Biol. Biochem. 99, 137–149. doi: 10.1016/j.soilbio.2016.05.005
Liu, H., Huang, X., Tan, W., Di, H., Xu, J., and Li, Y. (2020). High manure load reduces bacterial diversity and network complexity in a paddy soil under crop rotations. Soil Ecol. Lett. 2, 104–119. doi: 10.1007/s42832-020-0032-8
Liu, J., Shu, A., Song, W., Shi, W., Li, M., Zhang, W., et al. (2021). Long-term organic fertilizer substitution increases rice yield by improving soil properties and regulating soil bacteria. Geoderma 404:115287. doi: 10.1016/j.geoderma.2021.115287
Lou, J., Fu, L., Peng, Y., and Zhou, L. (2013). Metabolites from alternaria fungi and their bioactivities. Molecules 18, 5891–5935. doi: 10.3390/molecules18055891
Luan, H., Gao, W., Huang, S., Tang, J., Li, M., Zhang, H., et al. (2020). Substitution of manure for chemical fertilizer affects soil microbial community diversity, structure and function in greenhouse vegetable production systems. PLoS One 15:e0214041. doi: 10.1371/journal.pone.0214041
Ma, X., Liu, M., and Li, Z. (2016). Shifts in microbial biomass and community composition in subtropical paddy soils under a gradient of manure amendment. Biol. Fertil. Soils 52, 775–787. doi: 10.1007/s00374-016-1118-4
Magoč, T., and Salzberg, S. L. (2011). FLASH: fast length adjustment of short reads to improve genome assemblies. Bioinformatics 27, 2957–2963. doi: 10.1093/bioinformatics/btr507
Maharachchikumbura, S. S. N., Hyde, K. D., Jones, E. B. G., Mckenzie, E. H. C., Bhat, J. D., Dayarathne, M. C., et al. (2016). Families of Sordariomycetes. Fungal Divers. 79, 1–317. doi: 10.1007/s13225-016-0369-6
Maharachchikumbura, S. S. N., Hyde, K. D., Jones, E. B. G., Mckenzie, E. H. C., Huang, S.-K., Abdel-Wahab, M. A., et al. (2015). Towards a natural classification and backbone tree for Sordariomycetes. Fungal Divers. 72, 199–301. doi: 10.1007/s13225-015-0331-z
Nilsson, R. H., Larsson, K.-H., Taylor, A. F. S., Bengtsson-Palme, J., Jeppesen, T. S., Schigel, D., et al. (2019). The UNITE database for molecular identification of fungi: handling dark taxa and parallel taxonomic classifications. Nucleic Acids Res. 47, D259–D264. doi: 10.1093/nar/gky1022
Pruesse, E., Quast, C., Knittel, K., Fuchs, B. M., Ludwig, W., Peplies, J., et al. (2007). SILVA: a comprehensive online resource for quality checked and aligned ribosomal RNA sequence data compatible with ARB. Nucleic Acids Res. 35, 7188–7196. doi: 10.1093/nar/gkm864
Ren, J., Liu, X., Yang, W., Yang, X., Li, W., Xia, Q., et al. (2021). Rhizosphere soil properties, microbial community, and enzyme activities: short-term responses to partial substitution of chemical fertilizer with organic manure. J. Environ. Manag. 299:113650. doi: 10.1016/j.jenvman.2021.113650
Ren, Y., Yu, G., Shi, C., Liu, L., Guo, Q., Han, C., et al. (2022). Majorbio cloud: a one-stop, comprehensive bioinformatic platform for multiomics analyses. iMeta 1:e12. doi: 10.1002/imt2.12
Schloss, P. D. (2020). Reintroducing mothur: 10 years later. Appl. Environ. Microbiol. 86:e02343-19. doi: 10.1128/AEM.02343-19
Schloter, M., Nannipieri, P., Sørensen, S. J., and Van Elsas, J. D. (2018). Microbial indicators for soil quality. Biol. Fertil. Soils 54, 1–10. doi: 10.1007/s00374-017-1248-3
Segata, N., Izard, J., Waldron, L., Gevers, D., Miropolsky, L., Garrett, W. S., et al. (2011). Metagenomic biomarker discovery and explanation. Genome Biol. 12:R60. doi: 10.1186/gb-2011-12-6-r60
Sharma, M., and Reynnells, R. (2016). Importance of soil amendments: survival of bacterial pathogens in manure and compost used as organic fertilizers. Microbiol. Spectr. 4:10.
Shen, P., Xu, M., Zhang, H., Yang, X., Huang, S., Zhang, S., et al. (2014). Long-term response of soil Olsen P and organic C to the depletion or addition of chemical and organic fertilizers. Catena 118, 20–27. doi: 10.1016/j.catena.2014.01.020
Song, H., Peng, L., Li, Z., Deng, X., Shao, J., and Gu, J.-D. (2019). Metal distribution and biological diversity of crusts in paddy fields polluted with different levels of cadmium. Ecotoxiol. Environ. Saf. 184:109620. doi: 10.1016/j.ecoenv.2019.109620
Sun, R., Chen, Y., Han, W., Dong, W., Zhang, Y., Hu, C., et al. (2020). Different contribution of species sorting and exogenous species immigration from manure to soil fungal diversity and community assemblage under long-term fertilization. Soil Biol. Biochem. 151:108049. doi: 10.1016/j.soilbio.2020.108049
Tang, H., Li, C., Xiao, X., Shi, L., Cheng, K., Wen, L., et al. (2020). Effects of short-term manure nitrogen input on soil microbial community structure and diversity in a double-cropping paddy field of southern China. Sci. Rep. 10:13540. doi: 10.1038/s41598-020-70612-y
Tang, Q., Ti, C. P., Xia, L. L., Xia, Y. Q., Wei, Z. J., and Yan, X. Y. (2019). Ecosystem services of partial organic substitution for chemical fertilizer in a peri-urban zone in China. J. Clean. Product. 224, 779–788. doi: 10.1016/j.jclepro.2019.03.201
Wallander, H., Nilsson, L. O., Hagerberg, D., and Rosengren, U. (2003). Direct estimates of C:N ratios of ectomycorrhizal mycelia collected from Norway spruce forest soils. Soil Biol. Biochem. 35, 997–999. doi: 10.1016/S0038-0717(03)00121-4
Wang, H., Xu, J., Liu, X., Zhang, D., Li, L., Li, W., et al. (2019). Effects of long-term application of organic fertilizer on improving organic matter content and retarding acidity in red soil from China. Soil Till. Res. 195:104382. doi: 10.1016/j.still.2019.104382
Wang, H., Zhang, Y., Chen, A., Liu, H., Zhai, L., Lei, B., et al. (2017). An optimal regional nitrogen application threshold for wheat in the North China Plain considering yield and environmental effects. Field Crop. Res. 207, 52–61. doi: 10.1016/j.fcr.2017.03.002
Wang, J., Song, Y., Ma, T., Raza, W., Li, J., Howland, J. G., et al. (2017). Impacts of inorganic and organic fertilization treatments on bacterial and fungal communities in a paddy soil. Appl. Soil Ecol. 112, 42–50. doi: 10.1016/j.apsoil.2017.01.005
Wang, J., Xue, C., Song, Y., Wang, L., Huang, Q., and Shen, Q. (2016). Wheat and rice growth stages and fertilization regimes alter soil bacterial community structure, but not diversity. Front. Microbiol. 7:1207. doi: 10.3389/fmicb.2016.01207
Wang, P., Wang, X., Nie, J., Wang, Y., Zang, H., Peixoto, L., et al. (2022). Manure application increases soil bacterial and fungal network complexity and alters keystone taxa. J. Soil Sci. Plant Nutr. 22, 607–618. doi: 10.1007/s42729-021-00673-z
Wang, Q., Garrity, G. M., Tiedje, J. M., and Cole, J. R. (2007). Naïve bayesian classifier for rapid assignment of rRNA sequences into the new bacterial taxonomy. Appl. Environ. Microb. 73, 5261–5267. doi: 10.1128/AEM.00062-07
Wei, M., Hu, G., Wang, H., Bai, E., Lou, Y., Zhang, A., et al. (2017). 35 years of manure and chemical fertilizer application alters soil microbial community composition in a Fluvo-aquic soil in Northern China. Eur. J. Soil Biol. 82, 27–34. doi: 10.1016/j.ejsobi.2017.08.002
Wei, Z., Ying, H., Guo, X., Zhuang, M., Cui, Z., and Zhang, F. (2020). Substitution of mineral fertilizer with organic fertilizer in maize systems: a meta-analysis of reduced nitrogen and carbon emissions. Agronomy 10:1149. doi: 10.3390/agronomy10081149
Wu, H., Cai, A., Xing, T., Huai, S., Zhu, P., Xu, M., et al. (2021). Fertilization enhances mineralization of soil carbon and nitrogen pools by regulating the bacterial community and biomass. J. Soil. Sediment. 21, 1633–1643. doi: 10.1007/s11368-020-02865-z
Xin, X., Qin, S., Zhang, J., Zhu, A., Yang, W., and Zhang, X. (2017). Yield, phosphorus use efficiency and balance response to substituting long-term chemical fertilizer use with organic manure in a wheat-maize system. Field Crop Res. 208, 27–33. doi: 10.1016/j.fcr.2017.03.011
Ye, C., Huang, S., Sha, C., Wu, J., Cui, C., Su, J., et al. (2022). Changes of bacterial community in arable soil after short-term application of fresh manures and organic fertilizer. Environ. Technol. 43, 824–834. doi: 10.1080/09593330.2020.1807608
Ye, G., Banerjee, S., He, J.-Z., Fan, J., Wang, Z., Wei, X., et al. (2021). Manure application increases microbiome complexity in soil aggregate fractions: results of an 18-year field experiment. Agric. Ecosyst. Environ. 307:107249. doi: 10.1016/j.agee.2020.107249
Yoshida, N. (2022). Seasonal dynamics of the pink root fungus (Setophoma terrestris) in rhizosphere soil: effect of crop species and rotation. Plant Pathol. 71, 361–372. doi: 10.1111/ppa.13464
Zechmeister-Boltenstern, S., Keiblinger, K. M., Mooshammer, M., Penuelas, J., Richter, A., Sardans, J., et al. (2015). The application of ecological stoichiometry to plant-microbial-soil organic matter transformations. Ecol. Monogr. 85, 133–155. doi: 10.1890/14-0777.1
Zhang, M., Yao, Y., Tian, Y., Ceng, K., Zhao, M., Zhao, M., et al. (2018). Increasing yield and N use efficiency with organic fertilizer in Chinese intensive rice cropping systems. Field Crop Res. 227, 102–109. doi: 10.1016/j.fcr.2018.08.010
Zhang, M., Zhang, X., Zhang, L., Zeng, L., Liu, Y., Wang, X., et al. (2021). The stronger impact of inorganic nitrogen fertilization on soil bacterial community than organic fertilization in short-term condition. Geoderma 382:114752. doi: 10.1016/j.geoderma.2020.114752
Zhang, W.-F., Dou, Z.-X., He, P., Ju, X.-T., Powlson, D., Chadwick, D., et al. (2013). New technologies reduce greenhouse gas emissions from nitrogenous fertilizer in China. Proc. Natl. Acad. Sci. U.S.A. 110, 8375–8380. doi: 10.1073/pnas.1210447110
Zhang, X., Zhang, J., Jiang, L., Yu, X., Zhu, H., Zhang, J., et al. (2021). Black soldier fly (Hermetia illucens) larvae significantly change the microbial community in chicken manure. Curr. Microbiol. 78, 303–315. doi: 10.1007/s00284-020-02276-w
Zhao, S., Qiu, S., Cao, C., Zheng, C., Zhou, W., and He, P. (2014). Responses of soil properties, microbial community and crop yields to various rates of nitrogen fertilization in a wheat–maize cropping system in north-central China. Agric. Ecosyst. Environ. 194, 29–37. doi: 10.1016/j.agee.2014.05.006
Zhao, Y., Yan, Z., Qin, J., and Xiao, Z. (2014). Effects of long-term cattle manure application on soil properties and soil heavy metals in corn seed production in Northwest China. Environ. Sci. Pollut. Res. 21, 7586–7595. doi: 10.1007/s11356-014-2671-8
Zhong, W., Gu, T., Wang, W., Zhang, B., Lin, X., Huang, Q., et al. (2010). The effects of mineral fertilizer and organic manure on soil microbial community and diversity. Plant Soil 326, 511–522. doi: 10.1007/s11104-009-9988-y
Zhong, X.-Z., Zeng, Y., Wang, S.-P., Sun, Z.-Y., Tang, Y.-Q., and Kida, K. (2020). Insight into the microbiology of nitrogen cycle in the dairy manure composting process revealed by combining high-throughput sequencing and quantitative PCR. Bioresour. Technol. 301:122760. doi: 10.1016/j.biortech.2020.122760
Zhou, J., Deng, Y., Luo, F., He, Z., Tu, Q., Zhi, X., et al. (2010). Functional molecular ecological networks. mBio 1:e00169-10. doi: 10.1128/mBio.00169-10
Zhou, J., Jiang, X., Zhou, B., Zhao, B., Ma, M., Guan, D., et al. (2016). Thirty four years of nitrogen fertilization decreases fungal diversity and alters fungal community composition in black soil in northeast China. Soil Biol. Biochem. 95, 135–143. doi: 10.1016/j.soilbio.2015.12.012
Keywords: manure, soil, microbial community structure, microbial community diversity, co-occurrence network, winter wheat
Citation: Jin H, Zhang D, Yan Y, Yang C, Fang B, Li X, Shao Y, Wang H, Yue J, Wang Y, Cheng H, Shi Y and Qin F (2022) Short-term application of chicken manure under different nitrogen rates alters structure and co-occurrence pattern but not diversity of soil microbial community in wheat field. Front. Microbiol. 13:975571. doi: 10.3389/fmicb.2022.975571
Received: 22 June 2022; Accepted: 12 August 2022;
Published: 07 September 2022.
Edited by:
Yongchun Li, Zhejiang Agriculture and Forestry University, ChinaReviewed by:
Jia Liu, Jiangxi Academy of Agricultural Sciences (CAAS), ChinaCopyright © 2022 Jin, Zhang, Yan, Yang, Fang, Li, Shao, Wang, Yue, Wang, Cheng, Shi and Qin. This is an open-access article distributed under the terms of the Creative Commons Attribution License (CC BY). The use, distribution or reproduction in other forums is permitted, provided the original author(s) and the copyright owner(s) are credited and that the original publication in this journal is cited, in accordance with accepted academic practice. No use, distribution or reproduction is permitted which does not comply with these terms.
*Correspondence: Xiangdong Li, aG5seGRAMTI2LmNvbQ==; Deqi Zhang, ZHF6aGFuZzNAMTYzLmNvbQ==
†These authors have contributed equally to this work
Disclaimer: All claims expressed in this article are solely those of the authors and do not necessarily represent those of their affiliated organizations, or those of the publisher, the editors and the reviewers. Any product that may be evaluated in this article or claim that may be made by its manufacturer is not guaranteed or endorsed by the publisher.
Research integrity at Frontiers
Learn more about the work of our research integrity team to safeguard the quality of each article we publish.