- Wenzhou Key Laboratory of Sanitary Microbiology, Key Laboratory of Laboratory Medicine, Ministry of Education, School of Laboratory Medicine and Life Sciences, Wenzhou Medical University, Wenzhou, Zhejiang, China
Aeromonas hydrophila is an emerging waterborne and foodborne pathogen with pathogenicity to humans and warm water fishes, which severely threatens human health, food safety and aquaculture. A novel method for the rapid, accurate, and sensitive detection of pathogenic A. hydrophila is still needed to reduce the impact on human health and aquaculture. In this work, we developed a rapid, accurate, sensitive, and visual detection method (dRAA-CRISPR/Cas12a), without elaborate instruments, integrating the dualplex recombinase-assisted amplification (dRAA) assay and CRISPR/Cas12a system to detect pathogenic A. hydrophila expressing aerA and/or hlyA virulence genes. The dRAA-CRISPR/Cas12a method has high sensitivity, which can rapidly detect (about 45 min) A. hydrophila with the limit of detection in 2 copies of genomic DNA per reaction, and has high specificity for three pathogenic A. hydrophila strains (aerA+hlyA−, aerA−hlyA+, and aerA+hlyA+). Moreover, dRAA-CRISPR/Cas12a method shows satisfactory practicability in the analysis of the spiked human blood and stool and fish samples. These results demonstrate that our developed pathogenic A. hydrophila detection method, dRAA-CRISPR/Cas12a, is a promising potential method for the early diagnosis of human A. hydrophila infection and on-site detection of A. hydrophila in food and aquaculture.
Introduction
Aeromonas hydrophila is a widespread aquatic and zoonotic pathogen (Daskalov, 2006; Li et al., 2011). In animals, A. hydrophila mainly causes diseases in aquaculture animals, such as the motile Aeromonas septicemia and red sore disease in warm water fishes (Janda and Abbott, 2010), resulting in huge economic losses and food safety risks. In humans, A. hydrophila was initially thought to be an opportunistic pathogen for immunocompromised populations (Balsalobre et al., 2009), but a growing body of research indicates that it is an emerging enteric (Özbaş et al., 2000), waterborne (Chauret et al., 2001), and foodborne (Daskalov, 2006) pathogen regardless of the immune status of the host (Balsalobre et al., 2009).
The pathogenicity of A. hydrophila is closely related to its production of virulence factors (Janda and Abbott, 2010; Tichoniuk et al., 2010; Li et al., 2011). It has been reported that the pathogenic A. hydrophila can survive and multiply in water, soil and various foods (milk, fish, raw meat, etc.) at room temperature and low temperature (Balakrishna et al., 2010; Janda and Abbott, 2010), and produce virulence factors aerolysin and/or hemolysin (Wong et al., 1998; Heuzenroeder et al., 1999; Balakrishna et al., 2010; Janda and Abbott, 2010). Patients infected with A. hydrophila can develop sepsis and necrotizing fasciitis, which have an acute onset and rapid progression (Tsai et al., 2015; Sun et al., 2021) and are life-threatening, mainly through ingestion of contaminated water and food and exposed wounds, respectively (Meng et al., 2015); moreover, sepsis can reach a state of systemic toxicity within 24 h (Zhu et al., 2021). In view of these aspects, a rapid, accurate, and sensitive detection method of pathogenic A. hydrophila would contribute to improving the treatment and control strategies and then reducing the hazard of this bacterial infection.
Nowadays, many methods can be used for the detection of A. hydrophila. The traditional culture method is accurate, but it takes a long time. The biochemical test is complicated, and only typical strains can be detected because of the limited biochemical characteristics (Abbott et al., 2003). Enzyme-linked immunosorbent assay (ELISA; Swain et al., 2003), dot blotting (Longyant et al., 2010), and serotyping (Nielsen et al., 2001) have low diagnostic sensitivity. Over the past decade, a large number of assays based on PCR technology have been developed to detect A. hydrophila by targeting virulence gene (Wang et al., 2003; Hussain et al., 2014). However, these methods require professional personnel and specialized equipment and are not suitable for on-site application or resource-constrained areas. In recent years, isothermal nucleic acid amplification technologies, such as recombinase-aided amplification (RAA; Piepenburg et al., 2006; Subsoontorn et al., 2020) and loop-mediated isothermal amplification (Nagamine et al., 2002), have promoted the development of nucleic acid amplification without thermal cyclers. RAA assay that only requires one primer set and can be reacted even at body temperature of operator (Wang et al., 2017) has been used for the detection of pathogens such as A. hydrophila (Qu et al., 2021) and Enterocytozoon hepatopenaei (Zhou et al., 2020), but this method has low sensitivity (Xiao et al., 2021) and the result is complicated to obtain (Zhou et al., 2020).
Recently, the discovery of the trans-cleavage activity of clustered regularly interspaced short palindromic repeats (CRISPR)-associated protein Cas12a has made the CRISPR/Cas12a system a hot spot in the field of in vitro diagnostics (Chen et al., 2018; Li et al., 2018a,b). Chen et al. developed a DETECTR platform, consisting of a RPA assay and the CRISPR/Cas12a system, with aM sensitivity and high specificity to distinguish between HPV16 and HPV18 (Chen et al., 2018). Zhang et al. used PCR assay and CRISPR/Cas12a system to develop an integrated naked-eye detection method for the tlh gene of Vibrio parahaemolyticus with a detection limit of 1.02 × 102 copies/μl (Zhang et al., 2020). Because of its speed, sensitivity, specificity, and simplicity, DETECTR platform is a promising method to be used for on-site detection, and has been performed to detect pathogens including Listeria monocytogenes (Li et al., 2021), Vibrio vulnificus (Xiao et al., 2021), and SARS-CoV-2 (Broughton et al., 2020).
The virulence factors play roles through cooperation or alone in the establishment of A. hydrophila infection (Nawaz et al., 2010; Li et al., 2011; Nagar et al., 2011; Igbinosa and Okoh, 2013), and the detection methods only targeting one virulence gene of pathogenic A. hydrophila always lead to false negative results (Elsheshtawy et al., 2019). Here, a dRAA-CRISPR/Cas12a method targeting aerA and hlyA genes for the detection of pathogenic A. hydrophila without elaborate instruments was developed by integrating dualplex RAA (dRAA) and CRISPR/Cas12a system (Figure 1), and was compared with the dPCR-CRISPR/Cas12a method to investigate their sensitivity in detecting A. hydrophila stains and practicability in spiked samples. The whole process only takes 45 min, and the sensitivity is as low as 2 copies per reaction. The dRAA-CRISPR/Cas12a method we developed may be a promising method for rapid, accurate, and sensitive detection of pathogenic A. hydrophila in samples from human, fish, and food.
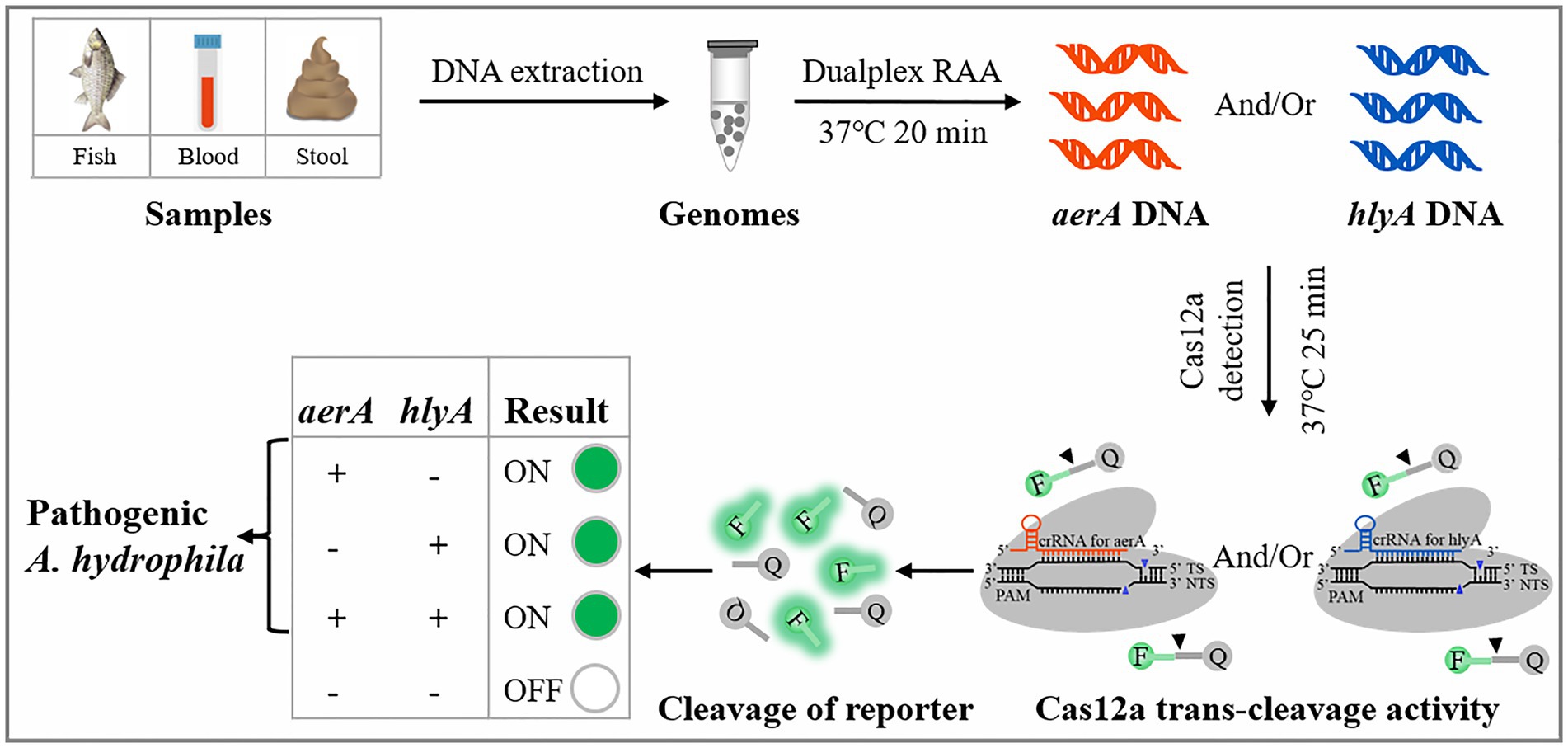
Figure 1. Schematic illustration of the developed dRAA-CRISPR/Cas12a method in the detection of pathogenic Aeromonas hydrophila.
Materials and methods
Bacterial strains
Bacterial strains preserved in our laboratory were used in this study and consisted of eight standard strains and eight isolation strains. The standard strains were A. hydrophila (ATCC 7966), Vibrio vulnificus (ATCC 27562), Vibrio alginolyticus (ATCC 17749), Vibrio harvey (ATCC 14126), Staphylococcus aureus (ATCC 25923), Escherichia coli (ATCC 25922), Pseudomonas aeruginosa (ATCC 27853) and Bacillus cereus (ATCC 14579). The isolation strains were A. hydrophila strain 1, A. hydrophila strain 2, A. hydrophila strain 3, A. veronii, A. sobria, Vibrio parahaemolyticus, Edwardsiella piscicida, and Salmonella typhimurium, which were isolated from affected aquatic animal, patients, and environment. The aerA and hlyA genes expressed in these four A. hydrophila strains were detected by dualplex RAA assay, and the results showed that A. hydrophila strain 1 (NQ201810) is aerA−hlyA−, A. hydrophila strain 2 (NQ201906) is aerA+hlyA−, A. hydrophila strain 3 (AS1.1801) is aerA−hlyA+, and A. hydrophila strain 4 (ATCC 7966) is aerA+hlyA+ (Supplementary Figure S1). Moreover, the expression of aerA and hlyA genes in A. veronii and A. sobria was also detected by dualplex RAA assay, and the results showed that these two species of Aeromonas are aerA+hlyA− (Supplementary Figure S2).
Genomic DNA extraction
Bacterial strains were inoculated into 2216E broth or LB medium and cultured with shaking at 28°C or 37°C for 12–16 h, and then the genomic DNA of them were extracted by Kit-based and NaOH-based method. The Kit-based method for the extraction of genomic DNA was conducted with a MiniBEST Bacteria Genomic DNA Extraction Kit Ver.3.0 (9,763; TaKaRa Bio Inc., Japan) according to the Kit manual. The NaOH-based method was conducted to extract the genomic DNA of A. hydrophila according to the method mentioned in two published papers (Zhang et al., 2020; Xiao et al., 2021). The genomic DNA was stored at −20°C and then used as the templates of RAA and PCR assays.
Nucleic acid preparation
The primer sets for aerA (F: 5′-AAGACGGCCATCAAGGTCAG-3′; R: 5′- ACGAAGGTGTGGTTCCAGT-3′) and hlyA (F: 5′-CCGGCATCTCTTTTGATGCG-3′; R: 5′-GGATGTTGACCGAGGAGTCG-3′) were used to amplify the aerA gene and hlyA gene of A. hydrophila by PCR assay respectively, and then the PCR products were sequenced by GENEWIZ (GENEWIZ, China).
Several sequences of Aeromonas aerA and hlyA genes obtained from GenBank were aligned using the online tool, Clustal Omega. The aligned results and the conserved regions of A. hydrophila aerA and hlyA genes were shown in Supplementary Figures S4, S5, respectively. The regions of aerA and hlyA sequences that were highly conserved among A. hydrophila were selected as templates for the design of RAA primers, which was conducted with NCBI Primer-BLAST. Five primer sets for aerA gene and four primer sets for hlyA gene were designed according to the design instruction of RPA primer1, and the sequences of them were listed in Supplementary Table S1.
According to the complementary pairing characteristics of crRNA with target sequence (Chen et al., 2018), PAM sequences (TTTN) were first found from RAA amplicon of aerA or hlyA, and then 15–25 bp was selected from the adjacent sequence of PAM as the target sequence. The specific sequences that can distinguish A. hydrophila from other pathogens were selected from the candidate target sequences (Supplementary Figures S6, S7), and the crRNA sequence was comprised of the repeat sequence (UAA UUU CUA CUA AGU GUA GAU) and the selected specific sequence. The ssDNA-FQ reporter (5′−/6-FAM/TTATT/BHQ1/−3′) was used to indicate the existence of the target gene. The crRNA and ssDNA-FQ were purchased from GENEWIZ (GENEWIZ, China).
The genomic DNA of three pathogenic A. hydrophila strains (aerA+hlyA−, aerA−hlyA+, and aerA+hlyA+) extracted by Kit-based method were gradiently diluted with 1 × NEB buffer 2.1, and the obtained diluents of A. hydrophila genomic DNA with different concentrations (1 × 100 to 1 × 107 copies/μl) were stored at −80°C.
Single and dualplex RAA assay
The RAA Nucleic Acid Amplification Kit (B00000; Jiangsu Qitian Gene Biological Co., China) was employed to conduct single and dualplex RAA assay. Single RAA assay was carried out according to the Kit manual. Briefly, 25 μl of buffer V, 16.5 μl of purified water, 2 μl of primer F (10 μm), 2 μl of primer R (10 μm), 2.5 μl of magnesium acetate, and 2 μl of genomic DNA were added to the reaction tube. After being softly vortexed for 8 s, the reaction tube was incubated for 20 min at a 37°C water bath. The procedure for dRAA assay was similar to single RAA assay, and the only difference was that the addition volume of each primer (20 μm) in dRAA assay was 1 μl.
Dualplex RAA-CRISPR/Cas12a assay
The product of dRAA assay was used as the target of CRISPR-Cas12a system. The Cas12a trans-cleavage reaction was performed as follows: 5 μl of 1,000 nm Cas12a (M0653T; New England Biolabs Inc., MA, United States) and 5 μl of 400 nm crRNAmix (crRNA mixture), consisting of crRNA for aerA (ACR) and crRNA for hlyA (HCR), were preincubated at 37°C for 20 min to form Cas12a-crRNA complex. 10 μl of 1,000 nm ssDNA-FQ, 10 μl of 1 × NEB buffer 2.1, and 2 μl of dRAA product were added to the tube containing 10 μl of Cas12a-crRNA complex. After softly vortexed for 8 s, the tube containing 32 μl of mixture was incubated at 37°C for 35 min. The results can be read with an UV flashlight or a multifunctional microplate reader (λex: 485 nm and λem: 520 nm). In this study, we optimized the concentration of Cas12a, the concentration of ssDNA-FQ, and the Cas12a cleavage time.
Dualplex PCR-CRISPR/Cas12a assay
The procedure for dPCR-CRISPR/Cas12a assay was similar to the dRAA-CRISPR/Cas12a assay. Briefly, dualplex PCR assay was carried out using Phanta® Max Super-Fidelity DNA Polymerase (P505-d1; Vazyme Biotechnology Co. LTD., China) in a 50 μl reaction mixture, containing 25 μl of 2 × Phanta Max Buffer, 1 μl of 10 μm forward and reverse primers (total of 4 μl; Supplementary Table S1), 1 μl of dNTP Mix (10 mm each), 1 U Phanta Max Super-Fidelity DNA Polymerase, 2 μl of genomic DNA, and 17 μl of H2O. 2 μl of dPCR product was then added to the reaction mixture containing the ssDNA-FQ and Cas12a-crRNA complex.
Spiked sample testing
This experiment was conducted by two operators. Firstly, 15 healthy crucian carps were purchased from supermarket. One operator took out the livers of crucian carp, cut them into small pieces, and then added 20 mg of tissue to the 15 tubes containing 200 μl of 0.5 M NaOH solution at clean workbench. Then, 15 tubes were numbered, and 1 × 103 CFU of A. hydrophila were added to some tubes. These tissue samples were grinded with a Disposable Tissue Grinding Pestle (Sangon, China) for 3 min, and upon 20-fold dilution with H2O, 2 μl of lysate was used as the DNA template for the dRAA assay. Above was the NaOH-based method for the extraction of A. hydrophila genomic DNA from spiked fish (Zhang et al., 2020; Xiao et al., 2021; Zhao et al., 2022), and meanwhile, the Kit-based extraction method was also conducted as a comparative test. The other operator who did not know the number of spiked samples performed the dRAA-CRISPR/Cas12a assay.
Application of dRAA-CRISPR/Cas12a assay for human blood and stool specimens
Human blood and stool samples were provided by three healthy volunteers. The spiked samples were prepared by adding 100 μl of human blood or 200 mg of stool into the tubes containing 1 × 103 CFU of A. hydrophila (aerA+hlyA+). The MiniBEST Universal Genomic DNA Extraction Kit (9,765; TaKaRa Bio Inc., Japan) and the TIANamp Stool DNA Kit (DP328; TIANGEN BIOTECH CO. LTD, China) were employed to extract genomic DNA from these spiked samples and samples without A. hydrophila, which acted as negative controls. The genomic DNA of these samples were then detected by dRAA-CRISPR/Cas12a assay.
Statistical analysis
Statistical analysis was performed using SPSS 13.0 software (SPSS Inc., Chicago, IL, USA). The data were analyzed by Student’s t test. p < 0.05 (indicated by *) was considered statistically significant.
Results
Screening the RAA primer pairs targeting aerA gene and hlyA gene
High amplification efficiency of the amplification assay can increase the detection sensitivity of the method (Clementi et al., 1993; Pfaffl, 2004). To obtain the primer pair with high amplification efficiency, we designed five primer pairs and four primer pairs targeting aerA gene and hlyA gene, respectively, and then screened an optimal primer pair according to the relative intensity of RAA product band for each primer pair. The results showed that all the predicted product bands were clearly distinguishable (Figure 2A). As for the aerA gene, the band intensity of the No.5 lane obtained with the primer set AF5 (5′-GCCATCAAGGTCAGCAATTTTGCGTACAAC-3′)/AR5 (5′- CACTTGAACTTGTTCTTGGTGGTCACCTTCTC-3′) was the strongest among the five bands, while as for the hlyA gene, it was the No.8 lane obtained with the primer set HF3 (5′- CACGTGGCCTTCTACCTCAACGTCAACC-3′)/HR3 (5′- CCTTGGTGTTGGACGCCTCGATGCTGAA-3′). Furthermore, these two primer sets were used to amplify aerA gene and hlyA gene through dRAA assay, and the result showed that two predicted bands with satisfactory intensity were present at the No.10 lane. Therefore, the RAA primer sets, AF5/AR5 and HF3/HR3, were chosen as the optimal primers for the dRAA reaction, and the amplicon of AF5/AR5 and HF3/HR3 was used to design crRNA for aerA and hlyA, respectively.
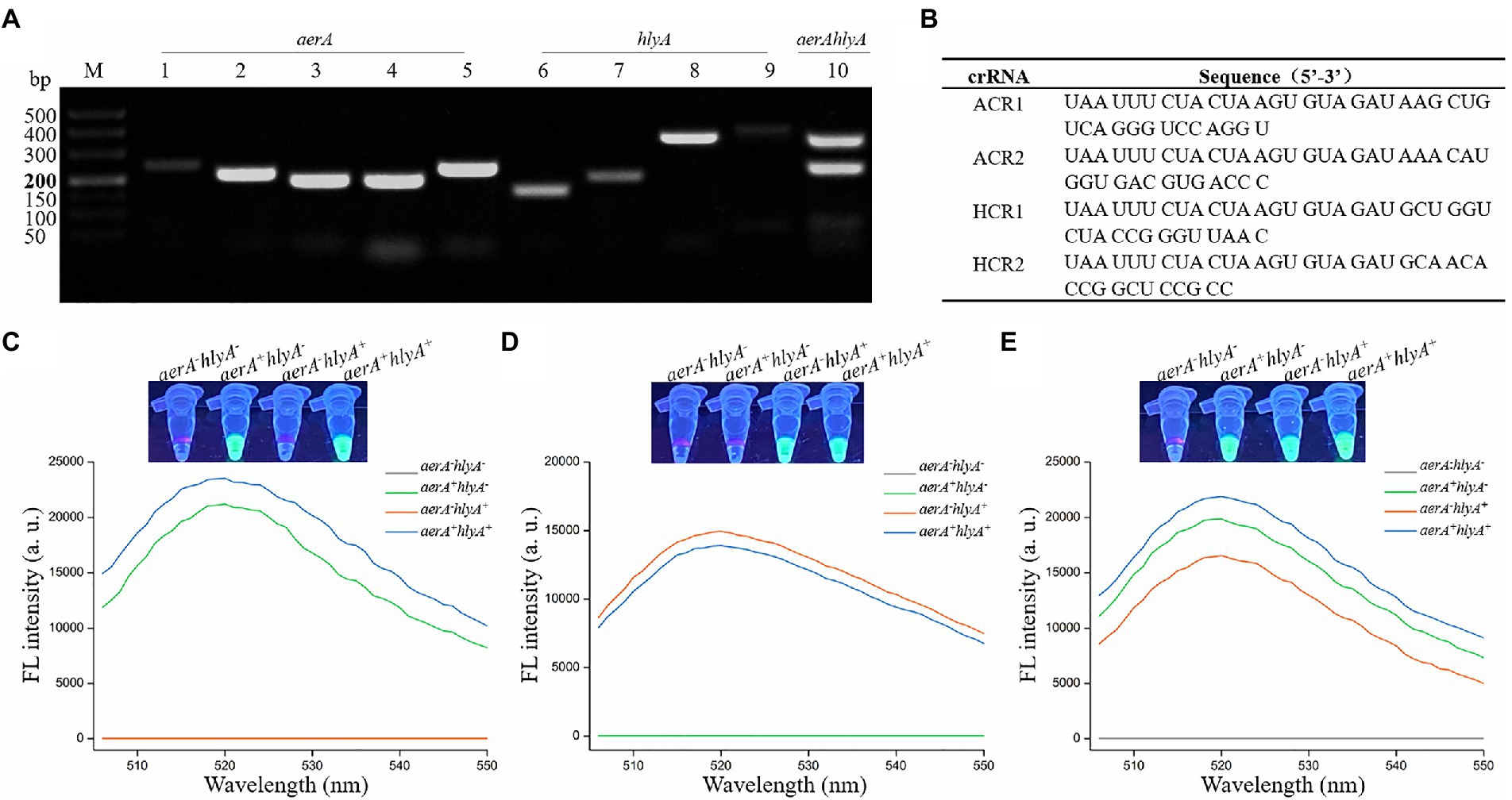
Figure 2. Feasibility verification of the dRAA-CRISPR/Cas12a assay in the detection of Aeromonas hydrophila. (A) Screening the RAA primer pairs. The single and dualplex RAA assays were performed using the genomic DNA extracted from aerA+hlyA+ A. hydrophila strain as the template. Lanes 1–5, screening the primer pair targeting for aerA gene. Lanes 6–9, screening the primer pair targeting for hlyA gene. Lane 10, feasibility of dRAA assay with the optimal aerA and hlyA primer pairs. (B) Sequences of crRNA used in this study. (C–E) Feasibility verification of the dRAA-CRISPR/Cas12a assay using the ACR1 and ACR2 (C), HCR1 and HCR2 (D), and ACR1, ACR2, HCR1 and HCR2 (E) in the detection of four A. hydrophila strains. Fluorescence signals were read using an UV flashlight (upper) or a multifunctional microplate reader (below).
Feasibility verification of the dRAA-CRISPR/Cas12a assay in the detection of Aeromonas hydrophila
According to the principle of crRNA design mentioned in Materials and Methods, ACR1 and ACR2, targeting aerA gene, and HCR1 and HCR2, targeting hlyA gene, were designed, and the sequences of these four crRNAs were listed in Figure 2B. To investigate the validity of the designed crRNA and verify the feasibility of the dRAA-CRISPR/Cas12a method, the dRAA-CRISPR/Cas12a assays for the detection of four A. hydrophila strains (aerA−hlyA−, aerA+hlyA−, aerA−hlyA+ and aerA+hlyA+) were performed using the ACR1 and ACR2 (Figure 2C), HCR1 and HCR2 (Figure 2D), and ACR1, ACR2, HCR1 and HCR2 (Figure 2E), respectively. As shown in Figure 2C, only the genomic DNA samples extracted from aerA+hlyA− and aerA+hlyA+ A. hydrophila strains could generate fluorescence signals, indicating that ACR1 and ACR2 were valid and specific crRNAs in the detection of A. hydrophila expressing aerA gene. Our results also indicated that HCR1 and HCR2 were valid and specific crRNAs in the detection of A. hydrophila expressing hlyA gene (Figure 2D). Furthermore, as shown in Figure 2E, only the genomic DNA samples extracted from A. hydrophila expressing aerA and/or hlyA genes could generate fluorescence signals, indicating that our developed method, dRAA-CRISPR/Cas12a, using ACR1, ACR2, HCR1 and HCR2 as the crRNAmix could be used to detect the pathogenic A. hydrophila expressing aerA and/or hlyA genes.
Optimization of the conditions of dRAA-CRISPR/Cas12a assay
The concentrations of Cas12a and ssDNA-FQ and the Cas12a cleavage time are related to cleavage efficiency, signal output, and reaction speed of the CRISPR-based detection method (Broughton et al., 2020; Sun et al., 2020; Fu et al., 2022). To achieve an ideal reaction performance, dRAA-CRISPR/Cas12a assay was conducted to optimize the concentration of Cas12a, the concentration of ssDNA-FQ and the Cas12a cleavage time with the aerA+hlyA+ A. hydrophila genomic DNA (1 × 104 copies/μL) and H2O as templates. The ratio of the fluorescence intensity triggered by A. hydrophila (F) to the fluorescence intensity triggered by H2O (F0) was employed to assess the reaction concentrations of Cas12a and ssDNA-FQ. As shown in Figure 3A, both the F and F0 increased with the increasing of the Cas12a concentrations, and when the Cas12a concentration was 600 nm, the ratio of F/F0 reached maximum. As shown in Figure 3B, the results indicated that the optimal concentration of ssDNA-FQ was 500 nm. The cleavage time of Cas12a was further explored using the optimized concentrations of Cas12a (600 nm) and ssDNA-FQ (500 nm). The results showed that the increasing of F reached a plateau after 25 min, which indicated that the optimal cleavage time of Cas12a was 25 min. Therefore, the reaction conditions of our developed method, dRAA-CRISPR/Cas12a, were as followed: 20 min of RAA reaction, 5 μl of 600 nm Cas12a, 5 μl of 400 nm crRNAmix, 10 μl of 500 nm ssDNA-FQ, and 25 min of Cas12a cleavage, and in the subsequent experiments, dRAA-CRISPR/Cas12a assays were conducted according to the above conditions.
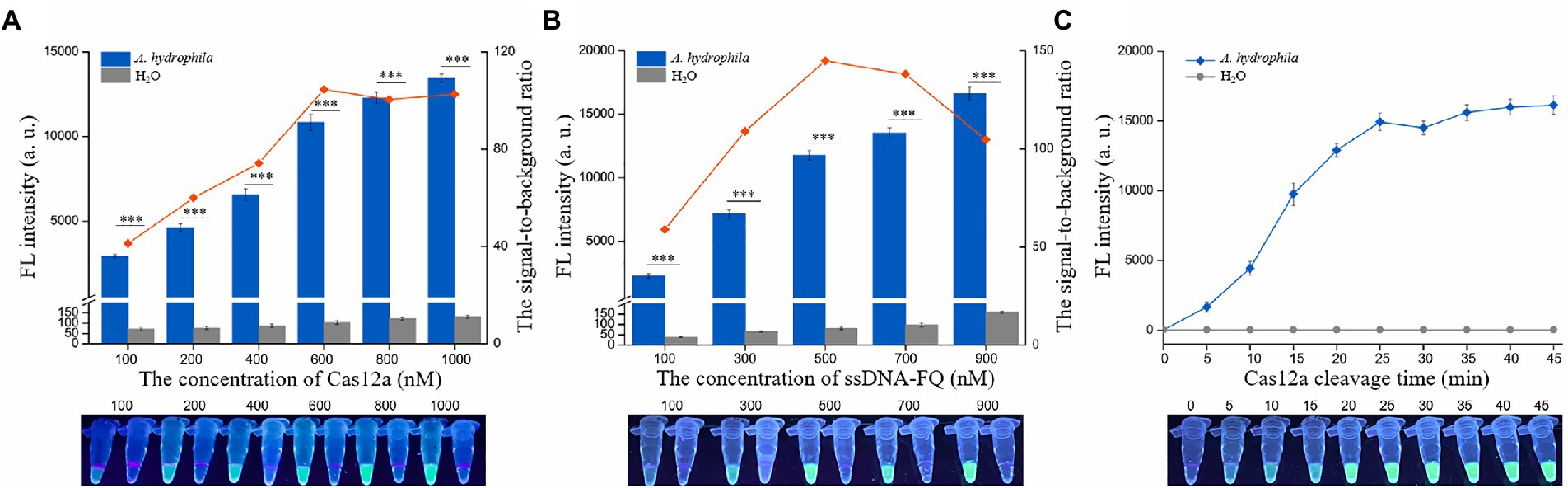
Figure 3. Optimization of the conditions of dRAA-CRISPR/Cas12a assay. The dRAA-CRISPR/Cas12a assays were conducted using AF5/AR5 and HF3/HR3 as RAA primer sets, aerA+hlyA+ A. hydrophila genomic DNA (1 × 104 copies/μl) or H2O as a RAA template, and ACR1, ACR2, HCR1 and HCR2 as crRNAmix to optimize the concentration of Cas12a (A) and ssDNA-FQ (B) and the cleavage time of Cas12a (C). The results were read using an UV flashlight (below) or a multifunctional microplate reader (upper). (A,B) n = 3 technical replicates; two-tailed Student’s t test; ***p < 0.001; bars represent mean ± SEM.
Sensitivity of dRAA-CRISPR/Cas12a assay for detecting Aeromonas hydrophila
To investigate the detection sensitivity of dRAA-CRISPR/Cas12a assay for pathogenic A. hydrophila, 2 μl of the genomic DNA with different concentrations (1 × 100–1 × 106 copies /μL) extracted from three A. hydrophila strains (aerA+hlyA−, aerA−hlyA+ and aerA+hlyA+) were used as templates to perform the dRAA assay, and then 2 μl of dRAA product was subjected to Cas12a cleavage test. The dPCR-CRISPR/Cas12a assay was conducted as a comparative test. The results of dRAA-CRISPR/Cas12a assays showed that fluorescence signals were detected in all samples except negative control (H2O; Figure 4A), and the limit of detection (LOD) of dRAA-CRISPR/Cas12a assay for each A. hydrophila strain reached 2 copies per reaction, which was in line with the LOD of dPCR-CRISPR/Cas12a assay (Figure 4B). Therefore, our developed detection method, dRAA-CRISPR/Cas12a, showed high sensitivity (2 copies/reaction) for pathogenic A. hydrophila expressing aerA and/or hlyA genes.
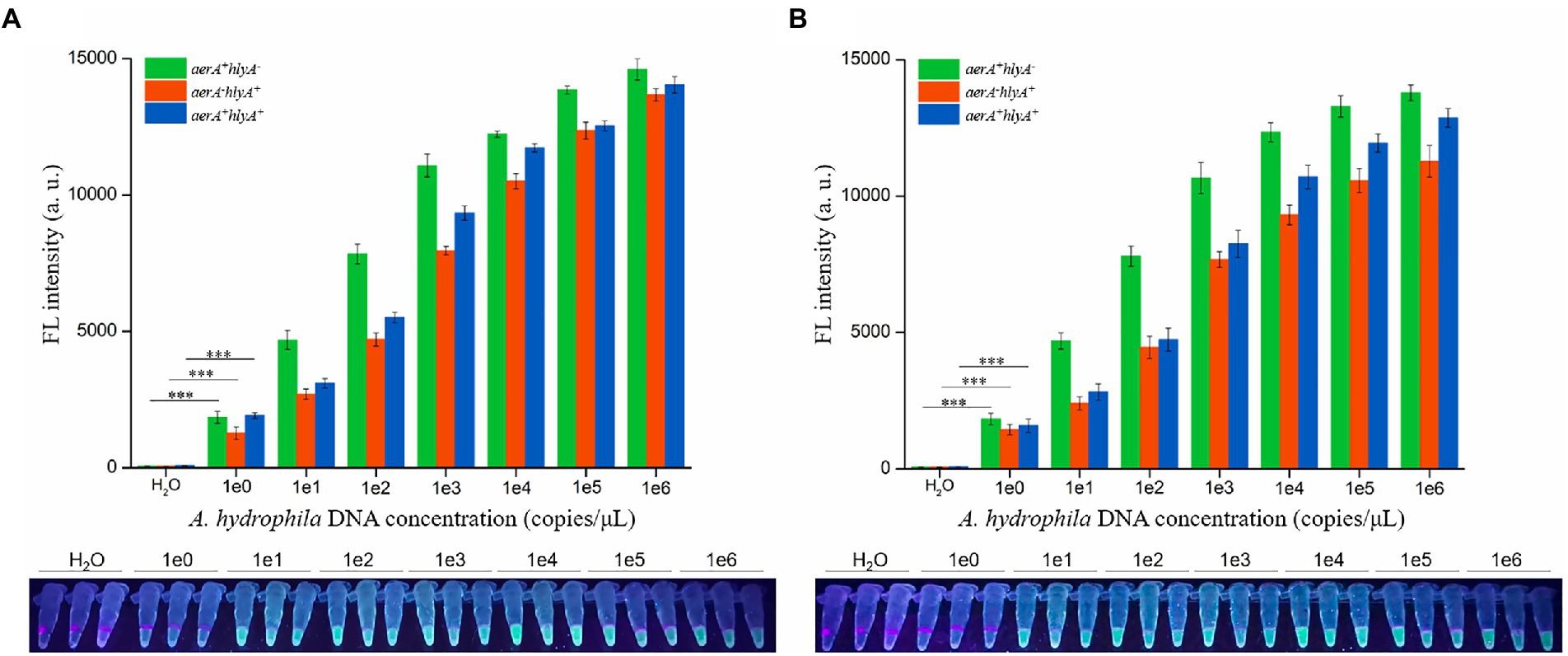
Figure 4. Sensitivity evaluation. Sensitivity of dRAA-CRISPR/Cas12a (A) and dPCR-CRISPR/Cas12a (B) assays were evaluated in the detection of pathogenic Aeromonas hydrophila. The different concentrations (1 × 100–1 × 106 copies/μl) of A. hydrophila genomic DNA were used as the detection template, and H2O was used as a negative control. The results were read using an UV flashlight (below) or a multifunctional microplate reader (upper). n = 3 technical replicates; two-tailed Student’s t test; ***p < 0.001, experimental group versus corresponding negative control group (only shown the 1e0 vs. H2O); bars represent mean ± SEM.
Specificity of dRAA-CRISPR/Cas12a assay for detecting Aeromonas hydrophila
To evaluate the specificity of dRAA-CRISPR/Cas12a assay in A. hydrophila detection, 16 genomic DNA samples extracted from four A. hydrophila strains and 12 other strains of waterborne and/or foodborne pathogenic bacteria were used as dRAA templates, and H2O was used as negative control. As shown in Figure 5, one A. hydrophila strain (aerA−hlyA−) and 12 other bacterial strains did not generate the fluorescence signal, while it was only detected in three A. hydrophila strains expressing aerA and/or hlyA genes, indicating no cross-reactions with non-pathogenic A. hydrophila and non-Aeromonas hydrophila bacteria of dRAA-CRISPR/Cas12a assay in pathogenic A. hydrophila detection. Therefore, our developed detection method, dRAA-CRISPR/Cas12a, showed high specificity for pathogenic A. hydrophila expressing aerA and/or hlyA genes.
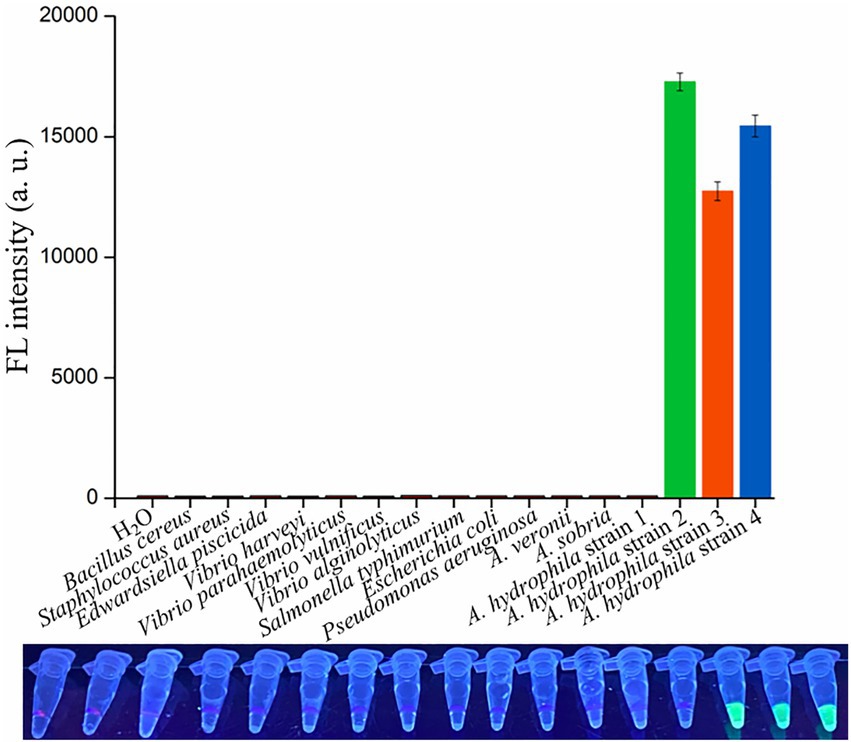
Figure 5. Specificity and practicability evaluation of dRAA-CRISPR/Cas12a assay. Specificity evaluation. 16 bacterial strains were employed to evaluate the specificity of dRAA-CRISPR/Cas12a assay in the detection of A. hydrophila. A. hydrophila strain 1, 2, 3, and 4 were aerA−hlyA−, aerA+hlyA−, aerA−hlyA+, and aerA+hlyA+, respectively. H2O was used as a negative control. n = 3 technical replicates; bars represent mean ± SEM.
Practicability evaluation of the dRAA-CRISPR/Cas12a assay in the detection of spiked samples
Finally, to evaluate the practicability of the dRAA-CRISPR/Cas12a assay, fish and human samples were employed to conduct this experiment by two operators. For fish samples, livers were taken out from 15 healthy crucian carps, nine liver samples (20 mg/sample) were spiked with 1 × 103 CFU of A. hydrophila, and then the genomic DNA was extracted using the NaOH-based method from these 15 liver samples by one operator, while the other one did the dRAA-CRISPR/Cas12a and dPCR-CRISPR/Cas12a assays with these 15 liver samples. The results of dRAA-CRISPR/Cas12a assays showed that only the spiked samples could generate the fluorescence signal (Figure 6A). This result was consistent with the result obtained from dPCR-CRISPR/Cas12a assays (Figures 6B,C). In addition, the genomic DNA extracted from the other 15 liver samples using Kit-based method were detected by dRAA-CRISPR/Cas12a assay, and the same result with Figure 6A was obtained (Supplementary Figure S3). Therefore, these results indicated that our developed method, dRAA-CRISPR/Cas12a, could rapidly and accurately detect A. hydrophila from fish samples.
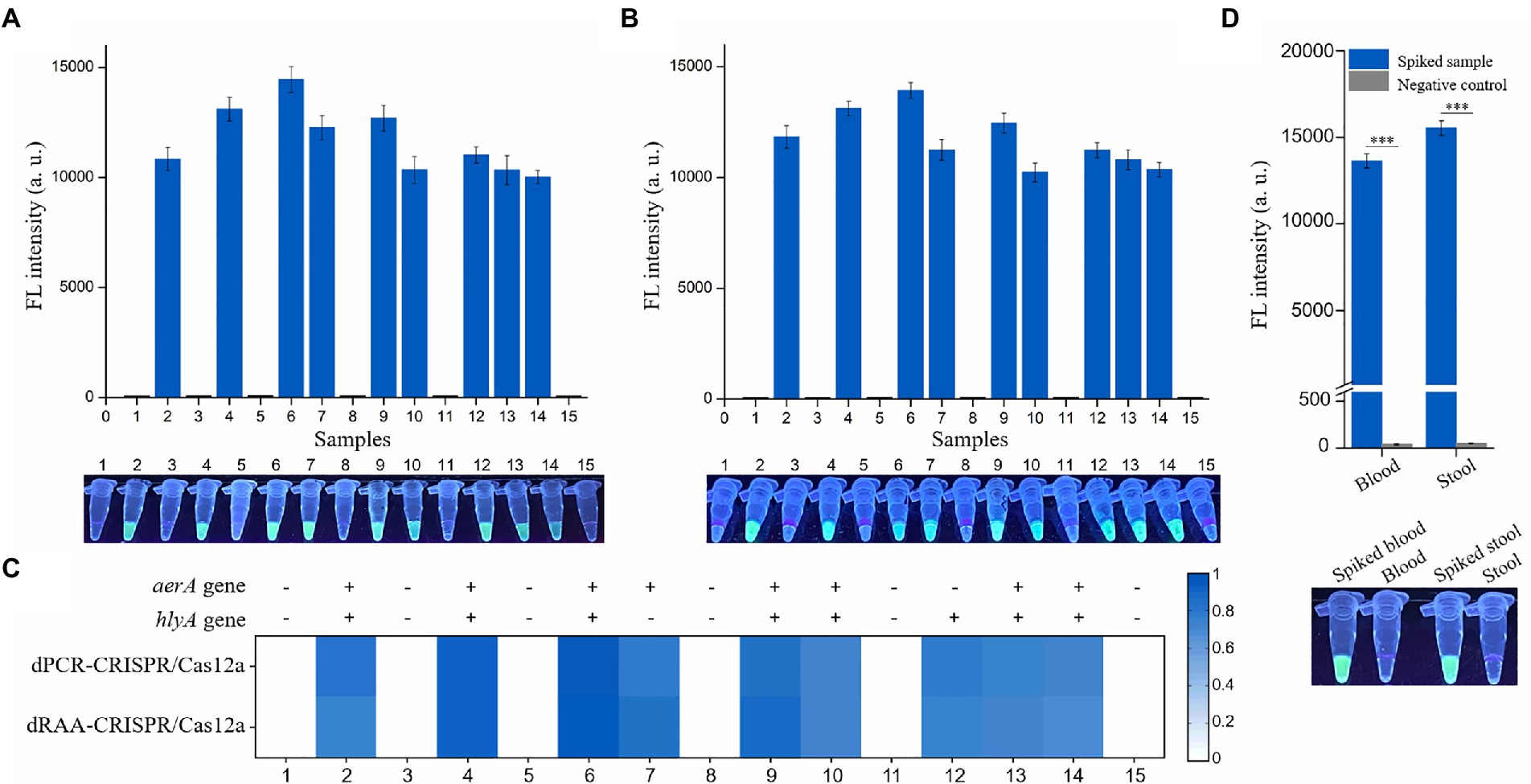
Figure 6. Practicability evaluation of the dRAA-CRISPR/Cas12a and dPCR-CRISPR/Cas12a assays in the detection of spiked samples. Six normal liver samples and nine liver samples spiked with 1 × 103 CFU of A. hydrophila were used to extract genomic DNA according to the NaOH-based method. These genomic DNA samples were detected using dRAA-CRISPR/Cas12a (A) and dPCR-CRISPR/Cas12a (B) assays. n = 3 technical replicates; bars represent mean ± SEM. (C) Heatmap of the results of the two assays for the detection of A. hydrophila from 15 crucian carps. (D) Human blood and stool samples were spiked with 1 × 103 CFU of A. hydrophila (aerA+hlyA+). Normal blood and stool samples were served as negative controls. The fluorescence signals were read using an UV flashlight (below) or a multifunctional microplate reader (upper). n = 3 technical replicates; two-tailed Student’s t test; ***p < 0.001; bars represent mean ± SEM.
For human samples, blood and stool samples were collected from three healthy volunteers, and some blood and stool were spiked with 1 × 103 CFU of A. hydrophila (aerA+hlyA+). As shown in Figure 6D, only the spiked samples could generate the fluorescence signal. These results indicated that our developed method, dRAA-CRISPR/Cas12a, could be used to detect clinical sepsis and gastroenteritis caused by A. hydrophila.
Discussion
Aeromonas hydrophila is an emerging pathogen with pathogenicity to humans and fishes (Tichoniuk et al., 2010; Li et al., 2011). Increasingly, A. hydrophila is posing a serious threat to human health, aquaculture and food safety, and foodborne outbreaks of its infection have occurred in many countries (Morena et al., 1993; Krovacek et al., 1995; Zhang et al., 2012; Tsheten et al., 2016). Many studies have reported that the pathogenicity of A. hydrophila is determined by multiple virulence factors (Janda and Abbott, 2010; Tichoniuk et al., 2010; Li et al., 2011), and based on this reason, there are many methods targeting one or more virulence genes to detect pathogenic A. hydrophila (Tichoniuk et al., 2010; Uma et al., 2010; Li et al., 2011; Elsheshtawy et al., 2019; Qu et al., 2021). However, these methods have some flaws, such as long operation time, low sensitivity, complicated operation, and missed detection. In this study, to avoid these flaws, we developed a novel method, dRAA-CRISPR/Cas12a, for the detection of pathogenic A. hydrophila targeting aerA and hlyA genes based on dRAA assay and CRISPR/Cas12a system (Figure 1). This method performed according to our optimized conditions only takes 45 min to obtain the accurate result without an elaborate instrument (Figure 3), and the LOD of it is as low as 2 copies of A. hydrophila genomic DNA per reaction (Figure 4A).
The target genes, aerA and hlyA, encoding aerolysin and hemolysin respectively, of our proposed method are two important virulent genes of A. hydrophila (Wong et al., 1998; Biscardi et al., 2002; Ørmen et al., 2003; Janda and Abbott, 2010). Aerolysin and hemolysin are most common in clinical and environmental strains of A. hydrophila (Wong et al., 1998; González-Rodríguez et al., 2002; Janda and Abbott, 2010) and play key roles through cooperation or alone in the pathogenicity of it (Biscardi et al., 2002; Singh et al., 2008; Nawaz et al., 2010; Igbinosa and Okoh, 2013). Moreover, the study reported by Heuzenroeder et al. indicated that a method targeting aerA and hlyA genes was a trustworthy approach to detect pathogenic A. hydrophila (Heuzenroeder et al., 1999). Therefore, aerA and hlyA genes were selected for the detection of pathogenic A. hydrophila in this study. Because aerA and hlyA genes are also present in some other species of Aeromonas, the published aerA and hlyA sequences were downloaded from GenBank and aligned to seek the sequences which are highly conserved in A. hydrophila but not in other Aeromonas species (Supplementary Figures S4–S7), and based on these sequences, we successfully designed two crRNAs for aerA and two crRNAs for hlyA (Figures 2B–E). Furthermore, the results of specificity experiment indicated that only three A. hydrophila strains expressing aerA gene and/or hlyA gene could be detected by our developed method, dRAA-CRISPR/Cas12a (Figure 5), which further confirmed the validity of our designed crRNA and RAA primer sets.
In addition, we investigated the sensitivity of dRAA-CRISPR/Cas12a in the detection of three pathogenic A. hydrophila strains (aerA+hlyA−, aerA−hlyA+ and aerA+hlyA+), and found that the LOD for each strain reached 2 copies of genomic DNA per reaction (Figure 4A), which was consistent with the results of dPCR-CRISPR/Cas12a (Figure 4B). However, because the samples used to investigate the sensitivity of dRAA-CRISPR/Cas12a were the pure bacteria, the result of this experiment may not reflect the real sensitivity of dRAA-CRISPR/Cas12a in detecting the clinical and environmental samples with a large number of impurities that may influence the amplification efficiency of RAA assay. Finally, we evaluated the practicability of the dRAA-CRISPR/Cas12a assay in the detection of spiked fish samples. Our proposed method dRAA-CRISPR/Cas12a could only detect the presence of A. hydrophila from all the spiked liver samples (Figure 6A), and this result completely matched the results got from dPCR-CRISPR/Cas12a assay (Figures 6B,C), indicating that dRAA-CRISPR/Cas12a method is a promising candidate for on-site A. hydrophila detection in fish samples. Because A. hydrophila infection mainly causes sepsis and gastroenteritis in humans (González-Serrano et al., 2002; Zhu et al., 2021), we also performed dRAA-CRISPR/Cas12a method to detect the spiked blood and stool samples, and found that the fluorescence signal could only be detected in spiked blood and stool samples (Figure 6D), indicating that the dRAA-CRISPR/Cas12a method can also be used to diagnose patients infected by A. hydrophila.
In conclusion, the dRAA-CRISPR/Cas12a method we developed can rapidly, accurately, and sensitively detect pathogenic A. hydrophila expressing aerA gene and/or hlyA gene, and the results can be read using an UV flashlight. It is very beneficial for early diagnosis and on-site detection of A. hydrophila infection especially in resource-poor areas. Apart from dRAA-CRISPR/Cas12a method, dPCR-CRISPR/Cas12a used in this study can also be a candidate to detect A. hydrophila in Lab.
Data availability statement
The original contributions presented in the study are included in the article/Supplementary material, further inquiries can be directed to the corresponding authors.
Author contributions
XX and YL conceived and designed the study. XX and ZL wrote the manuscript. ZL, JL, and SW performed the experiments and analyzed the data. XL and LZ reviewed the manuscript. All authors read and approved the submitted version.
Funding
This study was supported by the National Natural Science Foundation of China (grant no. 82002117), the Science and Technology Bureau of Wenzhou (grant no. Y20210109), and the Key Discipline of Zhejiang Province in Medical Technology (First Class, Category A).
Conflict of interest
The authors declare that the research was conducted in the absence of any commercial or financial relationships that could be construed as a potential conflict of interest.
Publisher’s note
All claims expressed in this article are solely those of the authors and do not necessarily represent those of their affiliated organizations, or those of the publisher, the editors and the reviewers. Any product that may be evaluated in this article, or claim that may be made by its manufacturer, is not guaranteed or endorsed by the publisher.
Supplementary material
The Supplementary material for this article can be found online at: https://www.frontiersin.org/articles/10.3389/fmicb.2022.973996/full#supplementary-material
Footnotes
References
Abbott, S. L., Cheung, W. K., and Janda, J. M. (2003). The genus Aeromonas: biochemical characteristics, atypical reactions, and phenotypic identification schemes. J. Clin. Microbiol. 41, 2348–2357. doi: 10.1128/JCM.41.6.2348-2357.2003
Balakrishna, K., Murali, H. S., and Batra, H. V. (2010). Detection of toxigenic strains of Aeromonas species in foods by a multiplex PCR assay. Indian J. Microbiol. 50, 139–144. doi: 10.1007/s12088-010-0038-5
Balsalobre, L. C., Dropa, M., Matte, G. R., and Matte, M. H. (2009). Molecular detection of enterotoxins in environmental strains of Aeromonas hydrophila and Aeromonas jandaei. J. Water Health 7, 685–691. doi: 10.2166/wh.2009.082
Biscardi, D., Castaldo, A., Gualillo, O., and De Fusco, R. (2002). The occurrence of cytotoxic Aeromonas hydrophila strains in Italian mineral and thermal waters. Sci. Total Environ. 292, 255–263. doi: 10.1016/s0048-9697(01)01132-9
Broughton, J. P., Deng, X., Yu, G., Fasching, C. L., Servellita, V., Singh, J., et al. (2020). CRISPR-Cas12-based detection of SARS-CoV-2. Nat. Biotechnol. 38, 870–874. doi: 10.1038/s41587-020-0513-4
Chauret, C., Volk, C., Creason, R., Jarosh, J., Robinson, J., and Warnes, C. (2001). Detection of Aeromonas hydrophila in a drinking-water distribution system: a field and pilot study. Can. J. Microbiol. 47, 782–786. doi: 10.1139/w01-070
Chen, J. S., Ma, E., Harrington, L. B., Da Costa, M., Tian, X., Palefsky, J. M., et al. (2018). CRISPR-Cas12a target binding unleashes indiscriminate single-stranded DNase activity. Science 360, 436–439. doi: 10.1126/science.aar6245
Clementi, M., Menzo, S., Bagnarelli, P., Manzin, A., Valenza, A., and Varaldo, P. E. (1993). Quantitative PCR and RT-PCR in virology. PCR Methods Appl. 2, 191–196. doi: 10.1101/gr.2.3.191
Daskalov, H. (2006). The importance of Aeromonas hydrophila in food safety. Food Control 17, 474–483. doi: 10.1016/j.foodcont.2005.02.009
Elsheshtawy, A., Yehia, N., Elkemary, M., and Soliman, H. (2019). Direct detection of unamplified Aeromonas hydrophila DNA in clinical fish samples using gold nanoparticle probe-based assay. Aquaculture 500, 451–457. doi: 10.1016/j.aquaculture.2018.10.046
Fu, X., Sun, J., Ye, Y., Zhang, Y., and Sun, X. (2022). A rapid and ultrasensitive dual detection platform based on Cas12a for simultaneous detection of virulence and resistance genes of drug-resistant salmonella. Biosens. Bioelectron. 195:113682. doi: 10.1016/j.bios.2021.113682
González-Rodríguez, M., Santos, J., Otero, A., and García-López, M. (2002). PCR detection of potentially pathogenic aeromonads in raw and cold-smoked freshwater fish. J. Appl. Microbiol. 93, 675–680. doi: 10.1046/j.1365-2672.2002.01739.x
González-Serrano, C., Santos, J., García-López, M., and Otero, A. (2002). Virulence markers in Aeromonas hydrophila and Aeromonas veronii biovar sobria isolates from freshwater fish and from a diarrhoea case. J. Appl. Microbiol. 93, 414–419. doi: 10.1046/j.1365-2672.2002.01705.x
Heuzenroeder, M. W., Wong, C. Y., and Flower, R. L. (1999). Distribution of two hemolytic toxin genes in clinical and environmental isolates of Aeromonas spp.: correlation with virulence in a suckling mouse model. FEMS Microbiol. Lett. 174, 131–136. doi: 10.1111/j.1574-6968.1999.tb13559.x
Hussain, I. A., Jeyasekaran, G., Shakila, R. J., Raj, K. T., and Jeevithan, E. (2014). Detection of hemolytic strains of Aeromonas hydrophila and A. sobria along with other Aeromonas spp. from fish and fishery products by multiplex PCR. J. Food Sci. Technol. 51, 401–407. doi: 10.1007/s13197-013-1190-9
Igbinosa, I. H., and Okoh, A. I. (2013). Detection and distribution of putative virulence associated genes in Aeromonas species from freshwater and wastewater treatment plant. J. Basic Microbiol. 53, 895–901. doi: 10.1002/jobm.201200351
Janda, J. M., and Abbott, S. L. (2010). The genus Aeromonas: taxonomy, pathogenicity, and infection. Clin. Microbiol. Rev. 23, 35–73. doi: 10.1128/CMR.00039-09
Krovacek, K., Dumontet, S., Eriksson, E., and Baloda, S. B. (1995). Isolation, and virulence profiles, of Aeromonas hydrophila implicated in an outbreak of food poisoning in Sweden. Microbiol. Immunol. 39, 655–661. doi: 10.1111/j.1348-0421.1995.tb03253.x
Li, S. Y., Cheng, Q. X., Liu, J. K., Nie, X. Q., Zhao, G. P., and Wang, J. (2018a). CRISPR-Cas12a has both cis- and trans-cleavage activities on single-stranded DNA. Cell Res. 28, 491–493. doi: 10.1038/s41422-018-0022-x
Li, S. Y., Cheng, Q. X., Wang, J. M., Li, X. Y., Zhang, Z. L., Gao, S., et al. (2018b). CRISPR-Cas12a-assisted nucleic acid detection. Cell Discov. 4:20. doi: 10.1038/s41421-018-0028-z
Li, J., Ni, X., Liu, Y., and Lu, C. (2011). Detection of three virulence genes alt, ahp and aerA in Aeromonas hydrophila and their relationship with actual virulence to zebrafish. J. Appl. Microbiol. 110, 823–830. doi: 10.1111/j.1365-2672.2011.04944.x
Li, F., Ye, Q., Chen, M., Zhou, B., Zhang, J., Pang, R., et al. (2021). An ultrasensitive CRISPR/Cas12a based electrochemical biosensor for listeria monocytogenes detection. Biosens. Bioelectron. 179:113073. doi: 10.1016/j.bios.2021.113073
Longyant, S., Chaiyasittrakul, K., Rukpratanporn, S., Chaivisuthangkura, P., and Sithigorngul, P. (2010). Simple and direct detection of Aeromonas hydrophila infection in the goldfish, Carassius auratus (L.), by dot blotting using specific monoclonal antibodies. J. Fish Dis. 33, 973–984. doi: 10.1111/j.1365-2761.2010.01197.x
Meng, S., Wang, Y., Wang, Y., Liu, D., and Ye, C. (2015). Development of cross-priming amplification assays for rapid and sensitive detection of Aeromonas hydrophila. Lett. Appl. Microbiol. 61, 171–178. doi: 10.1111/lam.12439
Morena, M. L., Van, R., Singh, K., Brian, M., Murray, B. E., and Pickering, L. K. (1993). Diarrhea associated with Aeromonas species in children in day care centers. J. Infect. Dis. 168, 215–218. doi: 10.1093/infdis/168.1.215
Nagamine, K., Hase, T., and Notomi, T. (2002). Accelerated reaction by loop-mediated isothermal amplification using loop primers. Mol. Cell. Probes 16, 223–229. doi: 10.1006/mcpr.2002.0415
Nagar, V., Shashidhar, R., and Bandekar, J. R. (2011). Prevalence, characterization, and antimicrobial resistance of Aeromonas strains from various retail food products in Mumbai, India. J. Food Sci. 76, M486–M492. doi: 10.1111/j.1750-3841.2011.02303.x
Nawaz, M., Khan, S. A., Khan, A. A., Sung, K., Tran, Q., Kerdahi, K., et al. (2010). Detection and characterization of virulence genes and integrons in Aeromonas veronii isolated from catfish. Food Microbiol. 27, 327–331. doi: 10.1016/j.fm.2009.11.007
Nielsen, M. E., Høi, L., Schmidt, A., Qian, D., Shimada, T., Shen, J., et al. (2001). Is Aeromonas hydrophila the dominant motile Aeromonas species that causes disease outbreaks in aquaculture production in the Zhejiang Province of China? Dis. Aquat. Org. 46, 23–29. doi: 10.3354/dao046023
Ørmen, Ø., Regue, M. Q., Tomás, J. M., and Granum, P. E. (2003). Studies of aerolysin promoters from different Aeromonas spp. Microb. Pathog. 35, 189–196. doi: 10.1016/s0882-4010(03)00124-4
Özbaş, Z. Y., Lehner, A., and Wagner, M. (2000). Development of a multiplex and semi-nested PCR assay for detection of Yersinia enterocolitica and Aeromonas hydrophila in raw milk. Food Microbiol. 17, 197–203. doi: 10.1006/fmic.1999.0298
Piepenburg, O., Williams, C. H., Stemple, D. L., and Armes, N. A. (2006). DNA detection using recombination proteins. PLoS Biol. 4:e204. doi: 10.1371/journal.pbio.0040204
Qu, Y., Wang, Q., Li, Y., Wang, Y., Yin, J., Ren, Y., et al. (2021). Development of a real-time recombinase polymerase amplification assay for rapid detection of Aeromonas hydrophila. J. Fish Dis. 44, 469–477. doi: 10.1111/jfd.13291
Singh, V., Rathore, G., Kapoor, D., Mishra, B., and Lakra, W. (2008). Detection of aerolysin gene in Aeromonas hydrophila isolated from fish and pond water. Indian J. Microbiol. 48, 453–458. doi: 10.1007/s12088-008-0056-8
Subsoontorn, P., Lohitnavy, M., and Kongkaew, C. (2020). The diagnostic accuracy of isothermal nucleic acid point-of-care tests for human coronaviruses: a systematic review and meta-analysis. Sci. Rep. 10, 22349–22313. doi: 10.1038/s41598-020-79237-7
Sun, H.-H., He, F., Wang, T., Yin, B.-C., and Ye, B.-C. (2020). A Cas12a-mediated cascade amplification method for microRNA detection. Analyst 145, 5547–5552. doi: 10.1039/d0an00370k
Sun, Y., Zhao, Y., Xu, W., Fang, R., Wu, Q., He, H., et al. (2021). Taxonomy, virulence determinants and antimicrobial susceptibility of Aeromonas spp. isolated from bacteremia in southeastern China. Antimicrob. Resist. Infect. Control 10:43. doi: 10.1186/s13756-021-00911-0
Swain, P., Nayak, S., Sahu, A., Meher, P., and Mishra, B. (2003). High antigenic cross-reaction among the bacterial species responsible for diseases of cultured freshwater fishes and strategies to overcome it for specific serodiagnosis. Comp. Immunol. Microbiol. Infect. Dis. 26, 199–211. doi: 10.1016/s0147-9571(02)00059-0
Tichoniuk, M., Gwiazdowska, D., Ligaj, M., and Filipiak, M. (2010). Electrochemical detection of foodborne pathogen Aeromonas hydrophila by DNA hybridization biosensor. Biosens. Bioelectron. 26, 1618–1623. doi: 10.1016/j.bios.2010.08.030
Tsai, Y. H., Shen, S. H., Yang, T. Y., Chen, P. H., Huang, K. C., and Lee, M. S. (2015). Monomicrobial necrotizing fasciitis caused by Aeromonas hydrophila and Klebsiella pneumoniae. Med. Princ. Pract. 24, 416–423. doi: 10.1159/000431094
Tsheten, T., Tshering, D., Gyem, K., Dorji, S., Wangchuk, S., Tenzin, T., et al. (2016). An outbreak of Aeromonas hydrophila food poisoning in deptsang village, Samdrup Jongkhar, Bhutan, 2016. J. Res. Health. Sci. 16, 224–227.
Uma, A., Rebecca, G., Meena, S., and Saravanabava, K. (2010). PCR detection of putative aerolysin and hemolysin genes in an Aeromonas hydrophila isolate from infected Koi carp (Cyprinus carpio). Tamil. J. Vet. Anim. Sci. 6, 31–33.
Wang, G., Clark, C. G., Liu, C., Pucknell, C., Munro, C. K., Kruk, T. M., et al. (2003). Detection and characterization of the hemolysin genes in Aeromonas hydrophila and Aeromonas sobria by multiplex PCR. J. Clin. Microbiol. 41, 1048–1054. doi: 10.1128/JCM.41.3.1048-1054.2003
Wang, R., Zhang, F., Wang, L., Qian, W., Qian, C., Wu, J., et al. (2017). Instant, visual, and instrument-free method for on-site screening of GTS 40-3-2 soybean based on body-heat triggered recombinase polymerase amplification. Anal. Chem. 89, 4413–4418. doi: 10.1021/acs.analchem.7b00964
Wong, C. Y., Heuzenroeder, M. W., and Flower, R. L. (1998). Inactivation of two haemolytic toxin genes in Aeromonas hydrophila attenuates virulence in a suckling mouse model. Microbiology 144, 291–298. doi: 10.1099/00221287-144-2-291
Xiao, X., Lin, Z., Huang, X., Lu, J., Zhou, Y., Zheng, L., et al. (2021). Rapid and sensitive detection of Vibrio vulnificus using CRISPR/Cas12a combined with a recombinase-aided amplification assay. Front. Microbiol. 12:767315. doi: 10.3389/fmicb.2021.767315
Zhang, M., Liu, C., Shi, Y., Wu, J., Wu, J., and Chen, H. (2020). Selective endpoint visualized detection of Vibrio parahaemolyticus with CRISPR/Cas12a assisted PCR using thermal cycler for on-site application. Talanta 214:120818. doi: 10.1016/j.talanta.2020.120818
Zhang, Q., Shi, G. Q., Tang, G. P., Zou, Z. T., Yao, G. H., and Zeng, G. (2012). A foodborne outbreak of Aeromonas hydrophila in a college, Xingyi City, Guizhou, China, 2012. Western Pac. Surveill Response J. 3, 39–43. doi: 10.5365/WPSAR.2012.3.4.018
Zhao, G., Wang, J., Yao, C., Xie, P., Li, X., Xu, Z., et al. (2022). Alkaline lysis-recombinase polymerase amplification combined with CRISPR/Cas12a assay for the ultrafast visual identification of pork in meat products. Food Chem. 383:132318. doi: 10.1016/j.foodchem.2022.132318
Zhou, S., Wang, M., Liu, M., Jiang, K., Wang, B., and Wang, L. (2020). Rapid detection of Enterocytozoon hepatopenaei in shrimp through an isothermal recombinase polymerase amplification assay. Aquaculture 521:734987. doi: 10.1016/j.aquaculture.2020.734987
Keywords: Aeromonas hydrophila, dualplex recombinase-aided amplification assay, CRISPR/Cas12a, detection, diagnosis
Citation: Lin Z, Lu J, Wu S, Lin X, Zheng L, Lou Y and Xiao X (2022) A novel detection method for the pathogenic Aeromonas hydrophila expressing aerA gene and/or hlyA gene based on dualplex RAA and CRISPR/Cas12a. Front. Microbiol. 13:973996. doi: 10.3389/fmicb.2022.973996
Edited by:
Kamal El Bissati, The University of Chicago, United StatesReviewed by:
Rui Pang, Guangdong Academy of Science, ChinaMehdi Soltani, Murdoch University, Australia
Copyright © 2022 Lin, Lu, Wu, Lin, Zheng, Lou and Xiao. This is an open-access article distributed under the terms of the Creative Commons Attribution License (CC BY). The use, distribution or reproduction in other forums is permitted, provided the original author(s) and the copyright owner(s) are credited and that the original publication in this journal is cited, in accordance with accepted academic practice. No use, distribution or reproduction is permitted which does not comply with these terms.
*Correspondence: Yongliang Lou, bHlsQHdtdS5lZHUuY24=; Xingxing Xiao, eGlhb3hpbmd4aW5nQHdtdS5lZHUuY24=
†These authors have contributed equally to this work