- Department of Integrated Traditional Chinese and Western Medicine, Peking University First Hospital, Institute of Integrated Traditional Chinese and Western Medicine, Peking University, Beijing, China
Background: Helicobacter pylori (H. pylori) infects half of the human population globally. Eradication rates with triple or quadruple therapy have decreased owing to the increasing rate of antibiotic resistance. Jinghua Weikang capsule (JWC) is the first and most popular Chinese patent medicine approved by the state for the treatment of gastritis and peptic ulcers caused by H. pylori infection in China. Previous studies have found that JWC has a certain bactericidal effect on drug-resistant H. pylori and its major component, Chenopodium ambrosioides L. inhibits biofilm formation, but the mechanism remains unclear. This study focused on drug-resistant H. pylori and explored whether JWC could reverse drug resistance and its related mechanisms.
Method: The agar plate dilution method, E-test method, and killing kinetics assay were used to evaluate the bactericidal effect of JWC on antibiotic-resistant H. pylori and its effect on antibiotic resistance. Sanger sequencing was used to detect mutations in drug resistance genes. The crystal violet method, scanning electron microscopy, and confocal laser scanning microscopy were used to evaluate the effects of JWC on biofilms. qPCR was performed to evaluate the effect of JWC on the expression of efflux pump-related genes. qPCR and immunofluorescence were used to evaluate the effects of JWC on H. pylori adhesion.
Results: JWC showed considerable antibacterial activity against drug-resistant H. pylori strains, with minimum inhibitory concentration (MIC) values ranging from 64 to 1,024 μg/ml. The MIC of metronidazole (MTZ) against H. pylori 26,695–16R decreased from 64 to 6 μg/ml after treatment with 1/2 MIC of JWC. The resistance of H. pylori 26,695–16R to MTZ was reversed by JWC, and its effect was better than that of PaβN and CCCP. H. pylori 26,695–16R is a moderate biofilm-forming strain, and JWC (16–64 μg/ml) can inhibit the formation of biofilms in H. pylori 26,695–16R. JWC reduced the expression of HP0605-HP0607 (hefABC), HP0971-HP0969 (hefDEF), HP1327-HP1329 (hefGHI), and HP1489-HP1487. JWC reduced the adhesion of H. pylori to GES-1 cells and the expression of adhesives NapA, SabA, and BabA.
Conclusion: The reversal of MTZ resistance by JWC may be achieved through the adhesin/efflux pump-biofilm pathway.
Introduction
Helicobacter pylori (H. pylori) infects half of the global population and can cause a variety of gastric diseases, such as peptic ulcers, chronic gastritis, gastric cancer, and extragastric diseases (Zamani et al., 2018; Ren et al., 2022). The eradication of H. pylori has significantly reduced the incidence and mortality rates of gastric cancer (Chiang et al., 2021). However, large-scale eradication has led to increasing rates of H. pylori resistance to multiple antibiotics, the main cause of eradication failure (Zhong et al., 2022). The eradication rate of triple therapy is currently less than 70% (Liu et al., 2018). Since 2017, H. pylori has been listed by the World Health Organization as one of the 20 pathogens that pose the most serious threat to human health owing to its drug resistance (Tacconelli et al., 2018). It is difficult to reduce the resistance rate. The causes and mechanisms of antibiotic resistance are complicated and include specific resistance factors against a particular antibiotic (resistance gene mutation; Tshibangu-Kabamba and Yamaoka, 2021), as well as nonspecific resistance factors, such as biofilms and efflux pumps (Zanotti and Cendron, 2019). Bacterial biofilms are complexes composed of bacteria and extracellular polymers (EPS) such as proteins, polysaccharides, lipids, and DNA secreted by bacteria, which create a protective environment for bacteria (Høiby et al., 2010; Rather et al., 2021). Bacteria that form biofilm structures are highly resistant to harsh external environments such as antibiotic exposure. It has been demonstrated that bacteria are 10–1,000 times more resistant to antibiotics when they form biofilms (Chen and Wen, 2011; Yonezawa et al., 2019; Hou et al., 2022). The formation of H. pylori biofilms includes four steps: adhesion, growth, maturation, and diffusion (Hou et al., 2022). Adhesion is the first step and a prerequisite for biofilm formation. The adhesion of H. pylori is mediated by dozens of specific adhesin receptors, among which blood group antigen-binding adhesin (BabA), sialic acid adhesin (SabA), and neutrophil-activating protein A (NapA) play major roles (Fu, 2014; Doohan et al., 2021; Matos et al., 2021). The bacterial efflux pump is a transmembrane transporter protein that mediates the pumping of intracellular drugs out of the cell, thereby reducing the intracellular drug concentration and promoting drug resistance. The efflux effect of the active efflux pump system in bacteria is an important mechanism underlying nonspecific drug resistance. The resistance nodulation and cell division (RND) family, major facilitator super (MFS) family, and ATP-binding cassette (ABC) family are the predominant efflux pump families in H. pylori. Several studies have shown that the efflux pump expression in biofilm-forming bacteria is higher than that in planktonic cells (Soto, 2013). The expression levels of HP0605–HP0607 (hefABC), HP0971-HP0969 (hefDEF), HP1327–HP1329 (hefGHI), and HP1489–HP1487 in biofilm-forming strains are higher than those in planktonic bacteria (Yonezawa et al., 2019). The expression level of Hp1174 [glucose/galactose transporter (gluP)] also follows these rules (Ge et al., 2018). This suggests that efflux pumps and biofilms may interact or act synergistically to increase drug resistance.
As an exogenous pathogenic factor, H. pylori is equivalent to “evil qi” in traditional Chinese medicine (TCM). According to TCM, H. pylori infection mostly presents with basic symptoms of cold and heat in complexity and deficiency in complexity. Jinghua Weikang capsules (JWC) were obtained from Chenopodium ambrosioides L. (CAL) and Rubiaceae adina pilulifera (RAP). CAL regulates qi, disperses cold, and kills insects, while RAP clears heat and removes blood stasis. The two are used in combination to harmonize the spleen and stomach. It is widely used in digestive diseases related to H. pylori infection and has a good basis for application and clinical efficacy (Hui and Xuezhi, 2014). Previous studies have found that JWC and its main component, CAL, can kill and inhibit standard drug-resistant H. pylori, and CAL can inhibit the formation of drug-resistant H. pylori biofilms (Liu et al., 2013; Ye et al., 2015; Enen et al., 2020). In the remedial treatment of patients with chronic gastritis or peptic ulcer suffering from H. pylori infection relapses, the addition of JWC improved the eradication rate of H. pylori compared to bismuth quadruple therapy (90.0 vs. 82.0%; Hong et al., 2016). Antibiotic resistance is the main reason for H. pylori eradication failure. JWC can contribute toward improving the eradication rate of H. pylori in remedial treatment, however, its mechanism is still unknown. This study explores whether JWC can reverse the drug resistance and related mechanisms in H. pylori.
Materials and methods
Drug preparation
The volatile oil of JWC (Tasly Pharmaceutical Group Co. LTD, Tianjin, China) was mixed with dimethyl sulfoxide (DMSO, Thermo Fisher Scientific, Waltham, MA, United States) at a 1:4 volume ratio and dissolved in sterile deionized water. The density of JWC was 937 mg/ml.
Bacterial culture and identification
The H. pylori strains used in this experiment (Table 1) were all obtained from the Department of Gastroenterology, Peking University First Hospital, among which H. pylori Strains1–6 were isolated from clinical patients who had previously failed H. pylori eradication therapy and required drug sensitivity test to guide the eradication regimen and H. pylori 26,695–16R is an rdxA null deletion mutant derivative of H. pylori 26,695 (Sisson et al., 2000). H. pylori strains were frozen in –80°C refrigerator. The cryopreservation solution was prepared by brain heart infusion (OXOID, Basingstoke, United Kingdom) and glycerol (Solarbio, Beijing, China). H. pylori was inoculated on Columbia blood agar (OXOID, Basingstoke, United Kingdom) plates containing 8% sheep blood (Lablead, Beijing, China), placed upside down at 37°C in a microaerophilic (85% N2, 10% CO2, 5% O2) environment for 48–72 h, and the positive ones were sub-cultured, generally no more than seven generations. The strains were identified by colony morphology, Gram staining, and rapid urease tests.
Drug susceptibility test
E-test method
Helicobacter pylori cultured for 48–72 h was uniformly ground into a cryopreservation solution, and the bacterial solution was diluted to 3 × 108 CFU/ml. One hundred microliters of bacterial solution was pipetted onto the surface of the medium, smeared evenly with L sticks, placed on an E-test drug susceptibility test strip (Liofilchem, Roseto degli Abruzzi, Italy), and incubated at 37°C for 72 h in a microaerophilic environment to read the results. The value corresponding to the ring region where the bacteria stops growing is the minimum inhibitory concentration (MIC) of an antibiotic for H. pylori. According to EUCAST Clinical Breakpoint standard 2022, H. pylori strains that could grow in medium containing amoxicillin (MIC > 0.125 μg/ml), levofloxacin (MIC > 1 μg/ml), clarithromycin (MIC > 0.5 μg/ml), and metronidazole (MIC > 8 μg/ml) were identified as drug-resistant strains. Phenylalanine-arginine β-naphthylamide (PaβN, Sigma-Aldrich, St. Louis, MO, United States) and carbonyl cyanide m-chlorophenylhydrazonequinoline (CCCP, Sigma-Aldrich, St. Louis, MO, United States) are the most common inhibitors of efflux pumps and have also been found to inhibit biofilm formation (Zhang et al., 2010; Kinana et al., 2016; Tang et al., 2020; Dawan et al., 2022).
Agar plate dilution method
Media containing different concentrations of JWC (2048, 1,024, 512, 256, 128, 64, 32, and 16 μg/ml) were prepared. Drug-free and DMSO (Sigma-Aldrich, St. Louis, MO, United States) -containing media were used as controls. H. pylori cultured for 48–72 h was uniformly ground into the cryopreservation solution, and the bacterial solution was diluted to 3 × 108 cfu/ml. A 1 μl sterile inoculating ring was used to inoculate the bacterial solution on the surface of the drug-containing medium, and observed after 72 h of culture in a microaerobic environment at 37°C. The MIC of the lowest drug concentration on a medium without colonies was the MIC for JWC.
Inhibiting kinetics and killing kinetics assay
The inhibition and killing kinetics assays were performed as previously reported (Shen et al., 2021; Peng et al., 2022). For the inhibition kinetics assay, H. pylori 26,695–16R cultured for 48–72 h was collected at 0 (control), 0.25, 0.5, and 1 times the MIC concentration for JWC in Brucella Broth (BD, Franklin Lakes, NJ, United States) containing 10% Foetal Bovine Serum (FBS, BI, Kibbutz Beit Haemek, Israel) and shaken (100–120 rpm) at 37°C. Then, at 0, 12, 24, 36, 46, 48, 60, and 72 h, 100 μl of each sample was tested for absorbance at 600 nm. Three holes were set in each sample, and the experiment was repeated three times. For the killing kinetics assay, H. pylori 26,695–16R cultured for 48–72 h was collected at 0 (control), 1, 2, and 4 times the MIC concentration of JWC in Brucella broth containing 10% FBS and treated in a shaker (100–120 rpm) at 37°C. At 0, 4, 8, 12, and 24 h, 30 μl was removed from each sample, and serial 10-fold dilutions were prepared in Brucella broth. One hundred microliters of the diluted solution were plated on Columbia blood agar plates and incubated at 37°C, and colonies were counted and averaged after 3 days. The results are expressed as Log10 (CFU/ml). This experiment was repeated twice.
Sanger sequencing
Bacterial genomic DNA was extracted using the QIAamp DNA Mini Kit (QIAGEN GmbH, Hilden, Germany), and the samples were stored at –80°C. The 23S rRNA, gyrA (hp0701), gyrB (hp0501), rdxA (hp0954), and frxA (hp0642) fragments were amplified using PCR. Primers used are listed in Table 1 (Teh et al., 2014). PCR amplification products were examined on 1.0% agarose gels and bands were observed. The PCR product was separated and purified using magnetic beads (Ensure Biologicals, Shanghai, China). The PCR product sequencing was performed by Beijing Liuhe Bgi Co. Ltd. (Beijing, China).
Quantitative real-time PCR
Helicobacter pylori cultured for 48–72 h was collected in Brucella broth containing different concentrations of drugs and shaken (100–120 rpm) at 37°C for 2 h. Total RNA of H. pylori was extracted using the TRIzol method, mRNA was reverse transcribed into cDNA using a High Capacity cDNA Reverse Transcription Kit (Thermo Fisher Scientific, Waltham, MA, United States), and PCR amplification was carried out using PowerUp™ SYBR™Green Master Mix (Thermo Fisher Scientific, Waltham, MA, United States). Primers used in this experiment are listed in Table 2 (Yonezawa et al., 2019; Zhong et al., 2021).
Crystal violet staining
The biofilm of H. pylori was cultured using the 96-well plate method. H. pylori cultured for 48–72 h was collected in Brucella broth containing different concentrations of drugs and treated in a microaerobic environment at 37°C for 72 h. Each group was provided with nine holes. After incubation, the upper bacterial solution was gently discarded, the plate was rinsed three times with Phosphate Buffer Saline (PBS, Thermo Fisher Scientific, Waltham, MA, United States), 200 μl of anhydrous methanol (Beijing Tongguang Fine Chemical, Beijing, China) was added to each well for 15 min, the methanol was discarded, and the plate was air-dried. Then, 200 μl of 1% ammonium oxalate crystal violet reagent (Solarbio, Beijing, China) was added to each well for staining for 5 min and then washed with running water. After natural drying, 200 μl 95% ethanol (Beijing Tongguang Fine Chemical, Beijing, China) was added to each well and dissolved in a shaker (80 rpm) at 37°C for 30 min. The Optical Density (OD) value was measured using a microplate reader (TECAN, Männedorf, Switzerland; absorbance at 590 nm). Dc is the OD value of the blank wells and D is the mean value of the remaining OD values after removing the outliers. A value of D > 4 × Dc, was determined to be a strong biofilm-forming H. pylori strain; 2 × Dc < D ≤ 4 × Dc, a moderate biofilm-forming H. pylori strain; Dc < D ≤ 2 × Dc, a weak biofilm-forming H. pylori strain; and D ≤ Dc, a non-biofilm-forming H. pylori strain.
Scanning electron microscope
The nitrocellulose (NC) membrane (GE, Boston, Mass, United States) was cut into 1 × 1 cm pieces to prepare a solid medium containing NC membranes and different concentrations of drugs. H. pylori cultured for 48–72 h was uniformly ground into the cryopreservation solution, and the bacterial solution was diluted to 3 × 108 cfu/ml. A total of 10 μl of bacterial solution was pipetted onto the NC membrane, spread evenly, and incubated in a microaerophilic environment at 37°C for 72 h. The NC membrane was then removed and placed in a 6-well plate, and an appropriate amount of glutaraldehyde (Regen Biotechnology Co., LTD, Beijing, China) was added to fix it at 4°C for 2 h. After fixation, the membrane was air-dried and the results were observed using a field emission scanning electron microscope (JEOL, Tokyo, Japan). Metronidazole used in this study was obtained from Aladdin, Shanghai, China.
Confocal laser scanning microscope
A LIVE/DEAD™ BacLight™ Bacterial Cell Activity Assay Kit (Thermo Fisher Scientific, Waltham, MA, United States) was used for fluorescence staining. Dye A (SYTO™ 9 dye) penetrates the bacterial cell membrane and binds to DNA to stain bacteria green. Dye B (propidium iodide) only penetrates incomplete bacterial cell membranes; when the bacteria die, the permeability of the cell membrane changes, and dye B dyes the dead bacteria red. Glycerin was used for microscopic observations.
The biofilm was constructed as described above, and the incubated NC membrane was removed aseptically, placed in a 24-well plate and rinsed 3 times in PBS. Mix 3 μl A and B 1:1, add 1 ml normal saline, add 100 μl to each well, and incubate in the dark for 15 min. The NC membrane was removed and placed on a glass slide, and glycerol was added for observation under a microscope. The samples were observed within 1 h to avoid the effects of bacterial death caused by prolonged exposure. CLSM (Leica, Wetzlar, Germany) was performed using an argon laser at 488 nm excitation, with the blue channel receiving the green signal and the 560 nm green channel receiving the red signal, and scanning from the free side of the H. pylori biofilm to the attached side of the slide layer-by-layer at an interval of 1 μm.
Immunofluorescent staining
GES-1 cells (1 × 105) were infected with H. pylori (multiplicity of infection [MOI] = 200:1) for 2 h. The drug group was then pre-treated for 2 h. After 2 h, the culture was aspirated, 4% paraformaldehyde (Regen Biotechnology, Beijing, China) was added, incubated at room temperature for 20 min, paraformaldehyde was discarded, and the cells were washed thrice with PBST [PBS + 0.05% Tween 20 (Solarbio, Beijing, China)] and maintained for 5 min each time. After washing, PBST containing 0.1% Triton (Solarbio, Beijing, China) was added for 20 min to permeabilize the cells. Next, blocking solution [TBST containing 5% BSA (Lablead, Beijing, China)] was added for 30 min at room temperature, and the blocking solution was removed and cleaned twice with PBST. Then, the primary anti-Helicobacter pylori antibody (ab20459, Abcam, Cambridge, United Kingdom) was added and incubated in a wet box overnight at 4°C. The cells were washed three times with PBST and Alexa Fluor® 488-labeled goat anti-rabbit IgG secondary antibody (Zhongshan Jinqiao Biotechnology, Beijing, China), incubated at room temperature, and protected from light for 1 h. After 1 h, the secondary antibody was aspirated, washed three times with PBST, and stained with mounting medium containing DAPI (Zhongshan Jinqiao Biotechnology, Beijing, China) for 5 min. The DAPI and fluorescein isothiocyanate (FITC) channels were selected under a fluorescence microscope (Nikon, Tokyo, Japan) and photographed for analysis. The results were expressed as H. pylori fluorescence area/DAPI fluorescence area calculated using ImageJ software.
Statistical analysis
Data are presented as mean ± standard deviation. Differences between groups were assessed using one-way ANOVA. Pairwise comparisons were performed using Dunnett’s or Tukey’s post hoc test. p was set at p < 0.05. Statistical analysis was performed using the GraphPad Prism 8.1 software.
Results
Screening of drug-resistant Helicobacter pylori strains by E-test
According to EUCAST Clinical Breakpoint standard 2022, H. pylori strains with AML MIC > 0.125 μg/ml, CLR MIC > 0.5 μg/ml, LEV MIC > 1 μg/ml, and MTZ MIC > 8 μg/ml were antibiotic resistant. Seven drug-resistant H. pylori strains were screened, including six multidrug-resistant strains (Nos. 1–6) and one single drug-resistant strain (26695–16R). There were five CLR-resistant strains (Nos. 1, 2, 4, 5, and 6), five LEV-resistant strains (Nos. 1, 2, 3, 4, 6, and 26,695–16R), six MTZ-resistant strains (Nos. 1, 2, 3, 4, 6, and 26,695–16R), and one AML-resistant strain (No. 1). The MICs of the antibiotics against H. pylori are listed in Table 3.
In vitro antibacterial activities of JWC on Helicobacter pylori
The MICs of JWC against the drug-resistant strains were determined using the agar dilution method. The results showed that JWC had considerable antibacterial activity against drug-resistant H. pylori strains, with MIC values ranging from 64 to 1,024 μg/ml (Table 4), suggesting that there were differences in the antibacterial activity against different strains.
The MICs of antibiotics after JWC intervention
JWC at ½ MIC was used to inhibit drug-resistant H. pylori strains. Based on previous studies (Hirata et al., 2010; Tsugawa et al., 2011), the concentration of PaβN used in this study was 20 μg/ml, which had no inhibitory effect on H. pylori 26,695–16R growth (Supplementary Table S1). Owing to the toxicity of CCCP, which had an obvious inhibitory effect on H. pylori 26,695–16R growth, its MIC against H. pylori 26,695–16R was determined to be 5 μg/ml using the agar dilution method (Supplementary Table S2); to exclude the bactericidal effect of CCCP itself, we used a concentration of 1 μg/ml for the test (Supplementary Figure S1). The results showed that JWC and efflux pump inhibitors PaβN (20 μg/ml) and CCCP (1 μg/ml) had no effect on the MICs of LEV-resistant strains but had a slight effect on the MICs of CLA-resistant strains. However, the MIC of MTZ against H. pylori 26,695–16R decreased from 64 μg/ml to 6 μg/ml after treatment with ½ MIC of JWC. The drug resistance of H. pylori 26,695–16R to MTZ was reversed, and its effect was better than that of efflux pump inhibitors PaβN (20 μg/ml) and CCCP (1 μg/ml; Tables 5–7).
In this study, JWC had different MICs for different drug-resistant H. pylori strains and showed a unique effect on reducing MTZ resistance in H. pylori 26,695–16R, suggesting that its effect on reducing drug resistance may also be affected by bacterial characteristics. To further explore the possible mechanism based on the confirmed effect, H. pylori 26,695–16R was selected as the research object in subsequent experiments.
Inhibiting kinetics and killing kinetics assay
The kinetics of the inhibition and killing of H. pylori 26,695–16R by JWC were time-and dose-dependent (Figure 1). JWC inhibited the growth of H. pylori 26,695–16R at concentrations as low as 16 μg/ml (1/4 MIC). The OD600 of the bacterial solution did not increase significantly after treatment with 32 μg/ml (1/2 MIC) and 64 μg/ml (MIC) concentrations of JWC (Figure 1A). JWC killed H. pylori 26,695–16R at 64–256 μg/ml (MIC to 4 MIC), which indicated a 1,000-fold reduction in the number of bacteria compared with the initial inoculation. JWC at 64–256 μg/ml (MIC to 4 MIC) completely killed H. pylori 26,695–16R after 8–24 h of intervention (Figure 1B).
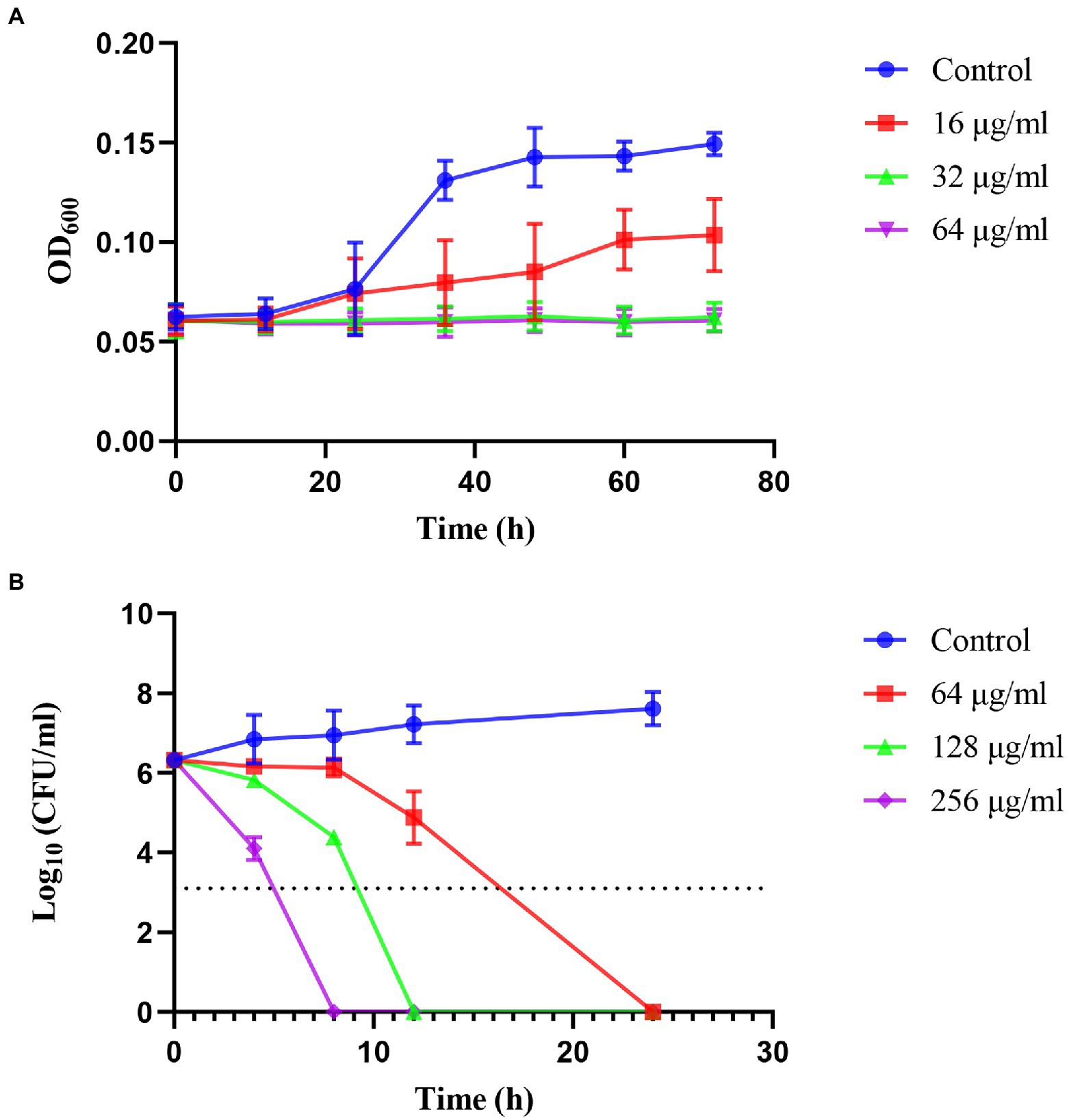
Figure 1. Inhibiting kinetic curves and killing kinetic curves. (A) Inhibiting kinetics curves of JWC on Helicobacter pylori 26,695–16R. (B) Killing kinetics curves of JWC on H. pylori 26,695–16R. The dotted line represents a 1,000-fold reduction in the number of bacteria compared to the initial inoculation.
Detection of drug resistance-related genes in Helicobacter pylori 26,695–16R
Gene sequencing results showed that only the G210T point mutation occurred in the MTZ resistance-related gene rdxA among several genes detected in H. pylori 26,695–16R, and intervention with the efflux pump inhibitors PaβN (20 μg/ml), CCCP (1 μg/ml), and JWC did not affect the mutation, as shown by the red ellipse in Figure 2. Primers used in these experiments are listed in Table 1.
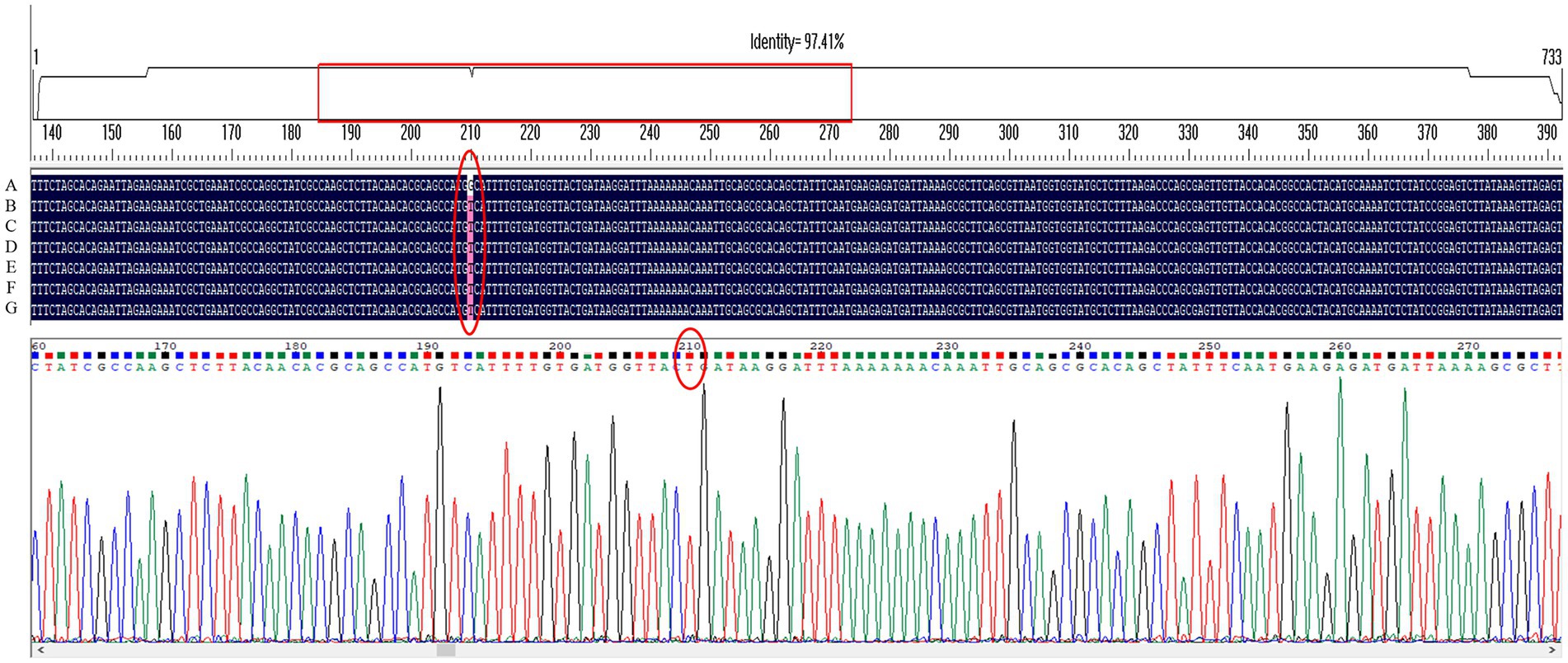
Figure 2. G210T point mutation in the MTZ resistance-related gene rdxA of H. pylori 26,695–16R under different drug interventions. A, H. pylori 26,695; B, H. pylori 26,695–16R; C, Treated with PaβN (20 μg/ml); D, Treated with CCCP (1 μg/ml); E, Treated with JWC 16 μg/ml; F, Treated with JWC 32 μg/ml; G, Treated with JWC 64 μg/ml.
Effect of JWC on the biofilm of Helicobacter pylori 26,695–16R by crystal violet method
When D/Dc > 4, it was determined to be a strong biofilm-forming strain; 2 < D/Dc ≤ 4, a moderate biofilm-forming strain; and 1 < D/Dc ≤ 2, a weak biofilm-forming strain. D/Dc ≤ 1 was defined as a strain without biofilm formation. The results showed that H. pylori 26,695–16R is a moderate biofilm-forming strain, and CCCP (1 μg/ml) and JWC (16–64 μg/ml) inhibited biofilm formation in H. pylori 26,695–16R, and the difference was statistically significant (Figure 3).
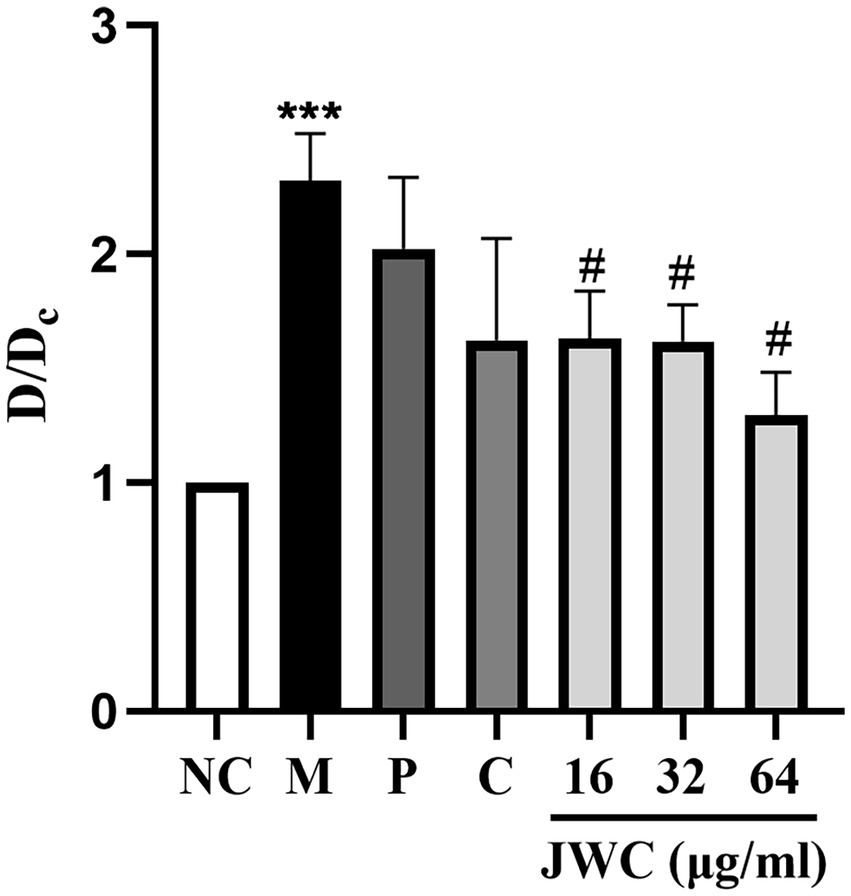
Figure 3. Effect of JWC, efflux pump inhibitors and MTZ on the biofilm of H. pylori 26,695–16R. Dc is the OD value of the blank control group, and D is the OD value of the other groups. NC: the blank control group, culture medium without H. pylori 26,695–16R; M, Model group, culture medium with H. pylori 26,695–16R; P, PaβN (20 μg/ml); C, CCCP (1 μg/ml). ***p < 0.001, compared with the results of the control group. #p < 0.05, compared with the results of the model group.
Effect of JWC on the biofilm of Helicobacter pylori 26,695–16R by SEM
The normal group showed a biofilm structure formed by H. pylori 26,695–16R. Bacteria and extracellular matrix that are closely linked to bacteria can be found in biofilms. The bacteria were mostly rod-shaped with tight connections and fewer voids. After treatment with CCCP (1 μg/ml) and JWC, the biofilm structure of bacteria was disrupted, connections between bacteria became sparse, and the number of voids increased. The degree of destruction of the bacterial biofilm structure increased with increasing JWC concentrations. After treatment with MTZ at a concentration of 64 μg/ml, H. pylori 26,695–16R cells converted into a coccoid shape, which is a stress response to MTZ and one of the survival mechanisms of H. pylori (Figure 4).
Effect of JWC on the biofilm of Helicobacter pylori 26,695–16R by CLSM
CLSM images provide a rough outline of H. pylori biofilms and the viability of the bacteria. Green represents live bacteria and red represents dead bacteria. In the control group, we observed that H. pylori 26,695–16R formed a dense biofilm of a certain thickness and good bacterial activity. After treatment with PaβN (20 μg/ml), CCCP (1 μg/ml), and JWC (16–64 μg/ml), the biofilm structure of H. pylori 26,695–16R loosened, with a reduced proportion of live bacteria and an increased proportion of dead bacteria, indicating that biofilm formation was inhibited. When the concentration of JWC increased, the ratio of live/dead bacteria decreased and the amount of biofilm formed by bacterial aggregation decreased (Figure 5).
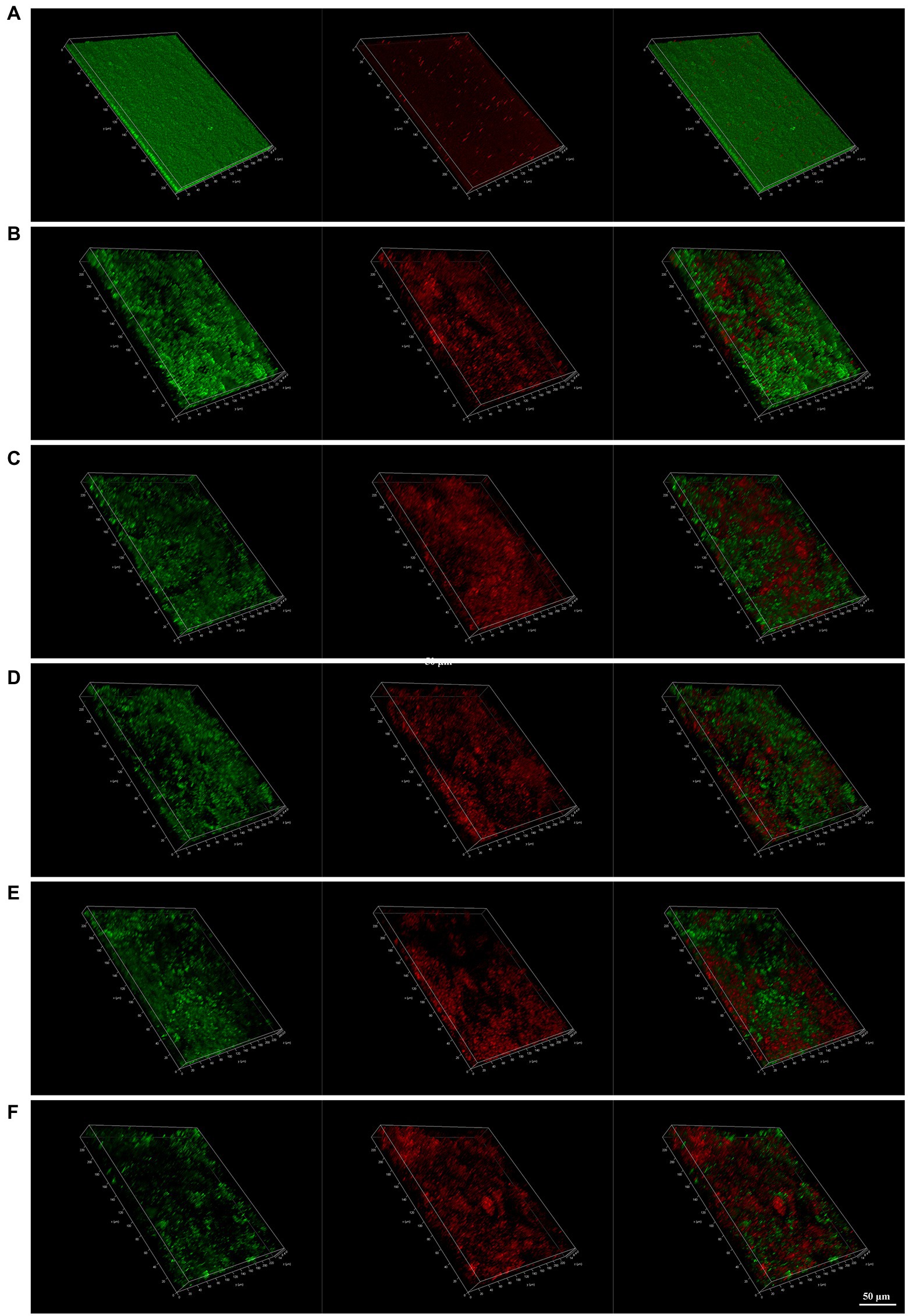
Figure 5. Confocal laser scanning microscope (CLSM) images of H. pylori 26,695–16R strain biofilms. Cells stained with membrane-permeant SYTO 9 (green) and membrane-impermeant propidium iodide (red) were visualized by CLSM. (A) Control group; (B) treated with PaβN (20 μg/ml); (C) treated with CCCP (1 μg/ml); (D) treated with JWC 16 μg/ml; (E) treated with JWC 32 μg/ml; (F) treated with JWC 64 μg/ml.
Influence of JWC on efflux pump gene expression in Helicobacter pylori 26,695–16R
qPCR results showed that both CCCP and JWC inhibited the expression of HP0605 and HP0971. CCCP and JWC at 64 μg/ml (MIC) and 128 μg/ml (2 MIC) inhibited the expression of HP1327. CCCP and JWC at 32 μg/ml (1/2 MIC) and 128 μg/ml (2 MIC) inhibited the expression of HP1489, and the difference was statistically significant, as shown in Figure 6. The primers used are listed in Table 2.
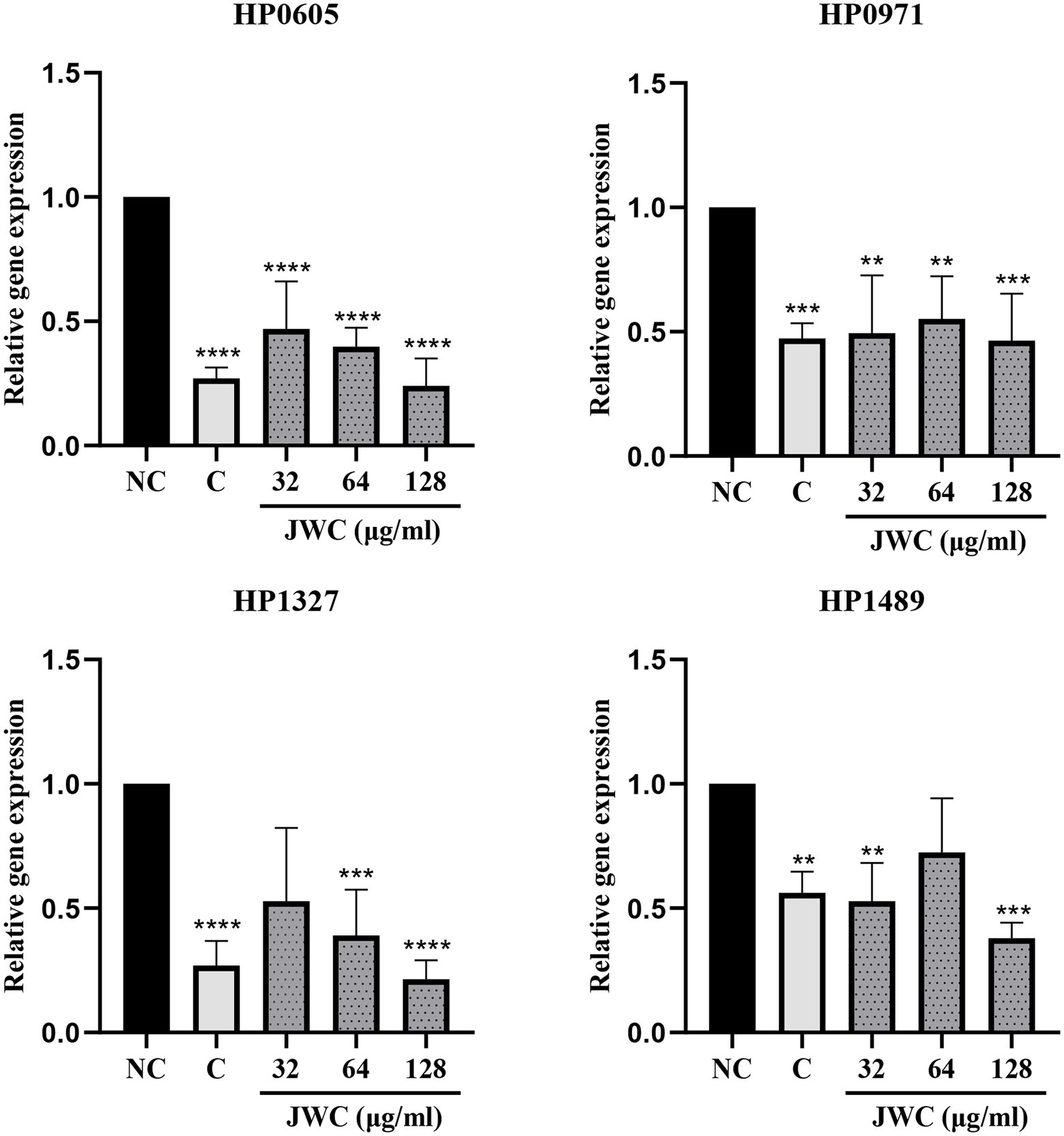
Figure 6. Expression of different efflux effect genes of H. pylori 26,695–16R after incubation with PaβN, CCCP and different concentrations of JWC. NC, Normal control group; C, CCCP (1 μg/ml); **p < 0.01, ***p < 0.001, ****p < 0.0001, compared with the results of the control group.
Jinghua Weikang capsule inhibited the adhesion of Helicobacter pylori 26,695–16R to GES-1 cells
As shown in Figure 7, blue fluorescence indicates the nucleus of GES-1 cells and green fluorescence indicates H. pylori. After observing the pictures in different channels, they were merged using a filter function. The merged results showed that H. pylori can adhere to GES-1 cells and that 16, 32, and 64 μg/ml JWC dose-dependently reduced the amount of H. pylori adherence (Figure 7A). According to the results of fluorescence intensity analysis using Image J software, the adhesion of H. pylori was inhibited by 40–60% when 16–64 μg/ml JWC was used (Figure 7B).
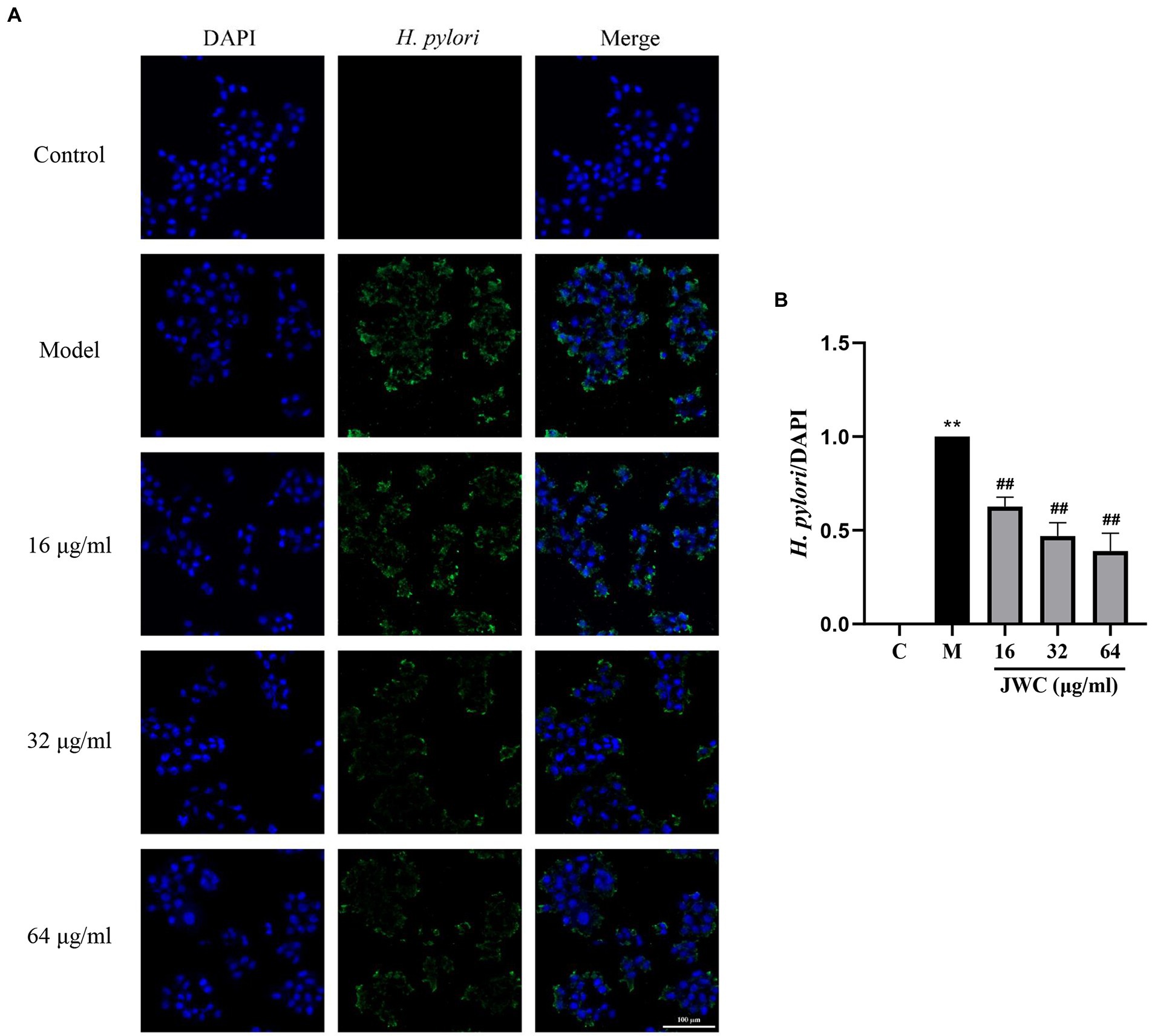
Figure 7. Effect of JWC on the adhesion of H. pylori 26,695–16R to GES-1 cells. (A) Immunofluorescence images of H. pylori and GES-1 adhesion after JWC intervention. Control group, only GES-1 cells; Model group, GES-1 and H. pylori. (B) The H. pylori fluorescence area/DAPI fluorescence area calculated by ImageJ software. **p < 0.01, compared with the results of the control group. ##p < 0.01, compared with the results of the model group. Bold values represent a meaningful MIC change of antibiotic and the H. pylori strain used in subsequent experiments.
Effect of JWC on adhesins of Helicobacter pylori 26,695–16R
The results showed that 32 μg/ml and 64 μg/ml JWC and CCCP decreased the expression of SabA, BabA and NapA. PaβN only decreased SabA. On the whole. JWC decreased the expression of adhesins in a concentration-dependent manner (Figure 8).
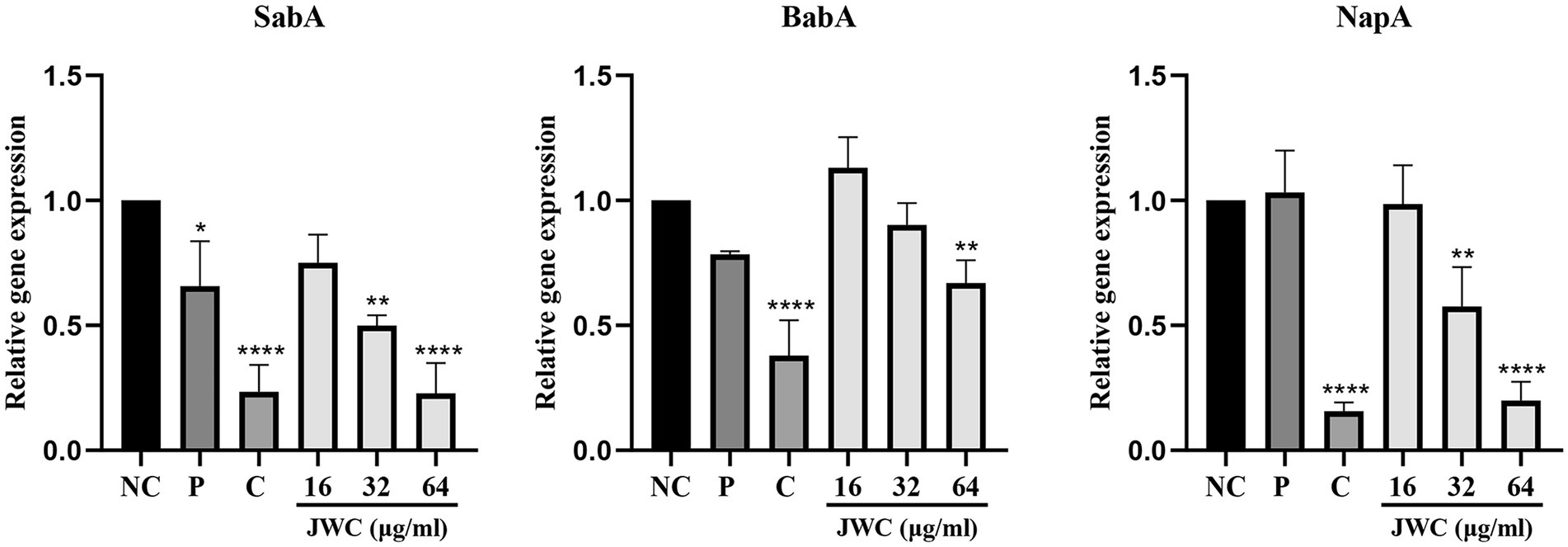
Figure 8. Effect of JWC on adhesions of H. pylori 26,695–16R. NC, Normal control group, H. pylori 26,695–16R; P, PaβN (20 μg/ml); C, CCCP (1 μg/ml). *p < 0.05, **p < 0.01, ****p < 0.0001, compared with the results of the control group.
Discussion
Antibiotic resistance of H. pylori has been increasing worldwide (Tshibangu-Kabamba and Yamaoka, 2021; Ho et al., 2022; Veenendaal et al., 2022; Zhong et al., 2022). Resistance of H. pylori to MTZ is more common than that of other antibiotics (Gerrits et al., 2006; Ho et al., 2022). MTZ resistance rates range from 42 to 96% worldwide, with higher rates in developing countries (Geng et al., 2022; Ho et al., 2022; Liu et al., 2022; Tian et al., 2022; Veenendaal et al., 2022). In triple, quadruple, and sequential therapies, including MTZ, eradication failure is often associated with MTZ resistance (Fischbach and Evans, 2007; Gatta et al., 2009). The nitro moiety of MTZ is reduced to a highly active compound that exerts antibacterial activity against H. pylori (Jenks and Edwards, 2002). The inactivation of rdxA (which encodes an oxygen-insensitive NADPH nitroreductase), frxA (which encodes NADPH flavin oxidoreductase), and fdxB (which encodes a ferredoxin-like protein) was closely associated with the failure of enzymatic reduction and MTZ resistance (Jenks and Edwards, 2002). In this study, seven resistant strains were screened, and JWC was found to have a significant anti-drug resistance effect on the MTZ-resistant strain H. pylori 26,695–16R, reducing its MIC value against MTZ from 64 μg/ml to 6 μg/ml and reversing resistance. For H. pylori 26,695–16R, the effect of JWC on reducing MTZ resistance was better than that of the efflux pump inhibitors PaβN and CCCP. The G210T point mutation in the rdxA gene of H. pylori 26,695–16R was found by sequencing, but the point mutation did not change after drug treatment, suggesting that the mechanism by which JWC reverses MTZ resistance is not related to the rdxA mutation, and that JWC may influence MTZ resistance through other mechanisms.
In addition to gene mutations, biofilm formation in vivo is an important mechanism leading to drug resistance. Biofilms are communities of microorganisms attached to a surface, and the surrounding EPS matrix is composed of extracellular polysaccharides, DNA, and proteins (Rabin et al., 2015). Biofilms play an important role in the persistence of bacterial infections, reducing bacterial susceptibility to antibiotics, and counteracting host immune mechanisms, allowing bacteria to survive in hostile environments (Rabin et al., 2015; Del Pozo, 2018). In the initial stage of biofilm formation, H. pylori is helical, and after effective adhesion and proliferation on the surface, the morphology changes to helical, rod-shaped, curved, spherical, and filamentous. However, in prolonged culture, all cells in the biofilm eventually transformed into globular cells, indicating that they were involved in survival and had a higher tolerance to adverse environmental factors (Krzyżek et al., 2020). The crystal violet method showed that H. pylori 26,695–16R is a moderate biofilm-forming strain, and CCCP and JWC (16–64 μg/ml) could inhibit biofilm formation in H. pylori 26,695–16R. After treatment with CCCP and JWC, the biofilm structure of bacteria was disrupted, connections between bacteria became sparse, and the number of voids increased. The degree of destruction of the bacterial biofilm structure increased with increasing JWC concentrations. However, after treatment with MTZ at a concentration of 64 μg/ml, the morphology of H. pylori 26,695–16R became spherical, indicating that MTZ is a powerful toxic substance to bacteria, and that bacteria obtain stronger self-protection ability through sphericity, which is also the reason for their resistance to MTZ.
The efflux pump system is one of the mechanisms of biofilm formation and is a key nonspecific mechanism of drug resistance in gram-negative bacteria (Hall and Mah, 2017). The efflux pump expels toxic substances such as antibiotics from the bacterial cytosol, thereby reducing the intracellular concentration of antibiotics and conferring antibiotic resistance to the bacteria (Poole, 2007). The RND family, the most studies in regards to their involvement in bacterial biofilm formation, is composed of inner membrane, periplasmic membrane fusion, and outer membrane proteins (Nikaido, 2011). At least one efflux pump, AcrAB-TolC, belongs to the RND family, and four gene clusters encoding RND, namely, HP0605-HP0607 (hefABC), HP0971-HP0969 (hefDEF), HP1327-HP1329 (hefGHI), and HP1489-HP1487, are currently detected in H. pylori (Ye et al., 2020). An enhanced efflux system is the first step in the development of MTZ resistance in H. pylori (Tsugawa et al., 2011). Several studies have shown that the expression of the efflux pump gene in biofilm-forming cells was significantly higher than that in planktonic cells (Soto, 2013; Attaran et al., 2017). For example, the expression levels of HP0605-HP0607 (hefABC), HP0971-HP0969 (hefDEF), HP1327-HP1329 (hefGHI), and HP1489-HP1487 in biofilm-forming strains are higher than those in planktonic bacteria (Yonezawa et al., 2019). This study found that JWC reduced the expression of HP0605-HP0607 (hefABC), HP0971-HP0969 (hefDEF), HP1327-HP1329 (hefGHI), and HP1489-HP1487, suggesting that JWC may reduce H. pylori resistance to MTZ by reducing the expression of efflux pump genes, and may also indirectly affect biofilm formation by reducing the expression of efflux pump genes to reduce H. pylori resistance to MTZ. However, the specific mechanism by which efflux pumps affect biofilm formation is not clear at present, which may be related to the pumping of substances related to biofilm formation. A previous study found that emodin, baicalin, schizandrin, and berberine significantly decreased the MIC of amoxicillin and tetracycline against some H. pylori strains, and the mechanism may be related to the reduction in hefA mRNA expression (Huang et al., 2015). Our study is consistent with this view and complements the study of efflux pump genes and related biofilms.
Adhesion is the first step and prerequisite for biofilm formation. The expression of adhesins increases during the transition from the planktonic to the biofilm phase (Krzyżek et al., 2020). Several studies have shown that adhesive proteins, such as NapA, AlpB, SabA, BabA, Homb, LabA and HopZ are involved in biofilm formation (Cooksley et al., 2003; Yang et al., 2011; Acio-Pizzarello et al., 2017; Servetas et al., 2018; Zhao et al., 2021). Compared to wild-type strains, ArsRS mutants had high surface attachment and biofilm production, and the expression of genes encoding outer membrane proteins was increased in these mutants, including AlpB, SabA, BabA, Homb, LabA and HopZ (Acio-Pizzarello et al., 2017; Servetas et al., 2018). NapA is a surface protein that attracts and activates neutrophils and promotes endothelial adhesion and production of oxygen radicals and chemokines (D’Elios et al., 2007). When Helicobacter pylori is exposed to oxidative stress, NapA is highly expressed to resist oxygen stress injury, thus relieving bacterial survival pressure and promoting the formation and aggregation of EPS to promote biofilm formation (Cooksley et al., 2003; Zhao et al., 2021). Another study also showed that NapA plays a role in adhesion to a substratum and H. pylori and hence influences biofilm formation (Yang et al., 2011). In this study, we found that JWC dose-dependently reduced the adhesion of H. pylori to GES-1 cells and the expression of adhesives NapA, sabA and babA, suggesting that JWC may reduce the adhesion between H. pylori and H. pylori or GES-1 by decreasing the expression of adhesins, thus affecting the formation of biofilms and inhibiting drug resistance.
Although it was found in this study that JWC considerably reduced the drug resistance of MTZ-resistant strain 26,695–16R, it did not reduce the drug resistance of other strong MTZ-resistant strains, indicating that the ability of JWC to reduce MTZ resistance is not universal and may be related to the strength of MTZ resistance of the strain itself. The reversal of metronidazole resistance by JWC may be achieved through the adhesin/RND efflux pump-biofilm pathway.
Conclusion and outlook
JWC had good antibacterial effects against drug-resistant H. pylori strains and reversed the drug resistance of the MTZ-resistant strain 26,695–16R in vitro, the mechanism of which was related to the adhesin/RND efflux pump-biofilm pathway. However, biofilms are difficult to construct in animals because of the low ratophilic nature of H. pylori, which requires further experimental exploration in vivo. The mechanisms by which efflux pumps and adhesins inhibit biofilm formation require further investigation. In addition to its bactericidal effect, JWC has the advantages of reducing drug resistance and multi-targeting, reflecting the concept of potentiation rather than pure antagonism against drug-resistant H. pylori. This study provides an explanation for the mechanism by which JWC inhibits drug-resistant H. pylori, experimental support for the clinical application of JWC in combination with triple or quadruple therapy, and ideas for the clinical treatment of H. pylori and the development of new drugs.
Data availability statement
The raw data supporting the conclusions of this article will be made available by the authors, without undue reservation.
Author contributions
XJ performed the experiments, wrote the manuscript, and summarized and analyzed the data. QH and ML participated in performing the experiments. HY and ZS revised the manuscript. YC participated in the data analysis. HY designed the study. XZ and HY finally reviewed and approved the article for publication. All authors contributed to the article and approved the submitted version.
Funding
The study is supported by the National Science Foundation of China (nos. 81803910 and 81973615) and Qi-Huang Scholar Chief Scientist Program of National Administration of Traditional Chinese Medicine Leading Talents Support Program (2021).
Acknowledgments
Thanks to Hong Cheng and Jiang Li from the Department of Gastroenterology, Peking University First Hospital for providing the Helicobacter pylori strains.
Conflict of interest
The authors declare that the research was conducted in the absence of any commercial or financial relationships that could be construed as a potential conflict of interest.
Publisher’s note
All claims expressed in this article are solely those of the authors and do not necessarily represent those of their affiliated organizations, or those of the publisher, the editors and the reviewers. Any product that may be evaluated in this article, or claim that may be made by its manufacturer, is not guaranteed or endorsed by the publisher.
Supplementary material
The Supplementary material for this article can be found online at: https://www.frontiersin.org/articles/10.3389/fmicb.2022.962354/full#supplementary-material
References
Acio-Pizzarello, C. R., Acio, A. A., Choi, E. J., Bond, K., Kim, J., Kenan, A. C., et al. (2017). Determinants of the regulation of helicobacter pylori adhesins include repeat sequences in both promoter and coding regions as well as the two-component system ArsRS. J. Med. Microbiol. 66, 798–807. doi: 10.1099/jmm.0.000491
Attaran, B., Falsafi, T., and Ghorbanmehr, N. (2017). Effect of biofilm formation by clinical isolates of helicobacter pylori on the efflux-mediated resistance to commonly used antibiotics. World J. Gastroenterol. 23, 1163–1170. doi: 10.3748/wjg.v23.i7.1163
Chen, L., and Wen, Y. M. (2011). The role of bacterial biofilm in persistent infections and control strategies. Int. J. Oral Sci. 3, 66–73. doi: 10.4248/IJOS11022
Chiang, T. H., Chang, W. J., Chen, S. L., Yen, A. M., Fann, J. C., Chiu, S. Y., et al. (2021). Mass eradication of helicobacter pylori to reduce gastric cancer incidence and mortality: a long-term cohort study on Matsu Islands. Gut 70, 243–250. doi: 10.1136/gutjnl-2020-322200
Cooksley, C., Jenks, P. J., Green, A., Cockayne, A., Logan, R. P. H., and Hardie, K. R. (2003). NapA protects helicobacter pylori from oxidative stress damage, and its production is influenced by the ferric uptake regulator. J. Med. Microbiol. 52, 461–469. doi: 10.1099/jmm.0.05070-0
Dawan, J., Li, Y., Lu, F., He, X., and Ahn, J. (2022). “Role of efflux pump-mediated antibiotic resistance in quorum sensing-regulated biofilm formation by salmonella Typhimurium,” in Pathogens. ed. L. S. Young (Switzerland: Basel).
Del Pozo, J. L. (2018). Biofilm-related disease. Expert Rev. Anti-Infect. Ther. 16, 51–65. doi: 10.1080/14787210.2018.1417036
D'Elios, M. M., Amedei, A., Cappon, A., Del Prete, G., and de Bernard, M. (2007). The neutrophil-activating protein of helicobacter pylori (HP-NAP) as an immune modulating agent. FEMS Immunol. Med. Microbiol. 50, 157–164. doi: 10.1111/j.1574-695X.2007.00258.x
Doohan, D., Rezkitha, Y. A. A., Waskito, L. A., Yamaoka, Y., and Miftahussurur, M. (2021). Helicobacter pylori BabA-SabA key roles in the adherence phase: the synergic mechanism for successful colonization and disease development. Toxins 13:485. doi: 10.3390/toxins13070485
Enen, Z., Hui, Y., xiaofen, J., Qiuyue, H., Xuezhi, Z., and Yu, L. (2020). Effect of Chenopodium ambrosioides L. on biofilm formation of helicobacter pylori in vitro. Chin. J. Integr. Traditional Western Med. Digest. 40, 1241–1245. doi: 10.7661/j.cjim.20200904.334
Fischbach, L., and Evans, E. L. (2007). Meta-analysis: the effect of antibiotic resistance status on the efficacy of triple and quadruple first-line therapies for Helicobacter pylori. Aliment. Pharmacol. Ther. 26, 343–357. doi: 10.1111/j.1365-2036.2007.03386.x
Fu, H. W. (2014). Helicobacter pylori neutrophil-activating protein: from molecular pathogenesis to clinical applications. World J. Gastroenterol. 20, 5294–5301. doi: 10.3748/wjg.v20.i18.5294
Gatta, L., Vakil, N., Leandro, G., Di Mario, F., and Vaira, D. (2009). Sequential therapy or triple therapy for helicobacter pylori infection: systematic review and meta-analysis of randomized controlled trials in adults and children. Am. J. Gastroenterol. 104, 3069–3079. doi: 10.1038/ajg.2009.555
Ge, X., Cai, Y., Chen, Z., Gao, S., Geng, X., Li, Y., et al. (2018). Bifunctional enzyme SpoT is involved in biofilm formation of Helicobacter pylori with multidrug resistance by upregulating efflux pump Hp1174 (gluP). Antimicrob. Agents Chemother. 62:e00957-18. doi: 10.1128/AAC.00957-18
Geng, T., Yu, Z. S., Zhou, X. X., Liu, B., Zhang, H. H., and Li, Z. Y. (2022). Antibiotic Resistance of Helicobacter pylori Isolated from children in Chongqing, China. European Journal of Pediatrics, 2715–2722.
Gerrits, M. M., van Vliet, A. H., Kuipers, E. J., and Kusters, J. G. (2006). Helicobacter pylori and antimicrobial resistance: molecular mechanisms and clinical implications. Lancet Infect. Dis. 6, 699–709. doi: 10.1016/S1473-3099(06)70627-2
Hall, C. W., and Mah, T. F. (2017). Molecular mechanisms of biofilm-based antibiotic resistance and tolerance in pathogenic bacteria. FEMS Microbiol. Rev. 41, 276–301. doi: 10.1093/femsre/fux010
Hirata, K., Suzuki, H., Nishizawa, T., Tsugawa, H., Muraoka, H., Saito, Y., et al. (2010). Contribution of efflux pumps to clarithromycin resistance in Helicobacter pylori. J. Gastroenterol. Hepatol. 25, S75–S79. doi: 10.1111/j.1440-1746.2009.06220.x
Ho, J. J. C., Navarro, M., Sawyer, K., Elfanagely, Y., and Moss, S. F. (2022). Helicobacter pylori antibiotic resistance in the United States between 2011-2021: A systematic review and meta-analysis. Am. J. Gastroenterol. 117, 1221–1230. doi: 10.14309/ajg.0000000000001828
Høiby, N., Bjarnsholt, T., Givskov, M., Molin, S., and Ciofu, O. (2010). Antibiotic resistance of bacterial biofilms. Int. J. Antimicrob. Agents 35, 322–332. doi: 10.1016/j.ijantimicag.2009.12.011
Hong, C., Fulian, H., Jianqiu, S., Hejuan, A., and Le, X. (2016). Jinghuaweikang capsules combined with furazolidone-based triple or quadruple therapy as the rescue treatment for helicobacter pylori infection:a multicenter randomized controlled clinical trial. Natl. Med. J. China 96, 3206–3212. doi: 10.3760/cma.j.issn.0376-2491.2016.40.002
Hou, C., Yin, F., Wang, S., Zhao, A., Li, Y., and Liu, Y. (2022). Helicobacter pylori biofilm-related drug resistance and new developments in its anti-biofilm agents. Infect. Drug Resist. 15, 1561–1571. doi: 10.2147/IDR.S357473
Huang, Y. Q., Huang, G. R., Wu, M. H., Tang, H. Y., Huang, Z. S., Zhou, X. H., et al. (2015). Inhibitory effects of emodin, baicalin, schizandrin and berberine on hefA gene: treatment of helicobacter pylori-induced multidrug resistance. World J. Gastroenterol. 21, 4225–4231. doi: 10.3748/wjg.v21.i14.4225
Hui, Y., and Xuezhi, Z. (2014). Clinical research progress of Jinghua Weikang capsule in the treatment of Helicobacter pylori related upper digestive tract diseases. Chin. J. Integr. Traditional Western Med. Digest. 22, 694–698.doi: 10.3969/j.issn.1671-038X.2014.11.21
Jenks, P. J., and Edwards, D. I. (2002). Metronidazole resistance in Helicobacter pylori. Int. J. Antimicrob. Agents 19, 1–7. doi: 10.1016/S0924-8579(01)00468-X
Kinana, A. D., Vargiu, A. V., May, T., and Nikaido, H. (2016). Aminoacyl β-naphthylamides as substrates and modulators of AcrB multidrug efflux pump. Proc. Natl. Acad. Sci. U. S. A. 113, 1405–1410. doi: 10.1073/pnas.1525143113
Krzyżek, P., Grande, R., Migdał, P., Paluch, E., and Gościniak, G. (2020). “Biofilm formation as a complex result of virulence and adaptive responses of helicobacter pylori” in Pathogens (Switzerland: Basel)
Liu, W., Liu, Y., Zhang, X. Z., Li, N., and Cheng, H. (2013). In vitro bactericidal activity of Jinghua Weikang capsule and its individual herb Chenopodium ambrosioides L. against antibiotic-resistant helicobacter pylori. Chin. J. Integr. Med. 19, 54–57. doi: 10.1007/s11655-012-1248-y
Liu, Y., Wang, S., Yang, F., Chi, W., Ding, L., Liu, T., et al. (2022). Antimicrobial resistance patterns and genetic elements associated with the antibiotic resistance of helicobacter pylori strains from Shanghai. Gut pathogens 14:14. doi: 10.1186/s13099-022-00488-y
Liu, W. Z., Xie, Y., Lu, H., Cheng, H., Zeng, Z. R., Zhou, L. Y., et al. (2018). Fifth Chinese National Consensus Report on the management of helicobacter pylori infection. Helicobacter 23:e12475. doi: 10.1111/hel.12475
Matos, R., Amorim, I., Magalhães, A., Haesebrouck, F., Gärtner, F., and Reis, C. A. (2021). Adhesion of helicobacter species to the human gastric mucosa: a deep look Into Glycans role. Front. Mol. Biosci. 8:656439. doi: 10.3389/fmolb.2021.656439
Nikaido, H. (2011). Structure and mechanism of RND-type multidrug efflux pumps. Adv. Enzymol. Relat. Areas Mol. Biol. 77, 1–60. doi: 10.1002/9780470920541.ch1
Peng, C., Sang, S., Shen, X., Zhang, W., Yan, J., Chen, P., et al. (2022). In vitro anti-helicobacter pylori activity of Syzygium aromaticum and the preliminary mechanism of action. J. Ethnopharmacol. 288:114995. doi: 10.1016/j.jep.2022.114995
Poole, K. (2007). Efflux pumps as antimicrobial resistance mechanisms. Ann. Med. 39, 162–176. doi: 10.1080/07853890701195262
Rabin, N., Zheng, Y., Opoku-Temeng, C., Du, Y., Bonsu, E., and Sintim, H. O. (2015). Biofilm formation mechanisms and targets for developing antibiofilm agents. Future Med. Chem. 7, 493–512. doi: 10.4155/fmc.15.6
Rather, M. A., Gupta, K., and Mandal, M. (2021). Microbial biofilm: formation, architecture, antibiotic resistance, and control strategies. Braz. J. Microbiol. 52, 1701–1718. doi: 10.1007/s42770-021-00624-x
Ren, S., Cai, P., Liu, Y., Wang, T., Zhang, Y., Li, Q., et al. (2022). Prevalence of helicobacter pylori infection in China: a systematic review and meta-analysis. J. Gastroenterol. Hepatol. 37, 464–470. doi: 10.1111/jgh.15751
Servetas, S. L., Doster, R. S., Kim, A., Windham, I. H., Cha, J. H., Gaddy, J. A., et al. (2018). ArsRS-dependent regulation of homB contributes to helicobacter pylori biofilm formation. Front. Microbiol. 9:1497. doi: 10.3389/fmicb.2018.01497
Shen, X., Zhang, W., Peng, C., Yan, J., Chen, P., Jiang, C., et al. (2021). In vitro anti-bacterial activity and network pharmacology analysis of Sanguisorba officinalis L. against helicobacter pylori infection. Chin. Med. 16:33. doi: 10.1186/s13020-021-00442-1
Sisson, G., Jeong, J. Y., Goodwin, A., Bryden, L., Rossler, N., Lim-Morrison, S., et al. (2000). Metronidazole activation is mutagenic and causes DNA fragmentation in helicobacter pylori and in Escherichia coli containing a cloned H. pylori RdxA(+) (Nitroreductase) gene. J. Bacteriol. 182, 5091–5096. doi: 10.1128/JB.182.18.5091-5096.2000
Soto, S. M. (2013). Role of efflux pumps in the antibiotic resistance of bacteria embedded in a biofilm. Virulence 4, 223–229. doi: 10.4161/viru.23724
Tacconelli, E., Carrara, E., Savoldi, A., Harbarth, S., Mendelson, M., Monnet, D. L., et al. (2018). Discovery, research, and development of new antibiotics: the WHO priority list of antibiotic-resistant bacteria and tuberculosis. Lancet Infect. Dis. 18, 318–327. doi: 10.1016/S1473-3099(17)30753-3
Tang, M., Wei, X., Wan, X., Ding, Z., Ding, Y., and Liu, J. (2020). The role and relationship with efflux pump of biofilm formation in Klebsiella pneumoniae. Microb. Pathog. 147:104244. doi: 10.1016/j.micpath.2020.104244
Teh, X., Khosravi, Y., Lee, W. C., Leow, A. H., Loke, M. F., Vadivelu, J., et al. (2014). Functional and molecular surveillance of Helicobacter pylori antibiotic resistance in Kuala Lumpur. PLoS One 9:e101481. doi: 10.1371/journal.pone.0101481
Tian, L., Yao, Y., Yin, L., Wang, L., An, Z., Kang, L., et al. (2022). Direct detection of antibiotic resistance in Chinese helicobacter pylori clinical isolates by sequencing-based approach. J. Healthc. Eng. 2022, 1–6. doi: 10.1155/2022/6436256
Tshibangu-Kabamba, E., and Yamaoka, Y. (2021). Helicobacter pylori infection and antibiotic resistance—from biology to clinical implications. Nat. Rev. Gastroenterol. Hepatol. 18, 613–629. doi: 10.1038/s41575-021-00449-x
Tsugawa, H., Suzuki, H., Muraoka, H., Ikeda, F., Hirata, K., Matsuzaki, J., et al. (2011). Enhanced bacterial efflux system is the first step to the development of metronidazole resistance in Helicobacter pylori. Biochem. Biophys. Res. Commun. 404, 656–660. doi: 10.1016/j.bbrc.2010.12.034
Veenendaal, R. A., Woudt, S. H. S., Schoffelen, A. F., de Boer, M. G. J., van den Brink, G., Molendijk, I., et al. (2022). Important rise in antibiotic resistance rates in Helicobacter pylori in the Netherlands. Ned. Tijdschr. Geneeskd. 166:D6434.
Yang, F. L., Hassanbhai, A. M., Chen, H. Y., Huang, Z. Y., Lin, T. L., Wu, S. H., et al. (2011). Proteomannans in biofilm of helicobacter pylori ATCC 43504. Helicobacter 16, 89–98. doi: 10.1111/j.1523-5378.2010.00815.x
Ye, H., Liu, Y., Li, N., Yu, J., Cheng, H., Li, J., et al. (2015). Anti-helicobacter pylori activities of Chenopodium ambrosioides L. in vitro and in vivo. World J. Gastroenterol. 21, 4178–4183. doi: 10.3748/wjg.v21.i14.4178
Ye, L., Meng, F., Mao, X., Zhang, Y., Wang, J., Liu, Y., et al. (2020). Using next-generation sequencing to analyze helicobacter pylori clones with different levofloxacin resistances from a patient with eradication failure. Medicine 99:e20761. doi: 10.1097/MD.0000000000020761
Yonezawa, H., Osaki, T., Hojo, F., and Kamiya, S. (2019). Effect of Helicobacter pylori biofilm formation on susceptibility to amoxicillin, metronidazole and clarithromycin. Microb. Pathog. 132, 100–108. doi: 10.1016/j.micpath.2019.04.030
Zamani, M., Ebrahimtabar, F., Zamani, V., Miller, W. H., Alizadeh-Navaei, R., Shokri-Shirvani, J., et al. (2018). Systematic review with meta-analysis: the worldwide prevalence of helicobacter pylori infection. Aliment. Pharmacol. Ther. 47, 868–876. doi: 10.1111/apt.14561
Zanotti, G., and Cendron, L. (2019). Structural aspects of helicobacter pylori antibiotic resistance. Adv. Exp. Med. Biol. 1149, 227–241. doi: 10.1007/5584_2019_368
Zhang, Z., Liu, Z. Q., Zheng, P. Y., Tang, F. A., and Yang, P. C. (2010). Influence of efflux pump inhibitors on the multidrug resistance of helicobacter pylori. World J. Gastroenterol. 16, 1279–1284. doi: 10.3748/wjg.v16.i10.1279
Zhao, Y., Cai, Y., Chen, Z., Li, H., Xu, Z., Li, W., et al. (2021). SpoT-mediated NapA upregulation promotes oxidative stress-induced helicobacter pylori biofilm formation and confers multidrug resistance. Antimicrob. Agents Chemother. 65. doi: 10.1128/AAC.00152-21
Zhong, Y., Tang, L., Deng, Q., Jing, L., Zhang, J., Zhang, Y., et al. (2021). Unraveling the novel effect of patchouli alcohol against the antibiotic resistance of Helicobacter pylori. Front. Microbiol. 12:674560. doi: 10.3389/fmicb.2021.674560
Keywords: Helicobacter pylori, antibiotic resistance, metronidazole, biofilm, efflux pump, adhesion, Jinghua Weikang capsule
Citation: Jia X, Huang Q, Lin M, Chu Y, Shi Z, Zhang X and Ye H (2022) Revealing the novel effect of Jinghua Weikang capsule against the antibiotic resistance of Helicobacter pylori. Front. Microbiol. 13:962354. doi: 10.3389/fmicb.2022.962354
Edited by:
Maria Teresa Mascellino, Sapienza University of Rome, ItalyReviewed by:
Abbas Yadegar, Shahid Beheshti University of Medical Sciences, IranXia Ding, Beijing University of Chinese Medicine, China
Wei Wei, Wangjing Hospital, China Academy of Chinese Medical Sciences, China
Copyright © 2022 Jia, Huang, Lin, Chu, Shi, Zhang and Ye. This is an open-access article distributed under the terms of the Creative Commons Attribution License (CC BY). The use, distribution or reproduction in other forums is permitted, provided the original author(s) and the copyright owner(s) are credited and that the original publication in this journal is cited, in accordance with accepted academic practice. No use, distribution or reproduction is permitted which does not comply with these terms.
*Correspondence: Xuezhi Zhang, emhhbmcueHVlemhpQDI2My5uZXQ=; Hui Ye, YnJpZ2h0bGVhZjcyM0AxNjMuY29t