- College of Veterinary Medicine, Sichuan Agriculture University, Chengdu, China
Methicillin-resistant Staphylococcus aureus (MRSA) is among the common drug resistant bacteria, which has gained worldwide attention due to its high drug resistance and infection rates. Biofilms produced by S. aureus are known to increase antibiotic resistance, making the treatment of S. aureus infections even more challenging. Hence, inhibition of biofilm formation has become an alternative strategy for controlling persistent infections. In this study, we evaluated the efficacy of geraniol as a treatment for MRSA biofilm infection. The results of crystal violet staining indicated that 256 μg/mL concentration of geraniol inhibited USA300 biofilm formation by 86.13% and removed mature biofilms by 49.87%. Geraniol exerted its anti-biofilm effect by influencing the major components of the MRSA biofilm structure. We found that geraniol inhibited the synthesis of major virulence factors, including staphyloxanthin and autolysins. The colony count revealed that geraniol inhibited staphyloxanthin and sensitized USA300 cells to hydrogen peroxide. Interestingly, geraniol not only reduced the release of extracellular nucleic acids (eDNA) but also inhibited cell autolysis. Real-time polymerase chain reaction data revealed the downregulation of genes involved in biofilm formation, which verified the results of the phenotypic analysis. Geraniol increased the effect of vancomycin in eliminating USA300 biofilms in a mouse infection model. Our findings revealed that geraniol effectively inhibits biofilm formation in vitro. Furthermore, in combination with vancomycin, geraniol can reduce the biofilm adhesion to the implant in mice. This suggests the potential of geraniol as an anti-MRSA biofilm drug and can provide a solution for the clinical treatment of biofilm infection.
Introduction
Staphylococcus aureus is a common gram-positive bacterium that remains the main cause of healthcare-associated infections (Tenover et al., 2006). Due to the unreasonable use of antibiotics, many antibiotic-resistant strains have been found (Catalano et al., 2022). Methicillin-resistant Staphylococcus aureus (MRSA) is a well-known drug-resistant bacterium. In adults, MRSA is the main pathogen that causes bacteremia, pneumonia, and endocarditis (Turner et al., 2019). To date, vancomycin has always been the drug of choice for the treatment of MRSA infections and the last line of defense against MRSA. Unfortunately, vancomycin-resistant strains have been isolated since the beginning of the twenty-first century, worsening the current situation (Cong et al., 2020). Therefore, finding novel drugs that exhibit antibacterial activity but do not cause antibiotic resistance has attracted increasing attention worldwide.
Numerous infections caused by MRSA are closely related to biofilms. The biofilm structure prevents the complete eradication of pathogenic bacteria using antibiotics. MRSA biofilms mainly comprise polysaccharide intercellular adhesin (PIA), extracellular nucleic acids (eDNA), and proteins called extracellular polymeric substances (EPS). EPS provide protection against bacteria wrapped in biofilms, including evading the immune system and avoiding exposure to antibiotics (Sharma et al., 2019). Biofilm formation is an extremely complex and closely regulated process. SarA, a well-known S. aureus regulatory system, regulates the expression of many important virulence factors and induces S. aureus to produce biofilms, mainly by inhibiting the secretion of proteases (Trotonda et al., 2005; Abdelhady et al., 2014). It has been reported that not only is the biofilm-forming ability of sarA mutants seriously damaged but it also leads to changes in the expression levels of at least 120 genes (Mauro et al., 2016; Oriol et al., 2021). Numerous natural compounds, including thymol, carvacrol, and hesperidin, have been found to combat MRSA biofilms by targeting SarA (Selvaraj et al., 2020; Yuan et al., 2020; Vijayakumar et al., 2022). Taken together, these results indicated that sarA is a promising anti-biofilm target.
Geraniol is a terpenoid compound that is well known as the main component of many essential oils (Xu et al., 2022). Geraniol is found mainly in aromatic herbaceous plants and is cost-effective and easy to produce (Prasad and Muralidhara, 2017; Younis et al., 2021). In previous studies, geraniol was found to have anti-neurotoxicity properties (Lin et al., 2021), inhibit gastric cancer cell proliferation (Yang et al., 2021), and relieve arthritis (Wu et al., 2020). In recent years, geraniol has been proven to have significant antibacterial activity against gram-negative bacteria, such as Escherichia coli and Salmonella Typhimurium, (Friedman et al., 2002), and Streptococcus pneumoniae and S. aureus (Inouye et al., 2001). It has also been reported that geraniol can inhibit the growth of MRSA and relieve the symptoms of systemic infection caused by MRSA in mice (Lin et al., 2021). Furthermore, geraniol inhibits biofilm production by a variety of bacteria (Gupta et al., 2021; Kwiatkowski et al., 2022; Yu et al., 2022). However, the inhibitory effect and mechanism of action of geraniol on MRSA biofilms have rarely been reported. This study shows that geraniol inhibits the formation of MRSA biofilms by regulating the expression of the sarA system regulatory genes, genes related to virulence factors, and other genes that regulate biofilm formation. Vancomycin is clinically used in the treatment of MRSA infections. However, it causes nephrotoxicity and large doses may cause bacterial drug resistance. In an aim to establish the efficacy of geraniol in combination with vancomycin and reduce the dose of vancomycin, we conducted the current study.
Results
Geraniol has an effect on the growth of USA300
Geraniol exhibited antibacterial activity against USA300 with minimum inhibitory concentration (MIC) and minimum bactericidal concentration values of 512 and 1,024 μg/mL, respectively. The MIC optical density values at 600 nm (OD600 nm) are shown in Supplementary Table 1. The growth curve (Figure 1A and Supplementary Table 2) showed that geraniol did not inhibit the growth of USA300 at concentrations of 0, 16, 32, 64, 128, and 256 μg/mL.
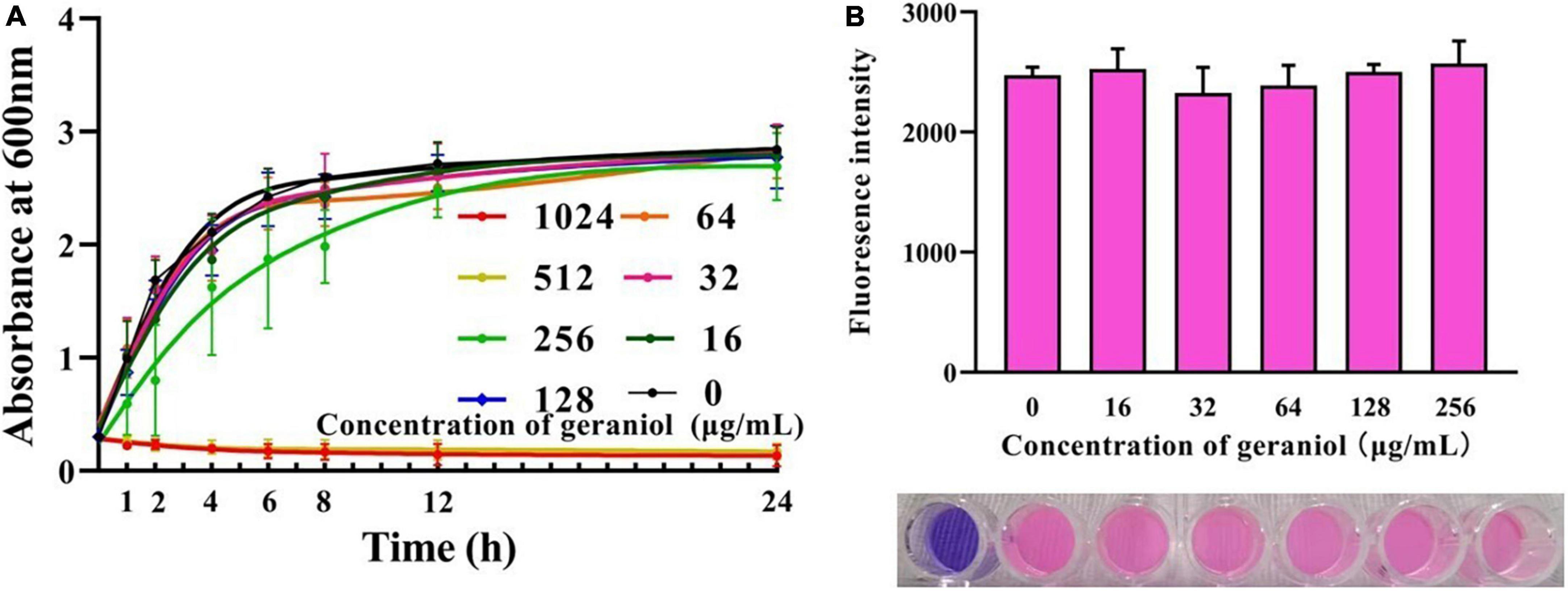
Figure 1. Effects of geraniol at different concentrations on the activity of USA300 assessed by the (A) growth curve assay and (B) Alamar blue analysis.
Biofilm formation inhibition effects of geraniol against USA300 and several other Staphylococcus aureus strains
Biofilm experiments were performed at sub-inhibitory concentrations of geraniol. The results of the Alamar Blue test (Figure 1B) showed that there was a negligible difference in the fluorescence intensity between the drug treatment group and untreated group, indicating that the activity of USA300 was hardly affected by the sub-MICs of geraniol. Geraniol significantly inhibited biofilm formation induced by USA300. When the concentration of geraniol was 128 and 256 μg/mL, biofilm formation decreased by 70.29 ± 7.58% and 86.13 ± 5.22%, respectively (Figure 2A). Figure 2B shows that the slides containing samples from the untreated group were densely covered with cells. However, as the geraniol concentration increased, the number of cells decreased, and PIAs had exuded. Only a small number of unaggregated cells was observed in the group treated with 256 μg/mL geraniol. These results indicated that the sub-MICs of geraniol (16, 32, 64, 128, and 256 μg/mL) significantly inhibited USA300 biofilm formation in a dose-dependent manner. In addition to USA300, the effect of geraniol on biofilm formation of several other S. aureus strains was also evaluated (Supplementary Figure 1). The biofilm inhibition rate of geraniol on these strains was more than 50%. The MIC of geraniol on these strains is shown in Supplementary Table 3.
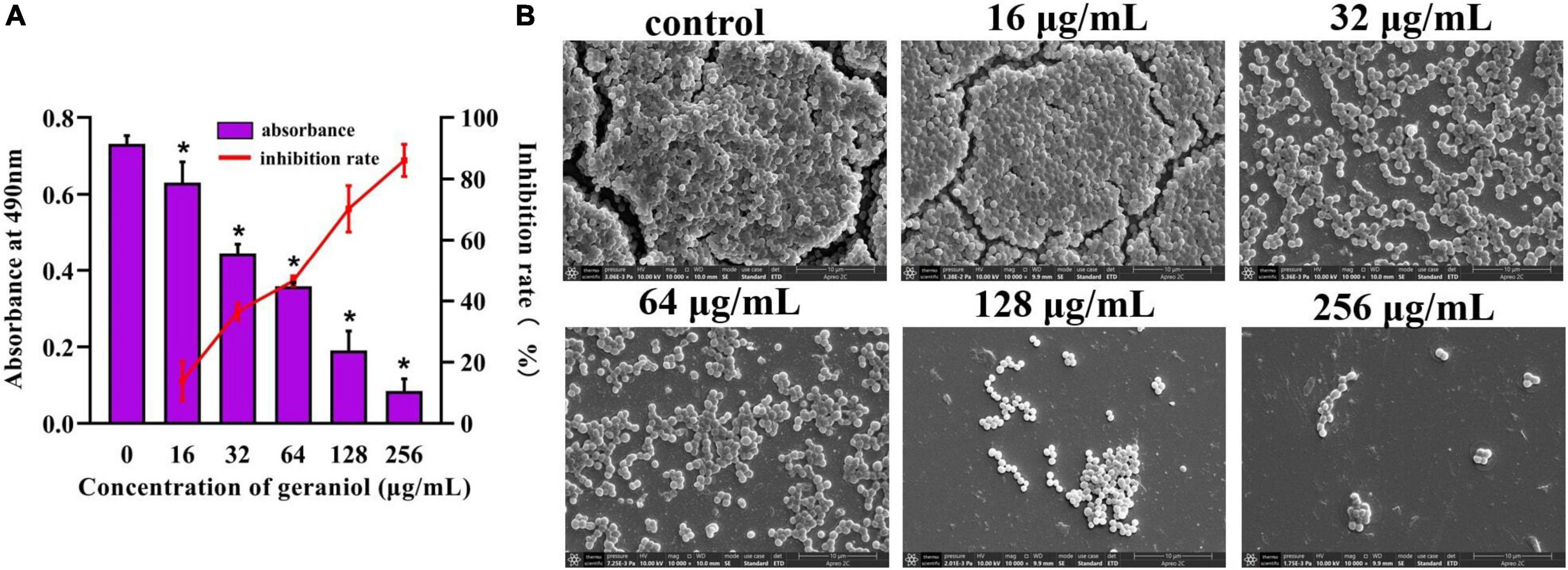
Figure 2. Effect of geraniol with sub-minimum inhibitory concentrations on the formation of USA300 biofilms assessed by (A) crystal violet staining and (B) scanning electron microscopy images at 10,000 × magnification. *p < 0.05 compared with the 0 μg/mL group.
Geraniol removed preformed USA300 biofilms and several other Staphylococcus aureus strains
The biofilm removal assay revealed that geraniol, at concentrations of 256 μg/mL, effectively disrupted preformed biofilms, decreasing them by 49.87 ± 5.11% (Figure 3A). The scanning electron microscopy (SEM) observations (Figure 3B) showed that the slides without geraniol treatment were completely covered by bacteria and the bacterial cells were closely arranged like pavers blocks. As the concentration increased, bacteria gradually decreased, and the distribution of bacterial cells became scattered. Geraniol destroyed the structure of the biofilm. Geraniol also had different scavenging effects on preformed biofilms of other S. aureus strains. However, the effect on 26FS31, YFC18 and 2ZG3 clinical strains was not significant (Supplementary Figure 2).
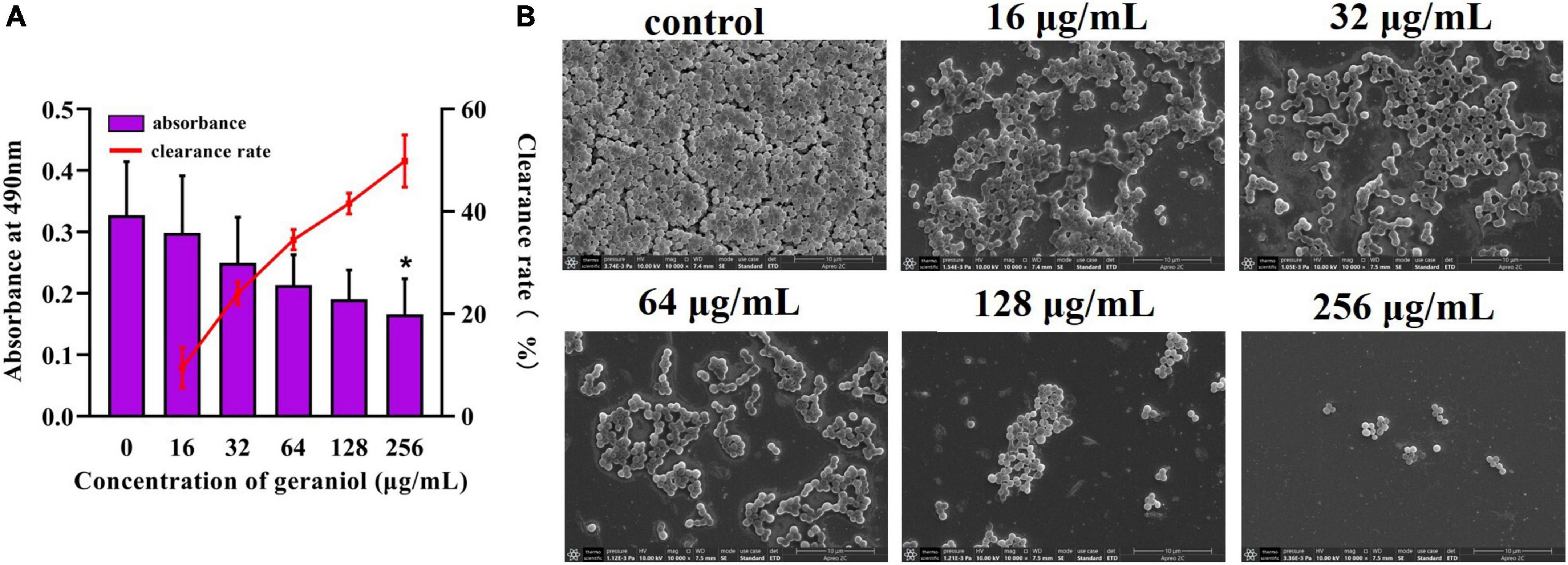
Figure 3. Effect of geraniol with sub-minimum inhibitory concentrations removing on preformed biofilms of USA300. (A) Crystal violet quantification and (B) scanning electron microscopy images at 10,000 × magnification. *p < 0.05 compared with the 0 μg/mL group.
Qualitative analysis of polysaccharide intercellular adhesin production
In the Congo Red (CR) plate, the production of PIA was indicated by the number of black colonies, with the PIA-positive bacterial strain being completely black. The results presented in Figure 4 show that as the geraniol concentration increased, the PIA synthesis gradually inhibited (observed as reduced color intensity of the black colonies). This suggests that geraniol inhibited USA300 biofilms by reducing the synthesis of PIA.
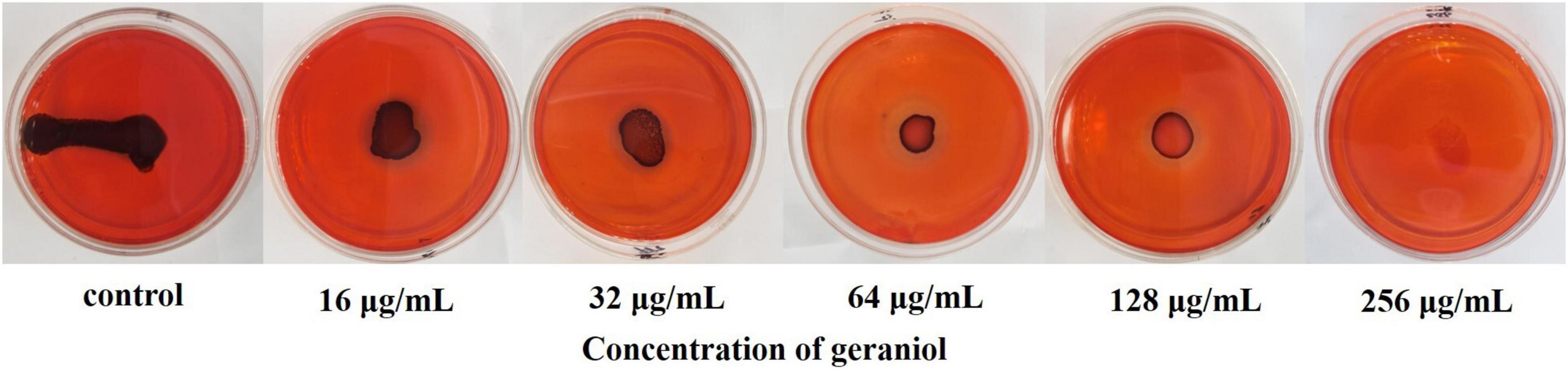
Figure 4. Qualitative analysis of the polysaccharide intercellular adhesin of USA300 upon geraniol treatment.
Qualitative analysis of extracellular DNA release
The amount of eDNA released by USA300 cells was evaluated in the absence and presence of geraniol. As shown in Figure 5A, at low concentrations of geraniol, the release of eDNA from MRSA biofilms was significantly inhibited. Compared to that in the untreated group, eDNA release was decayed by 42.50 ± 1.57% and 57.10 ± 4.59% after treatment with 128 and 256 mg/mL geraniol, respectively.
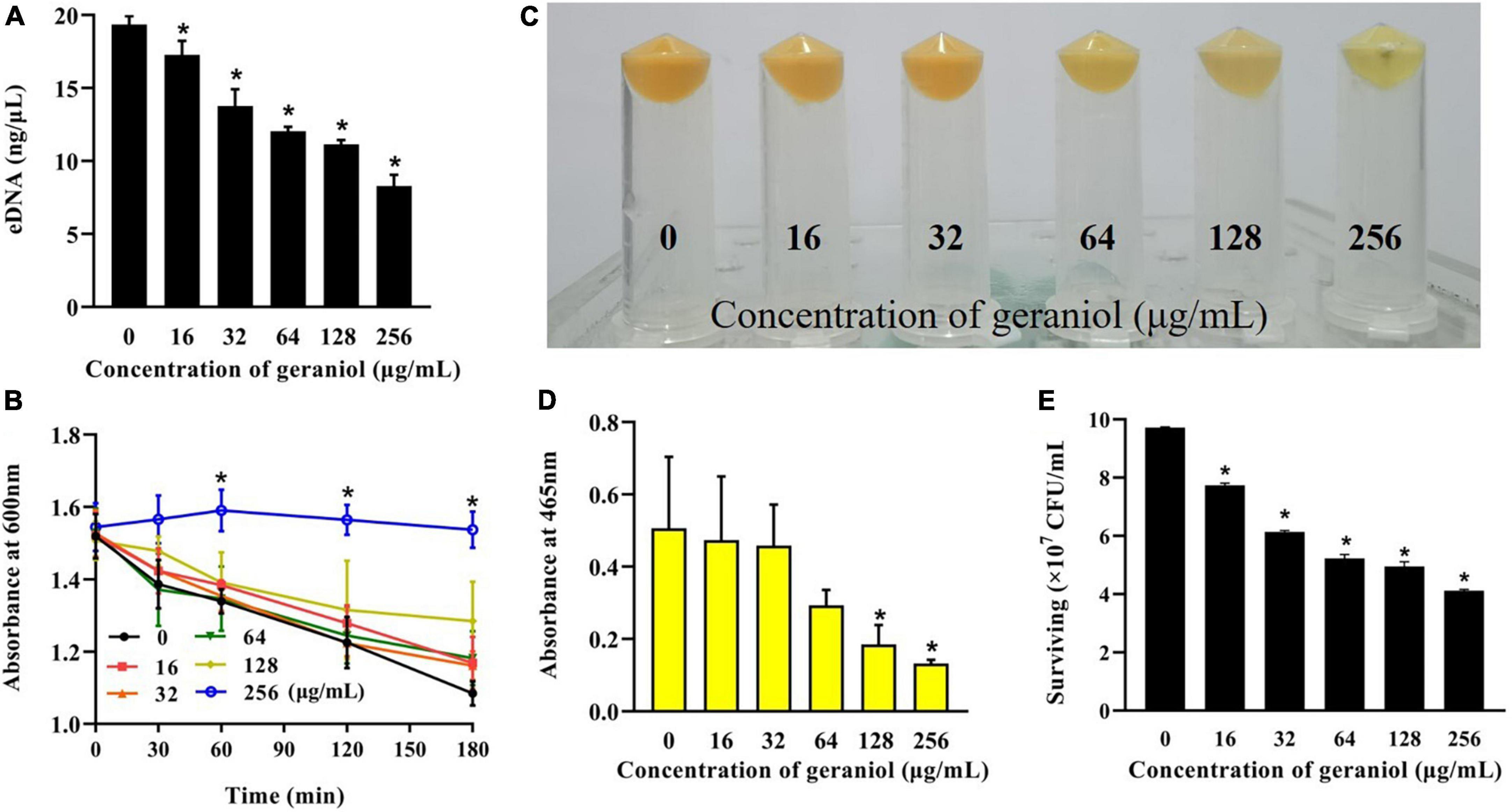
Figure 5. Inhibitory effects of geraniol on the release of extracellular nucleic acids and staphyloxanthin biosynthesis of USA300. (A) eDNA release, (B) autolysis, (C) cell color, (D) qualitative analysis of staphyloxanthin, and (E) effect of geraniol treatment on the survival of USA300 in H2O2. *p < 0.05 compared with the 0 μg/mL group.
Cell autolysis assay
Autolysis of USA300 with 128 μg/mL geraniol was negligible in the first 30 min, whereas autolysis of USA300 treated with 256 μg/mL geraniol was effectively inhibited (Figure 5B).
Geraniol inhibited the synthesis of staphyloxanthin and sensitized USA300 to H2O2
In geraniol-treated cells, the color of the bacteria turned pale (Figure 5C), and staphyloxanthin production (Figure 5D) was inhibited up to 70.70 ± 12.34%, with this inhibition being concentration-dependent. As shown in Figure 5E, the sensitivity of USA300 to H2O2 increased significantly in the 256 μg/mL geraniol-treated group (the number of live bacteria was 4.12 × 107 CFU/mL) compared with the untreated group (the number of live bacteria was 9.72 × 107 CFU/mL).
Effect of geraniol on the expression of genes
The results of the quantitative polymerase chain reaction (qPCR) (Figure 6) showed the effect of 256 μg/mL geraniol on the genes involved in biofilm formation and virulence factor production in USA300. Geraniol downregulated the expression of sarA, fnbA, fnbB, clfA, icaA, icaB, atlA, and crtM.
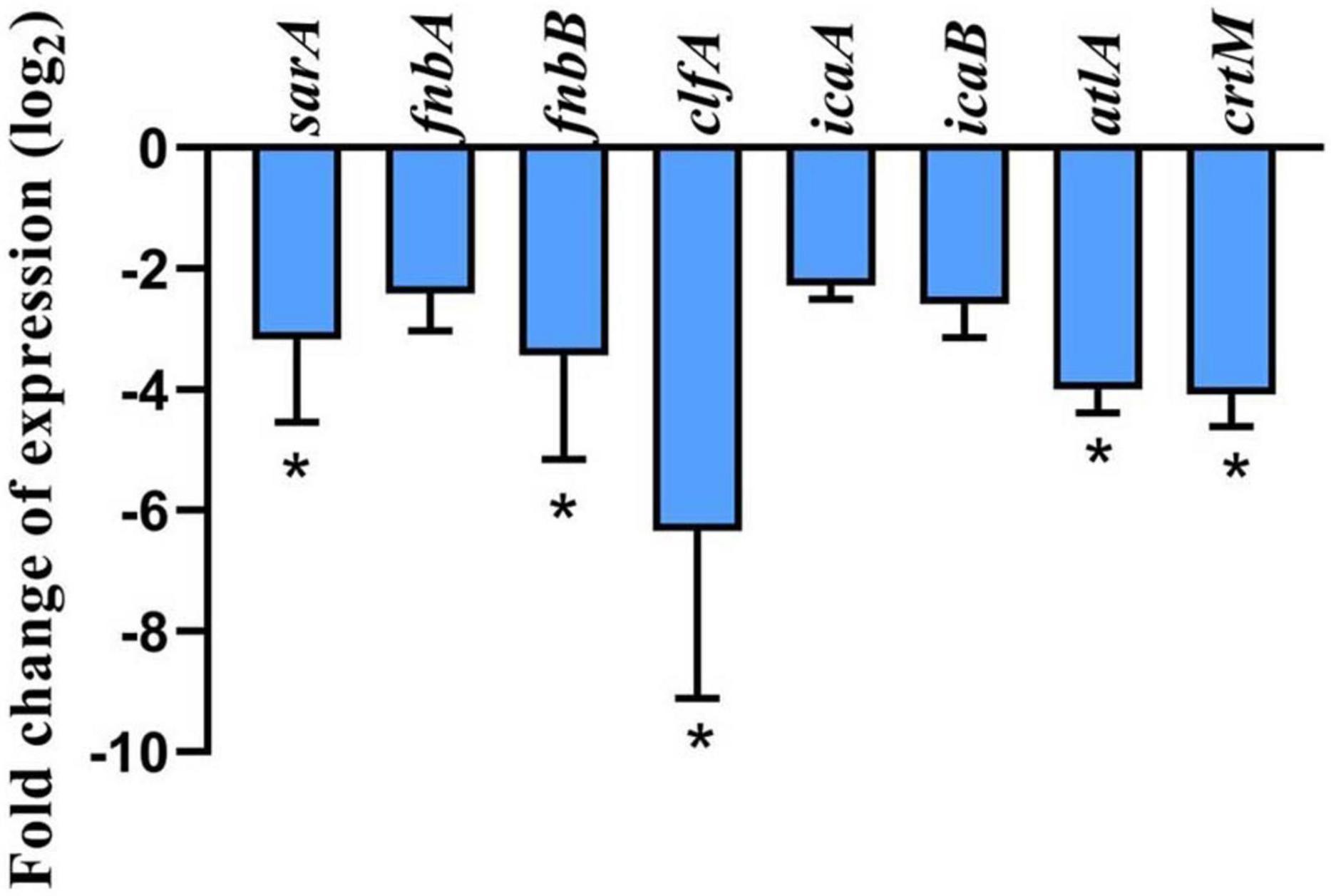
Figure 6. Effect of geraniol at 256 μg/mL concentration (half of the minimum inhibitory concentration) on the expression of genes involved in biofilm formation and related virulence factors of USA300. *p < 0.05 compared with the 0 μg/mL group.
Vancomycin combined with geraniol reduced intraperitoneal foreign-body biofilm infection caused by methicillin-resistant Staphylococcus aureus in mice
Vancomycin combination with geraniol removed preformed biofilms
The microscopic analysis showed that biofilms were removed to varying degrees. As shown in Figure 7, in the physiological saline group, the implant was tightly covered with dense biofilm bacteria, and many bacteria adhered to each other in clumps. A reticular structure was observed between the bacteria. In the high-dose combination group, the preformed biofilms were almost completely removed, and only a few bacteria were observed. A completely disrupted biofilm architecture was observed, with reticula-connecting bacteria that had already been destroyed.
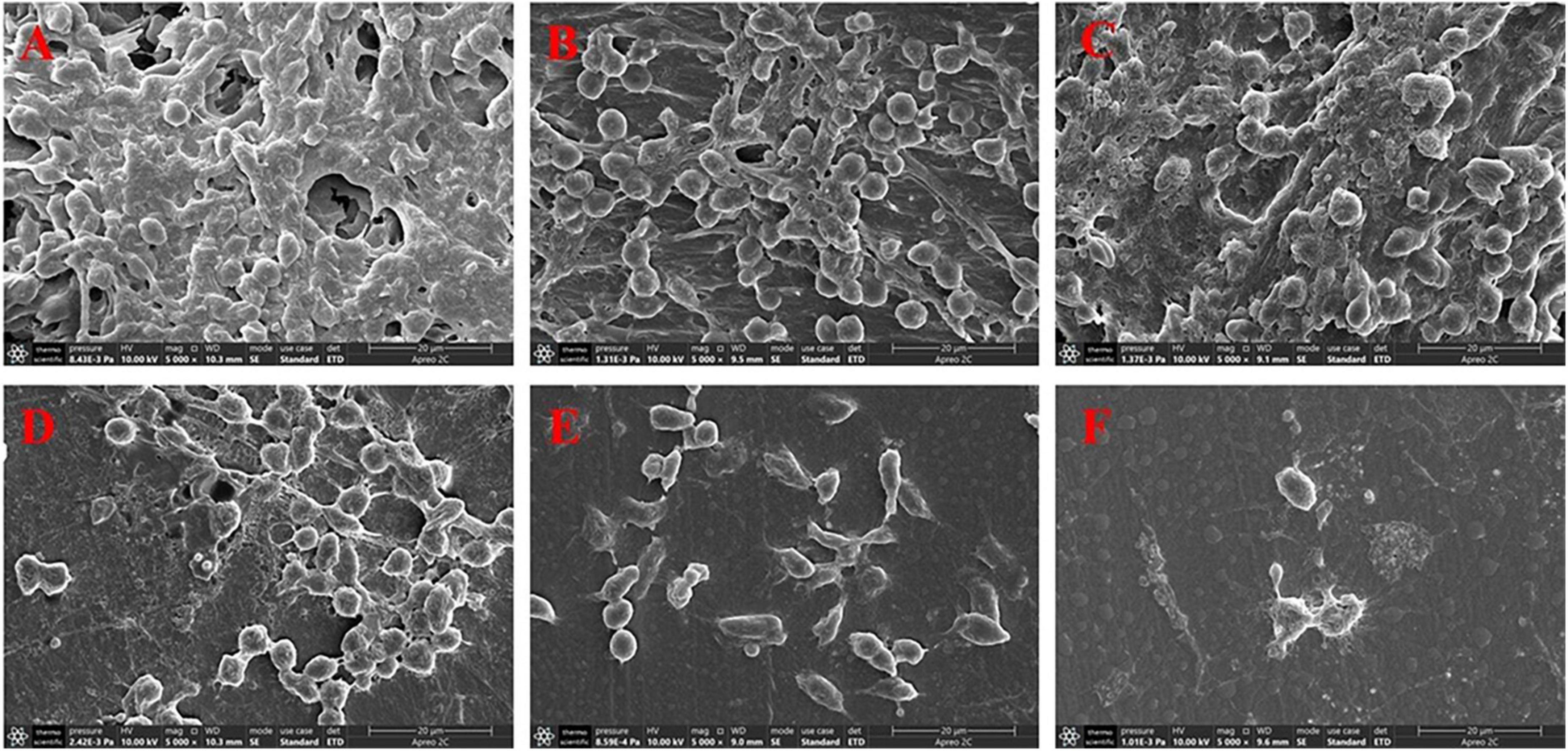
Figure 7. Scanning electron microscopy images at 5,000 × magnification of biofilms in peritoneal implants from mice. The doses of the drug were (A) physiological saline, (B) 40 mg/kg geraniol, (C) 40 mg/kg vancomycin, (D) 10 mg/kg geraniol + 40 mg/kg vancomycin, (E) 20 mg/kg geraniol + 40 mg/kg vancomycin, and (F) 40 mg/kg geraniol + 40 mg/kg vancomycin.
Vancomycin combined with geraniol reduced bacterial adhesion
The bacterial burden on the implants was calculated using colony counting. The implants treated with a combination of geraniol (40 mg/kg) and vancomycin (40 mg/kg), which showed the most significant effect, exhibited minimal bacterial adhesion, and bacterial counts (Figure 8A) were lower than those observed in the physiological saline group (99.94% ± 0.06%).
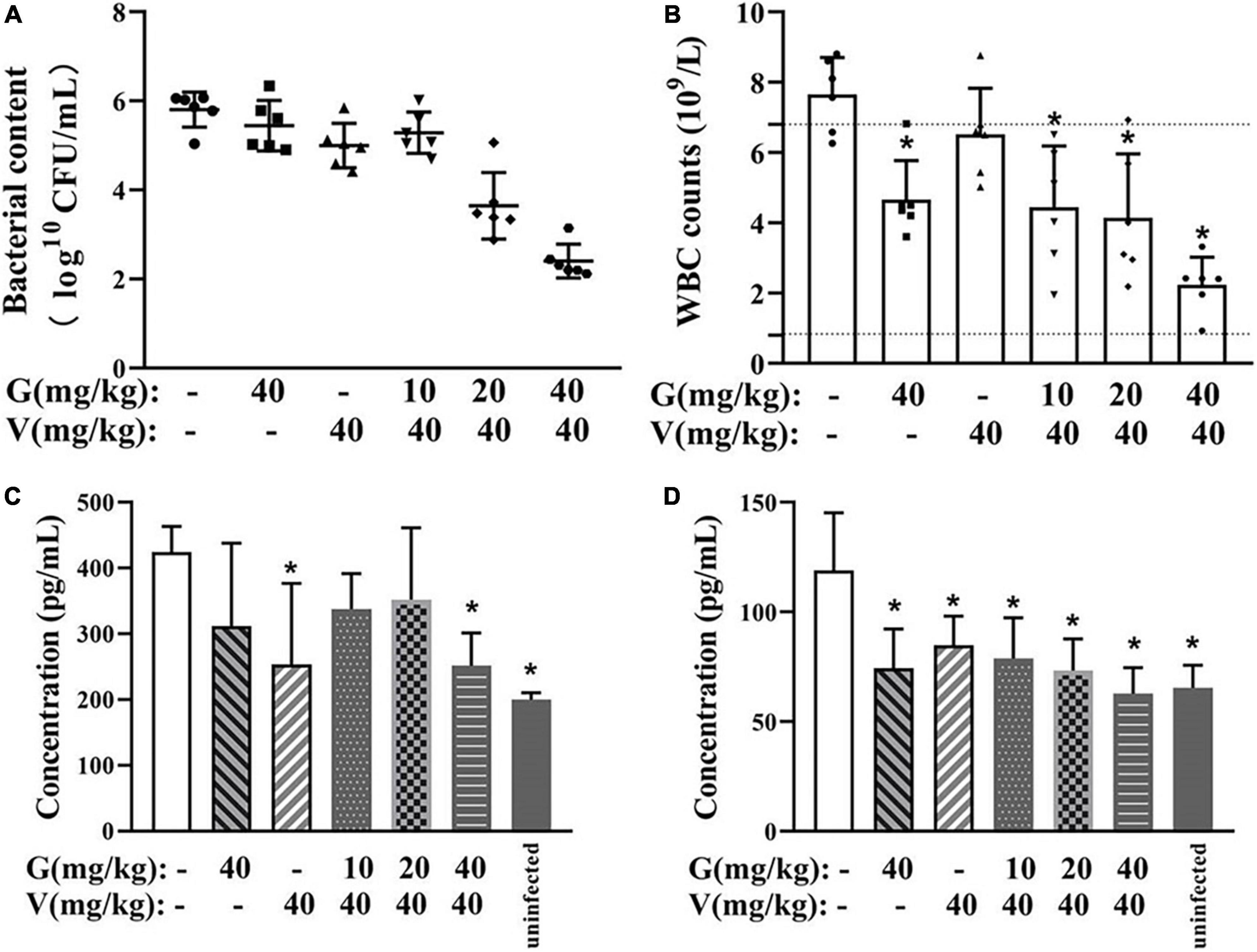
Figure 8. Therapeutic effect of combinations of vancomycin and/or geraniol on the mice infection model. (A) Bacterial count in implants, (B) white blood cell counts in mice, serum (C) TNF-α and (D) IL-6 levels in model mice after treatment. *p < 0.05 compared with the 0 μg/mL group.
Vancomycin combined with geraniol reduced the inflammatory responses in mice
In all treatment groups, the white blood cell (WBC) count decreased to a normal level, and the levels of TNF-α and IL-6 were inhibited. Particularly, compared with the physiological saline group, the WBC count in the high-dose group (geraniol 40 mg/kg + vancomycin 40 mg/kg) decreased by 70.11% ± 10.70% (Figure 8B), and the levels of TNF-α and IL-6 were significantly lower by 46.33 ± 7.45% and 41.01 ± 7.90%, respectively (Figures 8C,D).
Discussion
The limitation of antibiotic resistance caused by drug-resistant bacteria, such as MRSA and vancomycin-resistant S. aureus, has raised widespread concern worldwide. MRSA can cause chronic nosocomial infections and adhere to medical devices, especially implantable medical devices, by forming biofilms. Therefore, the demand for compound-targeted biofilms has increased in recent years. Anti-biofilm is a promising strategy for the treatment of MRSA-induced infections (Roy et al., 2018). Several natural compounds, such as limonene, andrographolide sulfonate, and luteolin, have been found to inhibit biofilm formation (Zhang et al., 2021; Gambino et al., 2022; Yuan et al., 2022). In this study, the growth curves and Alamar Blue assay indicated that the tested concentration of geraniol did not affect the activity of bacteria. Indicating that the drug concentration will not have an effect on subsequent biofilm formation. This property is not easy to cause drug resistance in bacteria (Roy et al., 2018). Moreover, we found that geraniol has a transcendent activity of inhibiting MRSA biofilm formation and has a superior mature biofilms elimination effect. Furthermore, to evaluate the inhibitory activity of geraniol on biofilm as comprehensively as possible, we observed the effect of geraniol on biofilm formation of methicillin-sensitive S. aureus (MSSA) and several other MRSA strains in vitro. Geraniol also has an apparent biofilm formation effect on these strains, which indicates that geraniol has a wide range of effects.
The PIA produced by S. aureus is an important contributor to biofilm formation. PIA contributes to stabilizing the interaction between bacterial cells and the adhesion of S. aureus to medical devices (Schilcher and Horswill, 2020). Nguyen et al. (2020) reported that wild-type strains cause more severe infections and show higher survival rates than PIA mutant strains in infected mouse models. Overall, determining the effect of geraniol on PIA production is important for exploring the potential mechanisms of geraniol. Our findings indicated that geraniol reduced PIA production in USA300 cells, as qualitatively evaluated using the CR plate method. PIA biosynthesis is regulated by the icaADBC operon. icaA encodes the main PIA synthesis, icaB is critical to the PIA adhesion function because it introduces a positive charge into the PIA via deacetylation, allowing the bacteria to adhere stably to surfaces (Nuryastuti and Krom, 2017; Lerch et al., 2019; Schilcher and Horswill, 2020). However, icaA cannot be expressed without sarA, since the promoter of the ica operon requires binding to sarA (Tamber and Cheung, 2009). sarA is an anti-biofilm target that has been discovered recently. It was reported that for individual strains, ica is not necessary for biofilm formation (Trotonda et al., 2005; Dengler Haunreiter et al., 2019). However, in ica-independent strains, sarA regulates biofilm formation in other ways. For instance, sarA positively regulates fnbA, fnbB, and clfA (determinants of S. aureus surface adhesins) (Pantrangi et al., 2015; Swarupa et al., 2018). Therefore, we selected several core genes for this study and found that they were significantly downregulated under the influence of geraniol. This is in agreement with phenotypic experiments (Zhang et al., 2021; Yehia et al., 2022).
In addition to PIA, eDNA is another major constituent of MRSA biofilms. The addition of DNase I (an enzyme that degrades eDNA) significantly reduces the formation of biofilms and facilitates its eradication (Nagasawa et al., 2017; Soler-Arango et al., 2019). The amount of eDNA released in this study (Figure 5A) supported the biofilm results, that is, geraniol inhibited the formation of biofilms and decreased the release of eDNA. Similar to apoptosis, S. aureus undergoes a cleavage process regulated by conserved genes, which is called autolysis (Rice et al., 2007). Autolysin is encoded by atlA and is involved in bacterial cell wall homeostasis and peptidoglycan conversion. Previous studies have shown that the release of eDNA by S. aureus is mediated by autolysis and biofilm formation (Rice et al., 2007; Houston et al., 2011). When the expression level of atlA was downregulated, the biofilm formation ability of S. aureus decreased sharply (Biswas et al., 2006). Therefore, we studied the autolysis of USA300 cells treated with geraniol, and found that geraniol significantly inhibited cell autolysis. This is consistent with the results of previous studies (Selvaraj et al., 2019; Valliammai et al., 2019) and suggests that geraniol reduces the release of eDNA by inhibiting cell autolysis. The gene expression level also confirmed that geraniol downregulated atlA gene. Hence, geraniol affected the USA300 biofilm architecture by modulating the expression of atlA.
Notably, geraniol changed the color of USA300 in this experiment. We speculate that this was associated with staphyloxanthin biosynthesis. Staphyloxanthin is the main component of the bacterial pigment that provides S. aureus its unique yellow or orange appearance. Staphyloxanthin protects S. aureus from escaping the immune system and from the bactericidal effect of oxides, which promotes bacterial survival (Liu and Nizet, 2009). This is due to the fact that the alternate bonds in the staphyloxanthin structure can absorb excess energy from reactive oxygen species (El-Agamey et al., 2004). Previous studies have shown that mutants encoding staphyloxanthin synthase CrtM not only cause S. aureus to lose its yellow appearance but are also more likely to be killed by peroxides in whole blood (Clauditz et al., 2006). The results of the lethal analysis and qPCR showed that geraniol could improve the sensitivity of USA300 to H2O2 and downregulate crtM gene expression, further verifying the results of phenotypic experiments. These results suggest that geraniol may be beneficial in the clinical treatment of MRSA.
Despite many preventive measures, medical device infections caused by biofilms still occur often, particularly implanted device infections. It is especially important to evaluate the biofilm scavenging activity of drugs in vivo. Therefore, we explored the therapeutic effects of geraniol combined with vancomycin on foreign body infections in mice. After 24 h of treatment, the pathological damage in mice was significantly alleviated. The therapeutic effect was the most obvious in the high-dose group. Neither geraniol nor vancomycin effectively killed bacteria that adhered to the surface. However, the number of bacteria significantly decreased with the addition of geraniol. Based on these results, we presumed that geraniol could effectively destroy the biofilm structure in mice and increase the effect of vancomycin. Infection with S. aureus causes an increase in WBCs and a large release of proinflammatory factors. In this study, the WBC count and the release of TNF-α and IL-6 in the dosing group were downregulated. Due to the high bacteriostatic concentration of geraniol (MIC = 512 μg/mL), we speculate that the anti-inflammatory effect of geraniol alleviates inflammatory symptoms in mice (Li et al., 2018; Toiu et al., 2019; Yuan et al., 2020). Owing to vancomycin’s non-negligible toxicity, the dosage of vancomycin should not be increased in clinical practice. The in vivo results indicated that the combination of geraniol and vancomycin could effectively treat biofilm infection, which is critical for the treatment of MRSA biofilms.
In summary, geraniol not only effectively prevented MRSA biofilm formation but also removed mature MRSA biofilms. Geraniol inhibited the secretion of PIA and released eDNA, mainly by inhibiting the gene expression of sarA and atlA. Furthermore, geraniol reduced staphyloxanthin production by downregulating crtM, thereby increasing the sensitivity of MRSA to peroxides. In this study, the ability of geraniol in combination with vancomycin to remove biofilms in vivo was also evaluated. Although geraniol alone did not significantly remove the biofilm from the implant in mice, it is speculated that geraniol improved the therapeutic effect of vancomycin by destroying the structure of the biofilms, relieving inflammatory symptoms. In conclusion, geraniol is a potential drug for treating MRSA biofilms. However, the pharmacodynamics, pharmacokinetics, and toxicity of geraniol require further exploration.
Materials and methods
Bacterial strain and drug reagent
The MRSA strain USA300 (ATCC® BAA-1717™) [obtained from the American Type Culture Collection (ATCC)] was used in the current study and was cultivated in brain heart infusion (BHI) broth (Hopebio, Qingdao, China). The bacteria were cultured in an air bath constant temperature oscillator (BS-2F, Ningbo Jingda Formal Equipment Co., Ltd., Jintan, China) at a temperature of 37°C and a rotational speed of 150–200 rpm. Geraniol (>98% HPLC purity; CAS No. 106-24-1) was purchased from Shanghai McLean Biochemical Technology Co., Ltd. (Shanghai, China) and dissolved in dimethyl sulfoxide to obtain a stock solution. Vancomycin was purchased from Beijing Solarbio Science and Technology Co., Ltd. MSSA (ATCC® 25923, ATCC® 29213) were obtained from the ATCC. MRSA (ATCC® 43300, PHY6, 26FS18, C2Y, 2ZG3, YFC31) were presented by Professor Yanhua Li, School of Animal Medicine, Northeast Agricultural University.
Ethics statement
All animal studies were performed in accordance with the approved experimental practices and standards of the Animal Ethics Committee of Sichuan Agricultural University (Chengdu, China), and the experimental protocols were approved and conducted under the supervision of the Animal Care Committee (permitnumberDKY-B2020203001; date of approval: June 11, 2021).
Effect of geraniol on the activity of USA300
Susceptibility testing and growth curve assay
The MIC of geraniol for S. aureus strains was determined using the double dilution method according to the Clinical and Laboratory Standards Institute (Arendrup et al., 2017). For USA300, after the bacteria were static cultured at 37°C for 18 h, the OD600 nm of each bacteria group was determined. In the determination of the growth curve according to Yuan’s experimental method (Yuan et al., 2019), the OD600 nm was measured at different time points, which indicated the growth of USA300 (at 37°C and 200 rpm shaking speed) co-cultured with different concentrations (0, 16, 32, 64, 128, 256, 512, and 1,024 μg/mL) of geraniol. An appropriate amount of DMSO was used instead of geraniol as the control group in all experiments.
Alamar blue assay
Cell viability was evaluated using the Alamar Blue (Solaibao, Beijing, China) assay according to Khan’s experimental method (Khan et al., 2022). Briefly, Alamar Blue reagent was mixed with USA300 cells treated with sub-MIC (0, 16, 32, 64, 128, and 256 μg/mL) of geraniol. Samples were incubated in opaque 96-well plates (BKMAM BIOTECHNOLOGY Co., Ltd., Hunan, China) for 30 min at 37°C, before the fluorescence intensity was determined.
Effect on biofilm formation
The effect of geraniol on the formation of methicillin-resistant Staphylococcus aureus biofilms
Referring to the method of Peng et al. (2020), MSSA and MRSA strains cells (treated with sub-MICs of geraniol or appropriate concentration of DMSO) were cultured in 96-well plates (BKMAM BIOTECHNOLOGY Co., Ltd., Hunan, China) under anaerobic condition at 37°C for 18 h, and the mature biofilm was gently washed with non-heat source phosphate-buffered saline (PBS) to remove planktonic bacteria. Then the cultures were fixed by formaldehyde solution for more than 8 h and mature biofilms were stained with crystal violet for 2 h. Acetic acid (290 μL/well) was added to each well to dissolve the adhered dye, and the OD490 nm was determined. SEM was used to observe morphological and structural changes in biofilms. The biofilms were cultured on slides (2 × 2 cm) using the same method as that used by Yuan et al. (2020). Finally, the biofilms were visualized using SEM (Apreo 2; Thermo Fisher Scientific Inc., Waltham, MA, United States).
Biofilm removal assay
Preformed biofilms were treated with sub-MICs of geraniol or appropriate concentration of DMSO (diluted with PBS) for 24 h at 37°C. Then, similar to the steps in the previous experiment, the supernatant was removed and the planktonic bacteria were gently washed out with non-heat source PBS once. After crystal violet staining, the OD490 nm was determined. SEM samples were prepared using the same method as previously described (Selvaraj et al., 2019).
Polysaccharide intercellular adhesin analysis
To determine the effect of geraniol PIA production, the overnight (12 h) cultured USA300 suspension was inoculated on CR agar plates containing sub-MIC geraniol, and colony color was observed after incubation at 37°C for 24 h (Freeman et al., 1989).
Extraction of extracellular DNA
USA300 was diluted to 107 CFU/mL, and fresh BHI broth (Hopebio) with sub-MICs of geraniol was added to a 6-well plate (NEST Biotechnology Co., Ltd., Zhejiang, China), co-cultured for 18 h to construct a mature biofilm, and extracted according to the Rice method (Rice et al., 2007). Briefly, the mature biofilm was re-suspended by TEN buffer and the supernatant was absorbed for centrifugation (4°C, 18,000 rpm, 5 min). The supernatant was mixed with TE buffer and mixed solution of organic reagent (phenol, chloroform, and isoamyl alcohol) and extracted. After the extract was centrifuged (4°C, 12,000 rpm, 10 min), the supernatant was re-extracted by chloroform and isoamyl alcohol. Finally, eDNA release was detected using an ultra-micro spectrophotometer (NanoDrop One, Thermo Fisher Scientific Inc., Waltham, MA, United States).
Autolysis analysis
USA300 cells were cultured overnight (12 h) in the presence of sub-MICs of geraniol. After washing thrice with PBS, the cells were resuspended in PBS containing 0.02% Triton Xmur100 (Solaibao, Beijing, China). These cells were cultured at 37°C and the OD600 nm value of the bacterial suspension was determined every 30 min (Valliammai et al., 2019).
Pigment production assay
Overnight (12 h) cultured USA300 was added to BHI broth containing sub-MICs of geraniol at 37°C and 180 rpm shaking speed for 24 h. Suspended cells were collected and extracted with methanol for more than 8 h. Thereafter, the supernatant was collected, and the OD465 nm value was determined (Yuan et al., 2020).
H2O2 killing assay
USA300 cells treated with sub-MICs of geraniol were added to PBS with H2O2 (1 mM) and incubated at 37°C for 1 h. The cells were then washed twice with PBS, diluted, evenly coated on BHI agar plates (Hopebio, Qingdao, China), cultured at 37°C for 24 h, and the colony count was performed (Valliammai et al., 2019).
Quantitative real-time PCR
Total RNA was extracted from USA300 cells grown in BHI broth to the late-logarithmic phase in the absence and presence of geraniol (256 μg/mL) using an RNA kit (Tianmo Biotech, Beijing, China) and converted to cDNA using 5X RT Mix (Beijing Biomed Gene Technology Co., Ltd., China). PCR was performed in a 20 μL reaction volume containing BlasTaq™ 2XqPCR MasterMix (abm, Vancouver, Canada) according to the manufacturer’s instructions. Real-time PCR was performed on a CFX Connect™ Real-Time PCR System (Bio-Rad Laboratories, Hercules, CA, United States) using specific primers (Table 1) designed using Primer 5.0. (PREMIER Biosoft, Canada) The levels of the target transcripts were calculated relative to those of 16S rRNA (housekeeping gene) using the 2–ΔΔCt method (Schmittgen and Livak, 2008).
Animals
Establishment of a murine intraperitoneal foreign-body biofilm infection model in murine
The murine model was constructed according to the method by Yuan et al. (2020). Briefly, a USA300 biofilm was grown on the implants (a 1 mm in length medical PVC tubes, Yongkang, Shandong, China). Then, in the sterile environment, the mice were anesthetized with pentobarbital (40 mg/kg), a small incision was made in the left groin, the implants were placed carefully, and a professional sutured the incision. The process of establishing the mouse model is shown in Supplementary Figure 3. Finally, the mice were randomly divided into four groups with eight mice in each group, and the mice were injected with 0.1 mL of the drugs (40 mg/kg geraniol, 40 mg/kg vancomycin, 10 mg/kg geraniol + 40 mg/kg vancomycin, 20 mg/kg geraniol + 40 mg/kg vancomycin, 40 mg/kg geraniol + 40 mg/kg vancomycin, and physiological saline). All drugs were injected intraperitoneally twice daily (24 h) with a 12 h interval.
Colony counting
The implants were removed from the mouse and gently rinsed with non-heat source PBS to remove the attached impurities. Thereafter, the colonies on the implants were counted (Tursi et al., 2020).
Scanning electron microscopy
The implants were removed from the mice and fixed with 2.5% glutaraldehyde (Solaibao, Beijing, China) for 24 h; then the SEM (Apreo 2; Thermo Fisher Scientific Inc., Waltham, MA, United States) was used to observe the morphology and structure of the biofilms (Inoue et al., 2017).
White blood cell counts
At 12 h after the second administration, the blood of the mice was collected, and a blood cell analyzer (BC-5120; Mindray, Shenzhen, China) was used to count the WBCs.
Enzyme-linked immunosorbent assay
Blood was collected from the mice, and the serum was prepared according to the instructions of the enzyme-linked immunosorbent assay (ELISA) kit (Jiangsu Meimian industrial Co., Ltd., Yancheng, China) for ELISA.
Statistical analysis
All in vitro experiments were performed in triplicate, and the values are presented as mean ± standard deviation. Two-tailed Student’s t-tests and analysis of variance were used to analyze statistically significant differences. GraphPad Prism 8 software was used for the analysis. Differences with p-values less than 0.05 were considered statistically significant.
Data availability statement
The raw data supporting the conclusions of this article will be made available by the authors, without undue reservation.
Ethics statement
The animal study was reviewed and approved by the Animal Ethics Committee of Sichuan Agricultural University (Chengdu, China).
Author contributions
LY and ZX conceived and designed the experiments. KG, YH, YD, CH, XL, GS, and TT performed the experiments. TT and LZ contributed to preparing the reagents, materials, and analysis tools. KG and OP wrote the manuscript. All authors have read and agreed to the published.
Funding
This study was supported by the Sichuan Science and Technology Program (Grant no. 2021YFH0156), the National Natural Science Foundation of China (Grant no. 31702284), and the Central Government Funds of Guiding Local Scientific and Technological Development for Sichuan Province (Grant no. 2021ZYD0071).
Acknowledgments
We sincerely thank Prof. Yanhua Li for providing the strains. We are grateful the Editage (www.editage.cn) for English language editing.
Conflict of interest
The authors declare that the research was conducted in the absence of any commercial or financial relationships that could be construed as a potential conflict of interest.
Publisher’s note
All claims expressed in this article are solely those of the authors and do not necessarily represent those of their affiliated organizations, or those of the publisher, the editors and the reviewers. Any product that may be evaluated in this article, or claim that may be made by its manufacturer, is not guaranteed or endorsed by the publisher.
Supplementary material
The Supplementary Material for this article can be found online at: https://www.frontiersin.org/articles/10.3389/fmicb.2022.960728/full#supplementary-material
Supplementary Figure 1 | Inhibition rate of geraniol with sub-minimum inhibitory concentrations on the formation of MSSA standard strains (A) ATCC 29213, (B) ATCC 25923 and MRSA strains (C) ATCC 43300, (D) HYP6, (E) 26FS31, (F) C2Y, (G) 2ZG3, (H) YFC18.
Supplementary Figure 2 | Clearance rate of geraniol with sub-minimum inhibitory concentrations on preformed biofilms of MSSA standard strains (A) ATCC 29213, (B) ATCC 25923 and MRSA strains (C) ATCC 43300, (D) HYP6, (E) 26FS31, (F) C2Y, (G) 2ZG3, (H) YFC18.
References
Abdelhady, W., Bayer, A., Seidl, K., Moormeier, D., Bayles, K., Cheung, A., et al. (2014). Impact of vancomycin on sarA-mediated biofilm formation: role in persistent endovascular infections due to methicillin-resistant Staphylococcus aureus. J. Infect. Dis. 209, 1231–1240. doi: 10.1093/infdis/jiu007
Arendrup, M. C., Anupam, P., Joseph, M., Cheshta, S., and Anuradha, C. (2017). Comparison of EUCAST and CLSI reference microdilution MICs of eight antifungal compounds for candida auris and associated tentative epidemiological cutoff values. Antimicrob. Agents Chemother. 61:e00485-17. doi: 10.1128/AAC.00485-17
Biswas, R., Voggu, L., Simon, U., Hentschel, P., Thumm, G., and Götz, F. (2006). Activity of the major staphylococcal autolysin Atl. FEMS Microbiol. Lett. 259, 260–268. doi: 10.1111/j.1574-6968.2006.00281.x
Catalano, A., Iacopetta, D., Ceramella, J., Scumaci, D., Giuzio, F., Saturnino, C., et al. (2022). Multidrug Resistance (MDR): a widespread phenomenon in pharmacological therapies. Molecules 27:616. doi: 10.3390/molecules27030616
Clauditz, A., Resch, A., Wieland, K., Peschel, A., and Götz, F. (2006). Staphyloxanthin plays a role in the fitness of Staphylococcus aureus and its ability to cope with oxidative stress. Infect. Immun. 74, 4950–4953. doi: 10.1128/iai.00204-06
Cong, Y., Yang, S., and Rao, X. (2020). Vancomycin resistant Staphylococcus aureus infections: a review of case updating and clinical features. J. Adv. Res. 21, 169–176. doi: 10.1016/j.jare.2019.10.005
Dengler Haunreiter, V., Boumasmoud, M., Häffner, N., Wipfli, D., Leimer, N., Rachmühl, C., et al. (2019). In-host evolution of Staphylococcus epidermidis in a pacemaker-associated endocarditis resulting in increased antibiotic tolerance. Nat. Commun. 10:1149. doi: 10.1038/s41467-019-09053-9
El-Agamey, A., Lowe, G., McGarvey, D., Mortensen, A., Phillip, D., Truscott, T., et al. (2004). Carotenoid radical chemistry and antioxidant/pro-oxidant properties. Arch. Biochem. Biophys. 430, 37–48. doi: 10.1016/j.abb.2004.03.007
Freeman, D., Falkiner, F., and Keane, C. (1989). New method for detecting slime production by coagulase negative staphylococci. J. Clin. Pathol. 42, 872–874. doi: 10.1136/jcp.42.8.872
Friedman, M., Henika, P., and Mandrell, R. (2002). Bactericidal activities of plant essential oils and some of their isolated constituents against Campylobacter jejuni, Escherichia coli, Listeria monocytogenes, and Salmonella enterica. J. Food Prot. 65, 1545–1560. doi: 10.4315/0362-028x-65.10.1545
Gambino, E., Maione, A., Guida, M., Albarano, L., Carraturo, F., Galdiero, E., et al. (2022). Evaluation of the pathogenic-mixed biofilm formation of Pseudomonas aeruginosa/Staphylococcus aureus and treatment with limonene on three different materials by a dynamic model. Int. J. Environ. Res. Public Health 19:3741. doi: 10.3390/ijerph19063741
Gupta, P., Gupta, H., and Poluri, K. (2021). Geraniol eradicates Candida glabrata biofilm by targeting multiple cellular pathways. Appl. Microbiol. Biotechnol. 105, 5589–5605. doi: 10.1007/s00253-021-11397-6
Houston, P., Rowe, S., Pozzi, C., Waters, E., and O’Gara, J. (2011). Essential role for the major autolysin in the fibronectin-binding protein-mediated Staphylococcus aureus biofilm phenotype. Infect. Immun. 79, 1153–1165. doi: 10.1128/iai.00364-10
Inoue, D., Kabata, T., Ohtani, K., Kajino, Y., Shirai, T., and Tsuchiya, H. (2017). Inhibition of biofilm formation on iodine-supported titanium implants. Int. Orthop. 41, 1093–1099. doi: 10.1007/s00264-017-3477-3
Inouye, S., Takizawa, T., and Yamaguchi, H. (2001). Antibacterial activity of essential oils and their major constituents against respiratory tract pathogens by gaseous contact. J. Antimicrob. Chemother. 47, 565–573. doi: 10.1093/jac/47.5.565
Khan, M., Butt, S., Chaudhry, A., Rashid, A., Ijaz, K., Majeed, A., et al. (2022). Osteogenic induction with silicon hydroxyapatite using modified autologous adipose tissue-derived stromal vascular fraction: in vitro and qualitative histomorphometric analysis. Materials 15:1826. doi: 10.3390/ma15051826
Kwiatkowski, P., Sienkiewicz, M., Pruss, A., Łopusiewicz, Ł, Arszyńska, N., Wojciechowska-Koszko, I., et al. (2022). Klebsiella pneumoniaeantibacterial and anti-biofilm activities of essential oil compounds against New Delhi Metallo-β-lactamase-1-producing uropathogenic strains. Antibiotics 11:147. doi: 10.3390/antibiotics11020147
Lerch, M., Schoenfelder, S., Marincola, G., Wencker, F., Eckart, M., Förstner, K., et al. (2019). A non-coding RNA from the intercellular adhesion (ica) locus of Staphylococcus epidermidis controls polysaccharide intercellular adhesion (PIA)-mediated biofilm formation. Mol. Microbiol. 111, 1571–1591. doi: 10.1111/mmi.14238
Li, S., Chen, L., Wang, G., Xu, L., Hou, S., Chen, Z., et al. (2018). Anti-ICAM-1 antibody-modified nanostructured lipid carriers: a pulmonary vascular endothelium-targeted device for acute lung injury therapy. J. Nanobiotechnol. 16:105. doi: 10.1186/s12951-018-0431-5
Lin, L., Long, N., Qiu, M., Liu, Y., Sun, F., and Dai, M. (2021). The inhibitory efficiencies of geraniol as an anti-inflammatory, antioxidant, and antibacterial, natural agent against methicillin-resistant Staphylococcus aureus infection in vivo. Infect. Drug Resist. 14, 2991–3000. doi: 10.2147/IDR.S318989
Liu, G., and Nizet, V. (2009). Color me bad: microbial pigments as virulence factors. Trends Microbiol. 17, 406–413. doi: 10.1016/j.tim.2009.06.006
Mauro, T., Rouillon, A., and Felden, B. (2016). Insights into the regulation of small RNA expression: SarA represses the expression of two sRNAs in Staphylococcus aureus. Nucleic Acids Res. 44, 10186–10200. doi: 10.1093/nar/gkw777
Nagasawa, R., Sato, T., and Senpuku, H. (2017). Raffinose induces biofilm formation by Streptococcus mutans in low concentrations of sucrose by increasing production of extracellular DNA and fructan. Appl. Environ. Microbiol. 83:e00869-17. doi: 10.1128/aem.00869-17
Nguyen, H. T. T., Nguyen, T. H., and Otto, M. (2020). The staphylococcal exopolysaccharide PIA – Biosynthesis and role in biofilm formation, colonization, and infection. Comput. Struct. Biotechnol. J. 18, 3324–3334. doi: 10.1016/j.csbj.2020.10.027
Nuryastuti, T., and Krom, B. (2017). Ica-status of clinical Staphylococcus epidermidis strains affects adhesion and aggregation: a thermodynamic analysis. Antonie Van Leeuwenhoek 110, 1467–1474. doi: 10.1007/s10482-017-0899-2
Oriol, C., Cengher, L., Manna, A., Mauro, T., Pinel-Marie, M., Felden, B., et al. (2021). Expanding the Staphylococcus aureus SarA regulon to small RNAs. mSystems 6:e0071321. doi: 10.1128/mSystems.00713-21
Pantrangi, M., Singh, V., and Shukla, S. (2015). Regulation of staphylococcal superantigen-like gene, ssl8, expression in Staphylococcus aureus strain, RN6390. Clin. Med. Res. 13, 7–11. doi: 10.3121/cmr.2014.1226
Peng, Q., Lin, F., and Ling, B. (2020). In vitro activity of biofilm inhibitors in combination with antibacterial drugs against extensively drug-resistant Acinetobacter baumannii. Sci. Rep. 10:18097. doi: 10.1038/s41598-020-75218-y
Prasad, S. N., and Muralidhara, M. (2017). Analysis of the antioxidant activity of geraniol employing various in-vitro models: relevance to neurodegeneration in diabetic neuropathy. Asian J. Pharmaceutical Clin. Res. 10, 101–105.
Rice, K., Mann, E., Endres, J., Weiss, E., Cassat, J., Smeltzer, M., et al. (2007). The cidA murein hydrolase regulator contributes to DNA release and biofilm development in Staphylococcus aureus. Proc. Natl. Acad. Sci. U S A. 104, 8113–8118. doi: 10.1073/pnas.0610226104
Roy, R., Tiwari, M., Donelli, G., and Tiwari, V. (2018). Strategies for combating bacterial biofilms: a focus on anti-biofilm agents and their mechanisms of action. Virulence 9, 522–554. doi: 10.1080/21505594.2017.1313372
Schilcher, K., and Horswill, A. R. (2020). Staphylococcal biofilm development: structure, regulation, and treatment strategies. Microbiol. Mol. Biol. Rev. MMBR 84:e00026-19. doi: 10.1128/MMBR.00026-19
Schmittgen, T., and Livak, K. (2008). Analyzing real-time PCR data by the comparative C(T) method. Nat. Protocols 3, 1101–1108. doi: 10.1038/nprot.2008.73
Selvaraj, A., Jayasree, T., Valliammai, A., and Pandian, S. K. (2019). Myrtenol attenuates MRSA biofilm and virulence by suppressing sarA expression dynamism. Front. Microbiol. 10:2027. doi: 10.3389/fmicb.2019.02027
Selvaraj, A., Valliammai, A., Muthuramalingam, P., Priya, A., Suba, M., Ramesh, M., et al. (2020). Carvacrol targets SarA and CrtM of methicillin-resistant Staphylococcus aureus to mitigate biofilm formation and staphyloxanthin synthesis: an in vitro and in vivo approach. ACS Omega 5, 31100–31114. doi: 10.1021/acsomega.0c04252
Sharma, D., Misba, L., and Khan, A. (2019). Antibiotics versus biofilm: an emerging battleground in microbial communities. Antimicrobial Resistance Infect. Control 8:76. doi: 10.1186/s13756-019-0533-3
Soler-Arango, J., Figoli, C., Muraca, G., Bosch, A., and Brelles-Marino, G. (2019). The Pseudomonas aeruginosa biofilm matrix and cells are drastically impacted by gas discharge plasma treatment: a comprehensive model explaining plasma-mediated biofilm eradication. PLoS One 14:e0216817. doi: 10.1371/journal.pone.0216817
Swarupa, V., Chaudhury, A., and Sarma, P. (2018). Staphylococcus aureusIron enhances the peptidyl deformylase activity and biofilm formation in. 3 Biotech 8:32. doi: 10.1007/s13205-017-1050-9
Tamber, S., and Cheung, A. (2009). SarZ promotes the expression of virulence factors and represses biofilm formation by modulating SarA and agr in Staphylococcus aureus. Infect. Immun. 77, 419–428. doi: 10.1128/iai.00859-08
Tenover, F. C., McDougal, L. K., Goering, R. V., Killgore, G., Projan, S. J., Patel, J. B., et al. (2006). Characterization of a strain of community-associated methicillin-resistant Staphylococcus aureus widely disseminated in the United States. J. Clin. Microbiol. 44, 108–118. doi: 10.1128/JCM.44.1.108-118.2006
Toiu, A., Mocan, A., Vlase, L., Pârvu, A., Vodnar, D., Gheldiu, A., et al. (2019). In VivoComparative phytochemical profile, antioxidant, antimicrobial and anti-inflammatory activity of different extracts of traditionally used romanian L. and L. (Lamiaceae). Molecules 24:1597. doi: 10.3390/molecules24081597
Trotonda, M., Manna, A., Cheung, A., Lasa, I., and Penadés, J. (2005). SarA positively controls bap-dependent biofilm formation in Staphylococcus aureus. J. Bacteriol. 187, 5790–5798. doi: 10.1128/jb.187.16.5790-5798.2005
Turner, N. A., Sharma-Kuinkel, B. K., Maskarinec, S. A., Eichenberger, E. M., Shah, P. P., Carugati, M., et al. (2019). Methicillin-resistant Staphylococcus aureus: an overview of basic and clinical research. Nat. Rev. Microbiol. 17, 203–218. doi: 10.1038/s41579-018-0147-4
Tursi, S. A., Puligedda, R. D., Szabo, P., Nicastro, L. K., Miller, A. L., Qiu, C., et al. (2020). Salmonella Typhimurium biofilm disruption by a human antibody that binds a pan-amyloid epitope on curli. Nat. Commun. 11:1007. doi: 10.1038/s41467-020-14685-3
Valliammai, A., Sethupathy, S., Priya, A., Selvaraj, A., Bhaskar, J., Krishnan, V., et al. (2019). 5-Dodecanolide interferes with biofilm formation and reduces the virulence of methicillin-resistant Staphylococcus aureus (MRSA) through up regulation of agr system. Sci. Rep. 9:13744. doi: 10.1038/s41598-019-50207-y
Vijayakumar, K., Muhilvannan, S., and Arun Vignesh, M. (2022). Hesperidin inhibits biofilm formation, virulence and staphyloxanthin synthesis in methicillin resistant Staphylococcus aureus by targeting SarA and CrtM: an in vitro and in silico approach. World J. Microbiol. Biotechnol. 38:44. doi: 10.1007/s11274-022-03232-5
Wu, Y., Wang, Z., Fu, X., Lin, Z., and Yu, K. (2020). Geraniol-mediated osteoarthritis improvement by down-regulating PI3K/Akt/NF-κB and MAPK signals: in vivo and in vitro studies. Int. Immunopharmacol. 86:106713. doi: 10.1016/j.intimp.2020.106713
Xu, L., Liu, M., Yang, Y., Wang, Y., Hua, X., Du, L., et al. (2022). Geraniol enhances inhibitory inputs to the paraventricular thalamic nucleus and induces sedation in mice. Phytomedicine 98:153965. doi: 10.1016/j.phymed.2022.153965
Yang, H., Liu, G., Zhao, H., Dong, X., and Yang, Z. (2021). Inhibiting the JNK/ERK signaling pathway with geraniol for attenuating the proliferation of human gastric adenocarcinoma AGS cells. J. Biochem. Mol. Toxicol. 35:e22818. doi: 10.1002/jbt.22818
Yehia, F., Yousef, N., and Askoura, M. (2022). Celastrol mitigates staphyloxanthin biosynthesis and biofilm formation in Staphylococcus aureus via targeting key regulators of virulence; in vitro and in vivo approach. BMC Microbiol. 22:106. doi: 10.1186/s12866-022-02515-z
Younis, N., Elsewedy, H., Soliman, W., Shehata, T., and Mohamed, M. (2021). Geraniol isolated from lemon grass to mitigate doxorubicin-induced cardiotoxicity through Nrf2 and NF-κB signaling. Chem. Biol. Interact. 347:109599. doi: 10.1016/j.cbi.2021.109599
Yu, H., Liu, Y., Yang, F., Xie, Y., Guo, Y., Cheng, Y., et al. (2022). The combination of hexanal and geraniol in sublethal concentrations synergistically inhibits quorum sensing in Pseudomonas fluorescens-in vitro and in silico approaches. J. Appl. Microbiol. Online ahead of print. doi: 10.1111/jam.15446
Yuan, Q., Feng, W., Wang, Y., Wang, Q., Mou, N., Xiong, L., et al. (2022). Luteolin attenuates the pathogenesis of Staphylococcus aureus by interfering with the agr system. Microb. Pathog. 165:105496. doi: 10.1016/j.micpath.2022.105496
Yuan, Z., Dai, Y., Ouyang, P., Rehman, T., Hussain, S., Zhang, T., et al. (2020). Thymol inhibits biofilm formation, eliminates pre-existing biofilms, and enhances clearance of methicillin-resistant Staphylococcus aureus (MRSA) in a mouse peritoneal implant infection model. Microorganisms 8:99. doi: 10.3390/microorganisms8010099
Yuan, Z., Ouyang, P., Gu, K., Rehman, T., Zhang, T., Yin, Z., et al. (2019). The antibacterial mechanism of oridonin against methicillin-resistant Staphylococcus aureus (MRSA). Pharm. Biol. 57, 710–716. doi: 10.1080/13880209.2019.1674342
Keywords: geraniol, MRSA, biofilm, PIA, eDNA, sarA, staphyloxanthin, implant model
Citation: Gu K, Ouyang P, Hong Y, Dai Y, Tang T, He C, Shu G, Liang X, Tang H, Zhu L, Xu Z and Yin L (2022) Geraniol inhibits biofilm formation of methicillin-resistant Staphylococcus aureus and increase the therapeutic effect of vancomycin in vivo. Front. Microbiol. 13:960728. doi: 10.3389/fmicb.2022.960728
Received: 03 June 2022; Accepted: 16 August 2022;
Published: 06 September 2022.
Edited by:
Xiaolin Hou, Beijing University of Agriculture, ChinaReviewed by:
Alaguvel Valliammai, Ben-Gurion University of the Negev, IsraelHong-Ning Wang, Sichuan University, China
Arunachalam Kannappan, Shanghai Jiao Tong University, China
Copyright © 2022 Gu, Ouyang, Hong, Dai, Tang, He, Shu, Liang, Tang, Zhu, Xu and Yin. This is an open-access article distributed under the terms of the Creative Commons Attribution License (CC BY). The use, distribution or reproduction in other forums is permitted, provided the original author(s) and the copyright owner(s) are credited and that the original publication in this journal is cited, in accordance with accepted academic practice. No use, distribution or reproduction is permitted which does not comply with these terms.
*Correspondence: Zhiwen Xu, abtcxzw@126.com; Lizi Yin, yinlizi@siacu.edu.cn
†These authors have contributed equally to this work