- 1Department of Agricultural and Food Sciences, Alma Mater Studiorum - University of Bologna, Bologna, Italy
- 2Department of Food Science and Technology, University of Córdoba, Agrifood Campus of International Excellence ceiA3, Córdoba, Spain
- 3Department of Veterinary Medical Sciences, Alma Mater Studiorum - University of Bologna, Bologna, Italy
Artisanal cheeses are produced in small-scale production plants, where the lack of full automation and control of environmental and processing parameters suggests a potential risk of microbial contamination. The aim of this study was to perform a longitudinal survey in an Italian artisanal factory producing a spreadable soft cheese with no rind to evaluate the inter- and intra-batch variability of physicochemical and microbial parameters on a total of 720 environmental and cheese samples. Specifically on cheese samples, the evaluation was additionally performed on physicochemical parameters. Cheese samples were additionally collected during 15 days of storage at constant temperatures of 2 and 8°C, as well as a dynamic profile of 2°C for 5 days and 8°C for 10 days. Furthermore, Enterobacteriaceae isolates were identified at species level to have a better knowledge of the environmental and cheese microbiota potentially harboring human pathogens. High inter-batch variability was observed for lactic acid bacteria (LAB) and total bacteria count (TBC) in cheese at the end of production but not for pH and water activity. A temperature of 8°C was associated with a significantly higher load of Enterobacteriaceae in cheeses belonging to batch 6 at the end of storage, and this temperature also corresponded with the highest increase in LAB and TBC loads over cheese shelf life. Results from generalized linear mixed models (GLMMs) indicated that drains in the warm room and the packaging area were associated with higher levels of TBC and Enterobacteriaceae in cheese. Regarding foodborne pathogens, no sample was positive for verotoxigenic Escherichia coli (VTEC) or Listeria monocytogenes, whereas six Staphylococcus aureus and one Salmonella pullorum isolates were collected in cheese samples during storage and processing, respectively. Regarding Enterobacteriaceae, 166 isolates were identified at species level from all batches, with most isolates belonging to Klebsiella oxytoca and pneumoniae, Enterobacter cloacae, Hafnia alvei, and Citrobacter freundii evidencing the need to focus on standardizing the microbial quality of cow milk and on hygienic procedures for cleaning and disinfection especially in warm and maturation rooms. Further studies should be performed to investigate the potential pathogenicity and antimicrobial resistance of the identified Enterobacteriaceae species in artisanal cheeses.
Introduction
Artisanal foods are increasing in popularity since they are perceived as homemade and more genuine products (Cirne et al., 2019). Moreover, these products usually benefit from an undeniable and recognized richness of taste, providing valuable organoleptical properties which are highly appreciated by consumers. The practices or technologies applied and the know-how of the artisanal food producers are protected from imitation and misuse by the European Union (EU) geographical indication schemes such as Protected Designation of Origin (PDO) (European Union [EU], 2012). As regards quality standards, Italy is the EU country with the highest number of PDOs (European Commission [EC], 2022).
These quality standards are also linked to various biodiversity factors, such as microbial communities brought by ingredients and production environments. Due to the lack of standardization in the artisanal productions, data on the microbial parameters of these products are scarce.
As artisanal cheeses are produced in small-scale production plants where control of the process is often challenging due to the lack of full automation and control of environmental parameters and processing variables, there is a suggested potential risk of microbial contamination and growth (Jaramillo-Bedoya et al., 2021). In this regard, it is important to monitor the microbial quality of the food and the environment to reduce the exposure of consumers to microbial hazards. In the dairy industry, standard microbial testing focuses on the enumeration of total mesophilic bacteria, coagulase-positive staphylococci and Enterobacteriaceae as contamination indicators (Fusco et al., 2020). The presence of Enterobacteriaceae, although recognized as part of the natural microbiota of many cheeses, in high concentrations is usually indicative of poor microbiological quality (Maifreni et al., 2013).
Microbial testing is particularly relevant in those cheeses posing a higher risk for consumers, namely, those which have a short maturation time and with a higher water content (> 45%) (named “soft”), with no rind, and a short shelf life (e.g., < 15 days). Italy has a long tradition of artisanal soft cheeses with PDO; Squacquerone di Romagna, Casatella Trevigiana, and Robiola di Roccaverano are some examples. For soft cheese made with pasteurized cows’ milk, according to EU regulations No. 853/2004 and No. 2073/2005, microbiological analyses by food business operators should focus on raw cows’ milk and the final cheese product (European Union [EU], 2004, 2007). Processed cows’ milk cannot exceed a load of 100,000 cfu/mL at 30°C when directly used to produce dairy products (European Union [EU], 2004). In soft cheese at the end of the manufacturing process, coagulase-positive staphylococci cannot exceed 100 cfu/g in more than two over five samples tested.
From a food safety perspective, soft cheese can be a vehicle of different microbial hazards. Because of its tolerance to a wide range of environmental conditions, Listeria monocytogenes can grow and survive in the processing plant and contaminate food during production. L. monocytogenes outbreaks associated with the consumption of contaminated fresh and/or soft cheeses have been reported worldwide, including the United States, Sweden, Canada, Czechia, Austria, Portugal among other countries (Jackson et al., 2018; Martinez-Rios and Dalgaard, 2018). Staphylococcus aureus often causes mastitis in cows, leading to milk contamination (Rabello et al., 2007). It also inhabits the skin and nasal cavity of food handlers, who might act as vehicles of transmission of virulent strains to food (Castro et al., 2016). Outbreaks of S. aureus have been associated with the consumption of contaminated milk or cheese (Johler et al., 2015a,b). In Italy, S. aureus has been isolated from raw milk and artisanal raw milk cheeses (Johler et al., 2018). Salmonella spp. has also been a common causative agent of foodborne outbreaks linked to cheese products being contaminated with microbes associated with the primary production and processing environment (Robinson et al., 2018). Regarding Escherichia coli, Shiga toxin-producing E. coli (STEC) was associated to outbreaks related to the consumption of raw milk and raw milk cheeses in the United States in 2009–2014 and in France in 2019 (Costard et al., 2017; Jones et al., 2019). Although the pathogen is inactivated by pasteurization, STEC can be detected in the final product as a result of post-process contamination (Dos Santos Rosario et al., 2021).
Based on EU regulations, when process hygiene criteria are not fulfilled, corrective measures are required during cheesemaking operations. Therefore, knowledge on microbial loads at each stage of production is of paramount importance to select specific and suitable control measures in real time. The microbiological status at each stage of production of both the food product and the environment could be helpful to describe the whole food production chain and to specifically evaluate the intra- and inter-batch variability of artisanal productions. At present, very few scientific papers have focused on the microbial quality of soft cheese made from raw or pasteurized cow or goat cheese worldwide (Pappa et al., 2017; Barukèiæ et al., 2020; Silva et al., 2021), and none focused on artisanal PDO products.
The aim of this study was to perform a longitudinal survey in an Italian artisanal factory producing a spreadable soft cheese with no rind to evaluate the physicochemical and microbial parameters during the different production stages. Quantitative data collected over a year were analyzed through statistical models to identify the intra- and inter-batch variability of total bacterial count (TBC), Enterobacteriaceae, and lactic acid bacteria (LAB) from raw materials, environmental and product samples. Among these data, potential correlations within different stages of the production chain were investigated. Moreover, a durability study was carried out to investigate the microbial fate of artisanal cheeses during 15 days at constant temperatures of 2 and 8°C, as well as a dynamic profile of 2°C for 5 days and 8°C for 10 days. Furthermore, Enterobacteriaceae isolates were identified at species level to have a better knowledge of the environmental and cheese microbiota.
Materials and methods
Experimental design
The cheese production chain of one Italian artisanal factory was sampled over 14 months (January 2020–March 2021). The factory is producing a spreadable soft cheese with no rind made of pasteurized cow milk. Regarding processing, after milk pasteurization, starter cultures, calf rennet, and enzymes were added to the milk. After coagulation and clot disruption, curd was drained. After storage in the warm room at 25°C for 1.5 h, cheese was ripened at 5°C for 1–4 days in the maturation room, and then it was packed in the packaging area. Raw materials and intermediate and final products were sampled along with the swabs from the processing environment. A total of 720 samples were tested, namely, unpasteurized cow milk (n = 24), pasteurized milk (n = 24), calf rennet (n = 30), and cheese (n = 78) and environmental samples [walls (n = 56) and drains (n = 72)] from the warm room, the maturation room, and the packaging area. Additionally, workers’ gloves (n = 20) at the packaging area were sampled. Environmental samples were collected by swabbing a 100 cm2 area with a sterile cotton swab (Copan Italia, Brescia, Italy) that has been moistened in 10 mL of saline solution (0.9% NaCl). Environmental samples were collected before cleaning and disinfection procedures. Additionally, within a durability study, samples of soft cheese (n = 416) were analyzed at specific time intervals (i.e., 0, 1, 4, 8, 11, and 15 days) during storage at 2°C, 8°C, and dynamic temperatures (i.e., 2°C for the first 5 days and 8°C for the remaining 10 days of storage). Five sample units per matrix (i.e., food and environment) were tested for each batch. Overall, six batches were investigated: batch 1 (January 2020), batch 2 (May 2020), batch 3 (July 2020), batch 4 (November 2020), batch 5 (January 2021), and batch 6 (March 2021).
Microbiological analyses
Total bacterial counts (TBCs) were enumerated in all samples collected at different production stages along with pH and water activity (aw) of cheese samples following international standard protocols (ISO, 1999, 2004a,2013). LAB (ISO, 1998) and Enterobacteriaceae (ISO, 2017a) were also quantified on raw materials and final products during shelf life lasting for 15 days. The occurrence of L. monocytogenes (ISO, 2017b), coagulase-positive Staphylococci (ISO, 2004b), verotoxigenic E. coli (VTEC) (using ISO protocols and subsequent PCR for detection of Shiga toxin genes) (ISO, 2001; Perelle et al., 2004), and Salmonella spp. (ISO, 2017c) was also investigated on cheese samples and environmental sites. Moreover, to isolate and identify bacteria belonging to the Enterobacteriaceae family, 25 g of cheese was diluted in 225 mL of Buffer Peptone Water (BPW, Thermo Scientific, Milan, Italy) and incubated for 24 h at 37°C. For Enterobacteriaceae species identification, BPW pre-enriched cultures were then streaked on MacConkey agar (Thermo Scientific) and incubated for 24 h at 37°C. Five colonies per plate were harvested and submitted for confirmation by biochemical test (RapID ONE System and RapID STAPH PLUS System, Thermo Scientific) and PCR (Wesley et al., 2002; Perelle et al., 2004; Chander et al., 2011; Saraiva et al., 2018). If confirmation was obtained, one isolate per species per sample was retained.
Minimum inhibitory concentration
Since the relevance of multidrug-resistant Klebsiella spp. as human bacterial pathogen of nosocomial importance and since the number of Klebsiella spp. isolates was the most abundant within Enterobacteriaceae, the minimum inhibitory concentration of Klebsiella oxytoca and K. pneumoniae species was evaluated using Sensititre EUVSEC plates (Thermo Fisher Scientific Sensititre EU Surveillance Salmonella/E. coli EUVSEC Plate).
Data analysis and modeling
Data analysis and modeling were performed using R (R Core Team, 2020). Microbial counts determined from different food batches and different environmental samples were statistically compared by ANOVA (p ≤ 0.05). The Tukey HSD test was performed to identify the different homogeneous groups. Boxplots and barplots were constructed to enable the visualization of intra- and inter-batch variability on microbial counts determined on cheese samples stored at different temperatures during their shelf life.
Generalized linear mixed models
Generalized linear mixed models (GLMMs) were adjusted to longitudinal data sets from environmental surfaces, food contact surfaces, intermediate products, finished cheeses, and stored cheeses, including the estimation of inter-batch variability as random effect. To do so, the R packages, lme4 (Bates et al., 2015) and nlme, were used. Main effects considered the influence of the variables “Stage” (warm room, maturation room, packaging area, and storage) according to the form:
where Yis(b) is the count (log cfu/g) of a given microbial group (TBC, Enterobacteriaceae, and LAB) determined in the processing stage s, belonging to batch b; β0 is the model intercept that can take random shifts ub according to batch b; Stages is the processing stage s; and εis(b) is the error of the microbial count i determined in the processing stage s, belonging to batch b.
Samples (environment and drains swabs, workers’ gloves, intermediate cheese product, finished cheese, and stored cheese) taken from each processing stage were included in another main effects model as follows:
where Stages (Environmenti) is the sample i taken from the processing stage s belonging to batch b.
The between-batch variability (within a factory) was determined from the squared standard deviation of the normal distribution for the random effects. Errors are also assumed to follow a normal distribution.
Results and discussion
Enumeration of total bacterial count, lactic acid bacteria, and Enterobacteriaceae
As regards environmental swabs collected at the processing plant, statistical differences were recorded on TBC within the different types of samples (p ≤ 0.05). Drains samples presented the highest loads of TBC compared to the other tested environmental sites. More specifically, drains samples of the warm room (WW) showed the highest TBC with a mean value of 7.07 ± 1.10 log cfu/cm2, followed by drains of the packaging area (WP, mean value 5.43 ± 0.94 log cfu/cm2) and drains of the maturation room (WM, mean value 4.83 ± 1.02 log cfu/cm2) (Figure 1).
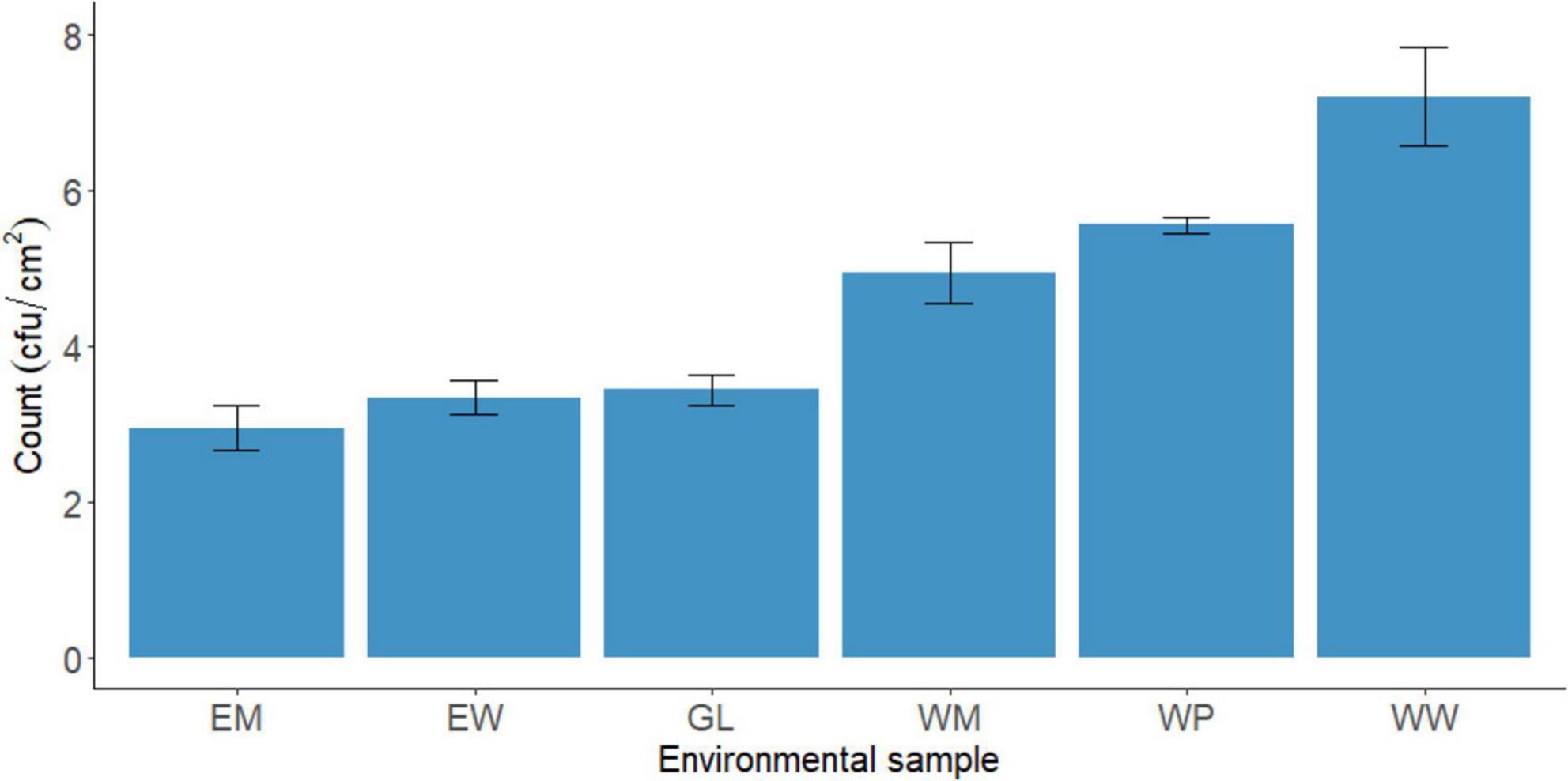
Figure 1. Total bacterial count (TBC) on environmental samples collected at the processing plant. EM, walls, maturation room; EW, walls, warm room; GL, gloves of workers, packaging area; WM, drains, maturation room; WP, drains, packaging area; WW, drains, warm room (mean ± standard deviation of six batches).
As regards milk samples, statistical differences in TBC were detected between the investigated batches (p ≤ 0.05), with batch 2 and batch 6 showing the highest and the lowest TBC values, respectively (Table 1). Pasteurization (T ≥ 72°C for 20 s, 6,000 L/h) led to a decrease of microbial loads of 3 log reductions on average. In particular, TBC decreased from 6.48 ± 0.49 to 3.34 ± 0.76 log cfu/mL. The levels of TBC in milk after pasteurization were compliant with the requirements of EU regulation 853/2004 for processed cows’ milk intended for the elaboration of dairy products (TBC no higher than 100,000 cfu/mL) (European Union [EU], 2004).
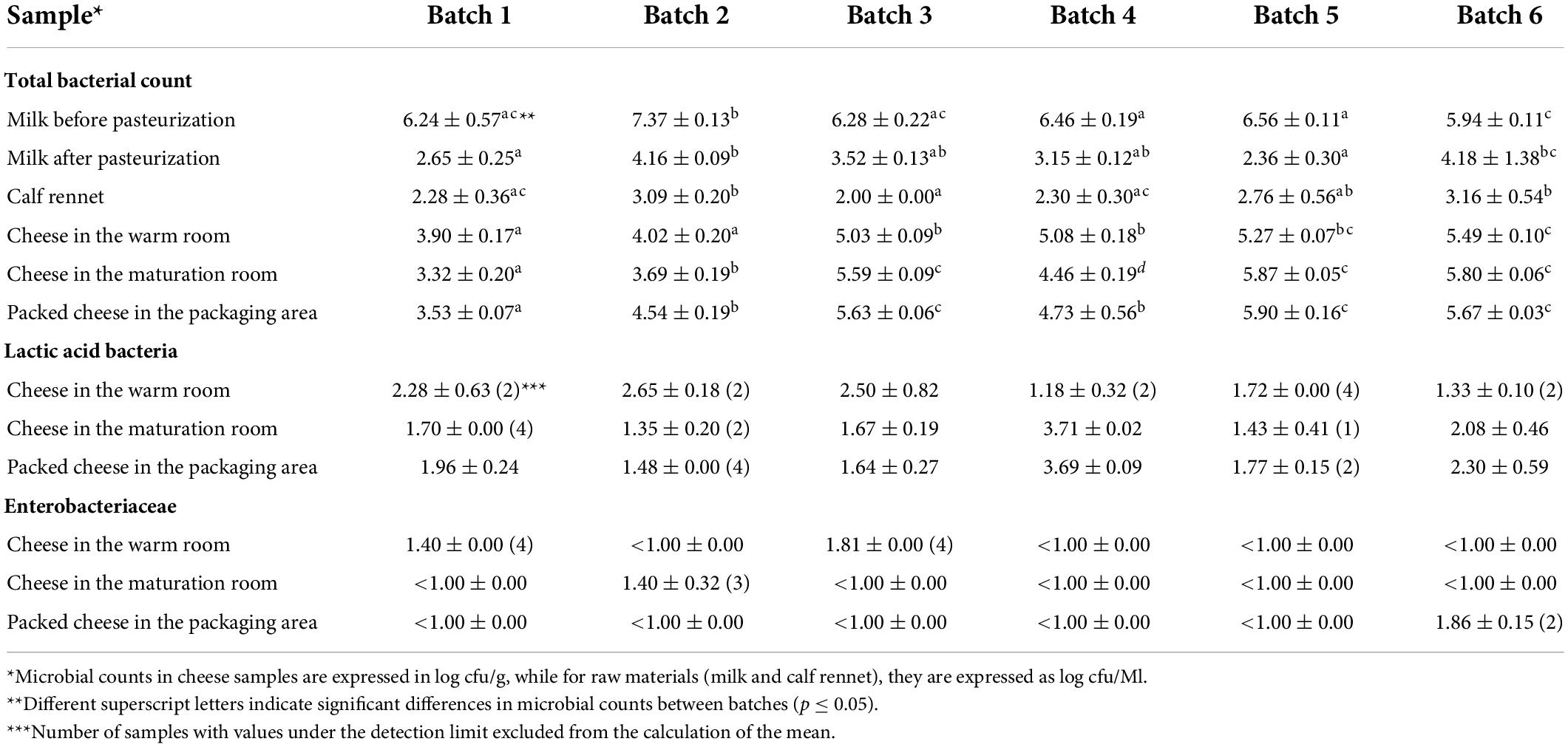
Table 1. Enumeration of total bacterial count, lactic acid bacteria, and Enterobacteriaceae in raw materials and cheese during processing.
For the soft cheese samples collected at different stages of the production process, the TBC counts were constant during the production stages with no differences when compared to cheese sampled in the warm, maturation, and packaging rooms in each batch. Low intra-batch variability was observed in these samples (Table 1). However, inter-batch variability was observed with average differences of TBC up to 2.4 log cfu/g [i.e., 3.53 (batch 1) vs. 5.90 (batch 5) log cfu/g quantified on packed cheese collected at the packaging area] with batches 3, 5, and 6 showing the highest loads (Figure 2). Inter-batch variability was observed for LAB especially in packed cheese [i.e., 1.06 (batch 2) vs. 3.69 (batch 4) log cfu/g] with batch 4 showing the highest load (Figure 2). Moreover, LAB counts showed higher intra-batch variability compared to TBC especially in batches 5 and 6 (Figure 2). At the end of processing, LAB showed higher counts in batch 4 in comparison with the other batches (Figure 2).
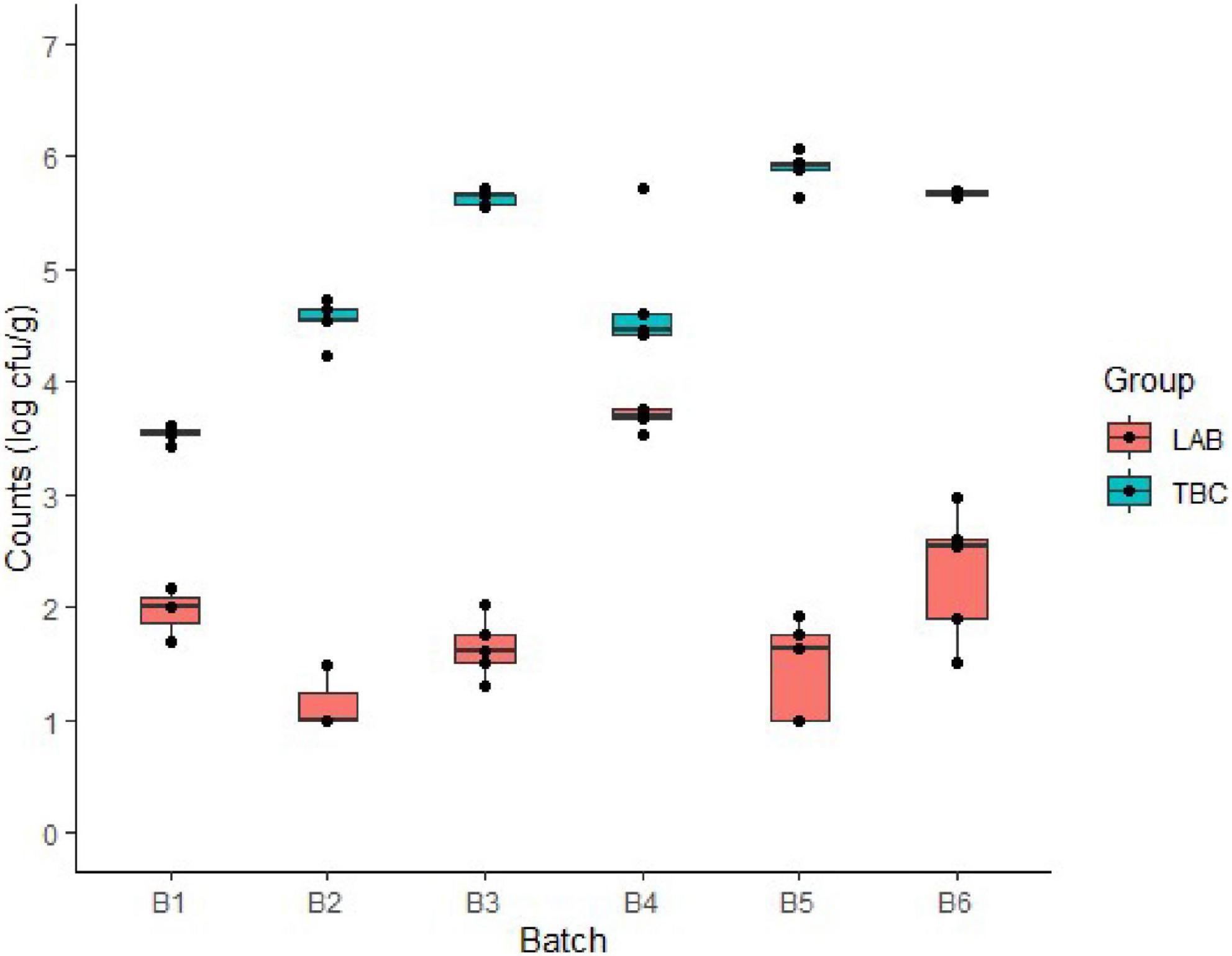
Figure 2. Lactic acid bacteria (LAB) and total bacterial counts (TBCs) in packaged cheese samples belonging to the six tested batches (B) at the end of the production process.
The high variability of LAB counts might be due to the fat composition of milk. In summer the fat composition of milk is lower than in winter (summer fat content 3.70–3.80%, winter fat content approximately 4.10–4.20%) (unpublished data). Although it is clear that the fat content plays a role, different effects are reported on different starter cultures and non-starter culture Lactobacilli in dairy products. Yogurt with a lower fat content was already described as associated with higher growth capability of Lactobacillus acidophilus (Tavakoli et al., 2019). Similarly, high LAB content was observed in low-fat feta cheese at the end of 14 days storage at 4°C (Ahmed et al., 2021). In contrast, non-starter culture Lactobacillus casei showed higher growth performances with higher fat content in cheddar cheese (Tan et al., 2012).
In the packed cheese collected at the packaging area, Enterobacteriaceae loads were below the detection limit of 1 log10 cfu/g in all samples tested from batches 1–5, whereas in batch 6 the mean Enterobacteriaceae concentration was 1.86 ± 0.15 log cfu/g (Table 1).
Regarding cheese stored at different temperatures, the fate of TBC and LAB showed an increase over cheese shelf life, although storage at 2°C was associated to a slower increase (Table 2). Specifically, approx. 4–5 log cfu/g at day 8 at 8°C (batch 4), at day 11 at 2/8°C (batches 2, 4, and 5) and at day 15 at 2°C (batch 5) (Figure 2). Regarding inter-batch variability, after 15 days of storage, differences of up to approximately 2 log cfu/g at 2°C and 3 log cfu/g at 8 and 2/8°C were observed for LAB (Figure 3). Similar inter-batch variability was observed at the same day of sampling for TBC with differences of approximately 2.5–3.5 log cfu/g at 8°C and 2/8°C. However, no differences were observed at 2°C (Figure 4). Both bacterial groups showed the highest concentrations in batch 5, reaching 5.31 and 8.03 log cfu/g, respectively, for LAB and TBC after 15 days of storage at 8°C (Figures 3, 4).
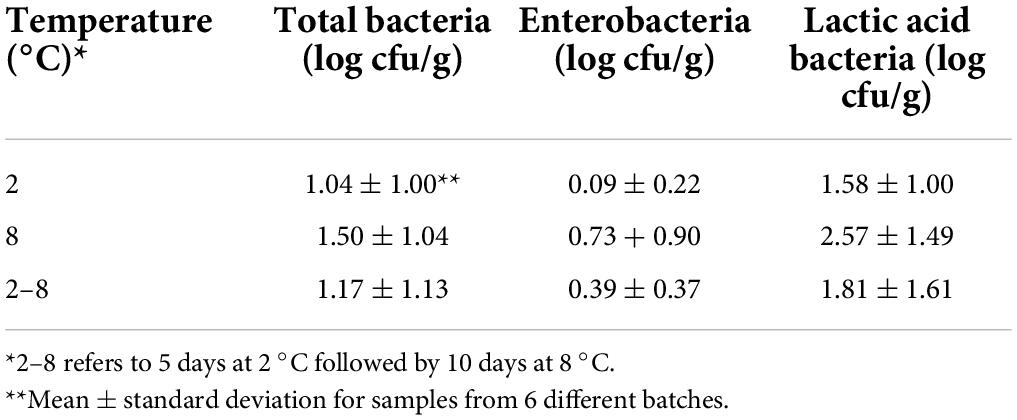
Table 2. Mean increase of microbial concentrations on Italian soft cheeses during 15 days of storage at different temperatures.
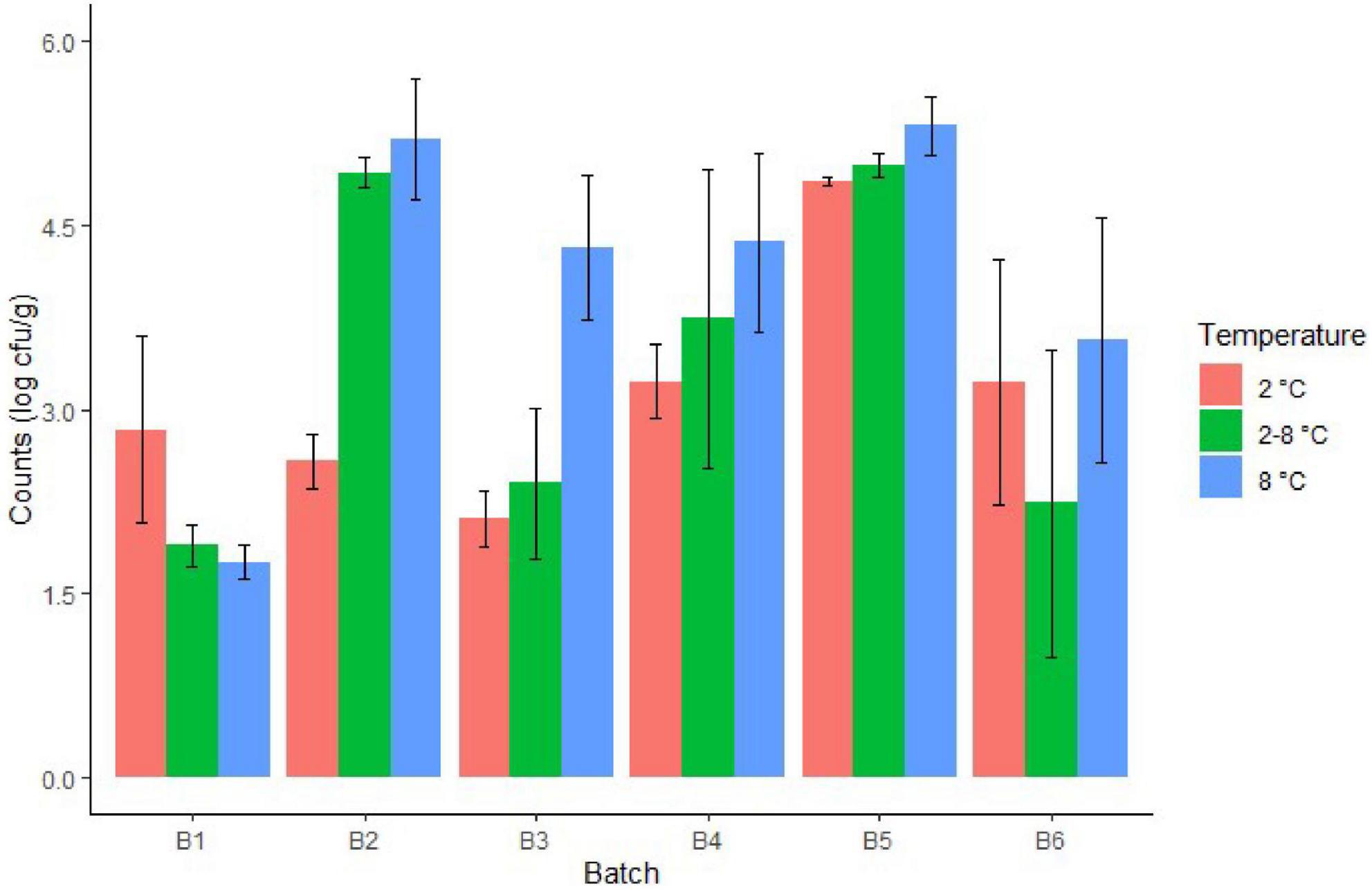
Figure 3. Lactic acid bacteria count in cheeses from different batches after 15 days of storage at different temperatures.
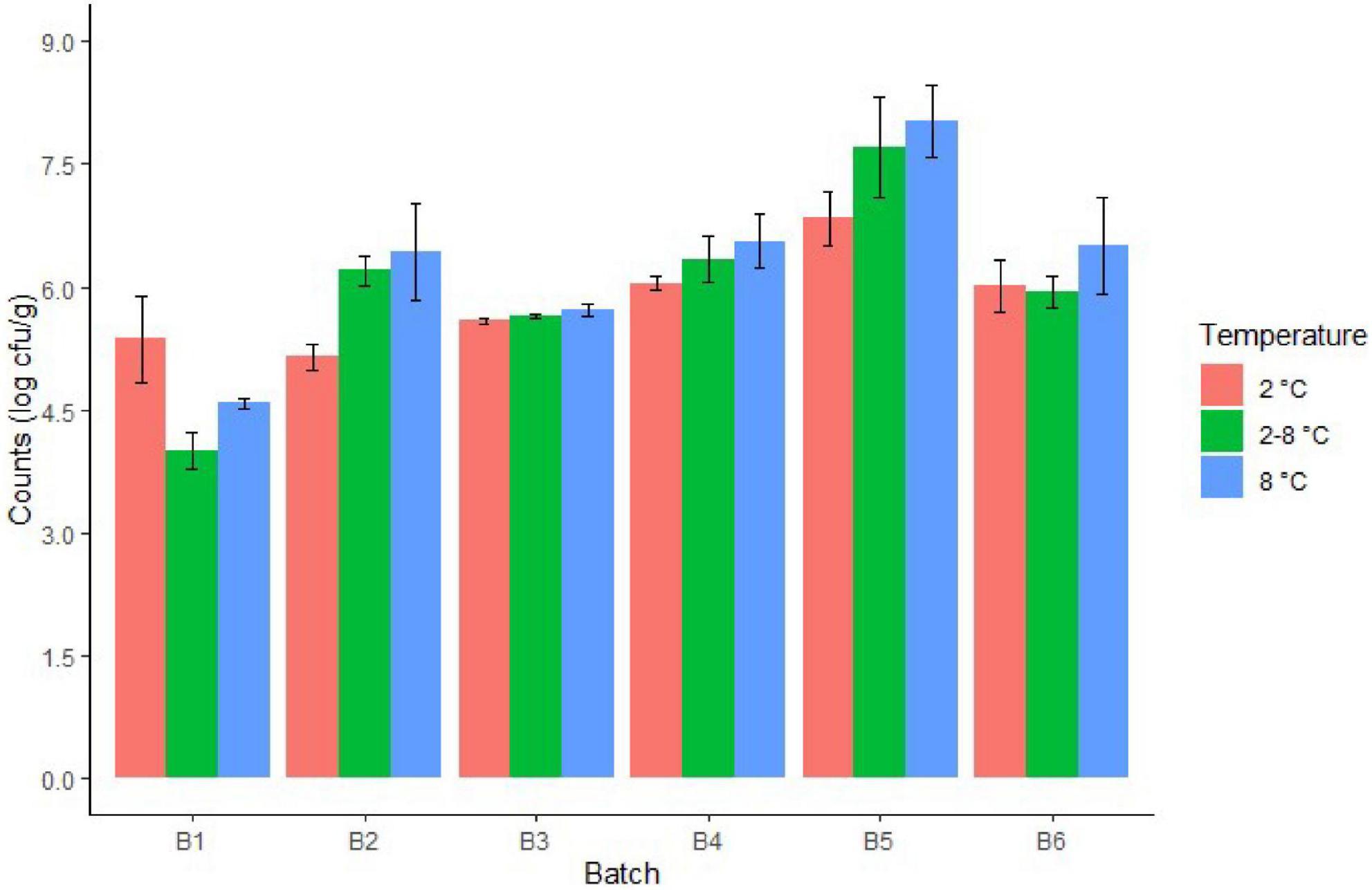
Figure 4. Total bacteria counts in cheeses from different batches after 15 days of storage at different temperatures.
Of all the investigated batches, batch 6 showed the highest intra-batch variability for LAB after 15 days of storage at all three tested temperatures (Figure 3). Reduced intra-batch variability was observed for TBC in cheese at the end of storage, whereas high variability was observed in batches 5 and 6 at the end of production (Figures 2, 4).
The counts of Enterobacteriaceae were under or close to the detection limit in all batches for all cheese samples during storage, except for batch 6 at day 15 at 8°C where a concentration of 3.06 ± 1.17 log cfu/g was observed (data not shown). Although not statistically significant, this result might be linked to a lower efficiency of the pasteurization process which was associated to a lower TBC reduction (1.76 log cfu/mL reduction in batch 6 in comparison with 3 log cfu/mL reduction on average in the other batches) (Table 1). A high coefficient of correlation has been previously observed between TBC and coliforms counts in bulk milk (Pantoja et al., 2009).
Temperature of 8°C is confirmed as an abuse temperature and associated in batch 6 with a significantly higher load of Enterobacteriaceae in the cheese at the end of production and the end of storage. In addition, the highest increase in LAB and TBC loads was recorded during cheese storage at this temperature (Table 2). In the literature, 8°C was determined as the lowest temperature that allows the growth of Salmonella in queso fresco (Kasrazadeh and Genigeorgis, 1994). In soft cheese, coliforms showed a higher increase rate during storage at 18°C in comparison with 4°C (Mohammed et al., 2019).
Moreover, no statistical differences were detected between the growth potential of a given microbial group at the different temperatures evaluated during cheese storage throughout its shelf life.
Evaluation of physicochemical parameters (pH and aw)
As regards raw materials such as milk, calf rennet, and cheese during production, as expected the pH decreased after the inclusion of the starter culture (Streptococcus thermophilus) (Table 3). However, only in batch 3 did the pH reach a value lower than 5.3 in packed cheese immediately at the end of production. This value is within the range of 4.9–5.3 reported for similar types of cheeses (Lante et al., 2006). During storage, the higher the temperature the lower the pH in line with the higher LAB count at 8°C (Figures 3, 5). As expected, aw of cheese at 15 days of storage was lower than that of cheese at day 0 (end of processing) (Figure 5).
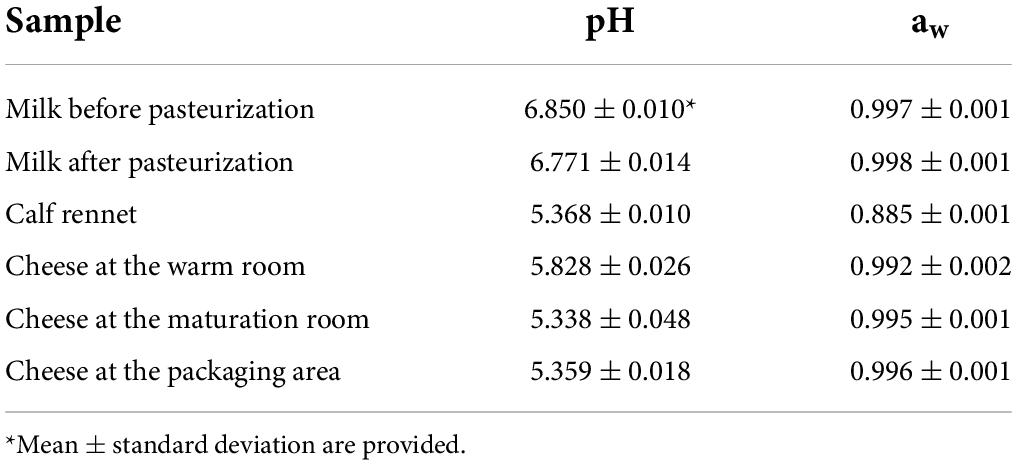
Table 3. pH and water activity (aw) of raw materials and Italian soft cheese during the production process.
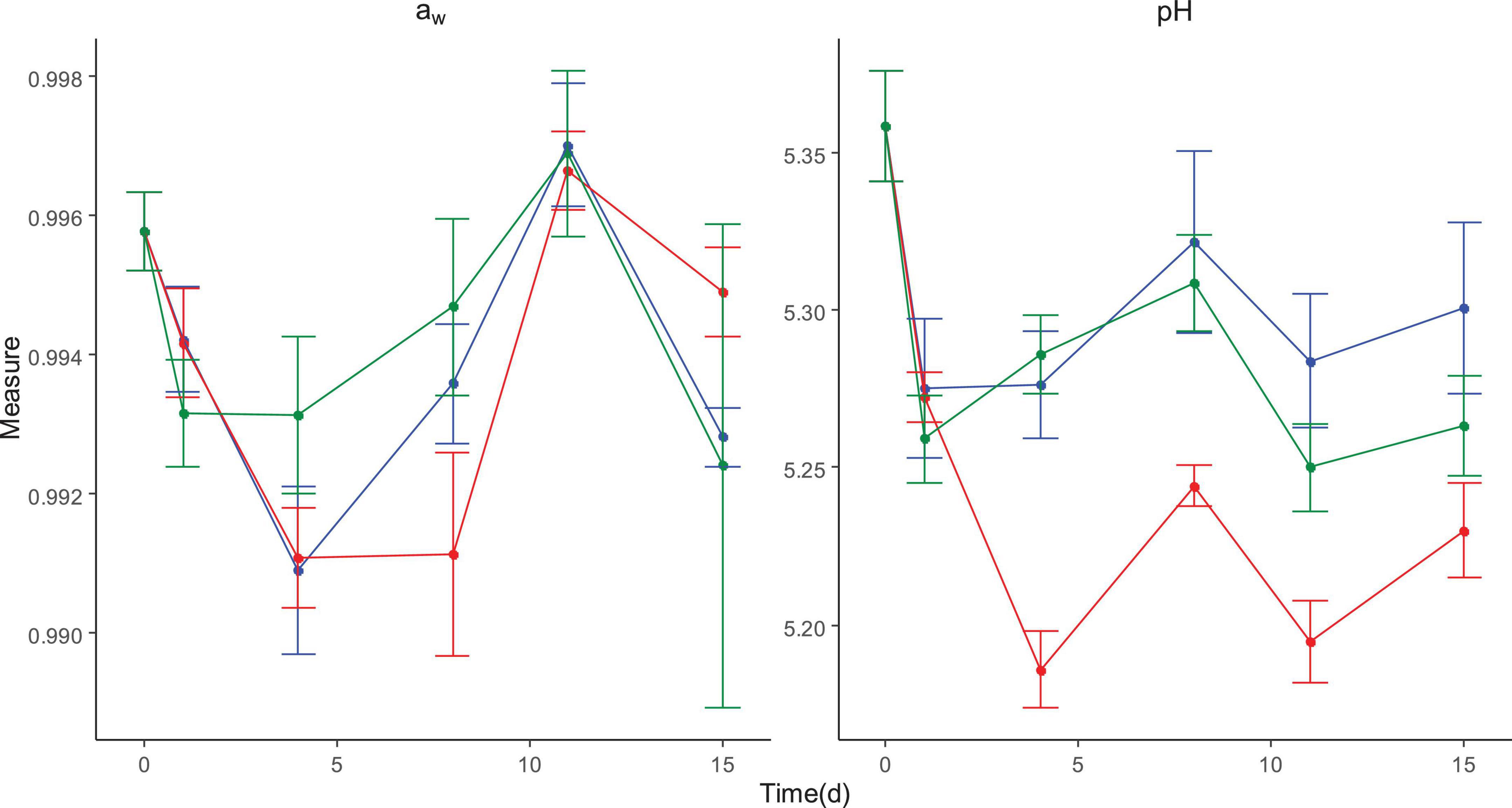
Figure 5. Mean ± standard deviation of aw and pH of cheeses from all batches during storage for 15 days at 2°C (blue line), 2–8°C (green line), and 8°C (red line).
For the cheese samples at the packaging area, pH and aw did not show significant differences during production between batches with pH values ranging from a minimum of 5.24 to a maximum of 5.47 and aw values ranging from 0.992 to 0.999.
Generalized linear mixed models
GLMMs were developed to investigate whether the production stage and the environment could partially explain the inter-batch differences in TBC and Enterobacteriaceae counts in cheeses. The results of this analysis are shown in Table 4.
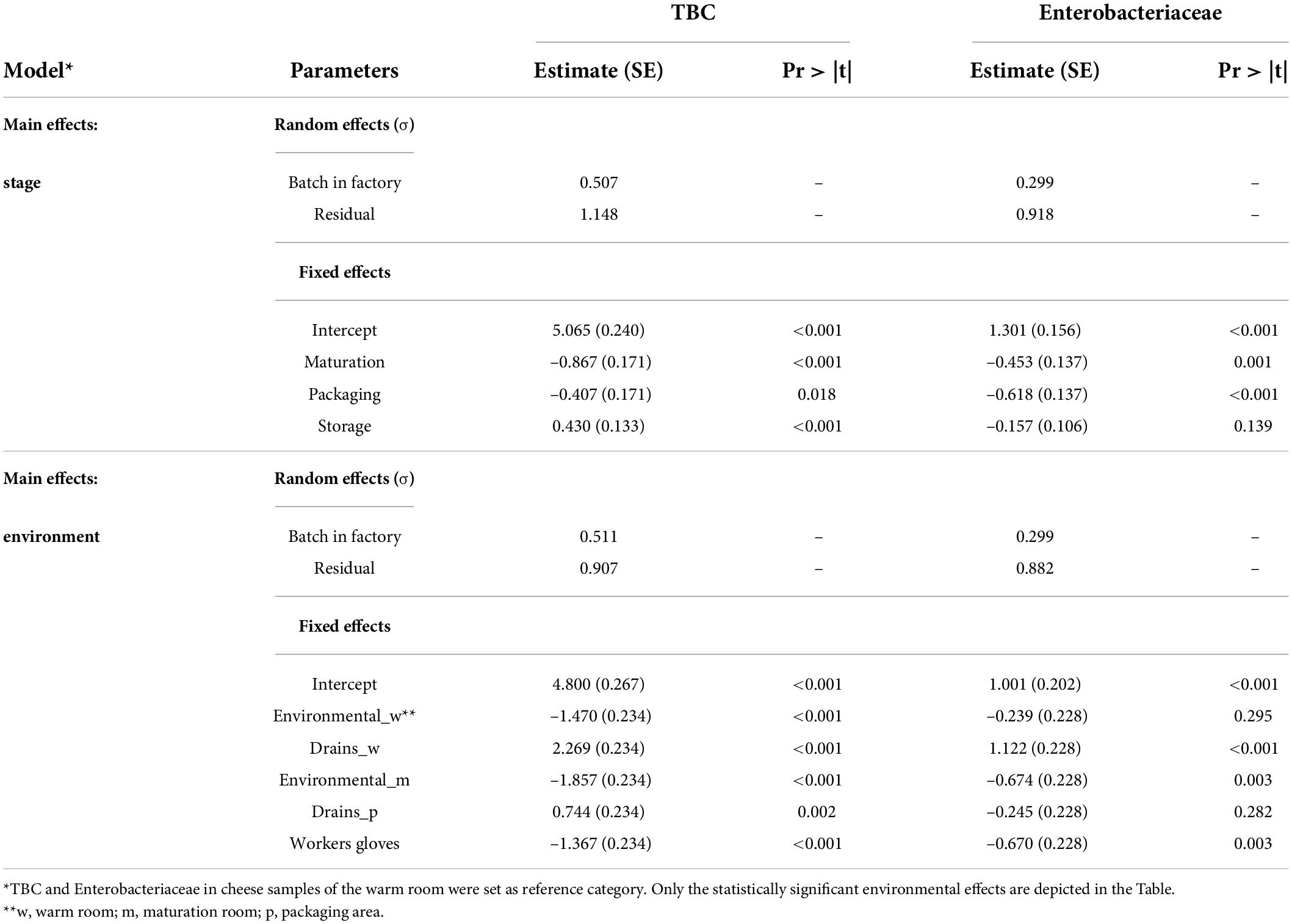
Table 4. Parameter estimates of the linear mixed models with random effects assessing the variables “Stage” and “Environment” as main effects on total bacterial counts (TBCs) and Enterobacteriaceae counts in the artisanal soft cheese, as well as estimates of inter-batch variability.
Results from GLMMs indicated that the storage stage had a significant positive effect on TBC in cheeses (0.430 ± 0.133), while drains in the warm room (2.269 ± 0.234) and the packaging area (0.744 ± 0.234) were also associated with higher levels of TBC in the products. In addition, drains in the warm room also had a positive effect on Enterobacteriaceae loads in cheeses (1.122 ± 0.228). Regarding the inter-batch variability, random effects indicate a higher variability between batches with respect to TBC compared to Enterobacteriaceae.
Occurrence of bacterial pathogens
Regarding isolation of bacterial pathogens, out of the 720 samples analyzed, L. monocytogenes and VTEC were not found. Six isolates were positive for S. aureus and one for Salmonella spp. Regarding Enterobacteriaceae, 166 isolates were identified at species level, with most isolates belonging to Klebsiella oxytoca and K. pneumoniae (44), Enterobacter cloacae (39), Hafnia alvei (26), and Citrobacter freundii (21) (Table 5). Hafnia alvei is generally described as commensal bacteria. However, in some circumstances, they have been reported as pathogenic (Günthard and Pennekamp, 1996). Bacteria were collected from cheese and swab environmental samples along production and during storage with a particular lower occurrence in batch 1. The latter showed a lower load of TBC from milk after pasteurization (2.65 log cfu/g) (Table 1), through packed cheese (3.53 log cfu/g) and cheese after 15 days of storage (5.37 log cfu/g at 2°C, 4.58 log cfu/g at 8°C, and 3.99 log cfu/g at 2/8°C) (Table 2).
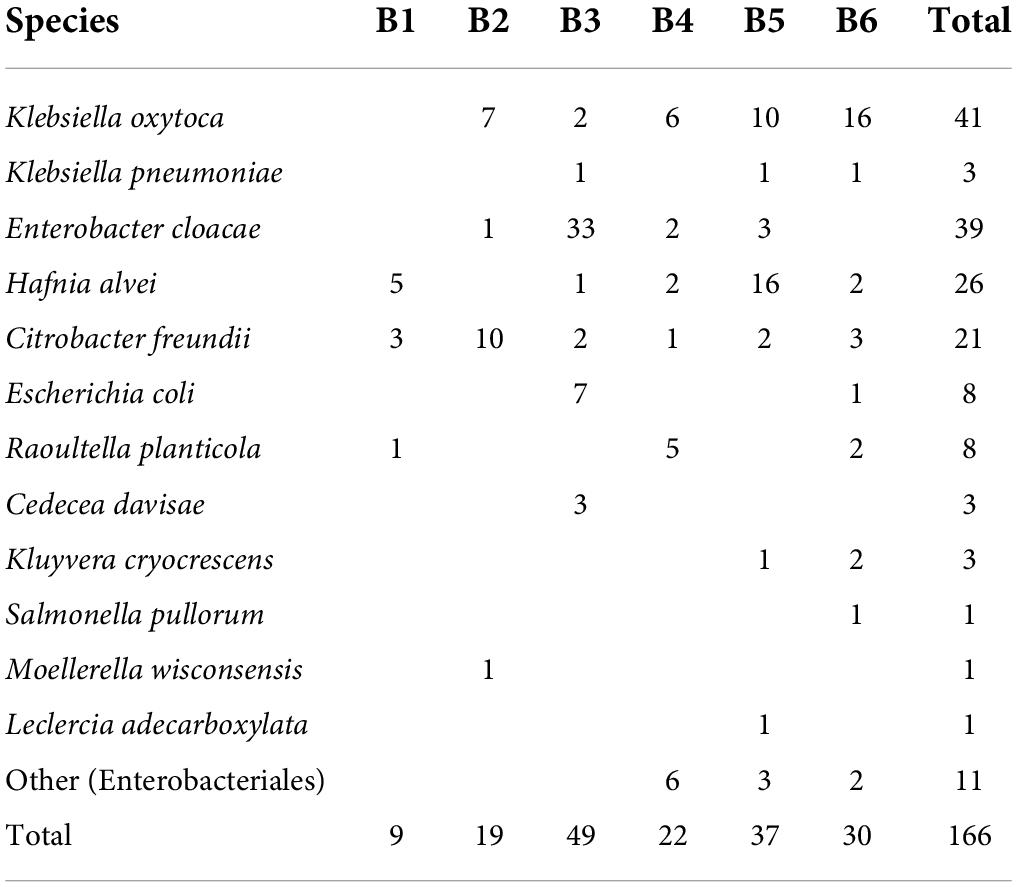
Table 5. Results of the species identification from the 166 Enterobacteriaceae isolates in the six tested batches (B1–B6).
S. aureus and Salmonella spp. were isolated from batches 2, 5, and 6. Specifically, six isolates of S. aureus were collected in cheese during storage at 2°C (batch 2), 8°C (batch 5), and 2/8°C (batch 6), and one isolate of Salmonella spp. was collected from cheese at the maturation room in batch 6 (Table 5). In 2020, Salmonella spp. maintained its position as the first causative agent of foodborne outbreaks in Europe (EFSA and ECDC (European Food Safety Authority and European Centre for Disease Prevention and Control)., 2021). In the last 20 years, serovars Enteritidis, Stanley, Typhimurium, Muenster, Montevideo, Dublin, and Newport were identified as causative agents of salmonellosis outbreaks associated with the consumption of contaminated cheese of different types (soft and hard, from cow, goat, and sheep milk mainly unpasteurized) in Italy, Switzerland, the Netherlands, and France (Pastore et al., 2008; Dominguez et al., 2009; van Cauteren et al., 2009; Van Duynhoven et al., 2009; Robinson et al., 2018; Ung et al., 2019; Napoleoni et al., 2021). Of relevance, in this study one isolate of Salmonella spp. was collected from cheese in the maturation room in batch 6. Although no further Salmonella spp. isolates were collected in cheese during storage, this finding evidence specific concerns for food safety.
S. aureus has been associated with bovine mastitis (Barkema et al., 2006). Raw cows’ milk might be a source of introduction of S. aureus within the dairy production chain. Food handlers might be another source since S. aureus is part of the common microbiota found on the skin and mucous membranes where it is predominantly methicillin-susceptible (Strommenger et al., 2018). In Italian artisanal cheeses, an occurrence of 80% has been recently described, although outbreaks due to the consumption of contaminated cheese are rarely reported (Johler et al., 2015a,2018). The occurrence of this pathogen was also described in this study: six isolates of S. aureus were collected from soft cheese of batches 5 and 6 during storage. Further investigations should be performed to evaluate the source of cheese contamination (i.e., milk, food handlers). Moreover, further characterizations of S. aureus enterotoxins and antimicrobial susceptibility are needed to assess the potential pathogenicity of collected isolates and their similarity to the methicillin-resistant S. aureus mainly found in hospitals.
Regarding Enterobacteriaceae, Klebsiella spp. isolates were the most abundant identified from all batches but one (batch 1) (Table 5). Batch 6 harbored the majority of K. oxytoca isolates (Table 6). Accordingly, batch 6 was characterized by the highest load of Enterobacteriaceae (reaching 3.06 log cfu/g at day 15 of storage at 8°C). Regarding the different steps of processing, all K. oxytoca isolates were collected in the warm and maturation rooms and the packaging area both in food and the environment (data not shown). Regarding storage of cheese samples, Klebsiella spp. was collected from day 1 to day 15 at all three tested temperatures. Abuse temperatures were not correlated to a higher occurrence of Klebsiella spp., indicating its ability to survive in soft cheese also at 2°C. K. pneumoniae and K. oxytoca have not been previously associated to foodborne-confirmed human cases. These species might cause bovine mastitis in dairy cows (Massé et al., 2020). These microorganisms are also found in soil environments and as saprophyte in the nasal cavity and in the intestinal tract. Pathogenic K. pneumoniae and K. oxytoca are often linked to nosocomial outbreaks of particular concern due to their multi-resistance character (Podschun and Ullmann, 1998). In this study, all isolates were resistant to sulfamethoxazole and all but one to ampicillin, and none was resistant to meropenem (Table 5). Both penicillins and sulfonamides are among the three antimicrobial agents most sold in Europe for food-producing animals [European Surveillance of Veterinary Antimicrobial Consumption (ESVAC), 2021]. The isolate collected from the environment of the warm room in batch 6 was resistant to sulfamethoxazole, ciprofloxacin, nalidixic acid, colistin, ampicillin, and gentamicin. The isolate collected from a cheese sample of batch 6 in the first day of storage at 2°C was resistant to sulfamethoxazole, colistin, and ampicillin.
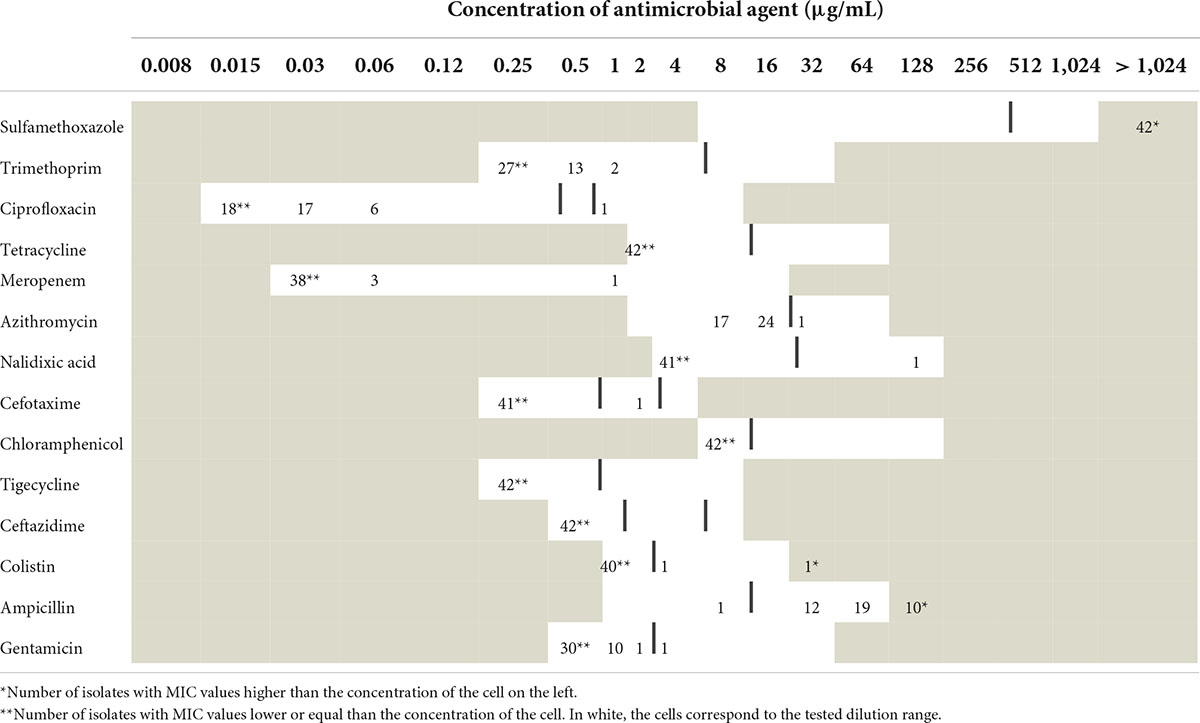
Table 6. Distribution of MIC values in Klebsiella spp. isolates (vertical lines—clinical breakpoints).
In foods, Klebsiella oxytoca and K. pneumoniae were described as spoilage microorganisms of mozzarella cheese (Massa et al., 1992). In this study, 42 isolates of both species belonging to environmental and food samples were found, with a prevalence of 5.8% (42/720). Klebsiella isolates were selected after pre-enrichment of samples. This consideration can explain why they were isolated in batches in which the Enterobacteriaceae counts, performed without enrichment, were under the detection limit in all cheese samples collected at the packaging area and during storage. Although on a significantly lower number of samples (87), higher occurrences (43%) in dairy processing plants have been reported in Czechia by Gelbíčová et al. (2021), who isolated Klebsiella spp. in the processing environment, personnel, and raw material. None of the Czech strains were hypervirulent or multidrug-resistant. Further investigations should be performed on Klebsiella spp. isolates of this study, to evaluate the origin of cheese contamination (i.e., milk, personnel, processing environment), their antimicrobial susceptibility, and potential pathogenicity.
Similar to Klebsiella spp., E. cloacae, Citrobacter freundii, and Hafnia alvei were detected in cheese and environmental samples from the maturation room up to the end of processing and during storage. E. cloacae is widely encountered in nature. Similar to Klebsiella spp., some strains have been described as associated to mastitis in dairy cows and nosocomial infections of particular concern due to their resistance to antimicrobial agents including critical important ones of the highest priority (colistin, extended-spectrum cephalosporins, and carbapenems) (Schukken et al., 2012; Davin-Regli and Pagès, 2015). In foods, E. cloacae was collected from cheese and infant milk formulas (Maifreni et al., 2013; Amorim and Nascimento, 2017). In this study, 39 E. cloacae isolates were collected of which 33 were found in samples from batch 3. In this batch, E. cloacae was isolated during processing and storage at all three temperatures tested in cheese and environmental samples.
H. alvei and C. freundii are common inhabitants of the gastrointestinal tract, although, rarely, they have been associated to human infections. H. alvei was associated to respiratory tract infections (Ramos-Vivas, 2020), whereas C. freundii was associated to different human infections from urinary tract infections to bloodstream (Liu et al., 2018). In this study, 26 isolates of H. alvei and 21 of C. freundii were collected from batches 5 and 2, respectively. In the literature, H. alvei and C. freundii were isolated during cheese processing and ripening (Maifreni et al., 2013; Tabla et al., 2018).
Conclusion
In conclusion, high inter-batch variability was observed among six batches of artisanal soft cheese production with batch 6 showing the highest Enterobacteriaceae counts at the end of the storage period of 15 days at 8°C. Isolation of Salmonella, S. aureus, K. pneumoniae and K. oxytoca, E. cloacae, H. alvei, and C. freundii after pre-enrichment in all batches during processing and storage suggests the need to focus attention on standardizing the processing steps from pasteurization to hygienic procedures. This includes cleaning and disinfection especially of warm and maturation rooms and the microbial quality and fat content of cows’ milk. Moreover, attention should be addressed to identify Enterobacteriaceae species occurring during processing and storage. Further studies should be performed to investigate the potential pathogenicity and antimicrobial resistance of the identified Enterobacteriaceae species in artisanal cheeses.
Data availability statement
The original contributions presented in the study are included in the article/supplementary files, further inquiries can be directed to the corresponding author/s.
Author contributions
FP contributed to data analysis, performed the literature search, and drafted the manuscript. AV and AP performed data and statistical analyses. CC and LG contributed to microbiological analyses. AL analyses of molecular biology. GM and AD contributed to data analysis. AD supervised the work. All authors contributed to data interpretation, manuscript revision and approved the final version as submitted.
Funding
This study was funded by EU H2020 PRIMA ARTISANEFOOD project “Innovative Bio-interventions and Risk Modeling Approaches for Ensuring Microbial Safety and Quality of Mediterranean Artisanal Fermented Foods.”
Conflict of interest
The authors declare that the research was conducted in the absence of any commercial or financial relationships that could be construed as a potential conflict of interest.
Publisher’s note
All claims expressed in this article are solely those of the authors and do not necessarily represent those of their affiliated organizations, or those of the publisher, the editors and the reviewers. Any product that may be evaluated in this article, or claim that may be made by its manufacturer, is not guaranteed or endorsed by the publisher.
References
Ahmed, M. E., Rathnakumar, K., Awasti, N., Elfaruk, M. S., and Hammam, A. R. A. (2021). Influence of probiotic adjunct cultures on the characteristics of low-fat Feta cheese. Food Sci. Nutr. 9, 1512–1520. doi: 10.1002/fsn3.2121
Amorim, A. M. B., and Nascimento, J. D. S. (2017). A highlight for non-Escherichia coli and non-Salmonella sp. Enterobacteriaceae in dairy foods contamination. Front. Microbiol. 8:930. doi: 10.3389/fmicb.2017.00930
Barkema, H. W., Schukken, Y. H., and Zadoks, R. N. J. (2006). Invited review: The role of cow, pathogen, and treatment regimen in the therapeutic success of bovine Staphylococcus aureus mastitis. J. Dairy Sci. 89, 1877–1895. doi: 10.3168/jds.S0022-0302(06)72256-1
Barukèiæ, I., Šèetar, M., Marasoviæ, I., Lisak Jakopoviæ, K., Galiæ, K., and Božaniæ, R. (2020). Evaluation of quality parameters and shelf life of fresh cheese packed under modified atmosphere. J. Food Sci. Technol. 57, 2722–2731. doi: 10.1007/s13197-020-04308-6
Bates, D., Mäechler, M., Bolker, B., and Walker, S. (2015). Fitting linear mixed-effects models using lme4. J. Stat. Softw. 67, 1–48. doi: 10.18637/jss.v067.i01
Castro, A., Santos, C., Meireles, H., Silva, J., and Teixeira, P. (2016). Food handlers as potential sources of dissemination of virulent strains of Staphylococcus aureus in the community. J. Infect. Public Health 9, 153–160. doi: 10.1016/j.jiph.2015.08.001
Chander, Y., Ramakrishnan, M. A., Jindal, N., Hanson, K., and Goyal, S. M. (2011). Differentiation of Klebsiella pneumoniae and K. oxytoca by multiplex polymerase chain reaction. Intern. J. Appl. Res. Vet. Med. 9:138.
Cirne, C. T., Tunick, M. H., and Trout, R. E. (2019). The chemical and attitudinal differences between commercial and artisanal products. NPJ Sci. Food 3:19. doi: 10.1038/s41538-019-0053-9
Costard, S., Espejo, L., Groenendaal, H., and Zagmutt, F. J. (2017). Outbreak-related disease burden associated with consumption of unpasteurized cow’s milk and cheese, United States, 2009–2014. Emerg. Infect. Dis. 23, 957–964. doi: 10.3201/eid2306.151603
Davin-Regli, A., and Pagès, J. M. (2015). Enterobacter aerogenes and Enterobacter cloacae; versatile bacterial pathogens confronting antibiotic treatment. Front. Microbiol. 6:392. doi: 10.3389/fmicb.2015.00392
Dominguez, M., Jourdan-Da Silva, N., Vaillant, V., Pihier, N., Kermin, C., Weill, F. X., et al. (2009). Outbreak of Salmonella enterica serotype Montevideo infections in France linked to consumption of cheese made from raw milk. Foodborne Pathog. Dis. 6, 121–128. doi: 10.1089/fpd.2008.0086
Dos Santos Rosario, A. I. L., da Silva Mutz, Y., Castro, V. S., da Silva, M. C. A., Conte-Junior, C. A., and da Costa, M. P. (2021). Everybody loves cheese: Crosslink between persistence and virulence of Shiga-toxin Escherichia coli. Crit. Rev. Food Sci. Nutr. 61, 1877–1899. doi: 10.1080/10408398.2020.1767033
EFSA and ECDC (European Food Safety Authority and European Centre for Disease Prevention and Control). (2021). The European Union one health 2020 zoonoses report. EFSA J. 19:6971. doi: 10.2903/j.efsa.2021.6971
European Commission [EC] (2022). eAmbrosia – the EU Geographical Indications Register. Available Online at: https://ec.europa.eu/info/food-farming-fisheries/food-safety-and-quality/certification/quality-labels/geographical-indications-register/ [accessed February 18, 2022].
European Surveillance of Veterinary Antimicrobial Consumption [ESVAC] (2021). Sales of veterinary antimicrobial agents in 31 European countries in 2019 and 2020. Available Online at: https://www.ema.europa.eu/en/documents/report/sales-veterinary-antimicrobial-agents-31-european-countries-2019-2020-trends-2010-2020-eleventh_en.pdf (accessed June 24, 2022).
European Union [EU] (2004). Regulation (EU) No 853/2004 of the European Parliament and of the Council of 29 April 2004 laying down specific hygiene rules for on the hygiene of foodstuffs. Off. J. Eur. Union 139, 55–205.
European Union [EU] (2007). Regulation (EU) No. No 1441/2007of 5 December 2007 amending regulation (EC) No 2073/2005 on microbiological criteria for foodstuff. Off. J. Eur. Union 322, 12–29.
European Union [EU] (2012). Regulation (EU) No. 1151/2012 of the European Parliament and of the Council of 21 November 2012 on quality schemes for agricultural products and foodstuffs. Off. J. Eur. Union 343, 1–29.
Fusco, V., Chieffi, D., Fanelli, F., Logrieco, A. F., Cho, G.-S., Kabisch, J., et al. (2020). Microbial quality and safety of milk and milk products in the 21st century. Compr. Rev. Food Sci. Food Saf. 19, 2013–2049. doi: 10.1111/1541-4337.12568
Gelbíčová, T., Kořená, K., Pospíšilová-Hlucháňová, L., Straková, N., and Karpíšková, R. (2021). Dissemination and characteristics of Klebsiella spp. at processed cheese plant. Czech J. Food Sci. 39, 113–121. doi: 10.17221/232/2020-CJFS
Günthard, H., and Pennekamp, A. (1996). Clinical significance of extraintestinal Hafnia alvei isolates from 61 patients and review of the literature. Clin. Infect. Dis. 22, 1040–1045. doi: 10.1093/clinids/22.6.1040
ISO (1998). Microbiology of food and animal feeding stuffs. Horizontal method for the numeration of mesophilic lactic acid bacteria - Colony-count technique at 30 degrees C. ISO Norm 15214:1998. Geneva: International Standardization Organization.
ISO (1999). International standards meat and meat products. Measurement of the pH (reference method). ISO Norm 2917:1999. Geneva: International Standardization Organization.
ISO (2001). Microbiology of the food chain — Horizontal method for the enumeration of beta-glucuronidase-positive Escherichia coli — Part 2: Colony-count technique at 44 degrees C using 5-bromo-4-chloro-3-indolyl beta-D-glucuronide. ISO Norm 16649:2001. Geneva: International Standardization Organization.
ISO (2004a). Microbiology of food and animal feeding stuffs. Determination of water activity. ISO Norm 21807:2004. Geneva: International Standardization Organization.
ISO (2004b). Microbiology of food and animal feeding stuffs. Horizontal method for the enumeration of coagulase-positive staphylococci (Staphylococcus aureus and other species) - Part 1: Technique using Baird-Parker agar medium. ISO Norm ISO 6888-1/A1:2004. Geneva: International Standardization Organization.
ISO (2013). Microbiology of the food chain. Horizontal method for the enumeration of microorganisms - Part 2: Colony count at 30 °C by the surface plating technique. ISO Norm 4833-2:2013. Geneva: International Standardization Organization.
ISO (2017c). Microbiology of the food chain. Horizontal method for the detection, enumeration and serotyping of Salmonella - Part 1: Detection of Salmonella spp. ISO Norm 6579-1:2017. Geneva: International Standardization Organization.
ISO (2017b). Microbiology of the food chain. Horizontal method for the detection and enumeration of Listeria monocytogenes and of Listeria spp. - Part 1: Detection method. ISO Norm 11290-1:2017. Geneva: International Standardization Organization.
ISO (2017a). Microbiology of the food chain. Horizontal method for the detection and enumeration of Enterobacteriaceae - Part 2: Colony-count technique. ISO Norm 21528-2:2017. Geneva: International Standardization Organization.
Jackson, K. A., Gould, L. H., Hunter, J. C., Kucerova, Z., and Jackson, B. (2018). Listeriosis outbreaks associated with soft cheeses, United States, 1998–2014. Emerg. Infect. Dis. 24, 1116–1118. doi: 10.3201/eid2406.171051
Jaramillo-Bedoya, E., Trujillo-Alzate, Y. A., and Ocampo-Ibáñez, I. D. (2021). Surveillance of fresh artisanal cheeses revealed high levels of Listeria monocytogenes contamination in the department of Quindío, Colombia. Pathogens 10:1341. doi: 10.3390/pathogens10101341
Johler, S., Macori, G., Bellio, A., Acutis, P. L., Gallina, S., and Decastelli, L. (2018). Characterization of Staphylococcus aureus isolated along the raw milk cheese production process in artisan dairies in Italy. J. Dairy Sci. 101, 2915–2920. doi: 10.3168/jds.2017-13815
Johler, S., Giannini, P., Jermini, M., Hummerjohann, J., Baumgartner, A., and Stephan, R. (2015a). Further evidence for staphylococcal food poisoning outbreaks caused by egc-encoded enterotoxins. Toxins 7, 997–1004. doi: 10.3390/toxins7030997
Johler, S., Weder, D., Bridy, C., Huguenin, M. C., Robert, L., Hummerjohann, J., et al. (2015b). Outbreak of staphylococcal food poisoning among children and staff at a Swiss boarding school due to soft cheese made from raw milk. J. Dairy Sci. 98, 2944–2948. doi: 10.3168/jds.2014-9123
Jones, G., Lefèvre, S., Donguy, M. P., Nisavanh, A., Terpant, G., Fougère, E., et al. (2019). Outbreak of Shiga toxin-producing Escherichia coli (STEC) O26 paediatric haemolytic uraemic syndrome (HUS) cases associated with the consumption of soft raw cow’s milk cheeses, France, March to May 2019. Euro Surveill. 24:1900305. doi: 10.2807/1560-7917.ES.2019.24.22.1900305
Kasrazadeh, M., and Genigeorgis, C. (1994). Potential growth and control of Salmonella in Hispanic type soft cheese. Int. J. Food Microbiol. 22, 127–140. doi: 10.1016/0168-1605(94)90137-6
Lante, A., Lomolino, G., Cagnin, M., and Spettoli, P. (2006). Contentand characterisation of minerals in milk and in Crescenza and Squacquerone Italian fresh cheeses by ICP-OES. Food Control 17, 229–233. doi: 10.1016/j.foodcont.2004.10.010
Liu, L. H., Wang, N. Y., Wu, A. Y., Lin, C. C., Lee, C. M., and Liu, C. P. (2018). Citrobacter freundii bacteremia: Risk factors of mortality and prevalence of resistance genes. J. Microbiol. Immunol. Infect. 51, 565–572. doi: 10.1016/j.jmii.2016.08.016
Maifreni, M., Frigo, F., Bartolomeoli, I., Innocente, N., Biasutti, M., and Marino, M. (2013). Identification of the Enterobacteriaceae in Montasio cheese and assessment of their amino acid decarboxylase activity. J. Dairy Res. 80, 122–127. doi: 10.1017/S002202991200074X
Martinez-Rios, V., and Dalgaard, P. (2018). Prevalence of Listeria monocytogenes in European cheeses: A systematic review and meta-analysis. Food Control 84, 205–214. doi: 10.1016/j.foodcont.2017.07.020
Massa, S., Gardini, F., Sinigaglia, M., and Guerzoni, M. E. (1992). Klebsiella pneumoniae as a spoilage organism in mozzarella cheese. J. Dairy Sci. 75, 1411–1414. doi: 10.3168/jds.S0022-0302(92)77894-1
Massé, J., Dufour, S., and Archambault, M. (2020). Characterization of Klebsiella isolates obtained from clinical mastitis cases in dairy cattle. J. Dairy Sci. 103, 3392–3400. doi: 10.3168/jds.2019-17324
Mohammed, S., Eshetu, M., Tadesse, Y., and Hailu, Y. (2019). Rheological properties and shelf life of soft cheese made from camel milk using camel chymosin. Dairy Vet. Sci. J. 10:555794. doi: 10.19080/JDVS.2019.10.555794
Napoleoni, M., Villa, L., Barco, L., Busani, L., Cibin, V., Lucarelli, C., et al. (2021). A strong evidence outbreak of Salmonella Enteritidis in central Italy linked to the consumption of contaminated raw sheep milk cheese. Microorganisms 9:2464. doi: 10.3390/microorganisms9122464
Pantoja, J. C. F., Reinemann, D. J., and Ruegg, P. L. (2009). Associations among milk quality indicators in raw bulk milk. J. Dairy Sci. 92, 4978–4987.
Pappa, E. C., Bontinis, T. G., Tasioula-Margari, M., and Samelis, J. (2017). Microbial quality of and biochemical changes in fresh soft, acid-curd xinotyri cheese made from raw or pasteurized goat’s milk. Food Technol. Biotechnol. 55, 496–510. doi: 10.17113/ftb.55.04.17.5338
Pastore, R., Schmid, H., Altpeter, E., Baumgartner, A., Hächler, H., Imhof, R., et al. (2008). Outbreak of Salmonella serovar Stanley infections in Switzerland linked to locally produced soft cheese, September 2006 - February 2007. Euro Surveill. 13:18979. doi: 10.2807/ese.13.37.18979-en
Perelle, S., Dilasser, F., Grout, J., and Fach, P. (2004). Detection by 5’-nuclease PCR of Shiga-toxin producing Escherichia coli O26, O55, O91, O103, O111, O113, O145 and O157:H7, associated with the world’s most frequent clinical cases. Mol. Cell. Probes 18, 185–192. doi: 10.1016/j.mcp.2003.12.004
Podschun, R., and Ullmann, U. (1998). Klebsiella spp. as nosocomial pathogens: Epidemiology, taxonomy, typing methods, and pathogenicity factors. Clin. Microbiol. Rev. 11, 589–603. doi: 10.1128/CMR.11.4.589
R Core Team (2020). R: A Language and Environment for Statistical Computing. Vienna: R Foundation for Statistical Computing.
Rabello, R. F., Moreira, B. M., Lopes, R. M., Teixeira, L. M., Riley, L. W., and Castro, A. C. (2007). Multilocus sequence typing of Staphylococcus aureus isolates recovered from cows with mastitis in Brazilian dairy herds. J. Med. Microbiol. 56, 1505–1511. doi: 10.1099/jmm.0.47357-0
Ramos-Vivas, J. (2020). Microbiology of Hafnia alvei. Enferm. Infecc. Microbiol. Clin. 38, (Suppl. 1), 1–6. doi: 10.1016/j.eimc.2020.02.001
Robinson, E., Travanut, M., Fabre, L., Larréché, S., Ramelli, L., Pascal, L., et al. (2018). Outbreak of Salmonella Newport associated with internationally distributed raw goats’ milk cheese, France, 2018. Epidemiol. Infect. 148:e180. doi: 10.1017/S0950268820000904
Saraiva, M. M., De Leon, C. M., Santos, S. C., Stipp, D. T., Souza, M. M., Santos Filho, L., et al. (2018). Accuracy of PCR targeting different markers for Staphylococcus aureus identification: A comparative study using matrix-assisted laser desorption/ionization time-of-flight mass spectrometry as the gold standard. J. Vet. Diagn. Invest. 30, 252–255. doi: 10.1177/1040638717732370
Schukken, Y., Chuff, M., Moroni, P., Gurjar, A., Santisteban, C., Welcome, F., et al. (2012). The “other” gram-negative bacteria in mastitis: Klebsiella, Serratia, and more. Vet. Clin. North Am. Food Anim. Pract. 28, 239–256. doi: 10.1016/j.cvfa.2012.04.001
Silva, M. P., Carvalho, A. F., Andretta, M., and Nero, L. A. (2021). Presence and growth prediction of Staphylococcus spp. and Staphylococcus aureus in Minas Frescal cheese, a soft fresh cheese produced in Brazil. J. Dairy Sci. 104, 12312–12320. doi: 10.3168/jds.2021-20633
Strommenger, B., Layer, F., and Werner, G. (2018). “Chapter 9 - Staphylococcus aureus and methicillin-resistant Staphylococcus aureus in workers in the food industry,” in Staphylococcus aureus, ed. A. Fetsch (Cambridge, MA: Academic Press), 163–188. doi: 10.1016/B978-0-12-809671-0.00009-7
Tabla, R., Gómez, A., Simancas, A., Rebollo, J. E., Molina, F., and Roa, I. (2018). Early blowing in raw goats’ milk cheese: Gas production capacity of Enterobacteriaceae species present during manufacturing and ripening. J. Dairy Res. 85, 331–338. doi: 10.1017/S0022029918000511
Tan, W. S., Budinich, M. F., Ward, R., Broadbent, J. R., and Steele, J. L. (2012). Optimal growth of Lactobacillus casei in a Cheddar cheese ripening model system requires exogenous fatty acids. J. Dairy Sci. 95, 1680–1689. doi: 10.3168/jds.2011-4847
Tavakoli, M., Habibi Najafi, M. B., and Mohebbi, M. (2019). Effect of the milk fat content and starter culture selection on proteolysis and antioxidant activity of probiotic yogurt. Heliyon 5:e01204. doi: 10.1016/j.heliyon.2019.e01204
Ung, A., Baidjoe, A. Y., Van Cauteren, D., Fawal, N., Fabre, L., Guerrisi, C., et al. (2019). Disentangling a complex nationwide Salmonella Dublin outbreak associated with raw-milk cheese consumption, France, 2015 to 2016. Euro Surveill. 24:1700703. doi: 10.2807/1560-7917.ES.2019.24.3.1700703
van Cauteren, D., Jourdan-da Silva, N., Weill, F. X., King, L., Brisabois, A., Delmas, G., et al. (2009). Outbreak of Salmonella enterica serotype Muenster infections associated with goat’s cheese, France, March 2008. Euro Surveill. 14:19290. doi: 10.2807/ese.14.31.19290-en
Van Duynhoven, Y. T., Isken, L. D., Borgen, K., Besselse, M., Soethoudt, K., Haitsma, O., et al. (2009). Outbreak Investigation Team. A prolonged outbreak of Salmonella Typhimurium infection related to an uncommon vehicle: Hard cheese made from raw milk. Epidemiol. Infect. 137, 1548–1557. doi: 10.1017/S0950268809002337
Wesley, I. V., Harmon, K. M., Dickson, J. S., and Schwartz, A. R. (2002). Application of a multiplex polymerase chain reaction assay for the simultaneous confirmation of Listeria monocytogenes and other Listeria species in turkey sample surveillance. J. Food Prot. 65, 780–785. doi: 10.4315/0362-028x-65.5.780
Keywords: microbiological quality, bacterial pathogens, soft cheese, processing environment, artisanal production
Citation: Pasquali F, Valero A, Possas A, Lucchi A, Crippa C, Gambi L, Manfreda G and De Cesare A (2022) Occurrence of foodborne pathogens in Italian soft artisanal cheeses displaying different intra- and inter-batch variability of physicochemical and microbiological parameters. Front. Microbiol. 13:959648. doi: 10.3389/fmicb.2022.959648
Received: 01 June 2022; Accepted: 26 July 2022;
Published: 25 August 2022.
Edited by:
Alicia Rodríguez, University of Extremadura, SpainReviewed by:
Rashed Noor, Independent University, Bangladesh, BangladeshDon Schaffner, Rutgers, The State University of New Jersey, United States
Copyright © 2022 Pasquali, Valero, Possas, Lucchi, Crippa, Gambi, Manfreda and De Cesare. This is an open-access article distributed under the terms of the Creative Commons Attribution License (CC BY). The use, distribution or reproduction in other forums is permitted, provided the original author(s) and the copyright owner(s) are credited and that the original publication in this journal is cited, in accordance with accepted academic practice. No use, distribution or reproduction is permitted which does not comply with these terms.
*Correspondence: Antonio Valero, YnQydmFkaWFAdWNvLmVz