- 1Maternal and Child Health Development Research Center, Shandong Provincial Maternal and Child Health Care Hospital, Jinan, China
- 2Pharmaceutical Department, Shandong Provincial Taishan Hospital, Taian, China
- 3Department of Ophthalmology, Shandong Provincial Maternal and Child Health Care Hospital, Jinan, China
- 4Physical Examination Center, Shandong Provincial Maternal and Child Health Care Hospital, Jinan, China
- 5Department of Clinical Laboratory, Shandong Provincial Maternal and Child Health Care Hospital, Jinan, China
- 6Department of Pediatric Surgery, Shandong Provincial Maternal and Child Health Care Hospital, Jinan, China
β-Lactams have been a clinical focus since their emergence and indeed act as a powerful tool to combat severe bacterial infections, but their effectiveness is threatened by drug resistance in bacteria, primarily by the production of serine- and metallo-β-lactamases. Although once of less clinical relevance, metallo-β-lactamases are now increasingly threatening. The rapid dissemination of resistance mediated by metallo-β-lactamases poses an increasing challenge to public health worldwide and comprises most existing antibacterial chemotherapies. Regrettably, there have been no clinically available inhibitors of metallo-β-lactamases until now. To cope with this unique challenge, researchers are exploring multidimensional strategies to combat metallo-β-lactamases. Several studies have been conducted to develop new drug candidates or calibrate already available drugs against metallo-β-lactamases. To provide an overview of this field and inspire more researchers to explore it further, we outline some promising candidates targeting metallo-β-lactamase producers, with a focus on Escherichia coli, Klebsiella pneumoniae, Pseudomonas aeruginosa, and Acinetobacter baumannii. Promising candidates in this review are composed of new antibacterial drugs, non-antibacterial drugs, antimicrobial peptides, natural products, and zinc chelators, as well as their combinations with existing antibiotics. This review may provide ideas and insight for others to explore candidate metallo-β-lactamases as well as promote the improvement of existing data to obtain further convincing evidence.
Introduction
β-Lactams, mainly penicillins, cephalosporins, carbapenems, and monobactams, are the most important and frequently used classes of antibiotics in medicine and play a vital role in the treatment of severe gram-negative infections. They inhibit the synthesis of bacterial cell walls by mimicking the natural substrates of transpeptidase and preventing cross-linking of adjacent peptidoglycan strands (Bush and Bradford, 2016). In recent years, however, the efficacy of antibiotics has been threatened by a global rise in drug resistance, of which resistance to β-lactams is mainly mediated by β-lactamases (Eurosurveillance Editorial, 2015). Carbapenem-resistant Enterobacteriaceae, carbapenem-resistant Pseudomonas aeruginosa (P. aeruginosa), and carbapenem-resistant Acinetobacter baumannii (A. baumannii) have been listed by the World Health Organization (WHO) as pathogens that urgently need to be studied in research aiming to develop new antibiotics because of the emergence of these drug-resistant pathogens; due to the limited number of treatments, these resistant bacteria pose a serious public health problem (Tangden and Giske, 2015; WHO, 2017).
β-lactamases fall into four categories, namely, Ambler classes A, B, C, and D. Classes A, C, and D feature active-site serine β-lactamases (SBLs), and class B contains zinc-dependent metallo-β-lactamases (MBLs) (Tooke et al., 2019). MBLs require one or two zinc ions for enzyme activity and are further divided into three subclasses (i.e., B1, B2, and B3), based primarily on their metal content and the characteristics of different active sites (Boyd et al., 2020). Among antimicrobial resistance, the expression of β-lactamases, especially MBLs, is of particular interest (Boyd et al., 2020). Most MBLs that have been identified in clinical isolates thus far belong to subclass B1, among which the New Delhi metallo-β-lactamase (NDM), Verona imipenemase (VIM), and imipenemase (IMP) families are the three most common types (Hansen, 2021). MBLs are a particular challenge for several reasons: (i) They have the ability to hydrolyze and develop resistance to virtually all existing β-lactam antibiotics; (ii) there are almost no clinically useful drug regimens for MBLs; (iii) there is a rapid pace of isolation and discovery of new varieties; (iv) their coding genes are easily transferred; and (v) they are ubiquitous in hospitals and the natural environment. Over the past decade, great progress has been made in antibiotic development. Newly approved combinations of β-lactam/β-lactamase inhibitors, such as ceftazidime-avibactam (CAZ-AVI), have emerged as new options for the treatment of drug-resistant bacterial infections related to β-lactamases (Shirley, 2018). However, these new antibiotics only have activity against SBLs, not MBLs, making the fight against MBLs a challenging public health problem (Boyd et al., 2020). Infections caused by MBL-positive isolates not only contribute significantly to increased patient morbidity and mortality, length of hospital stay, and medical costs but also face limited therapeutic options (Boyd et al., 2020). Moreover, the current COVID-19 pandemic further aggravated this scenario (Falcone et al., 2021b; Westblade et al., 2021). Several recent reports have described that COVID-19 patients have an increased risk of MBL-CRE acquisition compared with usual patients, adding to the challenge of managing COVID-19 patients (Farfour et al., 2020; Nori et al., 2020; Porretta et al., 2020). Nori et al. reported five cases of NDM-producing Enterobacterales infections in New York City COVID-19 patients, which may be the first reported cases of NDM emergence in COVID-19 patients (Nori et al., 2020). An outbreak of NDM-5-producing Escherichia coli (E. coli) in a French hospital department dedicated to COVID-19 patients were described, which may be related to the following factors: the multiple-bedroom configuration of the department, uncomplete compliance for standard and contact precautions, overwork due to the burden of the disease, lack of training of staff for the care of ICU patients, and misuse of gloves (Farfour et al., 2020). In an Italian hospital, COVID-19 patients were found to have an increased risk of NDM-CRE acquisition versus the usual patients (75.9 vs. 25.3 cases/10,000 patient days) (Porretta et al., 2020).
Considering that no MBL inhibitors are clinically available to date, there is an urgent and unmet medical need for the development of therapies targeting MBL-producing pathogens. In this study, we summarize the major advances in the development of alternative drugs concerning B1 MBLs (mainly NDM, VIM, and IMP) in Enterobacteriaceae and non-fermenting gram-negative bacteria, with a focus on E. coli and Klebsiella pneumoniae (K. pneumoniae) for the former, as well as a focus on P. aeruginosa and A. baumannii for the latter. The in vitro activity and in vivo efficacy of novel drugs or compounds vs. MBL producers adapted are summarized in Tables 1, 2, respectively.
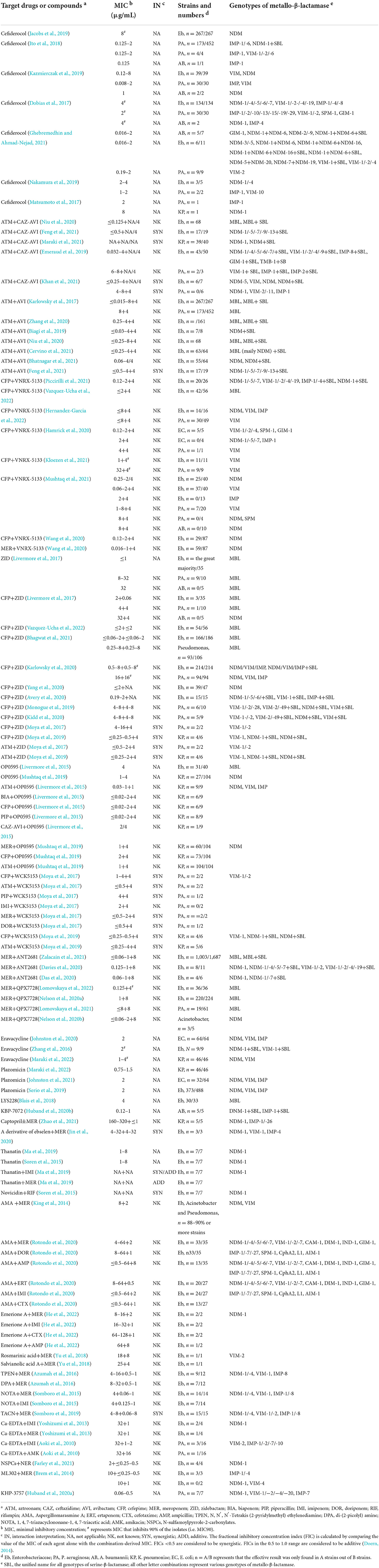
Table 1. In vitro activity of novel drug strategies vs. metallo-β-lactamase producers adapted from references.
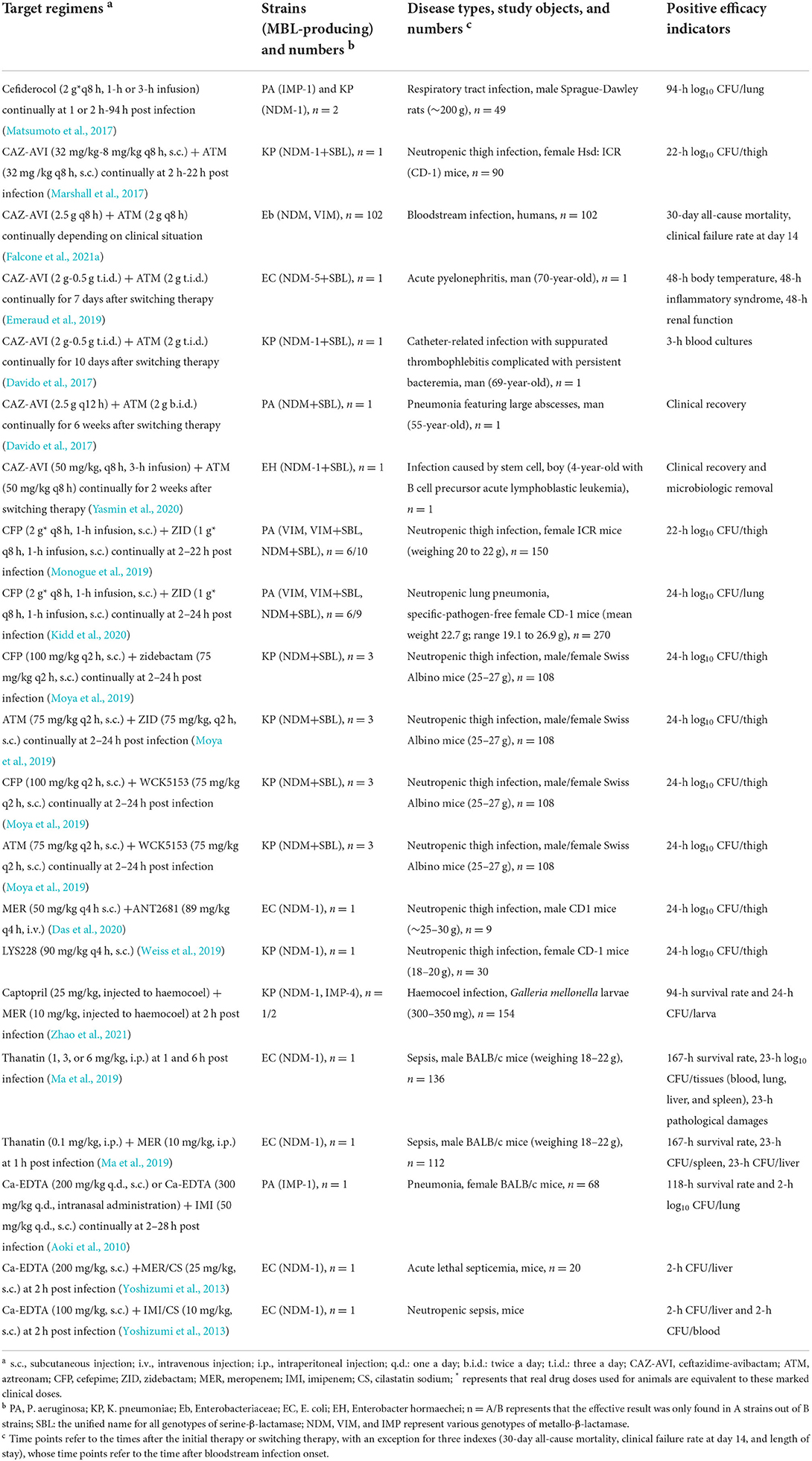
Table 2. In vivo efficacy of novel drug strategies vs. metallo-β-lactamase producers adapted from references.
Novel antibiotics and β-lactamase inhibitors in preclinical stages
There are many ongoing efforts to overcome the resistance mediated by MBLs, one of the most important of which is developing new antibiotics or β-lactamase inhibitors. In this context, attention is primarily given to introducing new antibiotics and combinations of β-lactams and new β-lactamase inhibitors, which exhibit active antibacterial effects against MBL producers.
Cefiderocol
Cefiderocol, a new siderophore cephalosporin that forms a complex with extracellular free iron, is transported through the extracellular membrane and exerts its bactericidal activity by inhibiting cell wall synthesis (Ito et al., 2018). Structurally, cefiderocol is similar to ceftazidime and cefepime, and the biggest highlight lies in the pyrrolidinium group on the C3 side chain and the carboxypropyl-oxyimino group on the C7 side chain, which confer its enhanced antibacterial activity and high stability to numerous β-lactamases of Ambler classes A to D, especially MBLs (Sato and Yamawaki, 2019).
A series of studies indicate that cefiderocol shows strong antibacterial activity against MBL-carrying Enterobacteriaceae, P. aeruginosa, and A. baumannii in vitro. Cefiderocol (0.016–8 μg/ml) has been proven to be effective against MBL-Enterobacteriaceae (Dobias et al., 2017; Matsumoto et al., 2017; Ito et al., 2018; Jacobs et al., 2019; Kazmierczak et al., 2019; Ghebremedhin and Ahmad-Nejad, 2021). Compared with MBL-carrying Enterobacteriaceae, cefiderocol has even more powerful antibacterial activity against MBL-carrying P. aeruginosa and MBL-carrying A. baumannii, with minimal inhibitory concentrations (MICs) in the range of 0.008–8 μg/ml (Dobias et al., 2017; Matsumoto et al., 2017; Ito et al., 2018; Jacobs et al., 2019; Kazmierczak et al., 2019; Nakamura et al., 2019; Ghebremedhin and Ahmad-Nejad, 2021). Moreover, Ghebremedhin et al. found that the antibacterial activity of cefiderocol against the same species of bacteria carrying different MBLs seems to be significantly different (Ghebremedhin and Ahmad-Nejad, 2021). NDM-1/-6-positive isolates (K. pneumoniae and A. baumannii) can be inhibited by cefiderocol at a concentration of ≤2 μg/ml, but this is not the case for NDM-5/-20, NDM-7/NDM-19, or NDM-9 (K. pneumoniae and A. baumannii), which exhibit MIC levels >16 μg/ml for cefiderocol (Ghebremedhin and Ahmad-Nejad, 2021). However, given the small number of strains involved in the study by Ghebremedhin et al., their results need to be interpreted with caution and studied further.
Animal models have also been used to evaluate the antibacterial activity of cefiderocol in vivo (Zhanel et al., 2019). In mouse models of neutropenic thigh and lung infection, cefiderocol (24–800 mg/kg, q24 h) was found to be effective against NDM-1-positive K. pneumoniae and IMP-1-positive P. aeruginosa at 24 h of treatment (Nakamura et al., 2019). In an immunocompetent rat respiratory infection model infected with NDM-1-positive K. pneumoniae and IMP-1-positive P. aeruginosa, cefiderocol (2 g q8 h, 1- or 3-h infusion) was reduced by >3 log10 CFU/lung below that in no drug therapy and ceftazidime (1 g q8 h, 0.5-h infusion) monotherapy (Matsumoto et al., 2017). These results indicate that cefiderocol has potent in vivo efficacy correlated with the in vitro activity against Enterobacteriaceae, P. aeruginosa, and A. baumannii producing MBLs.
Currently, cefiderocol has been approved by the Food and Drug Administration (FDA) and European Medicines Agency against different species of Enterobacteriaceae and non-fermenting gram-negative bacteria (Giacobbe et al., 2020). In summary, cefiderocol represents an interesting new option for multidrug resistance (MDR) gram-negative bacteria, and its structural stability makes it the most attractive new antibiotic currently available for the treatment of MBL producers.
Aztreonam+CAZ-AVI and aztreonam+AVI
Aztreonam was approved by the FDA in 1986 and is the only clinically available monocyclic lactam antibiotic against MBLs due to its structural stability in the presence of MBLs (Ramsey and MacGowan, 2016). However, the majority of MBL-carrying isolates coproduce one or more SBLs, which limits its usefulness as a monotherapy (Cervino et al., 2021). CAZ-AVI is a drug in phase 3 clinical trials and has been recommended by the Infectious Diseases Society of America in combination with aztreonam for the treatment of severe infections caused by MBL-carrying Enterobacteriaceae (Tamma et al., 2021).
As shown in Table 1, in vitro, aztreonam (≤0.03–4 μg/ml)+CAZ-AVI (4 μg/ml of the AVI component) exerts potent synergistic effects against most tested MBL-producing Enterobacteriaceae and few MBL-producing P. aeruginosa, which is superior to aztreonam alone or in combination with other drug combinations, such as aztreonam +amoxillin-clavulanate and aztreonam+ceftolozane-tazobactam (Emeraud et al., 2019; Niu et al., 2020; Khan et al., 2021; Maraki et al., 2021). With further research, the synergistic effect of aztreonam+CAZ-AVI on MBL producers was proven to be mainly caused by the combination of aztreonam and AVI (Feng et al., 2021). AVI is a novel β-lactamase inhibitor that effectively inhibits class A and C carbapenems (Das et al., 2019). The theoretical basis for the inactivation of class A, C, or D β-lactamases by AVI to restore aztreonam susceptibility is supported by its high efficacy in patients with severe infections and without conventional treatment options (Li et al., 2015). Therefore, aztreonam+AVI is considered an attractive combination for the treatment of MBL-positive Enterobacteriaceae. A series of in vitro studies on the combined effects of aztreonam+AVI against MBLs have been carried out. Aztreonam+AVI (≤0.015–4+4 μg/ml) has strong antibacterial activity on Enterobacteriaceae producing MBLs alone or both MBLs and SBLs (Karlowsky et al., 2017; Biagi et al., 2019; Niu et al., 2020; Zhang et al., 2020; Bhatnagar et al., 2021; Cervino et al., 2021; Feng et al., 2021). Karlowsky et al. found that aztreonam+AVI (4 μg/ml+4 μg/ml) also has a good inhibitory effect on a small portion of MBL-P. aeruginosa isolates (63/452) (Karlowsky et al., 2017). To date, no in vitro data have been found on the inhibition of MBL-carrying A. baumannii.
Although a phase 3 clinical trial of aztreonam+AVI is underway (ClinicalTrials.gov:NCT 03329092), in vivo studies of aztreonam+AVI are mainly performed by two FDA-approved drugs, i.e., aztreonam and CAZ-AVI. In vivo efficacy studies in human or animal models validate the solid in vitro data documenting the potential of aztreonam+CAZ-AVI (Table 2). In the neutropenic thigh mice infection model caused by NDM-1+SBLs positive K. pneumoniae, CAZ-AVI (32 mg/kg−8 mg/kg q8 h) plus aztreonam (32 mg/kg q8 h) reduced 24-h log10 CFU/thigh by ~2–4 log10 CFU below that in no drug therapy, CAZ-AVI (32 mg/kg−8 mg/kg q8 h), and CAZ+aztreonam (64 mg/kg q8 h) monotherapy (Marshall et al., 2017). In a relatively large clinical study for patients with bloodstream infections due to NDM- or VIM-producing Enterobacteriaceae, 30-day all-cause mortality was 19.2% for CAZ-AVI (2.5 g q8 h) plus aztreonam (2 g q8 h) vs. 44% for regimens of other active antibiotics, including colistin (4.5 M UI q12 h), tigecycline (100 mg b.i.d.), fosfomycin (4–6 mg q6 h), gentamicin (3–5 mg/kg q.d.), and meropenem (2 g q8 h); clinical failure rate at day 14 was 25% for CAZ-AVI+aztreonam vs. 52% for regimens of other active antibiotics (Falcone et al., 2021a). For a 70-year-old man with acute pyelonephritis and infection caused by NDM-5+SBL-positive E. coli, body temperature, resolution of the inflammatory syndrome, and improvement of renal function were found 48 h after antimicrobial treatment was switched from a regimen comprising colistin, fosfomycin, and gentamicin for 7–10 days to CAZ-AVI (2 g−0.5 g t.i.d.) plus aztreonam (2 g t.i.d.) (Emeraud et al., 2019). Several clinical case reports also show the potent in vivo efficacy of CAZ-AVI plus aztreonam against MBL-producing Enterobacteriaceae and P. aeruginosa. For a 69-year-old man with catheter-related infection due to NDM-1+SBL-positive K. pneumoniae, blood cultures became negative at 3 h after antimicrobial treatment was switched from a regimen comprising colistin, imipenem, fosfomycin, levofloxacin, and amikacin for 26 days to CAZ-AVI (2 g−0.5 g t.i.d.) plus aztreonam (2 g t.i.d.) (Davido et al., 2017). For a 55-year-old man with pneumonia featuring large abscesses caused by NDM+SBL-positive P. aeruginosa, clinical recovery was gained quickly after antimicrobial treatment was switched from a regimen comprising amikacin and colistin to CAZ-AVI (2.5 g q12 h) plus aztreonam (2 g b.i.d.) (Yasmin et al., 2020). For a 4-year-old leukemia boy infected by NDM-1+KPC-4 positive Enterobacter hormaechei due to stem cell, clinical recovery and microbiologic removal were gained at 2 weeks after antimicrobial treatment being switched from a regimen comprising piperacillin-tazobactam+vancomycin or meropenem+vancomycin to CAZ-AVI (50 mg/kg, q8 h, 3-h infusion) plus aztreonam (50 mg/kg q8 h) (Yasmin et al., 2020).
Overall, available data support the combination of aztreonam and AVI (sometimes CAZ-AVI) against MBL-producing Enterobacteriaceae and P. aeruginosa in vitro as well as in vivo, although data on MBL-producing A. baumannii are scarce.
Cefepime+VNRX-5133
VNRX-5133 (taniborbactam) is a new type of β-lactamase inhibitor in clinical development that inhibits important MBLs, including NDM-1 IMP-1 and VIM-1/2 (Krajnc et al., 2019; Lang et al., 2020). It should be noted that VNRX-5133 inhibited IMP less than NDM and VIM (Krajnc et al., 2019). Several in vitro studies have found that VNRX-5133 at 4 μg/ml can restore the antibacterial activity of cefepime against most Enterobacteriaceae producing NDM and VIM (Hamrick et al., 2020; Kloezen et al., 2021; Mushtaq et al., 2021; Piccirilli et al., 2021; Hernandez-Garcia et al., 2022; Vazquez-Ucha et al., 2022). However, cefepime+VNRX-5133 (0.12–8 μg/ml+4 μg/ml) has very weak or no antibacterial activity against Enterobacteriaceae-producing IMP (Hamrick et al., 2020; Wang et al., 2020; Kloezen et al., 2021; Mushtaq et al., 2021; Piccirilli et al., 2021; Hernandez-Garcia et al., 2022; Vazquez-Ucha et al., 2022). In addition, cefepime+VNRX-5133 (4–32 μg/ml+4 μg/ml) showed elevated MIC values against MBL-P. aeruginosa and its antibacterial effect against P. aeruginosa, with an emphasis on VIM producers, not NDM or SPM (Hamrick et al., 2020; Kloezen et al., 2021; Mushtaq et al., 2021; Hernandez-Garcia et al., 2022). No antibacterial activity was found for cefepime+ VNRX-5133 on MBL-producing A. baumannii (Mushtaq et al., 2021). In addition to being used in combination with cefepime, VNRX-5133 (4 μg/ml) can also be used in combination with meropenem (0.016–1 μg/ml) to play a considerable antibacterial role against the most MBL-positive Enterobacteriaceae (Wang et al., 2020).
Although there are no in vivo studies of cefepime+VNRX-5133 against MBLs in animal models, VNRX-5133 in combination with cefepime is currently in phase 3 clinical trials for the treatment of complex urinary tract infections (Dowell et al., 2021). We look forward to the formal clinical application of cefepime+VNRX-5133 in the near future.
Diazabicyclooctanes alone and in combination with antibiotics
In the mid-1990s, chemists first studied diazabicyclooctanes (DBOs) as β-lactamase analogs and demonstrated that they were a rich source of β-lactamase inhibitors (Coleman, 2011). First, DBOs inhibit most or all class A and C β-lactamases, as well as some class D β-lactamases (Mushtaq et al., 2019). AVI is the sole analog of DBOs thus far licensed for clinical use and is combined with CAZ to treat complex intraperitoneal infections and complex urinary tract infections associated with gram-negative bacteria (Shirley, 2018). Since then, DBOs have been promising non-β-lactam inhibitors of β-lactamases (Shlaes, 2013).
Surprisingly, DBOs have also been reported to have anti-MBL activity in recent years (Livermore et al., 2015, 2017). Some novel DBOs not only have synergistic activity with certain antibiotics but also have direct antibacterial activity against MBL producers (Livermore et al., 2017). Several developmental DBOs, notably zidebactam, nacubactam, and WCK5153, have significant affinity for PBP2 of many gram-negative species (Livermore et al., 2015, 2017; Moya et al., 2017). This allows for the antibiotics to exert both a direct antibacterial effect and an “enhancer” mechanism, potentiating partner β-lactams that bind to other PBPs (Livermore et al., 2015, 2017; Moya et al., 2017). This dual function of direct and enhancer-based activity means that combinations of MBL-labile β-lactams with DBOs can retain activity against MBL producers (Mushtaq et al., 2019). These DBOs are reviewed in the following pages.
Zidebactam alone and in combination with antibiotics
Zidebactam, as a new DBO, exerts a dual function involving selectively high affinity for gram-negative bacterial PBP2 binding and β-lactamase inhibition (Bhagwat et al., 2019). By binding to PBP2 binding, zidebactam exhibits antibacterial activity against various Enterobacteriaceae and P. aeruginosa isolates (Sader et al., 2017). In a study conducted by Livermore et al., zidebactam alone at ≤1 μg/ml and at 8–32 μg/ml exerted in vitro antibacterial activity against most of the tested MBL-producing Enterobacteriaceae and MBL-producing P. aeruginosa (Livermore et al., 2017). For MBL-carrying Enterobacteriaceae and P. aeruginosa isolates, zidebactam not only has an antibacterial effect but can also be used as a synergistic agent with antibiotics. Then, more attention was given to zidebactam application as an antibiotic sensitizer, and several studies were carried out to evaluate the in vitro antibacterial activity of cefepime+zidebactam, often described as “WCK5222.” Cefepime+zidebactam (≤0.06–8 μg/ml+ ≤ 0.06–8 μg/ml) was proven to be effective against most of the tested MBL-producing Enterobacteriaceae isolates in vitro (Livermore et al., 2017; Moya et al., 2019; Avery et al., 2020; Karlowsky et al., 2020; Yang et al., 2020; Bhagwat et al., 2021; Vazquez-Ucha et al., 2022). Similar results were shown for MBL-producing P. aeruginosa isolates. Cefepime+zidebactam (≤0.25–16 μg/ml+4–16 μg/ml) can inhibit most of the tested MBL-producing Pseudomonas (mainly P. aeruginosa) isolates (Livermore et al., 2017; Moya et al., 2017; Monogue et al., 2019; Karlowsky et al., 2020; Kidd et al., 2020; Bhagwat et al., 2021). What we want to emphasize is that no obvious inhibitory effect of zidebactam or cefepime+zidebactam was found on MBL-producing A. baumannii isolates (Livermore et al., 2017; Yang et al., 2020). In addition to cefepime, zidebactam (4 μg/ml) can also combine with aztreonam (0.25–2 μg/ml) against MBL-producing K. pneumoniae and MBL-producing P. aeruginosa isolates, which once again proves the potential of zidebactam as an antibiotic sensitizer against MBLs (Moya et al., 2017, 2019).
In vivo studies using animal models also demonstrated the efficacy of cefepime+zidebactam or aztreonam+zidebactam against MBLs. In a neutropenic thigh mouse infection model caused by P. aeruginosa producing VIM, VIM+SBL, or NDM+SBL, 22-h log10 CFU/thigh for cefepime (2 g q8 h, 1-h infusion) plus zidebactam (1 g q8 h, 1-h infusion) was significantly lower than that for no drug therapy, cefepime (2 g q8 h, 1-h infusion) monotherapy, and zidebactam (1 g q8 h, 1-h infusion) monotherapy (Monogue et al., 2019). In neutropenic lung pneumonia mice infected with P. aeruginosa producing VIM, VIM+SBLs, or NDM+SBLs, 24-h log10 CFU/lung for cefepime (2 g q8 h, 1-h infusion) plus zidebactam (1 g q8 h, 1-h infusion) was significantly lower than that for no drug therapy, cefepime (2 g q8 h, 1-h infusion, s.c.) monotherapy, and zidebactam (1 g q8 h, 1-h infusion, s.c.) monotherapy (Kidd et al., 2020). In a neutropenic mouse thigh infection model caused by NDM+SBL-positive K. pneumoniae, 24-h log10 CFU/thigh for cefepime (100 mg/kg q2 h) plus zidebactam (75 mg/kg q2 h) was significantly lower than that for no drug therapy, meropenem-cilastatin (37.5 mg/kg q4 h) monotherapy, cefepime (100 mg/kg q2 h) monotherapy, and zidebactam (75 mg/kg q2 h) monotherapy; 24-h log10 CFU/thigh for aztreonam (75 mg/kg q2 h) plus zidebactam (75 mg/kg, q2 h) was significantly lower than that for no drug therapy, meropenem-cilastatin (37.5 mg/kg q4 h) monotherapy, aztreonam (75 mg/kg q2 h) monotherapy, and zidebactam (75 mg/kg q2 h) monotherapy (Moya et al., 2019).
These results support the clinical development of cefepime in combination with antibiotics for the treatment of bacterial infections caused by MBL-producing Enterobacteriaceae and P. aeruginosa. Additionally, the cefepime/zidebactam combination (intravenous dose regimen: q8 h, 1-h infusion) is slated to enter phase 3 studies for infections caused by MDR gram-negative bacteria (Bhatia and Wockhardt, 2022).
OP0595 (Nacubactam) alone and in combination with antibiotics
Nacubactam, another new DBO, is also reported to show in vitro antibacterial activity against Enterobacteriaceae with MBLs either alone or in combination with PBP3-targeted β-lactams. Livermore et al. found that OP0595 at 4 μg/ml not only inhibited most MBL-producing Enterobacteriaceae but also achieved ≥8-fold potentiation of piperacillin, cefepime, and biapenem for MBL-producing K. pneumoniae isolates (Livermore et al., 2015). However, OP0595 at 4 mg/L achieved no significant potentiation of aztreonam, biapenem, piperacillin, or cefepime against the MLB-producing P. aeruginosa and A. baumannii (Livermore et al., 2015). In another study, OP0595 at 4 μg/ml potentiated meropenem (1 μg/ml), cefepime (2 μg/ml), and aztreonam (1 μg/ml) in most of the tested NDM-producing K. pneumoniae isolates, respectively (Mushtaq et al., 2019). No in vivo study of OP0595 against MBL producers in animal models was found.
WCK5153 in combination with antibiotics
No positive inhibitory effect on MBLs was reported for WCK5153 alone, a novel DBO. Nevertheless, WCK5153 has been proven to be effective against MBL-producing K. pneumoniae and P. aeruginosa isolates in vitro when used in combination with several antibiotics. Moya et al. found that WCK5153 (4 μg/ml) can enhance the effectiveness of several antibiotics against MBL-producing P. aeruginosa in vitro, including cefepime, aztreonam, piperacillin, imipenem, meropenem, and doripenem (Moya et al., 2017). Moya et al. confirmed the in vitro efficacy of cefepime (≤0.25–0.5 μg/ml) or aztreonam (≤0.25–4 μg/ml)+WCK5153 (4 μg/ml) on MBL-producing K. pneumoniae using a microdilution broth method (Moya et al., 2019). These authors also confirmed the in vivo efficacy of these two combinations using a neutropenic mouse thigh infection model caused by NDM+SBL-positive K. pneumoniae. Briefly, the 24-h log10 CFU/thigh for cefepime (100 mg/kg q2 h) plus WCK5153 (75 mg/kg q2 h) was significantly lower than that for no drug therapy, meropenem-cilastatin (37.5 mg/kg q4 h) monotherapy, cefepime (100 mg/kg q2 h) monotherapy, and WCK5153 (75 mg/kg q2 h) monotherapy; the 24-h log10 CFU/thigh for aztreonam (75 mg/kg q2 h) plus WCK5153 (75 mg/kg q2 h) was significantly lower than that for no drug therapy, meropenem-cilastatin (37.5 mg/kg q4 h) monotherapy, aztreonam (75 mg/kg q2 h) monotherapy, and WCK5153 (75 mg/kg q2 h) monotherapy (Moya et al., 2019).
Meropenem +ANT2681
ANT2681 is a specific, competitive inhibitor of MBLs with particularly potent activity against NDM, progressing to clinical development in combination with meropenem (Zalacain et al., 2021). Zalacain et al. verified the in vitro potent activity of meropenem+ANT2681 against NDM producers in a large population of Enterobacteriaceae strains (Zalacain et al., 2021). Meropenem+ANT2681 (≤0.06–1 μg/ml+8 μg/ml) can inhibit 80.7% of Enterobacteriaceae strains producing NDM, which is much higher than that producing VIM (29.1%) or IMP (10.2%). In addition, the antibacterial activity of meropenem+ANT2681 (≤0.06–1 μg/ml+8 μg/ml) against Enterobacteriaceae strains producing NDM+SBLs (10.6%) was much lower than that only producing NDM (80.7%), which likely indicated that meropenem+ANT2681 showed specific antibacterial activity against NDM-only Enterobacteriaceae. However, the specific potent antibacterial activity for NDM-only producers was not found in another study involving eleven Enterobacteriaceae strains, which may be related to the deviation of test results caused by too few strains (Davies et al., 2020). No study on meropenem+ANT2681 against MBL-producing P. aeruginosa or A. baumannii was found.
Regarding the in vivo efficacy, meropenem+ANT2681 was found to be effective against the NDM producer in a murine thigh infection model, which provides the underpinning evidence for meropenem+ANT2681 in clinical development. Specifically, in the neutropenic mouse thigh infection model caused by NDM-1-positive E. coli, the reduction of 24-h log10 CFU/thigh by meropenem (50 mg/kg q4 h) plus ANT2681 (89 mg/kg q4 h) was more than 1.5 log10 CFU/thigh in comparison to that by no drug therapy (Das et al., 2020).
Meropenem+QPX7728
QPX7728 is a recently discovered ultra-broad-spectrum β-lactamase inhibitor from a class of cyclic boronates, with potent activity against MBLs (Hecker et al., 2020). It has been proved to be effective against MBL-producing gram-negative bacteria in vitro when in combination with meropenem. QPX7728 (4–8 μg/ml) restores sensitivity to meropenem for almost all tested MBL-positive Enterobacteriaceae strains (Nelson et al., 2020a; Lomovskaya et al., 2022). It was also found that QPX7728 (8 μg/ml) restored sensitivity to meropenem for some NDM-positive Acinetobacter strains (Nelson et al., 2020b). Compared with Acinetobacter, this combination may have a weaker effect on MBL-positive P. aeruginosa. Meropenem+QPX7728 (≤8+8 μg/ml) was found to be effective in only one-third of the MBL-positive P. aeruginosa strains (Lomovskaya et al., 2021). No in vivo study of this combination against MBL producers in animal models was found.
Eravacycline
Eravacycline is a novel and fully synthetic fluorocycline antibacterial agent. Its structure is similar to that of tigecycline, with two modifications to the D-ring of its tetracycline core: a fluorine atom replaces the dimethylamine moiety at C-7, and a pyrrolidinoacetamido group replaces the 2-tertiary-butyl glycylamido at C-9 (Zhanel et al., 2016). Recently, eravacycline has been approved for use in complicated intra-abdominal infections, including infections caused by MBL producers (Solomkin et al., 2019). Eravacycline at 1–4 μg/ml exerts potent antibacterial activity on all or most of the tested Enterobacteriaceae strains producing MBLs (Zhang et al., 2016; Johnston et al., 2020; Maraki et al., 2022). However, no relevant studies have been conducted to test its antibacterial activity in MBL-producing P. aeruginosa or A. baumannii thus far.
Plazomicin
Plazomicin is an aminoglycoside developed from a sisomicin scaffold via chemical modification to evade the most common aminoglycoside resistance mechanisms in Enterobacteriaceae, such as β-lactamases, including MBLs, as well as fluoroquinolone and colistin resistance mechanisms (Castanheira et al., 2018). For this reason, plazomicin maintains its activity against many MDR Enterobacteriaceae and was approved in 2018 by the FDA for the treatment of complicated urinary tract infections related to E. coli, K. pneumoniae, Enterobacter cloacae, and Proteus mirabilis (Serio et al., 2019). Several studies in vitro have confirmed its antibacterial activity on MBL-producing Enterobacteriaceae at a concentration of 0.75–2 μg/ml (Serio et al., 2019; Johnston et al., 2021; Maraki et al., 2022). No study on its antibacterial activity against MBL-producing P. aeruginosa or A. baumannii was found.
KBP-7072
KBP-7072 is an effective, broad-spectrum, third-generation tetracycline (aminomethylcycline) antimicrobial agent under development (oral and intravenous formulations) for the treatment of community-acquired pneumonia, overcoming many common mechanisms of tetracycline resistance (Lepak et al., 2019). KBP-7072 shows excellent antibacterial activity against MBL-positive A. baumannii isolates with MIC values ranging from 0.12 to 1 μg/ml in vitro, which is significantly better than that of the comparator agents (MIC: 0.5–32 μg/ml), including tigecycline, ceftazidime, gentamicin, levofloxacin, and meropenem (Huband et al., 2020b). No other study on its antibacterial activity against MBL-producing Enterobacteriaceae or P. aeruginosa was found.
LYS228
LYS228 is a novel monobactam with potent antibacterial activity against carbapenem-resistant Enterobacteriaceae and is stable to MBLs. LYS228 at ≤0.06–4 μg/ml exerts potent in vitro antibacterial activity on most of the tested Enterobacteriaceae strains producing MBLs, which is obviously more active than aztreonam, ceftazidime, ceftazidime/avibactam, cefepime, and meropenem (Blais et al., 2018). Weiss et al. confirmed its in vivo efficacy using a neutropenic mice thigh infection model caused by NDM-1 positive K. pneumoniae. In brief, 24-h log10 CFU/thigh for LYS228 (90 mg/kg q4 h) was significantly lower than that for no drug therapy (Weiss et al., 2019). No study on its antibacterial activity against MBL-producing P. aeruginosa or A. baumannii was found.
Non-antibacterial drugs
Studies have shown that some non-antibacterial drugs also have antibacterial activity. According to a study published in JAMA Cardiol, the conventional antiplatelet dose of ticagrelor had good antibacterial activity against gram-positive bacteria (Lancellotti et al., 2019). Its bactericidal effect against methicillin-resistant Staphylococcus aureus and methicillin-resistant Staphylococcus epidermidis was better than that of vancomycin, and its bactericidal effect against methicillin-resistant S. aureus and vancomycin-resistant Enterococcus was similar to that of daptomycin (Lancellotti et al., 2019). Beyond that, non-antibacterial drugs with antibacterial activity to MBL producers have also been reported in recent years.
Captopril, a classical antihypertensive drug, chelates prosthetic groups and destroys the catalytic activity of MBL (Heinz et al., 2003). Captopril is reported to effectively inhibit NDM-1 with an IC50 value of 7.9 μM (Li et al., 2014). An in vitro study showed that captopril (10–320 μg/ml) potentiated meropenem activity and restored its efficacy against MBL-producing K. pneumoniae (Zhao et al., 2021). In Galleria mellonella infected by IMP-4-positive K. pneumoniae, the survival rate for captopril (25 mg/kg) plus meropenem (10 mg/kg) was significantly higher than that for no drug therapy, captopril (25 mg/kg) monotherapy, and meropenem (10 mg/kg) monotherapy; 24-h CFU/larva for captopril plus meropenem was significantly lower than that for no drug therapy and meropenem monotherapy (Zhao et al., 2021). Especially, captopril analogs based on a series of structure-guided designs that can inhibit the activity of MBLs are being discovered (Li et al., 2014; Yusof et al., 2016; Ma et al., 2021).
Ebselen is a drug used to treat cerebral ischemia and stroke in human clinical trials. Chiou et al. found that ebselen is capable of covalently binding to the Cys221 residue in the active site of the NDM-1 enzyme, which ultimately results in the loss of one zinc ion (Chiou et al., 2015). In vitro, ebselen alone has low intrinsic antibacterial activity but can reduce the MICs of ampicillin and meropenem by 16- and 128-fold, respectively, against NDM-1-positive E. coli (detailed data not presented) (Jin et al., 2020). Compared with ebselen, a 2-substituted 1,2-benzisothiazol-3(2H) derivative of ebselen exhibited much stronger activity against NDM-1 isolates. This derivative (4–32 μg/ml) in combination with meropenem (4–32 μg/ml) demonstrated potent synergistic activity against NDM-1-positive Enterobacteriaceae (Jin et al., 2020).
Antimicrobial peptides
Antimicrobial peptides (AMPs), also known as host defense peptides, can be found virtually in every life form, from prokaryotes to humans. To date, there have been many databases of natural AMPs, consisting of more than 2,000 peptides and exhibiting remarkable functional diversity (Wang, 2015). They not only possess direct and broad-spectrum antimicrobial activity but also are capable of modulating innate immunity in higher organisms to protect hosts from infectious diseases (Hancock and Sahl, 2006; Pasupuleti et al., 2012). Currently, AMPs have been reported to be effective against MBL-producing Enterobacteriaceae.
Thanatin is a 21-residue antimicrobial peptide with a disulfide bond between Cys11 and Cys18 that exerts potent activity against NDM-1-producing bacteria by a dual mechanism of action: disrupting the outer membrane of NDM-1 producers and inhibiting the enzymatic activity of NDM-1 by displacing zinc ions from the active site (Ma et al., 2019). It was found that thanatin (1–8 μg/ml) could exhibit a potent inhibitory effect on NDM-1-producing Enterobacteriaceae strains in vitro (Soren et al., 2015; Ma et al., 2019). The in vivo therapeutic effects of thanatin were confirmed in a sepsis model. In the sepsis mouse model caused by NDM-1-positive E. coli, the survival rates for thanatin (1, 3, or 6 mg/kg) increased by 30–100% compared with that for no drug therapy; 23-h log10 CFU/tissues (blood, lung, liver, and spleen) for thanatin was significantly lower than that for no drug therapy; obvious rescued pathological damages were found for thanatin, in comparison to no drug therapy (Ma et al., 2019). In addition, thanatin (concentration not described) exerted synergistic or additive effects with imipenem and meropenem against the NDM-1-producing K. pneumoniae strain in vitro (Ma et al., 2019). Similarly, the in vivo therapeutic effects of thanatin+meropenem were confirmed in a sepsis model. In the sepsis mouse model caused by NDM-1-positive E. coli, the survival rate for thanatin (0.1 mg/kg) plus meropenem (10 mg/kg) increased by 49–100% compared with that for no drug therapy, thanatin (0.1 mg/kg) monotherapy, and meropenem (10 mg/kg) monotherapy; 23-h log10 CFU/tissues (spleen and liver) in target regimen was significantly lower than that for no drug therapy, thanatin monotherapy, and meropenem monotherapy (Ma et al., 2019).
Novicidin, a novel 18-residue cat-ionic antimicrobial peptide, exerts bactericidal effects by disrupting the bacterial membrane (Dorosz et al., 2010; Nielsen and Otzen, 2010). The combination of novicidin with rifampin was shown to have synergistic activity against all tested NDM-1 Enterobacteriaceae strains in vitro (Soren et al., 2015).
Natural products
Natural products from microorganisms and plants have been a rich source of starting materials in the search for new drugs. Natural products may represent potential lead compounds for anti-MBL drug development. Natural products serve as natural antibiotics to make multidimensional strategies against MBLs more intact, which is of clinical importance.
Aspergillomarasmine A (AMA) is a fungal natural product considered a rapid and potent MBL inhibitor that electively sequesters Zn2+ (King et al., 2014; Sychantha et al., 2021). AMA itself is not antimicrobial but works in combination with β-lactam antibiotics or carbapenems against MBL producers, with an emphasis on VIM or NDM. King et al. found that AMA (8 μg/ml) fully restored the activity of meropenem against >88% of Enterobacteriaceae, Acinetobacter spp. and Pseudomonas spp. strains possessing either VIM or NDM (King et al., 2014). Rotondo et al. found that AMA (≤0.5–64 g/ml) could be used not only with carbapenems but also with β-lactam antibiotics against MBL producers Enterobacteriaceae, similar to an emphasis on VIM or NDM (Rotondo et al., 2020). Iminodiacetic acid, as the metal-binding pharmacophore core of AMA, has been used for fragment-based drug discovery of NDM-1 inhibitors (Chen et al., 2020). Emerione A, identified from fungal products by in silico screening, is the second fungal metabolite reported to exhibit NMD-1 inhibitory activity after AMA. Emerione A (8–128 μg/ml) potentiates the activity of four β-lactam antibiotics against two kinds of NDM-1-producing Enterobacteriaceae (He et al., 2022).
Rosmarinic acid is widely identified in the plant kingdom, such as the plant families Lamiaceae, Boraginaceae, and Marantaceae. Kinetic assays revealed that rosmarinic acid is a fully reversible, substrate-competitive VIM-2 inhibitor (Yu et al., 2018). Salvianolic acid A, a derivative of RA, manifests potent inhibition of VIM-2, more interestingly, which also shows inhibitory activity against NDM-1 (Yu et al., 2018). Both rosmarinic acid and salvianolic acid A resulted in a 2- to 4-fold MIC reduction of meropenem against VIM-2-producing E. coli (Yu et al., 2018).
Some other natural products, which have been reported to have anti-MBL activity at the enzymatic level but have not been studied at the cellular level, will not be described here, such as alcohol extracts of Schisandra chinensis and baicalin (Shi et al., 2019; Duan et al., 2021; Salari-Jazi et al., 2021). In addition, natural products with very limited anti-MBL activity are no longer described here, such as the aquo-ethanolic extract of Camellia sinensis (Thakur et al., 2016) and the methanolic extract of Punica granatum (Dey et al., 2012).
Zinc chelators
The zinc-dependent activity of MBL allows its inhibition by zinc-chelating agents. Several compounds have potential usage to treat MBL producers in combination with existing antibiotics.
Two zinc chelators, N, N, N′, N′-tetrakis (2-pyridylmethyl) ethylenediamine (TPEN) and di-(2-picolyl) amine (DPA), were able to inhibit MBLs and render MBL producers susceptible to meropenem (Azumah et al., 2016). TPEN at 46–32 μg/ml and DPA at 8–32 μg/ml regain susceptibility to meropenem for most tested Enterobacteriaceae strains harboring MBL (Azumah et al., 2016). 1,4,7-Triazacyclononane-1,4,7-triacetic acid (NOTA) is a superior chelator of copper and gallium (Boros et al., 2010; Zhang et al., 2011). It is found to chelate zinc, which may be due to zinc having a size between that of copper and gallium (Somboro et al., 2015). NOTA (4 μg/ml) was able to restore the activities of carbapenems against most tested MBL-producing Enterobacteriaceae strains. TACN, a cyclic organic compound within NOTA derived from cyclononane by the replacement of three equidistant CH2 groups with NH groups, significantly reduced the MIC of meropenem against MBL-producing Enterobacteriaceae strains (Somboro et al., 2019). In brief, the combination of meropenem (0.06–8 μg/ml) + TACN (4–8 μg/ml) was synergistic for all tested MBL-producing Enterobacteriaceae strains.
Ca-EDTA, a metallo-chelator, was created as an injectable form of chelator with low toxicity, which has been approved for the treatment of lead intoxication (Lin-Tan et al., 2007). Aoki et al. and Yoshizumi et al. successively reported the efficacy of Ca-EDTA as an inhibitor of MBL (Aoki et al., 2010; Yoshizumi et al., 2013). The addition of Ca-EDTA (32 μg/ml) drastically reduced the MICs of carbapenems (imipenem, meropenem, and amikacin) in a small proportion of MBL-positive Enterobacteriaceae and P. aeruginosa in vitro (Aoki et al., 2010; Yoshizumi et al., 2013). The in vivo efficacy of combining Ca-EDTA (200–300 mg/kg q.d.) with IPM (50 mg/kg/day) against MBL-positive P. aeruginosa was confirmed in a pneumonia mouse model. In the mouse model of pneumonia caused by IMP-1-positive P. aeruginosa, the survival rate for mice treated with Ca-EDTA (200 mg/kg q.d. or 300 mg/kg q.d.) plus imipenem (50 mg/kg q.d., s.c.) increased by ~70–100% compared with that for mice given no drug therapy, Ca-EDTA (200 mg/kg q.d or 300 mg/kg q.d.) monotherapy, and imipenem (50 mg/kg q.d.) monotherapy; the reduction in bacterial burden by Ca-EDTA plus imipenem was more than 1 log10 CFU/lung in comparison to that by no drug therapy and imipenem monotherapy (Aoki et al., 2010). Moreover, these in vivo data suggest the therapeutic potential of Ca-EDTA not only by blocking MBLs but also by neutralizing tissue-damaging metalloproteases in infections caused by P. aeruginosa (Aoki et al., 2010). In the acute lethal mouse septicemia model caused by NDM-1-positive E. coli, CFU/liver (not CFU/blood) was significantly reduced by Ca-EDTA (200 mg/kg) plus imipenem/cilastatin sodium (25 mg/kg) compared with that by no drug therapy, Ca-EDTA (200 mg/kg) monotherapy, and imipenem/cilastatin sodium (25 mg/kg) monotherapy (Yoshizumi et al., 2013). Furthermore, in a more severe model of infection (neutropenic sepsis mouse model) caused by NDM-1-positive E. coli, both CFU/liver and CFU/blood were significantly reduced by Ca-EDTA (100 mg/kg) plus imipenem/cilastatin sodium (10 mg/kg) compared with that by no drug therapy, Ca-EDTA (100 mg/kg) monotherapy, and imipenem/cilastatin sodium (10 mg/kg) monotherapy (Yoshizumi et al., 2013).
Other compounds with MBL inhibitory activity
As mentioned above, antibiotic resistance as a global threat has aroused widespread concern, indicating that we have entered the “postantibiotic era.” To combat resistance crises, it is crucial to adopt multidimensional strategies.
Farley et al. synthesized nine N-sulfamoylpyrrole-2-carboxylates (NSPCs), which exert particularly potent activity against NDM-1 and IMP-1 (Farley et al., 2021). Crystallography reveals that the N-sulfamoyl NH2 group of NSPCs displaces the dopamine bridging hydroxide/water of the B1 MBLs. At a fixed concentration of 2 μg/ml, any one of the nine NSPCs reduced the meropenem MIC from 256 to ≤0.25–5 μg/ml in NDM-1 Enterobacteriaceae.
ML302 is a rhodamine-based MBL inhibitor. Mechanistic studies show that ML302 undergoes hydrolysis to yield a thioenolate fragment (ML302F), which triggers the formation of a ternary complex among the MBL, intact ML302, and ML302F by zinc chelation to potentiate antibiotic efficacy through collaborative strategies. ML302 (10 μg/ml) conferred meropenem sensitivity on IMP producers of Enterobacteriaceae, not NDM or VIM producers (Brem et al., 2014).
KHP-3757 is the most recent LpxC inhibitor of the hydroxamate class currently being evaluated in preclinical development studies (Huband et al., 2020a). Huband et al. demonstrated that KHP-3757 (0.06–0.5 μg/ml) had potent in vitro activity against all tested MBL-producing P. aeruginosa isolates, outperforming nine comparator agents, including amikacin, aztreonam, cefepime, ceftazidime, ciprofloxacin, colistin, imipenem, meropenem, and piperacillin-tazobactam (Huband et al., 2020a).
Khan et al. have reported the discovery of four novel non-β-lactam inhibitors (M1, M17, M21, and M61) against NDM-1 by a multi-step virtual screening approach (Khan et al., 2017). For E. coli producing NDM-1, the lower MICs were obtained when each of these inhibitors was combined with ceftazidime, cefoxitin, meropenem, and imipenem. The MICs were lowered from 8 to 4 μg/ml (ceftazidime), 16 to 8 μg/ml (cefoxitin), and 2 to 1 μg/ml for meropenem and imipenem.
Discussion and future perspectives
Bacterial infections are one of the leading causes of human death worldwide. International antibacterial treatments mainly use antibiotics to inhibit the reproduction and growth of bacteria and can achieve efficient sterilization effects. β-Lactams have long played a vital role in the treatment of pathogenic bacterial infections. However, widespread and improper use of antibiotics for decades in turn has led to resistance in bacteria. The major resistance mechanism is the emergence of β-lactamases, especially the most relevant MBLs, which can hydrolyze the β-lactam ring. Thus, MBLs are capable of inactivating almost all β-lactams, with the exception of aztreonam. Nevertheless, the use of aztreonam was also limited by the fact that MBL-producing strains carried SBLs, which requires broad-spectrum inhibitors (Cervino et al., 2021). Therefore, the development of new drugs against MBL or MBL +SBL producers has become a global biomedical research hotspot.
This article reviews the current research progress on novel drugs, compounds, and drug combinations against MBL producers. As shown in Table 1, many novel drugs, compounds, and drug combinations with anti-MBL activity have not been studied in detail. For example, antimicrobial activity studies of some novel drugs have not included P. aeruginosa and A. baumannii that produce MBLs, and most compounds in this review have not been studied in vivo. It must be noted that the traditional development of antibiotics inevitably leads to the emergence of new resistant strains, making new drugs quickly ineffective. Developing a single inhibitor of MBLs is also technically too difficult, and overcoming in vivo toxicity due to cross-reactions with human metalloenzymes has been a concern. Therefore, restoring the therapeutic potential of existing antibiotics through drug combinations may be a very attractive strategy to solve clinically challenging MBLs. In this study, several drugs or compounds in this review that have not been studied for their synergistic effects on existing antibiotics should be complemented with further experimental data.
In addition, some of these novel drugs, compounds, and drug combinations have many desirable antimicrobial properties for the treatment of MBL-associated infections, but more in vivo efficacy and safety data are needed to fully determine their role in the treatment of infectious diseases. Therefore, further experimental data should be improved based on Table 2 to obtain stronger evidence of the efficacy and safety of MBL producers for these novel drugs, compounds, and drug combinations. Indeed, we are still encountering many setbacks on these roads, such as complicated preparation processes, uncertain PK and PD properties, and differential efficacy in vitro and in vivo.
Nevertheless, the evolution of MBLs never ends, which urges us to conduct continuing research. The field of antimicrobial resistance is a dynamic and rapidly evolving field, and the treatment of antimicrobial resistance will continue to challenge clinicians. The application of bioinformatics software tools and compound databases also holds great promise for the production of effective inhibitors against MBLs, which is not described in detail in this review.
Limitations to this study include that most of the drugs involved in this study only performed MIC measurements. Except for a few drugs tested for affinity against the MBLs, scarcely any of the other drugs have been studied for the mechanism of drug resistance against MBL producers. Therefore, we did not show the mechanisms, but it is hoped that this study will inspire more researchers to study the mechanism of these drugs.
In conclusion, these novel drugs, compounds, and their combinations with anti-MBL activity provide more therapeutic alternatives against complicated infections caused by MBLs, although further rigorous optimization is required for their clinical development. It is hoped that this review can provide ideas and enlightenment for others to study the methods against metalloenzymes and improve the existing research data to obtain convincing evidence.
Author contributions
XL, HW, and YZ designed the concept and wrote the manuscript. BZ and XD assisted in making tables. JJ and WW finalized and corrected the manuscript. JZ assisted in the revision of the manuscript. All authors read and approved the final manuscript.
Funding
This work was supported by the Shandong Provincial Natural Science Foundation [Grant no. ZR2020QH365], the Hospital Level Project of Shandong Province Maternal and Child Health Hospital [Grant no. 2021SFF062], and Shandong Province Medical and Health Science and Technology Development Plan [Grant no. 202006021519]. The funders will have no role in the study design, data collection and analysis, decision to publish, or preparation of the manuscript.
Conflict of interest
The authors declare that the research was conducted in the absence of any commercial or financial relationships that could be construed as a potential conflict of interest.
Publisher's note
All claims expressed in this article are solely those of the authors and do not necessarily represent those of their affiliated organizations, or those of the publisher, the editors and the reviewers. Any product that may be evaluated in this article, or claim that may be made by its manufacturer, is not guaranteed or endorsed by the publisher.
Abbreviations
P. aeruginosa, Pseudomonas aeruginosa; A. baumannii, Acinetobacter baumannii; SBL, serine β-lactamases; MBL, metallo-β-lactamases; NDM, New Delhi metallo-β-lactamase; VIM, Verona imipenemase; IMP, imipenemase; CAZ, ceftazidime; AVI, avibactam; E. coli, Escherichia coli; K. pneumoniae, Klebsiella pneumoniae; FDA, Food and Drug Administration; DBO, diazabicyclooctane; AMP, antimicrobial peptides; AMA, Aspergillomarasmine A.
References
Aoki, N., Ishii, Y., Tateda, K., Saga, T., Kimura, S., Kikuchi, Y., et al. (2010). Efficacy of calcium-EDTA as an inhibitor for metallo-beta-lactamase in a mouse model of Pseudomonas aeruginosa pneumonia. Antimicrob. Agents Chemother. 54, 4582–4588. doi: 10.1128/AAC.00511-10
Avery, L. M., Mullane, E. M., and Nicolau, D. P. (2020). Evaluation of the in vitro activity of WCK 5222 (cefepime/zidebactam) and currently available combination therapies against single- and double-carbapenemase producing Enterobacteriaceae: expanding the zone of hope. Int. J. Antimicrob. Agents 55, 105863. doi: 10.1016/j.ijantimicag.2019.105863
Azumah, R., Dutta, J., Somboro, A. M., Ramtahal, M., Chonco, L., Parboosing, R., et al. (2016). In vitro evaluation of metal chelators as potential metallo- beta -lactamase inhibitors. J. Appl. Microbiol. 120, 860–867. doi: 10.1111/jam.13085
Bhagwat, S. S., Legakis, N. J., Skalidis, T., Loannidis, A., Goumenopoulos, C., Joshi, P. R., et al. (2021). In vitro activity of cefepime/zidebactam (WCK 5222) against recent Gram-negative isolates collected from high resistance settings of Greek hospitals. Diagn. Microbiol. Infect. Dis. 100, 115327. doi: 10.1016/j.diagmicrobio.2021.115327
Bhagwat, S. S., Periasamy, H., Takalkar, S. S., Palwe, S. R., Khande, H. N., and Patel, M. V. (2019). The novel beta-lactam enhancer zidebactam augments the in vivo pharmacodynamic activity of cefepime in a neutropenic mouse lung Acinetobacter baumannii infection model. Antimicrob. Agents Chemother. 63, e02146-18. doi: 10.1128/AAC.02146-18
Bhatia, A., and Wockhardt, M. (2022). Study of Cefepime-zidebactam (FEP-ZID) in Complicated Urinary Tract Infection (cUTI) or Acute Pyelonephritis (AP). Available online at: https://clinicaltrials.gov/ct2/show/NCT04979806 (accessed Augest 24, 2022).
Bhatnagar, A., Boyd, S., Sabour, S., Bodnar, J., Nazarian, E., Peinovich, N., et al. (2021). Aztreonam-avibactam susceptibility testing program for metallo-beta-lactamase-producing Enterobacterales in the antibiotic resistance laboratory network, March 2019 to December 2020. Antimicrob. Agents Chemother. 65, e0048621. doi: 10.1128/AAC.00486-21
Biagi, M., Wu, T., Lee, M., Patel, S., Butler, D., and Wenzler, E. (2019). Searching for the optimal treatment for metallo- and serine-beta-lactamase producing Enterobacteriaceae: aztreonam in combination with ceftazidime-avibactam or meropenem-vaborbactam. Antimicrob. Agents Chemother. 63, e01426-19. doi: 10.1128/AAC.01426-19
Blais, J., Lopez, S., Li, C., Ruzin, A., Ranjitkar, S., Dean, C. R., et al. (2018). In vitro activity of LYS228, a novel monobactam antibiotic, against multidrug-resistant Enterobacteriaceae. Antimicrob. Agents Chemother. 62, e00552-18. doi: 10.1128/AAC.00552-18
Boros, E., Ferreira, C. L., Cawthray, J. F., Price, E. W., Patrick, B. O., Wester, D. W., et al. (2010). Acyclic chelate with ideal properties for (68)Ga PET imaging agent elaboration. J. Am. Chem. Soc. 132, 15726–15733. doi: 10.1021/ja106399h
Boyd, S. E., Livermore, D. M., Hooper, D. C., and Hope, W. W. (2020). Metallo-beta-lactamases: structure, function, epidemiology, treatment options, and the development pipeline. Antimicrob. Agents Chemother. 64, e00397-20. doi: 10.1128/AAC.00397-20
Brem, J., Van Berkel, S. S., Aik, W., Rydzik, A. M., Avison, M. B., Pettinati, I., et al. (2014). Rhodanine hydrolysis leads to potent thioenolate mediated metallo-beta-lactamase inhibition. Nat. Chem. 6, 1084–1090. doi: 10.1038/nchem.2110
Bush, K., and Bradford, P. A. (2016). β-lactams and β-lactamase inhibitors: an overview. Cold Spring Harb. Perspect. Med. 6, a025247. doi: 10.1101/cshperspect.a025247
Castanheira, M., Davis, A. P., Mendes, R. E., Serio, A. W., Krause, K. M., and Flamm, R. K. (2018). In vitro activity of plazomicin against gram-negative and gram-positive isolates collected from U.S. hospitals and comparative activities of aminoglycosides against carbapenem-resistant Enterobacteriaceae and isolates carrying carbapenemase genes. Antimicrob. Agents Chemother. 62, e00313-18. doi: 10.1128/AAC.00313-18
Cervino, I., Gonzalez, D., Nastro, M., Vega, J., Reyes, A. P., Buriano, G., et al. (2021). In vitro synergistic activity of aztreonam (AZT) plus novel and old beta-lactamase inhibitor combinations against metallo-beta-lactamase-producing AZT-resistant Enterobacterales. J. Med. Microbiol. 70. doi: 10.1099/jmm.0.001427
Chen, A. Y., Thomas, C. A., Thomas, P. W., Yang, K., Cheng, Z., Fast, W., et al. (2020). Iminodiacetic acid as a novel metal-binding pharmacophore for New Delhi metallo-beta-lactamase inhibitor development. ChemMedChem 15, 1272–1282. doi: 10.1002/cmdc.202000123
Chiou, J., Wan, S., Chan, K. F., So, P. K., He, D., Chan, E. W., et al. (2015). Ebselen as a potent covalent inhibitor of New Delhi metallo-beta-lactamase (NDM-1). Chem. Commun. 51, 9543–9546. doi: 10.1039/C5CC02594J
Coleman, K. (2011). Diazabicyclooctanes (DBOs): a potent new class of non-beta-lactam beta-lactamase inhibitors. Curr. Opin. Microbiol. 14, 550–555. doi: 10.1016/j.mib.2011.07.026
Das, S., Johnson, A., Mcentee, L., Farrington, N., Kirby, A., Unsworth, J., et al. (2020). Pharmacodynamics of the novel metallo-beta-lactamase inhibitor ANT2681 in combination with meropenem for the treatment of infections caused by NDM-producing Enterobacteriaceae. Antimicrob. Agents Chemother. 64, e01076-20. doi: 10.1128/AAC.01076-20
Das, S., Li, J., Riccobene, T., Carrothers, T. J., Newell, P., Melnick, D., et al. (2019). Dose selection and validation for ceftazidime-avibactam in adults with complicated intra-abdominal infections, complicated urinary tract infections, and nosocomial pneumonia. Antimicrob. Agents Chemother. 63, e02187-18. doi: 10.1128/AAC.02187-18
Davido, B., Fellous, L., Lawrence, C., Maxime, V., Rottman, M., and Dinh, A. (2017). Ceftazidime-avibactam and aztreonam, an interesting strategy to overcome beta-lactam resistance conferred by metallo-beta-lactamases in Enterobacteriaceae and Pseudomonas aeruginosa. Antimicrob. Agents Chemother. 61, e01008-17. doi: 10.1128/AAC.01008-17
Davies, D. T., Leiris, S., Sprynski, N., Castandet, J., Lozano, C., Bousquet, J., et al. (2020). ANT2681: SAR studies leading to the identification of a metallo-beta-lactamase inhibitor with potential for clinical use in combination with meropenem for the treatment of infections caused by NDM-producing Enterobacteriaceae. ACS Infect. Dis. 6, 2419–2430. doi: 10.1021/acsinfecdis.0c00207
Dey, D., Debnath, S., Hazra, S., Ghosh, S., Ray, R., and Hazra, B. (2012). Pomegranate pericarp extract enhances the antibacterial activity of ciprofloxacin against extended-spectrum beta-lactamase (ESBL) and metallo-beta-lactamase (MBL) producing Gram-negative bacilli. Food Chem. Toxicol. 50, 4302–4309. doi: 10.1016/j.fct.2012.09.001
Dobias, J., Denervaud-Tendon, V., Poirel, L., and Nordmann, P. (2017). Activity of the novel siderophore cephalosporin cefiderocol against multidrug-resistant Gram-negative pathogens. Eur. J. Clin. Microbiol. Infect. Dis. 36, 2319–2327. doi: 10.1007/s10096-017-3063-z
Doern, C. D. (2014). When does 2 plus 2 equal 5? A review of antimicrobial synergy testing. J. Clin. Microbiol. 52, 4124–4128. doi: 10.1128/JCM.01121-14
Dorosz, J., Gofman, Y., Kolusheva, S., Otzen, D., Ben-Tal, N., Nielsen, N. C., et al. (2010). Membrane interactions of novicidin, a novel antimicrobial peptide: phosphatidylglycerol promotes bilayer insertion. J. Phys. Chem. B 114, 11053–11060. doi: 10.1021/jp1052248
Dowell, J. A., Dickerson, D., and Henkel, T. (2021). Safety and pharmacokinetics in human volunteers of taniborbactam (VNRX-5133), a novel intravenous beta-lactamase inhibitor. Antimicrob. Agents Chemother. 65, e0105321. doi: 10.1128/AAC.01053-21
Duan, M., Bai, J., Yang, J., Shi, P., and Bian, L. (2021). Screening and identification of bioactive components resistant to metallo-beta-lactamase from Schisandra chinensis (Turcz.) Baill. by metalloenzyme-immobilized affinity chromatography. J. Chromatogr. B Analyt Technol. Biomed. Life Sci. 1165, 122524. doi: 10.1016/j.jchromb.2021.122524
Emeraud, C., Escaut, L., Boucly, A., Fortineau, N., Bonnin, R. A., Naas, T., et al. (2019). Aztreonam plus clavulanate, tazobactam, or avibactam for treatment of infections caused by metallo-beta-lactamase-producing gram-negative bacteria. Antimicrob. Agents Chemother. 63, e00010-19. doi: 10.1128/AAC.00010-19
Eurosurveillance Editorial, T. (2015). WHO member states adopt global action plan on antimicrobial resistance. Euro Surveill. 20, 21106. doi: 10.2807/1560-7917.ES2015.20.17.21106
Falcone, M., Daikos, G. L., Tiseo, G., Bassoulis, D., Giordano, C., Galfo, V., et al. (2021a). Efficacy of ceftazidime-avibactam plus aztreonam in patients with bloodstream infections caused by metallo-beta-lactamase-producing Enterobacterales. Clin. Infect. Dis. 72, 1871–1878. doi: 10.1093/cid/ciaa586
Falcone, M., Tiseo, G., Giordano, C., Leonildi, A., Menichini, M., Vecchione, A., et al. (2021b). Predictors of hospital-acquired bacterial and fungal superinfections in COVID-19: a prospective observational study. J. Antimicrob. Chemother. 76, 1078–1084. doi: 10.1093/jac/dkaa530
Farfour, E., Lecuru, M., Dortet, L., Le Guen, M., Cerf, C., Karnycheff, F., et al. (2020). Carbapenemase-producing Enterobacterales outbreak: another dark side of COVID-19. Am. J. Infect. Control 48, 1533–1536. doi: 10.1016/j.ajic.2020.09.015
Farley, A. J. M., Ermolovich, Y., Calvopina, K., Rabe, P., Panduwawala, T., Brem, J., et al. (2021). Structural basis of metallo-beta-lactamase inhibition by N-sulfamoylpyrrole-2-carboxylates. ACS Infect. Dis. 7, 1809–1817. doi: 10.1021/acsinfecdis.1c00104
Feng, K., Jia, N., Zhu, P., Sy, S., Liu, Y., Dong, D., et al. (2021). Aztreonam/avibactam effect on pharmacodynamic indices for mutant selection of Escherichia coli and Klebsiella pneumoniae harbouring serine- and New Delhi metallo-beta-lactamases. J. Antimicrob. Chemother. 76, 2875–2883. doi: 10.1093/jac/dkab292
Ghebremedhin, B., and Ahmad-Nejad, P. (2021). In-Vitro Efficacy of cefiderocol in carbapenem-non-susceptible gram-negative bacilli of different genotypes in sub-region of North Rhine Westphalia, Germany. Pathogens 10, 1258. doi: 10.3390/pathogens10101258
Giacobbe, D. R., Ciacco, E., Girmenia, C., Pea, F., Rossolini, G. M., Sotgiu, G., et al. (2020). Evaluating cefiderocol in the treatment of multidrug-resistant gram-negative bacilli: a review of the emerging data. Infect. Drug Resist. 13, 4697–4711. doi: 10.2147/IDR.S205309
Hamrick, J. C., Docquier, J. D., Uehara, T., Myers, C. L., Six, D. A., Chatwin, C. L., et al. (2020). VNRX-5133 (Taniborbactam), a broad-spectrum inhibitor of serine- and metallo-beta-lactamases, restores activity of cefepime in Enterobacterales and Pseudomonas aeruginosa. Antimicrob. Agents Chemother. 64, e01963-19. doi: 10.1128/AAC.01963-19
Hancock, R. E., and Sahl, H. G. (2006). Antimicrobial and host-defense peptides as new anti-infective therapeutic strategies. Nat. Biotechnol. 24, 1551–1557. doi: 10.1038/nbt1267
Hansen, G. T. (2021). Continuous evolution: perspective on the epidemiology of carbapenemase resistance among Enterobacterales and other gram-negative bacteria. Infect. Dis. Ther. 10, 75–92. doi: 10.1007/s40121-020-00395-2
He, Y., Zhou, S., Sun, W., Li, Q., Wang, J., and Zhang, J. (2022). Emerione A, a novel fungal metabolite as an inhibitor of New Delhi metallo-beta-lactamase 1, restores carbapenem susceptibility in carbapenem-resistant isolates. J. Glob. Antimicrob. Resist. 28, 216–222. doi: 10.1016/j.jgar.2021.12.019
Hecker, S. J., Reddy, K. R., Lomovskaya, O., Griffith, D. C., Rubio-Aparicio, D., Nelson, K., et al. (2020). Discovery of cyclic boronic acid QPX7728, an ultrabroad-spectrum inhibitor of serine and metallo-beta-lactamases. J. Med. Chem. 63, 7491–7507. doi: 10.1021/acs.jmedchem.9b01976
Heinz, U., Bauer, R., Wommer, S., Meyer-Klaucke, W., Papamichaels, C., Bateson, J., et al. (2003). Coordination geometries of metal ions in d- or l-captopril-inhibited metallo-beta-lactamases. J. Biol. Chem. 278, 20659–20666. doi: 10.1074/jbc.M212581200
Hernandez-Garcia, M., Garcia-Castillo, M., Ruiz-Garbajosa, P., Bou, G., Siller-Ruiz, M., Pitart, C., et al. (2022). In vitro activity of cefepime-taniborbactam against carbapenemase-producing Enterobacterales and Pseudomonas aeruginosa isolates recovered in Spain. Antimicrob. Agents Chemother. 66, e0216121. doi: 10.1128/aac.02161-21
Huband, M. D., Lindley, J. M., Mendes, R. E., Fedler, K. A., Benn, V. J., Zhang, J., et al. (2020a). In vitro activity of KHP-3757 (a novel LpxC inhibitor) and comparator agents against recent and molecularly characterized Pseudomonas aeruginosa isolates from a global surveillance program (2017-2018). Diagn. Microbiol. Infect. Dis. 98, 115191. doi: 10.1016/j.diagmicrobio.2020.115191
Huband, M. D., Mendes, R. E., Pfaller, M. A., Lindley, J. M., Strand, G. J., Benn, V. J., et al. (2020b). In vitro activity of KBP-7072, a novel third-generation tetracycline, against 531 recent geographically diverse and molecularly characterized Acinetobacter baumannii species complex isolates. Antimicrob. Agents Chemother. 64. doi: 10.1128/AAC.02375-19
Ito, A., Sato, T., Ota, M., Takemura, M., Nishikawa, T., Toba, S., et al. (2018). In vitro antibacterial properties of cefiderocol, a novel siderophore cephalosporin, against gram-negative bacteria. Antimicrob. Agents Chemother. 62, e01454-17. doi: 10.1128/AAC.01454-17
Jacobs, M. R., Abdelhamed, A. M., Good, C. E., Rhoads, D. D., Hujer, K. M., Hujer, A. M., et al. (2019). ARGONAUT-I: activity of cefiderocol (S-649266), a siderophore cephalosporin, against gram-negative bacteria, including carbapenem-resistant nonfermenters and Enterobacteriaceae with defined extended-spectrum beta-lactamases and carbapenemases. Antimicrob. Agents Chemother. 63. doi: 10.1128/AAC.01801-18
Jin, W. B., Xu, C., Cheung, Q., Gao, W., Zeng, P., Liu, J., et al. (2020). Bioisosteric investigation of ebselen: synthesis and in vitro characterization of 1,2-benzisothiazol-3(2H)-one derivatives as potent New Delhi metallo-beta-lactamase inhibitors. Bioorg. Chem. 100, 103873. doi: 10.1016/j.bioorg.2020.103873
Johnston, B. D., Thuras, P., Porter, S. B., Anacker, M., Vonbank, B., Snippes Vagnone, P., et al. (2020). Activity of cefiderocol, ceftazidime-avibactam, and eravacycline against carbapenem-resistant Escherichia coli isolates from the United States and international sites in relation to clonal background, resistance genes, coresistance, and region. Antimicrob. Agents Chemother. 64, e00797-20. doi: 10.1128/AAC.00797-20
Johnston, B. D., Thuras, P., Porter, S. B., Anacker, M., Vonbank, B., Snippes Vagnone, P., et al. (2021). Activity of plazomicin against carbapenem-intermediate or -resistant Escherichia coli isolates from the United States and international sites in relation to clonal background, resistance genes, co-resistance, and region. J. Antimicrob. Chemother. 76, 2061–2070. doi: 10.1093/jac/dkab150
Karlowsky, J. A., Hackel, M. A., Bouchillon, S. K., and Sahm, D. F. (2020). In vitro activity of WCK 5222 (cefepime-zidebactam) against worldwide collected gram-negative bacilli not susceptible to carbapenems. Antimicrob. Agents Chemother. 64. doi: 10.1128/AAC.01432-20
Karlowsky, J. A., Kazmierczak, K. M., De Jonge, B. L. M., Hackel, M. A., Sahm, D. F., and Bradford, P. A. (2017). In vitro activity of aztreonam-avibactam against Enterobacteriaceae and Pseudomonas aeruginosa isolated by clinical laboratories in 40 countries from 2012 to 2015. Antimicrob. Agents Chemother. 61:AAC.00472-17. doi: 10.1128/AAC.00472-17
Kazmierczak, K. M., Tsuji, M., Wise, M. G., Hackel, M., Yamano, Y., Echols, R., et al. (2019). In vitro activity of cefiderocol, a siderophore cephalosporin, against a recent collection of clinically relevant carbapenem-non-susceptible Gram-negative bacilli, including serine carbapenemase- and metallo-beta-lactamase-producing isolates (SIDERO-WT-2014 Study). Int. J. Antimicrob. Agents 53, 177–184. doi: 10.1016/j.ijantimicag.2018.10.007
Khan, A., Erickson, S. G., Pettaway, C., Arias, C. A., Miller, W. R., and Bhatti, M. M. (2021). Evaluation of susceptibility testing methods for aztreonam and ceftazidime-avibactam combination therapy on extensively drug-resistant gram-negative organisms. Antimicrob. Agents Chemother. 65, e0084621. doi: 10.1128/AAC.00846-21
Khan, A. U., Ali, A., Danishuddin, Srivastava, G., and Sharma, A. (2017). Potential inhibitors designed against NDM-1 type metallo-beta-lactamases: an attempt to enhance efficacies of antibiotics against multi-drug-resistant bacteria. Sci. Rep. 7, 9207. doi: 10.1038/s41598-017-09588-1
Kidd, J. M., Abdelraouf, K., and Nicolau, D. P. (2020). Efficacy of human-simulated bronchopulmonary exposures of cefepime, zidebactam and the combination (WCK 5222) against MDR Pseudomonas aeruginosa in a neutropenic murine pneumonia model. J. Antimicrob. Chemother. 75, 149–155. doi: 10.1093/jac/dkz414
King, A. M., Reid-Yu, S. A., Wang, W., King, D. T., De Pascale, G., Strynadka, N. C., et al. (2014). Aspergillomarasmine A overcomes metallo-beta-lactamase antibiotic resistance. Nature 510, 503–506. doi: 10.1038/nature13445
Kloezen, W., Melchers, R. J., Georgiou, P. C., Mouton, J. W., and Meletiadis, J. (2021). Activity of cefepime in combination with the novel beta-lactamase inhibitor taniborbactam (VNRX-5133) against extended-spectrum-beta-lactamase-producing isolates in in vitro checkerboard assays. Antimicrob. Agents Chemother. 65, e02338-20. doi: 10.1128/AAC.02338-20
Krajnc, A., Brem, J., Hinchliffe, P., Calvopina, K., Panduwawala, T. D., Lang, P. A., et al. (2019). Bicyclic boronate VNRX-5133 inhibits metallo- and serine-beta-lactamases. J. Med. Chem. 62, 8544–8556. doi: 10.1021/acs.jmedchem.9b00911
Lancellotti, P., Musumeci, L., Jacques, N., Servais, L., Goffin, E., Pirotte, B., et al. (2019). Antibacterial activity of ticagrelor in conventional antiplatelet dosages against antibiotic-resistant gram-positive bacteria. JAMA Cardiol. 4, 596–599. doi: 10.1001/jamacardio.2019.1189
Lang, P. A., Parkova, A., Leissing, T. M., Calvopina, K., Cain, R., Krajnc, A., et al. (2020). Bicyclic boronates as potent inhibitors of AmpC, the class C beta-lactamase from Escherichia coli. Biomolecules 10, 899. doi: 10.3390/biom10060899
Lepak, A. J., Zhao, M., Liu, Q., Wang, P., Wang, Y., Bader, J. C., et al. (2019). Pharmacokinetic/pharmacodynamic evaluation of a novel aminomethylcycline antibiotic, KBP-7072, in the neutropenic murine pneumonia model against Staphylococcus aureus and Streptococcus pneumoniae. Antimicrob. Agents Chemother. 63, e02404-18. doi: 10.1128/AAC.02404-18
Li, H., Estabrook, M., Jacoby, G. A., Nichols, W. W., Testa, R. T., and Bush, K. (2015). In vitro susceptibility of characterized beta-lactamase-producing strains tested with avibactam combinations. Antimicrob. Agents Chemother. 59, 1789–1793. doi: 10.1128/AAC.04191-14
Li, N., Xu, Y., Xia, Q., Bai, C., Wang, T., Wang, L., et al. (2014). Simplified captopril analogues as NDM-1 inhibitors. Bioorg. Med. Chem. Lett. 24, 386–389. doi: 10.1016/j.bmcl.2013.10.068
Lin-Tan, D. T., Lin, J. L., Yen, T. H., Chen, K. H., and Huang, Y. L. (2007). Long-term outcome of repeated lead chelation therapy in progressive non-diabetic chronic kidney diseases. Nephrol. Dial. Transplant 22, 2924–2931. doi: 10.1093/ndt/gfm342
Livermore, D. M., Mushtaq, S., Warner, M., Vickers, A., and Woodford, N. (2017). In vitro activity of cefepime/zidebactam (WCK 5222) against Gram-negative bacteria. J. Antimicrob. Chemother. 72, 1373–1385. doi: 10.1093/jac/dkw593
Livermore, D. M., Mushtaq, S., Warner, M., and Woodford, N. (2015). Activity of OP0595/beta-lactam combinations against Gram-negative bacteria with extended-spectrum, AmpC and carbapenem-hydrolysing beta-lactamases. J. Antimicrob. Chemother. 70, 3032–3041. doi: 10.1093/jac/dkv239
Lomovskaya, O., Rubio-Aparicio, D., Nelson, K., Sun, D., Tsivkovski, R., Castanheira, M., et al. (2021). In vitro activity of the ultrabroad-spectrum beta-lactamase inhibitor QPX7728 in combination with multiple beta-lactam antibiotics against Pseudomonas aeruginosa. Antimicrob. Agents Chemother. 65, e00210-21. doi: 10.1128/AAC.00210-21
Lomovskaya, O., Rubio-Aparicio, D., Tsivkovski, R., Loutit, J., and Dudley, M. (2022). The ultrabroad-spectrum beta-lactamase inhibitor QPX7728 restores the potency of multiple oral beta-lactam antibiotics against beta-lactamase-producing strains of resistant Enterobacterales. Antimicrob. Agents Chemother. 66, e0216821. doi: 10.1128/aac.02168-21
Ma, B., Fang, C., Lu, L., Wang, M., Xue, X., Zhou, Y., et al. (2019). The antimicrobial peptide thanatin disrupts the bacterial outer membrane and inactivates the NDM-1 metallo-beta-lactamase. Nat. Commun. 10, 3517. doi: 10.1038/s41467-019-11503-3
Ma, G., Wang, S., Wu, K., Zhang, W., Ahmad, A., Hao, Q., et al. (2021). Structure-guided optimization of D-captopril for discovery of potent NDM-1 inhibitors. Bioorg. Med. Chem. 29, 115902. doi: 10.1016/j.bmc.2020.115902
Maraki, S., Mavromanolaki, V. E., Magkafouraki, E., Moraitis, P., Stafylaki, D., Kasimati, A., et al. (2022). Epidemiology and in vitro activity of ceftazidime-avibactam, meropenem-vaborbactam, imipenem-relebactam, eravacycline, plazomicin, and comparators against Greek carbapenemase-producing Klebsiella pneumoniae isolates. Infection 50, 467–474. doi: 10.1007/s15010-021-01735-1
Maraki, S., Mavromanolaki, V. E., Moraitis, P., Stafylaki, D., Kasimati, A., Magkafouraki, E., et al. (2021). Ceftazidime-avibactam, meropenen-vaborbactam, and imipenem-relebactam in combination with aztreonam against multidrug-resistant, metallo-beta-lactamase-producing Klebsiella pneumoniae. Eur. J. Clin. Microbiol. Infect. Dis. 40, 1755–1759. doi: 10.1007/s10096-021-04197-3
Marshall, S., Hujer, A. M., Rojas, L. J., Papp-Wallace, K. M., Humphries, R. M., Spellberg, B., et al. (2017). Can ceftazidime-avibactam and aztreonam overcome beta-lactam resistance conferred by metallo-beta-lactamases in Enterobacteriaceae? Antimicrob. Agents Chemother. 61, e02243-16. doi: 10.1128/AAC.02243-16
Matsumoto, S., Singley, C. M., Hoover, J., Nakamura, R., Echols, R., Rittenhouse, S., et al. (2017). Efficacy of cefiderocol against carbapenem-resistant gram-negative bacilli in immunocompetent-rat respiratory tract infection models recreating human plasma pharmacokinetics. Antimicrob. Agents Chemother. 61, e00700-17. doi: 10.1128/AAC.00700-17
Monogue, M. L., Tabor-Rennie, J., Abdelraouf, K., and Nicolau, D. P. (2019). In vivo efficacy of WCK 5222 (cefepime-zidebactam) against multidrug-resistant Pseudomonas aeruginosa in the neutropenic murine thigh infection model. Antimicrob. Agents Chemother. 63, e00233-19. doi: 10.1128/AAC.00233-19
Moya, B., Barcelo, I. M., Bhagwat, S., Patel, M., Bou, G., Papp-Wallace, K. M., et al. (2017). WCK 5107 (Zidebactam) and WCK 5153 are novel inhibitors of PBP2 showing potent “beta-lactam enhancer” activity against Pseudomonas aeruginosa, including multidrug-resistant metallo-beta-lactamase-producing high-risk clones. Antimicrob. Agents Chemother. 61, e02529-16. doi: 10.1128/AAC.02529-16
Moya, B., Barcelo, I. M., Cabot, G., Torrens, G., Palwe, S., Joshi, P., et al. (2019). In vitro and in vivo activities of beta-lactams in combination with the novel beta-lactam enhancers zidebactam and WCK 5153 against multidrug-resistant metallo-beta-lactamase-producing Klebsiella pneumoniae. Antimicrob. Agents Chemother. 63. doi: 10.1128/AAC.00128-19
Mushtaq, S., Vickers, A., Doumith, M., Ellington, M. J., Woodford, N., and Livermore, D. M. (2021). Activity of beta-lactam/taniborbactam (VNRX-5133) combinations against carbapenem-resistant Gram-negative bacteria. J. Antimicrob. Chemother. 76, 160–170. doi: 10.1093/jac/dkaa391
Mushtaq, S., Vickers, A., Woodford, N., Haldimann, A., and Livermore, D. M. (2019). Activity of nacubactam (RG6080/OP0595) combinations against MBL-producing Enterobacteriaceae. J. Antimicrob. Chemother. 74, 953–960. doi: 10.1093/jac/dky522
Nakamura, R., Ito-Horiyama, T., Takemura, M., Toba, S., Matsumoto, S., Ikehara, T., et al. (2019). In vivo pharmacodynamic study of cefiderocol, a novel parenteral siderophore cephalosporin, in murine thigh and lung infection models. Antimicrob. Agents Chemother. 63. doi: 10.1128/AAC.02031-18
Nelson, K., Rubio-Aparicio, D., Sun, D., Dudley, M., and Lomovskaya, O. (2020a). In vitro activity of the ultrabroad-spectrum-beta-lactamase inhibitor QPX7728 against carbapenem-resistant Enterobacterales with varying intrinsic and acquired resistance mechanisms. Antimicrob. Agents Chemother. 64. doi: 10.1128/AAC.00757-20
Nelson, K., Rubio-Aparicio, D., Tsivkovski, R., Sun, D., Totrov, M., Dudley, M., et al. (2020b). In vitro activity of the ultra-broad-spectrum beta-lactamase inhibitor QPX7728 in combination with meropenem against clinical isolates of carbapenem-resistant Acinetobacter baumannii. Antimicrob. Agents Chemother. 64, e01406-20. doi: 10.1128/AAC.01406-20
Nielsen, S. B., and Otzen, D. E. (2010). Impact of the antimicrobial peptide Novicidin on membrane structure and integrity. J. Colloid Interface Sci. 345, 248–256. doi: 10.1016/j.jcis.2010.01.065
Niu, S., Wei, J., Zou, C., Chavda, K. D., Lv, J., Zhang, H., et al. (2020). In vitro selection of aztreonam/avibactam resistance in dual-carbapenemase-producing Klebsiella pneumoniae. J. Antimicrob. Chemother. 75, 559–565. doi: 10.1093/jac/dkz468
Nori, P., Szymczak, W., Puius, Y., Sharma, A., Cowman, K., Gialanella, P., et al. (2020). Emerging co-pathogens: New Delhi metallo-beta-lactamase producing Enterobacterales infections in New York City COVID-19 patients. Int. J. Antimicrob. Agents 56, 106179. doi: 10.1016/j.ijantimicag.2020.106179
Pasupuleti, M., Schmidtchen, A., and Malmsten, M. (2012). Antimicrobial peptides: key components of the innate immune system. Crit. Rev. Biotechnol. 32, 143–171. doi: 10.3109/07388551.2011.594423
Piccirilli, A., Segatore, B., Brisdelli, F., Amicosante, G., and Perilli, M. (2021). Potent inhibitory activity of taniborbactam towards NDM-1 and NDM-1(Q119X) mutants, and in vitro activity of cefepime/taniborbactam against MBLs producing Enterobacterales. Int. J. Antimicrob. Agents 57, 106228. doi: 10.1016/j.ijantimicag.2020.106228
Porretta, A. D., Baggiani, A., Arzilli, G., Casigliani, V., Mariotti, T., Mariottini, F., et al. (2020). Increased risk of acquisition of New Delhi metallo-beta-lactamase-producing carbapenem-resistant Enterobacterales (NDM-CRE) among a cohort of COVID-19 patients in a teaching hospital in Tuscany, Italy. Pathogens 9:635. doi: 10.3390/pathogens9080635
Ramsey, C., and MacGowan, A. P. (2016). A review of the pharmacokinetics and pharmacodynamics of aztreonam. J. Antimicrob. Chemother. 71, 2704–2712. doi: 10.1093/jac/dkw231
Rotondo, C. M., Sychantha, D., Koteva, K., and Wright, G. D. (2020). Suppression of beta-lactam resistance by aspergillomarasmine A is influenced by both the metallo-beta-lactamase target and the antibiotic partner. Antimicrob. Agents Chemother. 64, e01386-19. doi: 10.1128/AAC.01386-19
Sader, H. S., Castanheira, M., Huband, M., Jones, R. N., and Flamm, R. K. (2017). WCK 5222 (cefepime-zidebactam) antimicrobial activity against clinical isolates of gram-negative bacteria collected worldwide in 2015. Antimicrob. Agents Chemother. 61, e00072-17. doi: 10.1128/AAC.00072-17
Salari-Jazi, A., Mahnam, K., Sadeghi, P., Damavandi, M. S., and Faghri, J. (2021). Discovery of potential inhibitors against New Delhi metallo-beta-lactamase-1 from natural compounds: in silico-based methods. Sci. Rep. 11, 2390. doi: 10.1038/s41598-021-82009-6
Sato, T., and Yamawaki, K. (2019). Cefiderocol: discovery, chemistry, and in vivo profiles of a novel siderophore cephalosporin. Clin. Infect. Dis. 69, S538–S543. doi: 10.1093/cid/ciz826
Serio, A. W., Keepers, T., and Krause, K. M. (2019). Plazomicin is active against metallo-beta-lactamase-producing Enterobacteriaceae. Open Forum Infect. Dis. 6, ofz123. doi: 10.1093/ofid/ofz123
Shi, C., Bao, J., Sun, Y., Kang, X., Lao, X., and Zheng, H. (2019). Discovery of baicalin as NDM-1 inhibitor: virtual screening, biological evaluation and molecular simulation. Bioorg. Chem. 88, 102953. doi: 10.1016/j.bioorg.2019.102953
Shirley, M. (2018). Ceftazidime-avibactam: a review in the treatment of serious gram-negative bacterial infections. Drugs 78, 675–692. doi: 10.1007/s40265-018-0902-x
Shlaes, D. M. (2013). New beta-lactam-beta-lactamase inhibitor combinations in clinical development. Ann. N. Y. Acad. Sci. 1277, 105–114. doi: 10.1111/nyas.12010
Solomkin, J. S., Sway, A., Lawrence, K., Olesky, M., Izmailyan, S., and Tsai, L. (2019). Eravacycline: a new treatment option for complicated intra-abdominal infections in the age of multidrug resistance. Fut. Microbiol. 14, 1293–1308. doi: 10.2217/fmb-2019-0135
Somboro, A. M., Amoako, D. G., Osei Sekyere, J., Kumalo, H. M., Khan, R., Bester, L. A., et al. (2019). 1,4,7-Triazacyclononane restores the activity of beta-lactam antibiotics against metallo-beta-lactamase-producing Enterobacteriaceae: exploration of potential metallo-beta-lactamase inhibitors. Appl. Environ. Microbiol. 85, e02077-18. doi: 10.1128/AEM.02077-18
Somboro, A. M., Tiwari, D., Bester, L. A., Parboosing, R., Chonco, L., Kruger, H. G., et al. (2015). NOTA: a potent metallo-beta-lactamase inhibitor. J. Antimicrob. Chemother. 70, 1594–1596. doi: 10.1093/jac/dku538
Soren, O., Brinch, K. S., Patel, D., Liu, Y., Liu, A., Coates, A., et al. (2015). Antimicrobial peptide novicidin synergizes with rifampin, ceftriaxone, and ceftazidime against antibiotic-resistant Enterobacteriaceae in vitro. Antimicrob. Agents Chemother. 59, 6233–6240. doi: 10.1128/AAC.01245-15
Sychantha, D., Rotondo, C. M., Tehrani, K., Martin, N. I., and Wright, G. D. (2021). Aspergillomarasmine A inhibits metallo-beta-lactamases by selectively sequestering Zn(2). J. Biol. Chem. 297, 100918. doi: 10.1016/j.jbc.2021.100918
Tamma, P. D., Aitken, S. L., Bonomo, R. A., Mathers, A. J., Van Duin, D., and Clancy, C. J. (2021). Infectious Diseases Society of America Guidance on the treatment of Extended-Spectrum Beta-Lactamase Producing Enterobacterales (ESBL-E), Carbapenem-Resistant Enterobacterales (CRE), and Pseudomonas aeruginosa with difficult-to-treat resistance (DTR-P. aeruginosa). Clin. Infect. Dis. 72, e169–e183. doi: 10.1093/cid/ciaa1478
Tangden, T., and Giske, C. G. (2015). Global dissemination of extensively drug-resistant carbapenemase-producing Enterobacteriaceae: clinical perspectives on detection, treatment and infection control. J. Intern. Med. 277, 501–512. doi: 10.1111/joim.12342
Thakur, P., Chawla, R., Chakotiya, A. S., Tanwar, A., Goel, R., Narula, A., et al. (2016). Camellia sinensis ameliorates the efficacy of last line antibiotics against carbapenem resistant Escherichia coli. Phytother. Res. 30, 314–322. doi: 10.1002/ptr.5535
Tooke, C. L., Hinchliffe, P., Bragginton, E. C., Colenso, C. K., Hirvonen, V. H. A., Takebayashi, Y., et al. (2019). β-Lactamases and β-lactamase inhibitors in the 21st century. J. Mol. Biol. 431, 3472–3500. doi: 10.1016/j.jmb.2019.04.002
Vazquez-Ucha, J. C., Lasarte-Monterrubio, C., Guijarro-Sanchez, P., Oviano, M., Alvarez-Fraga, L., Alonso-Garcia, I., et al. (2022). Assessment of activity and resistance mechanisms to cefepime in combination with the novel beta-lactamase inhibitors zidebactam, taniborbactam, and enmetazobactam against a multicenter collection of carbapenemase-producing Enterobacterales. Antimicrob. Agents Chemother. 66, e0167621. doi: 10.1128/AAC.01676-21
Wang, G. (2015). Improved methods for classification, prediction, and design of antimicrobial peptides. Methods Mol. Biol. 1268, 43–66. doi: 10.1007/978-1-4939-2285-7_3
Wang, X., Zhao, C., Wang, Q., Wang, Z., Liang, X., Zhang, F., et al. (2020). In vitro activity of the novel beta-lactamase inhibitor taniborbactam (VNRX-5133), in combination with cefepime or meropenem, against MDR Gram-negative bacterial isolates from China. J. Antimicrob. Chemother. 75, 1850–1858. doi: 10.1093/jac/dkaa053
Weiss, W. J., Pulse, M. E., Nguyen, P., and Growcott, E. J. (2019). In vivo efficacy of novel monobactam LYS228 in murine models of carbapenemase-producing Klebsiella pneumoniae infection. Antimicrob. Agents Chemother. 63, e02214-18. doi: 10.1128/AAC.02214-18
Westblade, L. F., Simon, M. S., and Satlin, M. J. (2021). Bacterial coinfections in coronavirus disease 2019. Trends Microbiol. 29, 930–941. doi: 10.1016/j.tim.2021.03.018
WHO (2017). WHO Priority Pathogens List for RandD of New Antibiotics. Available online at: http://www.who.int/mediacentre/news/releases/2017/bacteria-antibiotics-needed/en/ (accessed Augest 24, 2022).
Yang, Y., Guo, Y., Yin, D., Zheng, Y., Wu, S., Zhu, D., et al. (2020). In vitro activity of cefepime-zidebactam, ceftazidime-avibactam, and other comparators against clinical isolates of Enterobacterales, Pseudomonas aeruginosa, and Acinetobacter baumannii: results from China Antimicrobial Surveillance Network (CHINET) in 2018. Antimicrob. Agents Chemother. 65, e01726-20. doi: 10.1128/AAC.01726-20
Yasmin, M., Fouts, D. E., Jacobs, M. R., Haydar, H., Marshall, S. H., White, R., et al. (2020). Monitoring ceftazidime-avibactam and aztreonam concentrations in the treatment of a bloodstream infection caused by a multidrug-resistant Enterobacter sp. carrying both Klebsiella pneumoniae carbapenemase-4 and New Delhi metallo-beta-lactamase-1. Clin. Infect. Dis. 71, 1095–1098. doi: 10.1093/cid/ciz1155
Yoshizumi, A., Ishii, Y., Livermore, D. M., Woodford, N., Kimura, S., Saga, T., et al. (2013). Efficacies of calcium-EDTA in combination with imipenem in a murine model of sepsis caused by Escherichia coli with NDM-1 beta-lactamase. J. Infect. Chemother. 19, 992–995. doi: 10.1007/s10156-012-0528-y
Yu, Z. J., Liu, S., Zhou, S., Li, H., Yang, F., Yang, L. L., et al. (2018). Virtual target screening reveals rosmarinic acid and salvianolic acid A inhibiting metallo- and serine-beta-lactamases. Bioorg. Med. Chem. Lett. 28, 1037–1042. doi: 10.1016/j.bmcl.2018.02.025
Yusof, Y., Tan, D. T. C., Arjomandi, O. K., Schenk, G., and Mcgeary, R. P. (2016). Captopril analogues as metallo-beta-lactamase inhibitors. Bioorg. Med. Chem. Lett. 26, 1589–1593. doi: 10.1016/j.bmcl.2016.02.007
Zalacain, M., Lozano, C., Llanos, A., Sprynski, N., Valmont, T., De Piano, C., et al. (2021). Novel specific metallo-beta-lactamase inhibitor ANT2681 restores meropenem activity to clinically effective levels against NDM-positive Enterobacterales. Antimicrob. Agents Chemother. 65, e00203-21. doi: 10.1128/AAC.00203-21
Zhanel, G. G., Cheung, D., Adam, H., Zelenitsky, S., Golden, A., Schweizer, F., et al. (2016). Review of eravacycline, a novel fluorocycline antibacterial agent. Drugs 76, 567–588. doi: 10.1007/s40265-016-0545-8
Zhanel, G. G., Golden, A. R., Zelenitsky, S., Wiebe, K., Lawrence, C. K., Adam, H. J., et al. (2019). Cefiderocol: a siderophore cephalosporin with activity against carbapenem-resistant and multidrug-resistant gram-negative bacilli. Drugs 79, 271–289. doi: 10.1007/s40265-019-1055-2
Zhang, B., Zhu, Z., Jia, W., Qu, F., Huang, B., Shan, B., et al. (2020). In vitro activity of aztreonam-avibactam against metallo-beta-lactamase-producing Enterobacteriaceae-a multicenter study in China. Int. J. Infect. Dis. 97, 11–18. doi: 10.1016/j.ijid.2020.05.075
Zhang, Y., Hong, H., Engle, J. W., Bean, J., Yang, Y., Leigh, B. R., et al. (2011). Positron emission tomography imaging of CD105 expression with a 64Cu-labeled monoclonal antibody: NOTA is superior to DOTA. PLoS ONE 6, e28005. doi: 10.1371/journal.pone.0028005
Zhang, Y., Lin, X., and Bush, K. (2016). In vitro susceptibility of beta-lactamase-producing carbapenem-resistant Enterobacteriaceae (CRE) to eravacycline. J. Antibiot. 69, 600–604. doi: 10.1038/ja.2016.73
Keywords: antibiotic resistance, metallo-β-lactamases, novel drug strategies, Escherichia coli, Klebsiella pneumoniae, Pseudomonas aeruginosa, Acinetobacter baumannii
Citation: Li X, Zhao J, Zhang B, Duan X, Jiao J, Wu W, Zhou Y and Wang H (2022) Drug development concerning metallo-β-lactamases in gram-negative bacteria. Front. Microbiol. 13:959107. doi: 10.3389/fmicb.2022.959107
Received: 01 June 2022; Accepted: 09 August 2022;
Published: 15 September 2022.
Edited by:
Nagendran Tharmalingam, Rhode Island Hospital, United StatesReviewed by:
Asad U. Khan, Aligarh Muslim University, IndiaCharuta Agashe, Icahn Institute for Genomics and Multiscale Biology, United States
Aditya Yashwant Sarode, Columbia University, United States
Copyright © 2022 Li, Zhao, Zhang, Duan, Jiao, Wu, Zhou and Wang. This is an open-access article distributed under the terms of the Creative Commons Attribution License (CC BY). The use, distribution or reproduction in other forums is permitted, provided the original author(s) and the copyright owner(s) are credited and that the original publication in this journal is cited, in accordance with accepted academic practice. No use, distribution or reproduction is permitted which does not comply with these terms.
*Correspondence: Yuxia Zhou, emd6aG91eXV4aWFAMTYzLmNvbQ==; Hefeng Wang, d2hmLTEzOUAxNjMuY29t
†These authors have contributed equally to this work