- 1Instituto de Ciencias, Benemérita Universidad Autonóma de Puebla, Puebla, Mexico
- 2Centro de Investigaciones y Estudios Avanzados, Instituto Politécnico Nacional de México (CINVESTAV), Mexico City, Mexico
- 3Facultad de Estudios Superiores Iztacala, Universidad Nacional Autonóma de Mexico, Los Reyes Iztacala, Tlalnepantla de Baz, Edo de México, Mexico
- 4Científicos Veterinarios de México, Tepatitlán, Mexico
Pasteurellaceae family members obtain iron directly from host proteins or through siderophore-dependent mechanisms. Although Gallibacterum anatis expresses different virulence factors, its response to growth under iron restriction is unknown. G. anatis cultured in the presence of 2,2′-dipyridyl, up-expressed an approximately 65 kDa protein and repressed the expression of a 70 kDa protein. MALDI-TOF analysis of those proteins indicated homology with CirA (65 kDa), a protein involved in iron-siderophore acquisition in Mannheimia succinoproducens and a TonB-dependent receptor (70 kDa protein), a protein that binds chicken hemoglobin; however, G. anatis siderophore production was not detected by chromo azurol S (CAS)-BHI agar determination. This putative G. anatis siderophore receptor is under Fur control, but not the hemoglobin binding protein, as observed in G. anatis 12656-12 fur mutant (Ω fur 126.13) grown in the presence or not of 2,2′-dipyridyl. The addition of FeCl3 to the culture medium diminished the growth and biofilm production in approximately 30% and 35%, respectively, in the wild-type strain, but the growth of Ω fur 126.13 strain was not affected and biofilm production increased in 35%. G. anatis Ω fur 126.13 presented lower virulence when it was inoculated to 35-day-old chickens in comparison to the wild-type strain. The induction of more than one iron uptake mechanism could benefit pathogenic microorganisms such as Gallibacterium.
Introduction
Gallibacterium is a genus of the Pasteurellaceae family (Christensen et al., 2003). This genus is composed of different species, of which only Gallibacterium anatis has been associated with chickens (Bisgaard et al., 2009). This microorganism has been considered part of the autochthonous microbiota of the respiratory and lower genital tracks of chickens; however, it has also been associated with different pathologies, including salpingitis, peritonitis, and oophoritis (Neubauer et al., 2009). Lesions of the reproductive organs are responsible for a reduced egg production and increased mortality of chickens, which cause economic losses to the poultry industry worldwide (Bojesen et al., 2008).
Few virulence factors have been described to be expressed by this bacterium: chicken IgG-degrading secreted proteases (García-Gómez et al., 2005), expression of a RTX (Repeat in toxin) protein (Kristensen et al., 2010), as well as the ability to agglutinate red blood cells (Zepeda et al., 2009; Montes-García et al., 2016), and the ability to adhere to plastic surfaces and to form biofilms on glass (Vaca et al., 2011; Persson and Bojesen, 2015). This last capability could be supported by the fimbriae expression described recently (Salgado-Lucio et al., 2012; Bager et al., 2013; Kudirkiene et al., 2014; López-Ochoa et al., 2017) or by the participation of other molecules as putative adhesins like the EF-Tu protein (López-Ochoa et al., 2017). However, the response to different stresses and the putative relationship with virulence factors expression have not been studied.
Iron is an elemental microelement necessary for most microorganisms (Krewulak and Vogel, 2008). Different bacteria can acquire iron from host molecules by expressing outer membrane receptors for transferrin or hemeproteins and by producing or using siderophores from other microorganisms; the expression of most of these receptors is controlled by the ferric uptake regulator (Fur) protein. Fur protein is responsible for controlling the acquisition and transport mechanisms of iron ions in different bacteria (Jin-Won and Helmann, 2007; Krewulak and Vogel, 2008). In the present work, we identified two iron restriction-related proteins, a putative siderophore receptor under Fur control, induced in response to a lack of iron and a hemoglobin-binding protein that is not Fur-regulated when G. anatis is grown in the presence of 2,2′-dipyridyl as an iron-chelating agent. In addition, we describe the construction of a G. anatis 12656-12 mutant fur gene obtained by interruption of this gene with a streptomycin cassette using an integration vector recently described (López-Ochoa et al., 2019). Furthermore, the effect of fur interruption was evaluated by comparing the biofilm formation capability with respect to the G. anatis 12656-12 wild-type. Both strains were also evaluated with a virulence bioassay in chickens. Results obtained suggest that different iron uptake and control mechanisms could confer advantages to this avian pathogenic microorganism to grow within its infected host.
Materials and methods
Bacterial strain and growth conditions
G. anatis 12656-12 hemolytic, virulent and F149T non-hemolytic and non-virulent varieties were grown in brain heart infusion (BHI) medium for 24 h at 37°C under agitation. E. coli DH5α was used to made constructs. E. coli and Pseudomonas aeruginosa PAO1 were cultured in LB-broth to test siderophores production. G. anatis F114 and ESV34 strains hemolytic varieties (Montes-García et al., 2016) were also included in this study. Several fur-mutant strains were obtained in this work, but the mutant assayed in vivo here was Ω fur 126.13.
To eliminate the iron from the glassware, the material was soaked overnight in 0.5% ethylenediaminetetraacetic acid (EDTA) and then washed with deionized water, as previously described (Abascal et al., 2009); deionized water was used for the preparation of all solutions and culture media.
To evaluate the effect of iron on protein expression, an overnight culture of G. anatis was diluted 1:100 in flasks containing fresh BHI and incubated at 37°C under shaking until the cultures reached 0.1 optical density units at 600 nm. Next, the specific iron-chelating agent, 2,2′-dipyridyl (Sigma Aldrich, St Louis, MO, United States), was added at 0.25 mM (final concentration), keeping one flask as a control without addition. All assays were done in triplicate at least 3 times.
Collection of outer membrane proteins
G. anatis was cultured for 24 h in a 500-mL flask containing 250 mL of BHI supplemented with 2,2′-dipyridyl as above. Cells were separated by centrifugation (10,500 g during 30 min at 8°C) and suspended in 5 mL of 20 mM HEPES, pH 7.4, and lysozyme (1 mg/ml). Samples were sonicated on ice for seven cycles of 10 s on/10 s off each. After sonication, samples were processed as described previously (Abascal et al., 2009). The protein pellet was suspended in 500 μL of 20 mM HEPES supplemented with 1% Triton X-100 (final concentration, Thermo Fisher Scientific, Waltham, MA, United States). Triton-soluble or insoluble proteins were evaluated. Protein concentration was determined by the Bradford method using bovine serum albumin as standard (Bradford, 1976).
Sodium dodecyl sulfate-polyacrylamide gel electrophoresis
To identify proteins expressed by bacteria cultured in iron-restricted or in normal medium, samples containing 15 μg per well were loaded and were electrophoretically separated by 10% sodium dodecyl sulfate-polyacrylamide gel electrophoresis (SDS-PAGE). After electrophoresis, gels were stained with Coomassie blue R.
Peptide mass fingerprint
The peptide mass fingerprints of the selected outer membrane proteins (OMPs) were determined by matrix-assisted laser desorption ionization time-of-flight mass spectrometry (MALDI-TOF MS) after trypsin digestion of the proteins identified through SDS-PAGE (Abascal et al., 2009).
Siderophores
To search for siderophore production, G. anatis 12656-12 and Pseudomonas aeruginosa were grown in M9 minimum medium with 0.1 g/L polypeptone and 0.15 g/L casamino acids. Cultures were incubated under agitation for 48 h at 37°C in the presence or absence of 0.25 mM 2,2′-dipyridyl. Cultures were centrifuged as above, and cell-free culture supernatants were filtered through a 0.22-μm nitrocellulose membrane. The spent medium (2 mL) was mixed with the same volume of a 2% (w/v) FeCl3 solution, and the production of siderophores was assayed by spectrophotometric measurements from 400 to 600 nm according to Neilands (1981). Siderophore production was also evaluated on chrome azurol S (CAS)-BHI agar as described by Tabatabai et al. (2008) using P. aeruginosa O1 and Escherichia coli DH5α as positive and negative (Chart et al., 2000) siderophore-producer strains, respectively.
Chicken hemoglobin procurement
Chicken hemoglobin was obtained according to Drabkin (1946). Briefly, 0.9% NaCl (final concentration) was added to 200 mL of chicken blood. Red blood cells were collected by centrifugation at 2,000 rpm for 5 min. Chicken erythrocytes were lysed with distilled water (2 volumes), and membrane cells were removed in the same conditions. Chicken hemoglobin was recovered by precipitation with 35%, 50%, and 70% ammonium sulfate. Proteins recovered with 70% ammonium sulfate were dialyzed against PBS and lyophilized for storage. Chicken hemoglobin was dissolved and biotinylated as previously described (Kittigul et al., 1998).
Immune recognition
To evaluate the immunogenicity of stressed and unstressed cultures of G. anatis, extracted proteins were separated by 10% SDS-PAGE and transferred to a nitrocellulose membrane, as described previously, to determine proteins over- or under-expressed in the iron-restricted medium (Abascal et al., 2009). In this assay, membranes were incubated under one of the three following conditions: with a 1:1,000 diluted polyclonal serum pooled from chickens experimentally infected with G. anatis (Salgado-Lucio et al., 2012), anti-transferrin binding proteins (TbpA and TbpB) from Avibacterium paragallinarum (Abascal et al., 2009), or biotinylated-chicken hemoglobin. Next, the membranes were washed three times with phosphate-buffered saline-0.5% Tween 20. The immune reaction was revealed with rabbit IgG anti-chicken antibody, goat IgG anti-rabbit antibody or avidin, all of which were peroxidase- labeled, using diaminobenzidine and H2O2 as substrates.
G. anatis 12656-12 locus fur amplification and cloning
To amplify a DNA fragment containing the G. anatis fur gene (593 bp), oligonucleotides GfurAm (ccacaaaacggcttatcaatctca) and GfurA2m (ttaatttatatgccggtgcgtgaa) were designed based on the genomic sequencing data (NC_015460.1). They were designed considering the left and right fragments flanking the G. anatis UMN179 fur gene sequence (Figure 1) to amplify a 2.2 kbp fragment and increase the possibility to obtain a double crossover recombination on this fragment when G. anatis cells were transformed (Kristensen et al., 2012). G. anatis 12656-12 genomic DNA was obtained according to Sambrook et al. (1989), and the fur locus was amplified by polymerase chain reaction (PCR) under the following conditions: 94°C, 5 min; 94°C, 30 s, 55.5°C, 30 s, and 72°C, 2.5 min (28 cycles); and 72°C, 10 min. The PCR amplification product was visualized in a 0.8% agarose gel and cloned into the pBluescript KS II(-) vector (Stratagene, Kirkland, WA, United States), generating the plasmid pSAMfur. Plasmid DNA was purified using the Wizard Plus SV Miniprep DNA Purification System (Promega, Madison, WI. United States) and sequenced in a Perkin-Elmer analyzer (ABI PRISM® 3100 sequencer) using M13 reverse and forward universal primers. The sequence obtained was analyzed through the BLASTn, BLASTp1, and ClustalW2 programs.
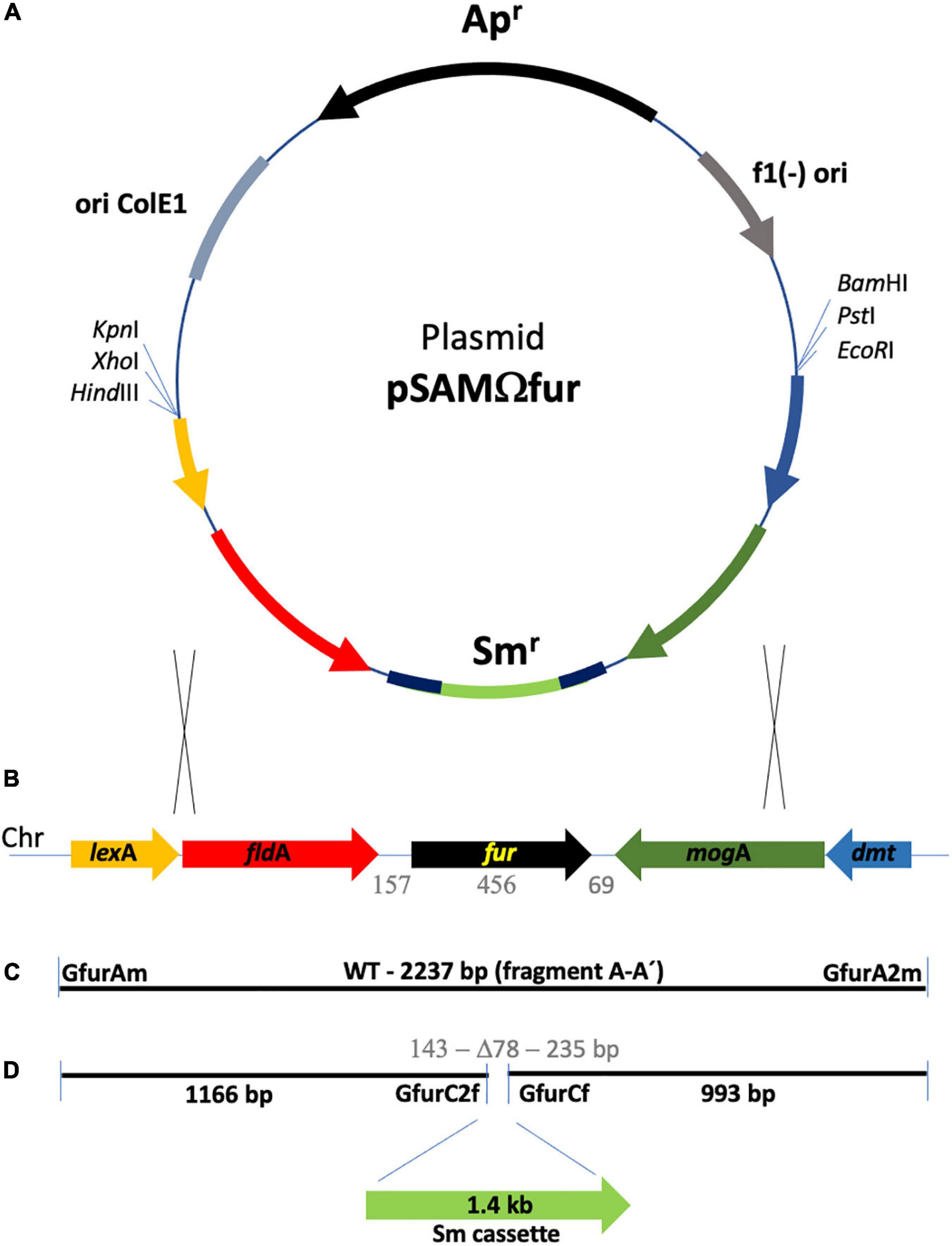
Figure 1. Schematic representation of the fur locus in the G. anatis 12656-12 genome and strategy of its disruption. (A) Diagram of plasmid pSAMΩfur, the one-step disruption cassette contains a streptomycin resistance gene flanked by regions of homology shown in red (left) and green (right). (B) Map of the fur locus located on the chromosome of G. anatis 12656-12; lexA, fldA, fur, mogA, and dmt are represented as arrows. The genes are homologous to those held in loci UNM179_R10075 to UMN179_R10095 from strain G. anatis UMN179 (accession NC_015460.1). (C) The A-A’ fragment is a PCR amplification product of 2,237 bp, using GfurAm and GfurA2m primers. The gray numbers indicate the size in base pairs of fur and adjacent intergenic sequences. (D) The sequences flanking the deletion site at the fur locus are 1,166 and 993 bp in size and were ligated to a 1.4 kb streptomycin cassette as part of the plasmid shown in A. pSAMΩfur is an integration plasmid that contains the colE1 replicon but is unable to replicate in G. anatis; therefore, resistance to streptomycin will only be possible after integration of the cassette into the chromosome. Replacing the fur WT locus with the one-step disruption cassette is indicated by two pairs of crossed lines; the interrupted A-A’ fragment increases the size of the locus by 1.4 kb. The gray numbers indicate the 78 bp deletion and, on the sides, the size of the remaining fur DNA in the construct.
G. anatis fur gene interruption
Once the presence of the fur gene in the locus (2.2 kb) cloned in pSAMfur was confirmed, an inverse PCR to get the fur gene interruption was performed to open the construct under the following conditions: 94°C, 5 min; 94°C, 30 s, 47°C, 30 s, and 72°C, 5 min (28 cycles); and 72°C, 10 min using primers GfurCf (tcgccataattttgaaggaa) and GfurC2f (aaccatttataaacatcttcag) (Figures 1B,C). The amplified product (5.1 kb) was used to clone a streptomycin cassette (1.4 kbp), obtained from a purified plasmid (pOV) from a multi-resistance pig Pasteurella multocida isolated and previously characterized (López-Ochoa et al., 2019), using primers Str2L (gatatcgaagggggtagttggt) and Str3U (gatatcatttgccggtgcttctgt) within the fur coding sequence, generating the plasmid pSAMΩfur (Figures 1A,D). Plasmid pSAMΩfur was sequenced to verify the construct and was used to transform competent G. anatis 12656-12 cells obtained, as described by Kristensen et al. (2012), using M-IV chemical transformation; double recombination events were selected by the streptomycin-resistant phenotype.
Genomic verification of the G. anatis 12656-12 fur mutants
Genomic DNA from G. anatis 126.13 and 126.5 Ω fur strains, as well as pSAMΩfur, and wild-type G. anatis cultures was extracted with phenol-chloroform and used to verify the replacement of the chromosomal fur gene in G. anatis 12656-12 by PCR amplification of the interrupted fur locus using primers GfurAm and GfurA2m, as above. This was also performed by DNA-DNA hybridization of genomic DNA digested with HindIII restriction enzyme and separated by electrophoresis on an agarose gel (0.8%). Separated DNA was transferred to a nylon membrane (Millipore-Merck, Sigma Aldrich, St. Louis, MO, United States) and hybridized with α32P-dCTP-labeled flavodoxin-fur (using primers Gmog-tgcgcccgttacttatacc and Gseq-cacctgatgcaacgttacgcc) or streptomycin cassette probes obtained by PCR. The signals representing hybridized DNA were: 4.2 kbp for WT-HindIII-fragment in size and 5.6 kbp for fur mutants and were observed by Kodak X-ray film-exposure (Vázquez-Cruz and Olmedo-Álvarez, 1997).
Effect of FeCl3 on G. anatis growth and biofilm formation
To know the effect of FeCl3 on the growth of G. anatis 12656-12 strain and its Ω fur 126.13 mutant, bacteria were grown in BHI medium supplemented with 100 μM FeCl3 in overnight cultures and determining the OD600 nm. The biofilm production by both strains was evaluated, as previously described, in a medium supplemented or not with 100 μM FeCl3 (Vaca et al., 2011). Biofilm quantification was determined through OD595 nm lectures of resolubilized Hucker’s crystal violet in 33% glacial acetic acid solution.
Virulence assay
To evaluate if the inactivation of G. anatis fur gene had any effect on the virulence, 35-day-old clinically healthy, specific-pathogen-freeleghorn chickens (SPF, Puebla, Mexico) were used in the study. Chickens were housed in isolators with antibiotics-free food and bottled water ad libitum. Groups of 10 chickens were separated in a single isolator and infected by nasal instillation with 0.2 mL of an overnight culture of wild type G. anatis or its mutant Ω fur 126.13 containing 1 × 108 CFU/mL (Trujillo-Ruíz et al., 2016). One group was instilled with 0.2 mL of PBS as a control. Chickens were examined for 10 days and euthanized after the last examination. Bacterial isolation was attained by sampling infraorbital sinuses and different organs and cultured on BHI blood agar.
Bioinformatics analysis of TonB-dependent receptors in G. anatis
The TonB-dependent receptors were analyzed using as a seed the TonB-dependent receptors included in tonB operons from G. anatis 12656-12, F149T, and UMN179 (AVOX01000000.1, JPHN01000000.1, NC_015460.1). The last one because it is in a circular genome and is a genomic reference. Regulatory sequences were predicted on nucleotide sequences from the TonB-dependent receptor and fur genes with Bprom from Softberry3. Proteins were analyzed by BLASTp4, in single and grouped mode. Alignments were done with Clustal Omega5 and the phylogenetic tree was redrawn with iTOL Tree viewer6. Amino acid sequences were also compared with 3D models in PDB7 and SWISS-MODEL8. Prediction of beta-barrel outer membrane protein was done with PRED (http://bioinformatics.biol.uoa.gr/PRED-TMBB) Supplementary Figure and Supplementary Data Sheet.
Results
Iron restriction conditions
The growth of G. anatis wild type or Ω fur 126.13 strains in the presence of 2,2′-dipyridyl exhibited a reduction of approximately 60% and 37%, respectively, compared with cultures with no addition of 2,2′-dipyridyl. The expression of an up-induced 65-kDa protein (Figure 2A lane 2) or a down-induced 70 kDa (Figure 2A, lane 3) in iron restriction condition was observed in triton-insoluble (lanes 1 and 2) and Triton-soluble (lanes 3 and 4) Omps from G. anatis 12656-12, respectively.
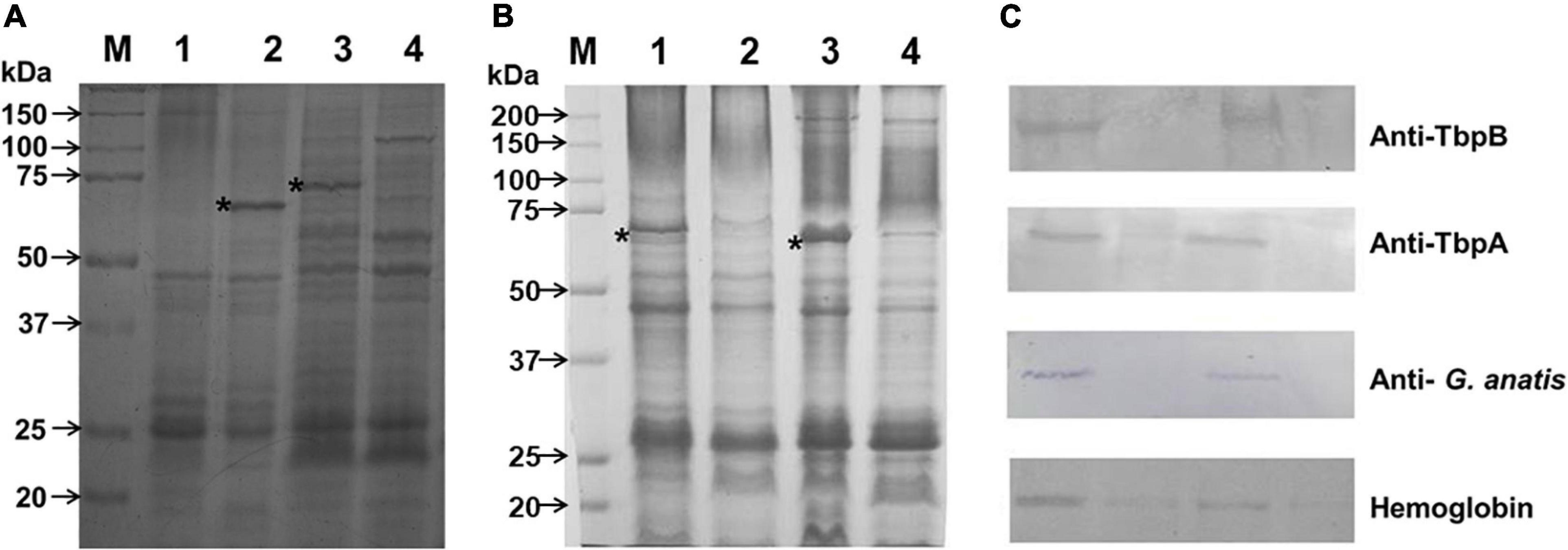
Figure 2. Up and down expression of proteins due to iron depletion. (A) Triton-insoluble (lanes 1 and 2) and Triton-soluble (lanes 3 and 4) proteins from G. anatis 12656-12 were separated by electrophoresis on 10% polyacrylamide gels and stained as described under Material and methods. The bacterium was grown under iron-restricted conditions (lanes 2 and 4) or normal conditions (lanes 1 and 3); the 65-kDa and 70-kDa proteins are shown in lanes 2 and 3, respectively (*). (B) Differential expression of the 65-kDa protein when G. anatis F149T (lanes 1 and 2) or 12656-12 (lanes 3 and 4) were grown under iron-restricted (lanes 1 and 3) or normal conditions (lanes 2 and 4). *Indicates 65- kDa protein in Triton-insoluble samples. (C) Immunological recognition of the 65- kDa iron-restricted protein from G. anatis F149T and 12656-12 by polyclonal sera against TbpB and TbpA proteins, both from A. paragallinarum, or by pooled sera from chickens infected with G. anatis; or interaction with biotinylated chicken hemoglobin.
Western and Far western blotting
By polyacrylamide gel electrophoresis, a band of approximately 65 kDa corresponding to a Triton-insoluble OMP was observed in samples of G. anatis grown in iron-restricted conditions but not in cultures without the addition of 2,2′-dipyridyl (Figure 2B). This OMP was immune-recognized by rabbit polyclonal antiserum against TbpB and TbpA from A. paragallinarum, indicating its relationship with the structure of iron-binding proteins (Figure 2C), or by sera from chickens infected with G. anatis (Figure 2C), suggesting its expression in vivo. Additionally, this protein was able to interact with biotin-labeled chicken hemoglobin (Figure 2C).
An approximately 70 kDa OMP, from total cell extracts (Figure 3B), interacted with biotin-labeled chicken hemoglobin in samples of G. anatis grown in the presence or absence of 2,2′-dipyridyl, and was expressed at lower levels by G. anatis 12656-12 in comparison with the F149T strain (Figure 3B); however, this interaction was more intense in samples from G. anatis grown in the absence of the chelating agent (Figure 3B). This protein was also immune-recognized by the serum of chickens infected with G. anatis, suggesting that this protein is expressed in vivo (Figure 2C).
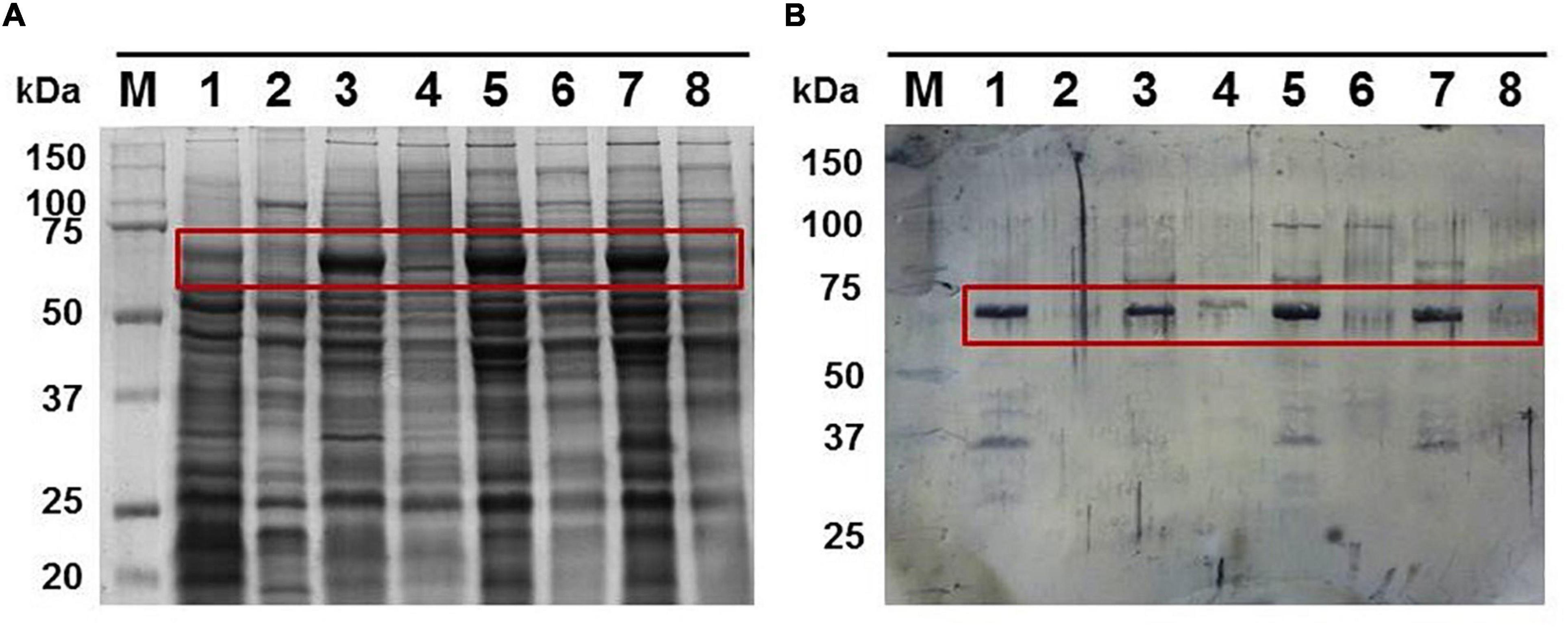
Figure 3. (A) Total cell extracts protein pattern in a 10% polyacrylamide gel from different G. anatis grown in normal (lanes 1, 3, 5, and 7) or iron-restricted conditions (lanes 2, 4, 6, and 8) of the 12566-12, F149T, F114, and ESV34 strains, respectively. The rectangle indicates the hemoglobin-binding protein. Twenty-five micrograms were loaded per well. (B) Interaction of proteins with biotinylated chicken hemoglobin.
OMPs identity
Tryptic digestion of the 65-kDa OMP produced four peptides with a mass-to-charge ratio (M/z) ranging from 440 to approximately 800. The peptide mass fingerprint was compared using the Mascot server9. The mass spectrometry analysis indicated that these peptides presented identity with a ferric iron-catecholate outer membrane transporter (CirA) from Mannheimia succiniciproducens MBEL55E (accession number: YP_087708) and with a TonB-dependent receptor from G. anatis F149T (accession number KGQ57895.1) (Supplementary data sheet 1). This result indicates that G. anatis could have the ability to uptake iron from siderophores.
The peptides obtained by MALDI-TOF analysis of the 70-kDa protein indicated that this protein was a TonB-dependent heme/hemoglobin receptor family member from G. anatis (access number: WP_013747074.1). A similar protein was also identified in OMP samples from G. anatis F114 and ESV34. This result indicates that this protein is conserved in other G. anatis strains and presents a similar response to iron stress (Figure 3A).
Siderophore search
To determine whether G. anatis, in addition to getting siderophores, could also produce them, a spectrophotometric determination was performed. Spent media from P. aeruginosa presented a maximum absorption at approximately 400–450 nm in the presence or absence of 2,2′-dipyridyl; being higher in the presence of the chelating agent and suggesting the presence of siderophores; however, this change was not observed with spent media from G. anatis. A similar result was also observed in CAS-BHI agar. A yellow-orange halo was observed with P. aeruginosa strains, indicating the production of siderophores, but not around G. anatis or E. coli DH5α, which was used as a negative control (data not shown).
G. anatis fur amplification
The possibility that the putative hemoglobin and siderophore receptors would be under positive or negative control by a Fur protein was answered by amplification, cloning, and interruption of the G. anatis 12656-12 fur gene. PCR amplification of the G. anatis 12656-12 locus fur gene gave a 2.2 kbp product containing a 593 bp fur gene flanked by the fldA and mogA genes in a similar manner as reported for G. anatis UMN179 (pSAMfur). The sequences of the G. anatis 12656-12 fur locus and fur gene share 97% similarity with those reported for the UMN179 strain (YP_004420910.1). pSAMfur was amplified by inverse PCR using primers GfurC2F and GfurCf to introduce a streptomycin cassette and interrupt the fur gene to generate pSAMΩfur (Figure 1A).
G. anatis fur mutant generation
Plasmid pSAMΩfur was introduced into G. anatis 12656-12 by a M-IV chemical transformation step. G. anatis 12656-12-pSAMΩfur was cultured in the presence of streptomycin to select pSAMΩfur. Cells were plated on BHI agar plus streptomycin and patched on the same agar plates to identify colonies where homologous recombination had occurred. In total, 12 putative mutants were recovered that were streptomycin resistant. Five of these putative mutants were selected to verify that homologous recombination had occurred in the selected DNA locus and were manipulated in vitro. Chromosomal DNA from the parent, 12656-12 strain, and from five putative mutants, 1: Ω fur 126.13, 2: Ω fur 126.5, 3: Ω fur A1, 4: Ω fur A2, and 5: Ω fur B2, were analyzed by PCR. Primers specific to the fur locus amplified a 3.6-kbp product from the putative mutants and a 2.2 kbp product from the wild-type strain 12656-12 (Figure 4). The latter suggested that all five putative mutants had a fur gene interrupted by the streptomycin cassette.
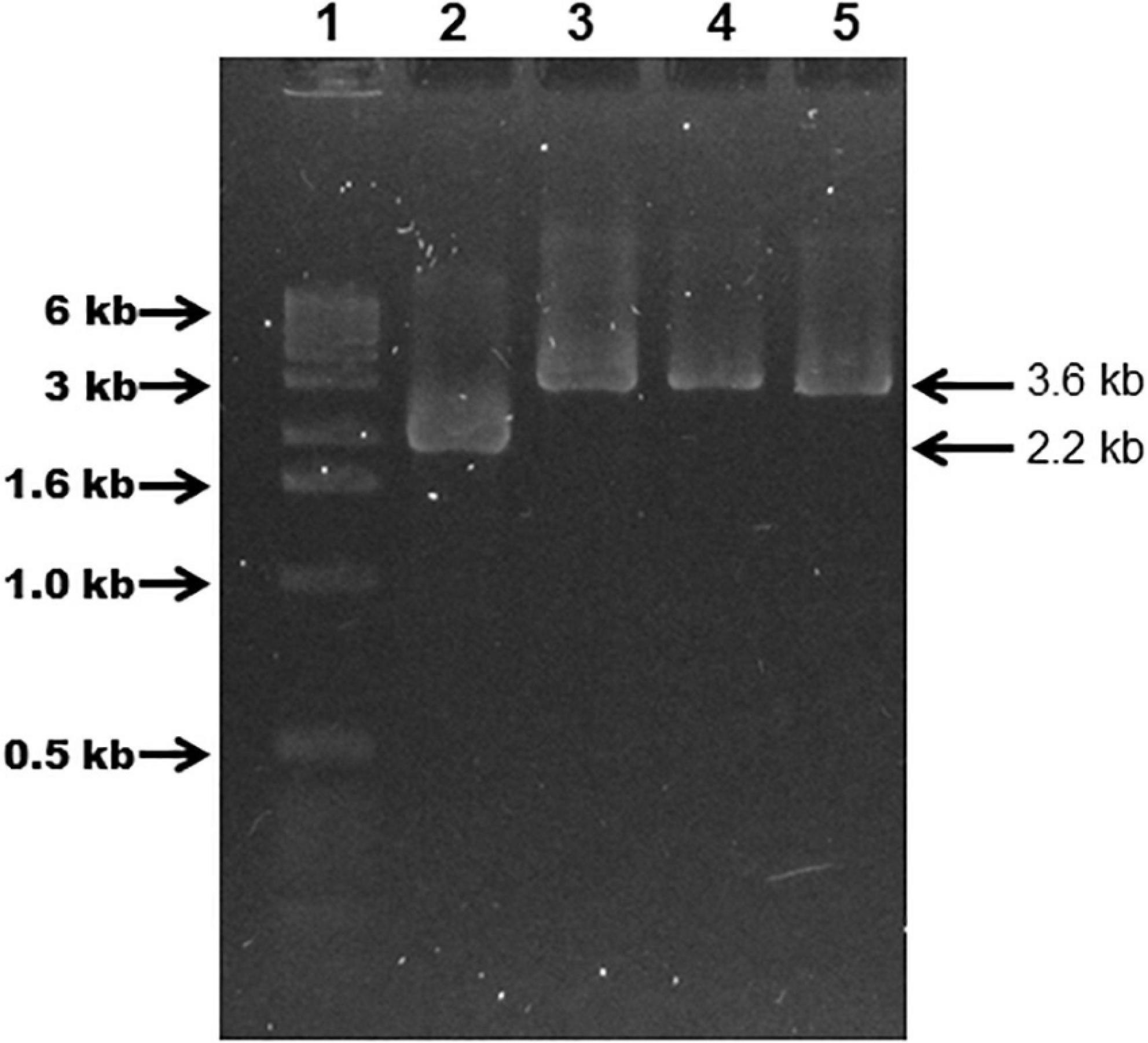
Figure 4. G. anatis 12656-12 (Ω fur) streptomycin-resistant mutants verified by PCR. Agarose gel (0.9%) showing 12656-12 fur WT (2.2 kbp band) and mutant fur::sm (3.6 kbp band) PCR amplification products obtained with G. anatis total DNA. (1) MWM, (2) G. anatis 12656-12, (3) construct pSAMΩfur in E. coli, (4) G. anatis Ω fur 126.3, (5) G. anatis Ω fur 126.5.
G. anatis fur mutant verification
Chromosomal DNA from the wild-type G. anatis 12656-12 and the putative fur mutants was digested with HindIII enzyme, separated by agarose gel electrophoresis (Figures 5A,C), transferred onto a nylon membrane, and hybridized with flavodoxin-fur (Figure 5B) or streptomycin cassette (Figure 5D) probes. The flavodoxin-fur probe hybridized with the 5.6-kbp DNA band in the G. anatis mutants and a 4.2-kbp band in the wild-type strain (Figure 5B). The streptomycin probe hybridized only with DNA from the putative G. anatis fur mutants (Figure 5D). All these results confirmed that the fur locus had been interrupted and replaced in these strains.
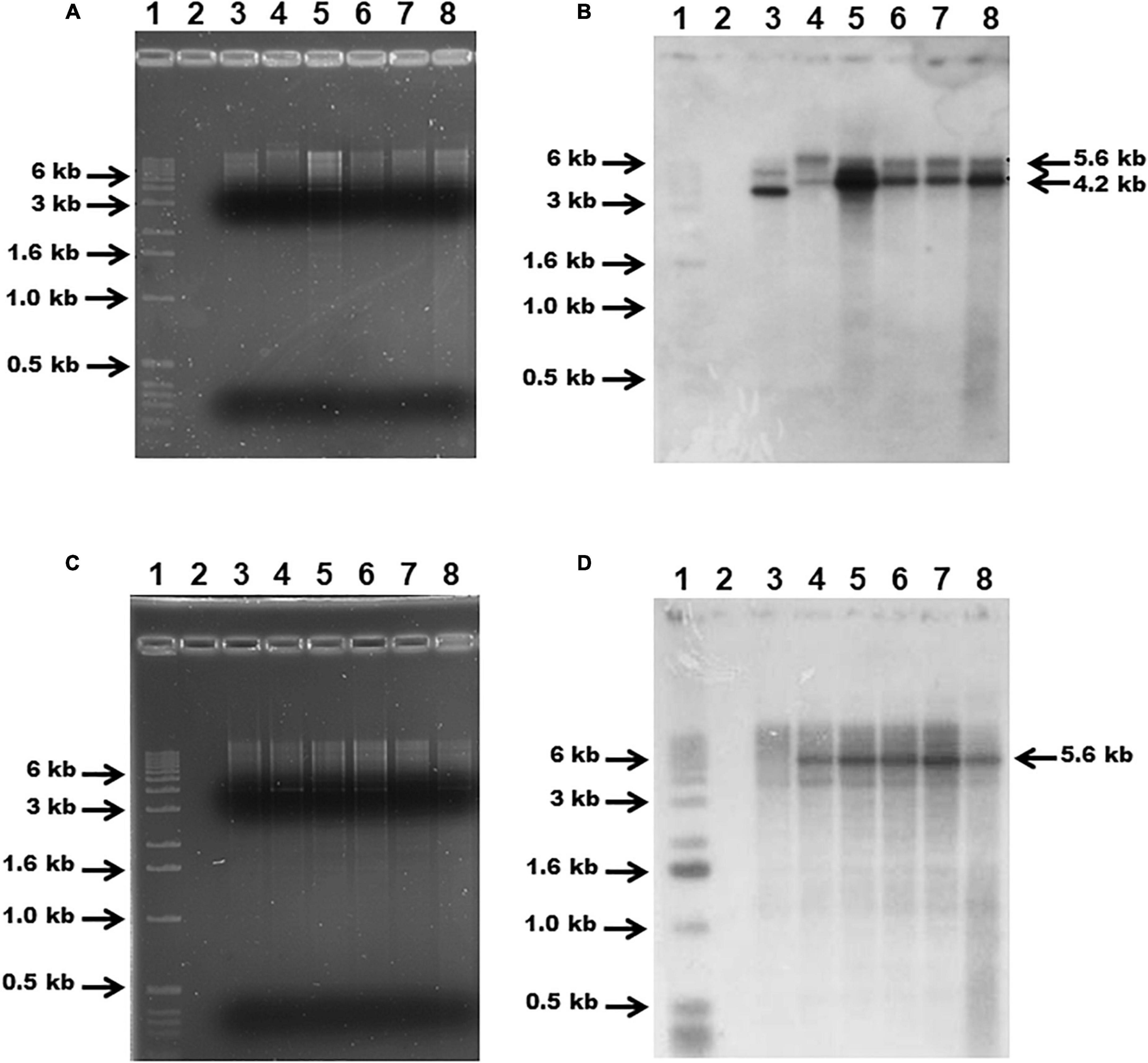
Figure 5. Agarose gel (1%) electrophoresis of G. anatis total DNA digested with HindIII (A,C) and DNA-DNA hybridization (B,D): using flavodoxin-fur [2.2 kbp, (B)] or streptomycin [1.5 kbp, (D)] gene probes, respectively. (1) 1 kbp MWM, (2) empty, (3) G. anatis 12656-12 (wt). The mutants are in the following lines: (4) Ω fur 126.13, (5) Ω fur 126.5, (6) Ω fur A1, (7) Ω fur A2, (8) Ω fur B2.
Putative siderophore receptor is under control of Fur
When the wild-type G. anatis 12656-12 and the 126.13 fur mutant were grown in the presence or absence of 2,2′-dipyridyl, a 65-kDa protein was expressed only in the presence of the chelating agent in the wild-type 12656-12 strain but was constitutively expressed in the Ω fur 126.13, in both conditions, confirming that the expression of the putative siderophore receptor is regulated by Fur (Figure 6A). However, expression of the 70-kDa protein diminished in the presence of 2,2′-dipyridyl in both wild-type 12656-12 and Ω fur 126.13 (Figure 6B), leading us to conclude that the putative hemoglobin-binding protein is not Fur controlled.
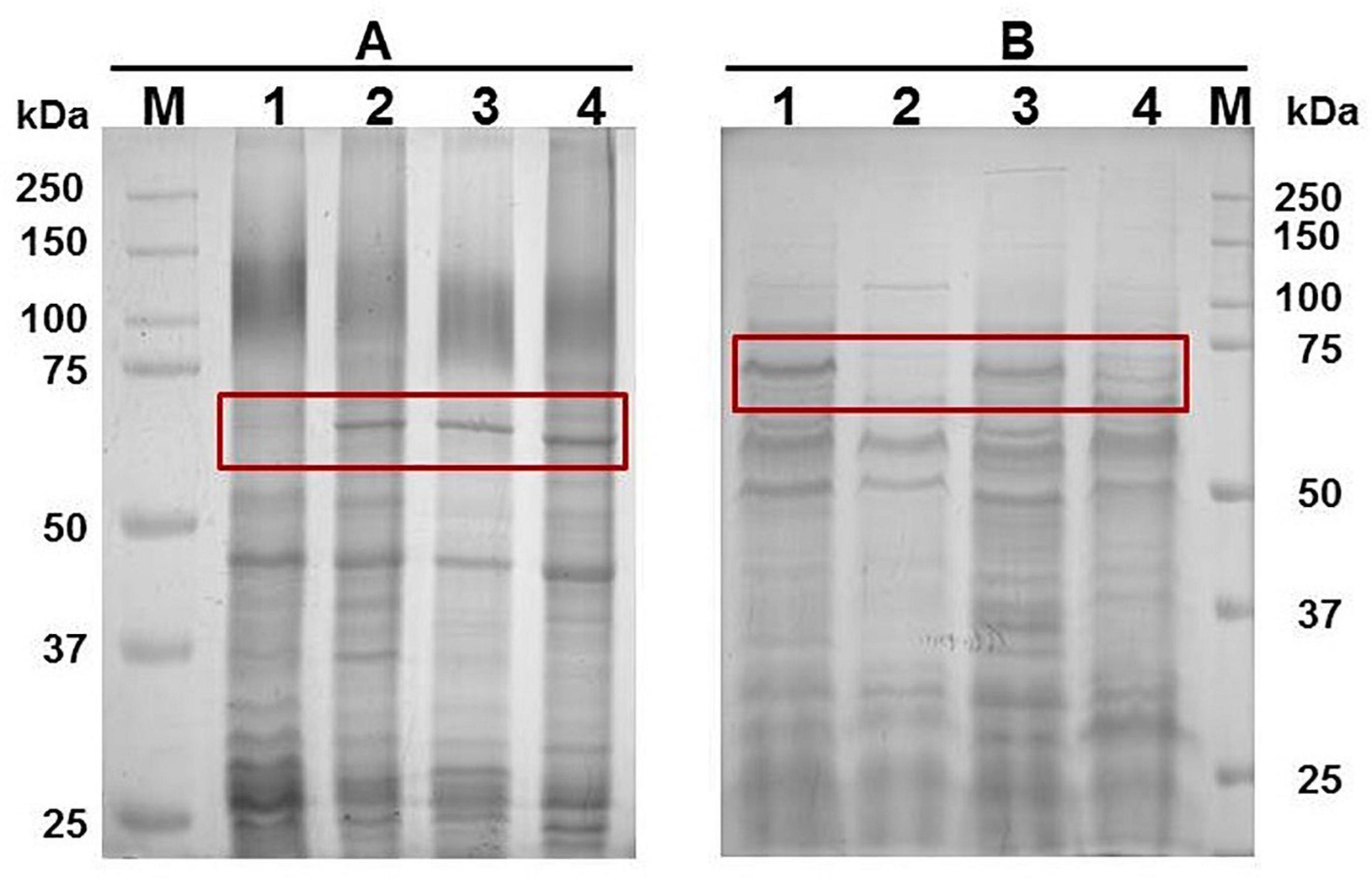
Figure 6. Ten percent polyacrylamide gel showing the 1% Triton-insoluble (A) or soluble (B) protein patterns of G. anatis 12656 WT (lanes 1 and 2) and Ω fur 126.13 (lanes 3 and 4) without (lanes 1 and 3) or with 2,2′-dipyridyl (lanes 2 and 4).
Effect of FeCl3 on G. anatis growth and biofilm production
The addition of FeCl3 to the 12656-12 strain culture medium diminished growth (approximately 30%) with respect to the culture without addition; however, the growth of Ω fur 126.13 was not affected (Figure 7). Also, in G. anatis 12656-12, presence of FeCl3 diminished biofilm production in 35%; in contrast, in the Ω fur 126.13, biofilm production increased in a similar percent (Figure 8), without a significant difference.
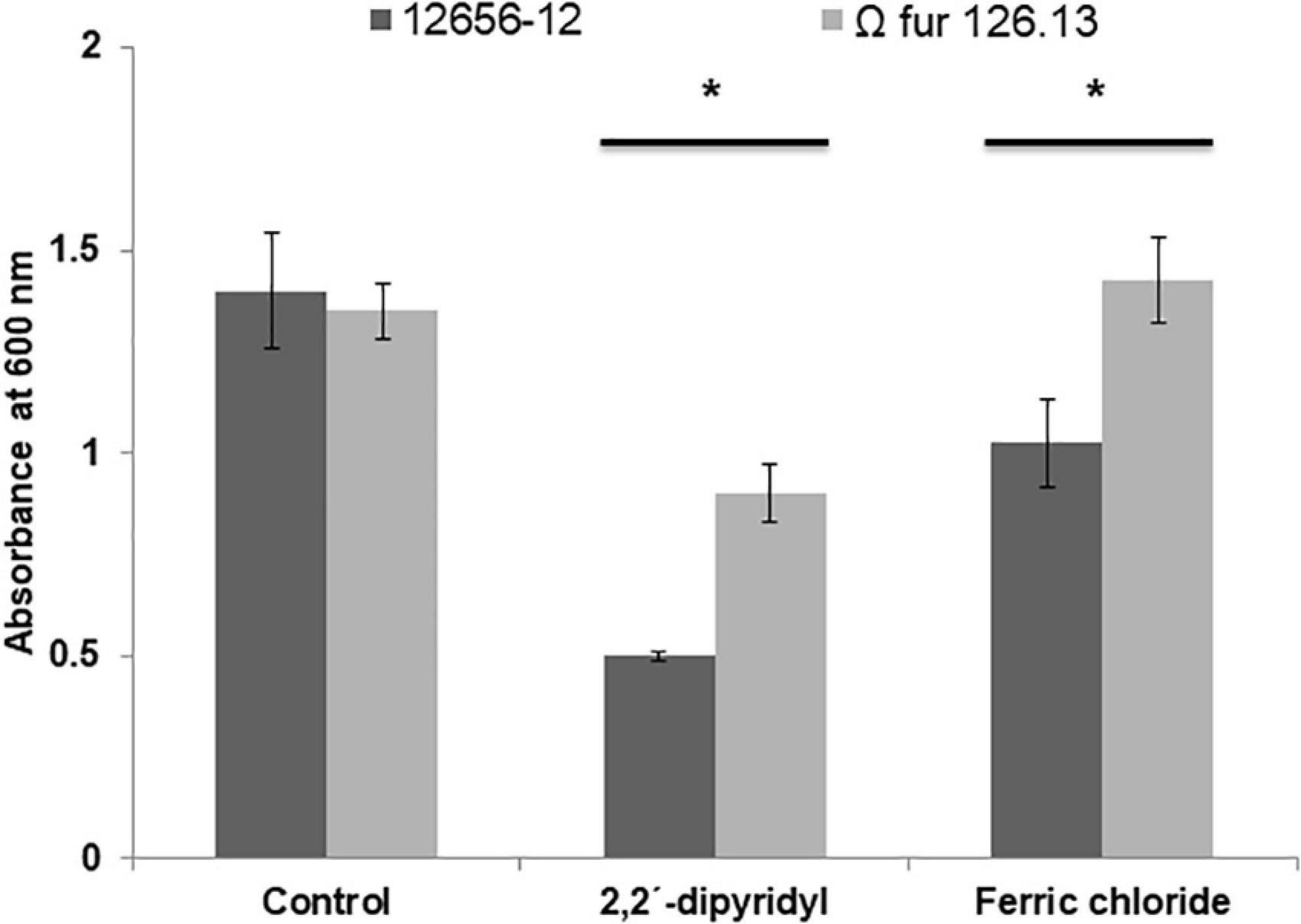
Figure 7. Growth of G. anatis 12656-12 and Ω fur 126.3 strains in 24-h cultures supplemented or not with 2,2′-dipyridyl or FeCl3. *Significant difference (P < 0.05), compared with the control.
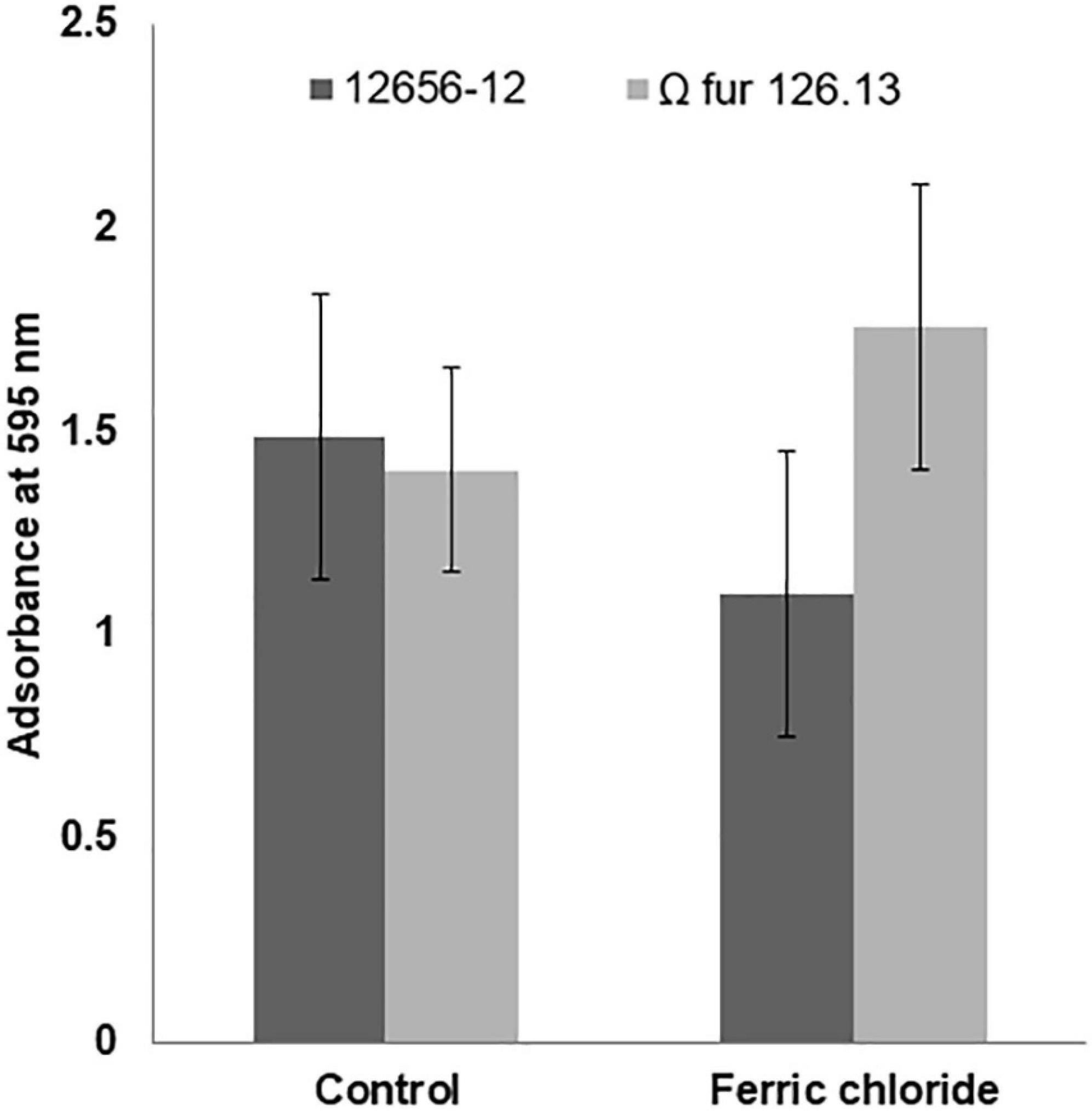
Figure 8. G. anatis 12656-12 and Ω fur 126.3 strains biofilm production in 24-h cultures supplemented or not with FeCl3.
G. anatis chicken inoculation
Chickens did not present any clinical signs along the 10 days post-inoculation. When re-isolation of G. anatis wild-type or Ω fur 126.13 strains were tried, 60% of the birds inoculated with the 126.13 strain were positive in heart samples; on the other hand, the wild-type strain was isolated in 20% of samples but from different organs (Table 1). G. anatis was not isolated from control group birds.
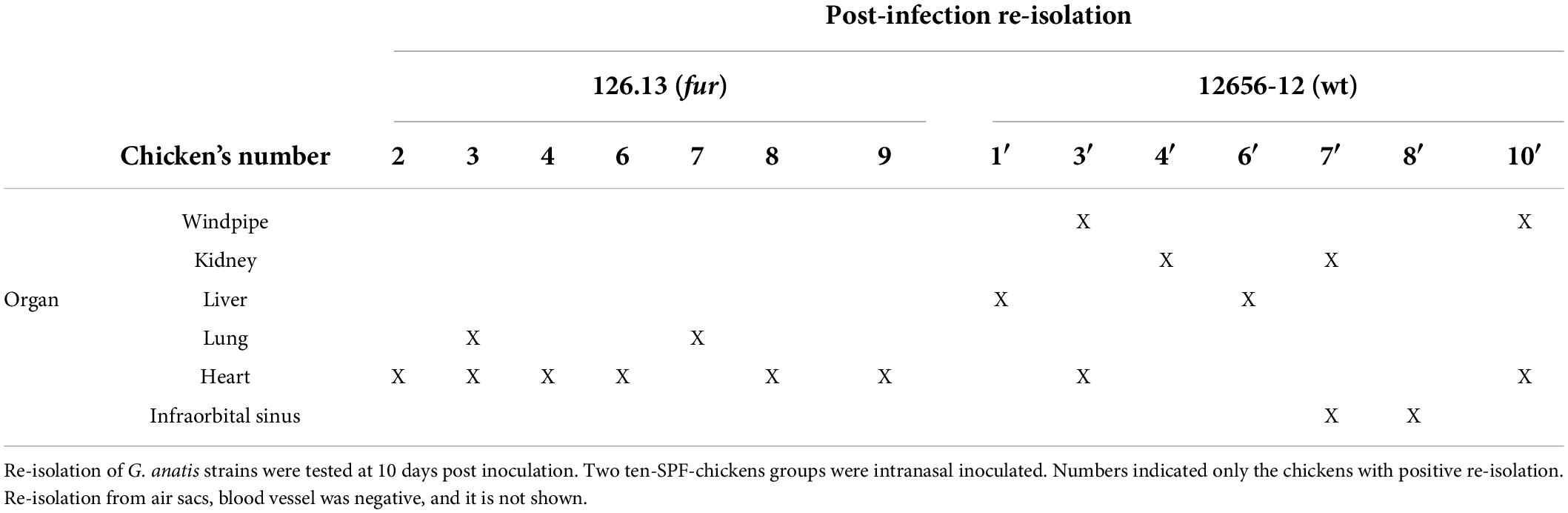
Table 1. Re-isolation of G. anatis 12656-12 and Ω fur 126.3 strains from SPF chickens intranasally inoculated.
Discussion
Iron restriction is a non-specific host mechanism that limits the optimal conditions for the reproduction of microorganisms. However, most microorganisms have a repertoire of different strategies, considered as virulence factors, to obtain the necessary micronutrients. To survive in a host with restricted micronutrients, such as iron, pathogenic microorganisms must be able to withdraw iron bound by the host’s iron-binding proteins. Iron can be obtained by the production of low molecular weight molecules with high affinity to iron, called siderophores, or of outer membrane proteins with the capability to bind host transferrins or hemoproteins. In this work, the ability to overexpress proteins from G. anatis grown under iron-restricted conditions was evaluated. G. anatis grown in the presence of 2,2′-dipyridyl exhibited a reduction (60%) in its growth with respect to cultures with no addition of 2,2′-dipyridyl (Figure 7), similarly as described for A. paragallinarum, another Pasteurellaceae member that also infects chickens (Abascal et al., 2009).
G. anatis iron-restricted growth induced the up-expression of a putative siderophore receptor (65-kDa protein), and the down-expression of a putative hemoglobin receptor (70-kDa protein), both proteins were immune-recognized by sera of chickens infected with G. anatis, indicating their in vivo expression. However, although G. anatis expresses a putative siderophore receptor, this bacterium does not produce siderophores as was observed by spectrophotometric determinations and chromo azurol-BHI agar assays. This result was also supported by the absence of genes involved in siderophore synthesis within the G. anatis genome (NC_015460.1). However, G. anatis possess, besides a putative siderophore receptor, other proteins, like the iron ABC transporter permease (WP_013745286.1 and WP_013746863.1) and an FTR1 family iron permease protein (WP_013745724.1), which could be involved in the transport of siderophores produced by other microorganisms. It has been described that Pasteurellaceae members do not produce siderophores (Cornelissen, 2003), but that they contain the genetic components to use those produced by other microorganisms (Morton et al., 2010).
There is evidence that iron-uptake genes’ regulation is largely due to Fur proteins. Fur proteins function mainly by negative regulation; however, there is evidence indicating that Fur proteins can also act as transcriptional activators (Jin-Won and Helmann, 2007; Runyen-Janecky, 2013). In this case, it seems that both negative and positive actions of gene regulation could be exerted by Fur or that other bacterial gene expression regulators could be participating, with respect to the putative G. anatis siderophore and hemoglobin receptors, respectively. This effect can be observed in the gel (Figure 2). The putative siderophore receptor (65-kDa protein) was found at a higher concentration in samples of G. anatis grown in iron-restricted conditions, and lower in samples under normal conditions. In contrast, the putative hemoglobin-binding protein (70-kDa protein from the culture without the added chelating agent) was at a higher concentration in samples from the F149T strain, and a tiny band was observed in iron-restricted conditions (Figure 4). G. anatis F149T is a non-hemolytic and non-virulent strain with few studies on its participation in gallibacteriosis (Montes-García et al., 2016; Zhang et al., 2017). However, this G. anatis strain also expresses different virulence factors as virulent strains do, but in a lower amount. The higher expression of the putative hemoglobin-binding protein by G. anatis F149T strain could be due to an incapacity to hemolyze red blood cells to get the necessary iron. A higher amount of this protein could ensure the supply of the necessary iron for this bacterium.
The constitutive expression of the putative G. anatis siderophore receptor in G. anatis fur mutants in the presence or not of 2,2′-dipyridyl indicates that this putative receptor is under control of the Fur protein, however, no changes were observed in the expression of the putative hemoglobin receptor in the G. anatis 12656-12 wild-type or its fur mutant, which indicates that the 70-kDa protein is not under Fur control. Besides the 70-kDa protein that interacts with the chicken biotinylated hemoglobin, two proteins of 35 kDa and 100 kDa also interact with hemoglobin (Figure 3B); but they will be the object of a future study.
Among bacteria from the Pasteurellaceae family several receptors have been implicated in the uptake of different sources of iron and annotated as proteins dependent on the TonB transport system (Whitby et al., 2009), i.e., the enterobactin, hemoglobin-transferrin, and siderophore receptors, in addition to others with unclear function (Lyles and Eichenbaum, 2018; Otero-Asman et al., 2019); moreover, along with these receptors, there is at least one that seems to be a pseudogene, as it is a truncated version of the aforementioned receptors containing only the carboxyl terminal sequence (accession ERF78509.1). In this work, we employed the G. anatis 12656-12 and F149T strains as an experimental system, and the G. anatis UMN179 as a reference strain to analyze the behavior of the protein-receptors in a Fe++-deficient environment. G. anatis UMN179 is the only known strain whose circularized genome has been publicly released (accession number NC_015460.1 under Material and methods). In this work, the locus containing an operon-like arrangement was identified, where the enterobactin or hemoglobin transporter gene was located, along with the putative genes encoding the periplasmic proteins ExbB and ExbD, and the plug or stopper protein TonB. Gene organization of the predicted operon is conserved in a single copy in the three strains, as seems to be its function, so disturbances affecting our system could be minimal. Two other genes encoding additional hemoglobin or siderophore transporters were harbored in the examined chromosomes, but they are not a share of the exbB, exbD, tonB array. In the Figure 9A image, co-transcription of the operon is hypothesized on the basis of the gene’s organization and the finding of a predicted promoter and a RpoD binding site; reinforcing the idea regarding the identification of a putative Fur-box motif that could contribute to the iron-dependent regulation of the Fur protein and the operon, which could induce the TonB-dependent protein of 65 kDa in response to iron depletion. In contrast, the 70-kDa protein seems to be a membrane protein, whose expression is not induced by dipyridyl (Figure 2A, lane 4). At first sight, a postranscriptional mechanism regulates its disruption to supply the 65 kDa protein, which is recognized by the same antibodies as the 70-kDa isoform (Figure 2C). Possibly the 70-kDa protein is a precursor of the 65- kDa protein, its production could be a consequence of the protein processing favored by Fur de-repression, or from mechanical disruption, rather than a change in the use of the transcription start site (Duval et al., 2017, Grove, 2022). Mass spectrometry analysis showed that amino-terminal amino acids were maintained in both the 65-kDa and 70-kDa proteins, demonstrating the presence of both isoforms of the protein.
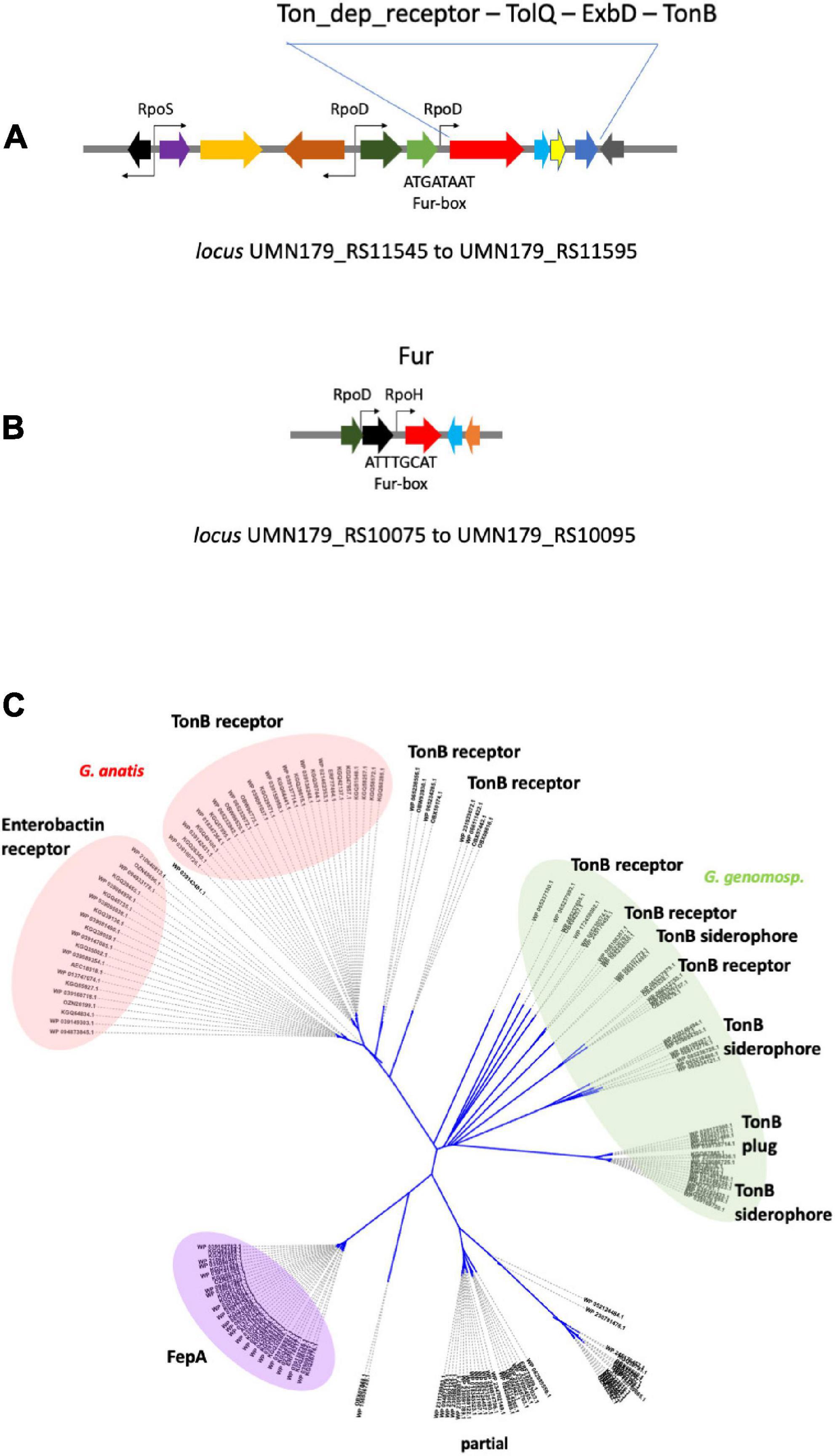
Figure 9. TonB-dependent receptors in Gallibacterium sp. (A) Map of the locus harboring the TonB-dependent receptor gene (FepAG) from G. anatis UMN179 chromosome. Gene encoding FepAG (boxed red arrow) is homologous to the enterobactin receptor gene (erf77464.1) and seems to be part of a four- members operon. The promoter nearest to fepAG was predicted as a RpoD-dependent promoter, indicated as RpoD above the tinny black arrow; a Fur-box with its consensus sequence was in the same intergenic region as indicated below the gray line. (B) Map of the locus encompassing the fur gene (red boxed arrow) in G. anatis UMN179 chromosome. The two promoters predicted to be close to fur are indicated as tinny black arrows in the drawing; one would depend on RpoH and the other on RpoD. One or both could control the gene expression of fur; below the proximal promoter, a Fur-box with its consensus sequence is indicated in the drawing. (C) Phylogeny of TonB-dependent receptors in the genus Gallibacterium sp. A total of 178 amino acid sequences were recovered from 41 sequenced genomes (GeneBank, Supplementary Information), of these: G. anatis (31 strains), G. genomospecies (7 strains), and G. salpindigitis (3 strains). An unrooted tree was built with the data; the phylogram shows five clades of proteins phylogenetically related to TonB-dependent receptor proteins from G. anatis. The first clade containing the protein from G. anatis UMN179 was termed enterobactin receptor (left, pink) because of the homology of proteins with the transferrin receptor sequence from Shigella boidii (accession EFX6075140.1). Another protein group (right, pink) was termed TonB receptor originating from G. anatis 12656-12. The disperse group, highlighted in green, encloses the TonB-dependent receptor and the TonB-dependent receptor of siderophores from G. anatis, G. salpingitidis, and G. genomospecies. The fourth clade, highlighted in purple, corresponds to receptors of the FepA-Like type. Clade marked as “partial” corresponds to incomplete sequence proteins of canonical receptors with b-barrel structure; the proteins of this group could correspond to the carboxyl-terminal domain of the TonB-dependent receptors.
The Fur-box sequences were predicted further in silico by the Bprom program (Figures 9A,B), when Fur-box sequences from the TonB-dependent receptor gene and the fur gene were compared, despite that both are AT-rich they were not identical, this was previously observed when Fur- boxes in fur regulated genes also varied in sequence in other bacterial species that contain more complex Fur boxes. Affinity of fur for Fur-boxes in the TonB-dependent receptor and fur genes of G. anatis need to be further investigated.
A great variety of proteins resembling TonB-dependent receptors were grouped in the phylogenetic tree (Figure 9C) based on: (1) the substantial number of sequenced and released genomes of the Gallibacterium genus, either as draft or complete genome sequences, from which we knew many different types of TonB-dependent receptor proteins. (2) Proteins related to the TonB-dependent receptor were three per genome, one of which was a truncated gene (Figure 9C). All these proteins are considered outer membrane proteins, with conserved β-barrel-like structure, so it was difficult to infer which type of receptor/transporter they were and if they can uptake and transport different types of iron-containing molecules (Roumia et al., 2021). We do not know whether the iron needed for essential functions stimulated the same acquisition pathway in the bacterial genus nor the mechanism to avoid the excessive accumulation of Fe or Fe complexes. It seems this diversity of related proteins is maintained to contribute to coping with iron influx to prevent severe cell damage (Whitby et al., 2009; Neumann et al., 2017; Parker et al., 2022). In the phylogenetic study, we include proteins from complete CDSs or those whose domains were as long as their predicted and annotated proteins in the GenBank. The proteins grouped in the colored ovoids (Figure 9C) share sequence identities more significant than 85%, with E values greater than 5e-100, and more than 90% coverage. Differences between groups were less than 50%, with E values of 5e-50 and less than 80% coverage. Considering the values specified above for the 65- and 70-kDa proteins, the existence of a specific proteolytic process may alter the β-barrel structure and possibly the permeability of the transport channels from the TonB-dependent receptors also. This unrecorded observation of mobility change, size change, and structure change of TonB-dependent receptors needs further understanding. Likewise, it is necessary to study the possible biochemical and molecular processes that could generate TonB-dependent receptor isoforms (Duval et al., 2017; Roumia et al., 2021; Parker et al., 2022). It is tempting to think of the DegP-type proteases from periplasmic spaces or external membranes as potential candidates (Ge et al., 2014; Gómez-Santos et al., 2019).
Other important regulators could be considered to control OMP changes, for example the general stress response regulator RpoS (Wang et al., 2014), and MarR, which controls the expression of genes involved in various adaptive molecular mechanisms, such as antibiotic and antimicrobial stress resistance, oxidative stress response, heat shock resistance and virulence, leading to transcriptional repression or activation (Wilkinson and Grove, 2006). The identification of the right/specific gene regulator of the 70-kDa protein and a wider characterization of the G. anatis 126.13 fur mutant will be performed in future works.
In Xanthomonas campestris, the fur gene mutation induces a growth reduction due to a high siderophore and iron transport genes expression (Jittawuttipoka et al., 2010). The addition of FeCl3 to the culture medium induced a diminishing growth of the wild-type G. anatis, suggesting a toxic effect, probably due to a Fenton reaction. However, the same addition did not affect the growth of the Ω fur 126.13 strain indicating Fur-independent regulator mechanisms in G. anatis. Among these mechanisms could be the anti-porter systems that expel the excess of iron to the cell’s exterior as described for Streptococcus suis (Jia et al., 2020). Another mechanism could be the up- expression of different antioxidant enzymes, such as the superoxide dismutase enzyme. In Stenotrophomonas maltophilia, it has been described that MnSOD increases activity in fur mutants, in comparison with strain type, indicating that MnSOD is negatively regulated by the fur gene (García et al., 2015). To understand this behavior, more experiments are needed.
In Staphylococcus aureus and Stenotrophomonas maltophilia, biofilm formation diminishes in the presence of iron and increases in iron-restriction conditions (Johnson et al., 2005; García et al., 2015). In S. maltophilia, a higher biofilm production was described by a spontaneous fur mutant, similarly as observed herein with the G. anatis fur mutant. This higher amount in S. maltophilia biofilm production was due to an increase in exopolymeric components produced by the fur mutant that were no different in composition from those produced by the wild-type strain (García et al., 2015). In Yersinia pestis, an alternative mechanism has been described in which Fur inhibits biofilm formation by repressing hmsT and, in consequence, inhibiting c-di-GMP synthesis (Porcheron and Dozois, 2015). High levels of c-di-GMP have been related with biofilm formation, and vice versa. The mechanisms involved in these effects in G. anatis will be considered in future work.
Interruption of the fur gene in G. anatis seems to diminish its pathogenic capacity because Ω fur 126.13 was only re-isolated from heart samples, whereas the wild-type strain was isolated, albeit at a lower percentage, but from different organs (Table 1). The reason about colonization mainly of heart tissues is unknown. Relevance of Fur in bacterial infections is different depending on the bacterial species and model of infection (Pasqua et al., 2017). The ability to count with different iron mechanisms could be an advantage for a pathogen microorganism, such as G. anatis.
Data availability statement
The original contributions presented in this study are included in the article/Supplementary material, further inquiries can be directed to the corresponding author.
Ethics statement
The animal study was reviewed and approved by Científicos Veterinarios de México. Av. Luis Donaldo Colosio 984-6, Tepatitlán, Jalisco, Mexico. Written informed consent was obtained from the owners for the participation of their animals in this study.
Author contributions
AC-G, SM-P, NR-R, IR-H, FM-G, HT-R, and IY-A performed the experiments. AC-G contributed to the microbiology, physiology, and genetics. SM-P contributed to the genetics. NR-R and IY-A contributed to the microbiology and physiology. IR-H contributed to the immunology. FM-G and HT-R contributed to pathogenicity. CV-C, PS-A, and EN-A made revision of results and data analysis and provided the funding. CV-C and EN-A wrote the manuscript. All authors critically reviewed, edited, and approved this work.
Funding
This work was supported by projects DGAPA-UNAM PAPIIT IN219919, BUAP-VIEP2022-00265, IN204122, CONACYT CB2015-01-259209 and Agro-biotechnological importance microbial models web (SEP-2016, Mexico).
Acknowledgments
We would like to thank J. López-Ochoa for her technical assistance.
Conflict of interest
The authors declare that the research was conducted in the absence of any commercial or financial relationships that could be construed as a potential conflict of interest.
Publisher’s note
All claims expressed in this article are solely those of the authors and do not necessarily represent those of their affiliated organizations, or those of the publisher, the editors and the reviewers. Any product that may be evaluated in this article, or claim that may be made by its manufacturer, is not guaranteed or endorsed by the publisher.
Supplementary material
The Supplementary Material for this article can be found online at: https://www.frontiersin.org/articles/10.3389/fmicb.2022.951173/full#supplementary-material
Abbreviations
BHI, brain heart infusion; CAS, chrome azurol S; CFU, colony-forming units; Fur, ferric uptake regulator; OMP, outer membrane protein; PCR, polymerase chain reaction; RTX, repeats in toxins; SDS-PAGE, sodium dodecyl sulfate-polyacrylamide gel electrophoresis.
Footnotes
- ^ www.ncbi.nlm.nih.gov
- ^ www.ebi.a-c.uk/Tools/clustalw2/index.html
- ^ http://www.softberry.com/berry.phtml?topic=bprom&group= programs&subgroup=gfindb
- ^ https://blast.ncbi.nlm.nih.gov/Blast.cgi
- ^ https://www.ebi.ac.uk/Tools/msa/clustalo/
- ^ https://itol.embl.de/
- ^ https://www.rcsb.org/
- ^ https://swissmodel.expasy.org/
- ^ http://www.matrixscience.com/server.html
References
Abascal, E. N., Guerra, A. C., Vázquez, A. S., Tenorio, V. R., Cruz, C. V., Zenteno, E., et al. (2009). Identification of iron-acquisition proteins of Avibacterium paragallinarum. Avian. Pathol. 38, 209–213. doi: 10.1080/03079450902912143
Bager, R. J., Nesta, B., Pors, S. E., Soriani, M., Serino, L., Boyce, J. D., et al. (2013). The fimbrial protein FlfA from Gallibacterium anatis is a virulence factor and vaccine candidate. Infect. Immun. 81, 1964–1973. doi: 10.1128/IAI.00059-13
Bisgaard, M., Korczak, B. M., Busse, H.-J., Kuhnert, P., Bojesen, A. M., and Christensen, H. (2009). Classification of the taxon 2 and taxon 3 complex of Bisgaard within Gallibacterium and description of Gallibacterium melopsittaci sp. nov. Gallibacterium trehalosifermentans sp. nov. and Gallibacterium salpingitidis sp. nov. Int. J. Syst. Evol. Microbiol. 59, 735–744. doi: 10.1099/ijs.0.005694-0
Bojesen, A. M., Christensen, J. P., and Bisgaard, M. (2008). “Gallibacterium infections and other avian pasteurellaceae,” in Poultry Diseases, eds M. Pattison, P. F. Mcmullin, J. M. Bradbury, and D. J. Alexander (Edinburg: Saunders Elsevier).
Bradford, M. M. (1976). A rapid and sensitive method for the quantitation of microgram quantities of protein utilizing the principle of protein-dye binding. Anal. Biochem. 72, 248–254.
Chart, H., Smith, H. R., La Ragione, R. M., and Woodward, M. J. (2000). An investigation into the pathogenic properties of Escherichia coli strains BLR, BL21, DH5α and EQ1. J. Appl. Microbiol. 89, 1048–1058. doi: 10.1046/j.1365-2672.2000.01211.x
Christensen, H., Bisgaard, M., Bojesen, A. M., Mutters, R., and Olsen, J. E. (2003). Genetic relationships among avian isolates classified as Pasteurella haemolytica, “Actinobacillus salpingitidis” or Pasteurella anatis with proposal of Gallibacterium anatis gen. nov., comb. nov. and description of additional genomospecies within Gallibacterium gen. nov. Int. J. System. Evol. Microbiol. 53, 275–287. doi: 10.1099/ijs.0.02330-2330
Cornelissen, C. N. (2003). Transferrin-iron uptake by gram-negative bacteria. Front. Biosci. 8:d836–d847. doi: 10.2741/1076
Drabkin, D. L. (1946). Spectrophotometric studies: the crystallographic and optical properties of the hemoglobin of man in comparison with those of other species. J. Biol. Chem. 164, 703–723.
Duval, V., Foster, K., Brewster, J., and Levy, S. B. (2017). A novel regulatory cascade involving BluR, YcgZ, and Lon Controls the expression of Escherichia coli OmpF porin. Front. Microbiol. 8, 1148. doi: 10.3389/fmicb.2017.01148
García, A. V., Alcaraz, E. S., Franco, M. A., Passerini, and de Rossi, B. N. (2015). Iron is a signal for Stenotrophomonas maltophilia biofilm formation, oxidative stress response, OMPs expression, and virulence. Front. Microbiol. 6:926. doi: 10.3389/fmicb.2015.00926
García-Gómez, E., Vaca, S., Pérez-Méndez, A., Ibarra-Caballero, J., Pérez-Márquez, V., Tenorio, V. R., et al. (2005). Gallibacterium anatis-secreted metalloproteases degrade chicken IgG. Avian. Pathol. 34, 426–429. doi: 10.1080/03079450500267866
Ge, X., Wang, R., Liu, Y., Ezemaduka, A. N., Chen, P. R., Fu, X., et al. (2014). DegP primarly function as a protease for the biogenesis of β-barrel outer membrane proteins in Gram-negative bacterium Escherichia coli. FEBS J. 281, 1226–1240. doi: 10.1111/febs.12701
Gómez-Santos, N., Glatter, T., Koebnik, R., Świątek-Połatyńska, M. A., and Søgaard-Andersen, L. A. (2019). TonB-dependent transporter is required for secretion of protease PopC across the bacterial outer membrane. Nat. Commun. 10:1360. doi: 10.1038/s41467-019-09366-9369
Grove, A. (2022). Extracytoplasmic function sigma factors governing production of the primary siderophores in pathogenic burkholderia species. Front. Microbiol. 13:851011. doi: 10.3389/fmicb.2022.851011
Jia, M., Wei, M., Zhang, Y., and Zheng, C. (2020). Transcriptomic analysis of Streptococcus suis in response to ferrous iron and cobalt toxicity. Genes 11:1035. doi: 10.3390/genes11091035
Jin-Won, L., and Helmann, J. D. (2007). Functional specialization within the fur family of metalloregulators. Biometals 20, 485–499.
Jittawuttipoka, T., Sallabhan, R., Vattanaviboon, P., Fuangthong, M., and Mongkolsuk, S. (2010). Mutations of ferric uptake regulator (fur) impair iron homeostasis, growth, oxidative stress survival, and virulence of Xanthomonas campestris pv. Campestris. Arch. Microbiol. 192, 331–339. doi: 10.1007/s00203-010-0558-8
Johnson, M., Cockayne, A., Williams, P. H., and Morrissey, J. A. (2005). Iron-responsive regulation of biofilm formation in Staphylococcus aureus involves fur-dependent and fur-independent mechanisms. J. Bacteriol. 187, 8211–8215. doi: 10.1128/JB.187.23.8211-8215.2005
Kittigul, L., Suthachana, S., Kittigul, C., and Pengruangrojanachai, V. (1998). Immunoglobulin M-capture biotin-streptavidin enzyme-linked immunosorbent assay for detection of antibodies to dengue viruses. Am. J. Trop. Med. Hyg. 59, 352–356. doi: 10.4269/ajtmh.1998.59.352
Krewulak, K. D., and Vogel, H. J. (2008). Structural biology of bacterial iron uptake. Biochim. Biophys. Acta 1778, 1781–1804. doi: 10.1016/j.bbamem.2007.07.026
Kristensen, B. M., Frees, D., and Bojesen, A. M. (2010). GtxA from Gallibacterium anatis, a cytolytic RTX-toxin with a novel domain organization. Vet. Res. 41:25. doi: 10.1051/vetres/2009073
Kristensen, B. M., Sinha, S., Boyce, J. D., Bojesen, A. M., Mell, J. C., and Redfield, R. J. (2012). Natural transformation of Gallibacterium anatis. App. Environ. Microbiol. 78, 4914–4922. doi: 10.1128/AEM.00412-412
Kudirkiene, E., Bager, R. J., Johnson, T. J., and Bojesen, A. M. (2014). Chaperone-usher fimbriae in a diverse selection of Gallibacterium genomes. BMC Genomics 15:1093. doi: 10.1186/1471-2164-15-1093
López-Ochoa, A. J., Sánchez-Alonso, M. P. G., Vázquez-Cruz, C., Horta-Valerdi, G., Negrete-Abascal, E., Vaca, S., et al. (2019). Molecular and genetic characterization of the pOV plasmid from Pasteurella multocida and construction of an integration vector for Gallibacterium anatis. Plasmid 103, 45–52. doi: 10.1016/j.plasmid.2019.04.003
López-Ochoa, J., Montes-García, J. F., Vázquez, C., Sánchez-Alonso, P., Pérez-Márquez, V. M., Blackall, P. J., et al. (2017). Gallibacterium elongation factor-Tu possesses amyloid-like protein characteristics, participates in cell adhesion, and is present in biofilms. J. Microbiol. 55, 745–752. doi: 10.1007/s12275-017-7077-7070
Lyles, K. V., and Eichenbaum, Z. (2018). From host heme to iron: the expanding spectrum of heme degrading enzymes used by pathogenic bacteria. Front. Cell. Infect. Microbiol. 8:198. doi: 10.3389/fcimb.2018.00198
Montes-García, J. F., Vaca, S., Vázquez, C., Soriano-Vargas, E., Aguilar-Romero, F., Blackall, P. J., et al. (2016). Identification of a hemagglutinin from Galllibacterium anatis. Curr. Microbiol. 72, 450–456. doi: 10.1007/s00284-015-0969-965
Morton, D. J., Turman, E. J., Hensley, P. D., VanWagoner, T. M., Seale, T. W., Whitby, P. W., et al. (2010). Identification of a siderophore utilization locus in nontypeable Haemophilus influenzae. BMC Microbiol. 10:113. doi: 10.1186/1471-2180-10-113
Neilands, J. B. (1981). Microbial iron compounds. Ann. Rev. Biochem. 50, 715–731. doi: 10.1146/annurev.bi.50.070181.003435
Neubauer, C., De Souza-Pilz, M., Bojesen, A. M., Bisgaard, M., and Hess, M. (2009). Tissue distribution of haemolytic Gallibacterium anatis isolates in laying birds with reproductive disorders. Avian. Pathol. 38, 1–7. doi: 10.1080/03079450802577848
Neumann, W., Hadley, R. C., and Nolan, E. M. (2017). Transition metals at the host-pathogen interface: how Neisseria exploit human metalloproteins for acquiring iron and zinc. Essays Biochem. 61, 211–223. doi: 10.1042/EBC20160084
Otero-Asman, J. R., García-García, A. I., Civantos, C., Quesada, J. M., and Llamas, M. A. (2019). Pseudomonas aeruginosa possesses three distinct systems for sensing and using the host molecule haem. Environ. Microbiol. 21, 4629–4647. doi: 10.1111/1462-2920.14773
Parker, A. C., Seals, N. L., Baccanale, C. L., and Rocha, E. R. (2022). Analysis of six tonB gene homologs in Bacteroides fragilis revealed that tonB3 is essential for survival in experimental intestinal colonization and intra-abdominal infection. Infect. Immun. 90:e0046921. doi: 10.1128/IAI.00469-421
Pasqua, M., Visaggio, D., Lo Sciuto, A., Genah, S., Banin, E., Visca, P., et al. (2017). Ferric uptake regulator Fur is conditionally essential in Pseudomonas aeruginosa. J. Bacteriol. 199:e00472-17. doi: 10.1128/JB.00472-417
Persson, G., and Bojesen, A. M. (2015). Bacterial determinants of importance in the virulence of Gallibacterium anatis in poultry. Vet. Res. 46:57. doi: 10.1186/s13567-015-0206-z
Porcheron, G., and Dozois, C. M. (2015). Interplay between iron homeostasis and virulence: fur and RyhB as major regulators of bacterial pathogenicity. Vet. Microbiol. 179, 2–14. doi: 10.1016/j.vetmic.2015.03.024
Roumia, A. F., Tsirigos, K. D., Theodoropoulou, M. C., Tamposis, I. A., Hamodrakas, S. J., and Bagos, P. G. (2021). OMPdb: a global hub of beta-barrel outer membrane proteins. Front. Bioinform. 1:646581. doi: 10.3389/fbinf.2021.646581
Runyen-Janecky, L. J. (2013). Role and regulation of heme iron acquisition in gram-negative pathogens. Front. Cell. Infect. Microbiol. 3:55. doi: 10.3389/fcimb.2013.00055
Salgado-Lucio, M. L., Vaca, S., Vázquez, C., Zenteno, E., Pérez-Márquez, V. M., Rea, I., et al. (2012). Adhesion of Gallibacterium anatis to chicken oral-pharyngeal epithelial cells and identification of putative fimbriae. Adv. Microbiol. 2, 505–510. doi: 10.4236/aim.2012.24064
Sambrook, J., Fritsch, E. F., and Maniatis, F. (1989). Molecular Cloning. a Laboratory Manual. 2nd Edn. Cold Spring Harbor Laboratory: Bungtown Rd
Tabatabai, L. B., Zehr, E. S., Zimmerli, M. K., and Nagaraja, K. V. (2008). Iron acquisition by Ornithobacterium rhinotracheale. Avian. Dis. 52, 419–425. doi: 10.1637/8185-113007-Reg
Trujillo-Ruíz, H. H., Shivaprasad, H. L., Morales-Erasto, V., Talavera-Rojas, M., Salgado-Miranda, C., Salazar-García, F., et al. (2016). Virulence of serovar C-1 strains of Avibacterium paragallinarum. Avian. Dis. 60, 837–840.
Vaca, S., Monroy, E., Rojas, L., Vazquez, C., Sanchez, P., Soriano-Vargas, E., et al. (2011). Adherence of Gallibacterium anatis to inert surfaces. J. Anim. Vet. Adv. 10, 1688–1693. doi: 10.3923/javaa.2011.1688.1693
Vázquez-Cruz, C., and Olmedo-Álvarez, G. (1997). Mechanism of decay of the cry1Aa mRNA in Bacillus subtilis. J. Bacteriol. 179, 6341–6348. doi: 10.1128/jb.179.20.6341-6348.1997
Wang, H., Ayala, J. C., Benitez, J. A., and Silva, A. J. (2014). The LuxR-type regulator VpsT negatively controls the transcription of rpoS, encoding the general stress response regulator, in Vibrio cholerae biofilms. J. Bacteriol. 196, 1020–1030. doi: 10.1128/JB.00993-13
Whitby, P. W., Seale, T. W., VanWagoner, T. M., Morton, D. J., and Stull, T. L. (2009). The iron/heme regulated genes of Haemophilus influenzae: comparative transcriptional profiling as a tool to define the species core modulon. BMC Genomics 10:6. doi: 10.1186/1471-2164-10-16
Wilkinson, S. P., and Grove, A. (2006). Ligand-responsive transcriptional regulation by members of the MarR family of winged helix proteins. Curr. Issues Mol. Biol. 8, 51–62.
Zepeda, A., Ramirez, S., Vega, V., Morales, V., Talavera, M., Salgado-Miranda, C., et al. (2009). Hemagglutinating activity of Gallibacterium strains. Avian Dis. 53, 115–118. doi: 10.1637/8375-060908-ResNote.1
Keywords: Gallibacterium, Fur, iron restriction, G. anatis fur-mutant, biofilm, virulence
Citation: Chantes-Guerra A, Maldonado-Puga S, Rojas-Ruiz N, Rea-Hernandez I, Montes-Garcia FJ, Trujillo-Ruiz H, Yañez-Aguilar IE, Vazquez-Cruz C, Sanchez-Alonso P and Negrete-Abascal E (2022) A putative siderophore receptor of Gallibacterium anatis 12656-12 under Fur control also binds hemoglobin. Front. Microbiol. 13:951173. doi: 10.3389/fmicb.2022.951173
Received: 23 May 2022; Accepted: 19 July 2022;
Published: 16 August 2022.
Edited by:
Juana Pérez, Universidad de Granada, SpainReviewed by:
Francisco Javier Marcos-Torres, University of Granada, SpainJosé Manuel Molina Guijarro, University of Alcalá, Spain
Copyright © 2022 Chantes-Guerra, Maldonado-Puga, Rojas-Ruiz, Rea-Hernandez, Montes-Garcia, Trujillo-Ruiz, Yañez-Aguilar, Vazquez-Cruz, Sanchez-Alonso and Negrete-Abascal. This is an open-access article distributed under the terms of the Creative Commons Attribution License (CC BY). The use, distribution or reproduction in other forums is permitted, provided the original author(s) and the copyright owner(s) are credited and that the original publication in this journal is cited, in accordance with accepted academic practice. No use, distribution or reproduction is permitted which does not comply with these terms.
*Correspondence: Candelario Vazquez-Cruz, ZWNvYmFjaWxvc0B5YWhvby5jb20=