- 1Key Laboratory of Animal Nutrition in Jiangxi Province, Jiangxi Agricultural University, Nanchang, China
- 2ZheJiang Cofine Biotechnology Company Limited, Haining, China
- 3Yangxin Yiliyuan Halal Meat Co., Ltd., Binzhou, China
This study was conducted to investigate the effects of yeast culture supplements on the physiological state and growth performance of growing bulls under heat stress conditions and the underlying mechanism. A total of 14 (6.0 ± 1.0 months old) growing bulls with similar body weight were randomly assigned into the control group (YC0g/d) and yeast culture supplement group (YC40g/d). YC0g/d contained three replicates, with two bulls in each replicate, which were fed a basal diet. Meanwhile, the YC40g/d treatment contained four replicates, with two bulls in each replicate, which were fed a basal diet supplemented with 40 g/day of yeast culture per cattle. Growth performance, nutrient digestibility, rumen fermentable metabolites, serum immunity, serum hormones, and serum antioxidant parameters were measured. Results showed that the average daily gain significantly increased (P < 0.05), while the feed-to-gain ratio significantly decreased (P < 0.01) after YC supplementation compared with the YC0g/d. The digestibility of neutral detergent fiber (P < 0.05) was higher in YC40g/d. There were no significant differences in ruminal pH, NH3-N, butyrate, or acetate/propionate (P > 0.05). Besides, the rumen MCP, acetate, propionate, and total VFA content remarkably increased with the supplement of YC (P < 0.05). Yeast culture supplementation increased the concentration of nicotinamide riboside, neuromedin B, peptides, and formyl-5-hydroxykynurenamine. The YC40g/d group had a significantly (P < 0.05) higher serum triiodothyronine level, serum glutathione peroxidase levels, and total antioxidant capacity while having a lower serum malondialdehyde level than the YC0g/d group. In conclusion, the addition of yeast culture in the diet improves the growth performance of growing bulls under heat stress by increasing nutrient digestibility, rumen fermentation function, antioxidant capacity, and rumen metabolites.
Introduction
Heat stress is one of the most stressful events for livestock, which might trigger detrimental consequences for animal health, productivity, and product quality (Gonzalez-Rivas et al., 2020). In terms of ruminants, heat generation was mainly attributed to the high temperature and high humidity combined with the feed fermentation in the rumen, which contributed to the increase in core temperature. Furthermore, long exposure time in the high temperature and humidity environment endangered the physiological ability to regulate temperature, which would be accompanied by a series of changes in physiological functions (Kadzere et al., 2002). Heat stress increases respiration and mortality, reduces fertility, modifies animal behavior, and suppresses the immune and endocrine systems (Thornton et al., 2022). Also, heat stress reduces total volatile fatty acid (VFA) production and leads to changes in rumen pH, and the passage rate and retention time of chyme are also affected by elevated ambient temperature, thereby affecting digestibility (Yadav et al., 2013). Although most relative studies focused on lactating cows, finishing cattle, or calves, correlated studies on growth periods had been rarely reported.
The growth period is critical for the production and economic performance of beef cattle, which requires not only the assurance of adequate necessary nutrients but also the improvement of the immunity and antioxidant function for healthy growth (Galyean et al., 1999). In Euramerican countries, this stage of lifecycle was received continuously grazing, supplemental feed, minerals, and vitamins to guarantee nutritional needs are met for optimal growth, which means less stress and better animal welfare. Nevertheless, in the southern provinces of China, the lack of natural pasture determines that intensive farming systems have to be selected for beef cattle production and may thereafter induce more challenges of stress, especially in summer with high temperatures and high humidity. Therefore, nutrition-dependent regulations were necessary to decrease the negative implication.
Yeast culture (YC) is a product obtained by active yeast through the liquid and solid fermentation, concentrated and dried substances (containing small peptides, amino acids, vitamins, various enzymes, and nucleic acids), extracellular metabolites such as malic acid, and the various nutrients contained in the culture medium after fermentation, the composition of which is mainly determined by the fermentation strain, the medium ratio, and fermentation process conditions. A considerable amount of research has indicated that yeast culture can increase fiber digestibility by optimizing rumen fermentation, relieving stress, improve production performance and animal welfare (Ghazanfar et al., 2019; Perdomo et al., 2020). In Shen’s study, feeding YC performed better or at least equal to antibiotics currently used in beef cattle rations and could be a natural alternative for beef cattle production (Shen et al., 2018).
Metabolomics has provided a useful method to characterize the metabolism of rumen fluid in dairy cows and beef cattle, which can be used to understand the underlying physiological mechanisms that contribute to differences in feed efficiency (Ametaj et al., 2010; Saleem et al., 2012; Artegoitia et al., 2017; Ogunade I. et al., 2019; Clemmons et al., 2020). Although untargeted metabolomics can provide rich information, no research has applied these techniques to analyze the impact of rumen fluid metabolome by YC yet. Therefore, in this study, the objectives were to evaluate the effects of supplemental dietary YC on growth performance and nutrient digestibility in heat-stressed growing bulls. We hypothesized that dietary YC would improve blood parameters, rumen fermentation characteristics, and rumen fluid metabolomics.
Materials and methods
Ethical statement
This trial was conducted following the Chinese guidelines for animal welfare and approved by the Committee for the Care and Use of Experimental Animals at Jiangxi Agricultural University (Ethics Approval Code: JXAULL-2021004).
Animal treatments and experimental design
This research was conducted on the beef cattle research and teaching farm, specifically Yufeng farming in Jiangxi province, China (28.25°N, 115.22°E). The highest temperature in summer in the region often exceeds 38°C.
A total of 14 Jinjiang bulls (6 ± 1 months old) with initial body weight (IBW) of 107.5 ± 10.6 kg were randomly allocated into two experimental groups for a 7-day adjustment period followed by a 62-day-long experimental period (69 feeding days in total) feeding process. The YC0g/d and YC40g/d contained six and eight bulls, respectively. All the bulls were fed in seven pens, with two bulls per pen. The two experimental groups received a YC supplement at 0 or 40 g/day, respectively. YC was acquired from Zhejiang Cofine Biotech. Co., Ltd. (Haining, Zhejiang, China). The fermentation strain is Saccharomyces cerevisiae. All bulls were regularly provided rations twice a day at 09:00 and 16:00. Bulls had free access to water during the experiment.
The temperature and humidity were recorded by a Temperature & Humidity Recorder (Renke COS-04, Renke Control Technology Co., Shangdong, China) at 9:00 and 16:00 h each day, and the temperature and humidity index (THI) was calculated using the following formula:
where T is temperature (°C) and RH is relative humidity percentage (%) (Domínguez-Mancera et al., 2017).
As shown in Figure 1, the average daily THI values during the experimental period were higher than 74 for the whole trial. THI values were interpreted as follows: ≤ 74, comfort; 75–78, alert; 79–83, dangerous; and ≥84, emergency (Domínguez-Mancera et al., 2017). The growth bulls were suffering from heat stress throughout the whole trial period.
The basal diet (Table 1) was designed to support the nutrient needs of protein, energy, vitamins, and minerals, which is consistent with the feeding standards of beef cattle in China, NY/T 815-2004 (Ministry of Agriculture of People’s Republic of China 2004). The ratio of concentrate:roughage was 40:60 DM (dry matter). The concentrate consisted of corn, soybean meal, NaHCO3, and premix. The roughage included straw and Baijiu fermented grains.
Dry matter intake (DMI) was recorded daily for each pen, and the BW of each bull was measured at the beginning and end of the trail, with an empty stomach in the morning. The recorded date was used to calculate the average daily gain (ADG).
Determination of apparent nutrient digestibility
Representative feed and fecal samples were collected during the last 3 days in two stages (days 28–30 and 60–62 of the experimental period) after the morning feeding, and results are displayed as the average of the total 3 days. Fecal samples were collected by the rectal sampling method. All feces were evenly mixed, and 10% H2SO4 was added to fix nitrogen, then stored at −20°C. Feed and fecal samples were dried in a forced air oven at 65°C for 72 h and then ground by the Wiley mill through a 1-mm screen sieve.
Dry matter (DM), crude protein (CP), crude fat (CF), ash, and ether extract (EE) of the feed and fecal samples were determined according to the Association of Analytical Communities (AOAC) using the methods 967.03, 984.13, 920.39, 924.05, and 920.39, respectively. Neutral detergent fiber (NDF) and acid detergent fiber (ADF) were determined using an Ankom A200i Fiber analyzer (ANKOM Technology Co., New York, NY, United States) according to Van Soest (Van Keulen and Young, 1977). The content of hydrochloric acid insoluble ash (AIA) was determined according to Block (Block et al., 1981), and AIA was used as a digestibility marker. The formula was as follows:
AD represents the apparent digestibility of nutrient content, a represents the nutrient content of the feed, b represents the nutrient content of feces, c represents the AIA content of the feed, and d represents the AIA content of feces.
Determination of rumen fermentation characteristics
Rumen fluid samples of 12 bulls (six bulls in each group, similar body weight) were collected on the last day through esophageal tubing in the morning before feeding. The first 150 ml of rumen fluid sample was discarded to minimize saliva contamination. The rumen fluid pH was measured immediately using a portable pH meter [Testo 206-pH1, Testo Instruments International (Shanghai) CO., Shanghai, China]. At the same time, 5 ml of rumen fluid was preserved at −80°C for further test and other samples were processed to analyze VFA, microbial protein (MCP), and ammonia-N (NH3-N). The ruminal MCP concentration was detected with a bicinchoninic acid protein quantification kit (Yeasen Biotechnology), and the concentration of NH3-N was determined using alkaline sodium hypochlorite-phenol spectrophotometry. The concentrations of VFA were determined by a gas chromatograph (GC-2014, Shimadzu, Tokyo, Japan) equipped with a capillary column (Stabilwax, Restek, Bellefonte, PA, United States). Notably, 1.00 ml of rumen supernatant was added to 0.20 ml of 25% (w/v) metaphosphoric acid and centrifuged at 10,000 r/min for 15 min. Then, the supernatant was used for the VFA test.
Metabolomics analysis of rumen fluid
For metabolomics, 12 rumen fluids from YC0g/d and YC40g/d were used. Non-targeted profiling of metabolites in rumen fluid was obtained according to the instructions of Majorbio Bio-Pharm Technology Co., Ltd. (Shanghai, China). A fluid sample of 100 μl was accurately weighted, and the metabolites were extracted using a 400 μl of cold methanol:water (4:1, v/v) solution. The mixture was treated by the high throughput tissue crusher Wonbio-96c at 50 Hz for 6 min, then followed by vortex for 30 s and ultrasound at 40 kHz for 30 min at 5oC. The samples were placed at −20oC for 30 min to precipitate proteins. After centrifugation at 13,000 g at 4oC for 15 min, the supernatant was taken for LC-MS (Waters ACQUITY Ultra-Performance Liquid Chromatography System, Milford, United States) coupled with a triple time-of-flight mass spectrometer (AB SCIEX TripleTOF 5600 System, Framingham, United States). A preheated hyper ACQUITY BEH C18 column (100 mm × 2.1 mm, 1.7 μm; Waters, Milford, United States) was used for chromatographic separation in the positive ion mode (ESI+) and negative ion modes (ESI-). The column temperature was maintained at 40oC. The sample injection volume was 2 μl, and the flow rate was set to 0.4 ml/min. The quality control samples consisting of equivalent mixtures of all rumen fluid samples were analyzed regularly to monitor the stability of the analysis.
Blood sampling and analysis
Blood samples were collected from the jugular vein into evacuated non-anticoagulative tubes before morning feeding on days 30 and 62. The blood samples were centrifuged at 3,000 g for 10 min to obtain serum samples and then stored at −20°C until required for further analysis.
The concentrations of serum immunoglobulin A (IgA), immunoglobulin B (IgG), immunoglobulin M (IgM), growth hormone (GH), interleukin-4 (IL-4), interleukin-6 (IL-6), tumor necrosis factor-α (TNF-α), cortisol (COR), triiodothyronine (T3), and thyroxine (T4) were measured using corresponding commercial kits (Beijing Sino-UK Institute of Biotechnology Technology, Beijing, China) and a microplate reader (DR-200BS, Wuxi DR-200BS, Wuxi, China).
The concentrations of serum malondialdehyde (MDA), the activities of superoxide dismutase (SOD), glutathione peroxidase (GSH-Px), and total antioxidant capacity (T-AOC) were measured with commercial kits (Nanjing Jiancheng Bioengineering Institute) and an Epoch 17121332 enzyme marker (Bio Tek, Vermont, United States).
Data analysis
A normal distribution test on growth performances and rumen fermentation analysis was first conducted using SPSS (Statistical Product Service Solutions) 23.0 software “proc univariate data = test normal” followed by a Student t-test (two-tail test). ANOVA repeated measures were performed to analyze digestibility and serum parameters at different time points (30 or 62 days). The results are shown as the mean and standard error mean (SME). P-value < 0.05 was considered to be significant.
The metabolomics data were subjected to partial least squares discriminant analysis (PCA), orthogonal partial least squares discriminate analysis (OPLS-DA), and differential metabolite screening on Majorbio Cloud Platform,1 with a false discovery rate (FDR) < 0.05 and variable weight importance ranking (VIP) > 1 as the screening criteria.
Result
Growth and feed efficiency
As shown in Table 2, there were no significant differences in ADFI (average daily feed intake), IBW (initial body weight), and FBW (final body weight) among groups (P > 0.05). However, the ADG of bulls was higher in the YC40g/d group than in the YC0g/d group (P < 0.05). The YC40g/d group had a lower F:G value than the YC0g/d group (P < 0.01).
Nutrient digestibility
The results of nutrient digestibility are presented in Table 3. There was no significant interaction between YC addition and time on the digestibility of feed. Supplementation with YC significantly increased the digestibility of NDF (P < 0.05). Except for ADF, the time of measurement had no effect on the group differences between treatments.
Rumen fermentation
As shown in Table 4, the levels of rumen MCP, acetate, propionate, and total VFA were increased significantly (P < 0.05) in the YC40g/d group when compared with the YC0g/d group. There were no significant differences in ruminal pH, NH3-N, butyrate, or acetate/propionate (P > 0.05).
Rumen fluid metabolomics
Rumen fluid metabolites from YC0g/d and YC40g/d were analyzed by LC-MS. A total of 777 differential peaks were selected, including 387 peaks in ESI+ and 390 peaks in ESI-. The PCA showed a primary unsupervised separation between the two groups (Figure 2A). To better distinguish the differences between the groups and improve effectiveness, an OPLS-DA score plot was performed to supervise the multivariate analysis (Figure 2B). The two groups can be clearly separated.
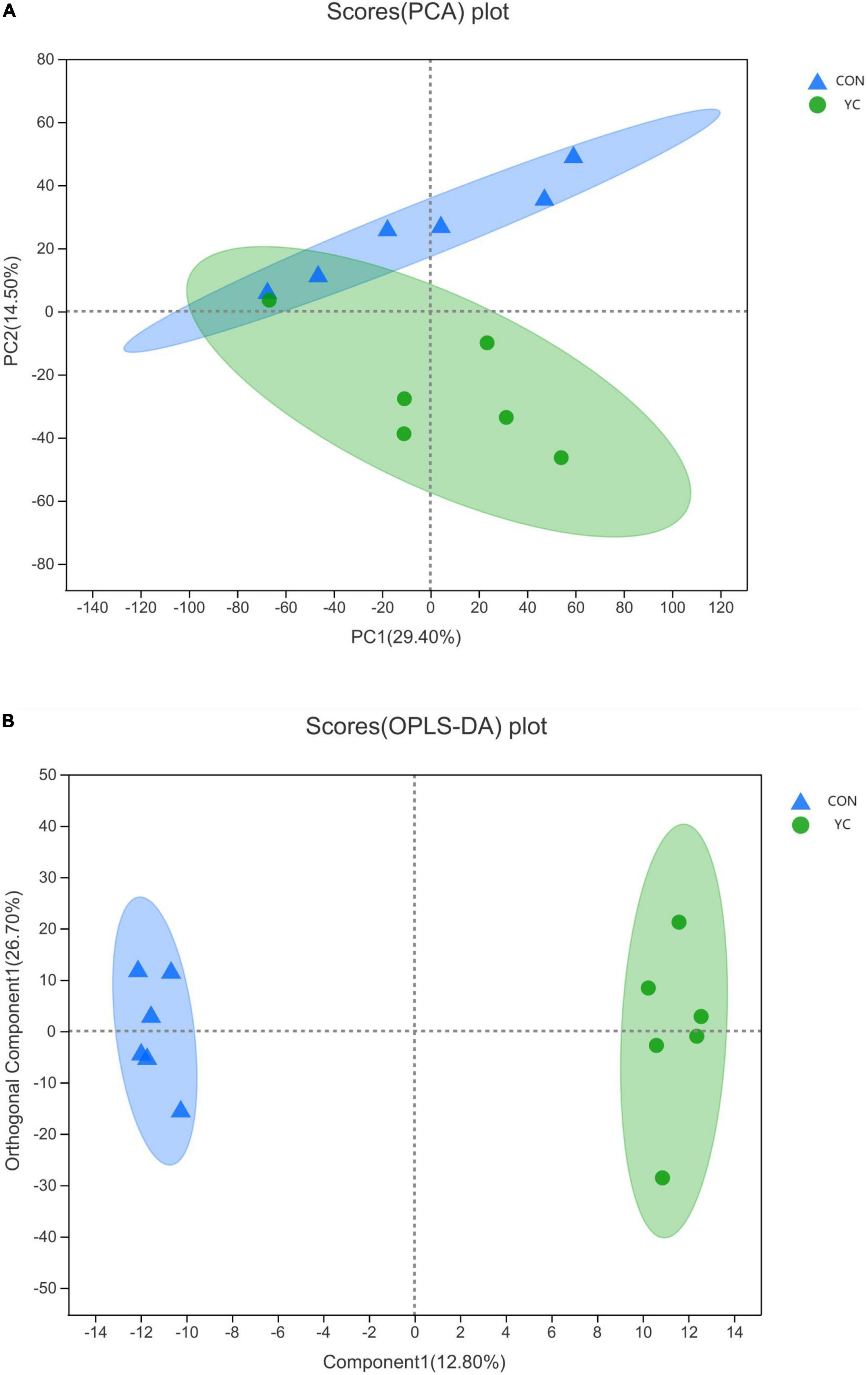
Figure 2. (A) A PCA of ruminal metabolites from bulls (n = 6) fed a control diet (control) and bulls fed 40 g/day of YC; (B) an OPLS-DA of ruminal metabolites from bulls (n = 6) fed a control diet (control) and bulls fed 40 g/day of YC.
As shown in Table 5, there were 29 differential metabolites between the YC0g/d and YC40g/d groups with a variable importance projection (VIP) value > 1.0 and P < 0.05.
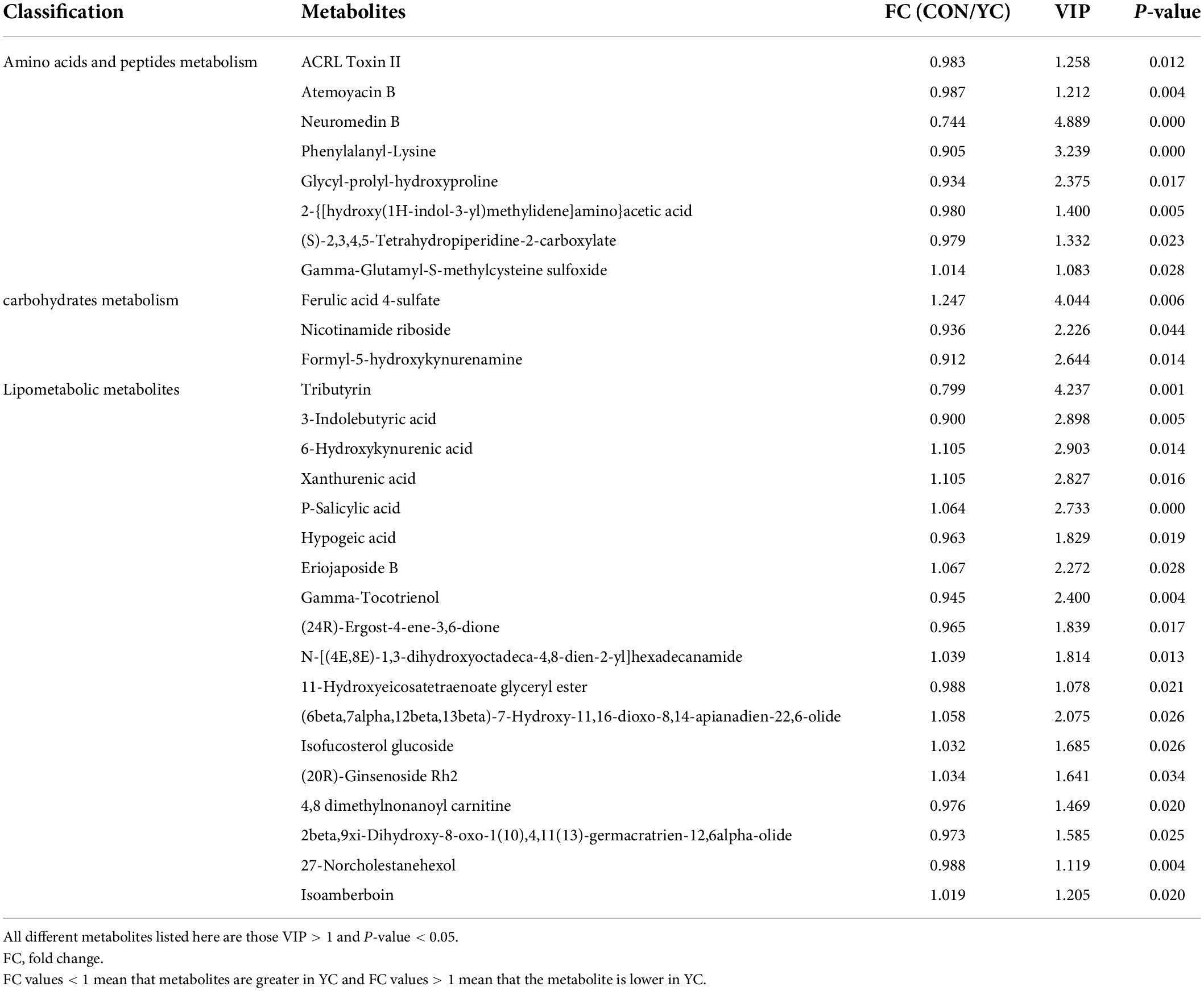
Table 5. Different metabolite contents in the rumen of bulls fed a control diet (CON) vs. bulls fed 40 g/day of YC.
The detected differential metabolites were screened, and the metabolites that could be enriched in the metabolic pathways in the KEGG database were selected for visual analysis of their metabolic pathways. Figure 3 shows the metabolic pathways enriched in the rumen. Based on the pathways of the YC40g/d group vs. control group were enriched, which included terpene lactones, cyclitols, coumaric acids, tripeptides, acetamide, quinoline carboxylic acid, C26 bile acids, alcohol, and derivatives, ceramides, fatty alcohols, vitamin E.
Blood immunological function, hormone, and antioxidant parameters
As presented in Table 6, there was no significant interaction between YC addition and time on blood immunological function, hormone, or antioxidant parameters. Supplementation with YC significantly decreased serum MDA (P < 0.05) and increased the concentration of serum T4 (P < 0.05), GSH (P < 0.01), and T-AOC (P < 0.05). Except for MDA, the time of measurement had no effect on the group differences between treatments.
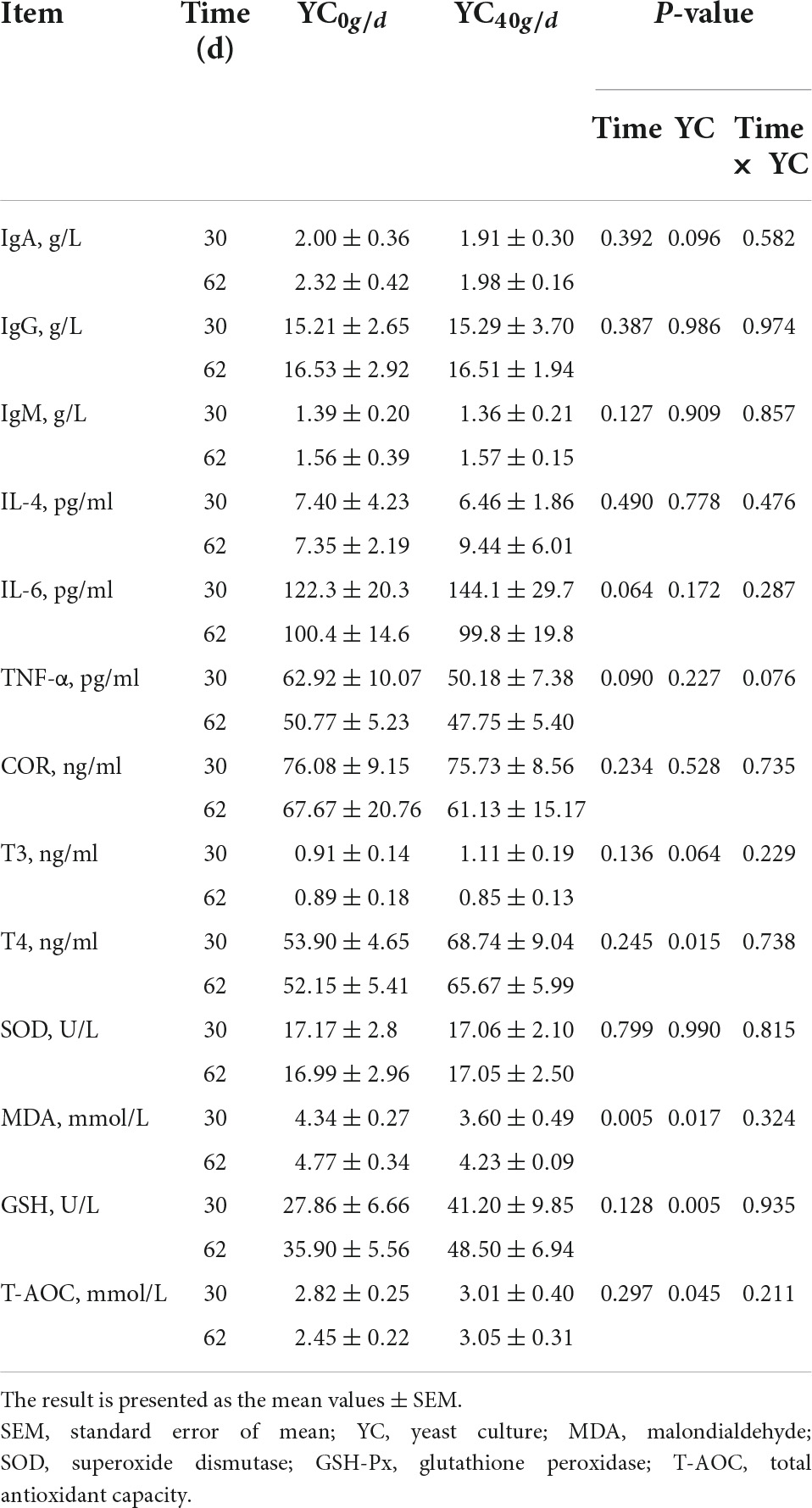
Table 6. Effect of feeding YC on the immunological function, anti-stress, and antioxidant parameters in the serum on days 30 and 62.
Discussion
This study has been widely used as a practical indicator of the heat stress degree (Habeeb et al., 2018). Livestock Weather Safety Index was developed by Livestock Conservation, Inc. and indicated that THI indexes greater than 74 were considered the occurrence of heat stress (Domínguez-Mancera et al., 2017). In this trial, the growing bulls spent the whole period of 62 days in THI > 74 conditions and a total of 40 days in THI > 79. This suggests that the growing bulls suffered from heat stress throughout the whole trial period.
Yeast culture improves the growth performance of heat-stressed growing bulls
Heat stress leads to a decrease in feed intake, digestion, and absorption of nutrients, resulting in poor growth performance of cattle (O’Brien et al., 2010; Wang et al., 2020). YC contains mass active yeast cells and compounds produced during fermentation, such as organic acid and VB, which showed positive effects on animals’ growth performance and health. In our study, there was an increasing ADG and a reducing F:G ratio in response to YC supplementation, and these findings were in line with a meta-analysis by Wagner (Wagner et al., 2016) and L. Armato’s study (Armato et al., 2016). Both of them showed that feeding beef cattle with YC enhanced the production performance, which included improved ADG, feed conversion ratio, rumen fermentation, and carcass quality.
Although feed intake was not affected by YC, increased NDF digestibility provides more nutrients to the growing bulls and further significantly improves growth performance. YC increases the level of nutrient metabolism (especially the carbohydrates) in the rumen, perhaps the most important reason for the improved production performance.
Effects of yeast culture supplementation on carbohydrate metabolism
The metabolic processes involving carbohydrates, fat, protein, and vitamins can help explain the difference in feed efficiency. However, the role of most of the differential rumen metabolites detected in this trial has not been described, which was similar to Ogunade’s study on live yeast (Ogunade I. M. et al., 2019).
Rumen pH was not influenced in this study, possibly because of the non-acidotic diet fed, which concurred with Gattass’ study (Gattass et al., 2008). Ruminal pH directly reflects the health of rumen fermentation function. The study found adding yeast increased the activity of lactate-utilizing bacteria and reduced the proliferation of lactate-producing bacteria, thus preventing lactic acid accumulation and increasing rumen pH (Gattass et al., 2008).
In this trial, NDF digestibility and VFA levels were significantly improved. The ability of yeast to stimulate the growth of fiber-digesting bacteria has been confirmed. Evidence suggests that the yeast can provide nutrients that stimulate the growth of cellulolytic bacteria such as Ruminococcus albus, Fibrobacter succinogenes, and Ruminococcus flavefaciens (Chaucheyras-Durand et al., 2008). Changes in the rumen microbiota, especially the abundance of fiber-digesting bacteria, lead to changes in the type and proportion of individual VFA produced in the rumen. The contents of acetate, propionate, and total VFA were significantly increased in this experiment. As the main source of glucose supplement for ruminants and a major substrate for glucose genesis, propionate will be rapidly absorbed by the rumen papilla and used for energy (Dijkstra et al., 2012). Butyrate is a direct energy donor for rumen epithelial cells and rumen papilla development (Laarman et al., 2012). Some experiments (Thrune et al., 2009; Zhu et al., 2017) found that the concentration and ratio of butyrate in the rumen increased after adding YC.
Nicotinamide riboside (NR) is a precursor of nicotinamide adenine dinucleotide (NAD) and a major NAD+ precursor vitamin in cow milk (Trammell et al., 2016), and represents a source of niacin (vitamin B3). Niacin is particularly important for ruminants, participating in the metabolism of carbohydrates, proteins, and fats, and can improve the synthesis capacity of rumen microbial protein and increase the content of rumen propionic acid (Trammell et al., 2016). Meanwhile, niacin synthesis in anaerobic microbial systems uses 3- and 4-carbon precursors such as propionate, glycerol, malic acid, succinic acid, or fumaric acid. In this trial, the YC40g/d group had increased propionate levels, which may explain the changes in VFA. Studies have found that supplementation of yeast cultures in heat-stressed dairy cows could increase plasma nicotinic acid concentrations (Salvati et al., 2015; Dias et al., 2018). Therefore, it is reasonable to suspect that yeast can increase the production of niacin and its related products in the rumen.
Effects of yeast culture supplementation on nitrogen metabolism
Supplementation with 40 g/day YC in the diet significantly increased the nitrogen-metabolic related contents of neuromedin B, phenylalanyl-lysine, and glycyl-prolyl-hydroxyproline. Neuromedin B regulates exocrine and endocrine secretion, blood glucose, body temperature, energy homeostasis, and cell growth (Salvati et al., 2015; Dias et al., 2018). However, its effects on rumen function in ruminants have not been reported. Phenylalanyl-lysine and glycyl-prolyl-hydroxyproline belong to the dipeptide and tripeptide, respectively. Peptides are potential nutrients for the growth of ruminal microorganisms but are also liable to be degraded to ammonia and lost from the rumen. The growth of mixed rumen bacteria is primarily driven by carbohydrate fermentation but is greatly stimulated by peptides that act as multipliers for microbial growth (Argyle and Baldwin, 1989), and this may help to explain the elevated MCP levels in rumen.
Formyl-5-hydroxykynurenamine is a metabolite of 5-hydroxytryptamine (5-HT). 5-HT is produced by tryptophan through the two-stage enzymatic reaction of tryptophan hydroxylase and aromatic amino acid decarboxylase and is involved in the tryptophan metabolism pathway (Bai et al., 2017). The supplement of YC could improve the metabolic utilization of 5-HT and the latter is involved in the hypothalamic-pituitary-adrenal axis (HPA) to help regulate stress (Dinan, 1996). This might help enhance the body’s stress-resistant capacity, improve body health, and further promote gastrointestinal digestibility.
Yeast culture improves serum antioxidant capacity
We found the addition of yeast culture in the diet can effectively improve the serum antioxidant indicators of growing bulls, which was consistent with the result of the previous study (Broadway et al., 2015). Under the condition of heat stress, the metabolic process of the animal body is disordered, the production of oxygen free radicals increases, and the antioxidant activity decreases so that the body cannot effectively use antioxidant enzymes to remove free radicals in a timely manner, resulting in an accumulation of free radicals and lipid peroxidation in the body reaction (Belhadj et al., 2016). The body needs to consume a large amount of antioxidant enzymes to scavenge excessive free radicals, resulting in a decrease in the activity of superoxidase dismutase, glutathione peroxidase, and total antioxidant capacity and an increase in the production of malondialdehyde (Sordillo and Aitken, 2009). Pancini (Pancini et al., 2020) considered that the yeast products would be of greater benefit to feedlot cattle experiencing stressful conditions. In this trial, coumaric acid (CA) is a phenolic acid of the hydroxycinnamic acid family, and it has many biological functions such as antioxidant, anti-inflammatory, antidiabetic, anti-ulcer, antiplatelet, and anticancer activities. Gamma-tocotrienol as a vitamin E isoform, a natural dietary antioxidant (Pathak et al., 2016), was significantly increased after YC supplementation compared with control. This may help to increase the body’s antioxidant capacity. In this study, time treatment significantly increased the serum MDA, which means that with the prolongation of heat stress, the body’s peroxidation response is strengthened.
Yeast cell walls consist of β-glucans, and mannan oligosaccharides (MOS) appear to possess the ability to bind the receptors of defense cells of the gut, which activates animal immune defenses such as phagocytosis and decreases morbidity (Broadway et al., 2015). Burdick found that supplementation of yeast products may improve energy availability during an immune challenge (Burdick et al., 2020). However, in this trial, the addition of yeast culture under a heat stress environment did not affect the immune function of the body, which was similar to a previous study (Ma et al., 2015).
As the major thyroid hormones, T3 and T4 are key regulators of energy metabolism in the body. To better adapt to the hot environment, the thyroid activity in animals will be relatively reduced, thereby reducing the release of thyroid hormones (Pereira et al., 2008; Sejian et al., 2010). In this study, compared with the control group, the content of serum T4 increased with the diet supplemented with YC; however, T3 and COR showed no difference. This may be related to the physical differences between different animals.
Conclusion
In conclusion, dietary supplementation with 40 g/day YC improved feed efficiency and increased antioxidant status and ruminal VFA that enhanced the production performance of growing bulls under heat stress. After adding YC, related metabolites such as NR and small peptides in the rumen increased. This partly explains the variation in rumen fermentation parameters. This study enhances our understanding of the effects of YC in the rumen, and more research is needed in the future.
Data availability statement
The original contributions presented in this study are included in the article/Supplementary material, further inquiries can be directed to the corresponding authors.
Ethics statement
The animal study was reviewed and approved by the Committee for the Care and Use of Experimental Animals at Jiangxi Agricultural University. Written informed consent was obtained from the owners for the participation of their animals in this study.
Author contributions
MQ designed the overall study. XZ, FX, HL, LX, BZ, and TZ performed the experiments. XZ and FX wrote the manuscript. All authors contributed to the article and approved the submitted version.
Funding
This study was supported by the China Agriculture Research System of MOF and MARA (CARS-37) and the Research Project of Zhejiang Province (Grant No. 2021C02007).
Acknowledgments
We thank the Nanchang Key Laboratory of Animal Health and Safety Production and Jiangxi Province Key Laboratory of Animal Nutrition/Engineering Research Center for Feed Development.
Conflict of interest
TZ was employed by ZheJiang Cofine Biotechnology Company Limited. FX was employed by Yangxin Yiliyuan Halal Meat Co., Ltd.
The remaining authors declare that the research was conducted in the absence of any commercial or financial relationships that could be construed as a potential conflict of interest.
Publisher’s note
All claims expressed in this article are solely those of the authors and do not necessarily represent those of their affiliated organizations, or those of the publisher, the editors and the reviewers. Any product that may be evaluated in this article, or claim that may be made by its manufacturer, is not guaranteed or endorsed by the publisher.
Supplementary material
The Supplementary Material for this article can be found online at: https://www.frontiersin.org/articles/10.3389/fmicb.2022.947822/full#supplementary-material
Footnotes
References
Ametaj, B. N., Zebeli, Q., Saleem, F., Psychogios, N., and Lewis, M. J. (2010). Metabolomics reveals unhealthy alterations in rumen metabolism with increased proportion of cereal grain in the diet of dairy cows. Metabolomics 6, 583–594. doi: 10.1007/s11306-010-0227-6
Argyle, J. L., and Baldwin, R. L. (1989). Effects of amino acids and peptides on rumen microbial growth yields. J. Dairy Sci. 72, 2017–2027. doi: 10.3168/jds.S0022-0302(89)79325-5
Armato, L., Gianesella, M., Morgante, M., Fiore, E., Ruzzo, M., Giudice, E., et al. (2016). Rumen volatile fatty acids × dietary supplementation with live yeast and yeast cell wall in feedlot beef cattle. Acta Agriculturae Scand. Sec. Animal Sci. 66, 119–124. doi: 10.1080/09064702.2016.1272628
Artegoitia, V. M., Foote, A. P., Lewis, R. M., and Freetly, H. C. (2017). Rumen fluid metabolomics analysis associated with feed efficiency on crossbred steers. Sci. Rep. 7:2864. doi: 10.1038/s41598-017-02856-0
Bai, M., Liu, H., Xu, K., Oso, A. O., Wu, X., Liu, G., et al. (2017). A review of the immunomodulatory role of dietary tryptophan in livestock and poultry. Amino Acids 49, 67–74. doi: 10.1007/s00726-016-2351-8
Belhadj, S. I., Najar, T., Ghram, A., and Abdrrabba, M. (2016). Heat stress effects on livestock: molecular, cellular and metabolic aspects, a review. J. Animal Physiol. Animal Nutrition 100, 401–412. doi: 10.1111/jpn.12379
Block, E., Kilmer, L. H., and Muller, L. D. (1981). Acid insoluble ash as a marker of digestibility for sheep fed corn plants or hay and for lactating dairy cattle fed hay. J. Anim. Sci. 52, 1164–1169. doi: 10.2527/jas1981.5251164x
Broadway, P. R., Carroll, J. A., and Sanchez, N. C. (2015). Live yeast and yeast cell wall supplements enhance immune function and performance in food-producing livestock: a review. Microorganisms 3, 417–427. doi: 10.3390/microorganisms3030417
Burdick, S. N., Carroll, J. A., Broadway, P. R., Edrington, T. S., Yoon, I., and Belknap, G. R. (2020). Some aspects of the acute phase immune response to a lipopolysaccharide (LPS) challenge are mitigated by supplementation with a Saccharomyces cerevisiae fermentation product in weaned beef calves. Trans. Animal Sci. 4:a156. doi: 10.1093/tas/txaa156
Chaucheyras-Durand, F., Walker, N. D., and Bach, A. (2008). Effects of active dry yeasts on the rumen microbial ecosystem: past, present and future. Animal Feed Sci. Technol. 145, 5–26. doi: 10.1016/j.anifeedsci.2007.04.019
Clemmons, B. A., Powers, J. B., Campagna, S. R., Seay, T. B., Embree, M. M., and Myer, P. R. (2020). Rumen fluid metabolomics of beef steers differing in feed efficiency. Metabolomics 16:23. doi: 10.1007/s11306-020-1643-x
Dias, J. D. L., Silva, R. B., Fernandes, T., Barbosa, E. F., Graças, L. E. C., Rafael, C., et al. (2018). Yeast culture increased plasma niacin concentration, evaporative heat loss, and feed efficiency of dairy cows in a hot environment. J. Dairy Sci. 101, 5924–5936. doi: 10.3168/jds.2017-14315
Dijkstra, J., Ellis, J. L., Kebreab, E., Strathe, A. B., López, S., France, J., et al. (2012). Ruminal pH regulation and nutritional consequences of low pH. Animal Feed Sci. Technol. 172, 22–33. doi: 10.1016/j.anifeedsci.2011.12.005
Dinan, T. G. (1996). Serotonin and the regulation of hypothalamic-pituitary-adrenal axis function. Life Sci. 58, 1683–1694. doi: 10.1016/0024-3205(96)00066-5
Domínguez-Mancera, B., Hernández-Beltrán, A., Rodríguez-Andrade, A., Cervantes-Acosta, P., Barrientos-Morales, M., and Pinos-Rodriguez, J. (2017). Changes in livestock weather security index (Temperature Humidity Index, THI) during the period 1917-2016 in Veracruz, Mexico. J. Animal Res. 7:983. doi: 10.5958/2277-940X.2017.00149.8
Galyean, M. L., Perino, L. J., and Duff, G. C. (1999). Interaction of cattle health/immunity and nutrition. J. Anim. Sci. 77:1120. doi: 10.2527/1999.7751120x
Gattass, C. B. A., Morais, M. D. G., Abreu, U. G. P. D., Franco, G. L., Stein, J., and Lempp, B. (2008). Efeito da suplementação com cultura de levedura na fermentação ruminal de bovinos de corte. Rev. Brasileira De Zootecnia 37, 711–716. doi: 10.1590/S1516-35982008000400018
Ghazanfar, S., Khalid, N., Ahmed, I., and Imran, M. (2019). “Probiotic yeast: Mode of action and its effects on ruminant nutrition,” in Yeast—industrial applications, ed. A. Morata (London: IntechOpen), 186–188.
Gonzalez-Rivas, P. A., Chauhan, S. S., Ha, M., Fegan, N., Dunshea, F. R., and Warner, R. D. (2020). Effects of heat stress on animal physiology, metabolism, and meat quality: a review. Meat Sci. 162:108025. doi: 10.1016/j.meatsci.2019.108025
Habeeb, A. A., Gad, A. E., and Atta, M. A. (2018). Temperature-Humidity indices as indicators to heat stress of climatic conditions with relation to production and reproduction of farm animals. Int. J. Biotechnol. Recent Adv. 1, 35–50. doi: 10.18689/ijbr-1000107
Kadzere, C. T., Murphy, M. R., Silanikove, N., and Maltz, E. (2002). Heat stress in lactating dairy cows: a review. Livestock Production Sci. 77, 59–91. doi: 10.1016/S0301-6226(01)00330-X
Laarman, A. H., Ruiz-Sanchez, A. L., Sugino, T., Guan, L. L., and Oba, M. (2012). Effects of feeding a calf starter on molecular adaptations in the ruminal epithelium and liver of Holstein dairy calves. J. Dairy Sci. 95, 2585–2594. doi: 10.3168/jds.2011-4788
Ma, T., Tu, Y., Zhang, N. F., Guo, J. P., Deng, K. D., Zhou, Y., et al. (2015). Effects of dietary yeast β-glucan on nutrient digestibility and serum profiles in pre-ruminant Holstein calves. J. Int. Agriculture 14, 749–757. doi: 10.1016/S2095-3119(14)60843-1
O’Brien, M. D., Rhoads, R. P., Sanders, S. R., Duff, G. C., and Baumgard, L. H. (2010). Metabolic adaptations to heat stress in growing cattle. Domest. Anim. Endocrinol. 38, 86–94. doi: 10.1016/j.domaniend.2009.08.005
Ogunade, I., Schweickart, H., McCoun, M., Cannon, K., and McManus, C. (2019). Integrating 16S rRNA sequencing and LC-MS-Based metabolomics to evaluate the effects of live yeast on rumen function in beef cattle. Animals 9:28. doi: 10.3390/ani9010028
Ogunade, I. M., Lay, J., Andries, K., McManus, C. J., and Bebe, F. (2019). Effects of live yeast on differential genetic and functional attributes of rumen microbiota in beef cattle. J. Animal Sci. Biotechnol. 10:68. doi: 10.1186/s40104-019-0378-x
Pancini, S., Cooke, R. F., Brandão, A. P., Dias, N. W., Timlin, C. L., Fontes, P. L. P., et al. (2020). Supplementing a yeast-derived product to feedlot cattle consuming monensin: impacts on performance, physiological responses, and carcass characteristics. Livestock Sci. 232:103907. doi: 10.1016/j.livsci.2019.103907
Pathak, R., Bachri, A., Ghosh, S. P., Koturbash, I., Boerma, M., Binz, R. K., et al. (2016). The vitamin e analog Gamma-Tocotrienol (GT3) suppresses Radiation-Induced cytogenetic damage. Pharm. Res. 33, 2117–2125. doi: 10.1007/s11095-016-1950-0
Perdomo, M. C., Marsola, R. S., Favoreto, M. G., Adesogan, A., Staples, C. R., Santos, J. E. P., et al. (2020). Effects of feeding live yeast at 2 dosages on performance and feeding behavior of dairy cows under heat stress. J. Dairy Sci. 103, 325–339. doi: 10.3168/jds.2019-17303
Pereira, A. M., Baccari, F. J., Titto, E. A., and Almeida, J. A. (2008). Effect of thermal stress on physiological parameters, feed intake and plasma thyroid hormones concentration in Alentejana, Mertolenga, Frisian and Limousine cattle breeds. Int. J. Biometeorol. 52, 199–208. doi: 10.1007/s00484-007-0111-x
Saleem, F., Ametaj, B. N., Bouatra, S., Mandal, R., Zebeli, Q., Dunn, S. M., et al. (2012). A metabolomics approach to uncover the effects of grain diets on rumen health in dairy cows. J. Dairy Sci. 95, 6606–6623. doi: 10.3168/jds.2012-5403
Salvati, G. G. S., Morais Júnior, N. N., Melo, A. C. S., Vilela, R. R., Cardoso, F. F., Aronovich, M., et al. (2015). Response of lactating cows to live yeast supplementation during summer. J. Dairy Sci. 98, 4062–4073. doi: 10.3168/jds.2014-9215
Sejian, V., Maurya, V. P., and Naqvi, S. M. K. (2010). Adaptive capability as indicated by endocrine and biochemical responses of Malpura ewes subjected to combined stresses (thermal and nutritional) in a semi-arid tropical environment. Int. J. Biometeorol. 54, 653–661. doi: 10.1007/s00484-010-0341-1
Shen, Y. Z., Wang, H. G., Ran, T., Yoon, I., Saleem, A. M., Yang, W. Z., et al. (2018). Influence of yeast culture and feed antibiotics on ruminal fermentation and site and extent of digestion in beef heifers fed high grain rations. J. Anim. Sci. 96, 3916–3927.
Sordillo, L. M., and Aitken, S. L. (2009). Impact of oxidative stress on the health and immune function of dairy cattle. Vet. Immunol. Immunopathol. 128, 104–109. doi: 10.1016/j.vetimm.2008.10.305
Thornton, P., Nelson, G., Mayberry, D., and Herrero, M. (2022). Impacts of heat stress on global cattle production during the 21st century: a modelling study. Lancet Planet Health 6, e192–e201. doi: 10.1016/S2542-5196(22)00002-X
Thrune, M., Bach, A., Ruiz-Moreno, M., Stern, M. D., and Linn, J. G. (2009). Effects of Saccharomyces cerevisiae on ruminal pH and microbial fermentation in dairy cows. Livestock Sci. 124, 261–265. doi: 10.1016/j.livsci.2009.02.007
Trammell, S. A., Yu, L., Redpath, P., Migaud, M. E., and Brenner, C. (2016). Nicotinamide riboside is a major NAD+ precursor vitamin in cow milk. J. Nutrition 146, 957–963. doi: 10.3945/jn.116.230078
Van Keulen, J., and Young, B. A. (1977). Evaluation of Acid-Insoluble ash as a natural marker in ruminant digestibility studies. J. Anim. Sci. 44, 282–287. doi: 10.2527/jas1977.442282x
Wagner, J. J., Engle, T. E., Belknap, C. R., and Dorton, K. L. (2016). Meta-analysis examining the effects of Saccharomyces cerevisiae fermentation products on feedlot performance and carcass. Prof. Animal Sci. 32, 172–182. doi: 10.15232/pas.2015-01438
Wang, J. J., Li, J. H., Wang, F. X., Xiao, J. X., Wang, Y. J., Yang, H. J., et al. (2020). Heat stress on calves and heifers: a review. J. Animal Sci. Biotechnol. 11, 1–79. doi: 10.1186/s40104-020-00485-8
Yadav, B., Singh, G., Verma, A. K., Dutta, N., and Sejian, V. (2013). Impact of heat stress on rumen functions. Vet. World 6, 992–996. doi: 10.14202/vetworld.2013.992-996
Zhu, W., Wei, Z. H., Xu, N. N., Yang, F., Yoon, I., Chung, Y. H., et al. (2017). Effects of Saccharomyces cerevisiae fermentation products on performance and rumen fermentation and microbiota in dairy cows fed a diet containing low quality forage. J. Animal Sci. Biotechnol. 8:36. doi: 10.1186/s40104-017-0167-3
Keywords: yeast culture, growing bulls, growth performance, metabolomics, heat stress, antioxidant variables
Citation: Zhang X, Liang H, Xu L, Zou B, Zhang T, Xue F and Qu M (2022) Rumen fermentative metabolomic and blood insights into the effect of yeast culture supplement on growing bulls under heat stress conditions. Front. Microbiol. 13:947822. doi: 10.3389/fmicb.2022.947822
Received: 19 May 2022; Accepted: 18 July 2022;
Published: 06 September 2022.
Edited by:
Haifeng Zhao, South China University of Technology, ChinaReviewed by:
Yangchun Cao, Northwest A&F University, ChinaShaoxun Tang, Institute of Subtropical Agriculture (CAS), China
Copyright © 2022 Zhang, Liang, Xu, Zou, Zhang, Xue and Qu. This is an open-access article distributed under the terms of the Creative Commons Attribution License (CC BY). The use, distribution or reproduction in other forums is permitted, provided the original author(s) and the copyright owner(s) are credited and that the original publication in this journal is cited, in accordance with accepted academic practice. No use, distribution or reproduction is permitted which does not comply with these terms.
*Correspondence: Fuguang Xue, eHVlZnVndWFuZzEwMjRAanhhdS5lZHUuY24=; Mingren Qu, cXVtaW5ncmVuQHNpbmEuY29t