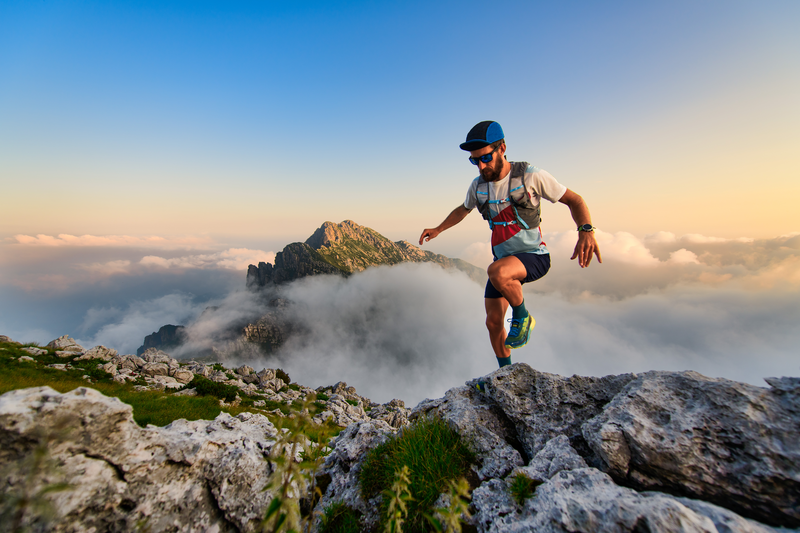
94% of researchers rate our articles as excellent or good
Learn more about the work of our research integrity team to safeguard the quality of each article we publish.
Find out more
METHODS article
Front. Microbiol. , 14 July 2022
Sec. Microbiotechnology
Volume 13 - 2022 | https://doi.org/10.3389/fmicb.2022.947821
Streptococcus suis is an important zoonotic pathogen, however, an efficient markerless genetic manipulation system is still lacking for further physiological and pathological studies on this bacterium. Several techniques have been developed for markerless genetic manipulation of S. suis utilizing either a temperature-sensitive vector or a counterselectable markers (CSMs), however, at present, the efficiency of these techniques is not very satisfactory. In this study, we developed a strategy for markerless genetic manipulation of S. suis employing a CSM based on a conditionally lethal mutant allele of pheS, which encodes the α-subunit of phenylalanyl-tRNA synthetase (PheS). This mutant pheS, mPheS, was constructed by introducing site-directed mutations for a T261S/A315G double-substitution and a number of silent mutations to decrease its similarity with the endogenous wild type pheS gene (wtPheS). Additionally, five potentially strong promoters from S. suis were screened for their ability to drive high-level expression of mPheS, thus endowing the carrier strain with sufficient sensitivity to the phenylalanine analog p-chloro-phenylalanine (p-Cl-phe). Insertion of these P-mPheS cassettes into a vector or into the chromosomal locus via a linked erythromycin resistance gene revealed that mPheS allele driven by promoters P0530 and P1503 renders S. suis sensitive to as low as 0.01% (or 0.5 mM) of p-Cl-phe. This offers two potential CSMs for S. suis with p-Cl-phe as a counterselective agent. P1503-mPheS was revealed to be 100% efficient for counter-selection in S. suis by application in a precise gene deletion. Using P1503-mPheS as a CSM, a two-step insertion and excision strategy for markerless genetic manipulation of S. suis were developed, supplying a powerful tool for markerless genetic manipulation of S. suis.
Streptococcus suis is a Gram-positive bacterium and is associated with a wide range of diseases in pigs, including meningitis, arthritis, septicemia, and death (Segura, 2009; Palmieri et al., 2012; Goyette-Desjardins et al., 2014). More seriously, it can be transmitted to humans by exposure to sick pigs, causing meningitis, streptococcal toxic shock-like syndrome, and death. S. suis was reported to be an emerging and re-emerging zoonotic pathogen, and seriously threatens the swine industry and human public health (Lun et al., 2007; Palmieri et al., 2012; Feng et al., 2014; Goyette-Desjardins et al., 2014). During the last two decades, S. suis has attracted widespread attention from researchers, and significant progress has been made in understanding its physiological and pathological process (Segura et al., 2017; Lin et al., 2019; Tan et al., 2019, 2021; Xie et al., 2019; Chen et al., 2020). However, further sophisticated and unbiased investigation of S. suis’s physiology and pathology process urgently needs an efficient genetic manipulation system for precise and clean genetic manipulation such as scarless gene-deletion, gene-fusion, and point-mutation.
In S. suis, the most frequently used genetic manipulation system is based on the pSET4s thermosensitive suicide vector (Takamatsu et al., 2001b). The procedure involved the introduction of vector into S. suis by electroporation, and two steps of allelic exchange. However, this system is labor-intensive and time-consuming, and electrotransformation does not work well for certain S. suis isolates. Recently, a cloning-independent method employing peptide-induced competence has been established in S. suis (Zaccaria et al., 2014). It allows high-throughput mutation, but the resulted mutant must carry an antibiotic-resistance gene for selection, limiting its use in markerless and multiple gene manipulations.
Counterselection systems have been reported as an efficient way to establish markerless mutants in many bacteria (Kristich et al., 2005; Kino et al., 2016; Argov et al., 2017). Only very recently, counterselectable markers (CSMs) were inspected for their use in S. suis genetic manipulation. The well-known Bacillus subtilis levansucrase SacB and the Vibrio parahaemolyticus toxin YoeB have been tested for their potential for counter-selection in S. suis (Zhang et al., 2019; Zheng et al., 2021). Though the target markerless mutations have been successfully selected by these CSMs, the notorious counter-selection escape of the SacB-based system and the incomplete growth inhibition of the YoeB-based system strongly limited the efficiency of these systems and urged us to explore a more effective CSM alternative for S. suis.
Among the known counterselectable genes, derivatives of the pheS gene, which encodes the α-subunit of phenylalanyl-tRNA ligase PheS, has recently been reported to be an effective CSM for genetic deletion in several bacteria (Kino et al., 2016; Zhou et al., 2017; Kharchenko et al., 2018; Liu et al., 2018, 2020; Wang et al., 2018; Schuster et al., 2019). The Escherichia coli PheS mutant, containing an A294G single-substitution, is able to misincorporate the phenylalanine analog p-chloro-phenylalanine (p-Cl-phe) into proteins during translation, thereby causing cell death (Kast and Hennecke, 1991). After the initial use for counter-selection in E. coli, various pheS markers were then adapted for the genetic engineering of both Gram-negative and Gram-positive bacteria (Kast, 1994; Kristich et al., 2007; Argov et al., 2017; Zhou et al., 2017). The drawback of this single-substitution mutant is its rather low selection efficiency, which requires the selection to be performed on a minimal or semi-rich medium with relatively high concentrations of p-Cl-phe (>5 mM). Later Miyazaki (2015) demonstrated that the T251S/A294G double-substituted PheS mutant possessed higher p-Cl-phe incorporation efficiency, and Kharchenko et al. (2018) proved that in Bacillus this double-substituted PheS mutant confers higher sensitivity to p-Cl-phe even in rich medium.
In order to develop an effective CSM, and establish a markerless genetic manipulation strategy for S. suis, in this study, a synthetic pheS gene mutant (mPheS) was designed with two site-directed mutations and a number of silent mutations from the native wild type pheS gene (wtPheS). Driven by a strong promoter, this mPheS enables an extremely efficient two-step strategy for markerless genetic manipulation of S. suis.
Streptococcus suis strain 05ZYH33 was used as a wild type strain (WT) (Chen et al., 2007). S. suis strains were cultured at 37°C with 5% CO2 on Tryptic Soy Agar (TSA) or in Tryptic Soy broth (TSB) supplemented with 5% (vol/vol) horse serum (Biosharp). E. coli strains were grown in Luria–Bertani (LB) broth or on LB agar at 37°C. All pSET2-derived plasmids were propagated in E. coli MC1061F-strain (Takamatsu et al., 2001a). When required, the indicated concentration of p-Cl-phe (Sigma) was added to the TSA before autoclaving. Spectinomycin was added to the medium at 50 μg/ml for E. coli and 100 μg/ml for S. suis. Erythromycin was added to the medium at 4 μg/ml for S. suis.
Wild type pheS gene was searched from the genome of S. suis 05ZYH33 (GenBank: CP000407) by BLAST with the pheS of S. mutans (Zhang et al., 2017). mPheS was then designed based on wtPheS nucleotide sequence by introducing a double-substitution and a number of silent mutations. To introduce the double-substitution corresponding to T251S/A294G of E. coli PheS, the threonine and alanine counterparts were identified by sequence alignment of PheS proteins from E. coli, S. mutans, Listeria monocytogenes, Bacillus amyloliquefaciens, and S. suis using ClustalW. To introduce silent mutations, codon adaptation software Jcat (Grote et al., 2005) was employed to perform one round of codon adaption using S. pyogenes codon usage as the reference, then manual modification was applied to further decrease the nucleotide sequence similarity between mPheS to wtPheS. The final modified mPheS gene was synthesized by BGI (Beijing, China).
Five potentially strong promoters P0177, P0530, P1503, P1815, and P1868 were used to drive the expression of mPheS, in comparison with the native pheS promoter (Pwt). These five promoters have previously been identified as strong promoters for two reasons. First, they are responsible for the expression of five high abundant proteins in S. suis 05ZYH33, with genome locus_tag of SSU05_0177, SSU05_0530, SSU05_1503, SSU05_1815, and SSU05_1868, respectively. Second, they could drive high level expression of exogenous GFP in S. suis (Liu et al., 2019). In order to construct the P-mPheS cassettes (mPheS gene driven by different promoters), mPheS gene was amplified from the above synthesized mPheS gene with primers mPheS-F/mPheS-R. Six promoters, including the five potentially strong promoters and Pwt were amplified from S. suis 05ZYH33 chromosomal DNA using corresponding primers listed in Table 1. The fragment Pwt-wtPheS which contains native pheS gene and its promoter was also amplified to be used as a control. The Pwt-mPheS, P0177-mPheS, P0530-mPheS, P1503-mPheS, P1815-mPheS, and P1868-mPheS cassettes were constructed using overlap-extension PCR strategy, with the amplicons of mPheS and each of the six promoters as templates. These cassettes and Pwt-wtPheS were recombinationally cloned into EcoRI/BamHI double-digested shuttle vector pSET2 using Trelief SoSoo cloning Kit Ver. 2 (Tsingke, Beijing), and verified by PCR and sequencing with primers pSET2-F/pSET2-R. All the primers used in this study are listed in Table 1.
To integrate the P-mPheS cassettes into the genome of S. suis, an appropriate integration site, and a linked positive-selection marker are needed. In this study, the site right downstream of gene ssu05-0630 was chosen as the integration site on the basis of our previous construction of an erythromycin-resistant-gene (erm) substituted ssu05-0630 gene-deletion strain (Chen et al., 2019, 2020). It has been shown that the gene modification at this site does not affect the growth of S. suis (Chen et al., 2019). Furthermore, the positive-selection marker erm and the downstream sequence can be conveniently amplified as one amplicon from the gene-deletion strain. To construct the fused DNA fragments for integration, the upstream sequence (UP0630) and the erm marker with the downstream sequence (Erm-DN0630) were amplified from the ssu05-0630 gene-deletion strain. The six P-mPheS cassettes were amplified from the above constructed plasmids with the corresponding primers listed in Table 1. Each of the P-mPheS cassettes was fused with UP0630 and erm-DN0630 using overlap-extension PCR in the order of UP0630-P-mPheS-erm-DN0630. The fused DNA fragments were transformed into S. suis 05ZYH33, and plated on TSAS-Erm medium (TSA supplemented with horse serum and erythromycin) for positive-selection. The integration of P-mPheS cassettes in positive clones was confirmed by PCR and sequencing using primers SeqF/SeqR.
A peptide-induced transformation was performed as previously described (Zaccaria et al., 2014) with slight modifications. The peptide (GNWGTWVEE) was synthesized by GenScript (China) at 95% purity. It was dissolved in deionized water at a final concentration of 5 mM, divided into aliquots, and stored at −20°C. Overnight culture of S. suis 05ZYH33 was diluted 1:100 in fresh TSBS medium and grown for 1.5, 2, 2.5, and 3 h. For each time point, a 50 μl culture was collected, and mixed with 2.5 μl of peptide and 1 μg of DNA (plasmid or PCR product). Following 4 h of incubation, the mixtures were plated on TSAS (TSA supplemented with horse serum) containing spectinomycin, erythromycin, or p-Cl-phe for selection.
To inspect the sensitivity of S. suis strains to p-Cl-phe, growth inhibition test was performed. Overnight cultures of S. suis strains were diluted in fresh medium and grown to OD600 nm 0.6. Each culture was undiluted or 10-fold serially diluted up to 10–5, and 5 μl of each dilution was spotted on to TSAS plate supplemented with indicated concentrations of p-Cl-phe. The growth of strains on the plates was photographically documented after 24 h incubation at 37°C, 5% CO2. The minimum inhibitory concentration (MIC) for each strain was defined as the lowest concentration of p-Cl-phe that inhibits the visible growth of that strain.
To test the efficiency of the P1503-mPheS as a CSM, it was used for markerless deletion of ireB gene (genome locus_tag SSU05_0066). It is a homolog of the ireB gene from Enterococcus faecalis and reoM gene from L. monocytogenes, both were reported to play important roles in peptidoglycan synthesis (Hall et al., 2013; Wamp et al., 2020). The whole process involved the construction of two fused DNA fragments and twice transformation. Using the corresponding primers listed in Table 1 for ireB gene-deletion, the upstream and downstream sequences, i.e., UP1, DN1, UP2, DN2, of ireB gene were amplified from the WT strain, and the P1503-mPheS-erm (designated as P1503PE) sequence was amplified from the strain with genome-integrated P1503PE cassette. For the first transformation, the first fused fragment of P1503PE flanked by UP1 and DN1 was prepared by overlap-extension PCR. The resulted UP1-P1503PE-DN1 fragment was transformed into WT and screened on TSAS-Erm plates. Positives colonies were inoculated on TSAS plate supplemented with 0.05% p-Cl-phe to confirm its p-Cl-phe sensitivity, yielding the P1503PE-substituted ireB gene-deletion intermediate strain, ireBΔPPE. For the second transformation, the second UP2-DN2 fusion fragment was prepared and transformed into the above p-Cl-phe-sensitive ireBΔPPE intermediate strain, and counter-selected on the TSAS plate supplemented with 0.05% p-Cl-phe. The positive clones were verified by PCR with primers ireB-F/ireB-R. The percentage of ireB gene-deleted colonies was determined by random analysis of 100 colonies. The experiment of ireB gene-deletion was performed three times to confirm the counter-selection efficiency.
The DNA sequence of the modified mPheS gene has been deposited in GenBank under the accession number: ON184273.
The wtPheS with locus_tag of SSU05_1152 was identified from S. suis 05ZYH33 genome. Sequence alignment revealed that T261 and A315 are the counterparts corresponding to the T251 and A294 of E. coli PheS protein (Figure 1A). To develop a mPheS-based CSM, functional similarity is important for competition with the endogenous wtPheS for PheT-PheS complex formation (Ibba et al., 1994; Argov et al., 2017), therefore, a mPheS gene mutant was directly derived from the wtPheS based on the following two considerations. First, to effectively misincorporate p-Cl-phe, T261 and A315 were substituted by serine and glycine, respectively. Second, in order to avoid unwanted homologous recombination, silent mutations were introduced into mPheS to decrease the nucleotide sequence homology between the wtPheS and mPheS allele. As shown in Figures 1A,B, after double-substitution, codon adaption and manual modification, the resulting mPheS showed 73.7% similarity to wtPheS gene, and the longest continuously matched sequence between them is no more than 8 bp. Meanwhile, the protein sequence encoded by the mPheS showed exact identity with the wtPheS protein, except for the T261S and A315G double-substitution (Figure 1C).
Figure 1. PheS-related sequence alignment. (A) Amino acid sequence alignment of PheS proteins from E. coli, S. mutans, Listeria monocytogenes, Bacillus amyloliquefaciens, and S. suis. The T251 and A294 of PheS from E. coli and corresponding counterparts were indicated by stars. (B) Nucleotide sequence alignment of the wtPheS and mPheS genes. (C) Amino acid sequence alignment of the wtPheS and mPheS proteins. The T261S and A315G substitutions were indicated by stars.
To explore whether mPheS has potential as a CSM for S. suis, shuttle vector pSET2 was used to carry mPheS gene into S. suis. Furthermore, it was reported that high level of mPheS expression is important for functional competition with the endogenous wtPheS, which is key for effective misincorporation of p-Cl-phe and growth inhibition (Argov et al., 2017). To achieve high-level expression of mPheS allele, five previously identified potentially strong promoters (P0177, P0530, P1503, P1815, and P1868) (Liu et al., 2019) were selected to drive the gene expression of mPheS in addition to the native promoter Pwt. As shown in Figure 2, to construct the S. suis strains with pSET2-carried P-mPheS cassettes, fragments of Pwt-wtPheS, P0177, P0530, P1503, P1815, P1868, Pwt, and mPheS were amplified accordingly (Figure 2A). Then the six promoters were individually fused with mPheS gene, producing P0177-mPheS, P0530-mPheS, P1503-mPheS, P1815-mPheS, P1868-mPheS, and Pwt-mPheS cassettes (Figure 2B). Pwt-wtPheS and the six P-mPheS cassettes were recombinationally inserted into pSET2, and transformed into WT, yielding strains containing pSET2-carried mPheS driven by different promoters, namely pPwt-wtPheS, pP0177-mPheS, pP0530-mPheS, pP1503-mPheS, pP1815-mPheS, pP1868-mPheS, and pPwt-mPheS (Figures 2C,D and Table 2).
Figure 2. Construction and growth inhibition test of S. suis strains with plasmid-carried P-mPheS cassettes. (A) Amplification of Pwt-wtPheS, the six promoters (P0177, P0530, P1503, P1815, P1868, Pwt) and mPheS fragments. (B) Fused P-mPheS cassettes. (C) PCR confirmation of the S. suis transformants containing plasmid-carried P-mPheS cassettes (pP-mPheS) with primers pSET2-F/pSET2-R. (D) Diagram for the constructed plasmids carrying P-mPheS cassettes. (E) Growth inhibition of pP-mPheS strains by the indicated concentrations of p-Cl-phe.
Next, the p-Cl-phe sensitivity endowed by P-mPheS cassettes to the carrier strains was inspected. Growth inhibition by p-Cl-phe was tested with TSAS plates containing 0, 0.1, and 0.2% p-Cl-phe. Strains containing pPwt-wtPheS and empty pSET2 were both used as control that expresses wtPheS but not mPheS. As shown in Figure 2D, all the strains grew well on the TSAS without p-Cl-phe. No growth was observed with 0.2% p-Cl-phe, even for the control strains without mPheS expression, indicating that 0.2% p-Cl-phe was too high for S. suis counter-selection. With 0.1% p-Cl-phe, clear differences in sensitivity were displayed by strains carrying different P-mPheS cassettes. Only slight growth inhibition was observed for control strains expressing no mPheS, as well as the strain carrying mPheS driven by Pwt (pPwt-mPheS). The latter indicates that mPheS protein could not compete well with the endogenous wtPheS protein when they have similar expression levels. In contrast, the growth of the five strains containing mPheS driven by potentially strong promoters was significantly inhibited. No growth was observed even for the un-diluted culture of strains containing P0530-mPheS and P1503-mPheS cassettes, while only a few colonies were observed for the un-diluted culture of strains containing P0177-mPheS, P1815-mPheS, and P1868-mPheS cassettes. This indicates that mPheS expression driven by all these five promoters is able to overwhelmingly compete with the endogenous wtPheS, thus endowing the carrier strains with sufficient sensitivity to p-Cl-phe. Therefore, P0177-mPheS, P0530-mPheS, P1503-mPheS, P1815-mPheS, and P1868-mPheS cassettes all have potential as a CSM for S. suis, among them P0530-mPheS and P1503-mPheS have the greatest potential.
To further test the potential of the P-mPheS cassettes as CSM for S. suis when they were integrated into the genome via a positive-selection marker, which is the real case for counter-selection, they were integrated into the site right downstream of ssu05_0630 gene via an erm marker. As shown in Figure 3, the upstream sequence (UP0630) and the erm marker with downstream sequence (Erm-DN0630) were amplified from the erm-substituted ssu05-0630 gene-deletion strain we previously constructed, while the six P-mPheS cassettes (including the Pwt-mPheS cassette) were amplified from the above-constructed plasmids (Figure 3A). Each of the six P-mPheS fused with UP0630 and erm-DN0630, yielding fused DNA fragments of UP-P0177PE-DN, UP-P0530PE-DN, UP-P1503PE-DN, UP-P1815PE-DN, UP-P1868PE-DN, and UP-PwtPE-DN (for short, P-mPheS-erm was designated as PPE) (Figure 3B). After transformation, it was confirmed that the cassettes P0177PE, P0530PE, P1503PE, P1815PE, and P1868PE were successfully integrated into the site right downstream of ssu05-0630 gene (Figure 3C), yielding strains gP0177PE, gP0530PE, gP1503PE, gP1815PE, and gP1868PE (Figure 3D and Table 2). However, no transformant was obtained for the UP-PwtPE-DN fragment, very possibly due to the high sequence identity to the endogenous Pwt-wtPheS locus, leading to off-target recombination and failure of targeted integration.
Figure 3. Construction and growth inhibition test of S. suis strains with genome-integrated P-mPheS cassettes. (A) Amplification of UP0630, the six P-mPheS cassettes, and Erm-DN0630 fragments. (B) Fused UP-PPE-DN fragments. (C) PCR confirmation of S. suis transformants with genome-integrated P-mPheS cassettes (gPPE) with primers SeqF/SeqR. (D) Diagram for the constructed S. suis with genome-integrated P-mPheS cassettes. (E) Growth inhibition of gPPE strains by the indicated concentrations of p-Cl-phe. The MIC for each strain was indicated with dashed cycle.
The p-Cl-phe sensitivity of these strains was tested with TSAS plates containing 0–0.15% p-Cl-phe. As shown in Figure 3E, the WT strain grew well with p-Cl-phe concentrations below 0.1%, with the MIC of p-Cl-phe above 0.15%. In contrast, all five strains integrated with PPE were sensitive to 0.1% of p-Cl-phe. The MIC of p-Cl-phe for strains gP0177PE, gP0530PE, gP1503PE, gP1815PE, and gP1868PE were 0.02, 0.01, 0.01, 0.08, and 0.06%, respectively (Figure 3E). These results are in good consistent with those obtained with the above pP-mPheS strains, further confirming that mPheS driven by all these five promoters has potential as a CSM for S. suis counter-selection. Furthermore, as the gP0530PE and gP1503PE strains have the lowest MIC of p-Cl-phe, which is as low as 0.01%, less than one fifteenth of the MIC for the WT strain, the P0530-mPheS and P1503-mPheS cassettes in these strains have the greatest potential as a CSM for S. suis. Considering the promoter is an endogenous sequence from S. suis, the shorter, the less possibility of unwanted recombination. Therefore, the P1503-mPheS cassette containing the shorter promoter (197 bp of P1503 vs. 530 bp of P0530) suits better as a CSM for S. suis, but P0530-mPheS cassette still can be a good option when needed.
To evaluate the efficiency of P1503-mPheS cassette as a CSM for S. suis, we applied it in the construction of a markerless ireB gene-deletion mutant. We choose this gene because when we tried to investigate the function of IreB protein in S. suis, we found that ireB gene locates in an operon containing 4–5 genes (data not shown), therefore, markerless deletion of ireB gene is necessary to avoid the unwanted polar effect. First, the upstream and downstream sequences of ireB gene, i.e., UP1 and DN1 and the P1503PE fragment was amplified from WT and gP1503PE strain, respectively (Figure 4A). Then they were fused to produce fragment UP1-P1503PE-DN1 (Figure 4B) and transformed WT strain. Screened by erythromycin, the P1503PE-substituted ireB gene-deletion intermediate strain ireBΔ PPE has been obtained (Figure 4C). The intermediate strains were confirmed to be resistant to erythromycin and sensitive to 0.05% p-Cl-phe (a mid-value concentration between MICs for WT and gP1503PE) (Figure 4D). Then an intermediate ireBΔ PPE strain was used for the second transformation. The upstream and downstream sequences of ireB gene, this time named UP2 and DN2 to distinguish from the sequences for the first transformation, were amplified (Figure 4E) and fused as UP2-DN2 fragments (Figure 4F). The UP2-DN2 fragment was transformed into the ireBΔ PPE strain, and plated on TSAS containing 0.05% p-Cl-phe for counter-selection. A total of 100 colonies were randomly picked and tested using PCR. The whole experiment was repeated three times. Each time, of the p-Cl-phe-resistant colonies, 100% harbored the corrected genetic deletion (Figure 4G), indicating that P1503-mPheS is 100% efficient as a CSM for S. suis counter-selection.
Figure 4. Markerless deletion of ireB gene using the P1503-mPheS cassette as a CSM. (A) Amplification of upstream sequence (UP1) and downstream sequence (DN1) of ireB gene and P1503PE cassette. (B) Fused UP1-P1503PE-DN1 fragment. (C) PCR confirmation of two ireBΔ PPE intermediate transformants with primers ireBseq-F/ireBseq-R. (D) Confirmation of the resistance to erythromycin and the sensitivity to 0.05% p-Cl-phe for two ireBΔ PPE intermediate strains (ireBΔ PPE1, ireBΔ PPE2). (E) Amplification of upstream sequence (UP2) and downstream sequence (DN2) of ireB gene. (F) Fused UP2-DN2 fragment. (G) PCR confirmation of the colonies survived from counter-selection with primers ireB-F/ireB-R.
The 100% counter-selection efficiency of P1503-mPheS provides an intermediate strain that can be effectively removed using p-Cl-phe as a counterselective agent, thereby shaping a two-step insertion and excision strategy for markerless genetic manipulation of S. suis. As shown in the example of ireB gene-deletion and summarized in Figure 5, this strategy is clone-independent, and only two fused fragments and two transformations were needed. First, the upstream and downstream sequences of the mutation site were fused with P1503PE marker to yield the UP-P1503PE-DN fragment for the first transformation, which was selected by the introduced positive-selection marker erm, yielding a P1503PE-containing intermediate strain. Second, the upstream and downstream sequences of the mutation site are either directly fused together (for gene-deletion) or fused with a gene (such as gene of a fluorescent protein for gene-fusion), or fused with a mutated gene (for gene-mutation), to yield the second fragment for the second transformation, which was counter-selected by p-Cl-phe.
Figure 5. Diagram for the two-step markerless gene-deletion strategy developed for S. suis in this study, using erm as a positive-selection marker and P1503-mPheS as a CSM.
Except for the above ireB gene-deletion, we have also applied the strategy to markerless construct gfp- or rfp-fusion strains (data not shown). In any case, the efficiency of the counter-selection is 100%, indicating that it is repeatable. This extremely high efficiency is not totally unexpected, as the mPheS-based CSM has been revealed as 100% efficient for counter-selection in S. mutans (Xie et al., 2011; Zhang et al., 2017). The high efficiency is further guaranteed by the wide difference between the MICs of p-Cl-phe to the strains with and without P1503-mPheS, which are 0.01 and >0.15%, respectively. Here in this study, we used a mid-value concentration (0.05%) between two MICs, there is still room to increase to ensure the 100% efficiency in future applications.
In contrast, the sacB gene and yeoB gene reported for S. suis is less efficient. When the sacB gene was introduced as a CSM for S. suis, the efficiency was not mentioned in that report (Zhang et al., 2019). We followed the report to use the native sacB gene and its promoter from B. subtilis for S. suis counter-selection, however, no target clone was obtained after screening several hundred colonies, suggesting a pretty low efficiency for counter-selection using this native B. subtilis sacB and promoter. We then adapted sacB gene codon for S. suis and utilized P1503 to drive the codon-adapted sacB gene expression. The efficiency was significantly increased and made sacB gene suitable for S. suis counter-selection. But in most cases, the counter-selection efficiency is about 50%. It is not surprising, as the MICs of sucrose for strains with and without P1503-sacB were around 7 and 10%, respectively (our unpublished data). This small difference between the two MICs makes counter-selection escape easy to happen, thus limiting the counter-selection efficiency. For the yeoB system, induction of the toxin only partially inhibits the growth of yeoB-containing strain, it is not surprising that the final culture needs to be enriched for several generations in presence of the inducer, but still, the efficiency could not reach 100% (Zheng et al., 2021). All in all, the P1503-mPheS developed in this study is the most efficient CSM for S. suis up to now.
Though the mPheS-based markerless gene manipulation strategy has been developed in several bacteria, the double-substituted mPheS-based CSM was newly developed and lacked extensive verification in other species. Therefore, it is still necessary to verify its efficacy in S. suis and determine many details for S. suis, such as the pheS gene itself, mutations to be introduced, appropriate selection concentration of p-Cl-phe, and especially the strong promoter specific for S. suis. All of these need to be carefully set up in the background of S. suis. Here in this study, we identified the wtPheS gene from S. suis. After the introduction of mutations and different promoters, we investigated the potential of these mPheS-based CSM for markerless gene manipulation in S. suis, either carried by plasmid or integrated into the genome. Finally, the P1503-mPheS was proved to be highly effective under 0.01% (or 0.5 mM) p-Cl-phe, and used to develop an efficient two-step strategy for markerless gene manipulation of S. suis. With this strategy, markerless gene manipulation of S. suis is time-saving and takes about 1 week, moreover, no unwanted recombination has been observed so far.
In consideration of convenience, mPheS-based CSM is also prior to the SacB- or YoeB-based CSM, as the counter-selection agent p-Cl-phe only needs to be added directly into the medium before autoclaving. In contrast, the sucrose needs to be sterilized by filtration separately and added after autoclaving, while the yoeB system needs several generations of enrichment with an inducer.
As both the wtPheS gene and P1503 are highly conserved in S. suis, the strategy developed in this study has great potential to be widely used in different S. suis strains. This strategy requires an efficient transformation method, which is not a problem because Zhu et al. (2019) recently developed the convenient peptide-induced transformation method for S. suis strains from different serotypes.
Though the two-step strategy is very efficient, it has limitations. It needs two rounds of transformation. This can be problematic if the transformability of the strain is negatively affected after the first transformation step. To address this problem in S. mutans, Zhang et al. (2017) developed a next-generation counterselection cassette by introducing a short repeat sequence in the fused DNA fragment for the first transformation step. After obtaining the intermediate strain with positive selection, the strain was passaged several generations in absence of the selective agent. During the passages, the repeat sequence will mediate an in vivo recombination to remove the marker, and the markerless strains were then counter-selected by p-Cl-phe, thus skipping the second transformation step (Zhang et al., 2017).
We practiced this repeat sequence-mediated one-step strategy in S. suis mediated by a 200-bp repeat. Guaranteed by the high efficacy of counter-selection by P1503-mPheS, we successfully got the target markerless gene mutation strain with one transformation (data now shown). However, it was much trickier to fuse one more fragment by overlap extension PCR. We prefer to use the two-step strategy for the first choice, and when it is problematic, use the repeat sequence-mediated one-step strategy as an option.
In summary, this study supplies a rapid and efficient tool for sophisticated genetic analysis of S. suis. Hopefully, it will accelerate the physiological and pathological studies of this important zoonotic pathogen.
The data presented in the study are deposited in the GenBank repository, accession number ON184273.
YZ, GG, DW, GL, PC, and LW performed the experiments and data analysis. YZ and SL wrote the manuscript. All authors contributed to the article and approved the submitted version.
This research was supported by grants from the National Key R&D Program of China (2021YFD1800401) and the National Natural Science Foundation of China (32172853).
The authors declare that the research was conducted in the absence of any commercial or financial relationships that could be construed as a potential conflict of interest.
All claims expressed in this article are solely those of the authors and do not necessarily represent those of their affiliated organizations, or those of the publisher, the editors and the reviewers. Any product that may be evaluated in this article, or claim that may be made by its manufacturer, is not guaranteed or endorsed by the publisher.
Argov, T., Rabinovich, L., Sigal, N., and Herskovits, A. A. (2017). An effective counterselection system for Listeria monocytogenes and its use to characterize the monocin genomic region of strain 10403S. Appl. Environ. Microbiol. 83, e02927–16. doi: 10.1128/AEM.02927-16
Chen, C., Tang, J., Dong, W., Wang, C., Feng, Y., and Wang, J. (2007). A glimpse of streptococcal toxic shock syndrome from comparative genomics of S. suis 2 Chinese isolates. PLoS One 2:e315. doi: 10.1371/journal.pone.0000315
Chen, P., Liu, R., Huang, M., Zhu, J., Wei, D., Castellino, F. J., et al. (2020). A unique combination of glycoside hydrolases in Streptococcus suis specifically and sequentially acts on host-derived aGal-epitope glycans. J. Biol. Chem. 295, 10638–10652. doi: 10.1074/jbc.RA119.011977
Chen, P., Liu, R., Huang, M., Zhu, J., Xie, F., Ni, H., et al. (2019). Identification and characterization of a surface-localized N-acetylglucosaminase in Streptococcus suis serotype 2 strain 05ZYH33 (in Chinese). Chin. J. Prev. Vet. Med. 41, 462–467.
Feng, Y. J., Zhang, H. M., Wu, Z. W., Wang, S. H., Cao, M., Hu, D., et al. (2014). Streptococcus suis infection: an emerging/reemerging challenge of bacterial infectious diseases? Virulence 5, 477–497. doi: 10.4161/viru.28595
Goyette-Desjardins, G., Auger, J. P., Xu, J., Segura, M., and Gottschalk, M. (2014). Streptococcus suis, an important pig pathogen and emerging zoonotic agent-an update on the worldwide distribution based on serotyping and sequence typing. Emerg. Microbes Infec. 3:e45. doi: 10.1038/emi.2014.45
Grote, A., Hiller, K., Scheer, M., Munch, R., Nortemann, B., Hempel, D. C., et al. (2005). JCat: a novel tool to adapt codon usage of a target gene to its potential expression host. Nucleic Acids Res. 33, W526–W531. doi: 10.1093/nar/gki376
Hall, C. L., Tschannen, M., Worthey, E. A., and Kristich, C. J. (2013). IreB, a Ser/Thr kinase substrate, influences antimicrobial resistance in Enterococcus faecalis. Antimicrob. Agents Chemother. 57, 6179–6186. doi: 10.1128/AAC.01472-13
Ibba, M., Kast, P., and Hennecke, H. (1994). Substrate specificity is determined by amino acid binding pocket size in Escherichia coli phenylalanyl-tRNA synthetase. Biochemistry 33, 7107–7112. doi: 10.1021/bi00189a013
Kast, P. (1994). pKSS–a second-generation general purpose cloning vector for efficient positive selection of recombinant clones. Gene 138, 109–114. doi: 10.1016/0378-1119(94)90790-0
Kast, P., and Hennecke, H. (1991). Amino acid substrate specificity of Escherichia coli phenylalanyl-tRNA synthetase altered by distinct mutations. J. Mol. Biol. 222, 99–124. doi: 10.1016/0022-2836(91)90740-w
Kharchenko, M. S., Teslya, P. N., Babaeva, M. N., and Zakataeva, N. P. (2018). Improving the selection efficiency of the counter-selection marker pheS* for the genetic engineering of Bacillus amyloliquefaciens. J. Microbiol. Meth. 148, 18–21. doi: 10.1016/j.mimet.2018.03.011
Kino, Y., Nakayama-Imaohji, H., Fujita, M., Tada, A., Yoneda, S., Murakami, K., et al. (2016). Counterselection employing mutated pheS for markerless genetic deletion in Bacteroides species. Anaerobe 42, 81–88. doi: 10.1016/j.anaerobe.2016.09.004
Kristich, C. J., Chandler, J. R., and Dunny, G. M. (2007). Development of a host-genotype-independent counterselectable marker and a high-frequency conjugative delivery system and their use in genetic analysis of Enterococcus faecalis. Plasmid 57, 131–144. doi: 10.1016/j.plasmid.2006.08.003
Kristich, C. J., Manias, D. A., and Dunny, G. M. (2005). Development of a method for markerless genetic exchange in Enterococcus faecalis and its use in construction of a srtA mutant. Appl. Environ. Microbiol. 71, 5837–5849. doi: 10.1128/AEM.71.10.5837-5849.2005
Lin, L., Xu, L., Lv, W., Han, L., Xiang, Y., Fu, L., et al. (2019). An NLRP3 inflammasome-triggered cytokine storm contributes to Streptococcal toxic shock-like syndrome (STSLS). PLoS Pathog. 15:e1007795. doi: 10.1371/journal.ppat.1007795
Liu, M. F., Huang, Y., Liu, J. J., Biville, F., Zhu, D. K., Wang, M. S., et al. (2018). Multiple genetic tools for editing the genome of Riemerella anatipestifer using a counterselectable marker. Appl. Microbiol. Biotechnol. 102, 7475–7488. doi: 10.1007/s00253-018-9181-4
Liu, R., Huang, M., Chen, P., Zhu, J., Xie, F., Liu, S., et al. (2019). Screening and identification of promoters from Streptococcus suis serotype 2 strain 05ZYH33 (in Chinese). Chin. J. Prev. Vet. Med. 41, 584–589.
Liu, Y. C., He, X. R., Zhu, P. P., Cheng, M. G., Hong, Q., and Yan, X. (2020). pheS(AG) based rapid and efficient markerless mutagenesis in Methylotuvimicrobium. Front. Microbiol. 11:441. doi: 10.3389/fmicb.2020.00441
Lun, Z. R., Wang, Q. P., Chen, X. G., Li, A. X., and Zhu, X. Q. (2007). Streptococcus suis: an emerging zoonotic pathogen. Lancet Infect. Dis. 7, 201–209. doi: 10.1016/S1473-3099(07)70001-4
Miyazaki, K. (2015). Molecular engineering of a PheS counterselection marker for improved operating efficiency in Escherichia coli. Biotechniques 58, 86–88. doi: 10.2144/000114257
Palmieri, C., Varaldo, P. E., and Facinelli, B. (2012). Streptococcus suis, an emerging drug-resistant animal and human pathogen. Front. Microbiol. 2:235. doi: 10.3389/fmicb.2011.00235
Schuster, C. F., Howard, S. A., and Grundling, A. (2019). Use of the counter selectable marker PheS* for genome engineering in Staphylococcus aureus. Microbiol. SGM 165, 572–584. doi: 10.1099/mic.0.000791
Segura, M. (2009). Streptococcus suis: an emerging human threat. J. Infect. Dis. 199, 4–6. doi: 10.1086/594371
Segura, M., Fittipaldi, N., Calzas, C., and Gottschalk, M. (2017). Critical Streptococcus suis virulence factors: are they all really critical? Trends Microbiol. 25, 585–599. doi: 10.1016/j.tim.2017.02.005
Takamatsu, D., Osaki, M., and Sekizaki, T. (2001a). Construction and characterization of Streptococcus suis-Escherichia coli shuttle cloning vectors. Plasmid 45, 101–113.
Takamatsu, D., Osaki, M., and Sekizaki, T. (2001b). Thermosensitive suicide vectors for gene replacement in Streptococcus suis. Plasmid 46, 140–148. doi: 10.1006/plas.2001.1532
Tan, C., Zhang, A., Chen, H., and Zhou, R. (2019). Recent proceedings on prevalence and pathogenesis of Streptococcus suis. Curr. Issues Mol. Biol. 32, 473–520. doi: 10.21775/cimb.032.473
Tan, M. F., Hu, Q., Hu, Z., Zhang, C. Y., Liu, W. Q., Gao, T., et al. (2021). Streptococcus suis MsmK: novel cell division protein interacting with Ftsz and maintaining cell shape. mSphere 6, e119–e121. doi: 10.1128/mSphere.00119-21
Wamp, S., Rutter, Z. J., Rismondo, J., Jennings, C. E., Moller, L., Lewis, R. J., et al. (2020). PrkA controls peptidoglycan biosynthesis through the essential phosphorylation of ReoM. eLife 9:e56048. doi: 10.7554/eLife.56048
Wang, Y. C., Yuan, L. S., Tao, H. X., Jiang, W., and Liu, C. J. (2018). pheS* as a counter-selectable marker for marker-free genetic manipulations in Bacillus anthracis. J. Microbiol. Meth. 151, 35–38. doi: 10.1016/j.mimet.2018.05.024
Xie, F., Zan, Y., Zhang, Y., Zheng, N., Yan, Q., Zhang, W., et al. (2019). The cysteine protease ApdS from Streptococcus suis promotes evasion of innate immune defenses by cleaving the antimicrobial peptide cathelicidin LL-37. J. Biol. Chem. 294, 17962–17977. doi: 10.1074/jbc.RA119.009441
Xie, Z. J., Okinaga, T., Qi, F. X., Zhang, Z. J., and Merritt, J. (2011). Cloning-independent and counterselectable markerless mutagenesis system in Streptococcus mutans. Appl. Environ. Microbiol. 77, 8025–8033. doi: 10.1128/AEM.06362-11
Zaccaria, E., van Baarlen, P., de Greeff, A., Morrison, D. A., Smith, H., and Wells, J. M. (2014). Control of competence for DNA transformation in Streptococcus suis by genetically transferable pherotypes. PLoS One 9:e99394. doi: 10.1371/journal.pone.0099394
Zhang, S., Zou, Z., Kreth, J., and Merritt, J. (2017). Recombineering in Streptococcus mutans using direct repeat-mediated cloning-independent markerless mutagenesis (DR-CIMM). Front. Cell. Infect. Microbiol. 7:202. doi: 10.3389/fcimb.2017.00202
Zhang, Y., Zhong, X., Lu, P., Zhu, Y., Dong, W., Roy, S., et al. (2019). A novel autolysin AtlASS mediates bacterial cell separation during cell division and contributes to full virulence in Streptococcus suis. Vet. Microbiol. 234, 92–100. doi: 10.1016/j.vetmic.2019.05.020
Zheng, C., Wei, M., Qiu, J., and Li, J. (2021). A markerless gene deletion system in Streptococcus suis by using the copper-inducible vibrio parahaemolyticus YoeB toxin as a counterselectable marker. Microorganisms 9:1095. doi: 10.3390/microorganisms9051095
Zhou, C. Y., Shi, L. L., Ye, B., Feng, H. C., Zhang, J., Zhang, R. F., et al. (2017). pheS (*), an effective host-genotype-independent counter-selectable marker for marker-free chromosome deletion in Bacillus amyloliquefaciens. Appl. Microbiol. Biotechnol. 101, 217–227. doi: 10.1007/s00253-016-7906-9
Keywords: Streptococcus suis, markerless genetic manipulation, mutated PheS, counterselectable marker, strong promoter
Citation: Gao G, Wei D, Li G, Chen P, Wu L, Liu S and Zhang Y (2022) Highly Effective Markerless Genetic Manipulation of Streptococcus suis Using a Mutated PheS-Based Counterselectable Marker. Front. Microbiol. 13:947821. doi: 10.3389/fmicb.2022.947821
Received: 19 May 2022; Accepted: 13 June 2022;
Published: 14 July 2022.
Edited by:
Yu Wang, Tianjin Institute of Industrial Biotechnology (CAS), ChinaReviewed by:
Xiaohui Zhou, University of Connecticut, United StatesCopyright © 2022 Gao, Wei, Li, Chen, Wu, Liu and Zhang. This is an open-access article distributed under the terms of the Creative Commons Attribution License (CC BY). The use, distribution or reproduction in other forums is permitted, provided the original author(s) and the copyright owner(s) are credited and that the original publication in this journal is cited, in accordance with accepted academic practice. No use, distribution or reproduction is permitted which does not comply with these terms.
*Correspondence: Yueling Zhang, emhhbmcueWxAZm94bWFpbC5jb20=; emhhbmd5dWVsaW5nQGNhYXMuY24=
†These authors have contributed equally to this work and share first authorship
Disclaimer: All claims expressed in this article are solely those of the authors and do not necessarily represent those of their affiliated organizations, or those of the publisher, the editors and the reviewers. Any product that may be evaluated in this article or claim that may be made by its manufacturer is not guaranteed or endorsed by the publisher.
Research integrity at Frontiers
Learn more about the work of our research integrity team to safeguard the quality of each article we publish.