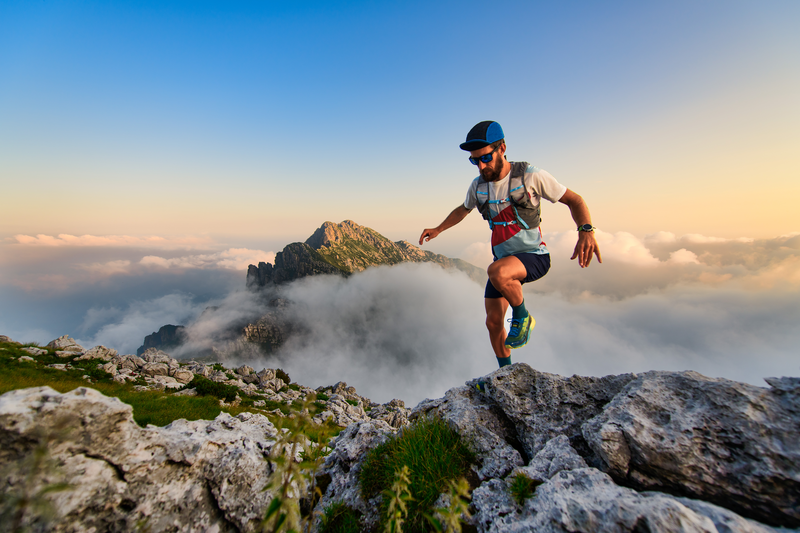
95% of researchers rate our articles as excellent or good
Learn more about the work of our research integrity team to safeguard the quality of each article we publish.
Find out more
ORIGINAL RESEARCH article
Front. Microbiol. , 10 August 2022
Sec. Microbiotechnology
Volume 13 - 2022 | https://doi.org/10.3389/fmicb.2022.944366
The study tested the water purification mechanism of the combination of microorganisms and purification materials via characteristic, enzymatic, and metagenomics methods. At 48 h, the removal rates of total nitrogen, total phosphorous, and Mn chemical oxygen demand in the combination group were 46.91, 50.93, and 65.08%, respectively. The alkaline phosphatase (AKP) activity increased during all times tested in the volcanic rock, Al@TCAP, and exogenous microorganism groups, while the organophosphorus hydrolase (OPH), dehydrogenase (DHO), and microbial nitrite reductase (NAR) activities increased at 36-48, 6-24, and 36-48 h, respectively. However, the tested activities only increased in the combination groups at 48 h. Al@TCAP exhibits a weak microbial loading capacity, and the Al@TCAP removal is primarily attributed to adsorption. The volcanic rock has a sufficient ability to load microorganisms, and the organisms primarily perform the removal for improved water quality. The predominant genera Pirellulaceae and Polynucleobacter served as the sensitive biomarkers for the treatment at 24, 36-48 h. Al@TCAP increased the expression of Planctomycetes and Actinobacteria, while volcanic rock increased and decreased the expression of Planctomycetes and Proteobacteria. The growth of Planctomycetes and the denitrification reaction were promoted by Al@TCAP and the exogenous microorganisms. The purification material addition group decreased the expression of Hyaloraphidium, Chytridiomycetes (especially Hyaloraphidium), and Monoblepharidomycetes and increased at 36-48 h, respectively. Ascomycota, Basidiomycota, and Kickxellomycota increased in group E, which enhanced the nitrogen cycle through microbial enzyme activities, and the growth of the genus Aspergillus enhanced the phosphorous purification effect.
In intensive aquaculture systems, such as cage and pond cultures, extra nutrients are often discharged without treatment (Santos et al., 2016). Approximately, 75% of aquaculture feed residues are converted into nitrogen and phosphorus in wastewater (Serpa et al., 2013). The use of purification materials can effectively reduce the contents of nutrients, such as nitrogen and phosphorus. Research on the actual treatment effect of aluminum-loaded attapulgite (Al@TCAP) on black and odorous water found that Al@TCAP can effectively reduce ammonia nitrogen (NH3-N) and total phosphorous (TP) in water (Yin et al., 2019). Volcanic rock can be used in the remediation of heavy metals (de Anda et al., 2019) and wastewater treatment (Wan et al., 2020).
However, the more effective and higher pollutant removal rate requires longer hydraulic resident times (HRT) in the tune of weeks to months. First, these nutrients typically promote the growth of aquatic plants because they readily absorb and utilize certain dissolved inorganic substances, such as ammonia, urea, and phosphate (i.e., PO43–-P, Franchino et al., 2016). Through the calculation, with the combined ammonia removal efficiencies of several nitrifying bacteria, the removal rate of NH3-N reached 71% (Sangnoi et al., 2017). The microorganisms have been used to convert dissolved organic matter into harmless substances, and the commercial micro-ecological products include effective microorganisms (EM), Bacillus, Streptococcus, Lactobacillus, and photosynthetic bacteria (Kuebutornye et al., 2019). The exogenous microorganisms were used to enhance the water purification effect when combined with purification materials that can provide a microorganism-loaded environment (Ren et al., 2021; Yin et al., 2022). The nitrifying and phosphorus-accumulating bacteria were enriched when added microorganisms in the activated sludge (Kuśnierz et al., 2022).
In Jiangsu province of China, the wastewater treatment system named “two dams and three districts” was constructed for water purification to remove suspended solids, nitrogen, and phosphorus. Two major problems in wastewater treatment systems should be solved urgently: the mechanism of the crosstalk in these interactive biochemical reactions (containing organics degradation, nitrification, denitrification, and microbial activities), and the enhancer method of water purification within the shorter HRTs in actual sewage treatment systems, even 40% removal rate obtained in our previous study via “Al@TCAP-volcanic rock + bacteria preparation + activated sludge.” Our recent study showed that the enhanced removal effect in this wastewater treatment system occurred when adding microorganisms and activated sludge (Yin et al., 2022), while the mode of action has not been determined.
In this study, the key factors governing the technical feasibility of the wastewater treatment system are evaluated: (1) the enhanced effect of exogenous microorganisms based on the two purification materials (Al@TCAP and volcanic rock) in the wastewater treatment system (superficial characteristic, nutrient removal, and enzyme activity), (2) the useful enhancers of bacterial and fungal category via 16S rRNA- and ITS-Seq methods. The purpose of this experiment was to explore the water purification mechanism effectiveness of a combination of microorganisms and purification materials.
The experimental wastewater was rearing water for tilapia Oreochromis niloticus from the base center of FFRC-CAFS. The water quality of the wastewater was determined during the experiment. The selected purification materials, Al@TCAP, and volcanic rock were prepared in our laboratory using the method reported by Yin et al. (2019) and Wan et al. (2020). The Al@TCAP and volcanic rock distribution method was tile and accumulation based on the optimal purification effect in the pre-experiment, and the total added amount was 6 g/L. The composed purification material added half of the amount (6 g/L) of Al@TCAP and volcanic rock. The exogenous microorganisms, included Bacillus licheniformis, Bacillus sp. (Jiangsu Suwei microbial research Co., Ltd.) (Ren et al., 2021), and activated sludge (Sk Hynix Semiconductor (Wuxi) Co., Ltd.) (Santorio et al., 2021; Kuśnierz et al., 2022), were named as three bacteria liquids. Their concentrations were 1 × 108-1 × 109 CFU/ml of a bacterial liquid dosage of 1 mg/L following Murray et al. (1981).
Five groups were used in the study: A, the control group (without purification materials or bacteria liquid); B, 6 g/L volcanic rock; C, 6 g/L Al@TCAP; D, 1 mg/L three bacteria liquids (actual detected as 4.6 × 108 CFU/mL); and E, 6 g/L Al@TCAP + 6 g/L volcanic rock + 1 mg/L three bacteria liquids (4.6 × 108 CFU/mL). First, the water samples collected at 0 h (s1), 6 h (s2), 12 h (s3), 24 h (s4), 36 h (s5), and 48 h (s6) after the start of the experiment were used to determine water quality and enzyme activity. The reasons for the sampling period selection mainly included the active enzymatic (Otte et al., 1996) and retention time (Alloul et al., 2021) for function, and the short centralized drainage time duration in the field culture of Jiangsu province. The purification materials were loaded with or without exogenous microorganisms, and their superficial characteristics were identified using SEM. The samples were fixed with 2.5% glutaric acid fixative solution before the electron microscopy analysis and then dried at 105°C for 6 h before the full-pore adsorption and desorption experiments.
The SEM method for the purification material is described in our previous studies (Zheng et al., 2020). The sample to be tested was vacuum degassed at 250°C for 3 h, the adsorption substance was N2, and the adsorption temperature was 77.3 K. The static adsorption equilibrium volume method was used to determine the gas adsorption isotherm of the sample. A specific surface area and porosity analyzer, ASAP 2460, was used for the full-pore adsorption and desorption experiments. Finally, the measured adsorption isotherm data were collected. The data for adsorption and desorption cumulative surface area of pores of the samples were determined.
The total nitrogen (TN), NH3-N, NO3–-N, nitrite nitrogen (NO2–-N), TP, PO43–-P, and Mn chemical oxygen demand (CODMn) were analyzed as described (State EPA of China, 2002). The dissolved oxygen (DO) was measured in situ with a YSI EXO2 multiparameter sonde (United States). The water removal rate was determined by dividing the decrease in water quality (treatment group minus control) by the original value.
A series of enzyme-linked immunosorbent assay kits including those for microbial ammonia monooxygenase (AMO), nitrate reductase (NAR), nitrite reductase (NIR), alkaline phosphatase (AKP), organophosphorus hydrolase (OPH), and dehydrogenase (DHO) were purchased from Meimian Biotechnology Company. The activities of AMO, NAR, NIR, AKP, OPH, and DHO were tested in the samples according to the protocols. Simply, the samples and embedded monoclonal antibodies were added to the microwells and then combined with a horseradish peroxidase enzyme. After thoroughly washing the samples, the substrate tetramethylbenzidine was revealed first in blue and then yellow before the acid stop solution was added. The intensity of the color was positively correlated with the enzyme tested in the sample. The absorbance (OD value) was measured at 450 nm using a microplate reader (Biomarker Technologies Corporation, Beijing, China). The activity concentration of the enzyme in the sample was calculated using the standard curve.
The metagenome used both 16SrRNA (bacterium) and ITS (fungus) sequences. In the 16SrRNA-Seq, the samples for M01-M03, M04-M06, M07-M09, M10-M12, and M13-M15 were named as groups A, B, C, D, and E at 0 h, respectively. The other samples for M16-M90 corresponded to the A-E group at 6, 12, 24, 36, and 48 h orderly. Total bacterial DNA extraction from samples, polymerase chain reaction amplification, and the methods described in Zheng et al. (2018) were used in the study, while for fungi, we followed the methods described in Fan et al.’s (2018) study. All PCR products were quantified using the Quant-iT dsDNA HS Reagent and pooled. High-throughput sequencing analysis of the fungi and bacterial rRNA genes was performed on the purified, pooled sample using the Illumina HiSeq 2500 platform (2 × 250 paired ends) at Biomarker Technologies Corporation (Beijing, China). The original data were spliced (FLASH, version 1.2.11), and the spliced sequences were quality filtered (Trimmomatic, version 0.33), and the illusions (UCHIME, version 8.1) were removed to obtain high-quality tag sequences. The sequences clustered with more than 97% similarity (USEARCH, version 10.0), while the operating taxonomical units (OTUs) were filtered with 0.005% as the threshold. Silva (release 132) was selected for the bacterial 16S database, and Unite (release 8.0) was chosen for the fungal ITS database. Using the RDP Classifier for species annotation, the confidence threshold was 0.8 (version 2.2). For multiple comparisons, the bacteria were PyNAST (version 1.2.2), the fungus was ClustalW2, and the phylogenetic tree was established using the neighbor-joining method.
The research aimed to identify the core microbiome in the same group and the presence of core bacteria that affect nitrification and denitrification in all the samples. The community indices we applied here include Chao1, Ace, Shannon, and Simpson indexes. A 0.5%, relative abundance threshold was used, which focused our analysis on PCR reproducible OTUs. To find the quality of 16SrRNA-Seq and ITS-Seq affected by the time (from M01 to M90) and different treatments (from groups A-E), the average data of five groups were taken as one group to obtain the new data for 0, 6, 12, 24, 36, and 48 h. The variation was calculated by selecting a significant increase or decrease in the bacterial community in a database constructed through a series of comparisons (e.g., s1 vs. s2 or s1 vs. s3). Differences can vary from the phylum to species level.
Mean comparison was performed using Fisher’s least significant difference test and the Duncan multiple range test with a significance level of p < 0.05, and the relevant graphs were drawn in Origin 9.4.
There were fewer microorganisms on the surface of the Al@TCAP, and no bacterial film was formed (Figure 1). The volcanic rocks carrying microorganisms contained many organisms that formed a bacterial film. The adsorption and desorption cumulative surface area of pores in Al@TCAP were 28.17 and 38.30 m2/g, respectively, while for volcanic rock, it became 9.59 and 18.22, respectively. The surface area indices of the Al@TCAP were higher than those of the volcanic rock.
Figure 1. SEM results for Al@TCAP and volcanic rock when loaded or unloaded with microbes. The red arrow showed loaded with microbes and bacterial film formation. The Al@TCAP surface was rough, uneven, and exhibited many granular protrusions. The surface of the granular protrusions was groove shaped, and there were pores under the granular protrusions. The surface of the volcanic rock had granular protrusions and more pores. The protrusion surface was rough and exhibited a block shape.
In the nitrogen cycle, the TN concentrations in groups C and E were lower at 48 h than that of the other groups (Figure 2A). The TN removal rate of group A was higher than that of other groups at 6–36 h and lower than that of groups C and E at 48 h. The NH3-N in each group displayed an upward trend. The concentration of NH3-N in group C was lower than that in other groups after 12 h, and the frequency of NH3-N in group D was higher than that in the other groups after 24 h (Figure 2B). The NO3–-N concentration in group A was higher than that in the other groups at 0 h, the NO3–-N concentration in group B was higher than that in group A at 6 h, and the NO3–-N concentrations were higher in groups C, D, and E at 12 h. The frequency of NO3–-N in group C was higher than that in group A at 24 h. The concentrations of NO3–-N in groups C and B were higher than that in group A at 36 h, and the concentrations of NO3–-N in the other groups were higher than that in group A at 48 h (Figure 2C). The concentration of NO2–-N in group A was low between 0 and 24 h. The concentration of NO2–-N in group A increased during 24-36 h, and the concentration of NO2–-N was higher at 36 and 48 h than that in the other groups. The NO2–-N concentrations in groups B, C, D, and E were low between 0 and 36 h, and they increased at 36-48 h (Figure 2D).
Figure 2. Measurement results of eight water quality indicators. (A–H) pictures are TN (removal rate), NH3-N, NO3–-N, NO2–-N, TP (removal rate), PO43–-P, DO, and CODMn (removal rate), respectively.
The TP removal rate was the highest at 6-24 h. The TP removal rate in group C was higher than that in group A at 36 h. The removal rates of TP in groups C and E were higher than that in group A at 48 h. The PO43–-P concentration typically exhibited an upward-decreasing trend (Figure 2E). Group A starts to decrease after a peak at 24 h, and the other groups drop after reaching a peak at 36 h. The PO43–-P concentration in group D was higher than that in group A at 0 h, and the PO43–-P concentrations in groups B, D, and E were higher than that in group A at 6 h. The PO43–-P concentrations in groups B and D were higher than that in group A at 12 h (Figure 2F). The DO concentration in group D was lower than that in other groups at 0, 6, and 24 h, and the DO concentration in group C was higher than that in other groups at 0, 6, and 48 h. At 48 h, the DO concentration in group A was lower than that in the other groups. The DO concentration of each group increased at 0-6 h, decreased at 6-12 h, and then slowly increased at 12-48 h (Figure 2G). The CODMn removal rates in groups D and E were higher than that in group A at 6 h, and the CODMn removal rate in group A was higher than that in other groups at 12 and 36 h. The CODMn removal rates were higher in groups C, D, and E than in group A at 24 and 48 h. The CODMn removal rates in groups D and E were higher than that in group A (Figure 2H). At 48 h, the removal rates of total nitrogen, total phosphorous, and Mn chemical oxygen demand in the purified materials plus the three exogenous microorganisms were 46.91, 50.93, and 65.08%, respectively.
In the nitrogen cycle, the ammonia monooxygenase (AMO) activity was higher in groups D and E than in group A at 0 h, in groups B, D, and E at 6 h than in group A, in group B at 12 h than in group A, and in group A at 24 and 36 h. Compared with other groups, group E exhibited more activity than group A at 48 h (Figure 3A). The nitrate reductase (NAR) activity was higher in group C than in group A at 0 h, and it was higher in each group that group A at 6 and 12 h. Groups B and C exhibited more NAR activity than group A at 24 h, and group B was more active than group A at 36 h. Group A exhibited more activity than the other groups at 48 h (Figure 3B). The nitrite reductase (NIR) activity was higher in groups C and E than in group A at 0 h, and group A activity was higher than that in other groups at 6-24 h, groups C, D, and E were more active than group A at 36 h, and all other groups exhibited more activity than group A at 48 h (Figure 3C).
Figure 3. Results of microbial enzyme activity determination. (A–F) pictures are AMO, NAR, NIR, AKP, OPH, and DHO, respectively. The NIR, NAR, and AMO participate in the nitrogen cycle. NIR degrades NO2–-N to N2 or NH3-N, reducing the accumulation of NO2–-N in the environment. NAR is an inducible enzyme that can directly reduce NO3–-N to NO2–-N. AMO catalyzes ammonia oxidation by helping ammonia-oxidizing bacteria, which can help ammonia-oxidizing bacteria convert NH3-N to NO2–-N. AKP can catalyze the decomposition of organic phosphorus and release orthophosphate, while OPH can decompose organic phosphorus compounds in water and reduce organic phosphorus compound pollution. DHO is an oxidoreductase that participates in the decomposition of organic matter.
It can be seen that the alkaline phosphatase (AKP) activity of group A was lower than that of the other groups at 0 h and higher than that of other groups at 6 h (Figure 3D). AKP activity was higher in group E than in group A at 12 h, groups B and C were more active than group A at 24 h, groups C and D were more active than group A at 36 h, and groups D and E were more active than group A at 48 h. The organophosphorus hydrolase (OPH) activity was higher in groups C and D than in group A at 0 h, groups B, C, and D exhibited greater OHP activity than group A at 6 h, group E activity was higher than group A at 12 h, and groups B, C, and D were more active than group A. Each group exhibited more OPH activity than group A at 36 h, and groups B, C, and D were more active than group A at 48 h (Figure 3E).
The dehydrogenase (DHO) activity was higher in groups B, D, and E than in group A at 0 h, groups D and E exhibited more DHO activity than group A at 6 h, and all other groups exhibited higher activity than group A at 12 and 24 h, and group B exhibited higher activity at 36 h. Group A was more active than other groups (except group B) at 36 and 48 h, and group activity A was higher than that of the other groups at 48 h (Figure 3F).
The 16S rRNA test results showed that Planctomycetes, Proteobacteria, and Bacteroidetes are the three primary group phyla. The predominant genera are Pirellulaceae, Polynucleobacter, and other Patescibacteria, Planctomycetes, Actinobacteria, Dependentiae, and Deinococcus-Thermus displayed significant differences between groups (Supplementary Figure 1). Results showed that from 24 h, optimization-CCS (Supplementary Figure 2) and Simpson diversity indices (Supplementary Figure 2) significantly increased, while OUT/Ace/Chao1 (Supplementary Figure 2), Shannon diversity indices (Supplementary Figure 2) decreased when compared to the controls. With the aspects of different treatments, group E decreased optimization-CCS. The maximum percentage of β-diversity variation measured by weighted UniFrac matrices in different experimental groups was 94.62% along PC1, 3.91% along PC2, and 0.51% along PC3 by PCA analysis (p < 0.05).
The relative abundances of Aerobic (Actinobacteria, Planctomycetes, Proteobacteria), Anaerobic (Bacteroidetes, Planctomycetes), Contains_Mobile_Elements (Proteobacteria), Facultatively_Anaerobic (Proteobacteria), Forms_Biofilms (Actinobacteria, Planctomycetes, Proteobacteria), Gram_Negative (Planctomycetes, Proteobacteria), Gram_Positive (Actinobacteria), Potentially_Pathogenic (Proteobacteria, TM7), and Stress_Tolerant (Proteobacteria) are revealed in Supplementary Figure 3. The branches of Actinobacteria, Planctomycetes, and Proteobacteria have been revealed as the significant biomarkers based on the total expression in all the treatment groups.
When we compared group C (named M52/53/54) with group D (named M55/56/57) at the class 2 level, the significant 17 COG categories have been enriched in the comparison between groups C and D at 24 h. The categories are ordered as amino acid transport and metabolism, carbohydrate transport and metabolism, coenzyme transport and metabolism, defense mechanisms, inorganic ion transport and metabolism, intracellular trafficking, secretion and vesicular transport, lipid transport and metabolism, secondary metabolites biosynthesis, and transport and catabolism.
In the KEGG pathway at the phylum class 1 level, environmental information processing and metabolism have been enriched. Interestingly, the significant 27 KEGG pathways in the comparison between groups C and D at 24 h, named as biosynthesis of other secondary metabolites, carbohydrate metabolism, energy metabolism, environmental adaptation, glycan biosynthesis and metabolism, and infectious diseases: bacterial, parasitic, and viral, membrane transport, metabolism of other amino acids, metabolism of terpenoids and polyketides, and xenobiotics biodegradation and metabolism.
In group C, when we compared 0 h (chosen M07 as a reference control) and 24 h (chosen M52 as the case), the significant biomarkers were Planctomycetes and Actinobacteria. Al@TCAP enhanced the expression of Pirellulaceae. In group B, when we compared 12 h (i.e., M35) and 48 h (i.e., M81), the expression of Planctomycetes and Proteobacteria increased and decreased in the volcanic rock group (Supplementary Figure 3).
In the network between the significant biomarkers in the process of water purification, the current study found the top 10 were Pirellulaceae and Polynucleobacter. Filimonas and Fluviicola are mainly involved in the degradation of high-concentration organic matter, while the relative abundance of Novosphingobium, Reyranella, Shinella, Nevskia, and Brevundimonas (both belong to Proteobacteria) was positively correlated with the content of polycyclic aromatic hydrocarbon analogs (Supplementary Figure 3). In the comparison between groups C and D, the significant KEGG pathway number of metabolism (other secondary metabolites, carbohydrate, energy, glycan, other amino acids, terpenoids, and polyketides), environmental response (xenobiotics biodegradation, adaptation), and disease prevention (infectious diseases: bacterial, parasitic, viral) are revealed in Supplementary Figure 3.
Based on the cluster images of species richness, 141 OTUs were collected. Chytridiomycota (71.51%) was the primary group phylum. Ascomycota, Basidiomycota, and Kickxellomycota displayed significant differences between the groups (Supplementary Figure 1). When assessing the samples, seven gates were detected, and there were differences between the collected samples.
Results showed from 48 h, optimization-CCS (Supplementary Figure 2) and Simpson diversity indices (Supplementary Figure 2) significantly increased, while OUT/ACE/Chao1 (Supplementary Figure 2) and Shannon diversity indices significantly increased when compared with those at 6 h. OTU, ACE, Chao1, and Shannon diversity indices at 36 and 48 h significantly decreased when compared with those at 0 h, while Simpson diversity indices significantly increased. With the aspects of different treatments, three purification material addition groups, containing groups B, C, and E decreased optimization-CCS, while the others showed no significant differences when compared to the controls.
The variation in β-diversity measured by Bray–Curtis in different experimental groups, based on an NMDS and Anosim analysis, found that S1, S2, and S3 are similar, and S4, S5, and S6 are extremely different between and within groups, indicating that the microbial communities gradually developed over time (Supplementary Table 1). There was little difference among samples. The maximum percentage of β-diversity variation measured by weighted UniFrac matrices in different experimental groups was 90.18% along PC1, 7.07% along PC2, and 0.88% along PC3 by PCA analysis (p < 0.05).
The decreased Chytridiomycetes (especially Hyaloraphidium) and increased Monoblepharidomycetes has been shown in the present study with the increasing time. Except for Basidiomycota, the branch of Aphelidiomycota, Ascomycota, Chytridiomycota, Kickxellomycota, and Rozellomycota showed as the significant biomarker between different time durations via ANOVA analysis (Figure 4). At 24 h, when we compared group C (chosen s4C1 as the reference control) and group D (chosen s4D3 as the case), the significant biomarkers belonged to Ascomycota. Kickxellomycota, Basidiomycota, and Ascomycota were the significant biomarkers between group A at 12 h (s3A2) and E at 48 h (s6E3) via the Ternary method. Compared with the S2 group, the genus Aspergillus, genus Penicillium, species Aspergillus penicillioides, and species Penicillium lemhiflumine in the S5 group were significantly reduced. Compared with the S2 group, the genus Penicillium and species Penicillium lemhiflumine in the S6 group decreased significantly (Figure 4). Compared with the S3 group, the genus Aspergillus in the S5 group was significantly reduced. Compared with the S4 group, the genus Penicillium in the S5 group decreased significantly. Compared with the S4 group, the genus Penicillium and species Penicillium lemhiflumin in the S6 group were significantly reduced. Compared with the S5 group, the genus Aspergillus in the S6 group increased significantly.
Figure 4. Fungal species-abundance clustering image at different category levels. (A), the significant difference of sample via heatmapby binary_jaccard_dm. method; (B), biomarkers via the lefse and cladogram method; (C), group ANOVA result in the phylum level, the relative abundance among the comparisons of s4C1 vs. s4D3, s3A2 vs. s6E3, while the relative abundance of the genus has also been revealed. Ascomycota (Nigrospora, Aspergillus, Cladosporium, Alternaria, Candida), Basidiomycota (Malassezia, Lyophyllum, Phallus), and Kickxellomycota (Smittium) increased. The relationship between the selected taxonomy group (abundant species, genera, classes, orders, or families) was calculated using SPSS 25.0 software.
Attapulgite and volcanic rock exhibit excellent adsorption performance and improve the environmental indicators of water quality (Fan et al., 2011; Derakhshan et al., 2016). It can be seen from the characterization experiments on the surface of the purification material that Al@TCAP has a rough and uneven surface with a large number of protruding, gully-like particles, and pores under the particles. The specific surface area is large, approximately 2.94 and 2.10 times for adsorption and desorption of the volcanic rock, but the microbial capacity on the surface is weak, and no bacterial film is formed. The study demonstrates that Al@TCAP exhibits a weak microbial loading capacity, and the Al@TCAP removal is primarily attributed to adsorption. At 48 h, the removal rates of TN, TP, and CODMn in the purified materials plus the three exogenous microorganisms were over 47%. The volcanic rock has a sufficient ability to load microorganisms, and the organisms primarily perform the removal for improved water quality.
The exogenous microorganism and activated sludge are often used for water pollution treatment, strengthening the denitrification capacity of the polluted water (Fan et al., 2020). In group E of the present study, the NO3–-N concentration increased and decreased within 6–12 h and 12–24 h, respectively, and the NO2–-N concentration continued to grow within 6–24 h, indicating that the nitrification reaction occurred after the actions of exogenous microorganisms. In the original group E, the concentration of NO3–-N decreased at 12–36 h, and the corresponding 24–48 h NAR increased. The 6–36 h NIR increased, indicating that denitrification continued to increase at 12–36 h and that nitrification and denitrification coexisted at 12–24 h (Kim et al., 2017; Zhang et al., 2020). At 12 h, the proportion of Planctomycetes increased in groups C, D, and E. In comparison, the percentage of Planctomycetes increased at 24 h in groups A and B. This shows that Al@TCAP and exogenous microorganisms accelerated the nitrification reaction and advanced the denitrification reaction (Zhang et al., 2020). In actual production, when aerating can be determined according to the NH3-N concentration of the primary precipitation unit, prolonging the nitrification reaction reduces the impact of NH3-N on the water body. The fungi detected by ITS-Seq contained Penicillium and Aspergillus, which have been proven to have PO43–-P-dissolving functions, and their PO43–-P-dissolving ability is typically more reliable than that of bacteria. Proteobacteria contain Nitrosomonas, which can oxidize ammonia (Ilgrande et al., 2018). Planctomycetes include a type of bacteria that can convert NO2–-N to NH3-N to form N2. This reaction is called anaerobic ammonium oxidation (Zheng Y. et al., 2019). The current study suggests that those bacteria play a vital role in the nitrogen cycle (Zhang et al., 2020). In addition, the correlation analysis of DO and NAR activities were significantly positively correlated (p < 0.05), indicating that increasing DO in water can increase NAR activity. Due to the decrease in DO, the AMO activity of the oxidized NH3-N decreased, and NIR reduced NO2––-N to NH3-N, which gradually increased the concentration of NH3-N in the water.
In this experiment, at 36 h, the OPH activity of each group was higher than that of the new group. Compared with other periods, the proportion of Penicillium at 36 h was significantly lower, which explains the flat TP removal rate at 24–36 h (Watanabe et al., 2010). The AKP activity and TP removal rate of group E at 48 h were higher than those of the group with the added purification materials and the exogenous microorganisms (Pundir et al., 2019; Zheng L. et al., 2019). The content of the genus Aspergillus at 48 h was higher than at 36 h, indicating that the purification materials assisted in the growth of certain exogenous microorganism environments and improved the growth of microorganisms associated with TP removal (Cheng et al., 2019).
The dehydrogenase activity of each group was higher than that of the control group at 12–24 h, and the CODMn removal rate of groups D and E with the added exogenous microorganisms was higher than that of the other groups, indicating that exogenous microorganisms can accelerate the initiation of organic matter degradation (Chen et al., 2020) and enhance the removal rate of CODMn.
In the comparison between groups C and D, the KEGG pathway of metabolism, environmental response, and disease prevention have been significantly affected, while Al@TCAP enhanced the expression of Pirellulaceae in the present study. Otherwise, Al@TCAP increased the expression of Planctomycetes and Actinobacteria. Planctomycetes were the dominant microbes (13% of the microbial community) under anaerobic conditions during the nitrogen cycle (Li et al., 2017). The relative occupied abundances of Proteobacteria offered their use in wastewater treatment, especially the bioremediation of hydrocarbon pollutants (Romero et al., 2019). The volcanic rock increased the expression of Planctomycetes, decreased the expression of Proteobacteria, and a recent study showed that volcanic rock had a retention capacity of heavy metals when combined with the use of biochar (Piscitelli et al., 2018).
Within the fungi in the present study, the purification material addition group decreased the expression of Hyaloraphidium, while no available data for Hyaloraphidium has been found. The decreased Chytridiomycetes (Hyaloraphidium) and the increased Monoblepharidomycetes were found with the increasing time, especially from 36 to 48 h. Chytridiomycetes was found to be associated with the degradation of organic matter and trace-organic contaminants (Maza-Márquez et al., 2016), the diversity indices of 16SrRNA- (24–48 h), ITS-Seq (36–48 h), and DHO of group C/D decreased at 36–48 h. The present study showed that organic matter degradation declined from 36 h. Ascomycota (Aspergillus and Candida; Assress et al., 2019) and Basidiomycota were the predominant fungal phylum during the thermophilic phase in the use of sewage sludge (Liu et al., 2019; Robledo-Mahón et al., 2020). Ascomycota, Basidiomycota, and Kickxellomycota increased in group D when compared with B at 24 h, while group E increased Ascomycota at 48 h. Actinobacteria, Proteobacteria, and Aspergillus significantly changed in the biochar applied treatments (Awasthi et al., 2017), Candida was useful for the removal of high-strength phenolic compounds (Basak et al., 2019) and similar to our study, it hinted that purification material addition may have the same effect as biochar in the wastewater treatment (Rocha et al., 2020).
The ability of Al@TCAP to load microorganisms is weak, and the water quality is predominantly purified by adsorption. The volcanic rock exhibits a strong ability to load microorganisms, and the water quality is primarily purified by microorganisms. At 48 h, the removal rates of TN, TP, and CODMn by the purification materials plus three exogenous microorganisms were 46.91, 50.93, and 65.08%, respectively. The study found that volcanic rock, Al@TCAP, and exogenous microorganisms can increase the purification effect by increasing the OPH, DHO, and NIR activities in a specific period. The combination of the composite purification material and three types of exogenous microorganisms can increase AKP activity and improve the TP purification effect at 48 h. In the experiment, Al@TCAP and exogenous microorganisms promoted the growth of Planctomycetes and accelerated denitrification. The purification material also provided a growth environment for microorganisms with phosphorus-decomposing functions and enhanced TP removal.
The data presented in the study is deposited in the SRA repository (https://www.ncbi.nlm.nih.gov/ PRJNA392982).
YZ, JY, and GX conceived and designed the experiments. YZ and YW analyzed the data. XY and JG contributed reagents, materials, and analysis tools. YZ contributed to the figures preparation. YZ prepared and wrote the manuscript. All authors reviewed the manuscript.
The work was supported by Central Public-Interest Scientific Institution Basal Research Fund, Freshwater Fisheries Research Center, CAFS (No. 2021JBFM19), China Agriculture Research System of MOF and MARA (No. CARS-46), and the open project of the Agriculture Ministry Key Laboratory of Healthy Freshwater Aquaculture (ZJK202102).
We thank Teresa (English editors both worked for editage) for providing grammar and spelling checks of the manuscript.
The authors declare that the research was conducted in the absence of any commercial or financial relationships that could be construed as a potential conflict of interest.
All claims expressed in this article are solely those of the authors and do not necessarily represent those of their affiliated organizations, or those of the publisher, the editors and the reviewers. Any product that may be evaluated in this article, or claim that may be made by its manufacturer, is not guaranteed or endorsed by the publisher.
The Supplementary Material for this article can be found online at: https://www.frontiersin.org/articles/10.3389/fmicb.2022.944366/full#supplementary-material
Alloul, A., Cerruti, M., Adamczyk, D., Weissbrodt, D. G., and Vlaeminck, S. E. (2021). Operational strategies to selectively produce purple bacteria for microbial protein in raceway reactors. Environ. Sci. Technol. 55, 8278–8286. doi: 10.1021/acs.est.0c08204
Assress, H. A., Selvarajan, R., Nyoni, H., Ntushelo, K., Mamba, B. B., and Msagati, T. A. M. (2019). Diversity, co-occurrence and implications of fungal communities in wastewater treatment plants. Sci. Rep. 9:14056. doi: 10.1038/s41598-019-50624-z
Awasthi, M. K., Li, J., Kumar, S., Awasthi, S. K., Wang, Q., Chen, H., et al. (2017). Effects of biochar amendment on bacterial and fungal diversity for co-composting of gelatin industry sludge mixed with organic fraction of municipal solid waste. Bioresour. Technol. 246, 214–223. doi: 10.1016/j.biortech.2017.07.068
Basak, B., Jeon, B. H., Kurade, M. B., Saratale, G. D., Bhunia, B., Chatterjee, P. K., et al. (2019). Biodegradation of high concentration phenol using sugarcane bagasse immobilized Candida tropicalis PHB5 in a packed-bed column reactor. Ecotoxicol. Environ. Saf. 180, 317–325. doi: 10.1016/j.ecoenv.2019.05.020
Chen, Z., Li, D., and Wen, Q. (2020). Investigation of hydrolysis acidification process during anaerobic treatment of coal gasification wastewater (CGW): Evolution of dissolved organic matter and biotoxicity. Sci. Total Environ. 723:137995. doi: 10.1016/j.scitotenv.2020.137995
Cheng, Z., Feng, K., Xu, D., Kennes, C., Chen, J., Chen, D., et al. (2019). An innovative nutritional slow-release packing material with functional microorganisms for biofiltration: Characterization and performance evaluation. J. Hazard. Mater. 366, 16–26. doi: 10.1016/j.jhazmat.2018.11.070
de Anda, J., Gradilla-Hernández, M. S., Díaz-Torres, O., de Jesús Díaz-Torres, J., and de la Torre-Castro, L. M. (2019). Assessment of heavy metals in the surface sediments and sediment-water interface of Lake Cajititlán, Mexico. Environ. Monit. Assess. 191:396. doi: 10.1007/s10661-019-7524-y
Derakhshan, Z., Ehrampoush, M. H., Mahvi, A. H., Faramarzian, M., Mokhtari, M., and Mazloomi, S. M. (2016). Evaluation of volcanic pumice stone as media in fixed bed sequence batch reactor for atrazine removal from aquatic environments. Water Sci. Technol. 74, 2569–2581. doi: 10.2166/wst.2016.424
Fan, F., Xu, R., Wang, D., and Meng, F. (2020). Application of activated sludge for odor control in wastewater treatment plants: Approaches, advances and outlooks. Water Res. 181:115915. doi: 10.1016/j.watres.2020.115915
Fan, L., Wu, W., Qiu, L., Song, C., Meng, S., Zheng, Y., et al. (2018). Methanogenic community compositions in surface sediment of freshwater aquaculture ponds and the influencing factors. Antonie Van Leeuwenhoek 111, 115–124. doi: 10.1007/s10482-017-0932-5
Fan, Q., Li, P., Chen, Y., and Wu, W. (2011). Preparation and application of attapulgite/iron oxide magnetic composites for the removal of U(VI) from aqueous solution. J. Hazard. Mater. 192, 1851–1859. doi: 10.1016/j.jhazmat.2011.07.022
Franchino, M., Tigini, V., Varese, G. C., Mussat Sartor, R., and Bona, F. (2016). Microalgae treatment removes nutrients and reduces ecotoxicity of diluted piggery digestate. Sci. Total Environ. 569-570, 40–45. doi: 10.1016/j.scitotenv.2016.06.100
Ilgrande, C., Leroy, B., Wattiez, R., Vlaeminck, S. E., Boon, N., and Clauwaert, P. (2018). Metabolic and proteomic responses to salinity in synthetic nitrifying communities of Nitrosomonas spp. and Nitrobacter spp. Front. Microbiol. 9:2914. doi: 10.3389/fmicb.2018.02914
Kim, H. T., Kim, S., Na, B. K., Chung, J., Hwang, E., and Hwang, K. Y. (2017). Structural insights into the dimer-tetramer transition of FabI from Bacillus anthracis. Biochem. Biophys. Res. Commun. 493, 28–33. doi: 10.1016/j.bbrc.2017.09.084
Kuebutornye, F. K. A., Abarike, E. D., and Lu, Y. (2019). A review on the application of Bacillus as probiotics in aquaculture. Fish Shellfish Immunol. 87, 820–828. doi: 10.1016/j.fsi.2019.02.010
Kuśnierz, M., Domańska, M., Hamal, K., and Pera, A. (2022). Application of integrated fixed-film activated sludge in a conventional wastewater treatment plant. Int. J. Environ. Res. Public Health 19:5985. doi: 10.3390/ijerph19105985
Li, K., Fang, F., Wang, H., Chen, Y., Guo, J., Wang, X., et al. (2017). Pathways of N removal and N2O emission from a one-stage autotrophic N removal process under anaerobic conditions. Sci. Rep. 7:42072. doi: 10.1038/srep42072
Liu, T., He, J., Cui, C., and Tang, J. (2019). Exploiting community structure, interactions and functional characteristics of fungi involved in the biodrying of storage sludge and beer lees. J. Environ. Manage. 232, 321–329. doi: 10.1016/j.jenvman.2018.11.089
Maza-Márquez, P., Vilchez-Vargas, R., Kerckhof, F. M., Aranda, E., González-López, J., and Rodelas, B. (2016). Community structure, population dynamics and diversity of fungi in a full-scale membrane bioreactor (MBR) for urban wastewater treatment. Water Res. 105, 507–519. doi: 10.1016/j.watres.2016.09.021
Murray, G. P., Costilow, R., Nester, E., Krieg, N., and Phillips, G. (eds). (1981). Manual of methods for general bacteriology. Washington, DC: American Society for Microbiology.
Otte, S., Grobben, N. G., Robertson, L. A., Jetten, M. S., and Kuenen, J. G. (1996). Nitrous oxide production by Alcaligenes faecalis under transient and dynamic aerobic and anaerobic conditions. Appl. Environ. Microbiol. 62, 2421–2426. doi: 10.1128/aem.62.7.2421-2426.1996
Piscitelli, L., Rivier, P. A., Mondelli, D., Miano, T., and Joner, E. J. (2018). Assessment of addition of biochar to filtering mixtures for potential water pollutant removal. Environ. Sci. Pollut. Res. Int. 25, 2167–2174. doi: 10.1007/s11356-017-0650-6
Pundir, C. S., Malik, A., and Preety. (2019). Bio-sensing of organophosphorus pesticides: A review. Biosens. Bioelectron. 140:111348. doi: 10.1016/j.bios.2019.111348
Ren, W., Wu, H., Guo, C., Xue, B., Long, H., Zhang, X., et al. (2021). Multi-strain tropical Bacillus spp. as a potential probiotic biocontrol agent for large-scale enhancement of mariculture water quality. Front. Microbiol. 12:699378. doi: 10.3389/fmicb.2021.699378
Robledo-Mahón, Gómez-Silván, C., Andersen, G. L., Calvo, C., and Aranda, E. (2020). Assessment of bacterial and fungal communities in a full-scale thermophilic sewage sludge composting pile under a semipermeable cover. Bioresour. Technol. 298:122550. doi: 10.1016/j.biortech.2019.122550
Rocha, L. S., Pereira, D., Sousa, É, Otero, M., Esteves, V. I., and Calisto, V. (2020). Recent advances on the development and application of magnetic activated carbon and char for the removal of pharmaceutical compounds from waters: A review. Sci. Total Environ. 718:137272. doi: 10.1016/j.scitotenv.2020.137272
Romero, F., Sabater, S., Font, C., Balcázar, J. L., and Acuña, V. (2019). Desiccation events change the microbial response to gradients of wastewater effluent pollution. Water Res. 151, 371–380. doi: 10.1016/j.watres.2018.12.028
Sangnoi, Y., Chankaew, S., and O-Thong, S. (2017). Indigenous Halomonas spp., the potential nitrifying bacteria for saline ammonium waste water treatment. Pak. J. Biol. Sci. 20, 52–58. doi: 10.3923/pjbs.2017.52.58
Santorio, S., Couto, A. T., Amorim, C. L., Val Del Rio, A., Arregui, L., Mosquera-Corral, A., et al. (2021). Sequencing versus continuous granular sludge reactor for the treatment of freshwater aquaculture effluents. Water Res. 201:117293. doi: 10.1016/j.watres.2021.117293
Santos, J. F., Soares, K. L., Assis, C. R., Guerra, C. A., Lemos, D. Jr., Carvalho, L. B., et al. (2016). Digestive enzyme activity in the intestine of Nile tilapia (Oreochromis niloticus L.) under pond and cage farming systems. Fish Physiol. Biochem. 42, 1259–1274. doi: 10.1007/s10695-016-0215-5
Serpa, D., Pousão-Ferreira, P., Caetano, M., Cancela da Fonseca, L., Dinis, M. T., and Duarte, P. (2013). A coupled biogeochemical-Dynamic Energy Budget model as a tool for managing fish production ponds. Sci. Total Environ. 463-464, 861–874. doi: 10.1016/j.scitotenv.2013.06.090
State EPA of China (2002). Monitoring and determination methods for water and wastewater, 4th Edn. Beijing: China Environmental Science Press.
Wan, Z., Zhang, Y., Lu, S., Zhang, X., Liu, X., and Yang, Y. (2020). Effect of packing substrates on the purification of municipal wastewater treatment plant effluent. Environ. Sci. Pollut. Res. Int. 27, 15259–15266. doi: 10.1007/s11356-020-08068-w
Watanabe, T., Tanaka, M., Masaki, K., Fujii, T., and Iefuji, H. (2010). Decolorization and treatment of Kokuto-shochu distillery wastewater by the combination treatment involving biodecolorization and biotreatment by Penicillium oxalicum d, physical decolorization by ozonation and treatment by activated sludge. Biodegradation 21, 1067–1075. doi: 10.1007/s10532-010-9367-7
Yin, H., Wang, J., Zhang, R., and Tang, W. (2019). Performance of physical and chemical methods in the co-reduction of internal phosphorus and nitrogen loading from the sediment of a black odorous river. Sci. Total Environ. 663, 68–77. doi: 10.1016/j.scitotenv.2019.01.326
Yin, T., Zheng, Y., Liu, T., Wang, X., Gao, J., Nie, Z., et al. (2022). Study on water purification effect and operation parameters of various units of wastewater circulation. Water 14:1743. doi: 10.3390/w14111743
Zhang, Z., Chen, H., Mu, X., Zhang, S., Pang, S., and Ohore, O. E. (2020). Nitrate application decreased microbial biodiversity but stimulated denitrifiers in epiphytic biofilms on Ceratophyllum demersum. J. Environ. Manage. 269:110814. doi: 10.1016/j.jenvman.2020.110814
Zheng, L., Ren, M., Xie, E., Ding, A., Liu, Y., Deng, S., et al. (2019). Roles of phosphorus sources in microbial community assembly for the removal of organic matters and ammonia in activated sludge. Front. Microbiol. 10:1023. doi: 10.3389/fmicb.2019.01023
Zheng, Y., Hou, L., Liu, M., and Yin, G. (2019). Dynamics and environmental importance of anaerobic ammonium oxidation (anammox) bacteria in urban river networks. Environ. Pollut. 254(Pt A):112998. doi: 10.1016/j.envpol.2019.112998
Zheng, Y., Wu, W., Hu, G., Qiu, L., Meng, S., Song, C., et al. (2018). Gut microbiota analysis of juvenile genetically improved farmed tilapia (Oreochromis niloticus) by dietary supplementation of different resveratrol concentrations. Fish Shellfish Immunol. 77, 200–207. doi: 10.1016/j.fsi.2018.03.040
Keywords: purification materials, exogenous microorganisms, water purification enhancement, microbial enzyme activity, metagenome
Citation: Zheng Y, Wang Y, Yang X, Gao J, Xu G and Yuan J (2022) Effective mechanisms of water purification for nitrogen-modified attapulgite, volcanic rock, and combined exogenous microorganisms. Front. Microbiol. 13:944366. doi: 10.3389/fmicb.2022.944366
Received: 25 May 2022; Accepted: 15 July 2022;
Published: 10 August 2022.
Edited by:
You-Peng Chen, Chongqing University, ChinaCopyright © 2022 Zheng, Wang, Yang, Gao, Xu and Yuan. This is an open-access article distributed under the terms of the Creative Commons Attribution License (CC BY). The use, distribution or reproduction in other forums is permitted, provided the original author(s) and the copyright owner(s) are credited and that the original publication in this journal is cited, in accordance with accepted academic practice. No use, distribution or reproduction is permitted which does not comply with these terms.
*Correspondence: Gangchun Xu, eHVnY0BmZnJjLmNu; Julin Yuan, eXVhbmp1bGluMTk4MkAxNjMuY29t
Disclaimer: All claims expressed in this article are solely those of the authors and do not necessarily represent those of their affiliated organizations, or those of the publisher, the editors and the reviewers. Any product that may be evaluated in this article or claim that may be made by its manufacturer is not guaranteed or endorsed by the publisher.
Research integrity at Frontiers
Learn more about the work of our research integrity team to safeguard the quality of each article we publish.