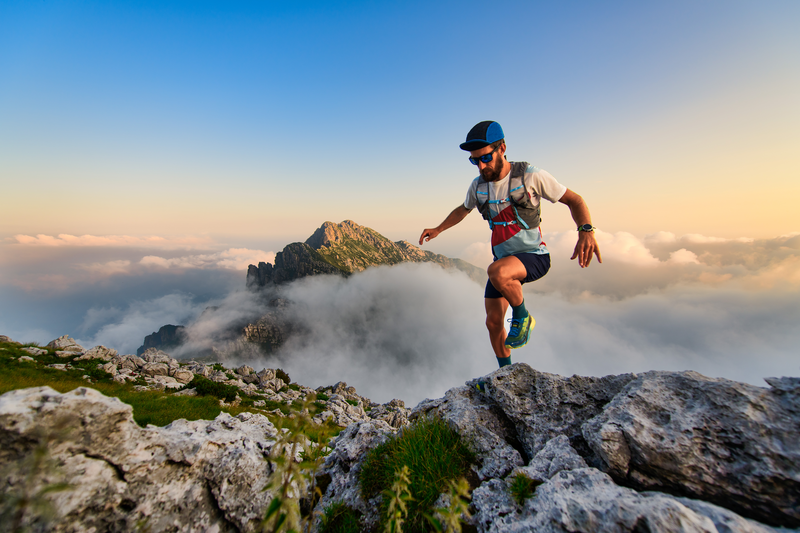
94% of researchers rate our articles as excellent or good
Learn more about the work of our research integrity team to safeguard the quality of each article we publish.
Find out more
ORIGINAL RESEARCH article
Front. Microbiol. , 14 July 2022
Sec. Infectious Agents and Disease
Volume 13 - 2022 | https://doi.org/10.3389/fmicb.2022.943441
While 16S rRNA PCR-Sanger sequencing has paved the way for the diagnosis of culture-negative bacterial infections, it does not provide the composition of polymicrobial infections. We aimed to evaluate the performance of the Nanopore-based 16S rRNA metagenomic approach, using both partial and full-length amplification of the gene, and to explore its feasibility and suitability as a routine diagnostic tool for bacterial infections in a clinical laboratory. Thirty-one culture-negative clinical samples from mono- and polymicrobial infections based on Sanger-sequencing results were sequenced on MinION using both the in-house partial amplification and the Nanopore dedicated kit for the full-length amplification of the 16S rRNA gene. Contamination, background noise definition, bacterial identification, and time-effectiveness issues were addressed. Cost optimization was also investigated with the miniaturized version of the flow cell (Flongle). The partial 16S approach had a greater sensitivity compared to the full-length kit that detected bacterial DNA in only 24/31 (77.4%) samples. Setting a threshold of 1% of total reads overcame the background noise issue and eased the interpretation of clinical samples. Results were obtained within 1 day, discriminated polymicrobial samples, and gave accurate bacterial identifications compared to Sanger-based results. We also found that multiplexing and using Flongle flow cells was a cost-effective option. The results confirm that Nanopore technology is user-friendly as well as cost- and time-effective. They also indicate that 16S rRNA targeted metagenomics is a suitable approach to be implemented for the routine diagnosis of culture-negative samples in clinical laboratories.
Rapid and accurate identification of the etiological agent of infection is the cornerstone of appropriate antimicrobial therapy and its successful management. While bacterial culture is the gold standard for microbiological diagnosis, it can be undermined and remain sterile in case of antimicrobial treatment prior to sampling, an infection caused by fastidious agents, or other viable but non-culturable (VBNC) microorganisms (Fenollar and Raoult, 2004; Laupland and Valiquette, 2013). Moreover, the typical turnaround time of 24–48 h for cultures can be extended to 7–14 days when slow-growing agents are involved. Molecular microbiology has helped overcome such limitations by providing bacterial identification in <24 h, enabling early-targeted therapy (Fenollar and Raoult, 2004; Renvoisé et al., 2013; Trotter et al., 2019). Specifically, 16S rRNA PCR has high sensitivity and specificity and is proven to be a powerful strategy for bacterial infection diagnosis in case of the absence of etiological orientation by broad-range DNA amplification (Fenollar et al., 2006; Renvoisé et al., 2013). The amplification of the 16S rRNA gene can cover all or some of the nine hypervariable regions scattered among highly conserved sequences. Ensuing sequencing of the amplicons and its comparison to nucleotide database allows assignment to a taxum. However, molecular diagnosis by conventional Sanger sequencing of 16S rRNA amplicons is hampered in case of a polybacterial infection. Metagenomic next-generation sequencing from biological samples has paved the way for a paradigm shift in microbiological diagnosis without a priori etiological assumption (Goodwin et al., 2016; Parize et al., 2017). This approach overcomes the shortcomings of both culture and PCR, by combining speed with comprehensive coverage of all the different microorganisms present in the sample. Nonetheless, the cost, complexity, and time needed for sample preparation, along with the bioinformatics, remained a pitfall in the deployment of such technology on a routine basis (Goodwin et al., 2016). Nanopore sequencing technology allows rapid and user-friendly library preparation, real-time data acquisition, and de-skilled and turnkey data analysis through an online platform with dedicated pipelines (Goodwin et al., 2016; Kai et al., 2019; Moon et al., 2019). It can be used either with regular flow cells or with the miniaturized cheaper version, the Flongle. Multiple studies have evaluated the gain in the information of both the 16S rRNA targeted and non-targeted metagenomic approaches, specifically in high pathogen load samples, such as respiratory infections, and its concordance with culture-positive samples (Mitsuhashi et al., 2017; Moon et al., 2017; Ashikawa et al., 2018; Charalampous et al., 2019; Dyrhovden et al., 2019; Hong et al., 2019; Kai et al., 2019; Yang et al., 2019; Chan et al., 2020; Leggett et al., 2020). However, little is known about the performance of the 16S rRNA-targeted approach on negative-culture samples and therefore no diagnosis (Moon et al., 2017, 2019; Cheng et al., 2018; Kai et al., 2019; Wang et al., 2020). Moreover, several issues remain to be addressed such as the risk of contamination, dealing with background noise, bacterial identification accuracy, and ensuing clinical-biological interpretation (Street et al., 2017; Sanderson et al., 2018).
Therefore, we aimed to evaluate the use of 16S rRNA Nanopore metagenomics as a routine diagnostic tool in negative-culture bacterial infections. We first addressed the risk of contamination, the definition of background noise, and bacterial identification accuracy. Performance was then compared between the in-house partial 16S rRNA PCR amplification and the Nanopore full-length 16S rRNA metagenomic kit; time-effectiveness and cost optimization were also evaluated.
Upon clinical suspicion of bacterial infection, amplification and Sanger-based sequencing of a portion of the 16S rRNA gene is routinely performed in our laboratory on culture-negative difficult-to-obtain samples such as bone and joint specimens, cardiac valve, brain abscesses, etc., as previously described (Gauduchon et al., 2003). Around 400 culture-negative and 16S rRNA, PCR-positive samples are obtained annually, of which 25% lead to unsuccessful Sanger sequencing. In the present study, we retrospectively searched the laboratory database for culture-negative and 16S rRNA PCR-positive samples with either a single DNA sequence or an uninterpretable polybacterial pattern chromatogram obtained from patients admitted between January and July 2019. The sample localisation and basic clinical context that came with the bacteriology analysis prescription were collected when available.
Remnant DNA extracts of the included samples were retrieved from −20°C storage in April–June 2020. Nanopore metagenomic analysis was performed using PCR products from both the V6/V8 region and full-length amplification of the 16S rRNA gene. Briefly, the in-house approach consists of the amplification of the V6/V8 portion of the 16S rRNA gene followed by barcoding of the amplicons with a dedicated Nanopore kit, while the full-length protocol consists of a one-step amplification and barcoding with another dedicated Nanopore kit (Figure 1).
Figure 1. Study design. On a routine basis, culture-negative clinical samples for which infection is suspected are sent to the molecular diagnosis platform for an in-house amplification of a portion of the 16S rRNA gene followed by Sanger-based sequencing. In the present study, DNA extracts were re-tested for (i) partial and, (ii) full-length 16S rRNA metagenomic analysis with Nanopore technology.
In-house partial (480 bp) 16S rRNA PCR was performed using the 91E and 13BS 5′-phosphorylated primers, as previously described (Gauduchon et al., 2003), and run for 40 cycles. Libraries were then prepared using the PCR Barcoding kit (SQK-PBK004; Oxford Nanopore Technologies, Oxford, UK) according to the manufacturer's protocol (version PBK_9073_v1_revF_23May2018) optimized as follows: phosphorylated 16S amplicons were directly purified using solid-phase reversible immobilization (SPRI) beads (NucleoMag NGS Clean-up and size selected, Macherey-Nagel, Hoerdt, France; 1.2×, 5 min), followed by PCR adapter ligation (Blunt/TA Ligase Master Mix; New England Biolabs, Evry, France), and an additional SPRI bead purification (1×, 5 min). After the barcoding PCR (LongAmp Taq 2× Master Mix, New England Biolabs), a final SPRI bead purification was performed (2×, 10 min).
Samples were prepared using the 16S Barcoding kit (SQK-RAB204; Oxford Nanopore Technologies) according to the manufacturer's protocol (version RAB_9053_v1_revL_14Aug19) except that the PCR program was changed to a run of 45 cycles instead of 25 to increase its sensitivity.
After the quantification of purified DNA barcoded amplicons (Qubit dsDNA HS Assay kit, Thermo Fischer Scientific, Illkirch-Graffenstaden, France), 60–80 fmol of sequencing library DNA were loaded into a regular flow cell (FLO-MIN 106D R9.4.1, Oxford Nanopore Technologies) or 20 fmol into its smaller version, the Flongle flow cell (FLO-FLG 001; Oxford Nanopore Technologies). Each sequencing run included a negative control consisting of PCR-grade water that was introduced at the 16S rRNA amplification step and underwent the full process.
Sequencing runs on the MinION device were driven by the MinKNOW software (version 3.6.5, Oxford Nanopore Technologies) to allow raw data acquisition and live base calling. Sequencing FASTQ reads were analyzed in real-time using the cloud-based platform EPI2ME Agent (v2020.2.10; Metrichor, Oxford, UK) using the 16S workflow. This turnkey tool includes quality-check, demultiplexing, primary and secondary analyses to obtain an identification of bacteria and archaea and an NCBI-based taxonomy tree for each sample.
The total amount of reagents required for one run was calculated in euros for each protocol and for both the regular flow cell and the Flongle and divided by the number of clinical samples tested in the associated run. The duration of each approach was estimated step-by-step based on observed times. Labour cost was not included since it can be very variable between countries. Cost-optimized runs on Flongle were performed with clinical samples 1–5.
This project was conducted according to the French law in force at the time of the study and ethical principles outlined in the Declaration of Helsinki. It obtained approval by the institutional ethics committee Hospices Civils de Lyon—CNIL (number 20-265).
The 31 clinical samples originated from bone and joint specimens (bone, n = 10; tissue, n = 4; joint fluid, n = 2; reaming product, n = 1; abscess, n =1), pleural aspirates (n = 3), aqueous humour (n = 2), cardiac valves (n = 2), abscesses (brain, n = 1; liver, n = 1; spleen, n = 1), and tissue biopsies (vascular, n = 1; soft tissue, n = 2).
Using the partial 16S approach with regular flow cells, the 31 clinical samples were sequenced in three consecutive runs, in which negative controls yielded 1–15 bacterial reads, accounting for <0.001–0.2% of the total number of reads in a run. None of these bacterial reads met the predominant bacteria detected in the samples of the associated run, ruling out the hypothesis of cross-contamination of samples or reagent contamination. Bacterial reads from the clinical samples that were present in a lower number than in the associated negative control were not taken into account for further analysis.
We used the results of the Sanger-based monobacterial infections (samples 1–10) as an estimate of background signals. Among them, nine [samples 1 (Figure 2A), 2, and 4–10] revealed the presence of one predominant bacterium; corresponding reads accounted for 97.05–99.65% of all reads (Supplementary Table 1). For each of these nine samples, the rest of the reads were assigned to a mean of 45 different bacteria (range: 6–96), with an infinitesimal (< 0.01–0.97%) presence of each, corresponding to an irrelevant signal phenomenon. Setting a threshold value of at least 1% of all reads defined the background noise. The last sample (number 3) had a first-ranked bacterium accounting for 60.28% of all reads, followed by five other bacteria above the 1% abundance cut-off, which revealed its polybacterial nature (Figure 2B).
Figure 2. The Bacterial Composition of Clinical Samples Is Based on Nanopore Technology. Examples of Partial 16S Approach Results From Sanger-Based Mono- and Polymicrobial Clinical Samples. An Abundance Histogram Represents the Proportion of Reads Assigned to up to 10 Predominant Bacteria. The Dotted Line Displays the 1% Abundance cut-off. (A) The Presence of one Single Bacterium Above the 1% cut-off, Followed by a Steep Fall Distribution of Other Detected Bacteria, Concluded to a Monomicrobial Sample With Some Background Noise. (B) The Presence of More Than one Bacterium Above the 1% cut-off Concluded to a Polymicrobial Sample, Along With Some Background Noise. (C) Sample 15 Displayed the Presence of 2 Bacteria Above the 1% cut-off, While Their Respective Abundances Were Greatly Different.
Of the 21 samples with a Sanger polybacterial pattern chromatogram, 4 (samples 11, 13, 14, and 16) revealed the presence of the first-ranked bacterium with an abundance of 96.50–99.26% followed by multiple bacteria with an abundance below the 1% threshold (0.2–0.9%; Supplementary Table 1) and were thus considered monomicrobial. Two other samples (samples 12 and 15) had a predominant bacterium with an abundance of 97.56 and 97.08%, respectively, followed by a second-ranked bacterium whose abundance was considerably lower (1.19 and 1.79%, respectively), while just above the 1% cut-off value (Supplementary Table 1, Figure 2C). The remaining 15 samples revealed the presence of at least three bacteria above the determined cut-off and were then categorized as polymicrobial samples (Supplementary Table 1). Noticeably, in sample 31 (pleural aspirate), Parvimonas micra and Mogibacterium diversum were considered as background noise due to a <1% abundance. Conversely, in sample 22 (aortic valve) six bacteria (Cutibacterium acnes, Enterobacter cloacae, Moraxella spp., S. mitis/oralis, Stenotrophomonas pavanii, and Chryseobacterium spp.) were >1% and considered notable.
All samples determined to be monomicrobial by Sanger sequencing were also determined to be so by Nanopore sequencing using the in-house partial 16S approach (Table 1). Regarding the 21 Sanger-based polymicrobial samples, Nanopore technology, and the partial 16S approach found that four of them were monomicrobial (samples 11, 13, 14, and 16) including Streptococcus dysgalactiae in a bone and joint infection (sample 11) and Raoultella spp. in an endophtalmitis (sample 16). Among the 17 remaining samples that were categorized as polymicrobial, Nanopore sequencing allowed the identification of the composing bacteria; for example, Porphyromonas gingivalis, Fusobacterium nucleatum, Tannerella forsythia, Filifactor alocis, and Staphylococcus aureus/simiae/haemolyticus in an infected mandible fragment (sample 29). Likewise, sample 31, a pleural aspirate, was found to be composed of Fusobaterium nucleatum, Treponema lecithinolyticum, Porphyromonas gingivalis, and Tannerella forsythia by the in-house partial 16S Nanopore approach, suggesting oropharyngeal flora; and sample 28, from a brain abscess, led to the identification of the Schaalia meyeri, Fusobacterium nucleatum, Campylobacter rectus, Neisseria artica, Kingella denitrificans, and Actinomyces israelii (Supplementary Table 1).
Table 1. Characteristics and bacterial identifications based on Sanger sequencing, full-length 16S rRNA Nanopore sequencing, and in-house partial 16S rRNA Nanopore sequencing.
Compared to the in-house partial 16S protocol, the full-length 16S protocol allowed both a shorter sample preparation time (5 h 30 min vs. 6 h) and a shorter hands-on time (1 h 45 min vs. 2 h 45 min; Figure 3).
Regarding sensitivity, the use of the full-length 16S rRNA barcoding kit allowed DNA amplification in only 24 of the 31 clinical samples; the remaining seven samples (samples 3–5, 11, 15, 16, and 19) did not yield a sufficient number of amplicons to be sequenced and further analyzed (Table 1). Of the positive samples, nine were found monomicrobial and with the same bacterial identification between the two approaches (Table 1). Noticeably, sample 12 was detected as monomicrobial with Streptococcus agalactiae using the full-length 16S protocol, while the partial 16S approach concluded the presence of both Streptococcus agalactiae and Porphyromonas endodontalis. The remaining 14 positive samples were categorized as polymicrobial with the same predominant bacteria using both the partial and full-length 16S protocol, even though not systematically ranked in the same order. Only samples 23 and 26 led to discordant bacterial identifications between the two approaches, with no overlap of the top-ranked bacteria (Table 1). Of note, the amplification signals were very weak, and the sequencing run yielded a majority of unclassified short fragments, probably primer dimers, leading to a difficult interpretation; these two observations strongly support the lack of clinical relevance.
Per sample cost was 106.1€ for a 12-plex regular flow cell (Figure 3). In order to improve cost-effectiveness, a switch to the miniaturized flow cell (Flongle) and multiplexing was considered. Multiplexing up to five samples (samples 1–5) plus a negative control was successful, yielding the same results for each sample (Table 1). This allowed dropping the cost to 48.2€/sample when switching to a 6-plex Flongle flow cell (Figure 3).
We assessed herein the performance and issues of a targeted 16S rRNA metagenomic approach using Nanopore sequencing on culture-negative mono- and polybacterial clinical samples, and compared the in-house 16S partial amplification with the full-length 16S dedicated Nanopore kit. The cost was also evaluated in order to implement the technology as a routine diagnostic procedure.
Addressing the widely discussed issue of background noise (Street et al., 2017; Sanderson et al., 2018; Charalampous et al., 2019; Yang et al., 2019; Chan et al., 2020), we found herein that setting a cut-off of 1% classified reads improved the accuracy of results, in line with many published studies (Sanderson et al., 2018; Charalampous et al., 2019; Yang et al., 2019; Chan et al., 2020). Nonetheless, using this cut-off in sample 31, collected from pleuropneumonia, led to categorising some bacteria as background noise while these are well-known oral flora bacteria (Nakazawa et al., 2002; Heintz et al., 2019). Conversely, the analysis of sample 22 (aortic valve in a context of infective endocarditis) concluded with the notable presence of six bacteria including environmental and oral flora bacteria, questioning their relevance as etiological agents. This highlights the challenge of identifying the true infective pathogen and the need for expertise by a skilled professional, on top of standard cut-offs.
Regarding identification accuracy, we confirmed the agreement between Nanopore and Sanger-based sequencing, which is in line with previous studies (Moon et al., 2017; Ashikawa et al., 2018; Cheng et al., 2018). The well-known major drawback of 16S rRNA gene sequencing relies on sequence similarities inducing poor discriminatory power at the species-level, and even at the genus-level for some bacterial clades. Jonhson et al. have shown that taxonomic resolution depends on the length of the analyzed 16S rRNA gene fragment and the chosen sub-region (Johnson et al., 2019). We here found a satisfactory balance between sensitivity of detection and accuracy of bacterial identification. Nonetheless, it should be reminded that, although often used for species-level identification (Yang et al., 2019; Chan et al., 2020), the Epi2Me pipeline was designed for genus-level identification only (manufacturer's recommendations) and should therefore be used with caution otherwise.
Cost is a major issue in metagenomics; we addressed this herein and found that Nanopore sequencing was a competitive option; the per-sample cost was 69.6€ for a 12-plex MinION regular flow-cell run and 48.2€ for a 6-plex Flongle run. In addition, we compared both partial and full-length 16S approaches and found that the latter offered a more user-friendly library preparation protocol with reduced hands-on time. However, the substantially greater sensitivity of the in-house partial 16S rRNA amplification, probably linked to the amplification of a shorter DNA fragment, prompted us to adopt the in-house protocol. The development of a kit using the partial 16S rRNA amplification could lead to a user-friendly and sensitive system.
Taken together, and from a clinical application point of view, herein, Nanopore sequencing allowed the identification of the bacteria present in both monomicrobial and polymicrobial samples, resolving the dead-end microbiological diagnoses. Bacterial identifications were consistent with well-known aetiologies (Plainvert et al., 2012; Selton-Suty et al., 2012; Vieira et al., 2015; Dyrhovden et al., 2019) but also scarcely reported aetiologies (Martiny et al., 2017), opening the way for the recognition of new aetiologies. In conclusion, Nanopore 16S rRNA targeted metagenomics appeared as a user-friendly, de-skilled, cost- and time-effective tool for clinical laboratories to ensure the routine diagnosis of culture-negative samples. This study paves the way for further prospective studies that will explore the clinical impact of this approach used in routine practise.
The datasets presented in this study can be found in online repositories. The names of the repository/repositories and accession number(s) can be found below: https://www.ebi.ac.uk/ena, PRJEB41337.
This project was conducted according to the French law in force at the time of the study and ethical principles outlined in the Declaration of Helsinki. It obtained approval by the institutional Ethics Committee (number 20-265). To be included in this research the patients gave a “non-opposition” form in line with French regulation.
CB was given the grant, designed and performed the study, and wrote the manuscript. CG helped design the study, trained CB in the Nanopore technology, provided technical expertise, and helped write the manuscript. YB performed part of the analyses and reviewed the manuscript. TG, HS, and OD helped designing the study and reviewing the manuscript. FL helped to obtain the grant, designing the study, and reviewing the manuscript. FV wrote the manuscript and helped to design the study. FV and FL gave medical input and expertise on the strategy of Nanopore technology development on a routine basis. All authors contributed to the article and approved the submitted version.
This work was supported by the Hospices Civils de Lyon with the Grant Innovation Award 2019 although funders had no role in study design, data collection, and interpretation.
The authors declare that the research was conducted in the absence of any commercial or financial relationships that could be construed as a potential conflict of interest.
All claims expressed in this article are solely those of the authors and do not necessarily represent those of their affiliated organizations, or those of the publisher, the editors and the reviewers. Any product that may be evaluated in this article, or claim that may be made by its manufacturer, is not guaranteed or endorsed by the publisher.
We are grateful to Philip Robinson, from the Clinical Research Department of the Hospices Civils de Lyon, for their help in manuscript preparation. We are thankful to Caroline Charre, from the department of virology, for our fruitful discussion on technical aspects.
The Supplementary Material for this article can be found online at: https://www.frontiersin.org/articles/10.3389/fmicb.2022.943441/full#supplementary-material
Ashikawa, S., Tarumoto, N., Imai, K., Sakai, J., Kodana, M., Kawamura, T., et al. (2018). Rapid identification of pathogens from positive blood culture bottles with the MinION nanopore sequencer. J. Med. Microbiol. 67, 1589–1595. doi: 10.1099/jmm.0.000855
Chan, W.-S., Au, C.-H., Leung, S. M., Ho, D. N., Wong, E. Y. L., To, M. Y., et al. (2020). Potential utility of targeted nanopore sequencing for improving etiologic diagnosis of bacterial and fungal respiratory infection. Diagn. Pathol. 15, 41. doi: 10.1186/s13000-020-00960-w
Charalampous, T., Kay, G. L., Richardson, H., Aydin, A., Baldan, R., Jeanes, C., et al. (2019). Nanopore metagenomics enables rapid clinical diagnosis of bacterial lower respiratory infection. Nat. Biotechnol. 37, 783–792. doi: 10.1038/s41587-019-0156-5
Cheng, J., Hu, H., Kang, Y., Chen, W., Fang, W., Wang, K., et al. (2018). Identification of pathogens in culture-negative infective endocarditis cases by metagenomic analysis. Ann. Clin. Microbiol. Antimicrob. 17, 43. doi: 10.1186/s12941-018-0294-5
Dyrhovden, R., Nygaard, R. M., Patel, R., Ulvestad, E., and Kommedal, Ø. (2019). The bacterial aetiology of pleural empyema. A descriptive and comparative metagenomic study. Clin. Microbiol. Infect. 25, 981–986. doi: 10.1016/j.cmi.2018.11.030
Fenollar, F., and Raoult, D. (2004). Molecular genetic methods for the diagnosis of fastidious microorganisms. APMIS 112, 785–807. doi: 10.1111/j.1600-0463.2004.apm11211-1206.x
Fenollar, F., Roux, V., Stein, A., Drancourt, M., and Raoult, D. (2006). Analysis of 525 samples to determine the usefulness of PCR amplification and sequencing of the 16S rRNA gene for diagnosis of bone and joint infections. J. Clin. Microbiol. 44, 1018–1028. doi: 10.1128/JCM.44.3.1018-1028.2006
Gauduchon, V., Chalabreysse, L., Etienne, J., Celard, M., Benito, Y., Lepidi, H., et al. (2003). Molecular diagnosis of infective endocarditis by PCR amplification and direct sequencing of DNA from valve tissue. J. Clin. Microbiol. 41, 763–766. doi: 10.1128/JCM.41.2.763-766.2003
Goodwin, S., McPherson, J. D., and McCombie, W. R. (2016). Coming of age: ten years of next-generation sequencing technologies. Nat. Rev. Genet. 17, 333–351. doi: 10.1038/nrg.2016.49
Heintz, E., Pettengill, M. A., Gangat, M. A., Hardy, D. J., Bonnez, W., and Sobhanie, M. M. (2019). Oral flora meningoencephalitis diagnosis by next-generation DNA sequencing. Access Microbiol. 1, e000056. doi: 10.1099/acmi.0.000056
Hong, N. T. T., Nghia, H. D. T., Thanh, T. T., Lan, N. P. H., Ny, N. T. H., Ngoc, N. M., et al. (2019). Cerebrospinal fluid MinION sequencing of 16S rRNA gene for rapid and accurate diagnosis of bacterial meningitis. J. Infect. 80, 469–496. doi: 10.1016/j.jinf.2019.12.011
Johnson, J. S., Spakowicz, D. J., Hong, B.-Y., Petersen, L. M., Demkowicz, P., Chen, L., et al. (2019). Evaluation of 16S rRNA gene sequencing for species and strain-level microbiome analysis. Nat. Commun. 10, 5029. doi: 10.1038/s41467-019-13036-1
Kai, S., Matsuo, Y., Nakagawa, S., Kryukov, K., Matsukawa, S., Tanaka, H., et al. (2019). Rapid bacterial identification by direct PCR amplification of 16S rRNA genes using the MinION™ nanopore sequencer. FEBS Open Bio 9, 548–557. doi: 10.1002/2211-5463.12590
Laupland, K. B., and Valiquette, L. (2013). The changing culture of the microbiology laboratory. Can. J. Infect. Dis. Med. Microbiol. 24, 125–128. doi: 10.1155/2013/101630
Leggett, R. M., Alcon-Giner, C., Heavens, D., Caim, S., Brook, T. C., Kujawska, M., et al. (2020). Rapid MinION profiling of preterm microbiota and antimicrobial-resistant pathogens. Nat. Microbiol. 5, 430–442. doi: 10.1038/s41564-019-0626-z
Martiny, D., Dauby, N., Konopnicki, D., Kampouridis, S., Jissendi Tchofo, P., Horoi, M., et al. (2017). MALDI-TOF MS contribution to the diagnosis of Campylobacter rectus multiple skull base and brain abscesses. New Microbes New Infect 19, 83–86. doi: 10.1016/j.nmni.2017.05.014
Mitsuhashi, S., Kryukov, K., Nakagawa, S., Takeuchi, J. S., Shiraishi, Y., Asano, K., et al. (2017). A portable system for rapid bacterial composition analysis using a nanopore-based sequencer and laptop computer. Sci. Rep. 7, 5657. doi: 10.1038/s41598-017-05772-5
Moon, J., Kim, N., Kim, T.-J., Jun, J.-S., Lee, H. S., Shin, H.-R., et al. (2019). Rapid diagnosis of bacterial meningitis by nanopore 16S amplicon sequencing: a pilot study. Int. J. Med. Microbiol. 309, 151338. doi: 10.1016/j.ijmm.2019.151338
Moon, J., Kim, N., Lee, H. S., Shin, H.-R., Lee, S.-T., Jung, K.-H., et al. (2017). Campylobacter fetus meningitis confirmed by a 16S rRNA gene analysis using the MinION nanopore sequencer, South Korea, 2016. Emerg. Microbes Infect. 6, e94. doi: 10.1038/emi.2017.81
Nakazawa, F., Poco, S. E., Sato, M., Ikeda, T., Kalfas, S., Sundqvist, G., et al. (2002). Taxonomic characterization of Mogibacterium diversum sp. nov. and Mogibacterium neglectum sp. nov., isolated from human oral cavities. Int. J. Syst. Evol. Microbiol. 52, 115–122. doi: 10.1099/00207713-52-1-115
Parize, P., Muth, E., Richaud, C., Gratigny, M., Pilmis, B., Lamamy, A., et al. (2017). Untargeted next-generation sequencing-based first-line diagnosis of infection in immunocompromised adults: a multicentre, blinded, prospective study. Clin. Microbiol. Infect. 23, 574.e1–574.e6. doi: 10.1016/j.cmi.2017.02.006
Plainvert, C., Doloy, A., Loubinoux, J., Lepoutre, A., Collobert, G., Touak, G., et al. (2012). Invasive group A streptococcal infections in adults, France (2006–2010). Clin. Microbiol. Infect. 18, 702–710. doi: 10.1111/j.1469-0691.2011.03624.x
Renvoisé, A., Brossier, F., Sougakoff, W., Jarlier, V., and Aubry, A. (2013). Broad-range PCR: past, present, or future of bacteriology? Med. Mal. Infect. 43, 322–330. doi: 10.1016/j.medmal.2013.06.003
Sanderson, N. D., Street, T. L., Foster, D., Swann, J., Atkins, B. L., Brent, A. J., et al. (2018). Real-time analysis of nanopore-based metagenomic sequencing from infected orthopaedic devices. BMC Genom. 19, 714–711. doi: 10.1186/s12864-018-5094-y
Selton-Suty, C., Celard, M., Le Moing, V., Doco-Lecompte, T., Chirouze, C., Iung, B., et al. (2012). Preeminence of Staphylococcus aureus in infective endocarditis: a 1-year population-based survey. Clin. Infect. Dis. 54, 1230–1239. doi: 10.1093/cid/cis199
Street, T. L., Sanderson, N. D., Atkins, B. L., Brent, A. J., Cole, K., Foster, D., et al. (2017). Molecular diagnosis of orthopedic-device-related infection directly from sonication fluid by metagenomic sequencing. J. Clin. Microbiol. 55, 2334–2347. doi: 10.1128/JCM.00462-17
Trotter, A. J., Aydin, A., Strinden, M. J., and O'Grady, J. (2019). Recent and emerging technologies for the rapid diagnosis of infection and antimicrobial resistance. Curr. Opin. Microbiol. 51, 39–45. doi: 10.1016/j.mib.2019.03.001
Vieira, G. de D., Mendonça, H. R., Alves, T. D. C., Araújo, D. F. de O., Silveira Filho, M. L. D., de Freitas, A. P. dos S. R., et al. (2015). Survey of infection in orthopedic postoperative and their causative agents: a prospective study. Rev. Assoc. Med. Bras. (1992) 61, 341–346. doi: 10.1590/1806-9282.61.04.341
Wang, C., Huang, Z., Li, W., Fang, X., and Zhang, W. (2020). Can metagenomic next-generation sequencing identify the pathogens responsible for culture-negative prosthetic joint infection? BMC Infect. Dis. 20, 253. doi: 10.1186/s12879-020-04955-2
Keywords: metagenomics, bacterial infections, molecular diagnosis, Nanopore sequencing, 16S rRNA
Citation: Bouchiat C, Ginevra C, Benito Y, Gaillard T, Salord H, Dauwalder O, Laurent F and Vandenesch F (2022) Improving the Diagnosis of Bacterial Infections: Evaluation of 16S rRNA Nanopore Metagenomics in Culture-Negative Samples. Front. Microbiol. 13:943441. doi: 10.3389/fmicb.2022.943441
Received: 13 May 2022; Accepted: 17 June 2022;
Published: 14 July 2022.
Edited by:
Axel Cloeckaert, Institut National de recherche pour l'agriculture, l'alimentation et l'environnement (INRAE), FranceReviewed by:
Pierre-Edouard Fournier, Aix-Marseille Université, FranceCopyright © 2022 Bouchiat, Ginevra, Benito, Gaillard, Salord, Dauwalder, Laurent and Vandenesch. This is an open-access article distributed under the terms of the Creative Commons Attribution License (CC BY). The use, distribution or reproduction in other forums is permitted, provided the original author(s) and the copyright owner(s) are credited and that the original publication in this journal is cited, in accordance with accepted academic practice. No use, distribution or reproduction is permitted which does not comply with these terms.
*Correspondence: Coralie Bouchiat, Y29yYWxpZS5ib3VjaGlhdEBjaHUtbHlvbi5mcg==
Disclaimer: All claims expressed in this article are solely those of the authors and do not necessarily represent those of their affiliated organizations, or those of the publisher, the editors and the reviewers. Any product that may be evaluated in this article or claim that may be made by its manufacturer is not guaranteed or endorsed by the publisher.
Research integrity at Frontiers
Learn more about the work of our research integrity team to safeguard the quality of each article we publish.