- Key Laboratory of Zoonosis Research, Ministry of Education, Institute of Zoonosis, Jilin University, Changchun, China
The fucosyltransferase 2 gene (FUT2) mediates the synthesis of histoblood group antigens (HBGA) that occur in vivo from multiple organs, particularly on the surface of intestinal epithelial cells and body fluids. To date, many studies have demonstrated that the interaction of HBGA with the host microbiota is the cause of pathogenesis of intestinal diseases, making FUT2 non-secretor a risk factor for inflammatory bowel disease (IBD) due to the lack of HBGA. As HBGA also acts as an attachment site for norovirus (NoV) and rotavirus (RV), the non-secretor becomes a protective factor for both viral infections. In addition, the interaction of norovirus and rotavirus with symbiotic bacteria has been found to play an important role in regulating enteroviral infection in IBD. Given the current incomplete understanding of the complex phenomenon and the underlying pathogenesis of intestinal diseases such as IBD, it has recently been hypothesized that the FUT2 gene regulates intestinal bacteria through attachment sites, may help to unravel the role of FUT2 and intestinal flora in the mechanism of intestinal diseases in the future, and provide new ideas for the prevention and treatment of intestinal diseases through more in-depth studies.
Introduction
ABO, known as the ABO blood group system, is found for classifying blood by specific antigens (agglutinogens) A and B on the surface of red blood cells. In the ABO blood group system, the antigen that eventually forms H antigens is a glycan chain catalyzed by a gene-encoded enzyme called fucosyltransferase (Barbé et al., 2018). In 1960, Watkins found that the antigen ABO is a carbohydrate, namely HBGA. The fucosyltransferase 2 (FUT2) gene mediates the synthesis of HBGA (Rouquier et al., 1995). In recent years, genome-wide association studies (GWAS) have highlighted the importance of FUT2 biology, and HBGA was found to serve as an attachment site for both intestinal flora and norovirus (NoV) and rotavirus (RV), identifying different polymorphisms of this gene resulting in distinct secretor statuses associated with the development of pathophysiological conditions between intestinal diseases (Maroni et al., 2015; Iliev and Cadwell, 2021; Tarris et al., 2021). This leads to new hypotheses for the study of complex mechanisms of intestinal diseases. In this review, we aimed to provide a brief overview of the effect of FUT2 on intestinal diseases, link HBGA to intestinal flora and enterovirus through some hypotheses about the way HBGA mediates intestinal flora, and investigate whether the FUT2 gene can be used to infer susceptibility to intestinal diseases so that the prevention can be better personalized for individuals in different regions. Therefore, we focused on the function of different FUT2 genotypes in terms of intestinal flora. As an outlook, the association of FUT2 with COVID-19 and psychiatric disorders is briefly discussed.
The Biosynthesis of HBGA
The Synthesis Process of HBGA
In recent years, four types of fucosyltransferases have been distinguished according to the specific site of fucose addition to substrates, including α-1,2-, α-1,3/4-, α-1,6-, and O-fucosyltransferases. Among these types of fucosyltransferases, FUT1, FUT2, and FUT3 catalyze α-1,2 and α-1,3/4 sites, respectively, which are indispensable for HBGA biosynthesis, and FUT1 and FUT2 play crucial catalytic roles in both types of H antigen formation (Kelly et al., 1995). Moreover, FUT1 and FUT2 have an apparent difference in acceptor specificity, and two types of H antigen precursors (type 1 and type 2) were regulated by the H enzyme encoded by the FUT2 and FUT1 gene, respectively, i.e., the acceptor specificity of FUT1 is type 2 chains (galactose-β-1→ 4-N-acetyl-glucosamine, N-acetyl-lactosamine) (GBD 2017 Inflammatory Bowel Disease Collaborators, 2020); in contrast, FUT2 gene-specified enzyme is more vigorous on type 1 (galactose-β-1→ 3-N-acetyl-glucosamine, lacto-N-biose) structures (Kyprianou et al., 1990). Two kinds of precursors serve as substrates for the FUT2 and FUT1 enzymes, which modify them by attaching an L-fucose to the galactose moiety via an α-1→ 2 linkage, generating type-1 and type-2 H antigens, respectively (Peña-Gil et al., 2021). However, if the FUT3 enzyme modifies the precursor, Lea (type 1 precursor) and Lex (type 2 precursor) antigens are produced. Different from the formation of H-type antigens, the modification of FUT3 involves the attachment of an L-fucose to N-acetyl-glucosamine with an α-1→ 4 linkage in the case of the type-1 precursor or an α-1→ 3 linkage in the case of the type-2 precursor (Monedero et al., 2018). Furthermore, H type-1 and type-2 antigens will be transformed to A and/or B blood groups catalyzed by two glycosyltransferases (A and B enzymes), which are coded by the ABO family. As a result, the FUT3 enzyme can apply to the H type-1 and type-2 antigens generating Leb and Ley antigens (Marionneau et al., 2001; Tan and Jiang, 2014) (Figure 1).
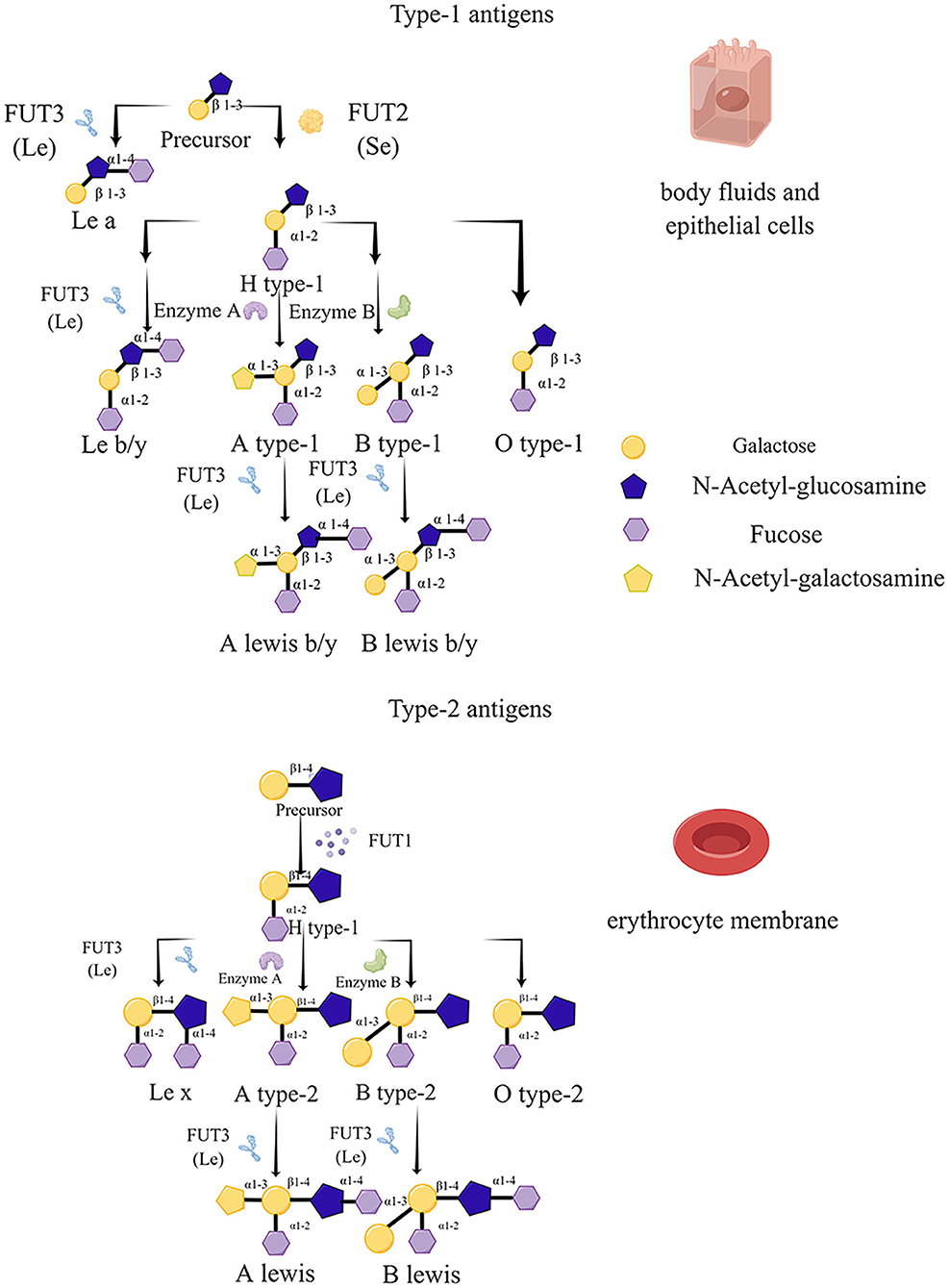
Figure 1. Biosynthesis routes and schematic structure of histo-blood group antigens (HBGAs). The antigen precursors on erythrocytes are mainly type 2 (N-Acetyl-galactosamine, N-Acetyl-Lac) catalyzed by the lactosyltransferases FUT1 and FUT3 for elongation, while those in other tissues and body fluids are type 1 precursors (Lacto-N-Biose, LNB), catalyzed by lactosyltransferase-2 (FUT2) and FUT3 enzymes further catalyze the synthesis to produce H and Lewis antigens, as well as A and B blood groups by A and B enzymes.
The Relevance of the HBGA and Secretor Status
In fact, HBGA can emerge from multiple organs in vivo probably. The reason is that the main function of the FUT1 gene (H) is to control the form of antigen type 2 on the red blood cell; nevertheless, the FUT2 gene (Se) mainly catalyzes the type 1 antigens of other mucosal tissues, body fluids, such as in saliva, breast milk and digestive juices (Ye and Yu, 2021), and epithelial cells. Therefore, we can determine, theoretically, when both FUT1 and FUT2 genes have normal expression functions, namely, the secretors genotype. Notably, it has been known that one-fifth of the population cannot express A, B, or H antigens in body fluids who are called as “non-secretor” (se). Actually, the existence of “non-secretor” is due to the nonfunctional mutations leading to the FUT2 homozygous gene, which results in the improper synthesis of antigens. Thus, ABO antigens are undetectable in their epithelial tissues and body secretions. However, the standard quantity levels of ABO antigens exist on the membranes of erythrocytes with the FUT1 gene being normal. Moreover, this phenomenon is consistent with the description of the tissue distribution of FUT1 and FUT2. In contrast, if the FUT1 gene is a recessive homozygote, a special o-type named Bombay type may occur.
Remarkably, the secretor status of the FUT2 gene was suggested as a close correlation with a variety of gastrointestinal diseases, including IBD (Tang et al., 2021), and diseases caused by viruses, mainly norovirus (Nov) (Lindesmith et al., 2003; Currier et al., 2015) and rotavirus (RV) (Galeev et al., 2021). In fact, the reason behind this phenotype determines the presence or absence of HBGA, which consists of an attachment site and a carbon source of intestinal microbiota (Tong et al., 2014; Kononova, 2017), and the major binding saccharide of some viruses, including NoV (Chen et al., 2011) and RV (Sharma et al., 2020). Recently, alteration of the composition and function of the gut microbiota, namely dysbiosis, which was suggested, may greatly contribute to the pathogenesis of IBD. Moreover, the interaction between enteric virus and commensal bacteria seem to play a significant role in the modulation of enteric virus infections in IBD. Due to the association with HBGA and enteric virus, GWAS has demonstrated the association between IBD and single nucleotide polymorphism (SNPs) of the FUT2 and FUT3 genes, which drive the synthesis of HBGA, and ligands for NoV and RV in the intestine (Tarris et al., 2021). SNPs can determine the secretion status of FUT2 gene, such as G428A (Caucasians population) (Soejima and Koda, 2021a), 778C>Del (Soejima and Koda, 2021a), A385T (Asian population) (Ye and Yu, 2021), 818C>A (Thr273Asn) (Soejima et al., 2012), 853G>A (Ala285Thr) (Soejima et al., 2012), 617 T>G (V206G) (Tian et al., 2014), 841G>A (G281R) (Tian et al., 2014), 244G>A (Soejima et al., 2008), 569G>A (Soejima et al., 2008), 950C>T (Soejima et al., 2008), 357C>T; 856T>C; and 863C>T (Soejima and Koda, 2021b); both are mutations that inactivate the enzyme and thus result in loss of Se enzyme activity, which mainly determines the non-secretor type. However, this is not a universal phenomenon in populations around the world, as SNPs of FUT2 are specific for population and region. For instance, a study described that the homozygosity for a nonsense mutation G428A in the FUT2 increases the risk of susceptibility to IBD in the Finnish population due to the absence of ABO blood groups in body fluids (Parmar et al., 2012). Similarly, a study from southeastern China suggested that FUT2 gene polymorphisms were considered to be associated with susceptibility to Crohn's disease (CD) (Wu et al., 2017). In another study, mutant allele and genotype of FUT2 (A385T and G428A) were dramatically increased in patients with CD (Wu et al., 2017). On the contrary, SNP 235G>A (Soejima and Koda, 2020), 304G>A (Soejima and Koda, 2020), 357C>T, 4G>A (Soejima et al., 2008), 489G>A (Soejima et al., 2008), and 665G>A (Soejima et al., 2008) were determined as the secretor type in a particular population, which were regarded as a protective factor for IBD, although it may be a risk locus for entering the virus genetically. As such, homozygous nonsense mutation (428G>A) in the FUT2 gene provides resistance to symptomatic NoV (GGII) infections (Thorven et al., 2005). Indeed, as discussed, the secretor status of FUT2 has shown a significant association with intestinal diseases.
FUT2 and IBD
Crohn's Disease
Inflammatory bowel disease (IBD), including ulcerative colitis (UC) and CD, is a multifactorial disease in which dietary, genetic, immunological, and microbial factors are at play (Khor et al., 2011; Nishida et al., 2018).
Recently, numerous studies have been conducted suggesting the involvement of FUT2 in genetic susceptibility to IBD, particularly CD. For instance, the association between FUT2 polymorphism and susceptibility to CD was observed in a Belgian sample (Forni et al., 2014), and this study provides strong evidence that non-secretor status increases susceptibility to CD (McGovern et al., 2010). A study in a small Japanese cohort reported that the FUT2 secretor status was also specifically associated with the colonic localization of CD (Miyoshi et al., 2011). Recently, an animal study using FUT2ΔIEC mice (FUT2 gene knockout mice) found more serious intestinal inflammation and destructive barrier functions that occurred in FUT2ΔIEC mice compared with WT mice when DSS was treated; simultaneously, while at the same time, less diversity of intestinal microbiota was observed in FUT2ΔIEC mice (Tang et al., 2021). It is found that N-acetylgalactosamine, one of the compounds synthesized by HGBA, may change gut microbiota in pigs (Yang et al., 2022). Contributing to the up-to-date progress in next-generation sequencing technology, IBD patients have been found to have alterations in the composition and function of the gut microbiota, termed dysbiosis, which is thought to play a central role in the pathogenesis of IBD (McGovern et al., 2010). In addition, dysbiosis may be one of the reasons for the increased susceptibility (McGovern et al., 2010); in fact, the composition and function of the intestinal microbiota were suggested to be affected by HBGA synthesis, which is mediated by both the FUT2 and ABO genes (Forni et al., 2014). The different secretor statuses result in the expression of ABO and Lewis glycan epitopes in the intestinal mucosa that may have affected the attachment of bacteria. For instance, probiotics Bifidobacteria (Wacklin et al., 2011) and Lactobacillus genus (Watanabe et al., 2010) show a significant decrease in the individuals with IBD genetic risk compared with healthy controls. Meanwhile, Rauschet al., indicated that compared with the secretor, the bacterial composition of non-secretors resembles more tightly to CD patients (Rausch et al., 2011). Given these observations, it comes as no surprise that the function of the FUT2 gene could have a positive impact on dysbiosis of intestinal microbiota.
A defective immune response against the commensal intestinal microbiota has been proposed as one of the most possible pathogenic mechanisms of CD (Xavier and Podolsky, 2007).
Ulcerative Colitis
According to the recent studies, the contribution of FUT2 to the pathogenesis of UC remains, to date, less clear. McGovern et al., reported that the non-secretor status is associated only with CD, and the association between UC and FUT2 is difficult to confirm (McGovern et al., 2010). Nevertheless, SNPs of the FUT2 gene leading to non-secretor status, including rs281377, rs1047781, and rs601338, have been associated with UC in a small sample population of Han and Uyghur ethnic groups in China (Aheman et al., 2012). In contrast to what has been discussed above for CD, a report based on a Finnish population showed that the homozygous rs601338-AA (G428A) SNP indicates a dominant protective role of the A allele against UC, while the GG genotype showed an increased risk of UC. That is to say, the mutant allele appears to be more protective of UC than of CD (Parmar et al., 2012). There is no doubt that IBD is caused by both UC and CD, but the two diseases are quite different in their genetic susceptibility. For example, the secretor statuses of FUT2 lead to opposite outcomes in susceptibility of the two diseases and have complex interactions with the extra genetic, environmental, and other factors that cause disease severity. The same is true for CD and UC: genetic variation of FUT2 was closely related to changes in different gut microbiota in patients with IBD.
The Hypothesis of Pathogenetic Mechanism of IBD: Interactions in Host-Microbe and Fucosylated Glycans
Although a few theories suggest that the taxonomic composition of the microbiota does not appear to be strongly associated with ABO or secretor status (Davenport et al., 2016), clinical and experimental data illustrate that dysbiosis could be a crucial element in the pathogenesis of IBD (Nishida et al., 2018). For instance, Bifidobacteria are important probiotics in the human intestine and the Bifidobacterial population detectable in the intestine of a healthy adult always consists of one to four species under normal conditions (Mättö et al., 2004), while one study from the fecal samples of 71 healthy individuals with different secretor status found that the diversity and abundance of dominant bacteria and Bifidobacteria were considerably lower in the samples from the non-secretor individuals as compared with those from the secretor individuals (Wacklin et al., 2011); similarly, the Lachnospiraceae family with lower abundances was noted in nonsecretors (Gampa et al., 2017); in other words, the non-secretors were considered to have lower species richness than the secretors (Wacklin et al., 2014). The reason was potentially explained, as the different secretor statuses may result in the expression of ABO and Lewis glycan epitopes in the intestinal mucosa that may have affected the attachment of bacteria. Recently, in animal experiments, it has been found that N-acetylgalactosamine, which is one of the compounds synthesized by HGBA, may change gut microbiota in pigs (Yang et al., 2022). Similarly, the probiotic Lactobacillus genus may hinder the attachment of potential pathogens to mucosal surfaces by possessing adhesins that bind to the ABO blood group antigens in the mucosa (Watanabe et al., 2010). Another study of the genus Roseburia showed a significant decrease in the individuals with IBD genetic risk compared with healthy controls (Imhann et al., 2018).
From the abovementioned data, we can draw a hypothesis that the FUT2 gene mediates the probiotic population by regulating the production of HBGA. Also, Roseburia spp functions to convert acetate into butyrate (Imhann et al., 2018), which protects against colon inflammation (Chang et al., 2014), counter-intuitively. Cheng et al., found more butyrate-producing bacteria and higher butyrate levels in sese individuals through metabolomic analysis (Cheng et al., 2021). This finding is against the abovementioned hypothesis. Thus, another hypothesis for the involvement of FUT2 in the mechanism of IBD is that nonfunctional mutation in FUT2 reduces the abundance of appressed bacteria by reducing their binding sites, which may allow the overgrowth of other bacteria, which in turn induces inflammatory T cells and leads to IBD (Cheng et al., 2021).
Given these observations, we agreed and emphasized the suggestion that the gut bacterial community in IBD patients and FUT2 with non-secretors differs from one of the secretors or healthy individuals (Nishida et al., 2018). The dysfunction of FUT2 is one of the most important causes of increased susceptibility to IBD including CD and UC. A brief summary of some variations and functions of common probiotic and pathogenic bacteria in IBD is shown in Table 1.
FUT2 Gene and Susceptibility to NoV and RV Infections
FUT2 Gene and NoV Infections
Norovirus, an RNA virus of the Caliciviridae family, is known as a human enteric pathogen that causes substantial morbidity (Robilotti et al., 2015), and is the leading cause of nonbacterial gastroenteritis and acute gastroenteritis (AGE) worldwide (Haga et al., 2020). Unlike the susceptibility to IBD, HBGA expression under the influence of the FUT2 gene is an important susceptibility risk for NoV infection, and secretor status is associated with susceptibility to NoV infection (Currier et al., 2015; Haga et al., 2020), while non-secretors lack enzyme coded by FUT2 resulting in high resistance to infection and gastroenteritis caused by numerous NoV strains. Moreover, GII.4 NoV infects secretors exclusively (Currier et al., 2015); it is the most commonly detected genotype of NoV, and the same indication was found in a community-based birth cohort of 194 children in Ecuador (Lopman et al., 2015). The next major genotypes were GII.3 and GII.6 (Mans, 2019). However, the non-secreting form is resistant to several NoV genotypes. After all, the link between FUT2 and NoV susceptibility has been explained by the fact that FUT2 secretor status alters HBGA expression in the intestine, while some GII group genotypes, mainly GII.4 and GII.6, use H or Lewis antigens as the main binding saccharides mediated by fucosyl- and glycosyltransferases under the genetic control of FUT2 (secretor), FUT3 (Lewis), and ABO(H) genes (Chen et al., 2011). This is the reason why some NoV are more susceptible in individuals with secretor status genotype rather than the non-secretor one.
The second factor affecting enterovirus infection is the host gut microbiome. Similar to IBD, for example, probiotics protect the host from viral infection by modulating the composition of the gut microbiota, enhancing intestinal barrier function, and promoting mucosal immunity (Peña-Gil et al., 2021). Additionally, they interfere with the binding of viruses to target cells by blocking viral receptors and binding to the surface in a competitive rejection manner, facilitating their excretion in the feces (Monedero and Rodríguez-Díaz, 2016). Furthermore, the presence of Bifidobacterium adolescentis inhibits the attachment of NoV GI.1 VLPs to epithelial cells in vitro (Li et al., 2016). In another study, Lactobacillus rhamnosus GG (LGG) and Escherichia coli Nissle 1917 (EcN) can bind NoV P particles containing genotype GII.3 and GII.4; this was investigated using the gnotobiotic pig model, and viral shedding in feces below the detection limit was observed, indicating significant inhibition on NoV infection by the colonization of such bacteria. Additionally, Lactobacillus rhamnosus GG (LGG) and E. coli Nissle 1917 (EcN) as a probiotic cocktail and supplemented with rice bran had a high protective effect against HuNoV diarrhea and shedding (Lei et al., 2016). There is also evidence which suggests that the members of the intestinal microbiota such as Enterobacter cloacae, E. coli, and Helicobacter pylori facilitate NoV infection (Rasko et al., 2000; Yi et al., 2005; Jones et al., 2014). However, studies on the negative effects of the microbiota on NoV infectivity are scarce. Epidemiological studies suggest that vitamin A intake has an anti-NoV impact. Research with murine NoV draws a conclusion that the amount of intestinal Lactobacilly ssp. increased after supplementation of vitamin A, which was speculated as the reason for the antiviral effect (Lee and Ko, 2016).
In brief, the abovementioned studies have clearly demonstrated that the major genotype GII.4, as well as several other less common genotypes, have clear specificity of secretor. A wide range of different NoV genotypes, including GI.3, GI.6, GII.1, GII.2, and GII.7 (Nordgren et al., 2013; Prystajecky et al., 2014; Currier et al., 2015; Lopman et al., 2015), have also been described in these studies that are also capable to infect an individual with non-secretors genotypes. However, no genotype has been found to exclusively infect non-secretors. In the previous discussion, the alteration of intestinal flora has been considered to be an important cause or consequence of intestinal diseases, and there are hypotheses suggesting that its function and attachment can be differentially influenced by FUT2, which has received great attention in the previous studies on IBD, and at the same time, some genera are also associated with viral infections, so it should also receive more attention in the studies about NoV, especially the pathogenic mechanism.
FUT2 Gene and RV Infections
Rotavirus (RV) is the most common causative agent of severe dehydrating gastroenteritis in children worldwide (Sharma et al., 2020). To some extent, the susceptibility effect of the FUT2 gene of RV is similar to that of NoV. HBGAs act as important attachment factors for human RV, especially VP8* (P-genotype) (Günaydin et al., 2016; Sharma et al., 2020). VP8* is the glycan-binding domain of VP4 protein (P genotype), which is used to define protease-sensitive (P) serotypes or genotypes; structural proteins (VP) were encoded by double-stranded RNA gene segments in triple-layered capsid (Sharma et al., 2020). In fact, the function of VP8* is responsible for binding to cellular receptors, namely HBGA, thereby facilitating viral attachment and entry (Sharma et al., 2020). Moreover, a French study suggested that RV P[8] infections were completely absent in individuals with the non-secretor phenotype, namely, the homozygous nonsense mutation FUT2 (Imbert-Marcille et al., 2014). In addition, a study based on children in Burkina Faso and Nicaragua found that P[8] and P[4] genotypes barely infected non-secretor and Lewis-negative children, while P[6] RV mostly infected children whose phenotype was independent of secretor status yielding the Lewis-negative population (Nordgren et al., 2014). However, most studies have suggested a strong association between positive secretor status and susceptibility of RV; some researchers have found individuals with Lewis positivity, independent of secretor status phenotype, as a susceptibility factor (Ayouni et al., 2015), while others have found that the individuals have both secretor- and Lewis-positive statuses, as well as Lewis b phenotype seemingly has a higher susceptibility risk than only secretor-positive status (Nordgren et al., 2014).
In terms of bacteria, RV-induced diarrhea may be reduced via an immune response, modulated by E. coli Nissle 1917 (Kandasamy et al., 2016; Vlasova et al., 2016). In addition, Ruminococcus gauvreauii, namely, a bacterium that has been isolated from human bile and seems to present in the small intestine, which is the site more likely to infect RV, has also been proved that can bind RV. Viral infectivity was reduced to threefold in the presence of R. gauvreauii, indicating that this bacterium has an anti-RV effect (Gozalbo-Rovira et al., 2021). Moreover, bacteria belonging to lactobacilli, Mucispirillum, Oscillospira, and Bilophila genera were negatively linked to RV infection in mice (Peña-Gil et al., 2021). In fact, the effect of HGBA on bacterial attachment, which may lead to interference with RV infection in vitro.
To summarize, we can conclude that secretor and Lewis antigens are important for susceptibility to RV such as in a P genotype–dependent way.
Discussion
As mentioned above, growing evidence and hypotheses support the involvement of FUT2 in the pathogenesis of many intestinal diseases. Among them, various inactivating polymorphisms resulting in a non-secretor phenotype is a susceptibility factor for IBD and conversely a protective factor for NoV and RV, the reasons behind which are due to the presence or absence of HBGA, in which synthesis is regulated by the FUT2 gene. The hypothesis explains that the FUT2 gene may regulate the synthesis of HBGA at the attachment site of intestinal bacteria, thus enabling the screening of the flora. An important factor in intestinal health is the flora that has been hypothesized to be influenced by the sugar chain called HBGA. Therefore, there is a non-negligible association between the FUT2 gene and intestinal diseases including IBD, which is caused by RV and NoV.
The disease caused by SARS-CoV-2 was subsequently named as COVID-19 by the WHO. Since the rapid global outbreak of COVID-19, extensive research suggested the ABO blood group system and secretor phenotype (Valenti et al., 2020) in potentially predisposing to infection with SARS-CoV-2 (Al-Youha et al., 2021). To date, a meta-analysis illustrates that the association between the ABO blood group and the infection of COVID-19 is that blood type A might be more susceptible to infecting COVID-19, while blood type O might be less susceptible to infecting COVID-19 (Wu et al., 2020). But the effect of the ABO blood group on the COVID-19 is not only in the infection but also contains severity and demise (Torres-Alarcón et al., 2021). A Chinese study analysis association between the ABO blood group and COVID-19 suggested that there was a statistically significant difference for blood type A rather than blood types B, AB, or O (Liu et al., 2021), particularly in women of Wuhan (Fan et al., 2020). The changes in host susceptibility to many infections are actually because of diversities in blood group antigen expression (Cooling, 2015). That is why more and more researches found out that the O blood type is a protection factor against COVID-19 infection and severe COVID-19 illness (Flegel, 2020; Wu et al., 2020; Liu et al., 2021; Ray et al., 2021), whereas a study reported that the interactions of the ABO and secretor types in the cohort of patients compared with the blood donors lack significant differences, so they suggested that that emphasis should not be put on the ABO antigenic structures, but rather on the isoagglutinin (Matzhold et al., 2021). In addition to using different antigens, different ABO blood groups also contain different situations of isoagglutininsin. ABO blood group O, with obligatory anti-A and anti-B antibodies present, is protective against COVID-19 (Matzhold et al., 2021). The Le (a–b–) type appeared to be at least a mitigating factor against hospitalization with COVID-19. Invariably, many study results reach a consensus that the O blood type acts as a protection factor against COVID-19, while no ABO blood group type can protect an individual from becoming infected (Flegel, 2020). Notably, individuals able to express these antigens on epithelial cells, known as the FUT2 gene secretion status, indicated a higher degree of exposure to SARS-CoV-2 (Shokri et al., 2022).
Moreover, mounting evidence currently suggested that neurological diseases including Parkinson's disease (PD) (Brudek, 2019; Lee et al., 2021) anxiety, and depressive disorder (Barberio et al., 2021) were highly related to the IBD. In addition, the gut microbiome is considered to be an indispensable element in the interaction between IBD and neurological disease (Needham et al., 2020; Nielsen et al., 2021). Currently, the existence of the brain-gut axis has been described by an increasing number of studies and is one of the most accepted reasons for this phenomenon (Sun and Shen, 2018; Carloni et al., 2021). The metabolites of the flora may enter certain areas of the brain through the brain-gut axis and affect the regular function of a specific brain. In the study of PD, the gut microbiome is required for microglial activation, α synuclein pathology, and motor deficits, which is a focus of research on the pathogenesis of PD (Nielsen et al., 2021). Whereas a possible chemical metabolite, 4-ethyl phenyl sulfate (4EPS), produced by intestinal bacteria from tyrosine metabolism (Hsiao et al., 2013), was identified in studies of depression and anxiety; 4EPS enters the mouse brain and affects the activation and connectivity of specific brain regions, disrupting brain oligodendrocyte maturation and myelination patterns, thereby modulating brain activity and anxiety-like behavior in mice. As a result, mice exposed to 4EPS exhibited anxiety-like behaviors and pharmacological treatment promoting oligodendrocyte differentiation blocked by the behavioral effects of 4EPS; pharmacological treatments that promote oligodendrocyte differentiation prevented the behavioral effects of 4EPS (Needham et al., 2022). In the future, animal studies using oral microbiota transplants from human PD cases could provide further insight into the entire mechanism. Therefore, the FUT2 gene, as mentioned above, has the function of regulating intestinal flora that may also affect the diseases associated with IBD, which can be regarded as a new research direction.
Author Contributions
MH, XZ, JL, and TS initiated the project, wrote, revised, and finalized the manuscript. LC, XH, and TS searched the database. All authors contributed to the article and approved the submitted version.
Funding
This study was financially supported by the National Natural Science Foundation of China (grant no. 32101226), the Young Elite Scientist Sponsorship Program by CAST (No. YESS20210189), the National Natural Science Foundation of China Program for Changjiang Scholars and Innovative Research Team in University (No. IRT_16R32), and the Science Foundation of Education Bureau of Jilin Province (Changchun, China; grant no. JJKH20211055KJ).
Conflict of Interest
The authors declare that the research was conducted in the absence of any commercial or financial relationships that could be construed as a potential conflict of interest.
Publisher's Note
All claims expressed in this article are solely those of the authors and do not necessarily represent those of their affiliated organizations, or those of the publisher, the editors and the reviewers. Any product that may be evaluated in this article, or claim that may be made by its manufacturer, is not guaranteed or endorsed by the publisher.
References
Aheman, A., Luo, H. S., and Gao, F. (2012). Association of fucosyltransferase 2 gene variants with ulcerative colitis in Han and Uyghur patients in China. World J. Gastroenterol. 18, 4758–64. doi: 10.3748/wjg.v18.i34.4758
Al-Youha, S. A., Alduaij, W., Al-Serri, A., Almazeedi, S. M., Al-Haddad, M., Jamal, M. H., et al. (2021). The impact of ABO blood groups on clinical outcomes and susceptibility to COVID-19: a retrospective study in an unselected population. Transfusion 61, 1631–41. doi: 10.1111/trf.16365
Ayouni, S., Sdiri-Loulizi, K., Rougemont, A., Estienney, M., Ambert-Balay, K., Aho, S., et al. (2015). Rotavirus P[8] infections in persons with secretor and nonsecretor phenotypes, Tunisia. Emerg. Infect. Dis, 21, 2055–8. doi: 10.3201/eid2111.141901
Barbé, L., Le Moullac-Vaidye, B., Echasserieau, K., Bernardeau, K., Carton, T., Bovin, N., et al. (2018). Histo-blood group antigen-binding specificities of human rotaviruses are associated with gastroenteritis but not with in vitro infection. Sci. Rep. 8, 12961. doi: 10.1038/s41598-018-31005-4
Barberio, B., Zamani, M., Black, C. J., Savarino, E. V., and Ford, A. C. (2021). Prevalence of symptoms of anxiety and depression in patients with inflammatory bowel disease: a systematic review and meta-analysis. Lancet Gastroenterol. Hepatol. 6, 359–70. doi: 10.1016/S2468-1253(21)00014-5
Brudek, T. (2019). Inflammatory bowel diseases and Parkinson's disease. J. Parkinsons Dis. 9, S331–44. doi: 10.3233/JPD-191729
Carloni, S., Bertocchi, A., Mancinelli, S., Bellini, M., Erreni, M., Borreca, A., et al. (2021). Identification of a choroid plexus vascular barrier closing during intestinal inflammation. Science 374, 439–48. doi: 10.1126/science.abc6108
Chang, P. V., Hao, L., Offermanns, S., and Medzhitov, R. (2014). The microbial metabolite butyrate regulates intestinal macrophage function via histone deacetylase inhibition. Proc. Natl. Acad. Sci. U. S. A. 111, 2247–52. doi: 10.1073/pnas.1322269111
Chen, Y., Tan, M., Xia, M., Hao, N., Zhang, X. C., Huang, P., et al. (2011). Crystallography of a Lewis-binding norovirus, elucidation of strain-specificity to the polymorphic human histo-blood group antigens. PLoS Pathog. 7, e1002152. doi: 10.1371/journal.ppat.1002152
Cheng, S., Hu, J., Wu, X., Pan, J. A., Jiao, N., Li, Y., et al. (2021). Altered gut microbiome in FUT2 loss-of-function mutants in support of personalized medicine for inflammatory bowel diseases. J. Genet. Genomics 48, 771–80. doi: 10.1016/j.jgg.2021.08.003
Cooling, L. (2015). Blood groups in infection and host susceptibility. Clin. Microbiol. Rev. 28, 801–70. doi: 10.1128/CMR.00109-14
Currier, R. L., Payne, D. C., Staat, M. A., Selvarangan, R., Shirley, S. H., Halasa, N., et al. (2015). Innate susceptibility to norovirus infections influenced by FUT2 genotype in a United States Pediatric Population. Clin. Infect. Dis., 60, 1631–8. doi: 10.1093/cid/civ165
Davenport, E. R., Goodrich, J. K., Bell, J. T., Spector, T. D., Ley, R. E., Clark, A. G., et al. (2016). ABO antigen and secretor statuses are not associated with gut microbiota composition in 1,500 twins. BMC Genomics 17, 941. doi: 10.1186/s12864-016-3290-1
Dejea, C. M., Fathi, P., Craig, J. M., Boleij, A., Taddese, R., Geis, A. L., et al. (2018). Patients with familial adenomatous polyposis harbor colonic biofilms containing tumorigenic bacteria. Science 359, 592–97. doi: 10.1126/science.aah3648
Fan, Q., Zhang, W., Li, B., Li, D. J., Zhang, J., Zhao, F., et al. (2020). Association between ABO blood group system and COVID-19 susceptibility in Wuhan. Front. Cell Infect. Microbiol. 10, 404. doi: 10.3389/fcimb.2020.00404
Fedorak, R. N., Feagan, B. G., Hotte, N., Leddin, D., Dieleman, L. A., Petrunia, D. M., et al. (2015). The probiotic VSL#3 has anti-inflammatory effects and could reduce endoscopic recurrence after surgery for Crohn's disease. Clin. Gastroenterol. Hepatol. 13, 928–35.e2. doi: 10.1016/j.cgh.2014.10.031
Flegel, W. A. (2020). COVID-19: risk of infection is high, independently of ABO blood group. Haematologica 105, 2706–08. doi: 10.3324/haematol.2020.266593
Forni, D., Cleynen, I., Ferrante, M., Cassinotti, A., Cagliani, R., Ardizzone, S., et al. (2014). ABO histo-blood group might modulate predisposition to Crohn's disease and affect disease behavior. J. Crohns Colitis 8, 489–94. doi: 10.1016/j.crohns.2013.10.014
Galeev, A., Suwandi, A., Cepic, A., Basu, M., Baines, J. F., Grassl, G. A., et al. (2021). The role of the blood group-related glycosyltransferases FUT2 and B4GALNT2 in susceptibility to infectious disease. Int. J. Med. Microbiol. 311, 151487. doi: 10.1016/j.ijmm.2021.151487
Gampa, A., Engen, P. A., Shobar, R., and Mutlu, E. A. (2017). Relationships between gastrointestinal microbiota and blood group antigens. Physiol. Genomics 49, 473–83. doi: 10.1152/physiolgenomics.00043.2017
GBD 2017 Inflammatory Bowel Disease Collaborators. (2020). The global, regional, and national burden of inflammatory bowel disease in 195 countries and territories, 1990-2017: a systematic analysis for the Global Burden of Disease Study 2017. Lancet Gastroenterol. Hepatol. 5, 17–30. doi: 10.1016/S2468-1253(19)30333-4
Göker, M., Gronow, S., Zeytun, A., Nolan, M., Lucas, S., Lapidus, A., et al. (2011). Complete genome sequence of Odoribacter splanchnicus type strain (1651/6). Stand. Genomic. Sci. 4, 200–9. doi: 10.4056/sigs.1714269
Gozalbo-Rovira, R., Rubio-Del-Campo, A., Santiso-Bellón, C., Vila-Vicent, S., Buesa, J., Delgado, S., et al. (2021). Interaction of intestinal bacteria with human rotavirus during infection in children. Int. J. Mol. Sci. 22. doi: 10.3390/ijms22031010
Günaydin, G., Nordgren, J., Sharma, S., and Hammarström, L. (2016). Association of elevated rotavirus-specific antibody titers with HBGA secretor status in Swedish individuals: the FUT2 gene as a putative susceptibility determinant for infection. Virus Res. 211, 64–8. doi: 10.1016/j.virusres.2015.10.005
Haga, K., Ettayebi, K., Tenge, V. R., Karandikar, U. C., Lewis, M. A., Lin, S. C., et al. (2020). Genetic manipulation of human intestinal enteroids demonstrates the necessity of a functional fucosyltransferase 2 gene for secretor-dependent human norovirus infection. mBio 11:e00251–20. doi: 10.1128/mBio.00251-20
Henke, M. T., Kenny, D. J., Cassilly, C. D., Vlamakis, H., Xavier, R. J., Clardy, J., et al. (2019). Ruminococcus gnavus, a member of the human gut microbiome associated with Crohn's disease, produces an inflammatory polysaccharide. Proc. Natl. Acad. Sci. U. S. A. 116, 12672–77. doi: 10.1073/pnas.1904099116
Hsiao, E. Y., McBride, S. W., Hsien, S., Sharon, G., Hyde, E. R., McCue, T., et al. (2013). Microbiota modulate behavioral and physiological abnormalities associated with neurodevelopmental disorders. Cell 155, 1451–63. doi: 10.1016/j.cell.2013.11.024
Iliev, I. D., and Cadwell, K. (2021). Effects of intestinal fungi and viruses on immune responses and inflammatory bowel diseases. Gastroenterology 160, 1050–66. doi: 10.1053/j.gastro.2020.06.100
Imbert-Marcille, B. M., Barbé, L., Dupé, M., Le Moullac-Vaidye, B., Besse, B., Peltier, C., et al. (2014). A FUT2 gene common polymorphism determines resistance to rotavirus A of the P[8] genotype. J. Infect. Dis. 209, 1227–30. doi: 10.1093/infdis/jit655
Imhann, F., Vich Vila, A., Bonder, M. J., Fu, J., Gevers, D., Visschedijk, M. C., et al. (2018). Interplay of host genetics and gut microbiota underlying the onset and clinical presentation of inflammatory bowel disease. Gut 67, 108–19. doi: 10.1136/gutjnl-2016-312135
Jones, M. K., Watanabe, M., Zhu, S., Graves, C. L., Keyes, L. R., Grau, K. R., et al. (2014). Enteric bacteria promote human and mouse norovirus infection of B cells. Science 346, 755–9. doi: 10.1126/science.1257147
Kandasamy, S., Vlasova, A. N., Fischer, D., Kumar, A., Chattha, K. S., Rauf, A., et al. (2016). Differential effects of escherichia coli nissle and lactobacillus rhamnosus strain GG on human rotavirus binding, infection, and B Cell immunity. J. Immunol. 196, 1780–9. doi: 10.4049/jimmunol.1501705
Kelly, R. J., Rouquier, S., Giorgi, D., Lennon, G. G., and Lowe, J. B. (1995). Sequence and expression of a candidate for the human Secretor blood group alpha(1,2)fucosyltransferase gene (FUT2). Homozygosity for an enzyme-inactivating nonsense mutation commonly correlates with the non-secretor phenotype. J. Biol. Chem. 270, 4640–9. doi: 10.1074/jbc.270.9.4640
Khor, B., Gardet, A., and Xavier, R. J. (2011). Genetics and pathogenesis of inflammatory bowel disease. Nature 474, 307–17. doi: 10.1038/nature10209
Kononova, S. V. (2017). How fucose of blood group glycotopes programs human gut microbiota. Biochemistry 82, 973–89. doi: 10.1134/S0006297917090012
Kyprianou, P., Betteridge, A., Donald, A. S., and Watkins, W. M. (1990). Purification of the blood group H gene associated alpha-2-L-fucosyltransferase from human plasma. Glycoconj. J. 7, 573–88. doi: 10.1007/BF01189078
Lee, H., and Ko, G. (2016). Antiviral effect of vitamin A on norovirus infection via modulation of the gut microbiome. Sci. Rep. 6, 25835. doi: 10.1038/srep25835
Lee, H. S., Lobbestael, E., Vermeire, S., Sabino, J., and Cleynen, I. (2021). Inflammatory bowel disease and Parkinson's disease: common pathophysiological links. Gut 70, 408–17. doi: 10.1136/gutjnl-2020-322429
Lei, S., Ramesh, A., Twitchell, E., Wen, K., Bui, T., Weiss, M., et al. (2016). High protective efficacy of probiotics and rice bran against human norovirus infection and diarrhea in gnotobiotic pigs. Front. Microbiol. 7, 1699. doi: 10.3389/fmicb.2016.01699
Li, D., Breiman, A., le Pendu, J., and Uyttendaele, M. (2016). Anti-viral effect of Bifidobacterium adolescentis against noroviruses. Front. Microbiol. 7, 864. doi: 10.3389/fmicb.2016.00864
Lindesmith, L., Moe, C., Marionneau, S., Ruvoen, N., Jiang, X., Lindblad, L. J., LePendu, Baric, R., et al. (2003). Human susceptibility and resistance to Norwalk virus infection. Nat. Med. 9, 548–53. doi: 10.1038/nm860
Liu, N., Zhang, T., Ma, L., Zhang, H., Wang, H., Wei, W., et al. (2021). The impact of ABO blood group on COVID-19 infection risk and mortality: a systematic review and meta-analysis. Blood Rev. 48, 100785. doi: 10.1016/j.blre.2020.100785
Lloyd-Price, J., Arze, C., Ananthakrishnan, A. N., Schirmer, M., Avila-Pacheco, J., Poon, T. W., et al. (2019). Multi-omics of the gut microbial ecosystem in inflammatory bowel diseases. Nature 569, 655–62. doi: 10.1038/s41586-019-1237-9
Lo Presti, A., Zorzi, F., Del Chierico, F., Altomare, A., Cocca, S., Avola, A., et al. (2019). Fecal and mucosal microbiota profiling in irritable bowel syndrome and inflammatory bowel disease. Front. Microbiol. 10, 1655. doi: 10.3389/fmicb.2019.01655
Lopman, B. A., Trivedi, T., Vicuña, Y., Costantini, V., Collins, N., Gregoricus, N., et al. (2015). Norovirus infection and disease in an ecuadorian birth cohort: association of certain norovirus genotypes with host FUT2 secretor status. J. Infect. Dis. 211, 1813–21. doi: 10.1093/infdis/jiu672
Mans, J. (2019). Norovirus infections and disease in lower-middle and low-income countries, 1997-2018. Viruses 11:341 doi: 10.3390/v11040341
Marionneau, S., Cailleau-Thomas, A., Rocher, J., Le Moullac-Vaidye, B., Ruvoën, N., Clément, M., et al. (2001). ABH and Lewis histo-blood group antigens, a model for the meaning of oligosaccharide diversity in the face of a changing world. Biochimie 83, 565–73. doi: 10.1016/S0300-9084(01)01321-9
Maroni, L., van de Graaf, S. F., Hohenester, S. D., Oude Elferink, R. P., and Beuers, U. (2015). Fucosyltransferase 2: a genetic risk factor for primary sclerosing cholangitis and Crohn's disease–a comprehensive review. Clin. Rev. Allergy Immunol. 48, 182–91. doi: 10.1007/s12016-014-8423-1
Mättö, J., Malinen, E., Suihko, M. L., Alander, M., Palva, A., Saarela, M., et al. (2004). Genetic heterogeneity and functional properties of intestinal bifidobacteria. J. Appl. Microbiol. 97, 459–70. doi: 10.1111/j.1365-2672.2004.02340.x
Matzhold, E. M., Berghold, A., Bemelmans, M. K. B., Banfi, C., Stelzl, E., Kessler, H. H., et al. (2021). Lewis and ABO histo-blood types and the secretor status of patients hospitalized with COVID-19 implicate a role for ABO antibodies in susceptibility to infection with SARS-CoV-2. Transfusion 61, 2736–45. doi: 10.1111/trf.16567
McGovern, D. P., Jones, M. R., Taylor, K. D., Marciante, K., Yan, X., Dubinsky, M., et al. (2010). Fucosyltransferase 2 (FUT2) non-secretor status is associated with Crohn's disease. Hum. Mol. Genet. 19, 3468–76. doi: 10.1093/hmg/ddq248
Miyoshi, J., Yajima, T., Okamoto, S., Matsuoka, K., Inoue, N., Hisamatsu, T., et al. (2011). Ectopic expression of blood type antigens in inflamed mucosa with higher incidence of FUT2 secretor status in colonic Crohn's disease. J. Gastroenterol. 46, 1056–63. doi: 10.1007/s00535-011-0425-7
Monedero, V., Buesa, J., and Rodríguez-Díaz, J. (2018). The interactions between host glycobiology, bacterial microbiota, and viruses in the Gut. Viruses, 10(2):96. doi: 10.3390/v10020096
Monedero, V., and Rodríguez-Díaz, J. (2016). “Chapter 62 - Intestinal microbiota and susceptibility to viral infections: role of probiotics,” in Probiotics, Prebiotics, and Synbiotics, eds R. R. Watson, V. R. Preedy. (Cambridge, MA: Academic Press). doi: 10.1016/B978-0-12-802189-7.00062-9
Needham, B. D., Funabashi, M., Adame, M. D., Wang, Z., Boktor, J. C., Haney, J., et al. (2022). A gut-derived metabolite alters brain activity and anxiety behaviour in mice. Nature 602, 647–53. doi: 10.1038/s41586-022-04396-8
Needham, B. D., Kaddurah-Daouk, R., and Mazmanian, S. K. (2020). Gut microbial molecules in behavioural and neurodegenerative conditions. Nat. Rev. Neurosci. 21, 717–31. doi: 10.1038/s41583-020-00381-0
Nielsen, S. D., Pearson, N. M., and Seidler, K. (2021). The link between the gut microbiota and Parkinson's disease: a systematic mechanism review with focus on α-synuclein transport. Brain Res. 1769, 147609. doi: 10.1016/j.brainres.2021.147609
Nishida, A., Inoue, R., Inatomi, O., Bamba, S., Naito, Y., Andoh, A., et al. (2018). Gut microbiota in the pathogenesis of inflammatory bowel disease. Clin. J. Gastroenterol. 11, 1–10. doi: 10.1007/s12328-017-0813-5
Nordgren, J., Nitiema, L. W., Ouermi, D., Simpore, J., and Svensson, L. (2013). Host genetic factors affect susceptibility to norovirus infections in Burkina Faso. PLoS ONE, 8, e69557. doi: 10.1371/journal.pone.0069557
Nordgren, J., Sharma, S., Bucardo, F., Nasir, W. G., Günaydin, D, Ouermi, L. W., et al. (2014). Both lewis and secretor status mediate susceptibility to rotavirus infections in a rotavirus genotype-dependent manner. Clin. Infect. Dis. 59, 1567–73. doi: 10.1093/cid/ciu633
Parmar, A. S., Alakulppi, N., Paavola-Sakki, P., Kurppa, K., Halme, L., Färkkilä, M., et al. (2012). Association study of FUT2 (rs601338) with celiac disease and inflammatory bowel disease in the Finnish population. Tissue Antigens. 80, 488–93. doi: 10.1111/tan.12016
Peña-Gil, N., Santiso-Bellón, C., Gozalbo-Rovira, R., Buesa, J., Monedero, V., Rodríguez-Díaz, J., et al. (2021). The role of host glycobiology and gut microbiota in rotavirus and norovirus infection, an update. Int. J. Mol. Sci. 22. doi: 10.3390/ijms222413473
Png, C. W., Lindén, S. K., Gilshenan, K. S., Zoetendal, E. G., McSweeney, C. S., Sly, L. I., et al. (2010). Mucolytic bacteria with increased prevalence in IBD mucosa augment in vitro utilization of mucin by other bacteria. Am. J. Gastroenterol. 105, 2420–8. doi: 10.1038/ajg.2010.281
Prystajecky, N., Brinkman, F. S., Auk, B., Isaac-Renton, J. L., and Tang, P. (2014). Personalized genetic testing and norovirus susceptibility. Can J. Infect. Dis. Med. Microbiol. 25, 222–4. doi: 10.1155/2014/708579
Rasko, D. A., Wang, G., Monteiro, M. A., Palcic, M. M., and Taylor, D. E. (2000). Synthesis of mono- and di-fucosylated type I Lewis blood group antigens by Helicobacter pylori. Eur. J. Biochem. 267, 6059–66. doi: 10.1046/j.1432-1327.2000.01683.x
Rausch, P., Rehman, A., Künzel, S., Häsler, R., Ott, S. J., Schreiber, S., et al. (2011). Colonic mucosa-associated microbiota is influenced by an interaction of Crohn disease and FUT2 (Secretor) genotype. Proc. Natl. Acad. Sci. U. S. A. 108, 19030–5. doi: 10.1073/pnas.1106408108
Ray, J. G., Schull, M. J., Vermeulen, M. J., and Park, A. L. (2021). Association between ABO and Rh blood groups and SARS-CoV-2 infection or severe COVID-19 illness: a population-based cohort study. Ann. Intern. Med. 174, 308–15. doi: 10.7326/M20-4511
Robilotti, E., Deresinski, S., and Pinsky, B. A. (2015). Norovirus. Clin. Microbiol. Rev. 28, 134–64. doi: 10.1128/CMR.00075-14
Rossi, O., van Berkel, L. A., Chain, F., Tanweer Khan, M., Taverne, N., Sokol, H., et al. (2016). Faecalibacterium prausnitzii A2-165 has a high capacity to induce IL-10 in human and murine dendritic cells and modulates T cell responses. Sci. Rep. 6, 18507. doi: 10.1038/srep18507
Rouquier, S., Lowe, J. B., Kelly, R. J., Fertitta, A. L., Lennon, G. G., Giorgi, D., et al. (1995). Molecular cloning of a human genomic region containing the H blood group alpha(1,2)fucosyltransferase gene and two H locus-related DNA restriction fragments. Isolation of a candidate for the human Secretor blood group locus. J. Biol. Chem. 270, 4632–9. doi: 10.1074/jbc.270.9.4632
Scaldaferri, F., Gerardi, V., Mangiola, F., Lopetuso, L. R., Pizzoferrato, M., Petito, V., et al. (2016). Role and mechanisms of action of Escherichia coli Nissle 1917 in the maintenance of remission in ulcerative colitis patients: an update. World J. Gastroenterol. 22, 5505–11. doi: 10.3748/wjg.v22.i24.5505
Sekizuka, T., Ogasawara, Y., Ohkusa, T., and Kuroda, M. (2017). Characterization of Fusobacterium varium Fv113-g1 isolated from a patient with ulcerative colitis based on complete genome sequence and transcriptome analysis. PLoS ONE 12, e0189319. doi: 10.1371/journal.pone.0189319
Sepehri, S., Khafipour, E., Bernstein, C. N., Coombes, B. K., Pilar, A. V., Karmali, M., et al. (2011). Characterization of Escherichia coli isolated from gut biopsies of newly diagnosed patients with inflammatory bowel disease. Inflamm. Bowel. Dis. 17, 1451–63. doi: 10.1002/ibd.21509
Sharma, S., Hagbom, M., Svensson, L., and Nordgren, J. (2020). The impact of human genetic polymorphisms on rotavirus susceptibility, epidemiology, and vaccine take. Viruses 12(3):324. doi: 10.3390/v12030324
Shokri, P., Golmohammadi, S., Noori, M., Nejadghaderi, S. A., Carson-Chahhoud, K., Safiri, S., et al. (2022). The relationship between blood groups and risk of infection with SARS-CoV-2 or development of severe outcomes: a review. Rev. Med. Virol. 32, e2247. doi: 10.1002/rmv.2247
Soejima, M., Fujimoto, R., Agusa, T., Iwata, H., Fujihara, J., Takeshita, H., et al. (2012). Genetic variation of FUT2 in a Vietnamese population: identification of two novel Se enzyme-inactivating mutations. Transfusion 52, 1268–75. doi: 10.1111/j.1537-2995.2011.03485.x
Soejima, M., and Koda, Y. (2020). FUT2 polymorphism in Latin American populations. Clin. Chim. Acta 505, 1–5. doi: 10.1016/j.cca.2020.02.011
Soejima, M., and Koda, Y. (2021a). Estimation of secretor status of ABO antigens by high-resolution melting analysis of rs601338 (428G > A). Clin. Chim. Acta 517, 86–91. doi: 10.1016/j.cca.2021.02.019
Soejima, M., and Koda, Y. (2021b). Survey and characterization of nonfunctional alleles of FUT2 in a database. Sci. Rep 11, 3186. doi: 10.1038/s41598-021-82895-w
Soejima, M., Nakajima, T., Fujihara, J., Takeshita, H., and Koda, Y. (2008). Genetic variation of FUT2 in ovambos, turks, and mongolians. Transfusion 48, 1423–31. doi: 10.1111/j.1537-2995.2008.01710.x
Sun, M. F., and Shen, Y. Q. (2018). Dysbiosis of gut microbiota and microbial metabolites in Parkinson's disease. Ageing Res. Rev. 45, 53–61. doi: 10.1016/j.arr.2018.04.004
Takahashi, K., Nishida, A., Fujimoto, T., Fujii, M., Shioya, M., Imaeda, H., et al. (2016). Reduced abundance of butyrate-producing bacteria species in the fecal microbial community in Crohn's disease. Digestion 93, 59–65. doi: 10.1159/000441768
Tan, M., and Jiang, X. (2014). Histo-blood group antigens: a common niche for norovirus and rotavirus. Expert. Rev. Mol. Med. 16, e5. doi: 10.1017/erm.2014.2
Tang, X., Wang, W., Hong, G., Duan, C., Zhu, S., Tian, Y., et al. (2021). Gut microbiota-mediated lysophosphatidylcholine generation promotes colitis in intestinal epithelium-specific Fut2 deficiency. J. Biomed. Sci., 28, 20. doi: 10.1186/s12929-021-00711-z
Tarris, G., Rougemont, A. d. e., Charkaoui, M., Michiels, C., Martin, L., Belliot, G., et al. (2021). Enteric viruses and inflammatory bowel disease. Viruses. 13(1):104. doi: 10.3390/v13010104
Thorven, M., Grahn, A., Hedlund, K. O., Johansson, H., Wahlfrid, C., Larson, G., et al. (2005). A homozygous nonsense mutation (428G–>A) in the human secretor (FUT2) gene provides resistance to symptomatic norovirus (GGII) infections. J. Virol. 79, 15351–5. doi: 10.1128/JVI.79.24.15351-15355.2005
Tian, L., Song, N., Yao, Z. Q., Li, X. J., and Zhang, R. (2014). Sequence analysis of the human fucosyltransferase 1 and 2 genes in Tibetan blood donors: identification of three novel alleles. Transfusion 54, 1847–50. doi: 10.1111/trf.12577
Tong, M., McHardy, I., Ruegger, P., Goudarzi, M., Kashyap, P. C., Haritunians, T. Jr., et al. (2014). Reprograming of gut microbiome energy metabolism by the FUT2 Crohn's disease risk polymorphism. Isme J. 8, 2193–206. doi: 10.1038/ismej.2014.64
Torres-Alarcón, C. G., García-Ruíz, A., Cañete-Ibáñez, C. R., Pogoda Morales, I. I., Muñoz-Arce, C. M., Cid-Domínguez, B. E., et al. (2021). Blood system ABO antigens as risk factor for severity of SARS-CoV-2 infection. Gac. Med. Mex. 157, 174–80. doi: 10.24875/GMM.20000498
Valenti, L., Villa, S., Baselli, G., Temporiti, R., Bandera, A., Scudeller, L., et al. (2020). Association of ABO blood group and secretor phenotype with severe COVID-19. Transfusion 60, 3067–70. doi: 10.1111/trf.16130
Vich Vila, A., Imhann, F., Collij, V., Jankipersadsing, S. A., Gurry, T., Mujagic, Z., et al. (2018). Gut microbiota composition and functional changes in inflammatory bowel disease and irritable bowel syndrome. Sci. Transl. Med. 10. doi: 10.1126/scitranslmed.aap8914
Vlasova, A. N., Shao, L., Kandasamy, S., Fischer, D. D., Rauf, A., Langel, S. N., et al. (2016). Escherichia coli Nissle 1917 protects gnotobiotic pigs against human rotavirus by modulating pDC and NK-cell responses. Eur. J. Immunol. 46, 2426–37. doi: 10.1002/eji.201646498
Wacklin, P., Mäkivuokko, H., Alakulppi, N., Nikkilä, J., Tenkanen, H., Räbinä, J., et al. (2011). Secretor genotype (FUT2 gene) is strongly associated with the composition of Bifidobacteria in the human intestine. PLoS ONE 6, e20113. doi: 10.1371/journal.pone.0020113
Wacklin, P., Tuimala, J., Nikkilä, J., Tims Sebastian, H., Mäkivuokko, N., Alakulppi, P., et al. (2014). Faecal microbiota composition in adults is associated with the FUT2 gene determining the secretor status. PLoS ONE, 9, e94863. doi: 10.1371/journal.pone.0094863
Watanabe, M., Kinoshita, H., Nitta, M., Yukishita, R., Kawai, Y., Kimura, K., et al. (2010). Identification of a new adhesin-like protein from Lactobacillus mucosae ME-340 with specific affinity to the human blood group A and B antigens. J. Appl. Microbiol. 109, 927–35. doi: 10.1111/j.1365-2672.2010.04719.x
Watanabe, Y., Nagai, F., and Morotomi, M. (2012). Characterization of Phascolarctobacterium succinatutens sp. nov., an asaccharolytic, succinate-utilizing bacterium isolated from human feces. Appl. Environ. Microbiol. 78, 511–8. doi: 10.1128/AEM.06035-11
Wick, E. C., Rabizadeh, S., Albesiano, E., Wu, X., Wu, S., Chan, J., et al. (2014). Stat3 activation in murine colitis induced by enterotoxigenic Bacteroides fragilis. Inflamm. Bowel Dis., 20, 821–34. doi: 10.1097/MIB.0000000000000019
Wu, B. B., Gu, D. Z., Yu, J. N., Yang, J., and Shen, W. Q. (2020). Association between ABO blood groups and COVID-19 infection, severity and demise: a systematic review and meta-analysis. Infect. Genet. Evol. 84, 104485. doi: 10.1016/j.meegid.2020.104485
Wu, H., Sun, L., Lin, D. P., Shao, X. X., Xia, S. L., Lv, M., et al. (2017). Association of fucosyltransferase 2 gene polymorphisms with inflammatory bowel disease in patients from Southeast China. Gastroenterol. Res. Pract. 2017, 4148651. doi: 10.1155/2017/4148651
Xavier, R. J., and Podolsky, D. K. (2007). Unravelling the pathogenesis of inflammatory bowel disease. Nature 448, 427–34. doi: 10.1038/nature06005
Yang, H., Wu, J., Huang, X., Zhou, Y., Zhange, Y, Liu, M., et al. (2022). ABO genotype alters the gut microbiota by regulating GalNAc levels in pigs. Nature 606, 358–367. doi: 10.1038/s41586-022-04769-z
Ye, Q., and Yu, J. (2021). A study on fucosyltransferase 2 gene polymorphism and secretion status related to neonatal necrotizing enterocolitis. J. Healthc. Eng., 2021, 7219850. doi: 10.1155/2021/7219850
Yi, W., Shao, J., Zhu, L., Li, M., Singh, M., Lu, Y., et al. (2005). Escherichia coli O86 O-antigen biosynthetic gene cluster and stepwise enzymatic synthesis of human blood group B antigen tetrasaccharide. J. Am. Chem. Soc., 127, 2040–1. doi: 10.1021/ja045021y
Keywords: FUT2, histoblood group antigens, inflammatory bowel disease, intestinal microbiota, ulcerative colitis (UC), Crohn's disease
Citation: Hu M, Zhang X, Li J, Chen L, He X and Sui T (2022) Fucosyltransferase 2: A Genetic Risk Factor for Intestinal Diseases. Front. Microbiol. 13:940196. doi: 10.3389/fmicb.2022.940196
Received: 11 May 2022; Accepted: 20 June 2022;
Published: 18 July 2022.
Edited by:
George Grant, University of Aberdeen, United KingdomReviewed by:
Alfredo Miccheli, Sapienza University of Rome, ItalyCopyright © 2022 Hu, Zhang, Li, Chen, He and Sui. This is an open-access article distributed under the terms of the Creative Commons Attribution License (CC BY). The use, distribution or reproduction in other forums is permitted, provided the original author(s) and the copyright owner(s) are credited and that the original publication in this journal is cited, in accordance with accepted academic practice. No use, distribution or reproduction is permitted which does not comply with these terms.
*Correspondence: Tingting Sui, suitingting@jlu.edu.cn
†These authors have contributed equally to this work