- School of Public Health, Qingdao University, Qingdao, China
Bacterial vaginosis (BV) is a common vaginal infection and has been associated with increased risk for a wide array of health issues. BV is linked with a variety of heterogeneous pathogenic anaerobic bacteria, among which Mobiluncus is strongly associated with BV diagnosis. However, their genetic features, pathogenicity, interspecific diversity, and evolutionary characters have not been illustrated at genomic level. The current study performed phylogenomic and comparative genomic analyses of Mobiluncus. Phylogenomic analyses revealed remarkable phylogenetic distinctions among different species. Compared with M. curtisii, M. mulieris had a larger genome and pangenome size with more insertion sequences but less CRISPR-Cas systems. In addition, these two species were diverse in profile of virulence factors, but harbored similar antibiotic resistance genes. Statistically different functional genome profiles between strains from the two species were determined, as well as correlations of some functional genes/pathways with putative pathogenicity. We also showed that high levels of horizontal gene transfer might be an important strategy for species diversification and pathogenicity. Collectively, this study provides the first genome sequence level description of Mobiluncus, and may shed light on its virulence/pathogenicity, functional diversification, and evolutionary dynamics. Our study could facilitate the further investigations of this important pathogen, and might improve the future treatment of BV.
Introduction
Bacterial vaginosis (BV), a common gynecological disease characterized by vaginal discharge, affects roughly a quarter of women worldwide and costs an estimated $4.8 billion annually (Sobel, 2000; Javed et al., 2019; Peebles et al., 2019). BV has been associated with an increased risk of various health problems, including sexually transmitted infections, adverse pregnancy outcomes (e.g., preterm births, premature rupture of membranes), pelvic inflammatory disease, increased susceptibility to HIV infection, and other chronic health issues (Taha et al., 1998; Schwebke and Desmond, 2005; Onderdonk et al., 2016). The pathogenesis of BV is still a subject of debate (Cherpes et al., 2008; Muzny and Schwebke, 2016), whereas it has been determined that BV is characterized by a decrease in the levels of Lactobacilli and an overgrowth of opportunistic bacteria including anaerobes or microaerophiles such as Prevotella, Gardnerella, and Mobiluncus genera (Hillier, 1993; Sha et al., 2005). Among these, the abundance of Gram-negatively stained and curved rod-shaped bacteria, represented by Mobiluncus, has been considered as one of the key indicators of Nugent score, the “gold standard” for BV diagnosis (Nugent et al., 1991; Srinivasan et al., 2013).
Mobiluncus organisms were initially recognized in vaginal fluid as early as 1895 and were first isolated in 1913 (Curtis, 1913). Nowadays, much interest has revolved around Mobiluncus since women with higher Nugent scores (predominantly because of morphotypes consistent with Mobiluncus) are more likely to fail therapy than those with lower scores (Schwebke and Desmond, 2007), and the presence and persistence of Mobiluncus spp. was found to be highly associated with recurrence of BV (Meltzer et al., 2008). In addition, the production of malic acid and trimethylamine by Mobiluncus strains have been reported to give rise to vaginal irritation and unpleasant odor (Africa et al., 2014). For these reasons, more efforts have been made to characterize their resistance mechanisms and virulence factors (Spiegel, 1987; Zeng et al., 2020; Zhang et al., 2020). Nevertheless, the definite role of Mobiluncus in BV pathogenesis still remains largely elusive.
Analysis of the 16S rRNA gene sequences has revealed that Mobiluncus genus mainly contains two distantly related species, M. curtisii and M. mulieris. Previous reports from a number of laboratories have shown that these two species can be differentiated based on physical and biochemical properties. It has been demonstrated that M. curtisii and M. mulieris comprise short curved and long straight (or slightly curved) rods (Hoyles et al., 2004; Onderdonk et al., 2016), respectively, and show variation in growth in different liquid media (Taylor-Robinson and Taylor-Robinson, 2002). In addition, antigenic profiles of the two species are also distinct (Roberts et al., 1985; Gatti et al., 1997), and M. mulieris can stimulate a TLR5-mediated response in host, while M. curtisii cannot (Dela Cruz et al., 2021). Antimicrobial susceptibility and clonality of Mobiluncus also vary widely among species and even strains (Spiegel, 1987; Zhang et al., 2020). Recently, a new species collected from pig gut, namely M. porci, has been described (Wylensek et al., 2020). However, these studies were mostly based on phenotypic data, while genomic features, including genetic diversity and evolutionary history, of/between Mobiluncus species have not been clearly elucidated yet.
In the present study, we carried out an in-depth comparative genomic analysis of 38 publicly available genomic sequences of Mobiluncus, aiming to investigate the genomic diversity of this taxon. We compared the status of various virulence and antibiotic resistance genes (ARGs) among the strains in order to unleash the potential underlying mechanism of pathogenicity and resistance. Moreover, we identified genes that may contribute to the differentiation between species, and tried to build linkages between genetic differences (gene functions and metabolic pathways) and potential pathogenicity. Finally, we uncovered the evolutionary events that may contribute to these variabilities, especially the horizontal gene transfer (HGT) events. Altogether, our study not only provides first insights into genomic features and evolution of the genus Mobiluncus, but also has implications for improved understanding of the pathogenic mechanism and putative treatment of this pathogen.
Materials and Methods
Genome Data Set
Contigs or scaffolds of the genome sequences for the members of the genus Mobiluncus were downloaded from the NCBI genomes FTP site (April 2021)1. To avoid bias, genomes with estimated contamination > 5% or completion < 95% were excluded based on CheckM results (Parks et al., 2015). Taxonomy assignment of these genomes was performed by the Genome Taxonomy Database (GTDB) toolkit (Chaumeil et al., 2019) and based on LPSN (List of Prokaryotic names with Standing in Nomenclature) database (Parte, 2018). Contigs of different species were reordered using the Move Contig tool in Mauve software (Darling et al., 2010) against the complete genome of the M. curtisii ATCC 43063 and M. mulieris DSM 2710, respectively. Pairwise genome alignment was carried out by the lastz program2, and the results were visualized using AliTV (Ankenbrand et al., 2017). Pairwise whole genome average nucleotide identity (ANI) values were computed by FastANI (Jain et al., 2018).
Genome Annotation
All genomes were reannotated using Prokka with default settings (Seemann, 2014). Functional annotation and classification of proteins were performed by sequence comparison using DIAMOND BLASTP (E-value 1e-05, coverage 0.5, and identity 40%) (Buchfink et al., 2015) against the recently updated Clusters of Orthologous Group (COG) database (Galperin et al., 2021). KEGG Automated Annotation Server (KAAS) (Moriya et al., 2007) was used for pathway mapping of species-specific genes. Insertion sequences (ISs) were identified by BLASTN against the ISFinder database (E-value 1e-05) (Siguier et al., 2006). The prediction of clustered regularly interspaced short palindromic repeat (CRISPR) in the genome was assessed by the CRISPRCasFinder tool (Couvin et al., 2018), and only CRISPRs classified with evidence levels 3 and 4 were considered. Potential ARGs and putative virulence factors (VFs) encoded in genomes were identified through BLASTP searches of the Comprehensive Antibiotic Resistance Database (CARD) (Alcock et al., 2020) and the Virulence Factors Database (VFDB) (Liu et al., 2019), respectively. The most differentiating COG entries between M. curtisii and M. mulieris were determined by SIMPER analysis by using “simper” function of the vegan R package3. “ordinate” function in the phyloseq R package (McMurdie and Holmes, 2013) was used to determine the variation in the functional profiles of HGT genes with non-metric multidimensional scaling (NMDS) analysis on BrayCurtis dissimilarity matrices. Pairwise comparisons of species-specific genomic regions were visualized by EasyFig software (Sullivan et al., 2011).
Pan-Genome and Phylogenomic Analyses
Homologous gene families were calculated using GET_HOMOLOGUES (Contreras-Moreira and Vinuesa, 2013) with the OrthoMCL clustering algorithm, and cloud, shell, and (soft-) core pangenome components were also derived. Pan-genome statistics were computed by PanGP (Zhao et al., 2014). Marker-based phylogenetic tree of the genus Mobiluncus was constructed by the GET_PHYLOMARKERS pipelines (Vinuesa et al., 2018) running in default mode based on nucleotide sequences of 329 high-quality marker genes. In addition. an absence/presence (0/1) matrix of dispensable genes was built according to GET_HOMOLOGUES results, and was subjected to R package pvclust (Suzuki and Shimodaira, 2006) for hierarchical clustering analysis with 1,000 bootstrap replicates with two types of p-values: AU (approximately unbiased) p-value and BP (bootstrap probability) value.
Identification of Potential Horizontal Genes
In order to predict the gain and loss of each homologous gene family across ancestral nodes during the evolution of Mobiluncus, the pangenome matrix and the rooted phylogenomic tree were used as inputs for COUNT software (Csûrös, 2010) to calculate posterior probabilities (cut-off was set at 70%). We also used HGTector software (Zhu et al., 2014) to detect genes in each genome that were potentially acquired through HGT. During this process, quality cutoffs for DIAMOND BLASTP results were E-value ≤ 1e-05, sequence identity ≥ 50%, and coverage of query sequence ≥ 50%. Mobiluncus (rank, genus; taxon identifier 2050) was set as the self group, and Actinomycetaceae (rank, family; taxon identifier 2049) was set as the close group.
Results
Phylogeny and Genome Overview of the Genus Mobiluncus
All 40 Mobiluncus genomes were downloaded from GenBank database (April 2021). Two genomes (strain FDAARGOS_303 and strain NCTC11820) were filtered out as they contained more contamination (8.53 and 11.87%, respectively). Therefore, a total of 38 high-quality Mobiluncus genomes were analyzed in this study, including four complete and 34 draft genome sequences (Supplementary Table 1). Based on the similarity observed by GTDB-tk, two valid species are present within the genus, with 18 genomes belonging to M. curtisii and 19 genomes belonging to M. mulieris. All of these strains were isolated from human vagina (except for two with missing data). In addition, based on LPSN database, strain RF-GAM-744-WT-7 (isolated from pig feces) was classified as M. porci (Wylensek et al., 2020). To further elucidate their genetic relatedness, a genome-wide ANI plot was generated (Figure 1A). The intraspecies ANI values of M. curtisii and M. mulieris were higher than 95.7 and 97.4%, respectively, which exceeded the recommended 95% threshold value for intraspecific prokaryotic strains (Richter and Rossello-Mora, 2009), while the inter-species values were lower than 78.4%. To further evaluate the intra-genus differentiation and evolutionary relationships within the genus, phylogenomic reconstruction was performed based on 329 high-quality phylogenomic marker (core) genes (Figure 2A). The resulting tree shows two major clades corresponding to M. curtisii and M. mulieris species, and one distinct branch formed by the single strain M. porci RF-GAM-744-WT-7 clustering with M. mulieris. A hierarchical clustering tree based on the content of dispensable genes showed similar topological structure (Figure 2B), but with M. porci RF-GAM-744-WT-7 located on the outskirt of M. curtisii. Given the representativeness, the following analyses will focus more on the two validly published BV-associated species, M. curtisii and M. mulieris.
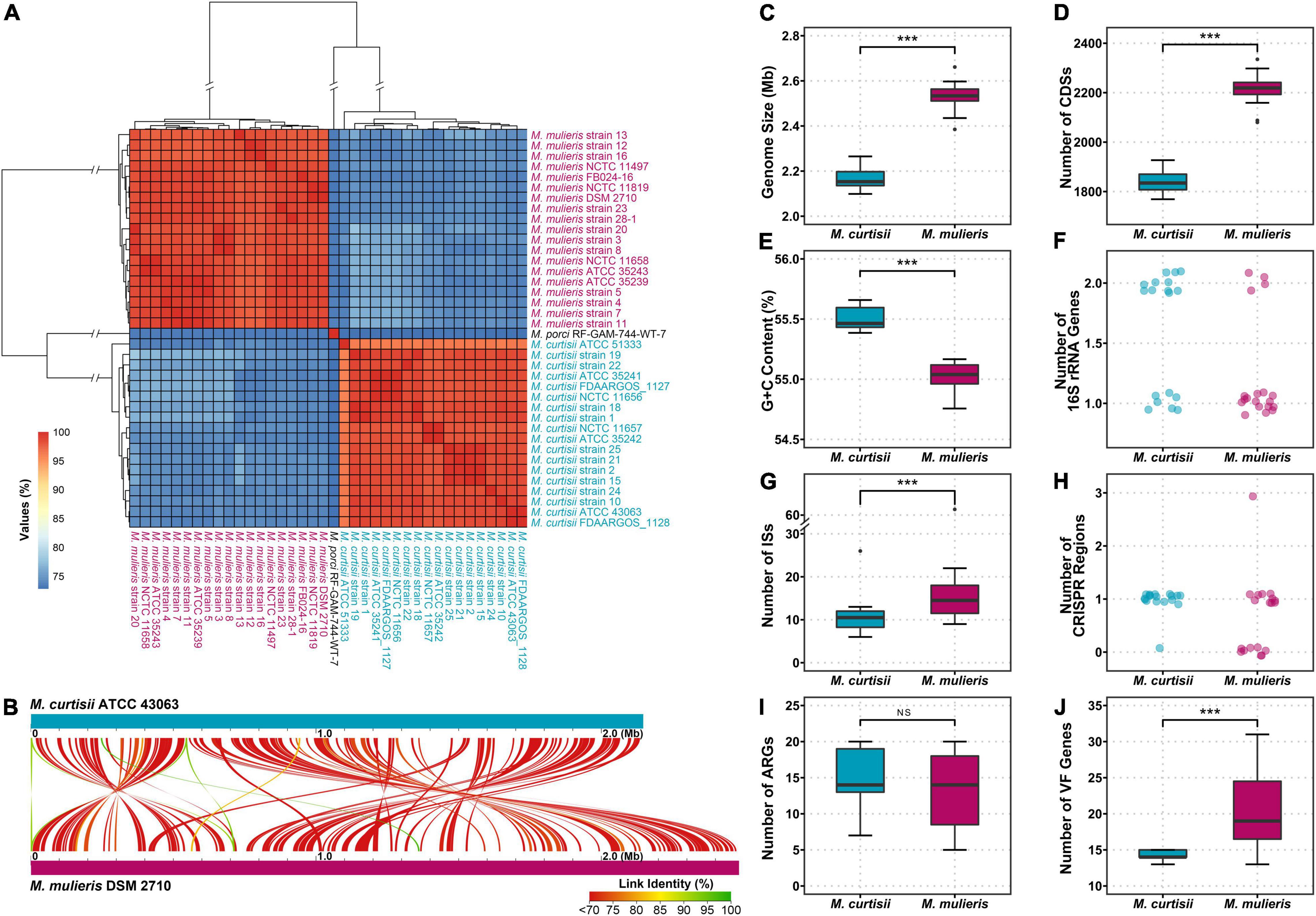
Figure 1. Genomic features of the genus Mobiluncus. (A) Hierarchical clustering based on ANI values of 38 Mobiluncus strains. (B) Whole genome alignment of M. curtisii and M. mulieris. Color denotes percent similarity of links (see legend). (C–J) Comparison of genomic characteristics between M. curtisii and M. mulieris. The boxplot shows the median, and the first and third quartiles as the lower and upper hinges. Outliers are indicated as dots. Asterisks (***) indicate significant differences (significance level of 0.001, Wilcoxon test). NS, not significant; ISs, insertion sequences; CRISPR, clustered regularly interspaced short palindromic repeat; ARGs, antibiotic resistance genes; VF, virulence factor.
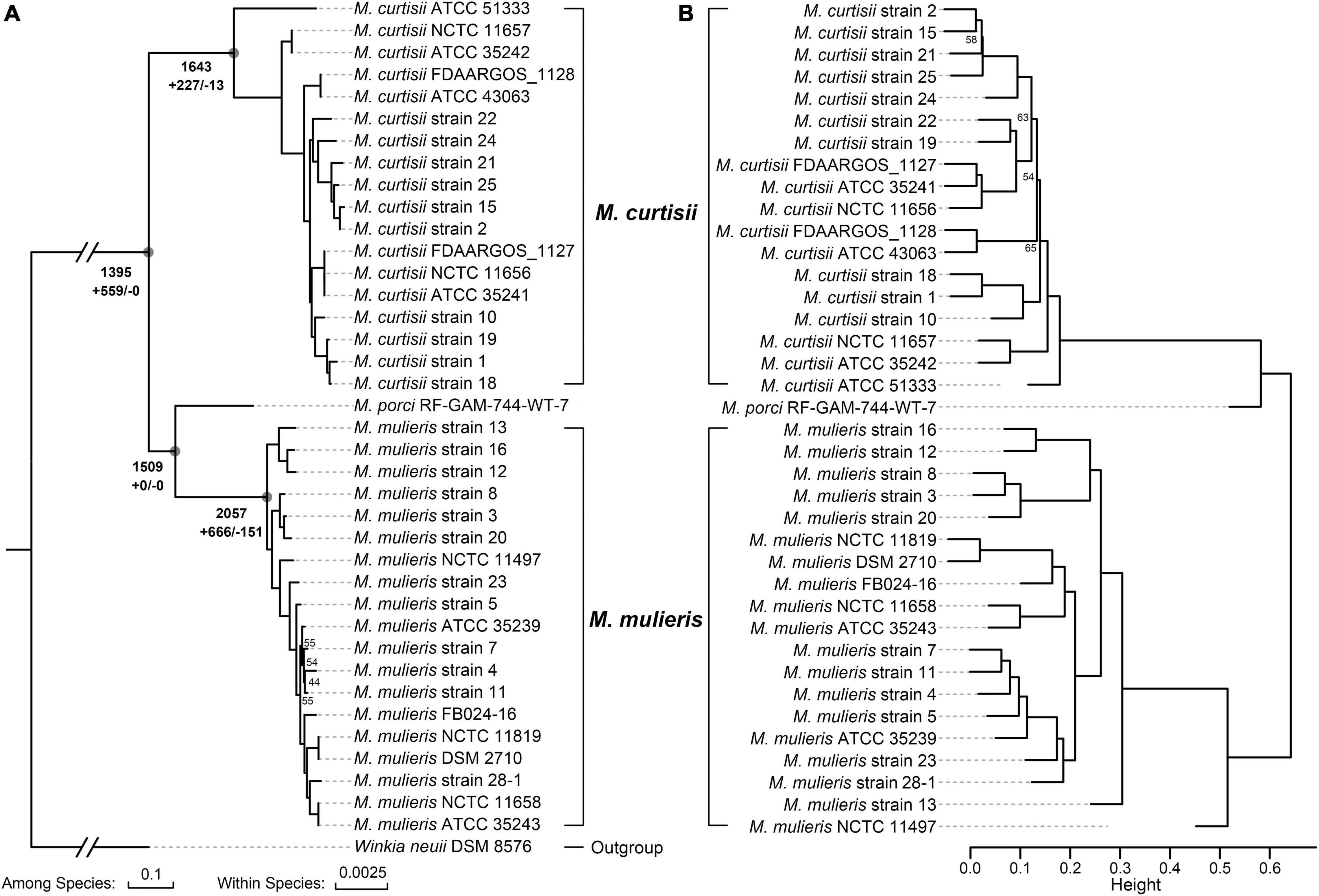
Figure 2. Phylogeny of the genus Mobiluncus. (A) Maximum-likelihood phylogenomic tree. The tree was constructed based on 329 core genes that could give a well-resolved tree topology without recombination. The total numbers of ancestral orthologous genes present at each of the nodes are shown next to the deep nodes, and the numbers of gene gain (+) and loss (–) events are indicated below. Bootstrap values less than 70% are shown at the nodes. The scale bars indicate 10 and 0.25% sequence divergences among and within species, respectively. (B) Hierarchical cluster analysis based on the presence or absence of dispensable genes. Height indicates the dissimilarity between genomes. BP (bootstrap probability) values less than 70% from 1,000 replicates are shown, and all AU (approximately unbiased) p-values are > 70%.
The characteristics of the genomes studied here are shown in Figures 1B–H and Supplementary Table 1. The average genome size of M. curtisii was 2.17 Mbp and was significantly less than M. mulieris at 2.53 Mbp (Wilcoxon test, p < 0.001). Consequently, M. curtisii on average contained fewer protein coding genes at 1,844 compared to M. mulieris at 2,213 (Wilcoxon test, p < 0.001), and between species showed collinearity with abundant gene arrangement. GC content levels were similar between species, with an average of 55.3%, although M. curtisii had a slightly larger GC content than M. mulieris (mean, 55.5 and 55.0%, respectively; Wilcoxon test, p < 0.001). Strikingly, 11 of 18 M. curtisii strains contained two copies of 16S rRNA genes, while only 4 of 19 for M. mulieris, and the remaining strains of the two species contained only one copy (Fisher exact test, p = 0.0201). The average number of ISs per genome was 10.8 in M. curtisii while 17.8 in M. mulieris, with M. mulieris strain NCTC11497 harboring the greatest number (n = 68). CRISPR-Cas system presented in nearly all (17 of 18) M. curtisii strains (16 TypeIE and 1 TypeIIC), but only 11 of 19 for M. mulieris (4 TypeIE and 7 TypeIIC) (Fisher exact test, p = 0.0188). Taken together, these genomic features suggested an apparent genomic divergence between M. curtisii and M. mulieris.
Antibiotic Resistance Genes and Potential Virulence Factors of Mobiluncus
A total of 26 distinctive putative ARGs were identified. Each genome contained 14.02 ARGs averagely, and no remarkable difference in the total number or profiles of ARGs was found between M. mulieris and M. curtisii (Figures 1I, 3A). Four ARGs were shared by all Mobiluncus strains, including rpoB and rpoB2 (ARO:3004480 and ARO:3000501, respectively; both conferring resistance to rifampicin), bcrA (ARO:3002987, conferring bacitracin resistance) and mtrA (ARO:3000816, encoding a transcriptional activator of the MtrCDE multidrug efflux pump). Specifically, otrC (ARO:3002894) was found to be M. curtisii-specific, which encoded a tetracycline resistance efflux pump.
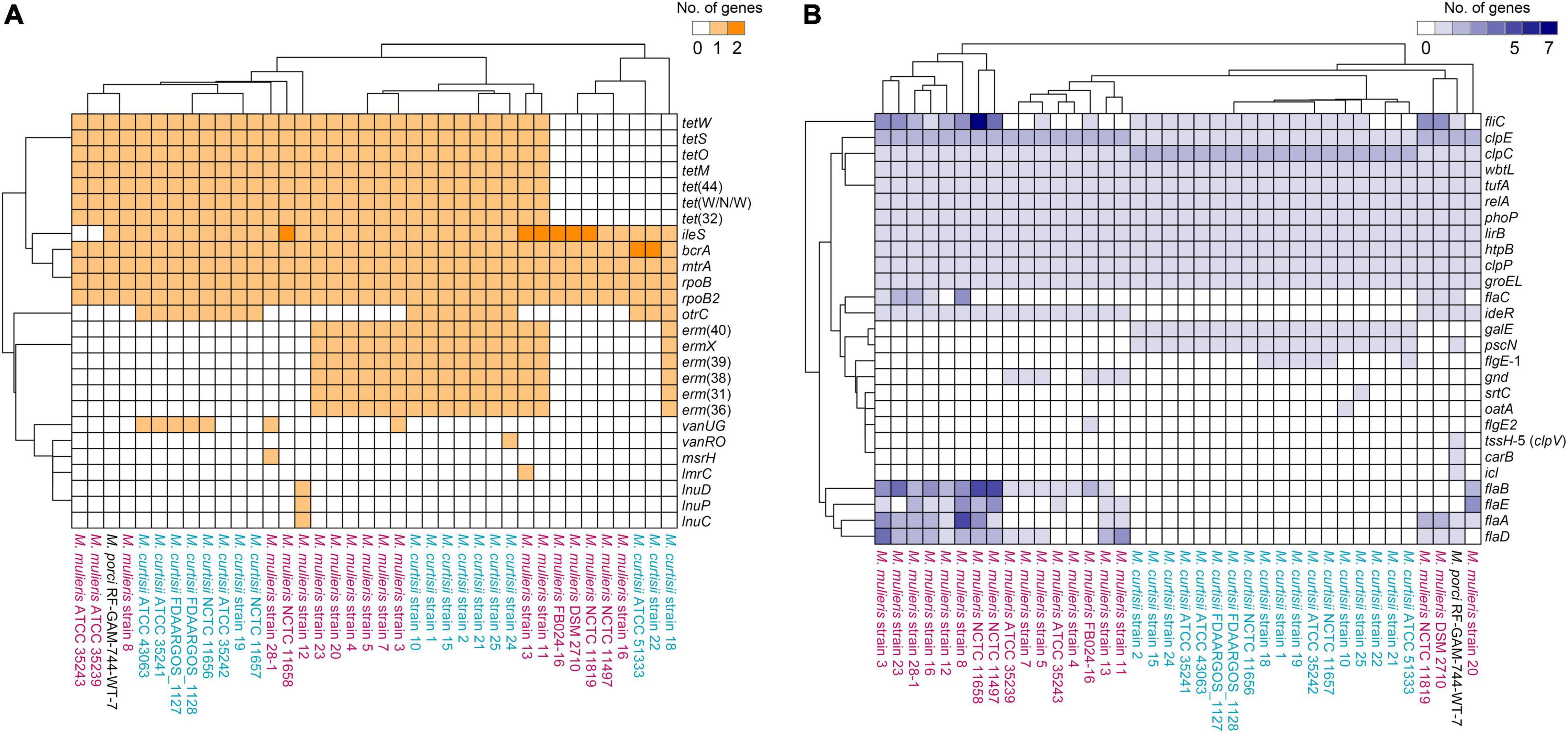
Figure 3. Hierarchically clustered heatmaps of the distributions of the putative antibiotic resistance genes (A) and virulence factors (B) in Mobiluncus genomes.
We next investigated the distribution of putative virulence genes. Overall, genomes of M. mulieris contained more VFs (mean, 20.42; median, 19) than those of M. curtisii (mean, 14.28; median, 14) (Figure 1J), and the profiles of the VFs could largely differentiate the two species (Figure 3B). Ten VFs were shared by all strains, including stress adaptation (clpC, clpE, and clpP), regulation (phoP and relA), adherence (groEL and tufA), secretion (lirB), immune evasion (wbtL) and others (htpB), suggesting they might play key roles in pathogenicity of Mobiluncus strains. In addition, six VFs were prevalent in M. mulieris but absent in M. curtisii strains. Among these, five genes (flaABCDE) are associated with bacterial flagellin proteins, which serve as mediators of pathogenicity and host immune responses (Ramos et al., 2004); and the last gene is ideR, protein of which has been reported to be the key regulator of VFs and iron homeostasis in Mycobacterium tuberculosis (Pandey and Rodriguez, 2014). Moreover, there were two VFs only present in all M. curtisii strains: one is galE, encoding a UDP-galactose-4-epimerase involved in the biosynthesis of capsular or O-antigen polysaccharide units in many bacterial pathogens (Agarwal et al., 2007; Li et al., 2014); and the other is pscN, which encoded ATPase of Type III secretion system as a main VF reported in Pseudomonas aeruginosa (Lee and Rietsch, 2015; Ngo et al., 2020).
Interspecific Pangenome Variation of Mobiluncus
To explore the interspecific pangenome variation, we characterized the core and pan-genomes of M. curtisii and M. mulieris separately (Figure 4). The pangenome of M. curtisii contained 2,576 genes, whereas that of M. mulieris contained 3,507 genes. There was little difference in the core genome size (1,540 and 1,539 genes, respectively; softcore: 1,598 and 1,619 genes, respectively) between the two species. However, it revealed that the pangenome of M. curtisii comprised 450 cloud genes (accounting for 17.47% of the total genes) and 528 shell genes (20.50%), much less than those of M. mulieris (814 and 23.21%, 1,074 and 30.62%, respectively). According to a power-law regression, both species pangenomes were “open”, with Bpan = 0.19 (M. curtisii) and 0.15 (M. mulieris). Taken together, both pangenomes appeared to be boundless, while that of the M. mulieris was relatively more extensive and heterogeneous.
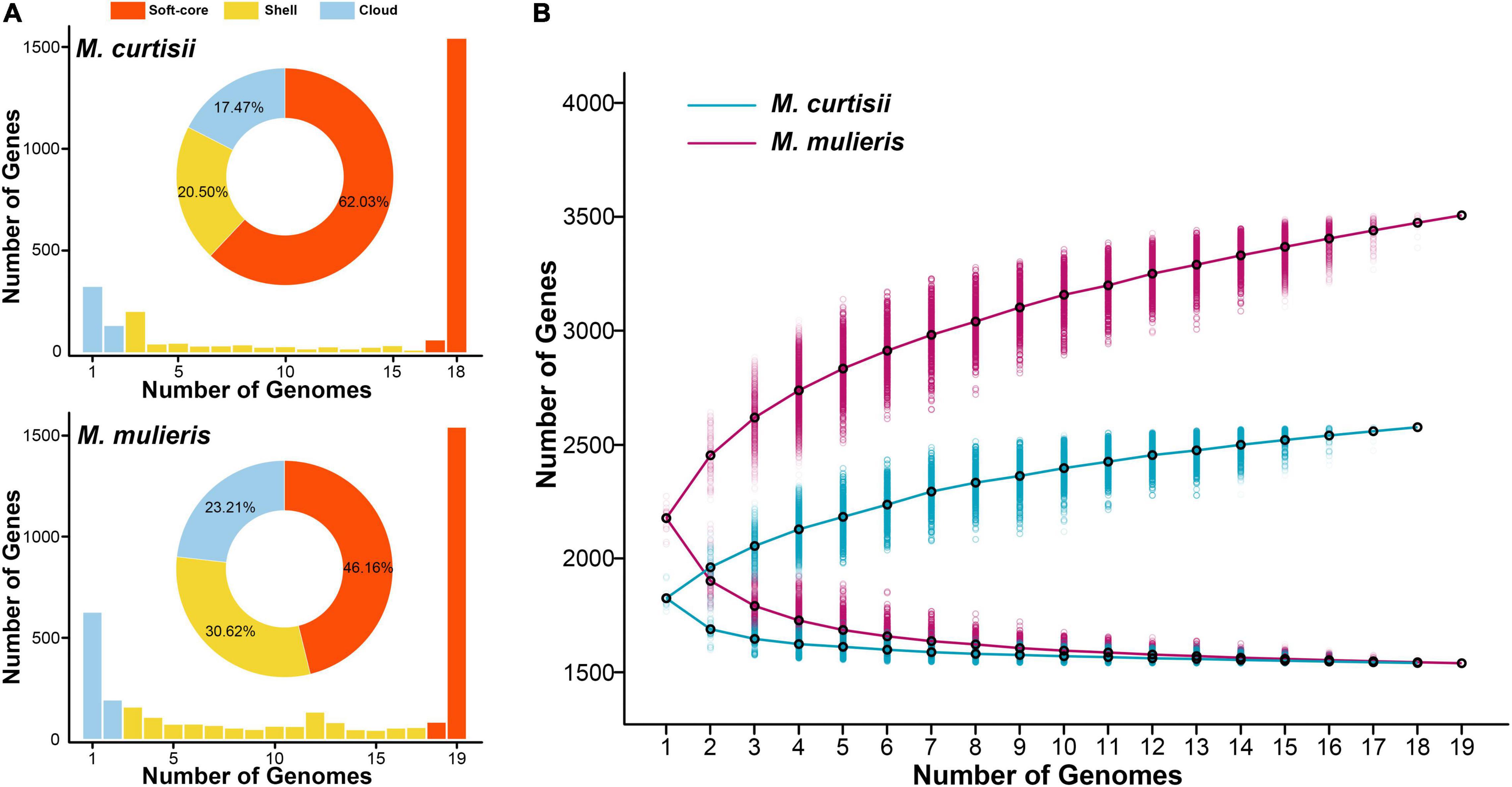
Figure 4. Pangenome summary statistics of M. curtisii and M. mulieris. (A) Histogram distributions of soft-core, shell, and cloud genes. Pie chart displays percentages of each part of the total genes. (B) The sizes of pan- and core-genomes in relation to numbers of genomes added into the gene pool.
Functional Divergence Between Mobiluncus Species
To investigate functional differentiation between M. curtisii and M. mulieris, we first explored the COG functional classification for all genes in each genome (Figure 5A). As a result, M. curtisii strains had a higher proportion of genes classified in COG categories E (amino acid transport and metabolism), H (coenzyme transport and metabolism), M (cell wall/membrane/envelope biogenesis) and P (inorganic ion transport and metabolism), while M. mulieris strains was significantly enriched for genes classified in COG categories G (carbohydrate transport and metabolism) and V (defense mechanisms). In addition, a total of 18 COGs were detected that significantly contributed most to the dissimilarity between species (SIMPER analysis, > 0.1% contribution, p < 0.01) (Figure 5B and Supplementary Table 2), with two-thirds were related to COGs G, V and M. For example, genes associated with cell wall binding/biosynthesis (COG2247 and COG0463) and lipoprotein transport (COG4591) are more abundant in M. curtisii, while M. mulieris strains contains more genes from RelBE/YafQ-DinJ/Txe-Axe toxin-antitoxin module (COG3077, COG2026, COG4115, and COG3041) and transmembrane proteins of sn-glycerol-3-phosphate transport system (COG0395 and COG1175).
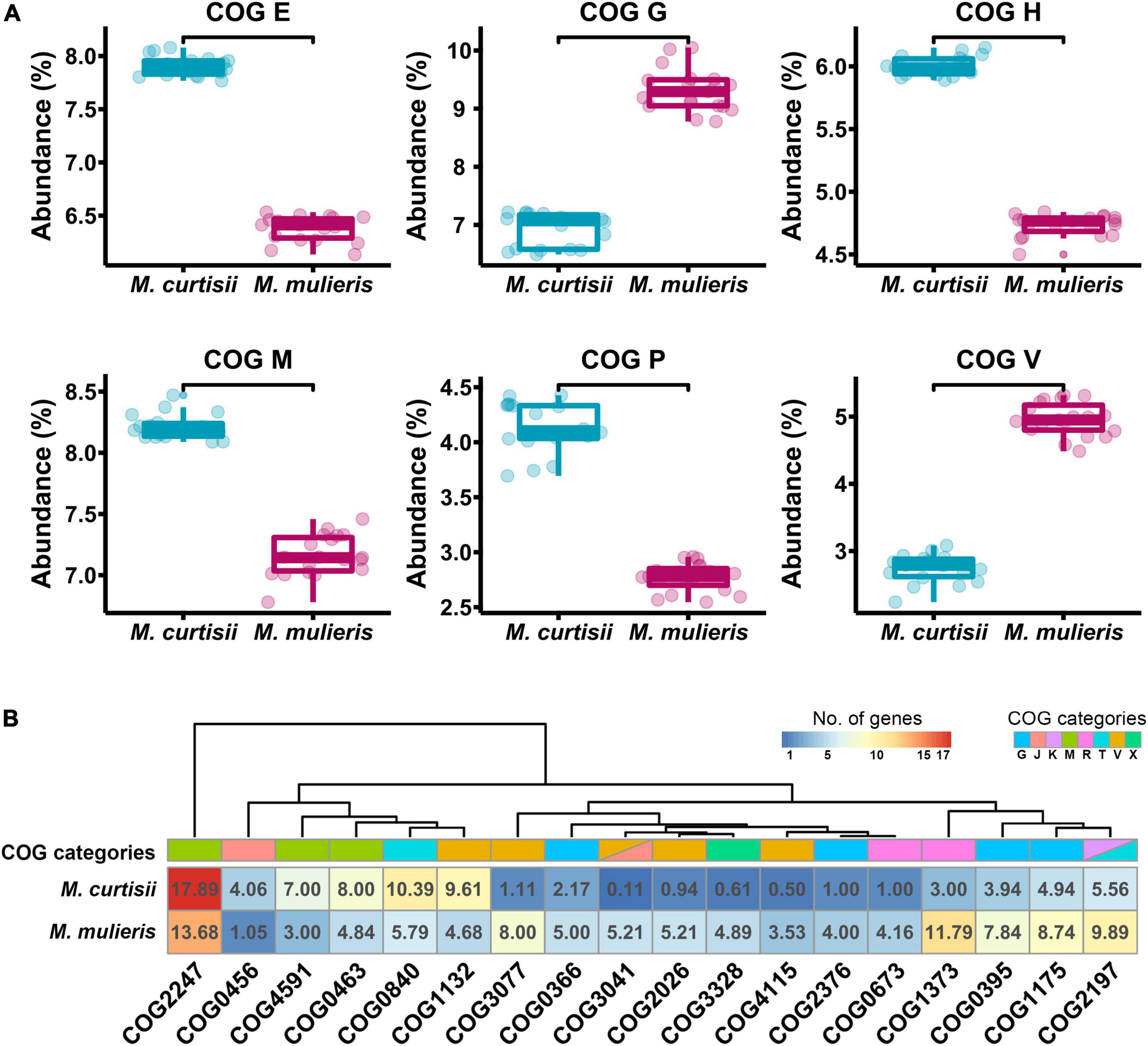
Figure 5. Functional divergences between M. curtisii and M. mulieris. (A) Boxplot of abundance of differential COG categories. All the distributions were significantly different (Wilcoxon test, p < 10-5). (B) Heatmap of COGs that significantly contributed most to the dissimilarity between M. curtisii and M. mulieris. Different COG categories are shown in different colors.
To further investigate functional differentiation between the species, we explored the species-specific genes that are universal (> 90%) in one species but absent in the other. We found 385 and 429 orthologous genes (OGs) that were specific to M. curtisii and M. mulieris, respectively (Supplementary Table 3). Some of the OGs were located physically adjacent and clustered into genomic regions (Figure 6), which might perform certain complicated or special roles in extending the metabolic/pathogenic pathways. One such region was composed of several arginine biosynthetic genes. Interestingly, besides operon argDRGH, within the region genomes of M. curtisii also contained genes of argCJB, whereas M. mulieris lacked but instead harbored operon carAB elsewhere, which has been reported to be necessary for pyrimidine nucleotide and arginine biosynthesis (Han and Turnbough, 1998). Another region contained two pathways, one was involved in the molybdopterin biosynthesis, encoding an ABC-type molybdate transport system and a biosynthetic gene cluster of molybdenum cofactor (MoCo), and another associated with nitrate respiration, encoding a nitrate reductase operon narKGHJI (only present in 73.7% M. curtisii strains). Moreover, although all genomes had a series of genes related to histidine biosynthesis, another eight his genes (hisF, hisI, hisG, hisA, hisH, hisB, hisC, and hisD) were unique to M. curtisii strains. Similarly, operon nadABC existed only in M. curtisii, enabling them biosynthesize NAD+ in both the salvage and the de novo pathways. Another M. curtisii-specific region contained genes of LIV system, which is responsible for the transport of branched-chain amino acids, such as leucine, isoleucine, and valine (Adams et al., 1990).
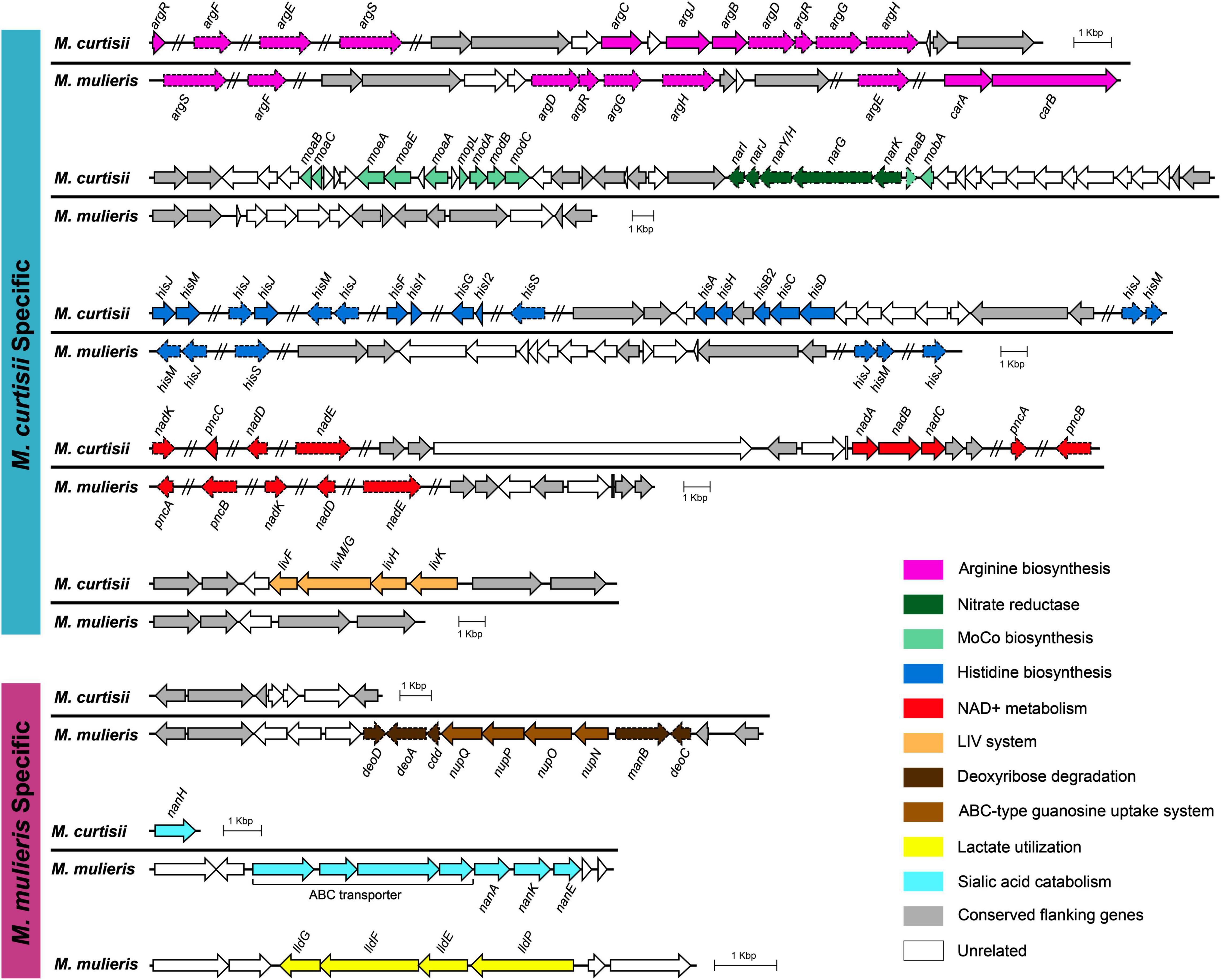
Figure 6. Genetic organizations of the regions containing species-specific genes. Only genes that are universal (> 90%) in one species but absent in the other are determined as species-specific genes. Conserved genomic regions flanking these genes are colored in gray. Non-specific genes are marked with dashed lines. MoCo, molybdenum cofactor.
We also detected three M. mulieris-specific genomic regions. One region was composed of genes of an ABC-type guanosine uptake system NupNOPQ, which inserted in an operon deoD-deoA-cdd-manB-DeoC, an unusual deoCABD-like operon perhaps serving as deoxynucleotide degradation also found in Mollicutes and other pathogens (Christensen et al., 2003; Bizarro and Schuck, 2007). The second was composed of a complete sialic acid catabolic gene cluster nanAKE. Interestingly, although M. curtisii did not contain the cluster, a sialidase gene (nanH) was found to be M. curtisii-specific. We also detected a lldPEFG operon (orthologs of lutABC and lctP) in M. mulieris, which was implicated in lactate utilization and has been reported to be involved in biofilm formation and pathogenesis in many pathogenic bacteria (Chai et al., 2009; Jiang et al., 2014).
Gain and Loss of Genes During the Evolution of Mobiluncus
To decipher the evolutionary history of the genus Mobiluncus, we first assessed the gain and loss events that have occurred of ancestral nodes of species on the phylogenomic tree (Figure 2A). The last common ancestor of the genus Mobiluncus was inferred to possess 1,395 gene families. Both of the M. curtisii and M. mulieris genomes have experienced a massive expansion, with 227 and 666 gene gains have been identified occurred at the divergence of the two species, with only 13 and 151 gene losses, respectively. Next, we further examined the potential horizontal genes in Mobiluncus genomes and tracked the potential donor. As a result, a total of 5,000 predicted HGT events were identified, with an average genome containing 131.58 (median, 130) horizontally transferred genes. Interestingly, although M. mulieris has a bigger genome than M. curtisii, predicted HGT events showed no significant variation between the two species (mean: 131.53 and 129.78, respectively). This may be because the transferred genes of M. mulieris comprised more shell and cloud genes (21.4%), while only 9.9% for M. curtisii. Correspondingly, 90.1% of the transferred genes of M. curtisii were soft-core genes, while only 78.6% for M. mulieris (Figure 7A). This content variation also presented at the gene family level.
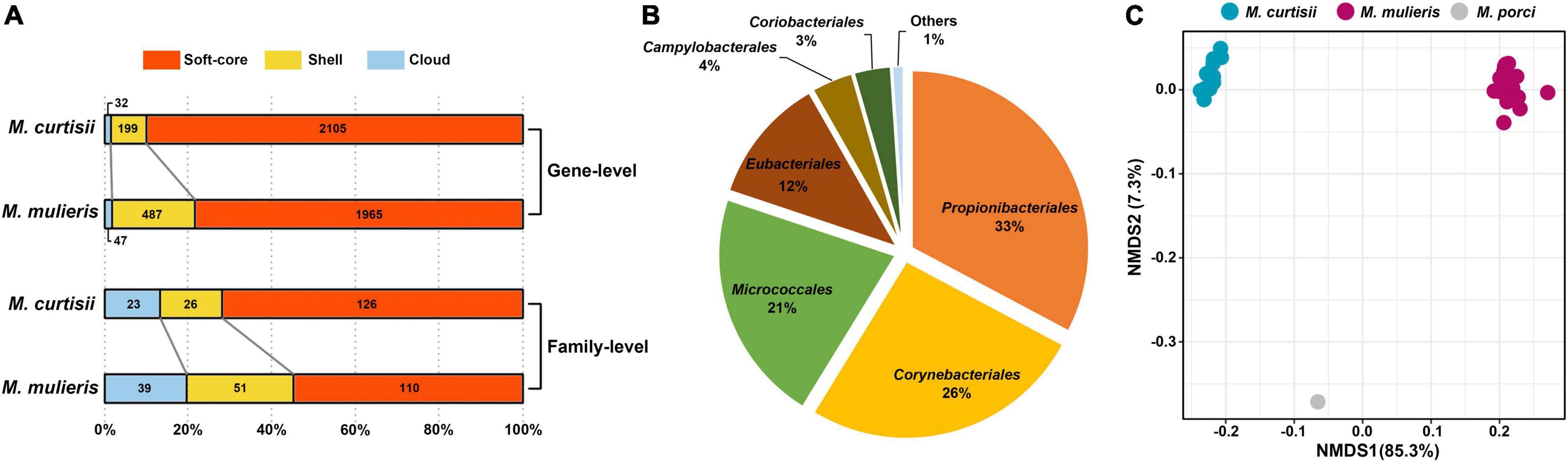
Figure 7. Analysis of predicted transferred genes. (A) Distribution of the predicted transferred genes in soft-core, shell, and cloud genes. Numbers of transferred genes and gene families are indicated. (B) Pie chart displays percentages of different donors of HGT events at order level. (C) Non-metric multidimensional scaling (NMDS) plot of COG entries showing distinct clustering of each species.
We next tracked the potential donor of the potential horizontal genes in Mobiluncus genomes. Among the 5,000 identified potential HGTs, 57.7% of the donors could be annotated at the phylum level, of which 94.7% were from Actinobacteria. The other phyla included Firmicutes (4.51%), Proteobacteria (0.76%) and Chloroflexi (0.035%). In addition, potential donor taxa of 473 genes could be identified at the order level (Figure 7B). The orders Propionibacteriales, Corynebacteriales, and Micrococcales appeared to be the main donor taxa, accounting for 80% of the total cross-order HGT genes, while order Eubacteriales was the most non-actinobacteria donor. We also revealed a different functional profile of putative transferred genes between species. M. curtisii acquired a higher proportion of genes classified in COG E (amino acid transport and metabolism), while M. mulieris was biased toward COG categories J (translation, ribosomal structure and biogenesis) and V (defense mechanisms) (Supplementary Figure 1). This result was partially consistent with the functional divergence between Mobiluncus species described above (Figure 5A). Meanwhile, based on the number of proteins annotated to each COG entries, the three species showed different functional profiles of the HGT genes (PERMANOVA test, p = 0.001; Figure 7C), implying HGT contributed to the functional divergence of Mobiluncus species.
Discussion
More than 60 years have passed since BV first described (Gardner and Dukes, 1955), and even now, its etiology and the reason for global prevalence remain unclear (Kenyon et al., 2013; Coudray and Madhivanan, 2020). Common opportunistic bacteria causing BV include Prevotella, Gardnerella vaginalis and Mobiluncus (Thorsen et al., 1998; Coudray and Madhivanan, 2020), and the abundance of Mobiluncus strains always represents a higher Nugent score and a higher possibility to fail therapy (Schwebke and Desmond, 2007; Meltzer et al., 2008). For the first time, in the current study we tried to reveal genomic details of the genus Mobiluncus, to gain more insights into the genomic features, VF and ARG profiles, functional repertoire and the evolutionary history of Mobiluncus diversification. Such information would provide theoretical foundation for further studies on the pathogenicity, therapy and discrimination of Mobiluncus species.
Efforts have been made to distinguish the two main species of Mobiluncus on the basis of morphological and biochemical differences, especially on the antigenic profiles (Roberts et al., 1984, 1985; Spiegel, 1987; Zhang et al., 2020). In this study, we performed a robust phylogenomic reconstruction to verify the degree of differentiation among species, emphasizing the genetic differences between M. curtisii and M. mulieris. We showed that the genome size of M. mulieris was relatively larger, with more gene family gains predicted across its evolution and a more open pangenome. This is consistent with the fact that M. mulieris strains comprised more ISs but less CRISPR-Cas systems within the genomes, both of which have been reported to play important roles in the bacterial genome instability (Darmon and Leach, 2014; Hatoum-Aslan and Marraffini, 2014). In addition, a genomic investigation on ARG and VF profiles showed four ARGs and ten VFs were found to be prevalent in all Mobiluncus strains, while the remaining other genes exhibited sporadic distribution patterns. Moreover, VFs profiles were able to distinguish M. curtisii from M. mulieris, whereas ARG profiles were not. Correspondingly, previous experimental studies have also revealed significant intra- and inter-species heterogeneity of antimicrobial susceptibility (Spiegel, 1987; Zhang et al., 2020). Also, it should be mentioned that although the role of Mobiluncus in the etiology and pathology of BV remains unclear, these two species may exhibit different pathogenicity and distribution during the disease process (Meltzer et al., 2008; Onderdonk et al., 2016; Arries and Ferrieri, 2022), sometimes even contradictory (Schwebke and Lawing, 2001; Salinas et al., 2020). Nevertheless, the VF and ARG profiles revealed in this work may provide guidance for the future treatment of Mobiluncus infection.
We also detected a series of metabolic pathways that showed apparent species specificity, most of which have been reported to be associated with virulence and adoption of pathogenic organisms. For example, the role of arginine biosynthesis in virulence has been reported to be crucial for full virulence of Aspergillus fumigatus in insects (Dietl et al., 2020), and we have showed that the pathway of arginine biosynthesis in M. curtisii and M. mulieris was different, perhaps suggesting a different utilizing efficiency. Furthermore, the capabilities of MoCo biosynthesis and nitrate reduction were only found in M. curtisii strains, both of which have been implicated in pathogenesis of a number of bacterial infections (Williams et al., 2011; Andreae et al., 2014; Almeida et al., 2017); more his genes were found in M. curtisii strains, perhaps enabling them capacity of biosynthesis of histidine and a crucial role in metal homeostasis and virulence (Dietl et al., 2016); and the extra NAD+ de novo biosynthesis pathway in M. curtisii could also enhance their virulence during host infection (Dom and Haesebrouck, 1992; Wang et al., 2019). On the contrary, two virulence-associated gene clusters, including genes associated with ABC-type guanosine uptake system NupNOPQ and lactate utilization, were only present in M. mulieris. Another noteworthy was the nan gene cluster for sialic acid catabolism (SAC). With these genes M. mulieris strains were more likely to consume host sialic acids as carbon source but could not cleave terminal Neu5Ac residues from host glycoconjugates (lacking nanH), whereas M. curtisii did just the opposite. This pattern perhaps implicated a cooperation between closely related species. However, this cooperation relationship seems not to be strictly necessary, as women with BV could harbor both or either of the two species (Holst, 1990). SAC associated genes have been detected in many BV-associated bacteria, which could enhance the pathogenicity of organisms by allowing easier invasion and destruction of tissues (Hardy et al., 2017; Jones, 2019; Li and Huang, 2022). These results could reinforce further discrimination of Mobiluncus species, perhaps by providing a simple and fast approach for identifying M. curtisii and M. mulieris using PCR or culture experiments, and in addition might facilitate the development of novel strategies to detect and prevent Mobiluncus infection of BV.
HGT is known to have great, perhaps the most conspicuous, impacts on bacterial diversity and speciation, especially for clinical microorganisms, where acquisition of foreign genes is crucial for pathogenicity (Smillie et al., 2011; Diard and Hardt, 2017; Arnold et al., 2021). In this study, we have used several methods to evaluate the HGT events. Firstly, both of the two Mobiluncus species have an open pangenome, which could be considered as an indicator of high HGT rates (Medini et al., 2005; Tettelin et al., 2008). Then, we reconstructed the evolutionary history of the genus. As expected, gene families undergoing gain events at ancestral nodes of species outnumbered those that experienced loss events. Therefore, differences in metabolism and pathogenicity between species have emerged. Finally, by using a BLAST-based HGT detection approach, we found that more than 5% of genes in each strain have suffered transfer events, and these genes perhaps further promoted the functional divergence between Mobiluncus species. Interestingly, no significant correlation between genome size and HGT frequency was observed, which probably means strains of M. mulieris, compared to M. curtisii, tend to acquire more dispensable genes, or meanwhile have suffered more gene loss events. Most of the transferred genes originated within the Actinobacteria phylum, with more from members of orders Propionibacteriales, Corynebacteriales, and Micrococcales. These orders have been reported to include many pathogenic species that could cause devastating diseases in humans and animals (Barka et al., 2016; Park et al., 2019), and also include microorganisms that are also present in the human vagina (Funke et al., 1997; Aleshkin et al., 2006; de Figueiredo Leite et al., 2010; Okoli et al., 2019). Taken together, these findings suggested that genome dynamic, mediated by gene gain and loss, might be an important strategy for Mobiluncus species diversification, host adaptation and pathogenicity.
Collectively, the present study largely extends the understanding of the genomic features, virulence and antibiotic resistance profiling, and evolution of the genus Mobiluncus. Our results also highlight the difference between M. curtisii and M. mulieris, providing more clues for distinguishing of the two species. Nevertheless, more experimental evidences are needed to verify these differences. Fully understanding the pathogenic potential of Mobiluncus strains remains a complex task with much to be explored in the future.
Data Availability Statement
The genomes analyzed in this study are all available in NCBI GenBank database with the accession numbers listed in Supplementary Table 1.
Author Contributions
YL designed the study, performed bioinformatic analyses, and wrote the draft manuscript. JL contributed to the conception of the study. YW and JL interpreted, discussed the results, and revised the manuscript. All authors contributed to manuscript revision and approved the submitted version.
Funding
This study was supported by the National Natural Science Foundation of China (32100002).
Conflict of Interest
The authors declare that the research was conducted in the absence of any commercial or financial relationships that could be construed as a potential conflict of interest.
Publisher’s Note
All claims expressed in this article are solely those of the authors and do not necessarily represent those of their affiliated organizations, or those of the publisher, the editors and the reviewers. Any product that may be evaluated in this article, or claim that may be made by its manufacturer, is not guaranteed or endorsed by the publisher.
Acknowledgments
We are grateful to Prof. Bin-Bin Xie (Shandong University) for his support to YL during funding application.
Supplementary Material
The Supplementary Material for this article can be found online at: https://www.frontiersin.org/articles/10.3389/fmicb.2022.939406/full#supplementary-material
Footnotes
- ^ https://www.ncbi.nlm.nih.gov/genomes
- ^ https://github.com/lastz/lastz
- ^ https://github.com/vegandevs/vegan
References
Adams, M. D., Wagner, L. M., Graddis, T. J., Landick, R., Antonucci, T. K., Gibson, A. L., et al. (1990). Nucleotide sequence and genetic characterization reveal six essential genes for the LIV-I and LS transport systems of Escherichia coli. J. Biol. Chem. 265, 11436–11443. doi: 10.1016/S0021-9258(19)38417-0
Africa, C. W., Nel, J., and Stemmet, M. (2014). Anaerobes and bacterial vaginosis in pregnancy: virulence factors contributing to vaginal colonisation. Int. J. Environ. Res. Public Health 11, 6979–7000. doi: 10.3390/ijerph110706979
Agarwal, S., Gopal, K., Upadhyaya, T., and Dixit, A. (2007). Biochemical and functional characterization of UDP-galactose 4-epimerase from Aeromonas hydrophila. Biochim. Biophys. Acta 1774, 828–837. doi: 10.1016/j.bbapap.2007.04.007
Alcock, B. P., Raphenya, A. R., Lau, T. T. Y., Tsang, K. K., Bouchard, M., Edalatmand, A., et al. (2020). CARD 2020: antibiotic resistome surveillance with the comprehensive antibiotic resistance database. Nucleic Acids Res. 48, D517–D525. doi: 10.1093/nar/gkz935
Aleshkin, V. A., Voropaeva, E. A., and Shenderov, B. A. (2006). Vaginal microbiota in healthy women and patients with bacterial vaginosis and nonspecific vaginitis. Microb. Ecol. Health Dis. 18, 71–74. doi: 10.1080/17482960600891473
Almeida, S., Sousa, C., Abreu, V., Diniz, C., Dorneles, E. M., Lage, A. P., et al. (2017). Exploration of nitrate reductase metabolic pathway in Corynebacterium pseudotuberculosis. Int. J. Genomics 2017:9481756. doi: 10.1155/2017/9481756
Andreae, C. A., Titball, R. W., and Butler, C. S. (2014). Influence of the molybdenum cofactor biosynthesis on anaerobic respiration, biofilm formation and motility in Burkholderia thailandensis. Res. Microbiol. 165, 41–49. doi: 10.1016/j.resmic.2013.10.009
Ankenbrand, M. J., Hohlfeld, S., Hackl, T., and Förster, F. (2017). AliTV—interactive visualization of whole genome comparisons. PeerJ Comput. Sci. 3:e116. doi: 10.7717/peerj-cs.116
Arnold, B. J., Huang, I. T., and Hanage, W. P. (2021). Horizontal gene transfer and adaptive evolution in bacteria. Nat. Rev. Microbiol. 20, 206–218. doi: 10.1038/s41579-021-00650-4
Arries, C., and Ferrieri, P. (2022). Mobiluncus curtisii bacteremia: case study and literature review. Infect. Dis. Rep. 14, 82–87. doi: 10.3390/idr14010009
Barka, E. A., Vatsa, P., Sanchez, L., Gaveau-Vaillant, N., Jacquard, C., Klenk, H. P., et al. (2016). Taxonomy, physiology, and natural products of Actinobacteria. Microbiol. Mol. Biol. Rev. 80, 1–43. doi: 10.1128/MMBR.00019-15
Bizarro, C., and Schuck, D. (2007). Purine and pyrimidine metabolism in Mollicutes. Genet. Mol. Biol. 30, 190–201. doi: 10.1590/S1415-47572007000200005
Buchfink, B., Xie, C., and Huson, D. H. (2015). Fast and sensitive protein alignment using diamond. Nat. Methods 12, 59–60. doi: 10.1038/nmeth.3176
Chai, Y., Kolter, R., and Losick, R. (2009). A widely conserved gene cluster required for lactate utilization in Bacillus subtilis and its involvement in biofilm formation. J. Bacteriol. 191, 2423–2430. doi: 10.1128/JB.01464-08
Chaumeil, P. A., Mussig, A. J., Hugenholtz, P., and Parks, D. H. (2019). GTDB-Tk: a toolkit to classify genomes with the genome taxonomy database. Bioinformatics 36, 1925–1927. doi: 10.1093/bioinformatics/btz848
Cherpes, T. L., Hillier, S. L., Meyn, L. A., Busch, J. L., and Krohn, M. A. (2008). A delicate balance: risk factors for acquisition of bacterial vaginosis include sexual activity, absence of hydrogen peroxide-producing lactobacilli, black race, and positive herpes simplex virus type 2 serology. Sex. Transm. Dis. 35, 78–83. doi: 10.1097/OLQ.0b013e318156a5d0
Christensen, M., Borza, T., Dandanell, G., Gilles, A. M., Barzu, O., Kelln, R. A., et al. (2003). Regulation of expression of the 2-deoxy-D-ribose utilization regulon, deoQKPX, from Salmonella enterica serovar Typhimurium. J. Bacteriol. 185, 6042–6050. doi: 10.1128/JB.185.20.6042-6050.2003
Contreras-Moreira, B., and Vinuesa, P. (2013). GET_HOMOLOGUES, a versatile software package for scalable and robust microbial pangenome analysis. Appl. Environ. Microbiol. 79, 7696–7701. doi: 10.1128/AEM.02411-13
Coudray, M. S., and Madhivanan, P. (2020). Bacterial vaginosis-A brief synopsis of the literature. Eur. J. Obstet. Gynecol. Reprod. Biol. 245, 143–148. doi: 10.1016/j.ejogrb.2019.12.035
Couvin, D., Bernheim, A., Toffano-Nioche, C., Touchon, M., Michalik, J., Neron, B., et al. (2018). CRISPRCasFinder, an update of CRISRFinder, includes a portable version, enhanced performance and integrates search for Cas proteins. Nucleic Acids Res. 46, W246–W251. doi: 10.1093/nar/gky425
Csûrös, M. (2010). Count: evolutionary analysis of phylogenetic profiles with parsimony and likelihood. Bioinformatics 26, 1910–1912. doi: 10.1093/bioinformatics/btq315
Curtis, A. H. (1913). A motile curved anaerobic Bacillus in uterine discharges. J. Infect. Dis. 12, 165–169. doi: 10.1093/infdis/12.2.165
Darling, A. E., Mau, B., and Perna, N. T. (2010). progressiveMauve: multiple genome alignment with gene gain, loss and rearrangement. PLoS One 5:e11147. doi: 10.1371/journal.pone.0011147
Darmon, E., and Leach, D. R. (2014). Bacterial genome instability. Microbiol. Mol. Biol. Rev. 78, 1–39. doi: 10.1128/MMBR.00035-13
de Figueiredo Leite, S. R., de Amorim, M. M., Calabria, W. B., de Figueiredo Leite, T. N., de Oliveira, V. S., Ferreira Junior, J. A., et al. (2010). Clinical and microbiological profile of women with bacterial vaginosis. Rev. Bras. Ginecol. Obstet. 32, 82–87. doi: 10.1590/s0100-72032010000200006
Dela Cruz, E. J., Fiedler, T. L., Liu, C., Munch, M. M., Kohler, C. M., Oot, A. R., et al. (2021). Genetic variation in Toll-like receptor 5 and colonization with flagellated bacterial vaginosis-associated bacteria. Infect. Immun. 89, e00060–20. doi: 10.1128/IAI.00060-20
Diard, M., and Hardt, W. D. (2017). Evolution of bacterial virulence. FEMS Microbiol. Rev. 41, 679–697. doi: 10.1093/femsre/fux023
Dietl, A. M., Amich, J., Leal, S., Beckmann, N., Binder, U., Beilhack, A., et al. (2016). Histidine biosynthesis plays a crucial role in metal homeostasis and virulence of Aspergillus fumigatus. Virulence 7, 465–476. doi: 10.1080/21505594.2016.1146848
Dietl, A. M., Binder, U., Bauer, I., Shadkchan, Y., Osherov, N., and Haas, H. (2020). Arginine auxotrophy affects siderophore biosynthesis and attenuates virulence of Aspergillus fumigatus. Genes (Basel) 11:423. doi: 10.3390/genes11040423
Dom, P., and Haesebrouck, F. (1992). Comparative virulence of NAD-dependent and NAD-independent Actinobacillus pleuropneumoniae strains. J. Vet. Med. B. 39, 303–306. doi: 10.1111/j.1439-0450.1992.tb01173.x
Funke, G., Hutson, R. A., Hilleringmann, M., Heizmann, W. R., and Collins, M. D. (1997). Corynebacterium lipophiloflavum sp. nov. isolated from a patient with bacterial vaginosis. FEMS Microbiol. Lett. 150, 219–224. doi: 10.1111/j.1574-6968.1997.tb10373.x
Galperin, M. Y., Wolf, Y. I., Makarova, K. S., Vera Alvarez, R., Landsman, D., and Koonin, E. V. (2021). COG database update: focus on microbial diversity, model organisms, and widespread pathogens. Nucleic Acids Res. 49, D274–D281. doi: 10.1093/nar/gkaa1018
Gardner, H. L., and Dukes, C. D. (1955). Haemophilus vaginalis vaginitis: a newly defined specific infection previously classified non-specific vaginitis. Am. J. Obstet. Gynecol. 69, 962–976. doi: 10.1016/0002-9378(55)90095-8
Gatti, M., Aschbacher, R., Cimmino, C., and Valentini, R. (1997). Antigenic profiles for the differentiation of Mobiluncus curtisii and Mobiluncus mulieris by immunoblotting technique. New Microbiol. 20, 247–252.
Han, X., and Turnbough, C. L. Jr. (1998). Regulation of carAB expression in Escherichia coli occurs in part through UTP-sensitive reiterative transcription. J. Bacteriol. 180, 705–713. doi: 10.1128/JB.180.3.705-713.1998
Hardy, L., Jespers, V., Van den Bulck, M., Buyze, J., Mwambarangwe, L., Musengamana, V., et al. (2017). The presence of the putative Gardnerella vaginalis sialidase A gene in vaginal specimens is associated with bacterial vaginosis biofilm. PLoS One 12:e0172522. doi: 10.1371/journal.pone.0172522
Hatoum-Aslan, A., and Marraffini, L. A. (2014). Impact of CRISPR immunity on the emergence and virulence of bacterial pathogens. Curr. Opin. Microbiol. 17, 82–90. doi: 10.1016/j.mib.2013.12.001
Hillier, S. L. (1993). Diagnostic microbiology of bacterial vaginosis. Am. J. Obstet. Gynecol. 169(2 Pt 2), 455–459. doi: 10.1016/0002-9378(93)90340-o
Holst, E. (1990). Reservoir of four organisms associated with bacterial vaginosis suggests lack of sexual transmission. J. Clin. Microbiol. 28, 2035–2039. doi: 10.1128/jcm.28.9.2035-2039.1990
Hoyles, L., Collins, M. D., Falsen, E., Nikolaitchouk, N., and McCartney, A. L. (2004). Transfer of members of the genus Falcivibrio to the genus Mobiluncus, and emended description of the genus Mobiluncus. Syst. Appl. Microbiol. 27, 72–83. doi: 10.1078/0723-2020-00260
Jain, C., Rodriguez, R. L., Phillippy, A. M., Konstantinidis, K. T., and Aluru, S. (2018). High throughput ANI analysis of 90K prokaryotic genomes reveals clear species boundaries. Nat. Commun. 9:5114. doi: 10.1038/s41467-018-07641-9
Javed, A., Parvaiz, F., and Manzoor, S. (2019). Bacterial vaginosis: an insight into the prevalence, alternative treatments regimen and it’s associated resistance patterns. Microb. Pathog. 127, 21–30. doi: 10.1016/j.micpath.2018.11.046
Jiang, T., Gao, C., Ma, C., and Xu, P. (2014). Microbial lactate utilization: enzymes, pathogenesis, and regulation. Trends Microbiol. 22, 589–599. doi: 10.1016/j.tim.2014.05.008
Jones, A. (2019). Bacterial vaginosis: a review of treatment, recurrence, and disparities. J. Nurse. Pract. 15, 420–423. doi: 10.1016/j.nurpra.2019.03.010
Kenyon, C., Colebunders, R., and Crucitti, T. (2013). The global epidemiology of bacterial vaginosis: a systematic review. Am. J. Obstet. Gynecol. 209, 505–523. doi: 10.1016/j.ajog.2013.05.006
Lee, P. C., and Rietsch, A. (2015). Fueling type III secretion. Trends Microbiol. 23, 296–300. doi: 10.1016/j.tim.2015.01.012
Li, C. T., Liao, C. T., Du, S. C., Hsiao, Y. P., Lo, H. H., and Hsiao, Y. M. (2014). Functional characterization and transcriptional analysis of galE gene encoding a UDP-galactose 4-epimerase in Xanthomonas campestris pv. campestris. Microbiol. Res. 169, 441–452. doi: 10.1016/j.micres.2013.08.005
Li, Y., and Huang, Y. (2022). Distribution and evolutionary history of sialic acid catabolism in the phylum Actinobacteria. Microbiol. Spectr. 10:e0238021. doi: 10.1128/spectrum.02380-21
Liu, B., Zheng, D., Jin, Q., Chen, L., and Yang, J. (2019). VFDB 2019: a comparative pathogenomic platform with an interactive web interface. Nucleic Acids Res. 47, D687–D692. doi: 10.1093/nar/gky1080
McMurdie, P. J., and Holmes, S. (2013). phyloseq: an R package for reproducible interactive analysis and graphics of microbiome census data. PLoS One 8:e61217. doi: 10.1371/journal.pone.0061217
Medini, D., Donati, C., Tettelin, H., Masignani, V., and Rappuoli, R. (2005). The microbial pan-genome. Curr. Opin. Genet. Dev. 15, 589–594. doi: 10.1016/j.gde.2005.09.006
Meltzer, M. C., Desmond, R. A., and Schwebke, J. R. (2008). Association of Mobiluncus curtisii with recurrence of bacterial vaginosis. Sex. Transm. Dis. 35, 611–613. doi: 10.1097/OLQ.0b013e318167b105
Moriya, Y., Itoh, M., Okuda, S., Yoshizawa, A. C., and Kanehisa, M. (2007). KAAS: an automatic genome annotation and pathway reconstruction server. Nucleic Acids Res. 35, W182–W185. doi: 10.1093/nar/gkm321
Muzny, C. A., and Schwebke, J. R. (2016). Pathogenesis of bacterial vaginosis: discussion of current hypotheses. J. Infect. Dis. 214(Suppl. 1), S1–S5. doi: 10.1093/infdis/jiw121
Ngo, T. D., Perdu, C., Jneid, B., Ragno, M., Novion Ducassou, J., Kraut, A., et al. (2020). The PopN gate-keeper complex acts on the ATPase PscN to regulate the T3SS secretion switch from early to middle substrates in Pseudomonas aeruginosa. J. Mol. Biol. 432:166690. doi: 10.1016/j.jmb.2020.10.024
Nugent, R. P., Krohn, M. A., and Hillier, S. L. (1991). Reliability of diagnosing bacterial vaginosis is improved by a standardized method of gram stain interpretation. J. Clin. Microbiol. 29, 297–301. doi: 10.1128/jcm.29.2.297-301.1991
Okoli, A. C., Agbakoba, N. R., Ezeanya, C. C., Oguejiofor, C. B., and Anukam, K. C. (2019). Comparative abundance and functional biomarkers of the vaginal and gut microbiome of Nigerian women with bacterial vaginosis: a study with 16S rRNA metagenomics. J. Med. Lab. Sci. 29, 1–26.
Onderdonk, A. B., Delaney, M. L., and Fichorova, R. N. (2016). The human microbiome during bacterial vaginosis. Clin. Microbiol. Rev. 29, 223–238. doi: 10.1128/CMR.00075-15
Pandey, R., and Rodriguez, G. M. (2014). IdeR is required for iron homeostasis and virulence in Mycobacterium tuberculosis. Mol. Microbiol. 91, 98–109. doi: 10.1111/mmi.12441
Park, C. J., Smith, J. T., and Andam, C. P. (2019). “Horizontal gene transfer and genome evolution in the phylum Actinobacteria,” in Horizontal Gene Transfer: Breaking Borders Between Living Kingdoms, eds T. G. Villa and M. Viñas (Cham: Springer International Publishing), 155–174.
Parks, D. H., Imelfort, M., Skennerton, C. T., Hugenholtz, P., and Tyson, G. W. (2015). CheckM: assessing the quality of microbial genomes recovered from isolates, single cells, and metagenomes. Genome Res. 25, 1043–1055. doi: 10.1101/gr.186072.114
Parte, A. C. (2018). LPSN - List of Prokaryotic names with Standing in Nomenclature (bacterio.net), 20 years on. Int. J. Syst. Evol. Microbiol. 68, 1825–1829. doi: 10.1099/ijsem.0.002786
Peebles, K., Velloza, J., Balkus, J. E., McClelland, R. S., and Barnabas, R. V. (2019). High global burden and costs of bacterial vaginosis: a systematic review and meta-analysis. Sex. Transm. Dis. 46, 304–311. doi: 10.1097/OLQ.0000000000000972
Ramos, H. C., Rumbo, M., and Sirard, J. C. (2004). Bacterial flagellins: mediators of pathogenicity and host immune responses in mucosa. Trends Microbiol. 12, 509–517. doi: 10.1016/j.tim.2004.09.002
Richter, M., and Rossello-Mora, R. (2009). Shifting the genomic gold standard for the prokaryotic species definition. Proc. Natl. Acad. Sci. U.S.A. 106, 19126–19131. doi: 10.1073/pnas.0906412106
Roberts, M. C., Baron, E. J., Finegold, S. M., and Kenny, G. E. (1985). Antigenic distinctiveness of Mobiluncus curtisii and Mobiluncus mulieris. J. Clin. Microbiol. 21, 891–893. doi: 10.1128/jcm.21.6.891-893.1985
Roberts, M. C., Hillier, S. L., Schoenknecht, F. D., and Holmes, K. K. (1984). Nitrocellulose filter blots for species identification of Mobiluncus curtisii and Mobiluncus mulieris. J. Clin. Microbiol. 20, 826–827. doi: 10.1128/jcm.20.4.826-827.1984
Salinas, A. M., Osorio, V. G., Pacha-Herrera, D., Vivanco, J. S., Trueba, A. F., and Machado, A. (2020). Vaginal microbiota evaluation and prevalence of key pathogens in ecuadorian women: an epidemiologic analysis. Sci. Rep. 10:18358. doi: 10.1038/s41598-020-74655-z
Schwebke, J. R., and Desmond, R. (2005). Risk factors for bacterial vaginosis in women at high risk for sexually transmitted diseases. Sex. Transm. Dis. 32, 654–658. doi: 10.1097/01.olq.0000175396.10304.62
Schwebke, J. R., and Desmond, R. A. (2007). A randomized trial of the duration of therapy with metronidazole plus or minus azithromycin for treatment of symptomatic bacterial vaginosis. Clin. Infect. Dis. 44, 213–219. doi: 10.1086/509577
Schwebke, J. R., and Lawing, L. F. (2001). Prevalence of Mobiluncus spp among women with and without bacterial vaginosis as detected by polymerase chain reaction. Sex. Transm. Dis. 28, 195–199. doi: 10.1097/00007435-200104000-00002
Seemann, T. (2014). Prokka: rapid prokaryotic genome annotation. Bioinformatics 30, 2068–2069. doi: 10.1093/bioinformatics/btu153
Sha, B. E., Chen, H. Y., Wang, Q. J., Zariffard, M. R., Cohen, M. H., and Spear, G. T. (2005). Utility of amsel criteria, nugent score, and quantitative PCR for Gardnerella vaginalis, Mycoplasma hominis, and Lactobacillus spp. for diagnosis of bacterial vaginosis in human immunodeficiency virus-infected women. J. Clin. Microbiol. 43, 4607–4612. doi: 10.1128/JCM.43.9.4607-4612.2005
Siguier, P., Perochon, J., Lestrade, L., Mahillon, J., and Chandler, M. (2006). ISfinder: the reference centre for bacterial insertion sequences. Nucleic Acids Res. 34, D32–D36. doi: 10.1093/nar/gkj014
Smillie, C. S., Smith, M. B., Friedman, J., Cordero, O. X., David, L. A., and Alm, E. J. (2011). Ecology drives a global network of gene exchange connecting the human microbiome. Nature 480, 241–244. doi: 10.1038/nature10571
Sobel, J. D. (2000). Bacterial vaginosis. Annu. Rev. Med. 51, 349–356. doi: 10.1146/annurev.med.51.1.349
Spiegel, C. A. (1987). Susceptibility of Mobiluncus species to 23 antimicrobial agents and 15 other compounds. Antimicrob. Agents Chemother. 31, 249–252. doi: 10.1128/AAC.31.2.249
Srinivasan, S., Morgan, M. T., Liu, C., Matsen, F. A., Hoffman, N. G., Fiedler, T. L., et al. (2013). More than meets the eye: associations of vaginal bacteria with gram stain morphotypes using molecular phylogenetic analysis. PLoS One 8:e78633. doi: 10.1371/journal.pone.0078633
Sullivan, M. J., Petty, N. K., and Beatson, S. A. (2011). Easyfig: a genome comparison visualizer. Bioinformatics 27, 1009–1010. doi: 10.1093/bioinformatics/btr039
Suzuki, R., and Shimodaira, H. (2006). Pvclust: an R package for assessing the uncertainty in hierarchical clustering. Bioinformatics 22, 1540–1542. doi: 10.1093/bioinformatics/btl117
Taha, T. E., Hoover, D. R., Dallabetta, G. A., Kumwenda, N. I., Mtimavalye, L. A., Yang, L. P., et al. (1998). Bacterial vaginosis and disturbances of vaginal flora: association with increased acquisition of HIV. AIDS 12, 1699–1706. doi: 10.1097/00002030-199813000-00019
Taylor-Robinson, A. W., and Taylor-Robinson, D. (2002). Evaluation of liquid culture media to support growth of Mobiluncus species. J. Med. Microbiol. 51, 491–494. doi: 10.1099/0022-1317-51-6-491
Tettelin, H., Riley, D., Cattuto, C., and Medini, D. (2008). Comparative genomics: the bacterial pan-genome. Curr. Opin. Microbiol. 11, 472–477.
Thorsen, P., Jensen, I. P., Jeune, B., Ebbesen, N., Arpi, M., Bremmelgaard, A., et al. (1998). Few microorganisms associated with bacterial vaginosis may constitute the pathologic core: a population-based microbiologic study among 3596 pregnant women. Am. J. Obstet. Gynecol. 178, 580–587. doi: 10.1016/s0002-9378(98)70442-9
Vinuesa, P., Ochoa-Sanchez, L. E., and Contreras-Moreira, B. (2018). GET_PHYLOMARKERS, a software package to select optimal orthologous clusters for phylogenomics and inferring pan-genome phylogenies, used for a critical geno-taxonomic revision of the genus Stenotrophomonas. Front. Microbiol. 9:771. doi: 10.3389/fmicb.2018.00771
Wang, Q., Hassan, B. H., Lou, N., Merritt, J., and Feng, Y. (2019). Functional definition of NrtR, a remnant regulator of NAD+ homeostasis in the zoonotic pathogen Streptococcus suis. FASEB J. 33, 6055–6068. doi: 10.1096/fj.201802179RR
Williams, M. J., Kana, B. D., and Mizrahi, V. (2011). Functional analysis of molybdopterin biosynthesis in mycobacteria identifies a fused molybdopterin synthase in Mycobacterium tuberculosis. J. Bacteriol. 193, 98–106. doi: 10.1128/JB.00774-10
Wylensek, D., Hitch, T. C. A., Riedel, T., Afrizal, A., Kumar, N., Wortmann, E., et al. (2020). A collection of bacterial isolates from the pig intestine reveals functional and taxonomic diversity. Nat. Commun. 11:6389. doi: 10.1038/s41467-020-19929-w
Zeng, W., Ma, H., Fan, W., Yang, Y., Zhang, C., Arnaud Kombe Kombe, J., et al. (2020). Structure determination of CAMP factor of Mobiluncus curtisii and insights into structural dynamics. Int. J. Biol. Macromol. 150, 1027–1036. doi: 10.1016/j.ijbiomac.2019.10.107
Zhang, X., Bai, Y., Zhang, L., Draz, M. S., Ruan, Z., and Zhu, Y. (2020). Antimicrobial susceptibility and clonality of vaginally derived multidrug-resistant Mobiluncus isolates in China. Antimicrob. Agents Chemother 64, e00780–20. doi: 10.1128/AAC.00780-20
Zhao, Y., Jia, X., Yang, J., Ling, Y., Zhang, Z., Yu, J., et al. (2014). PanGP: a tool for quickly analyzing bacterial pan-genome profile. Bioinformatics 30, 1297–1299. doi: 10.1093/bioinformatics/btu017
Keywords: Mobiluncus, comparative genomics, interspecific divergence, pathogenicity, horizontal gene transfer, bacterial vaginosis
Citation: Li Y, Wang Y and Liu J (2022) Genomic Insights Into the Interspecific Diversity and Evolution of Mobiluncus, a Pathogen Associated With Bacterial Vaginosis. Front. Microbiol. 13:939406. doi: 10.3389/fmicb.2022.939406
Received: 09 May 2022; Accepted: 16 June 2022;
Published: 05 July 2022.
Edited by:
Yufeng Wang, University of Texas at San Antonio, United StatesReviewed by:
Wei Ke, Rutgers, The State University of New Jersey, United StatesLiang Wang, Guangdong Provincial People’s Hospital, China
Copyright © 2022 Li, Wang and Liu. This is an open-access article distributed under the terms of the Creative Commons Attribution License (CC BY). The use, distribution or reproduction in other forums is permitted, provided the original author(s) and the copyright owner(s) are credited and that the original publication in this journal is cited, in accordance with accepted academic practice. No use, distribution or reproduction is permitted which does not comply with these terms.
*Correspondence: Jie Liu, amw1eWpAaG90bWFpbC5jb20=