- 1Istituto Zooprofilattico Sperimentale dell’Umbria e delle Marche “Togo Rosati,” Perugia, Italy
- 2Laboratorio Nazionale di Riferimento per Listeria Monocytogenes, Istituto Zooprofilattico Sperimentale dell’Abruzzo e del Molise G. Caporale, Teramo, Italy
- 3Centro di Referenza Nazionale per Sequenze Genomiche di Microrganismi Patogeni, Istituto Zooprofilattico Sperimentale dell’Abruzzo e del Molise G. Caporale, Teramo, Italy
In this study, we characterized 84 Listeria monocytogenes (Lm) strains having an atypical IVb-v1 profile and isolated in a meat producing plant of Central Italy. They were assigned to the new MLST type ST2801 (CC218). The new ST was widespread in the food-producing environment where it was able to persist for over a year even after cleaning and sanitation. Cluster analysis identified three main clusters genetically close to each other (0–22 allelic differences and 0–28 SNPs) from two different cgMLST types, suggesting a common source. The coexistence of closely related clusters over time could be the result of a different evolution path starting from a common ancestor first introduced in the plant and/or the consequence of the repetitive reintroduction of closely related clones probably by raw materials. All the strains presented several determinants for heavy metals resistance, stress response, biofilm production, and multidrug efflux pumps with no significant differences among the clusters. A total of 53 strains carried pLI100 and the j1776 plasmids, while in one strain, the pLM33 was found in addition to pLI100. Only the strains carrying plasmids presented cadA and cadC for cadmium resistance and the mco gene encoding a multicopper oxidase and gerN for an additional Na+/H+-K+ antiporter. All the strains presented a virulence profile including a full-length inlA gene and the additional LIPI-3. The isolation of a new ST with a large pattern of stress-adaptation genes and able to persist is an important contribution to deepening the current knowledge on the uncommon IVb-v1 and in general on the genomic diversity of Lm.
Introduction
Listeria monocytogenes (Lm) is the foodborne pathogen causing human listeriosis, the most serious foodborne disease under EU surveillance with the highest proportion of hospitalized cases and fatality rate (13%). Invasive forms of the disease mainly affect people at risk causing abortion and stillbirth in pregnant women and meningitis septicemia and death in the elderly, immunocompromised people, and newborns (European Food Safety Authority, and European Centre for Disease Prevention and Control, 2021). Lm is a genetically heterogeneous species including hypo- and hypervirulent clones (Maury et al., 2016, 2019) and strains able to survive and persist in food-producing environments (FPE) even for years, due to their adaptation to different environmental stresses such as cold temperatures, high salinity, low pH, oxidation, and desiccation (Palma et al., 2017; Pombinho et al., 2017; Maury et al., 2019; Parsons et al., 2020; Guidi et al., 2021; Palaiodimou et al., 2021).
Listeria monocytogenes isolates can be grouped into four lineages, five most common PCR serogroups (Orsi et al., 2011), 2,880 multilocus sequence typing (MLST) sequence types (ST) grouping in different clonal complexes (CCs) (Ragon et al., 2008), and thousands of core genome MLST (cgMLST) types (CTs) grouped into nearly 400 different sublineages (Moura et al., 2017) (accessed on February 2022).1
Although the most common Lm PCR serogroups known are IIa, IIb, IIc, L, and IVb (Doumith et al., 2004; Kérouanton et al., 2010; Leclercq et al., 2011), an atypical and novel PCR profile of serogroup IVb was identified in 2007 for Lm isolates from France (Leclercq et al., 2011) and other countries including the United States (Graves et al., 2007), Chile (de Vasconcelos et al., 2008), and Australia (Huang et al., 2011). These atypical strains harbored the lmo0737 gene, specific to serogroups IIa and IIc in addition to the four-target profile defining serogroup IVb (Doumith et al., 2004; Kérouanton et al., 2010; Leclercq et al., 2011; Lee et al., 2012). This rare profile was designated as “IVb-v1” (Lee et al., 2012).
Although the first studies published on this atypical IVb-v1 profile reported its isolation from milk products and meat products (Leclercq et al., 2011), the most recent works show a particular association with vegetable matrices such as caramel apple, stone fruits, leafy green, and radicchio as well as the associated processing environments (Torresi et al., 2020; Yang et al., 2020; Chen et al., 2022). Scientific reports on different IVb-v1 clinical isolates are also available (Leclercq et al., 2011; Lee et al., 2012; Scaltriti et al., 2020).
All the IVb-v1 strains previously isolated worldwide, from both food and humans, mainly belonged to four STs: ST218 (CC218), ST240 (CC240), ST382 (CC183), and ST554 (CC554) (Chen et al., 2017, 2022; Kim et al., 2018; Scaltriti et al., 2020; Yang et al., 2020).
To date, very few in-depth studies have been performed on the virulence genetic profile of Lm strains belonging to IVb-v1 and their genetic determinants involved in stress response. Chen et al. (2022), reported the presence of different stress response determinants in Lm IVb-v1 belonging to ST554 (low pH, cold, etc.) in the only published study describing the persistence of IVb-v1 strains in a food-processing plant. The same authors also defined the virulence profile for internalins and Listeria Pathogenicity Islands (LIPI) identifying the presence of inlA/B/C/E/F/H/J/K/P and the LIPI-3. The latter was also reported by de Tavares et al. (2020) in IVb-v1 Lm isolates belonging to ST218. In their report on a IVb-v1 Lm isolated from a vegetable matrix, Torresi et al. (2020) described the presence of a plasmid which carried heavy metal-resistance genes, but no information about the virulence profile was provided.
During the extensive environmental sampling plan for Lm performed in a pork meat-producing plant of Central Italy between 2020 and 2021, we isolated several strains having the atypical IVb-v1 serogroup profile and belonging to a new ST assigned by the Institute Pasteur (ST2801).
In this study, we characterized the new ST2801 isolates to (i) evaluate the genomic correlation existing among them, (ii) assess their persistence in the meat producing plant, (iii) investigate the presence of genetic determinants involved in environmental stress adaptation, and (iv) define virulence profiles.
Materials and Methods
Environmental Sampling and Listeria monocytogenes Detection
During the period between July 2020 and September 2021, an extensive environmental sampling for Lm was performed in a pork meat-producing plant in Central Italy. Three different sampling sessions were performed during production, in particular, on July 2020 (Production 1), May 2021 (Production 2), and September 2021 (Production 3). A sampling scheme of 63 surfaces including both food contact (FCS) and nonfood contact surfaces (NFCS) was defined focusing on the main niches for Lm presence (Supplementary Table 1). In each sampling session, the same surfaces were sampled using commercial sterile sponges.
In accordance with the European Union Reference Laboratory for Lm (EURL) guidelines (Carpentier and Barre, 2012), the total sampled area varied depending on the sampling site but was as large as possible to improve the probability of detecting Lm. The samples were tested according to ISO 11290-1:2017 for Lm detection.
If positive surfaces were found, they were sampled again after extraordinary cleaning and sanitation.
Strains Collection
Up to five Lm colonies from each positive sample were randomly selected and screened for their belonging to one of the five major serogroups (IIa, IIb, IIc, L, and IVb), using a multiplex PCR assay according to the EURL method (Doumith et al., 2004; Kérouanton et al., 2010). At least one isolate for each serogroup found in each sample was selected to be subjected to whole genome sequencing (WGS). In this study, we focused on Lm strains presenting the atypical serogroup IVb-v1 (Lee et al., 2012).
Whole Genome Sequencing and Bioinformatic Analysis
DNA extraction was performed according to Portmann et al. (2018), with minor modifications, using the QIAamp DNA Mini Kit (Qiagen Hilden, Germany) according to the manufacturer’s protocol.
The purity of the extracts was evaluated using NanoDrop2000 (ThermoFisher Scientific, Wältham, MA, United States). Starting from 1 ng of input DNA, the Nextera XT DNA chemistry (Illumina, San Diego, CA, United States) for library preparation was used according to the manufacturer’s protocols. WGS was performed on the NextSeq 500 platform (Illumina, San Diego, CA, United States) with the NextSeq 500/550 mid output reagent cartridge v2 (300 cycles, standard 150-bp paired-end reads).
For the analysis of WGS data, an in-house pipeline (Cito et al., 2018) was used, which included steps for trimming (Trimmomatic version 0.362; base quality parameters, namely, leading, 25; trailing, 25; and sliding window, 20:25) (Bolger et al., 2014) and a quality control check of the reads (FastQC version 0.11.53).
Genome de novo assembly of paired-end reads was performed using SPAdes version 3.11.14 (Bankevich et al., 2012) with the parameters suggested by the manual for the Illumina platform 2_150 chemistry (--only-assembler --careful -k 21, 33, 55, 77). Then, the genome assembly quality check was performed using QUAST version 4.35 (Gurevich et al., 2013). All the genomes that met the quality parameters recommended by Timme et al. (2020) were used for the subsequent analysis steps.
The genome assemblies were deposited at DDBJ/ENA/GenBank under the BioProject PRJNA821663.
Multilocus Sequence Typing Analysis, Core Genome Multilocus Sequence Typing, and Single-Nucleotide Polymorphisms Analysis
The sequence type (ST) and the clonal complex (CC) were deducted in silico using the specific tool available on the BIGSdb-Lm database (accessed on October 2021)6 and based on the MLST scheme including the seven housekeeping genes abcZ, bglA, cat, dapE, dat, ldh, and lhlA (Ragon et al., 2008).
To verify the relatedness among the isolates, identifying genomic clusters, a cgMLST analysis was performed using the chewBBACA7 allele calling algorithm (Silva et al., 2018) and the Pasteur Institute cgMLST scheme of 1,748 loci (Moura et al., 2017). According to the guidelines for Lm cgMLST typing (Moura et al., 2017), only the genomes with at least 1,660 called loci (95% of the full scheme) were considered. The software GrapeTree8 (Zhou et al., 2018) was used for the visualization of the minimum spanning tree (MSTreeV2 method).
A core single-nucleotide polymorphism (SNPs) analysis was performed using the reference-free tool KSNP39 with a kmer size of 21 (Morganti et al., 2016). The resulting neighbor-joining (NJ) tree was visualized using the interactive tree of life (iTOL).10
Genetic Determinants Involved in Stress Adaptation, Biofilm Formation, and Virulence Potential
All the genome assemblies were manually screened for the presence/absence of loci encoding for disinfectants and metal resistance and stress survival islets (SSIs) using the “Metal and Detergent Resistance” and the “Stress Islands” tools available on the BIGSdb-Lm platform (accessed on January 2022).
Moreover, the detection of additional determinants in the field, not included in these schemes (sugE, mdrl, lde, arsRDABC, cadAC), was performed automatically using Prokka v.1.1211 (Seemann, 2014).
Genes involved in biofilm production were also detected using the BIGSdb-Lm platform (inlA, actA, prfA, lmo0673, and lmo2504) and the Prokka software (see text footnote 11) (luxS, recO, inlL, and bapL).
The PlasmidFinder web tool (version 2.0.1 2020-02-07; accessed from October 2021 to January 2022; Carattoli et al., 2014)12 was used to detect the presence of presumptive plasmids.
The virulence profile of the strains was deduced using the “Virulence” tool provided by the BIGSdb-Lm platform, also investigating the presence of premature stop codon mutations in the inlA gene (accessed from October 2021 to January 2022).
Results
Environmental Sampling and Listeria monocytogenes Strain Collection
During the period between July 2020 and September 2021, 189 environmental samples were collected in the pork meat-producing plant of the study during production and 40 after cleaning and sanitation. A total of 35 samples of those collected during production (18.5%) and 7 samples of those collected after cleaning and sanitation (17.5%) tested positive for the presence of Lm. A total of 147 Lm strains were isolated from these samples and screened for serogroup. Among them, 84 Lm strains (Supplementary Table 2), isolated from 19 environmental surfaces, presented the atypical IVb-v1 serogroup profile carrying the lmo0737 target gene in addition to the four targets normally characterizing the serogroup IVb (ORF2110, ORF2819, prs and prfA). These atypical strains were selected for further genomic analysis.
Multilocus Sequence Typing Analysis, Core Genome Multilocus Sequence Typing, and Single-Nucleotide Polymorphisms Analysis
For all the Lm strains IVb-v1, the MLST analysis found exact allele matches for only six of the seven genes of the scheme. After submitting the genomes to the BIGSdb-Lm database (21 October 2021), a new allelic id was defined for the locus abcZ resulting in a new MLST profile (named ST2801 and belonging to clonal complex CC218).
The cgMLST analysis showed that the microbial population associated with the new ST was heterogeneous and allelic differences among the strains ranged from 0 to 22 (Figure 1). Based on a seven allele threshold, three main clusters were identified (Figure 1). Cluster A (cgMLST type L1-SL218-ST2801-CT10448) included 56 strains and was isolated in the FPE after all the sampling sessions performed during production and after two consecutive cleaning and sanitation sessions subsequent to Production 1. This cluster was isolated from 15 different surfaces: n°1, 2, 6, 9, 30, 32, 36, 37, 38, 43, 51, 56, 57, 62, and 63 (Supplementary Table 2). Among these surfaces, n°9, 30, 37, 38, and 63 were found to be contaminated with cluster A more than once over the course of time.
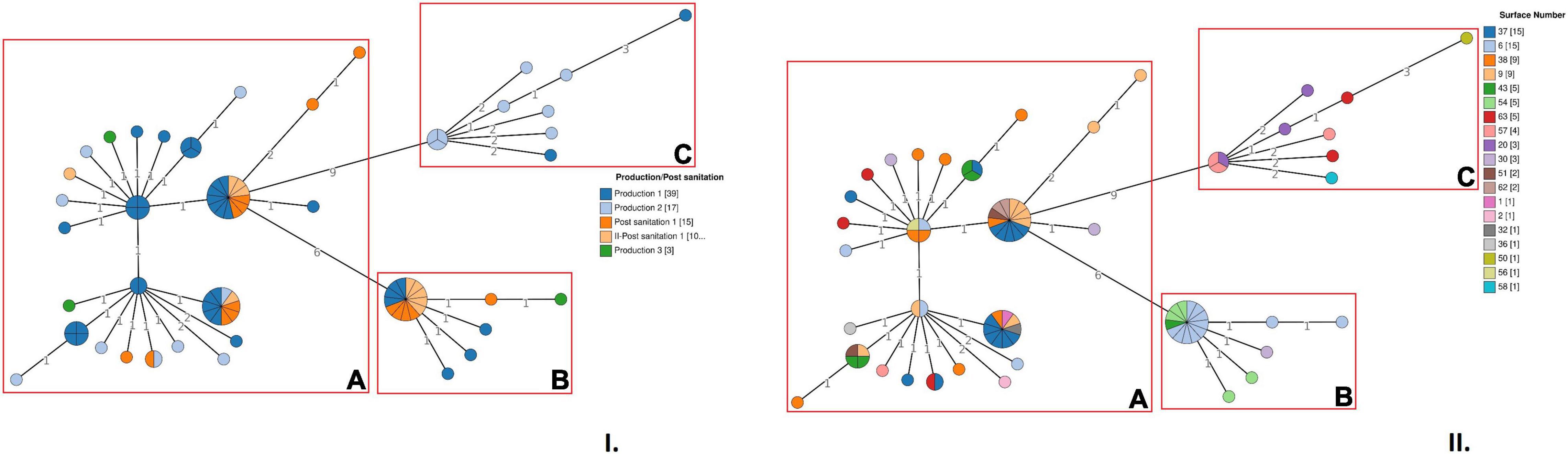
Figure 1. Cluster analysis of Lm strains belonging to ST2801 based on cgMLST profiles. (I) In the minimum spanning tree (MSTv2), strains are colored according to the sampling session. (II) In the MSTv2, strains are colored according to the environmental surface from which they were isolated. The number values between adjacent nodes indicate the number of allelic differences between nodes. Clusters are highlighted with red boxes.
A total of 18 Lm strains, isolated during Production 1 and Production 3 and after two consecutive cleaning and sanitation sessions subsequent to Production 1 (I-Post sanitation 1 and II-Post sanitation 1), were grouped in cluster B (cgMLST type L1-SL218-ST2801-CT10448). No strains isolated during Production 2 were grouped in this cluster. A total of 4 surfaces of the scheme, n°6, 30, 43 and 54, were found to be contaminated with cluster B, with surface n°6 resulting positive more than once (Figure 1 and Supplementary Table 2).
Finally, cluster C (cgMLST type L1-SL218-ST2801-CT11418) included 10 strains all isolated during production, in particular, Production 1 and Production 2. This cluster was recovered from 5 different surfaces of the sampling scheme, n°20, 50, 57, 58, and 63 (Figure 1).
The core SNPs analysis was performed to deepen the genetic relationships between the strains and the results were concordant with those of the cgMLST analysis (Figure 2). The number of SNP differences among all the isolates ranged from 0 to 28 (Supplementary Table 3). In particular, Lm strains grouped in cluster A differed by a number of SNPs ranging from 0 to 20 with a median of 4. Among strains of cluster B and cluster C, SNPs differences ranged from 0 to 5 (median 1) and from 0 to 11 (median 6), respectively.
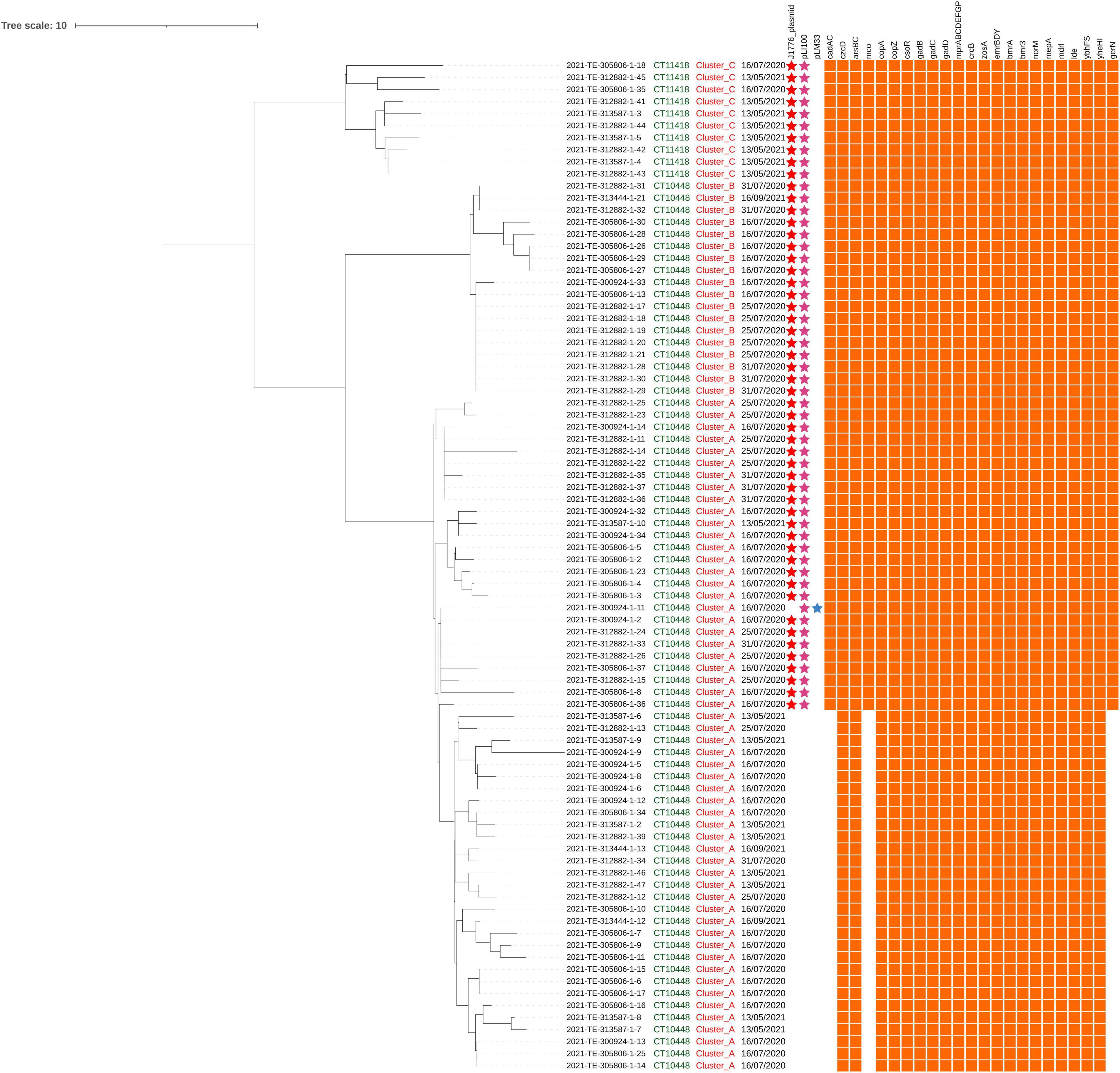
Figure 2. Single-nucleotide polymorphisms (SNPs) analysis of Lm strains belonging to ST2801. The first, the second, and the third columns indicate the CT, the cluster, and the sampling date, respectively. The presence/absence matrix represents, from left to right, plasmids (j1776 plasmid, pLI100, and pLM33) and genes involved in stresses adaptation.
Genetic Determinants Involved in Stress Adaptation and Biofilm Production
Using the BIGSdb-Lm platform together with the annotation results, several determinants for different heavy metal resistance were detected, as well as genes for multidrug efflux pumps and response to environmental stresses (Table 1).
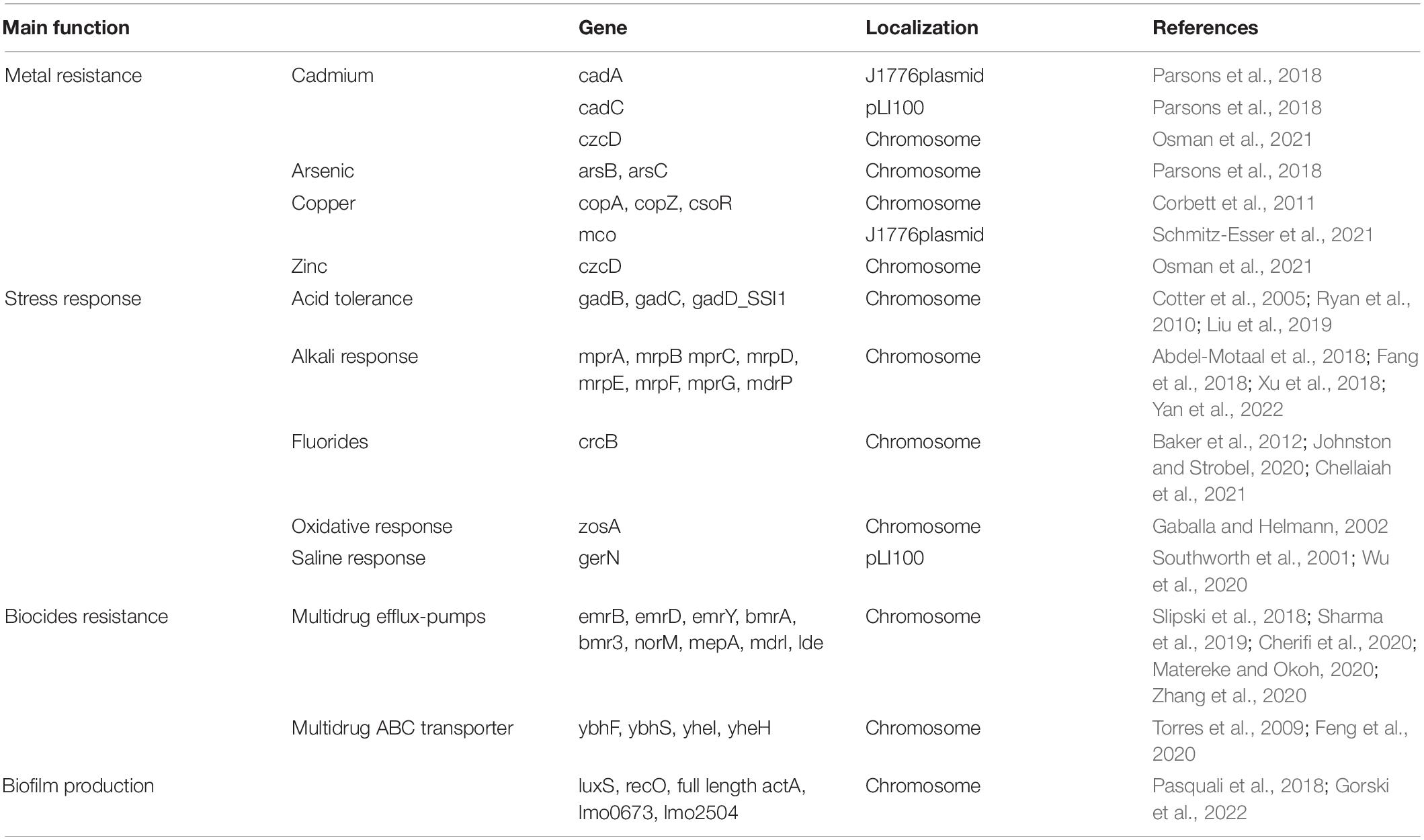
Table 1. Relevant features for environmental persistence of different clusters conveyed and not by plasmids.
In all the strains, the determinants for heavy metal resistance included czcD, arsB, arsC, and the csoR-copA-copZ copper-resistance operon. The zosA gene for Zn(II) uptake was also found.
The same genetic determinants for stress responses were detected in all the strains. In particular, for tolerance to acid stress, in addition to the gadB-gadC operon, gadD, was also found in all the strains and represented the only gene of the SSI-1.
Several determinants associated with monovalent cation/proton antiporters were found in all the ST2801 strains. These genes included the multiple resistance and pH operons mrpABCDEFG and mdrP.
All the strains of the new ST carried determinants for different multidrug efflux pumps (emrB, emrD, emrY, bmrA, bmr3, norM, mepA, mdrl, and lde) and multidrug ABC transporters (ybhF, ybhS, yheI, and yheH), as well as the fluoride resistance gene crcB.
A total of 26 strains from cluster A and all the strains from cluster B and cluster C carried two different plasmids and in particular, the pLI100 (Acc. Number AL592102) together with the j1776 plasmid (Acc. Number CP006612) or the pLM33 (Acc. Number GU244485; Figure 2).
Only the strains that carried these plasmids presented cadA and cadC for cadmium resistance, the mco gene for copper detoxification and the additional Na+/H+-K+ antiporter GerN.
All the strains carried the genes luxS, recO, lmo2504, and lmo0673 involved in biofilm formation.
Virulence Genes
A total of 66 virulence genes were detected on a scheme of 93 targets. All the strains showed the same virulence profile that presented the Listeria Pathogenicity Island (LIPI) 3 (llsA, llsG, llsH, llsX, llsB, llsY, llsD, and llsP) in addition to the conventional LIPI-1 (prfA, actA, hly, mpl, plcA, plcB, and iap), 10 internalin genes including a full length inlA, inlB, inlC, inlD, inlE, inlF, inlH, inlJ, and inlK, the viR/virS virulence regulatory system, the teichoic acid biosynthesis genes gltA and gltB, and the invasion gene aut_IVb.
Discussion
In this study, we reported the widespread isolation of Lm strains having an atypical IVb-v1 serogroup profile from the FPE of a meat-producing plant in Central Italy. To date, very little is known about the circulation of this uncommon variant in Italy, where only two studies reported the isolation of Lm strain IVb-v1 (Scaltriti et al., 2020; Torresi et al., 2020).
The first studies published by authors of other countries on this atypical IVb-v1 profile reported its isolation from milk products and meat products (Leclercq et al., 2011). However, the most recent works, including the one of Torresi et al. (2020), also show the association with vegetable matrices such as caramel apple, stone fruits, leafy green, and radicchio (Torresi et al., 2020; Yang et al., 2020; Chen et al., 2022). Moreover, IVb-v1 Lm strains have been previously isolated from FPEs. In more detail, Lee et al. (2012) typed three IVb-v1 strains isolated from a turkey processing plant of the United States during a 2-year sampling performed by Mullapudi et al. (2008) between 2017 and 2018. These strains were isolated from two drains and a chiller re-work table.
Chen et al. (2022) used WGS to type IVb-v1 strains isolated from several environmental surfaces of an apple packinghouse facilities mostly during a 2-year environmental survey performed between 2016 and 2017 by Simonetti et al. (2021). In this FPE, Lm isolates belonging to IVb-v1, counted for more than 90% of all isolates and spread across the facility mostly contaminating floors and other NFCSs and persisting throughout the sampling year (Chen et al., 2022). These results were very similar to those obtained in our study and seemed to indicate that once strains belonging to this uncommon serogroup are introduced into an FPE, they are able to spread and persist mainly on NFCSs such as drains, floors, and cleaning materials.
Lm IVb-v1 strains have been identified among the isolates from human cases of listeriosis occurred in France, Brazil, Switzerland, and United States (Leclercq et al., 2011; Lee et al., 2012). These strains were responsible for maternal-neonatal forms, infection of central nervous system, and bacteremia. Also in Italy, a human case of listeriosis, caused by a IVb-v1 strain in 2016 was reported by Scaltriti et al. (2020).
All the IVb-v1 strains previously isolated worldwide, from both food and humans, belonged to four main STs and in particular to ST218, ST240, ST382, and ST554 (Chen et al., 2017, 2022; Kim et al., 2018; Yang et al., 2020). In particular, all the Italian isolates reported before belonged to ST218 within the CC218.Very interestingly, the IVb-v1 strains described in this study presented a new MLST allelic profile also included in the CC218 and assigned as ST2801. As a future perspective, the evolutionary linkage between these STs isolated in Italy could be investigated.
In this study, the ST2801 was isolated from different environmental surfaces, mostly NFCS, representing the main source of environmental contamination in the FPE. Most of these NFCS, represented ideal niches since they were difficult to clean and sanitize because of inherent inaccessibility (e.g., drains), harborage sites (e.g., porous wall-floor connections), wear surfaces (e.g., door seals), and cleaning tools (e.g., water pullets). On some of these NFCS, such as the wall floor connection of a cold room, the wheels of meat trolleys, the door handle and seal of another cold room, and the water puller of the washing area, the ST2801 was able to persist over the time even after cleaning and sanitation. Since the surfaces were all identified, these results were reported to the FBOs in order to increase their level of attention in terms of more accurate cleaning and sanitation or replacement of worn materials. The persistence of this ST probably indicated an efficient environmental adaptation also suggested by the presence of several genetic determinants for stress response, as discussed below. In their previous study, Chen et al. (2022) also reported the isolation of Listeria monocytogenes IVb-v1 strains from several NFCS and their ability to persist in the FPE.
CgMLST analysis identified three main clusters from two different types (CT10448 and CT11418), genetically close to each other. The results of SNPs analysis confirmed the presence of the same three close-to-clonal groups.
In particular, cluster A was the most represented and was isolated during all the sampling sessions performed both during production and after sanitation, persisting in the plant from July 2020 to September 2021. The same persistence period was observed for the smaller cluster B, which, however, unlike cluster A, was not isolated during the intermediate Production 2. Cluster C, instead, was isolated during Production 1 and Production 2 but not during Production 3. These three near-clonal clusters were found to be coexistent in the meat-producing plant until Production 2, while during Production 3, only cluster A and cluster B were isolated. As observed by Knudsen et al. (2017) and Chiaverini et al. (2021), the coexistence of closely related clusters over the time could be the result of a different evolution path starting from a common ancestor first introduced in the plant and/or the repetitive reintroduction of closely related clones probably by raw materials.
All the ST2801 strains carried several determinants for different heavy metals resistance, stress response, and multidrugs efflux pumps with no significant differences among the clusters.
Genes for heavy metals resistance included czcD encoding a cation diffusion facilitator protein family transporter reducing Cd2+, Zn2+, and Co2+ accumulation in the cytoplasm (Osman et al., 2021), arsB and arsC encoding an ATPase and an arsenate reductase involved in arsenic resistance (Parsons et al., 2018), the csoR-copA-copZ copper resistance operon (Corbett et al., 2011), and zosA for Zn(II) uptake also contributing to oxidative stress resistance (Gaballa and Helmann, 2002). For tolerance to acid stress, in addition to the gadB-gadC operon, for a glutamate decarboxylase and a glutamate/GABS antiporter (Cotter et al., 2005; Liu et al., 2019), all the studied strains presented gadD, for a homologous decarboxylase. This last gene was the only one detected within the SSI-1 (Ryan et al., 2010), while no determinants belonging to the SSI-2 were found.
Several genes associated with monovalent cation/proton antiporters were found in all the strains and included the multiple resistance and pH operon mrpABCDEFG encoding for Na+/H+ antiporters and mdrP for a Na+ Li+ K+/H+ antiporter, both responsible for sodium and alkali resistance (Abdel-Motaal et al., 2018; Fang et al., 2018; Xu et al., 2018; Yan et al., 2022).
The crcB gene, associated with the riboswitch responsible for tolerance to the anion fluoride, was also detected in the studied genomes (Baker et al., 2012; Johnston and Strobel, 2020; Chellaiah et al., 2021).
Different multidrug efflux pumps (emrB, emrD, emrY, bmrA, bmr3, norM, mepA, mdrl, and lde) and multidrug ABC transporters (ybhF, ybhS, yheI, and yheH) were detected in all the strains (Torres et al., 2009; Slipski et al., 2018; Sharma et al., 2019; Cherifi et al., 2020; Feng et al., 2020; Matereke and Okoh, 2020; Zhang et al., 2020). Many of these genomic features are known to be involved in tolerance to disinfectants, including QAC, largely used in the food industry and specifically in the meat-producing plant studied (Slipski et al., 2018; Sharma et al., 2019; Cherifi et al., 2020; Matereke and Okoh, 2020; Zhang et al., 2020).
Although all the ST2801 strains mostly exhibited the same genetic pattern associated with environmental adaptations, there were some differences between the plasmid-carrying strains and those without. In more detail, two different Listeria plasmids were carried by 54 strains; in 53 of them pLI100 (Glaser et al., 2001; Kuenne et al., 2010) and the j1776 plasmid (Chiaverini et al., 2021; Mafuna et al., 2021; Maggio et al., 2021) were detected, while in one of them, the pLM33 (Kuenne et al., 2010) was found in addition to pLI100. Only the strains carrying these plasmids presented cadA and cadC for cadmium resistance (Parsons et al., 2018), the mco gene encoding a multicopper oxidase (MCO), and the additional Na+/H+-K+ antiporter GerN (Southworth et al., 2001; Wu et al., 2020). This finding suggests that the above determinants were carried on these plasmids. We used the nucleotide Basic Local Alignment Search Tool (BLASTn) to verify the alignment between the sequences of cadA, cadC, mco, and gerN as annotated by Prokka in the studied strains, and the ones found in the GenBank file of each plasmid on NCBI. A 100% score for coverage and identity was observed between the cadA gene carried by the strains and the one present in both pLI100 and j1776 plasmids. The same result was obtained for the cadC and gerN carried by the strains compared with the respective genes carried by pLI100. A maximum alignment score was also found comparing the mco gene with the homologous gene carried by the j1776 plasmid. All these findings were in agreement with previous authors reporting these plasmids as carrying determinants for cadmium, arsenic and copper resistance (Canchaya et al., 2010; Parsons et al., 2018; Chiaverini et al., 2021; Lachtara et al., 2021; Schmitz-Esser et al., 2021). Moreover, according to several studies, the specific plasmids found in the strains of this new ST are highly conserved between Lm strains on a large scale and across other STs, suggesting that they provide important advantages for survival in food and FPEs (Canchaya et al., 2010; Parsons et al., 2018; Chiaverini et al., 2021; Guidi et al., 2021; Lachtara et al., 2021; Maggio et al., 2021; Schmitz-Esser et al., 2021).
Moreover, the coexistence of Lm strains carrying and not carrying plasmids, even belonging to the same genetic cluster, indicated horizontal transfer dynamics within the microbial population studied, most probably driven by selective pressure.
Although not presenting a Premature Stop Codon Mutation in the inlA gene nor the SSI-1, both associated with an increased production of biofilm, as reported in previous studies (Keeney et al., 2018; Upham et al., 2019; Mahoney et al., 2022), all the strains carried several genetic markers for biofilm production. In particular luxS, for a S-ribosylhomocysteinase, recO encoding the DNA repair protein RecO and lmo2504 for a cell wall-binding protein were found in all the genomes (Pasquali et al., 2018; Gorski et al., 2022). The lmo0673 gene, encoding an hypothetical protein and reported as marker of biofilm production by Pasquali et al. (2018), was also found. These findings indicated the potential of biofilm formation of these strains. Regardless the effective amount of biofilm produced, if it is formed in niches that are difficult to reach during sanitation procedures, it can represent a persistent source of contamination. This could be another factor responsible for the survival of these ST2801 strains to disinfection.
Concerning the virulence profile, all the ST2801 strains presented the same identical pattern consistent with belonging to a IVb serogroup, albeit atypical and presenting hypervirulence features. Indeed, all the strains carried a full length inlA, encoding for a functional inlA, which is one of the essential virulence factors for Lm to cross the intestinal barrier. Other members of the internalin gene family were present in the strains, including inlC, inlD, inlE, inlF, inlH, inlJ, and inlK, which were known to contribute to the pathogenicity of the pathogen with different mechanisms (Su, 2019; Maćkiw et al., 2021). The aut_IVb, gltA, and gltB genes were also important virulence markers. These genes are involved in invasion and teichoic acid biosynthesis respectively, in serogroup IVb isolates, including IVb-v1, and are absent in other Lm (Moura et al., 2017).
The virulence profile of these ST2801 strains also included the additional LIPI-3, encoding a biosynthetic cluster involved in the production of listeriolysin S (LLS), a haemolytic, and cytotoxic factor conferring a greater virulence to Lm (Su, 2019; de Tavares et al., 2020). This finding was in agreement with previous studies reporting the presence of LIPI-3 in atypical IVb-v1 strains (Moura et al., 2017; de Tavares et al., 2020).
All these findings indicated this new ST, as presenting several determinants for stress response and environmental adaptation, probably responsible for its persistence in the meat-producing plant, together with virulence features. The isolation of a new MLST type with a large pattern of stress adaptation genes and able to persist over a year in the same FPE, also surviving cleaning and sanitation, is considered noteworthy.
Conclusion
In this study, we discovered a new MLST allelic profile of Lm designed as ST2801, never reported before, and belonging to the atypical serogroup IVb-v1. Moreover, only one study existing before ours witnessed the isolation of IV-v1 Lm strains from FPEs and their ability to persist over time.
The isolation of this new ST occurred during an intensive environmental sampling plan for Lm performed in a pork meat-producing plant of Central Italy, during production and, for positive surfaces, also after cleaning sanitation. We found widespread circulation of the ST2801 in the studied environment, where it was able to persist over a year and survive sanitation. Based on genomic characterization results, this new ST presents several determinants for stress response, environmental adaptation, and biofilm production, probably responsible for its persistence in the meat-producing plant, together with virulence features.
The isolation of a new ST with a large pattern of stress adaptation genes and the ability to persist in the same FPE for a year is an important contribution to deepen the current knowledge on the uncommon IVb-v1 and in general on genomic diversity of Lm. At the same time, the sampling approach adopted allowed us to provide specific recommendations to the FBO in order to improve the control of the pathogen, minimizing the risk of food contamination. All these findings pointed out how the application of intensive environmental sampling plans, which are specific to each FPE, would ensure improved surveillance and would provide the opportunity to increase knowledge about Lm.
Data Availability Statement
The datasets presented in this study can be found in online repositories. The names of the repository/repositories and accession number(s) can be found below: https://www.ncbi.nlm.nih.gov/bioproject/PRJNA821663.
Author Contributions
All authors listed have made a substantial, direct, and intellectual contribution to the work, and approved it for publication.
Funding
This study was funded by the Italian Ministry of Health (IZSUM RC0012019).
Conflict of Interest
The authors declare that the research was conducted in the absence of any commercial or financial relationships that could be construed as a potential conflict of interest.
Publisher’s Note
All claims expressed in this article are solely those of the authors and do not necessarily represent those of their affiliated organizations, or those of the publisher, the editors and the reviewers. Any product that may be evaluated in this article, or claim that may be made by its manufacturer, is not guaranteed or endorsed by the publisher.
Acknowledgments
We would like to thank the team of curators of the Institute Pasteur MLST databases (http://bigsdb.pasteur.fr/) for curating the data. Special thanks to Alexandra Moura (Institute Pasteur) for assigning the cgMLST CT type, for her technical support, and for the relevant professional advice regarding L. monocytogenes genotyping analyses.
Supplementary Material
The Supplementary Material for this article can be found online at: https://www.frontiersin.org/articles/10.3389/fmicb.2022.930895/full#supplementary-material
Supplementary Table 1 | Environmental sampling schema. FCS, food contact surface; NFCS, nonfood contact surface.
Supplementary Table 2 | IVb variant 1 Lm strains, environmental surfaces from which they have been isolated and sampling data.
Supplementary Table 3 | SNP distance matrix.
Footnotes
- ^ http://bigsdb.pasteur.fr/listeria
- ^ https://github.com/usadellab/Trimmomatic
- ^ https://github.com/s-andrews/FastQC
- ^ https://github.com/ablab/spades
- ^ https://github.com/ablab/quast
- ^ http://bigsdb.pasteur.fr/listeria
- ^ https://github.com/B-UMMI/chewBBACA
- ^ https://github.com/achtman-lab/GrapeTree
- ^ https://sourceforge.net/projects/ksnp/
- ^ https://itol.embl.de/
- ^ https://github.com/tseemann/prokka
- ^ https://cge.cbs.dtu.dk/services/PlasmidFinder/
References
Abdel-Motaal, H., Meng, L., Zhang, Z., Abdelazez, A. H., Shao, L., Xu, T., et al. (2018). An uncharacterized major facilitator superfamily transporter from Planococcus maritimus exhibits dual functions as a Na+(Li+, K+)/H+ antiporter and a multidrug efflux pump. Front. Microbiol. 9:1601. doi: 10.3389/fmicb.2018.01601
Baker, J. L., Sudarsan, N., Weinberg, Z., Roth, A., Stockbridge, R. B., and Breaker, R. R. (2012). Widespread genetic switches and toxicity resistance proteins for fluoride. Science 335, 233–235. doi: 10.1126/science.1215063
Bankevich, A., Nurk, S., Antipov, D., Gurevich, A. A., Dvorkin, M., Kulikov, A. S., et al. (2012). SPAdes: a new genome assembly algorithm and its applications to single-cell sequencing. J. Comput. Biol. 19, 455–477. doi: 10.1089/cmb.2012.0021
Bolger, A. M., Lohse, M., and Usadel, B. (2014). Trimmomatic: a flexible trimmer for Illumina sequence data. Bioinformatics 30, 2114–2120. doi: 10.1093/bioinformatics/btu170
Canchaya, C., Giubellini, V., Ventura, M., de los Reyes-Gavilán, C. G., and Margolles, A. (2010). Mosaic-Like sequences containing transposon, phage, and plasmid elements among Listeria monocytogenes plasmids. AEM 76, 4851–4857. doi: 10.1128/AEM.02799-2799
Carattoli, A., Zankari, E., García-Fernández, A., Larsen, M. V., Lund, O., Villa, L., et al. (2014). In silico detection and typing of plasmids using plasmidfinder and plasmid multilocus sequence typing. Antimicrobial Agents Chemotherapy 58:9. doi: 10.1128/AAC.02412-14
Carpentier, B., and Barre, L. (2012). Guidelines on Sampling the Food Processing Area and Equipment for the Detection of Listeria monocytogenes. French Agency for Food, Environmental and Occupational Health Safety – Maisons-Alfort. European Union Reference Laboratory for Listeria monocytogenes. Available online at: https://eurl-listeria.anses.fr/en/minisite/listeria-monocytogenes/mandate
Chellaiah, E. R., Ravi, P., and Uthandakalaipandian, R. (2021). Isolation and identification of high fluoride resistant bacteria from water samples of Dindigul district, Tamil Nadu, South India. Curr. Res. Microbial Sci. 2:100038. doi: 10.1016/j.crmicr.2021.100038
Chen, Y., Luo, Y., Curry, P., Timme, R., Melka, D., Doyle, M., et al. (2017). Assessing the genome level diversity of Listeria monocytogenes from contaminated ice cream and environmental samples linked to a listeriosis outbreak in the United States. PLoS One 12:e0171389. doi: 10.1371/journal.pone.0171389
Chen, Y., Simonetti, T., Peter, K., Jin, Q., Brown, E., LaBorde, L. F., et al. (2022). Genetic diversity of Listeria monocytogenes isolated from three commercial tree fruit packinghouses and evidence of persistent and transient contamination. Front. Microbiol. 12:756688. doi: 10.3389/fmicb.2021.756688
Cherifi, T., Arsenault, J., Pagotto, F., Quessy, S., Côté, J.-C., Neira, K., et al. (2020). Distribution, diversity and persistence of Listeria monocytogenes in swine slaughterhouses and their association with food and human listeriosis strains. PLoS One 15:e0236807. doi: 10.1371/journal.pone.0236807
Chiaverini, A., Guidi, F., Torresi, M., Acciari, V. A., Centorotola, G., Cornacchia, A., et al. (2021). Phylogenetic analysis and genome-wide association study applied to an Italian Listeria monocytogenes outbreak. Front. Microbiol. 12:750065. doi: 10.3389/fmicb.2021.750065
Cito, F., Di Pasquale, A., Cammà, C., and Cito, P. (2018). The Italian information system for the collection and analysis of complete genome sequence of pathogens isolated from animal, food and environment. Int. J. Infect. Dis. 73, 296–297. doi: 10.1016/j.ijid.2018.04.4090
Corbett, D., Schuler, S., Glenn, S., Andrew, P. W., Cavet, J. S., and Roberts, I. S. (2011). The combined actions of the copper-responsive repressor CsoR and copper-metallochaperone CopZ modulate CopA-mediated copper efflux in the intracellular pathogen Listeria monocytogenes: copper homeostasis in Listeria monocytogenes. Mol. Microbiol. 81, 457–472. doi: 10.1111/j.1365-2958.2011.07705.x
Cotter, P. D., Ryan, S., Gahan, C. G. M., and Hill, C. (2005). Presence of GadD1 glutamate decarboxylase in selected Listeria monocytogenes strains is associated with an ability to grow at low pH. Appl. Environ. Microbiol. 71, 2832–2839. doi: 10.1128/AEM.71.6.2832-2839.2005
de Tavares, R. M., Silva, D. A. L., da, Camargo, A. C., Yamatogi, R. S., and Nero, L. A. (2020). Interference of the acid stress on the expression of llsX by Listeria monocytogenes pathogenic island 3 (LIPI-3) variants. Food Res. Int. 132:109063. doi: 10.1016/j.foodres.2020.109063
de Vasconcelos, R. M., de Almeida, A. E. C. C., Hofer, E., da Silva, N. M. M., and Marin, V. A. (2008). Multiplex-PCR serotyping of Listeria monocytogenes isolated from human clinical specimens. Mem. Inst. Oswaldo Cruz 103, 836–838. doi: 10.1590/S0074-02762008000800016
Doumith, M., Buchrieser, C., Glaser, P., Jacquet, C., and Martin, P. (2004). Differentiation of the major Listeria monocytogenes serovars by multiplex PCR. J. Clin. Microbiol. 42, 3819–3822. doi: 10.1128/JCM.42.8.3819-3822.2004
European Food Safety Authority, and European Centre for Disease Prevention and Control (2021). The European Union One Health 2020 Zoonoses report. EFSA J. 19:6971. doi: 10.2903/j.efsa.2021.6971
Fang, H., Qin, X.-Y., Zhang, K.-D., Nie, Y., and Wu, X.-L. (2018). Role of the Group 2 Mrp sodium/proton antiporter in rapid response to high alkaline shock in the alkaline- and salt-tolerant Dietzia sp. DQ12-45-1b. Appl. Microbiol. Biotechnol. 102, 3765–3777. doi: 10.1007/s00253-018-8846-8843
Feng, Z., Liu, D., Wang, L., Wang, Y., Zang, Z., Liu, Z., et al. (2020). A putative efflux transporter of the ABC family, YbhFSR, in Escherichia coli functions in tetracycline efflux and Na+(Li+)/H+ transport. Front. Microbiol. 11:556. doi: 10.3389/fmicb.2020.00556
Gaballa, A., and Helmann, J. D. (2002). A peroxide-induced zinc uptake system plays an important role in protection against oxidative stress in Bacillus subtilis. Mol. Microbiol. 45, 997–1005. doi: 10.1046/j.1365-2958.2002.03068.x
Glaser, P., Frangeul, L., Buchrieser, C., Rusniok, C., Amend, A., Baquero, F., et al. (2001). Comparative genomics of listeria species. Science 294, 849–852. doi: 10.1126/science.1063447
Gorski, L., Cooley, M. B., Oryang, D., Carychao, D., Nguyen, K., Luo, Y., et al. (2022). Prevalence and clonal diversity of over 1,200 Listeria monocytogenes isolates collected from public access waters near produce production areas on the Central California Coast during 2011 to 2016. Appl. Environ. Microbiol. 88:e00357-22. doi: 10.1128/aem.00357-322
Graves, L. M., Broeker, R., Garette, N., and Swaminathan, B. (2007). “Comparison of a multiplex PCR assay and conventional serotyping for sero-classification of Listeria monocytogenes isolates in the USA, 2005-2006,” in Poster Communication P02. Proceedings of the 16th International Symposium on Problems of Listeriosis. ISOPOL XVI, (Savannah, GA).
Guidi, F., Orsini, M., Chiaverini, A., Torresi, M., Centorame, P., Acciari, V. A., et al. (2021). Hypo- and hyper-virulent Listeria monocytogenes clones persisting in two different food processing plants of Central Italy. Microorganisms 9:376. doi: 10.3390/microorganisms9020376
Gurevich, A., Saveliev, V., Vyahhi, N., and Tesler, G. (2013). QUAST: quality assessment tool for genome assemblies. Bioinformatics 29, 1072–1075. doi: 10.1093/bioinformatics/btt086
Huang, B., Fang, N., Dimovski, K., Wang, X., Hogg, G., and Bates, J. (2011). Observation of a new pattern in serogroup-related PCR typing of Listeria monocytogenes 4b isolates. J. Clin. Microbiol. 49, 426–429. doi: 10.1128/JCM.01207-1210
Johnston, N. R., and Strobel, S. A. (2020). Principles of fluoride toxicity and the cellular response: a review. Arch. Toxicol. 94, 1051–1069. doi: 10.1007/s00204-020-02687-2685
Keeney, K., Trmcic, A., Zhu, Z., Delaquis, P., and Wang, S. (2018). Stress survival islet 1 contributes to serotype-specific differences in biofilm formation in Listeria monocytogenes. Lett. Appl. Microbiol. 67, 530–536. doi: 10.1111/lam.13072
Kérouanton, A., Marault, M., Petit, L., Grout, J., Dao, T. T., and Brisabois, A. (2010). Evaluation of a multiplex PCR assay as an alternative method for Listeria monocytogenes serotyping. J. Microbiol. Methods 80, 134–137. doi: 10.1016/j.mimet.2009.11.008
Kim, S. W., Haendiges, J., Keller, E. N., Myers, R., Kim, A., Lombard, J. E., et al. (2018). Genetic diversity and virulence profiles of Listeria monocytogenes recovered from bulk tank milk, milk filters, and milking equipment from dairies in the United States (2002 to 2014). PLoS One 13:e0197053. doi: 10.1371/journal.pone.0197053
Knudsen, G. M., Nielsen, J. B., Marvig, R. L., Ng, Y., Worning, P., Westh, H., et al. (2017). Genome-wide-analyses of Listeria monocytogenes from food-processing plants reveal clonal diversity and date the emergence of persisting sequence types: emergence and diversity of L. monocytogenes. Environ. Microbiol. Rep. 9, 428–440. doi: 10.1111/1758-2229.12552
Kuenne, C., Voget, S., Pischimarov, J., Oehm, S., Goesmann, A., Daniel, R., et al. (2010). Comparative analysis of plasmids in the genus listeria. PLoS One 5:e12511. doi: 10.1371/journal.pone.0012511
Lachtara, B., Osek, J., and Wieczorek, K. (2021). Molecular typing of Listeria monocytogenes IVb serogroup isolated from food and food production environments in Poland. Pathogens 10:482. doi: 10.3390/pathogens10040482
Leclercq, A., Chenal-Francisque, V., Dieye, H., Cantinelli, T., Drali, R., Brisse, S., et al. (2011). Characterization of the novel Listeria monocytogenes PCR serogrouping profile IVb-v1. Int. J. Food Microbiol. 147, 74–77. doi: 10.1016/j.ijfoodmicro.2011.03.010
Lee, S., Ward, T. J., Graves, L. M., Wolf, L. A., Sperry, K., Siletzky, R. M., et al. (2012). Atypical Listeria monocytogenes serotype 4b strains harboring a lineage II-Specific gene cassette. Appl. Environ. Microbiol. 78, 660–667. doi: 10.1128/AEM.06378-6311
Liu, Y., Orsi, R. H., Gaballa, A., Wiedmann, M., Boor, K. J., and Guariglia-Oropeza, V. (2019). Systematic review of the Listeria monocytogenes σ B regulon supports a role in stress response, virulence and metabolism. Future Microbiol. 14, 801–828. doi: 10.2217/fmb-2019-2072
Maćkiw, E., Korsak, D., Kowalska, J., Felix, B., Stasiak, M., Kucharek, K., et al. (2021). Incidence and genetic variability of Listeria monocytogenes isolated from vegetables in Poland. Int. J. Food Microbiol. 339, 109023. doi: 10.1016/j.ijfoodmicro.2020.109023
Mafuna, T., Matle, I., Magwedere, K., Pierneef, R. E., and Reva, O. N. (2021). Whole genome-based characterization of Listeria monocytogenes isolates recovered from the food chain in South Africa. Front. Microbiol. 12:669287. doi: 10.3389/fmicb.2021.669287
Maggio, F., Rossi, C., Chiaverini, A., Ruolo, A., Orsini, M., Centorame, P., et al. (2021). Genetic relationships and biofilm formation of Listeria monocytogenes isolated from the smoked salmon industry. Int. J. Food Microbiol. 356:109353. doi: 10.1016/j.ijfoodmicro.2021.109353
Mahoney, D. B. J., Falardeau, J., Hingston, P., Chmielowska, C., Carroll, L. M., Wiedmann, M., et al. (2022). Associations between Listeria monocytogenes genomic characteristics and adhesion to polystyrene at 8 °C. Food Microbiol. 102:103915. doi: 10.1016/j.fm.2021.103915
Matereke, L. T., and Okoh, A. I. (2020). Listeria monocytogenes virulence, antimicrobial resistance and environmental persistence: a review. Pathogens 9:528. doi: 10.3390/pathogens9070528
Maury, M. M., Bracq-Dieye, H., Huang, L., Vales, G., Lavina, M., Thouvenot, P., et al. (2019). Hypervirulent Listeria monocytogenes clones’ adaption to mammalian gut accounts for their association with dairy products. Nat. Commun. 10:2488. doi: 10.1038/s41467-019-10380-10380
Maury, M. M., Tsai, Y.-H., Charlier, C., Touchon, M., Chenal-Francisque, V., Leclercq, A., et al. (2016). Uncovering Listeria monocytogenes hypervirulence by harnessing its biodiversity. Nat. Genet. 48, 308–313. doi: 10.1038/ng.3501
Morganti, M., Scaltriti, E., Cozzolino, P., Bolzoni, L., Casadei, G., Pierantoni, M., et al. (2016). Processing-Dependent and clonal contamination patterns of Listeria monocytogenes in the cured ham food chain revealed by genetic analysis. Appl. Environ. Microbiol. 82, 822–831. doi: 10.1128/AEM.03103-3115
Moura, A., Criscuolo, A., Pouseele, H., Maury, M. M., Leclercq, A., Tarr, C., et al. (2017). Whole genome-based population biology and epidemiological surveillance of Listeria monocytogenes. Nat. Microbiol. 2:16185. doi: 10.1038/nmicrobiol.2016.185
Mullapudi, S., Siletzky, R. M., and Kathariou, S. (2008). Heavy-Metal and benzalkonium chloride resistance of Listeria monocytogenes isolates from the environment of Turkey-Processing plants. AEM 74, 1464–1468. doi: 10.1128/AEM.02426-2427
Orsi, R. H., Bakker, H. C., den, and Wiedmann, M. (2011). Listeria monocytogenes lineages: genomics, evolution, ecology, and phenotypic characteristics. Int. J. Med. Microbiol. 301, 79–96. doi: 10.1016/j.ijmm.2010.05.002
Osman, D., Cooke, A., Young, T. R., Deery, E., Robinson, N. J., and Warren, M. J. (2021). The requirement for cobalt in vitamin B12: a paradigm for protein metalation. Biochim. Biophys. Acta (BBA) - Mol. Cell Res. 1868:118896. doi: 10.1016/j.bbamcr.2020.118896
Palaiodimou, L., Fanning, S., and Fox, E. M. (2021). Genomic insights into persistence of Listeria species in the food processing environment. J. Appl. Microbiol. 131, 2082–2094. doi: 10.1111/jam.15089
Palma, F., Pasquali, F., Lucchi, A., Cesare, A. D., and Manfreda, G. (2017). Whole genome sequencing for typing and characterisation of Listeria monocytogenes isolated in a rabbit meat processing plant. Italian J. Food Safety 6:6. doi: 10.4081/ijfs.2017.6879
Parsons, C., Lee, S., and Kathariou, S. (2018). Heavy metal resistance determinants of the foodborne pathogen Listeria monocytogenes. Genes 10:11. doi: 10.3390/genes10010011
Parsons, C., Lee, S., and Kathariou, S. (2020). Dissemination and conservation of cadmium and arsenic resistance determinants in Listeria and other Gram-positive bacteria. Mol. Microbiol. 113, 560–569. doi: 10.1111/mmi.14470
Pasquali, F., Palma, F., Guillier, L., Lucchi, A., De Cesare, A., and Manfreda, G. (2018). Listeria monocytogenes sequence types 121 and 14 repeatedly isolated within one year of sampling in a rabbit meat processing plant: persistence and ecophysiology. Front. Microbiol. 9:596. doi: 10.3389/fmicb.2018.00596
Pombinho, R., Camejo, A., Vieira, A., Reis, O., Carvalho, F., Almeida, M. T., et al. (2017). Listeria monocytogenes CadC regulates cadmium efflux and fine-tunes lipoprotein localization to escape the host immune response and promote infection. J. Infect. Dis. 215, 1468–1479. doi: 10.1093/infdis/jix118
Portmann, A.-C., Fournier, C., Gimonet, J., Ngom-Bru, C., Barretto, C., and Baert, L. (2018). A validation approach of an end-to-end whole genome sequencing workflow for source tracking of Listeria monocytogenes and Salmonella enterica. Front. Microbiol. 9:446. doi: 10.3389/fmicb.2018.00446
Ragon, M., Wirth, T., Hollandt, F., Lavenir, R., Lecuit, M., Le Monnier, A., et al. (2008). A new perspective on Listeria monocytogenes evolution. PLoS Pathog 4:e1000146. doi: 10.1371/journal.ppat.1000146
Ryan, S., Begley, M., Hill, C., and Gahan, C. G. M. (2010). A five-gene stress survival islet (SSI-1) that contributes to the growth of Listeria monocytogenes in suboptimal conditions: stress survival islet in L. monocytogenes. J. Appl. Microbiol. 109, 984–995. doi: 10.1111/j.1365-2672.2010.04726.x
Scaltriti, E., Bolzoni, L., Vocale, C., Morganti, M., Menozzi, I., Re, M. C., et al. (2020). Population structure of Listeria monocytogenes in Emilia-Romagna (Italy) and implications on whole genome sequencing surveillance of Listeriosis. Front. Public Health 8:519293. doi: 10.3389/fpubh.2020.519293
Schmitz-Esser, S., Anast, J. M., and Cortes, B. W. (2021). A large-scale sequencing-based survey of plasmids in Listeria monocytogenes reveals global dissemination of plasmids. Front. Microbiol. 12:653155. doi: 10.3389/fmicb.2021.653155
Seemann, T. (2014). Prokka: rapid prokaryotic genome annotation. Bioinformatics 30, 2068–2069. doi: 10.1093/bioinformatics/btu153
Sharma, A., Gupta, V., and Pathania, R. (2019). Efflux pump inhibitors for bacterial pathogens: from bench to bedside. Indian J. Med. Res. 149:129. doi: 10.4103/ijmr.IJMR_2079_17
Silva, M., Machado, M. P., Silva, D. N., Rossi, M., Moran-Gilad, J., Santos, S., et al. (2018). chewBBACA: a complete suite for gene-by-gene schema creation and strain identification. Microbial Genomics 4:e000166. doi: 10.1099/mgen.0.000166
Simonetti, T., Peter, K., Chen, Y., Jin, Q., Zhang, G., LaBorde, L. F., et al. (2021). Prevalence and distribution of Listeria monocytogenes in three commercial tree fruit packinghouses. Front. Microbiol. 12:652708. doi: 10.3389/fmicb.2021.652708
Slipski, C. J., Zhanel, G. G., and Bay, D. C. (2018). Biocide selective TolC-Independent efflux pumps in Enterobacteriaceae. J. Membrane Biol. 251, 15–33. doi: 10.1007/s00232-017-9992-9998
Southworth, T. W., Guffanti, A. A., Moir, A., and Krulwich, T. A. (2001). GerN, an endospore germination protein of Bacillus cereus, is an Na + /H + -K + Antiporter. J. Bacteriol. 183, 5896–5903. doi: 10.1128/JB.183.20.5896-5903.2001
Su, X. (2019). Characterization of internalin genes in Listeria monocytogenes from food and humans, and their association with the invasion of Caco-2 cells. Gut Pathogens 11:30. doi: 10.1186/s13099-019-0307-308
Timme, R. E., Wolfgang, W. J., Balkey, M., Gubbala Venkata, S. L., Randolph, R., Allard, M., et al. (2020). Optimizing open data to support one health: best practices to ensure interoperability of genomic data from microbial pathogens. One Health Outlook 2:20. doi: 10.1186/s42522-020-00026-23
Torres, C., Galián, C., Freiberg, C., Fantino, J.-R., and Jault, J.-M. (2009). The YheI/YheH heterodimer from Bacillus subtilis is a multidrug ABC transporter. Biochimica Biophys. Acta (BBA) - Biomembranes 1788, 615–622. doi: 10.1016/j.bbamem.2008.12.012
Torresi, M., Orsini, M., Acciari, V., Centorotola, G., Di Lollo, V., Di Domenico, M., et al. (2020). Genetic characterization of a Listeria monocytogenes serotype IVb variant 1 strain isolated from vegetal matrix in Italy. Microbiol. Resour. Announc. 9:e00782-20. doi: 10.1128/MRA.00782-720
Upham, J., Chen, S., Boutilier, E., Hodges, L., Eisebraun, M., Croxen, M. A., et al. (2019). Potential Ad hoc markers of persistence and virulence in canadian Listeria monocytogenes food and clinical isolates. J. Food Prot. 82, 1909–1921. doi: 10.4315/0362-028X.JFP-19-028
Wu, T., Wu, X.-Q., Xu, X., Kong, W., and Wu, F. (2020). Salt tolerance mechanism and species identification of the plant rhizosphere bacterium JYZ-SD2. Curr. Microbiol. 77, 388–395. doi: 10.1007/s00284-019-01835-1830
Xu, N., Zheng, Y., Wang, X., Krulwich, T. A., Ma, Y., and Liu, J. (2018). The lysine 299 residue endows the multisubunit Mrp1 antiporter with dominant roles in Na + Resistance and pH homeostasis in Corynebacterium glutamicum. Appl. Environ. Microbiol. 84:e00110-18. doi: 10.1128/AEM.00110-118
Yan, L., Wang, C., Jiang, J., Liu, S., Zheng, Y., Yang, M., et al. (2022). Nitrate removal by alkali-resistant Pseudomonas sp. XS-18 under aerobic conditions: performance and mechanism. Bioresource Technol. 344, 126175. doi: 10.1016/j.biortech.2021.126175
Yang, H., Hoffmann, M., Allard, M. W., Brown, E. W., and Chen, Y. (2020). Microevolution and gain or loss of mobile genetic elements of outbreak-related Listeria monocytogenes in food processing environments identified by whole genome sequencing analysis. Front. Microbiol. 11:866. doi: 10.3389/fmicb.2020.00866
Zhang, J., Buhe, C., Yu, D., Zhong, H., and Wei, Y. (2020). Ammonia stress reduces antibiotic efflux but enriches horizontal gene transfer of antibiotic resistance genes in anaerobic digestion. Bioresour. Technol. 295, 122191. doi: 10.1016/j.biortech.2019.122191
Keywords: Listeria monocytogenes, serogroup IVb-v1, ST2801 (CC218), food producing environment, persistence, WGS typing, environmental sampling
Citation: Guidi F, Lorenzetti C, Centorotola G, Torresi M, Cammà C, Chiaverini A, Pomilio F and Blasi G (2022) Atypical Serogroup IVb-v1 of Listeria monocytogenes Assigned to New ST2801, Widely Spread and Persistent in the Environment of a Pork-Meat Producing Plant of Central Italy. Front. Microbiol. 13:930895. doi: 10.3389/fmicb.2022.930895
Received: 28 April 2022; Accepted: 06 June 2022;
Published: 27 June 2022.
Edited by:
Tiziana Nardi, Council for Agricultural Research and Economics (CREA), ItalyReviewed by:
Itumeleng Matle, Agricultural Research Council of South Africa (ARC-SA), South AfricaFrederique Pasquali, University of Bologna, Italy
Copyright © 2022 Guidi, Lorenzetti, Centorotola, Torresi, Cammà, Chiaverini, Pomilio and Blasi. This is an open-access article distributed under the terms of the Creative Commons Attribution License (CC BY). The use, distribution or reproduction in other forums is permitted, provided the original author(s) and the copyright owner(s) are credited and that the original publication in this journal is cited, in accordance with accepted academic practice. No use, distribution or reproduction is permitted which does not comply with these terms.
*Correspondence: Fabrizia Guidi, Zi5ndWlkaUBpenN1bS5pdA==, Z3VpZGlmYWJyaXppYUB5YWhvby5pdA==