- 1School of Food and Engineering, Jilin Agricultural University, Changchun, China
- 2Key Laboratory of Jilin Province for Zoonosis Prevention and Control, Changchun Veterinary Research Institute, Chinese Academy of Agricultural Sciences, Changchun, China
- 3China-Japan Union Hospital, Jilin University, Changchun, China
Objective: To precisely determine the species of a carbapenem-resistant Pseudomonas strain 1809276 isolated from the urine of a Chinese patient and analyze its integrative and conjugative element (ICE) 1276 formation mechanism.
Methods: Single-molecule real-time (SMRT) sequencing was carried out on strain 18091276 to obtain the complete chromosome and plasmid (pCN1276) sequences, and average nucleotide identity (ANI) was used for precise species identification. The ICEs in GenBank with the same integrase structure as ICE 1276 were aligned. At the same time, the transfer ability of blaIMP−1 and the antibiotic sensitivity of Pseudomonas juntendi 18091276 were tested.
Results: This bacterium was P. juntendi, and its drug resistance mechanism is the capture of the accA4' gene cassette by the Tn402-like type 1 integron (IntI1-blaIMP−1) to form In1886 before its capture by the ΔTn4662a-carrying ICE 1276. The acquisition of blaIMP−1 confers carbapenem resistance to P. juntendi 18091276.
Conclusion: The formation of blaIMP−1-carrying ICE 1276, its further integration into the chromosomes, and transposition and recombination of other elements promote bacterial gene accumulation and transmission.
Introduction
Pseudomonas sp. is a genus of varied and complex Gram-negative bacteria commonly isolated from soil and water, with a wide host diversity, including animals and plants. It consists of 254 species (List of Prokaryotic names with Standing in Nomenclature, LPSN; www.bacterio.net) divided into three lineages and 13 groups based on the sequences of the 16S rRNA, gyrB, rpoB, and rpoD genes (Peix et al., 2018). Among them, several species of Pseudomonas sp. were regarded as important opportunistic pathogens affecting humans, including Pseudomonas aeruginosa, Pseudomonas asiatica, Pseudomonas fluorescens, Pseudomonas putida, Pseudomonas cepacia, Pseudomonas stutzeri, Pseudomonas maltophilia, and Pseudomonas putrefaciens (Tohya et al., 2019). Their capabilities of acquirement of exogenous genes lead to the prone of antibiotics resistance in this genus, including carbapenem antibiotics resistance, which enhances the difficulties of treatment. Pseudomonas sp. can acquire carbapenem resistance genes by integrons, which further can be captured by other mobile elements (such as plasmids, transposons, etc.), and then, these genes begin to spread among other bacteria.
In this study, we analyzed a P. juntendi strain separated from a urine specimen of a patient in China in 2018, which was tentatively identified as P. putida by VITEK2 (bioMerieux), and the strain carried a carbapenem resistance gene blaIMP−1. To our knowledge, this is the first report of clinical strain of P. juntendi carrying blaIMP−1 in a new gene context in China.
Materials and methods
Bacterial isolation and identification
Strain 18091276 was isolated from a urine specimen from a patient in a tertiary hospital in northeast China in 2018 and the species of the strain was determined by the part of the sequence of the 16S rRNA gene (Edwards et al., 1989). Then, a maximum likelihood (ML) evolutionary tree was constructed using MEGA 7.0 software to evaluate the sequence similarity of the 16S rRNA genes that were aligned and listed in the BLASTN program (top 100).
The minimum inhibitory concentration (MIC) of imipenem was determined by the broth microdilution method according to the Clinical and Laboratory Standards Institute (CLSI, 2020) guidelines, and Escherichia coli ATCC 25922 was used as a control. MICs of amikacin, gentamicin, meropenem, cefazolin, ceftazidime, cefotaxime, cefepime, aztreonam, ampicillin, piperacillin, amoxicillin-clavulanate, ampicillin-sulbactam, piperacillin-tazobactam, colistin, trimethoprim-sulfamethoxazole, chloramphenicol, ciprofloxacin, levofloxacin, moxifloxacin, and tetracycline were tested by BD Phoenix-100.
Sequencing and sequence assembly
Bacterial genomic DNA was extracted from the strain 18091276 using the UltraClean Microbial Kit (Qiagen, NW, Germany) and sequenced by a PacBio RSII sequencer (Pacific Biosciences, CA, USA). The reads were assembled de novo by using SMARTdenovo (http://github.com/ruanjue/smartdenovo).
Sequence annotation and comparison
Rast 2.0 (Brettin et al., 2015) and BLASTP/BLASTN (Boratyn et al., 2013) searches were used to predict open reading frames (ORFs), online databases CARD (https://card.mcmaster.ca/; Alcock et al., 2020), and ResFinder 4.1 (https://cge.cbs.dtu.dk/services/ResFinder/; Bortolaia et al., 2020) to find out resistance genes, and ISfinder (https://www-is.biotoul.fr/; Lastest Database Update 2021-9-21; Varani et al., 2011), TnCentral (https://tncentral.proteininformationresource.org/), and ICEberg 2.0 (http://db-mml.sjtu.edu.cn/ICEberg/; Liu et al., 2019) were used to find out the mobile elements. Pairwise sequence comparisons were carried out by BLASTN. Gene organization diagrams were drawn by Inkscape 1.0 (http://inkscape.org/en/).
Bacterial precise species identification and evolutionary tree construction
Bacterial precise species identification was performed using the pair-wise average nucleotide identity (ANI) analysis between strain 18091276 and the reference genome (http://www.ezbiocloud.net/tools/ani). A ≥95% ANI cutoff was used to define bacterial species (Yoon et al., 2017). CSI Phylogeny (https://cge.cbs.dtu.dk/services/CSIPhylogeny/; Kaas et al., 2014) calls and filters single nucleotide polymorphisms (SNPs) of strain 18091276, does site validation, and infers a phylogeny based on the concatenated alignment of the high-quality SNPs. In addition, the MUSCLE software program was used to align multiple single-copy core-encoded proteins identified by the core-/pan-genome analysis. The aligned sequences were then subjected to phylogenetic analysis using the TreeBeST (Version 1.9.2) program, a neighbor-joining tree reconstruction algorithm, and 1,000 bootstrap replicates (Nandi et al., 2010).
Conjugation experiments
Conjugation experiments were performed as described previously (Mizuno et al., 2020). Briefly, strain 18091276 was used as a donor and sodium azide-resistant E.coli J53 as a recipient. Donor and recipient strains were cultured overnight at 37°C separately. Then, 3 ml of 18091276 culture was mixed up with an equal volume of E.coli J53 culture. The mixed cells were harvested by centrifugation for 3 min at 12,000 × g, washed with 3 ml of lysogeny broth (LB), and resuspended in 150 μl of LB. The mixture was spotted on a 1 cm2 hydrophilic nylon membrane filter (Millipore) with a 0.45-μm pore size, which was then placed on an LB agar plate and then incubated for mating at 37°C for 6 h. The cells were recovered from the filter membrane and spotted on LB agar containing 100 μg/ml sodium azide and 4 μg/ml imipenem for selecting carbapenem-resistant E. coli transconjugant.
Nucleotide sequence accession numbers
The complete sequence of 18091276 has been submitted to GenBank under the accession number CP091311.
Results and discussion
After the 18091276 strain was cultured overnight at 37°C, 2 mm round protruding colonies with smooth and regular edges, non-fusion growth, non-pyocyanin production, and the absence of metallic sheen were found on brain heart infusion agar (imipenem concentration: 4 μg/ml).
Strain 18091276 was identified by the BD Phoenix-100 identification system and VITEK 2 as P. putida, and Table 1 shows its drug resistance spectrum. The similarity of the 16S rRNA sequence of this bacterium and P. putida, Pseudomonas monteilii, Pseudomonas plecoglossicida, and P. juntendi in the P. putida group was more than 99% and the strain was identified to be in the P. putida group (Supplementary Figure 1). After single-molecule real-time (SMRT) sequencing (basic information about SMRT sequence results was provided in Supplementary Table 1), it was found that the ANI value of strain 18091276 was more than 95% with the reference strain P. juntendi BML3 (GCA_009932375.1), and this strain was confirmed to be P. juntendi (ANI value of P. juntendi 18091276 were provided in Supplementary Table 2A). In 2019, Tohya M. was the first to carry out a systematic identification of P. juntendi and officially named this species (Tohya et al., 2019). The analysis of all 14 strains that were annotated as P. juntendi in GenBank (cutoff date was November 2021; Table 2) found that the clinical isolates before 2018 were from Brazil, and the isolates in China, the USA, Japan, and Russia only appeared after 2018. Although P. juntendi 18091276 and 14181154 were all isolated in China, they had a far phylogenetic relationship (Figure 1). According to the SNPs and core-genome phylogenetic tree, P. juntendi 18091276 was the closest relative to P. juntendi 12349 (Brazil, 2012) and P. juntendi PSB00036 (the USA, 2018; Figure 1). We speculate that P. juntendi 18091276 might be transferred from the USA or Brazil through international food (animal- and plant-based) trade and travel. The identification method at the time was not precise, many P. juntendi isolates were wrongly identified as P. putida or other species in the P. putida group (Morimoto et al., 2020), resulting in fewer epidemiological data and whole-genome data of this strain in other countries. ResFinder screening found that, out of the 14 P. juntendi strains, six Brazilian strains carried the carbapenem resistance genes blaIMP and blaVIM and two Chinese strains carried blaIMP (see Table 2 for strain information), showing that P. juntendi could acquire blaIMP and blaVIM, like P. aeruginosa and P. putida, and develop carbapenem resistance, and it is potentially harmful.
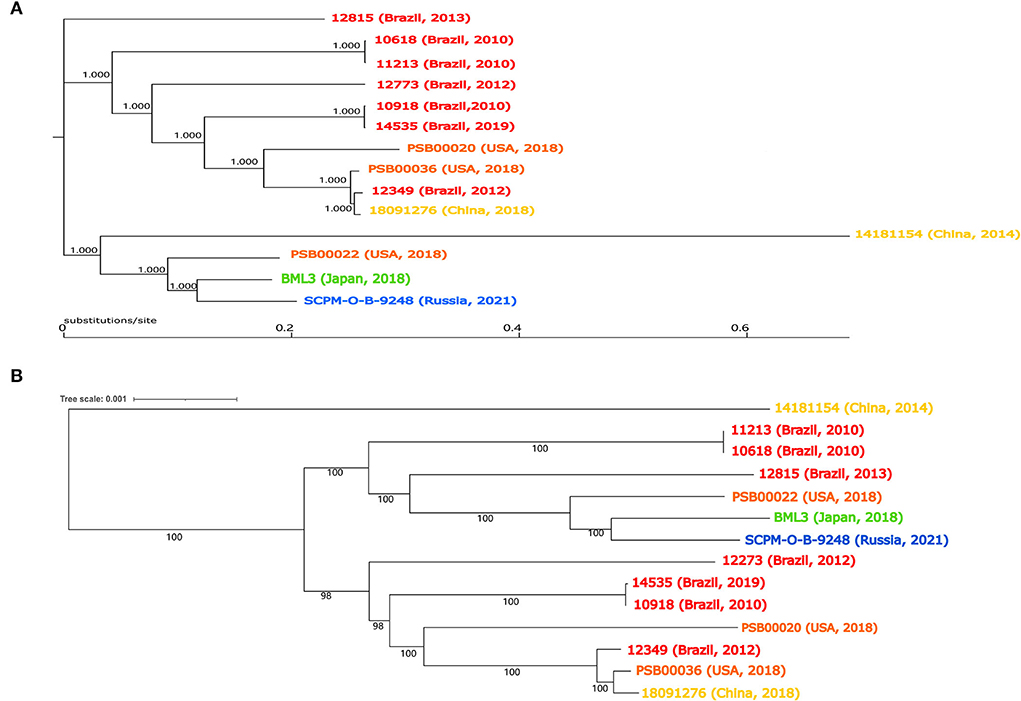
Figure 1. Pseudomonas juntendi genome phylogenetic tree. The top half is the phylogenetic tree constructed from SNPs of genomes (A). The bottom half of the figure is the phylogenetic tree constructed from core genomes (B). The Brazilian isolates are labeled in red, Chinese isolates are labeled in khaki, American isolates are labeled orange, Japanese isolates are labeled green, and Russian isolates are labeled blue.
The chromosome of P. juntendi 18091276 contained an 88.61 kb integrative and conjugative element (ICE) that was named ICE 1276. ICE 1276 contained attL and attR produced from recombination, a complete integrase gene (int), the F-type IV secretion system responsible for the ICE transfer, the conjugation gene cpl, and rlx responsible for autonomous rolling circle replication (Johnson and Grossman, 2015). ICE 1276 did not contain the cleavage enzyme xis or the origin of replication oriT. crpP (quinolone resistance gene) was also found in the backbone region of ICE 1276. crpP was mainly present in the ICEs of Pseudomonas aeruginosa and could increase ciprofloxacin resistance (Zhu et al., 2021). However, P. juntendi 18091276 did not possess quinolone resistance. In addition, a 15.96-kb accessory module was discovered in ICE 1276, and this structure had the closest phylogenetic relationship with Tn4662a (a Tn3 family transposon) in plasmid pDK1 (NC_014124.1; coverage: 58%, identity: 98.65%). Moreover, it had the same 38 bp inverted repeat sequence (IRs) as Tn4662a, and a 5-bp (AGTAT) directed repeat sequence (DRs) was generated during insertion (detailed information is shown in Supplementary Table 3). Tn4662a was first found on a plasmid pDK1 carried by P. putida HS (Yano et al., 2010). In contrast to Tn4662a (pDK1), the insertion structure of P. juntendi 18091276 also contained a Tn6811 remnant (Tn3 family), a single copy insertion element ISPsfu1 (IS5 family), and a complete In1886 (Tn402-like type 1 integron) carrying blaIMP−1 and aacA4'. However, the res_site_II, res_site_III, tnpR, and tnpA sequences in Tn4662a (ICE 1276) were partially deleted, which should have a Tn4662a-derived structure (Figure 2).
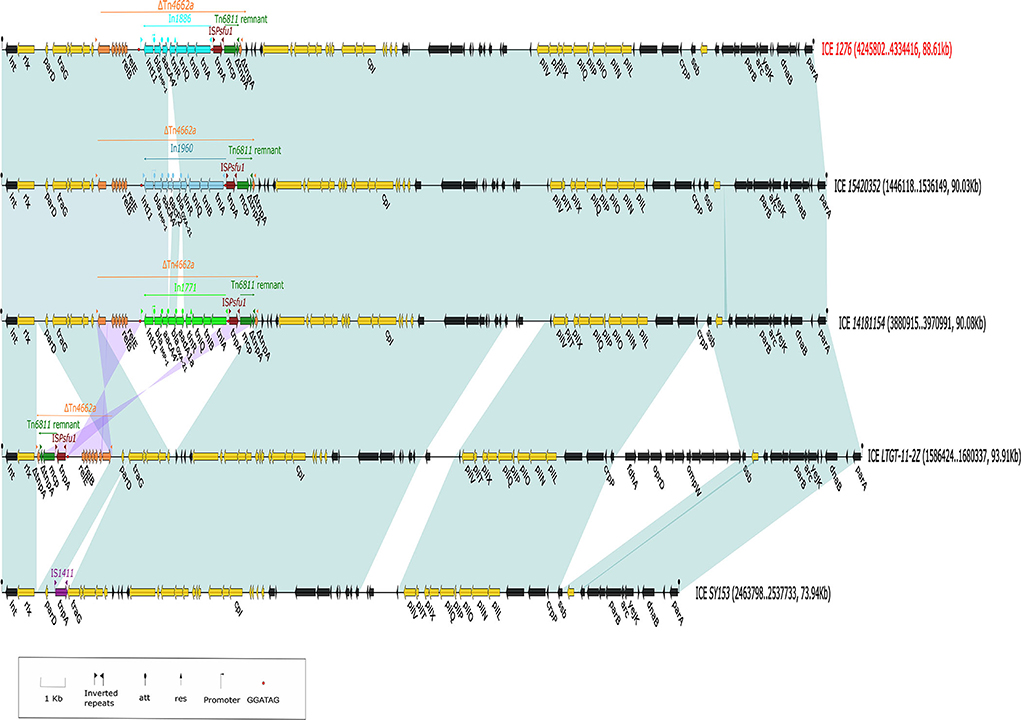
Figure 2. Linear alignment map of integrative and conjugative elements (ICEs). The integration region is yellow, the backbone region is black, In1886 is light blue, In1960 is blue, In1771 is green, Tn4662a is orange, ISPsfu1 is dark red, Tn6811 is dark green, and IS1411 is purple. The solid red dot is the integron inverted repeat (IR) recognition sequence GGATAG, and the shaded region represents regions with >90% nucleotide identity. Except for Pseudomonas putida SY153 ICE SY153, the other ICEs were all Tn4662a-derived structures. IS1411 (ISL3 family) recognizes the 8 bp binding site (ATCAAAGG) on traG through IR, thereby inserting it into ICE SY153 and causing the binding site to form directed repeat (DR). Except for the Tn4662a-derived structure in the ICE of Pseudomonas sp. LTGT-11-2Z that was inserted in the 257 bp site downstream of rlx after inversion, Tn4662a-derived structures from other strains were inserted in the positive direction 296 bp upstream of the integrating conjugative element protein of the TIGR03757 family in these ICEs. The integron IR recognition site (GATAGG) was similarly present at 1,153 bp upstream of the res site on Tn4662a (ICE LTGT-11-2Z), but no integron was inserted. A 14.29-kb accessory module (dark pink) was also inserted 74 bp upstream of the ssb (single-strand DNA binding protein) in the backbone region of ICE LTGT-11-2Z and contains fdhA (formate dehydrogenase), oprD (outer membrane porin protein), and ompW (outer membrane protein). Alignment of ICE 1276 with ICE 14181154 from P. juntendi 14181154 and ICE 15420352 from P. putida 15420352 found that only the number of gene cassettes captured by the Tn402-like integron was different, and other structures were identical.
Until November 2021, only 4 ICEs with the same integrase as ICE 1276 were indexed in GenBank (Table 3 shows the strain information). Interestingly, these ICE 1276-carrying strains were Pseudomonas sp. from China. ANI calculator was used to precisely analyze the identified Pseudomonas sp. 14181154 and LTGT-11-2Z. The ANI value of Pseudomonas sp. 14181154 and the reference strain P. juntendi BML3 was more than 95% (ANI value in Supplementary Table 2B), so Pseudomonas sp. 14181154 was identified as P. juntendi (Figure 1 shows the phylogenetic relationship with other P. juntendi strains), but the ANI of LTGT-11-2Z and all Pseudomonas species were lower than 95%. Therefore, LTGT-11-2Z was only identified as Pseudomonas sp. All ICEs were obtained from Pseudomonas sp. chromosomes, and E. coli transconjugants were not obtained after repeated conjugation experiments. Except for the exogenous insertion sites, the remaining structure of the ICEs was nearly identical. Another exception is that a 14.29 kb accessory module was inserted at 74 bp upstream of ssb (single-strand DNA binding protein) in the backbone region of ICE LTGT-11-2Z (plant-derived Pseudomonas sp. LTGT-11-2Z). This ICE seemed to have caused the transfer of large fragments between Pseudomonas species under specific conditions.
As the downstream region of Tn4662a is truncated by Tn6811, it is unable to carry out normal replicative transposition (Grindley and Reed, 1985), but it still contains an intact res_site_I (Supplementary Table 4A) and can recombine with In1886 and ISPsfu1 (Grindley and Reed, 1985; Brovedan et al., 2021) (Figure 2). IRs and DRs were absent at both ends of ISPsfu1-In1886 and did not constitute a composite transposon (Zong, 2014). Alignment with 10 Tn402-like integrons containing blaIMP−1 and aacA4' gene cassettes that were indexed before November 2021 (Table 4 shows the strain information) found that except for In1960, In1771, and In1886 located in ICEs, the ISPsfu1 element was not inserted upstream or downstream of the remaining integrons (regardless of whether it is on the plasmid or chromosome) and there were two independent insertion processes. The Tn4662a-drived structure in ICE LTGT-11-2Z (Figure 2) indicated that, after ISPsfu1 had undergone non-replicative transposition, In1886 was inserted upstream of the res_site_I of ΔTn4662a (Figure 3 shows the formation process of ICE 1276).
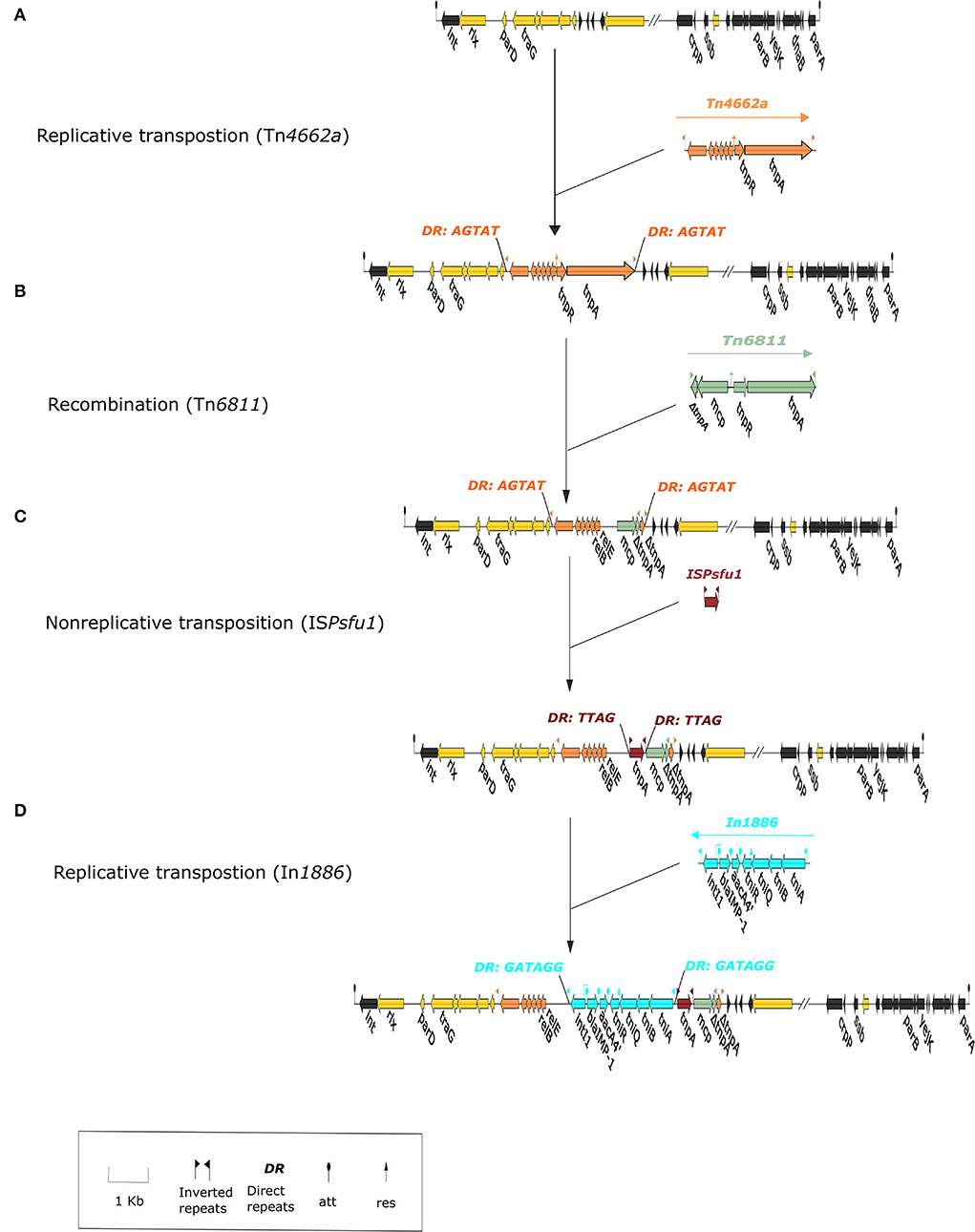
Figure 3. The formation process of integrative and conjugative element (ICE) 1276. The figure shows the ICE 1276 structure as a local structure. The integration region of ICE 1276 is yellow, the backbone region is black, In1886 is light blue; (A) Tn4662a wasrecombined in ICE 1276 through replicative transposition and produces bilateral directed repeat sequence (DRs) (AGTAT); (B) Tn6811 and Tn4662a underwent recombination to form the ΔTn4662a-ΔTn6811 structure; the truncated part of Tn4662a includes tnpA, complete deletion of tnpR, and the recombination sites res_site_II and res_site_III; (C) ISPsfu1 was inserted into ΔTn4662a-ΔTn6811 through non-replicative transposition and produced bilateral DRs (TTAG); (D) In1886 was inserted upstream of the res_site_I in ΔTn4662a through replicative transposition and produced bilateral DRs (GATAGG).
IMP-1 was first discovered in a P. aeruginosa strain from Japan (Walsh et al., 2005) and is now the most widely circulating IMP enzyme in Southeast Asia (Walsh et al., 2005). Up until November 2021, 86 IMP family members were indexed in GenBank, and most blaIMP−1 were carried and transferred by type 1 integrons (Diene and Rolain, 2014). After the Tn402 integron captures blaIMP−1, Tn402 possesses the self-transfer capability and can be integrated into transposons or res sites on plasmids to expand its transmission range (Gillings, 2017). In 2014, pNXM63 (MW150990) from Morganella morganii nx_m63 that was isolated in a hospital in China contained a Tn402-like type 1 integron (intI1-blaIMP−1; Xiang et al., 2021). Based on the repeat sequence (AACG) on both sides of the gene cassette, it can be deduced that In1886 is a structure formed after the integron on pNXM63 captured the aacA4' gene cassette (Figure 4). The Tn402-like transposon module at the 3' CS end of In1886 could capture other drug resistance genes. An example is In1886 in the PA15W plasmid in P. aeruginosa (GeneBank accession no.: MN961673). However, as this In1886 ISCfr1 fragment mediated the insertion of strA-strB-aac(3)-IId to the Tn402 recombination site on PA15W-In1886, it resulted in the truncation of the res_site_r1 site (Figure 4, Supplementary Table 4B), leading to irreversible recombination (Rowland et al., 2020). In1886 can also capture aadA6, blaOXA−21, aadA1a, qacF2, and guc162 gene cassettes through recombination at specific sites to obtain a series of derived structures (Labbate et al., 2009; Table 4). This integron and its derived structures were mostly present on Pseudomonas sp. chromosomes and were also discovered in K. pneumoniae and K. oxytoca plasmids (Table 4).
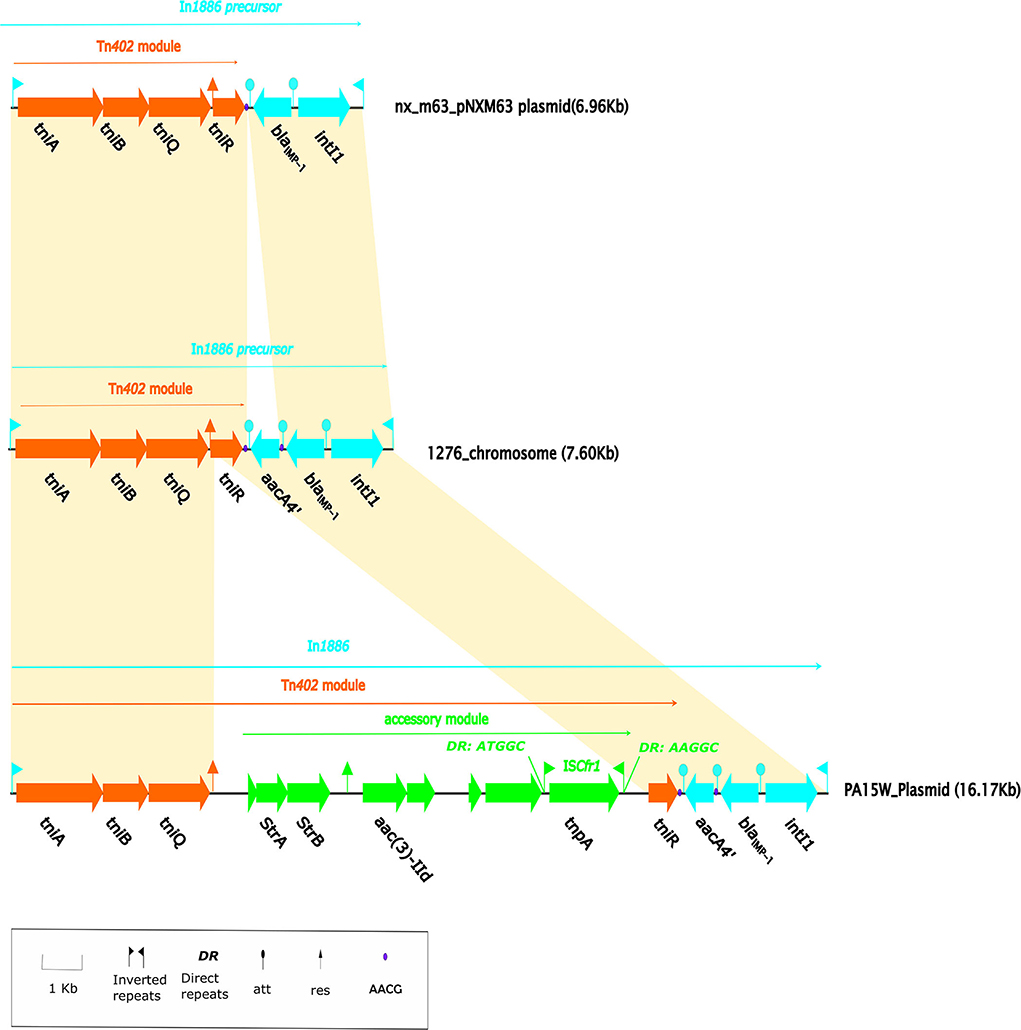
Figure 4. Linear alignment map of In1886. The Tn402 transposition module is orange-red, the 3'-CS of the integron is blue, and the exogenous insertion element is green. The solid purple dot is the AACG sequence that is recognized by the gene cassette att and forms two copies in In1886 due to the insertion of the aacA4' gene cassette. The shaded region represents a homologous region (>90% nucleotide identity). The pNXM63 (MW150990) from Morganella morganii nx_m63 contains an intI1-blaIMP−1-tniABQR structure. When this integron was compared with In1886, except for the gene cassette aacA4', all of the bases were completely identical, and there was no missing base. At the same time, the downstream region of the gene cassette blaIMP−1 in this integron contained the AACG site, which was present on both sides of the gene cassette aacA4' in In1886. In addition, In1886 was also present in the plasmid (GenBank accession no.: MN961673) in P. aeruginosa PA15W. However, a 7.45-kb exogenous insertion structure was inserted in the res_site_r1 recombination site of the Tn402 mobile module in the Tn402 of PA15W-In1886, causing res_site_r1 to be truncated (Supplementary Table 2). This exogenous insertion structure contained eight open reading frames (ORFs), including a complete ISCfr1 (IS1182 family), aminoglycoside resistance genes strA and strB, and aac(3)-IId.
Therefore, blaIMP−1 can be recombined upstream of res_site_I in Tn4662a using a Tn402-like integron as a medium to achieve transfer from other species to P. juntendi 18091276. After that, large fragments are transferred between Pseudomonas sp. through ICEs. The Tn4662a-derived structures in these ICEs contain the intact res_site_I sequence. It can also continue recombination with other elements. In addition, it was also found that, in addition to capturing gene cassettes, the res_site_r1 on tniABQR at 3' CS can also undergo further recombination, resulting in the production of multidrug resistance. Regarding different hosts, ICE 1276-like integration elements can also undergo recombination with exogenous genes through ssb to carry out adaptive evolution.
Conclusion
This study was the first to report a clinical isolate of P. juntendi in China. At the same time, it was found that this bacterium can capture Tn402-like type 1 integron containing blaIMP−1 through the ICE of Tn4662a. This provides a new vector and host for the horizontal transfer of blaIMP−1. Hence, there is a need to improve bacterial identification methods and drug resistance monitoring in opportunistic pathogens in hospitals. At the same time, the ICE 1276-Tn4662a-In1886 structure and its mutant-derived structures should be closely monitored, particularly its epidemiology in China.
Data availability statement
The datasets presented in this study can be found in online repositories. The names of the repository/repositories and accession number(s) can be found at: https://www.ncbi.nlm.nih.gov/genbank/, CP091311.
Author contributions
All strains were provided by China-Japan Union Hospital, Jilin University. PC, XG, JC, JL, and LZhu conceived, directed, and carried out the study. ML, GL, JJ, YW, XZ, and BJ prepared samples for sequence analysis. JG, SS, and LZhe acquired samples and analyzed the data. All authors have read and approved the final manuscript.
Funding
Funding for study design, data collection, data generation, and publication costs was provided by the National Science and Natural Science Foundation of China (Grant agreement 31872486).
Acknowledgments
We are grateful to the members of the China-Japan Union Hospital, Jilin University.
Conflict of interest
The authors declare that the research was conducted in the absence of any commercial or financial relationships that could be construed as a potential conflict of interest.
Publisher's note
All claims expressed in this article are solely those of the authors and do not necessarily represent those of their affiliated organizations, or those of the publisher, the editors and the reviewers. Any product that may be evaluated in this article, or claim that may be made by its manufacturer, is not guaranteed or endorsed by the publisher.
Supplementary material
The Supplementary Material for this article can be found online at: https://www.frontiersin.org/articles/10.3389/fmicb.2022.929800/full#supplementary-material
References
Alcock, B. P., Raphenya, A. R., Lau, T. T. Y., Tsang, K. K., Bouchard, M., Edalatmand, A., et al. (2020). CARD: antibiotic resistance surveillance with the Comprehensive Antibiotic Resistance Database. Nucl. Acids Res. 48, D517–D525. doi: 10.1093/nar/gkz935
Boratyn, G. M., Camacho, C., Cooper, P. S., Coulouris, G., Fong, A., Ma, N., et al. (2013). BLAST: a more efficient report with usability improvements. Nucl. Acids Res. 41, W29–33. doi: 10.1093/nar/gkt282
Bortolaia, V., Kaas, R. F., Ruppe, E., Roberts, M. C., Schwarz, S., Cattoir, V., et al. (2020). ResFinder 4.0 for predictions of phenotypes from genotypes. J. Antimicrobial Chemother. 75, 3491–3500. doi: 10.1093/jac/dkaa345
Brettin, T., Davis, J. J., Disz, T., Edwards, R. A., Gerdes, S., Olsen, G. J., et al. (2015). RASTtk: a modular and extensible implementation of the RAST algorithm for building custom annotation pipelines and annotating batches of genomes. Sci. Rep. 5:8365. doi: 10.1038/srep08365
Brovedan, M. A., Marchiaro, P. M., Diaz, M. S., Faccone, D., Corso, A., Pasteran, F., et al. (2021). Pseudomonas putida group species as reservoirs of mobilizable Tn402-like class 1 integrons carrying blaVIM-2 metallo-β-lactamase genes. Infect Genet Evol. 96:105131. doi: 10.1016/j.meegid.2021.105131
CLSI (2020). Performance Standards for Antimicrobial Susceptibility Testing. Wayne, PA: Clinical and Laboratory Standards Institute.
Diene, S. M., and Rolain, J. M. (2014). Carbapenemase genes and genetic platforms in Gram-negative bacilli: Enterobacteriaceae, Pseudomonas and Acinetobacter species. Clin. Microbiol. Infect. 20, 831–838. doi: 10.1111/1469-0691.12655
Edwards, U., Rogall, T., Blöcker, H., Emde, M., and Böttger, E. C. (1989). Isolation and direct complete nucleotide determination of entire genes. Characterization of a gene coding for 16S ribosomal RNA. Nucl. Acids Res. 17, 7843–53. doi: 10.1093/nar/17.19.7843
Gillings, M. R. (2017). Class 1 integrons as invasive species. Curr. Opin. Microbiol. 38, 10–15. doi: 10.1016/j.mib.2017.03.002
Grindley, N. D., and Reed, R. R. (1985). Transpositional recombination in prokaryotes. Annu. Rev. Biochem. 54, 863–896. doi: 10.1146/annurev.bi.54.070185.004243
Johnson, C. M., and Grossman, A. D. (2015). Integrative and Conjugative Elements (ICEs): what they do and how they work. Annu. Rev. Genet. 49, 577–601. doi: 10.1146/annurev-genet-112414-055018
Kaas, R. S., Leekitcharoenphon, P., Aarestrup, F. M., and Lund, O. (2014). Solving the problem of comparing whole bacterial genomes across different sequencing platforms. PLoS ONE 9:e104984. doi: 10.1371/journal.pone.0104984
Labbate, M., Case, R. J., and Stokes, H. W. (2009). The integron/gene cassette system: an active player in bacterial adaptation. Methods Mol. Biol. 532, 103–125. doi: 10.1007/978-1-60327-853-9_6
Liu, M., Li, X., Xie, Y., Bi, D., Sun, J., Li, J., et al. (2019). ICEberg 2.0: an updated database of bacterial integrative and conjugative elements. Nucl. Acids Res. 47, D660–D665. doi: 10.1093/nar/gky1123
Mizuno, S., Ogawa, M., Yano, H., Kasahara, K., and Mikasa, K. (2020). Molecular and epidemiological characteristics of carbapenemase-producing Klebsiella pneumoniae clinical isolates in Japan. mSphere 5, e00490–e00420. doi: 10.1128/mSphere.00490-20
Morimoto, Y., Tohya, M., Aibibula, Z., Baba, T., Daida, H., Kirikae, T., et al. (2020). Re-identification of strains deposited as Pseudomonas aeruginosa, Pseudomonas fluorescens and Pseudomonas putida in GenBank based on whole genome sequences. Int. J. Syst. Evol. Microbiol. 70, 5958–5963. doi: 10.1099/ijsem.0.004468
Nandi, T., Ong, C., Singh, A. P., Boddey, J., Atkins, T., Sarkar-Tyson, M., et al. (2010). A genomic survey of positive selection in Burkholderia pseudomallei provides insights into the evolution of accidental virulence. PLoS Pathog. 6:e1000845. doi: 10.1371/journal.ppat.1000845
Peix, A., Ramírez-Bahena, M. H., and Velázquez, E. (2018). The current status on the taxonomy of Pseudomonas revisited: an update. Infect. Genet. Evol. 57, 106–116. doi: 10.1016/j.meegid.2017.10.026
Rowland, S. J., Boocock, M. R., Burke, M. E., Rice, P. A., and Stark, W. M. (2020). The protein-protein interactions required for assembly of the Tn3 resolution synapse. Mol. Microbiol. 114, 952–965. doi: 10.1111/mmi.14579
Tohya, M., Watanabe, S., Teramoto, K., Shimojima, M., Tada, T., Kuwahara-Arai, K., et al. (2019). Pseudomonas juntendi sp. nov., isolated from patients in Japan and Myanmar. Int. J. Syst. Evol. Microbiol. 69, 3377–3384. doi: 10.1099/ijsem.0.003623
Varani, A. M., Siguier, P., Gourbeyre, E., Charneau, V., and Chandler, M. (2011). ISsaga: an ensemble of web-based methods for high throughput identification and semi-automatic annotation of insertion sequences in prokaryotic genomes. Genome Biol. 12:R30. doi: 10.1186/gb-2011-12-3-r30
Walsh, T. R., Toleman, M. A., Poirel, L., and Nordmann, P. (2005). Metallo-beta-lactamases: the quiet before the storm? Clin. Microbiol Rev. 18, 306–325. doi: 10.1128/CMR.18.2.306-325.2005
Xiang, G., Lan, K., Cai, Y., Liao, K., Zhao, M., Tao, J., et al. (2021). Clinical molecular and genomic epidemiology of Morganella morganii in China. Front. Microbiol. 12:744291. doi: 10.3389/fmicb.2021.744291
Yano, H., Miyakoshi, M., Ohshima, K., Tabata, M., Nagata, Y., Hattori, M., et al. (2010). Complete nucleotide sequence of TOL plasmid pDK1 provides evidence for evolutionary history of IncP-7 catabolic plasmids. J. Bacteriol. 192, 4337–4347. doi: 10.1128/JB.00359-10
Yoon, S. H., Ha, S. M., Lim, J. M., Kwon, S. J., and Chun, J. (2017). A large-scale evaluation of algorithms to calculate average nucleotide identity. Antonie van Leeuwenhoek 110, 1281–1286. doi: 10.1007/s10482-017-0844-4
Zhu, Z., Yang, H., Yin, Z., Jing, Y., Zhao, Y., Fu, H., et al. (2021). Diversification and prevalence of the quinolone resistance crpP genes and the crpP-carrying Tn6786-related integrative and conjuative elements in Pseudomonas aeruginosa. Virulence 12, 2162–2170. doi: 10.1080/21505594.2021.1962160
Keywords: Pseudomonas juntendi, integrative and conjugative, carbapenem-resistant, reorganization, blaIMP−1
Citation: Zheng L, Zhang X, Zhu L, Lu G, Guan J, Liu M, Jing J, Sun S, Wang Y, Sun Y, Ji X, Jiang B, Chen J, Liu J, Chen P and Guo X (2022) A clinical Pseudomonas juntendi strain with blaIMP−1 carried by an integrative and conjugative element in China. Front. Microbiol. 13:929800. doi: 10.3389/fmicb.2022.929800
Received: 27 April 2022; Accepted: 28 June 2022;
Published: 29 July 2022.
Edited by:
Ziad Daoud, Central Michigan University, United StatesReviewed by:
Po-Xing Zheng, Academia Sinica, TaiwanJeanette Teo, National University Hospital, Singapore
Copyright © 2022 Zheng, Zhang, Zhu, Lu, Guan, Liu, Jing, Sun, Wang, Sun, Ji, Jiang, Chen, Liu, Chen and Guo. This is an open-access article distributed under the terms of the Creative Commons Attribution License (CC BY). The use, distribution or reproduction in other forums is permitted, provided the original author(s) and the copyright owner(s) are credited and that the original publication in this journal is cited, in accordance with accepted academic practice. No use, distribution or reproduction is permitted which does not comply with these terms.
*Correspondence: Ping Chen, MTI0MzM3NzUyMyYjeDAwMDQwO3FxLmNvbQ==; Xuejun Guo, eHVlanVuZzIwMjEmI3gwMDA0MDsxNjMuY29t