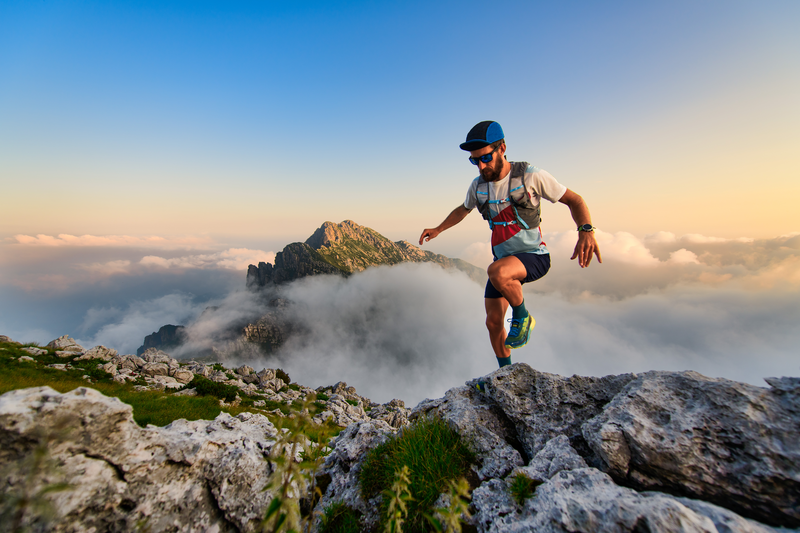
94% of researchers rate our articles as excellent or good
Learn more about the work of our research integrity team to safeguard the quality of each article we publish.
Find out more
ORIGINAL RESEARCH article
Front. Microbiol. , 07 July 2022
Sec. Microbiotechnology
Volume 13 - 2022 | https://doi.org/10.3389/fmicb.2022.926065
Silage Cassia alata (CA) can alleviate feed shortage in some areas to a certain extent and reduce feed costs. The present research evaluated the effect of cellulase (CE) and Lactiplantibacillus plantarum (LP) on the fermentation parameters, nutrients, and bacterial community of CA silage. Chopped CA was ensiled with three different treatments, namely, no inoculant (CK), CE, and LP, and the indexes were determined on the 2nd, 6th, 14th, and 30th days of silage fermentation. The fermentation parameters indicate that the pH value of the three groups decreased and then increased with the ensilage process, and the lowest value was observed on the 14th day. The CK and LP groups attained the highest value on the 30th day, while the CE group attained the highest value on the 2nd day. Additionally, the pH value and NH3-N content were significantly lower (P < 0.05) in the CE and LP groups than in the CK group. In terms of nutrients, crude protein (CP) contents significantly increased (P < 0.05) in the CE and LP groups on the 30th day. The neutral detergent fiber (NDF) and acid detergent fiber (ADF) contents of the CE group were significantly and negatively associated with fermentation time, and the water-soluble carbohydrate (WSC) contents of the three groups were significantly lower during ensiling. In comparison with the CK group, the NDF and ADF contents were significantly reduced (P < 0.05), and the WSC content increased (P < 0.05) in the CE group on day 30. Sequencing analysis of bacterial communities showed that Lactobacillus became the most dominant genus in the ensilage process. Moreover, both CE and LP groups increased the abundance of Lactobacillus and decreased that of Klebsiella, Weissella, and Acetobacter in comparison to the CK group, in which LP had a better effect. CE and LP could further improve the silage quality of CA, and LP had a more significant effect in reconstructing the bacterial community in the silage environment.
With the rapid development of animal husbandry, the shortage of high-quality silage is becoming increasingly serious. Thus, the development of new high-quality silage is essential. Cassia alata (CA), a major species of the Leguminosae family that originated from tropical South America, is cultivated in Yunnan and Guangdong provinces in China with a large plantation area and high yield. The fast growth, tolerance to barrenness, strong adaptability, and high protein content (Lim, 2014) of CA indicate its value in the development of feed. Although CA is mainly used for ornamental and medicinal purposes, it has also been used in feed. CA might have difficulty achieving satisfactory silage quality as a Leguminosae plant mainly because of the low content of water-soluble carbohydrate (WSC) and insufficient counts of epiphytic lactic acid bacteria (LAB). Therefore, the adverse effects should be addressed to improve the nutritional value and to obtain high-quality silage.
Considering the shortage of the low content of WSC in fresh CA, cellulase (CE) can be added. CE is widely recognized as the most commonly used enzyme for its applications in forage preservation (Hu et al., 2021). CE is a cell-wall degrading enzyme that can increase the rate of cell-wall degradation in plants and decrease the neutral detergent fiber (NDF) and acid detergent fiber (ADF) contents of silage by cleaving β-(1,4)-linkages in cellulose to release polysaccharides and then release glucose (Nadeau et al., 2000). Considering that glucose is the substrate for LAB fermentation, CE can indirectly promote LAB fermentation (Mu et al., 2020). Besides, the application of CE is beneficial for ensiling low-WSC forages. CE promotes fiber hydrolysis and releases glucose, which enables the multiplication of LAB and the production of lactic acid (LA), remarkably improving fermentation parameters and increasing the abundance of LAB in mixed silages of soybean residue and corn stover (Zhao et al., 2021). In addition, CE addition increases the content of true protein and decreases the fiber and NH3-N contents of mulberry leaf silage (He et al., 2019).
In addition to improving the content of WSC in fresh CA to obtain high-quality silage, the counts of epiphytic LAB can also be increased. The homofermentative LAB, such as Lactiplantibacillus plantarum (LP), is the most used fermentation prompter for silage production. LP can rapidly and effectively metabolize the WSC into LA, thus reducing the pH to 4 (Yitbarek and Tamir, 2014; Mu et al., 2020) and promoting silage fermentation. Low pH value and anaerobic conditions can inhibit the activity of undesirable microbiota and the inherent hydrolysis of plant protein, thereby reducing the production of NH3-N (Chen et al., 2020a). In the silage production, some spoilage bacteria reduce the quality of alfalfa silage, making the effect of silage less than expected. LP can rapidly eliminate pathogens under adverse conditions and improve the quality of silage (Ogunade et al., 2016). Adding LP reduces the pH value and the contents of acetic acid (AA), propionic acid (PA), and NH3-N in king grass silage. LP changes the bacterial community and increases the abundance of the desirable members of the Lactobacillus genus (Zi et al., 2021). In addition, the bacterial species of LP are considered by European Food Safety Authority to be suitable for the qualified presumption of safety approach (Additives EPo, 2021).
Harvesting CA for silage can alleviate feed shortage in some areas to a certain extent and reduce feed costs. Therefore, extending the use of CA in feed is essential for the diversification of the CA industry and meeting the rapid development of high-quality silage for ruminants. However, the microbial composition of CA silage and the reaction mechanism of additives remain unclear. The effects of CE and LP on the diversity of CA silage quality and microbiota need to be studied. However, CE and LP have beneficial effects on the fermentation and microbes of CA silage. This hypothesis was tested by assessing the effects of CE and LP on the fermentation parameters and microbial diversity of CA silage in the current research.
The whole plant CA in the blooming stage with a height of 1.2 m (with a stubble height of 30 cm) was harvested and chopped into 10–20 mm lengths by using a grass shredding machine on September 27, 2020 from the ecological breeding farm of the Guangshunhai Food Limited Company (116°133′N; 24°124′E, Meinan Town, Meixian District, Meizhou City, Guangdong Province, China). The raw material was used to determine the chemical composition. The chopped CA was divided into three groups and ensiled as follows: (1) CK group (control group without additives; sprayed with 1 g of distilled water), (2) CE group (Guangdong VTR Bio-Tech Co., Ltd., China mainland; mainly including C1-CE, CX-CE, and β-(1,4)-glucosidase; CE activity as 5 × 105 U/g; addition amount: 0.1 g/kg of fresh material (FM) dissolved in 1 g of distilled water), (3) LP group (LP application rate: 1 × 106 cfu/g FM suspended in 1 g of distilled water; No. 23490, a native strain isolated and selected from stylo silage based on its acid productivity as described by Wang et al., 2019a). After mixing CA with CE and LP thoroughly, approximately 200 g of silage material was placed into fermentation bags (20 × 30 cm) with a one-way breather valve, vacuum-sealed immediately, and then stored at room temperature (25 ± 3°C). Three replicate bags were prepared for each treatment, and four samples were uniformly obtained from each bag on days 2, 6, 14, and 30 of ensiling. The first sample was used to test the parameters of silage fermentation, the second one was dried (65°C for 48 h) and passed through a 1-mm sieve in a grinder for chemical composition analysis, the third one was used for microbial counts, and the fourth one was used for the microbial diversity analysis.
As described by Wang et al. (2019a), 5 g of sample of the silage was mixed with 45 mL of distilled water, stored at −4°C for 24 h, and filtered to obtain the extract to determine their fermentation characteristics. The pH was directly measured using a pH meter (Sartorius PB-10). Ammonia-N (NH3-N) content was determined using phenol-sodium hypochlorite colorimetry (Ke et al., 2017). LA was detected using the p-hydroxybiphenyl colorimetric method (Barker and Summerson, 1941). Volatile fatty acids, such as acetic acid (AA), propionic acid (PA), and butyric acid (BA), were analyzed using high-performance gas chromatography (Agilent 7890B). The vaporization chamber parameters are as follows: carrier gas, N2; split ratio, 40:1; injection volume, 0.4 μL; and temperature, 220°C. The column parameters are as follows: HP-INNOWax capillary column constant flow mode; flow, 2.0 ml/min; and average linear velocity, 38 cm/s. The column thermostat parameters are as follows: programmed heating 120 °C (3 min)-10 °C/min−180 °C (1 min). The detector parameters are as follows: H2 flow rate, 40 ml/min; airflow rate, 450 ml/min; column flow rate makeup air flow rate, 45 ml/min; FID temperature, 250 (Shao et al., 2005).
The removed samples were oven-dried at 65°C for 48 h to calculate the dry matter (DM), then crushed and passed through a 1-mm sieve for nutrient analysis. The results are expressed as DM. According to the methods of the Association of Official Analytical Chemists (Association of Official Analytical Chemists, 1990), crude protein (CP) was determined using a Kjeldahl nitrogen analyzer (Kjeltec 2300 Auto Analyzer, FOSS Analytical AB). Van Soest et al. (1991) measured NDF and ADF using an automatic fiber analyzer (ANKOM 2000i). The content of WSC was determined using the anthrone-sulfuric acid colorimetric method (Owens et al., 1999).
The trial procedures described in this section were similar to those previously reported (Wang et al., 2020a). Briefly, the silage was unpacked and sampled under aseptic conditions and subjected to microbiological analysis by plate counting. One portion of 5 g silage samples was homogenized with sterilized saline (45 mL) in an orbital shaker for 30 min, and the supernatants were serially diluted from 10−1 to 10−6. LAB and coliform bacteria (CB) were separately cultivated on Man Rogosa Sharpe agar and Violet Red Bile agar at 30°C for 48 h in an aerobic incubator. Yeasts and molds were cultured in Rose Bengal agar following incubation at 28°C for 48 h.
The bacterial community of silage was analyzed using high-throughput sequencing at the Guangzhou Genedenovo Biotechnology Co., Ltd. Microbial DNA was extracted from silage samples using the HiPure Soil DNA kit (Magen, Guangzhou, China) in accordance with the kit's instructions. The final DNA purification was determined using the NanoDrop 2000 microspectrophotometer (Thermo Fisher Scientific, USA), and the DNA integrity was assessed by 2% Biowest Agarose gel electrophoresis. Thereafter, the 16S rDNA V3-V4 regions of the ribosomal RNA gene were amplified using PCR using the primer pairs of 341F (5′-CCTACGGGNGGCWGCAG-3′) and 806R (5′-GGACTACHVGGGTATCTAAT-3′). After amplification, amplicons were purified using the AxyPrep DNA gel extraction kit (Axygen Biosciences, Union City, CA, USA) according to the manufacturer's instructions, and quantified using the ABI StepOnePlus real-time PCR system (Life Technologies, Foster City, USA). Then, sequencing was performed on the PE250 mode pooling of Novaseq 6000.
High-quality clean reads were obtained by filtering raw reads according to the rules using FASTP (version 0.18.0). Then, paired-end clean reads were merged as original tags using FLSAH (version 1.2.11) with a minimum overlap of 10 bp and a mismatch error rate of 2%. The noise sequences of the original tags were filtered under specific filtering conditions to obtain high-quality clean tags. The clean tags were clustered into operational taxonomic units (OTUs) of ≥97% similarity using the UPARSE (version 9.2.64) pipeline. All chimeric tags were removed using the UCHIME algorithm, and effective tags were obtained for further analysis. The tag sequence with the highest abundance was selected as the representative sequence within each cluster. The representative OTU sequences were classified into organisms by using a naive Bayesian model with the RDP classifier (version 2.2) based on the SILVA database (version 132) at a confidence threshold value of 0.8 to obtain taxonomic classification at the phylum, class, order, family, and genus levels. Alpha diversity was analyzed using QIIME (version 1.9.1). Beta diversity was analyzed in the R project Vegan package (version 2.5.3). The functions of microflora were predicted based on the Tax4Fun (version 1.0) database.
Fermentation parameters, chemical characteristics, microbial population, and alpha diversity index data were analyzed using a two-way analysis of variance (ANOVA) in the IBM SPSS 25.0. The model for data processing is as follows:
where Yij is the variable of interest, μ is the overall mean, αi is the fixed effect of ensiling days, βj is the fixed effect of different additions, αβij is the interaction of ensiling days and additions, and εij is the residual error. Duncan's multiple comparisons were used to examine the significant differences between various treatments (P < 0.05).
The chemical compositions of fresh CA before ensiling are listed in Table 1. The contents of DM, CP, NDF, ADF, and WSC were 22.82, 18.89, 64.37, 38.94, and 3.35%, respectively. Fresh CA has high NDF and ADF content and low WSC.
Table 2 shows the dynamics of fermentation parameters in CA silage. At each measurement time, the pH values in CE and LP groups were significantly lower (P < 0.05) than those in the CK group. With the increase of ensilage time, the pH values of each treatment group decreased and then increased, reaching the lowest on day 14. Among the samples, only the pH value in the CE group was below 4.2 on days 14 and 30. Besides, when the silage was fermented for 30 days, the pH values of the CE group were significantly lower (P < 0.05) than those of the CK and LP groups. The NH3-N content of the CK, CE, and LP groups increased significantly (P < 0.05) throughout the ensilage period. The NH3-N contents of the CE and LP groups were significantly lower (P < 0.05) than those of the CK group. The NH3-N content of the LP group was significantly lower than those of the two other groups (P < 0.05) after 30 days of fermentation, while the contents of NH3-N in the CK group were the highest. The LA content of each group increased (P < 0.05) during fermentation, and the LP group had the highest LA content on day 30. The AA content of each group increased significantly with the increase of silage days (P < 0.05) and reached the highest on day 30, where the CE group had the highest content and the LP group had the lowest content. On day 2 of ensilage, PA was not detected in the silage of each treatment group. After day 2, the contents of PA in each group increased significantly with fermentation (P < 0.05), but no significant differences (P < 0.05) were observed among the groups. The BA content of the CK group increased significantly (P < 0.05) with the increase of ensilage days. The BA content of the CK group was significantly (P < 0.05) higher than that of the CE and LP groups on day 30.
The nutrient analysis results are displayed in Table 3. The difference in DM contents among the three groups was insignificant (P > 0.05). The contents of CP in the CE and LP groups were significantly higher than those of the CK group on day 30 (P < 0.05). The contents of NDF and ADF in the CE group decreased significantly (P < 0.05) with the increase in fermentation time. In addition, the contents of NDF and ADF in the CE group significantly (P < 0.05) decreased compared with the CK group on day 30. In comparison with fresh CA, the contents of NDF and ADF in the CE group decreased to 34.27 and 28.09%, respectively, on day 30. The contents of WSC significantly (P < 0.05) decreased in the three groups during ensilage and were significantly (P < 0.05) higher in the CE group than in the CK and LP groups on day 30.
The dynamics of LAB, CB, yeasts, and molds are shown in Table 4. The LAB counts significantly increased (P < 0.05) followed by a significant decrease (P < 0.05) with prolonged ensiling time, and the peak value was observed on day 14. The LAB counts of the three silage groups were 8.06 (CK), 8.27 (CE), and 8.30 (LP) log10 CFU/g FM on day 14. The LAB counts of the CE and LP groups increased significantly (P < 0.05) compared with the CK group on day 14. The CB counts were highest on day 2 with measurements of 6.54, 5.48, and 5.04. Similarly, the count of yeasts was highest with measurements of 5.60, 5.31, and 5.08 on day 2. The counts of CB and yeasts were undetectable on day 30. Throughout the fermentation process, molds were not detected.
The alpha diversity of dynamic CA silage bacterial communities is shown in Table 5. The good coverage values for all treatments were above 99%, indicating that the sampling data were sufficiently representative of the entire flora of the different samples. The Sob, Chao, and Ace indexes of CE and LP groups decreased significantly (P < 0.05) throughout the ensilage period. The result of PCoA is shown in Figure 1. Principal coordinates 1 (PCo1) and 2 (PCo2) explain 20.67 and 10.79% of the total variance in CA silage, respectively. The 2- and 30-day CA silages were clustered in the third and second quadrants, whereas both the 6- and 14-day silages were in the first and fourth quadrants, respectively. All the three groups were significantly separated during different silage times, and then compared with themselves. In addition, the CE group formed a greater degree of separation from the CK group compared with the LP group on day 30.
Figure 1. Principal coordinates analysis (PCoA) of bacterial communities for cassia alata silage (CK, control; CE, cellulase; LP, Lactiplantibacillus plantarum, after 2, 6, 14, and 30 days of ensiling, respectively).
The relative abundances of bacterial communities of CA silage at the phylum and genus levels are presented in Figure 2 (Circos map) and Figure 3 (accumulation map). Supplementary Tables S1, S2 show the bacterial communities at the top 10 phylum and genus levels of ensiled CA. At the phylum level, Proteobacteria was the highest in CK (70.80%), CE (67.58%), and LP (60.19%) groups, and it was the most dominant phylum in CA silage on day 2. However, gradually, Firmicutes increased and Proteobacteria decreased significantly (P < 0.05) as the fermentation proceeded. Firmicutes was the most dominant phylum in the CA silage, accounting for 76.07% of CK, 78.76% of CE, and 86.13% of LP on day 30. The relative abundance of Firmicutes in the LP group was significantly higher (P < 0.05) than in the CK group. After refining the genus level, 31 colonies with relative abundance above 0.1% were detected in the silage. In the early stages of silage, the dominant genus was Klebsiella, while the subdominant genera were Lactobacillus, Weissella, and Acetobacter. Gradually, Lactobacillus replaced Klebsiella on the 6th, 14th, and 30th day. On day 30, Lactobacillus accounted for 66.83% (CK), 72.86% (CE), and 80.94% (LP), respectively. In comparison with the CK group, the relative abundance of Lactobacillus in the CE and LP groups were significantly higher (P < 0.05), while the relative abundance of Klebsiella and Weissella in the CE and LP groups significantly decreased (P < 0.05).
Figure 2. Circos map of bacterial communities at the phylum (A) and genus (B) levels for cassia alata silage (CK, control; CE, cellulase; LP, Lactiplantibacillus plantarum, after 2, 6, 14, and 30 days of ensiling, respectively).
Figure 3. Accumulation map of bacterial communities at the phylum (A) and genus (B) levels for cassia alata silage (CK, control; CE, cellulase; LP, Lactiplantibacillus plantarum, after 2, 6, 14, and 30 days of ensiling, respectively).
The functional and pathway profiles of bacterial colony prediction are shown in Figure 4. The first five predictive functions were carbohydrates metabolism, membrane transport, amino acid metabolism, nucleotide metabolism, and translation. The top five predicted pathways were ABC transporters, a two-component system, purine metabolism, aminoacyl-tRNA biosynthesis, and pyrimidine metabolism. The heatmap of the top 20 predicted functions and pathways implies that the addition of CE and LP might have affected the major functions and pathways.
Figure 4. Heatmap of the top 20 predicted functions (A) and pathways (B) of the bacterial communities analyzed via Tax4Fun for cassia alata silage (CK, control; CE, cellulase; LP, Lactiplantibacillus plantarum, after 2, 6, 14, and 30 days of ensiling, respectively).
The moisture content of raw material can affect the growth and reproduction rate of microorganisms during fermentation, and it is an important factor that affects the parameters of silage fermentation (Hu et al., 2009). The DM content in fresh CA was lower than the DM content of 35% required for good silage (Guyader et al., 2018). Therefore, it was necessary to add additives to avoided the problem of massive growth and reproduction of spoilage microbiota, such as Clostridium, that might exist in high-moisture silage and facilitated the preparation of high-quality silage for CA (Yitbarek and Tamir, 2014). However, increased NDF and ADF contents might contribute to the ruminant production performance. Silage can effectively reduce NDF and ADF contents and improve the palatability and nutritional value of CA. WSC is an essential substrate for LAB fermentation in the ensilage process. The WSC content of fresh CA was below the ideal content of 6% (Zhang et al., 2019), which was challenging for producing high-quality silage for CA. Therefore, silage additives and CE should be added to promote NDF and ADF hydrolysis to increase the WSC content, and LP should be added to promote LAB colonization, which effectively promotes the fermentation of CA. Collectively, CA was a high-protein forage. However, its disadvantages include high NDF and ADF contents and low WSC content, which hinder the ensilage process without silage additives. Therefore, additions were recommended to improve the quality of CA silage.
The pH value is an important indicator for evaluating the fermentation parameters of silage. During ensilage, the pH values of CE and LP groups were more significant than those in the CK group. Similar to the result of Mu et al. (2020), both CE and LP can decrease the pH value in mixed silage of high-moisture amaranth and rice straw. The pH values of each treatment group initially decreased and then increased during the fermentation. This phenomenon could be caused by the continuous growth and multiplication of Clostridium butyricum and the production of butyric acid in low pH and humid conditions, with partial fermentation of lactic acid to butyric acid (He et al., 2020). The lowest pH was observed on day 14, and the pH value of the CE group was below 4.2. Generally, silage also can be considered fermented at a pH below 4.2 (McDonald, 1981). The pH values of the CE group were significantly lower than those of CK and LP groups on day 30. Therefore, CE has a better ability to reduce the pH value of CA silage than LP.
During ensilage, proteins are eventually degraded into amines, amides, and ammonia by the action of plant proteases and microbiota (Kung et al., 2018). Therefore, NH3-N can reflect the nutritional value of silage, which is a common indicator of protein hydrolysis (McDonald, 1981). In this trial, the NH3-N contents of the CK, CE, and LP groups increased significantly with fermentation. The NH3-N contents of the CE and LP groups were significantly lower than those of the CK group. The results of Kung and Shaver (2001) indicated that the action of Clostridium and plant protein hydrolases might be the typical cause of NH3-N accumulation. Therefore, this phenomenon might have resulted from the effective inhibition of the action of Clostridium and plant protein hydrolases by CE and LP. Similar effects on other silage fermentation were achieved by applying CE or LP. The results of Li et al. (2019) show that the addition of CE significantly reduced NH3-N in cassava foliage silage. Cheng et al. (2021) found an obvious reduction in NH3-N content of paper mulberry treated with B17 (Lactiplantibacillus plantarum). Notably, the NH3-N content of the LP group was significantly lower than that of the two other groups on the 30th day. Apparently, LP was more conducive to inhibiting proteolysis through the influence of enzymes and microorganisms.
LAB are commonly homofermentative and produce more LA. However, some LAB shift to heterofermentative in forages lacking sugar (Li et al., 2018), thus producing LA and AA (Abdelazez et al., 2022). The LA content of each group increased, and the LP group was the highest on day 30. The AA content of each group remarkably increased during ensilage, of which the CE group had the highest content on day 30. AA can improve the aerobic stability of silage and effectively inhibit fungal reproduction (Schmidt and Kung, 2010). Thus, both LP and CE could promote LAB fermentation. Propionic acid (PA) and butyric acid (BA) reflect the nutritional loss of silage, and both compounds are not conducive to improving the quality of silage, which is undesirable in silage. After day 2, the contents of PA in each group increased significantly with fermentation. The BA content of the CK group remarkably increased during ensilage, indicating that CA ensilage possibly encountered harmful bacteria that multiplied and produced PA and BA. The BA content of the CK group was significantly higher than that of CE and LP group on day 30. Similarly, the results of Guo et al. (2014) showed that CE and LP lowered PA and BA contents compared with the control. Besides, the decrease in BA concentration supported that clostridial fermentation is inhibited by a rapid drop in pH. Therefore, LP and CE can both promote LAB fermentation and inhibit the increase in the number of deterioration bacteria, which can effectively improve the fermentation parameters of silage.
High-quality fermentation is accompanied by good preservation of nutrients in the silage. The DM contents among the three groups were lower than the ideal content of 30–35% reported by Guyader et al. (2018). CP content is an indicator for assessing the nutritional value of silage. The contents of CP in CE and LP groups were significantly higher than those of the CK group on day 30. This finding was obtained because both CE and LP facilitated the protection of proteins from catabolism (Li et al., 2017; Mu et al., 2020). NDF and ADF are important indicators of silage quality (Wang et al., 2011). The high contents of NDF and ADF in low-quality silage are not conducive to animal digestion and reduce the digestibility of the feed. With the increase in fiber content, the contents of protein and energy in the silage decreased. Therefore, the lower the fiber content in the feed, the better it is for improving the value of the feed (Xiang-Lin et al., 2008). The contents of NDF and ADF in the CE group were significantly and negatively correlated with ensilage time, and they significantly decreased compared with the CK group on day 30. CE significantly reduces the fiber content, especially NDF and ADF (Guo et al., 2014). WSCs are both decomposition products of plant fibrous materials and fermentation substrates for LAB. The consumption of WSC by LAB decreases the pH, which helps in inhibiting the activity of undesirable microbiota, reducing the loss of nutrients during fermentation, and improving the fermentation parameters of silage. The contents of WSC were significantly higher in the CE group than in the CK and LP groups on day 30. Both the decrease in NDF and ADF contents and the increase in WSC content in the CE group can be attributed to the ability of CE as a cell-wall-degrading enzyme to increase the rate of plant cell-wall degradation and release of glucose (Nadeau et al., 2000). Therefore, CE might be conducive to promoting LAB colonization during ensilage, consistent with the results of Li et al. (2017).
Microbial communities affect silage quality by affecting the ecosystem in silage (Zhao et al., 2021). LAB plays a leading role in the ensilage process and can produce a large amount of organic acids, which is closely related to the quality of silage (Ni et al., 2015). The LAB counts increase and then decrease during ensilage, reaching the peak value on day 14. This finding was consistent with the change in pH value. It indicates that the effect of the CE and LP groups was better than that of CK group on day 14 (P < 0.05), implying that both CE and LP promote LAB growth. Lactobacilli count must reach 105 CFU/g FM for high-quality silage (Cai et al., 1998). The count of LAB reached 8 log10 CFU/g FM in the three groups of this trial, indicating that LAB underwent massive multiplication during ensilage, which was beneficial for CA silage quality. CB, which is the main competitor of LAB, hydrolyzed the protein component and decreased the amino acid content, resulting in a loss of nutrients in the silage (Rauramaa et al., 1987). Yeasts, as a type of aerobic microorganisms, deplete oxygen and consume DM as the main flora and convert WSC into ethanol during fermentation (Lv et al., 2020). CB and yeast populations exceeded the limit of 2.00 log10 CFU/g FM (Wang et al., 2019b) during fermentation but were undetectable on day 30. Throughout the fermentation process, molds were not detected. CB, yeasts, and molds were not detected, which was beyond our expectations. This finding can be attributed to the continuous multiplication of LAB and the production of LA with a low pH value of the silage.
Microbial community structure, diversity, and function are hot topics in microbial ecology, which have been studied in different media (Wang et al., 2019b; Lv et al., 2020; Zhao et al., 2021). The OTUs (Sob index), diversity (Simpson and Shannon indexes), and richness (Chao and Ace indexes) were studied to estimate the bacterial alpha diversity. The significant changes in alpha diversity among silage periods are caused by the dynamic microbial response to the ensilage process, in which the composition and function of the bacterial flora can obviously vary (Sepehri and Sarrafzadeh, 2019). In the present study, the Sob, Chao, and Ace indexes of CE and LP groups decreased during storage time, indicating that the bacterial diversity in silage decreased. Wang et al. (2020b) confirmed that the addition of CE decreased the Chao and Ace indexes of alfalfa silage flora. Chen et al. (2020b) showed that LP could lower the Ace index of alfalfa silage. Therefore, the addition of CE and LP can reduce the bacterial diversity. This finding was obtained because CE provided more carbon and nitrogen sources for LAB, which promoted the multiplication of LAB. The increase in available nutrients (C, N) promoted LP growth, thereby increasing its proportion in the silage microbial populations, explaining the large LAB numbers observed. LAB produced large amounts of LA and lowered the pH value of silage, thus inhibiting the proliferation of harmful bacteria and decreasing bacterial diversity.
The results of beta diversity reflect the differences in bacterial communities in each individual or treatment (Tian et al., 2021). For further insight into the changes in bacterial communities of CA silage, PCoA of beta diversity was employed. The result of unweighted PCoA could demonstrate the variance in the bacterial community structure of CA silage. The PCoA results show that all three groups were significantly separated during different silage times compared with themselves, indicating obvious changes in bacterial communities. In addition, the CE group formed a greater degree of separation from the CK group compared with the LP group on day 30. Considering that the differences in microbial communities may be responsible for differences in silage quality, CE and LP might help in promoting the growth of different microbial communities, thus enhancing the fermentation parameters, with the CE effect being more remarkable.
The composition of the microbiota is related to silage quality (Tian et al., 2021). Changes in the dominant phylum and genus of CA silage material throughout the ensilage process indicated large changes in the composition and abundance of bacterial communities during ensilage. Therefore, the changes in the fermentation parameters and nutrients of CA are caused by the changes in these specific bacterial communities. On the phylum level, Proteobacteria was the most dominant in the early stage of ensilage. Firmicutes gradually replaced Proteobacteria during ensilage. Mu et al. (2020) reported similar results. After 30 days of ensiling, Firmicutes and Proteobacteria were the dominant phylum in the mixed silage of high-moisture amaranth and rice straw. Liu et al. (2019) found that Firmicutes and Proteobacteria were the most dominant phylum, accounting for 99% of the total relative abundance in the late stages of barley silage. Considering that all LAB belong to Firmicutes (Tian et al., 2021), with the decrease in pH and increase in LAB during ensilage, Firmicutes gradually became the most dominant phylum as the silage time changed. By contrast, Proteobacteria gradually decreased. This condition can be explained by the fact that the permeability of the outer membrane of Gram-negative bacteria was affected, thereby inhibiting the growth of Proteobacteria (Helander and Mattila-Sandholm, 2000). In conclusion, the addition of both CE and LP contributed to the growth of Firmicutes and the inhibition of Proteobacteria.
To further study the effect of additives on the bacterial community during ensilage, we examined the bacterial structure of CA silage at the genus level. In the early stages of silage, the dominant genus was Klebsiella, and the subdominant genera were Lactobacillus, Weissella, and Acetobacter. Li et al. (2021) found that the genes involved in NH3 formation were mainly classified as encoding nitrite reductase (nirB) and distributed mainly in Klebsiella. Klebsiella is an acid-intolerant facultative anaerobe that can grow well in anoxic environmental conditions using fermentation substrates (Stewart, 1988). Klebsiella is a harmful microorganism in silage; it destabilizes the forage aerobically and causes infectious diseases, such as mastitis (Zadoks et al., 2011). LAB is found in forage crops and silage, many of which isolates had been identified as part of Lactobacillus group (You et al., 2021a). Cai et al. (1999) found that Lactobacillus is the main microorganism in forage and silage. Lactobacillus is the dominant genus in high-quality silage, because it is responsible for driving substrate fermentation during ensilage and plays a crucial role in accumulating LA and lowering pH value (Zi et al., 2021). Some Lactobacillus species, such as Lactiplantibacillus plantarum and Lentilactobacillus buchneri, are commonly used as silage inoculants, because they grow rapidly and inhibit the reproduction of harmful bacteria, which help improve the quality of silage fermentation (Ranjit and Kung, 2000). Weissella, an obligately heterofermentative anaerobic bacterium, converts WSC to LA and AA during ensilage fermentation. Weissella is another major microorganism in all silages throughout the fermentation process and is an early colonizer (You et al., 2021b). Acetobacter is an AA-producing and nitrogen-fixing obligate aerobic bacterium (Pahlow et al., 2015) that usually acts simultaneously with yeast to trigger a metamorphic process in silage (Spoelstra et al., 1988). In barley silages, Liu et al. (2019) found that Acetobacter is dominant in the bacterial community once aerobic spoilage occurs. Therefore, as ensilage time changed, Lactobacillus, Weissella, and Acetobacter continued to produce acid to lower the pH value of the silage, resulting in less Klebsiella members. Furthermore, after 30 days of ensilage, Lactobacillus remarkably influenced the fermentation of silage by rebuilding the microbiota and became the most dominant genus, thus decreasing the abundance of Klebsiella, Weissella, and Acetobacter. The significant shift in the bacterial community from Proteobacteria to Firmicutes during ensilage can be explained by the increase in the total abundance of Lactobacillus and Weissella, which flourished under the environmental condition that develops during ensilage. Mu et al. (2020) found that the addition of both CE and LP was beneficial to increasing the abundance of Lactobacillus in the mixed silage of high-moisture amaranth and rice straw. In this trial, CE and LP further reduced the relative abundance of Klebsiella compared with the untreated CK group, suggesting that CE and LP had antibacterial ability against undesirable bacteria. LP group had the highest abundance of Lactobacillus. This finding corresponds to the result that both the content of LA and the number of LAB were the highest in the LP group, as mentioned previously. Therefore, LP was more favorable for the rapid accumulation of LA and multiplication of LAB and inhibited the activity of most acid intolerant and aerobic fungi.
The functional and pathway prediction of silage microflora was analyzed by Tax4Fun. In comparison with the CK group, the application of both CE and LP increased the abundance of carbohydrate metabolism, membrane transport, amino acid metabolism, nucleotide metabolism, translation, ABC transporters, purine metabolism, aminoacyl-tRNA biosynthesis, and pyrimidine metabolism. The heat maps of predicted functions and pathways imply that the addition of CE and LP might have affected major functions and pathways. Furthermore, the analysis of PCoA and bacterial abundance confirmed that LP plays a pronounced role in the predicted functions and pathways. Therefore, LP greatly influenced the functions of the bacterial community. These results can be attributed to the large changes in some functional bacteria caused by silage additives. However, the exact mechanism remains unclear, thus requiring further studies.
The study revealed the potential of CA as a raw material for ensilage. Moreover, CE and LP contributed to the fermentation parameters and nutrients of CA silage. The pH value and NH3-N content decreased, and the content of CP increased in the silage treated with CE and LP. The NDF and ADF contents were evidently reduced, and WSC content increased upon the addition of CE. The addition of CE and LP reduced bacterial diversity, increased the abundance of Lactobacillus, and decreased the abundance of Klebsiella, Weissella, and Acetobacter. LP had a better effect than CE. In conclusion, CE and LP could further improve the quality of CA silage, and LP had a greater effect on the bacterial community than CE.
The datasets presented in this study can be found in online repositories. The names of the repository/repositories and accession number(s) can be found in the article/Supplementary Material.
YG: conceptualization and project administration. MW and HT: methodology. ZX: software, validation, formal analysis, and data curation. MD: investigation and resources. ZX and JW: writing—original draft preparation. BS and DL: writing—review and editing. YL and GL: supervision. All authors have read and agreed to the published version of the manuscript.
This research was supported by the National Nature Science Foundation of China (31872382), the Guangdong Provincial Promotion Project on Preservation and Utilization of Local Breed of Livestock and Poultry (4300-F18260), and the Modern Agricultural Industrial Technology System of Guangdong Province (2019KJ127).
The authors declare that the research was conducted in the absence of any commercial or financial relationships that could be construed as a potential conflict of interest.
All claims expressed in this article are solely those of the authors and do not necessarily represent those of their affiliated organizations, or those of the publisher, the editors and the reviewers. Any product that may be evaluated in this article, or claim that may be made by its manufacturer, is not guaranteed or endorsed by the publisher.
The Supplementary Material for this article can be found online at: https://www.frontiersin.org/articles/10.3389/fmicb.2022.926065/full#supplementary-material
Abdelazez, A., Abdelmotaal, H., Evivie, S. E., Bikheet, M., Sami, R., Mohamed, H., et al. (2022). Verification of Lactobacillus brevis tolerance to simulated gastric juice and the potential effects of postbiotic gamma-aminobutyric acid in streptozotocin-induced diabetic mice. Food Sci. Hum. Well. 11, 165–176. doi: 10.1016/j.fshw.2021.07.017
Additives EPo Products or Substances used in, Animal, F, Bampidis, V, Azimonti, G, Bastos, ML, and Christensen, H. (2021). Safety and efficacy of a feed additive consisting of Lactiplantibacillus plantarum (formerly Lactobacillus plantarum) IMI 507027 for all animal species (ALL-TECHNOLOGY (IRELAND) LIMITED [Alltech Ireland]). EFSA J. 19, e06704. doi: 10.2903/j.efsa.2021.6704
Association of Official Analytical Chemists (1990). Official Methods of Analysis (Gaithersburg, MD).
Barker, S. B., and Summerson, W. H. (1941). The colorimetric determination of lactic acid in biological material. J. Biol. Chem. 138, 535–554. doi: 10.1016/S0021-9258(18)51379-X
Cai, Y., Benno, Y., Ogawa, M., Ohmomo, S., Kumai, S., Nakase, T., et al. (1998). Influence of lactobacillus spp. from an inoculant and of weissella and leuconostoc spp. from forage crops on silage fermentation. Appl. Environ. Microbiol. 64, 2982–2987. doi: 10.1128/AEM.64.8.2982-2987.1998
Cai, Y., Suyanandana, P., Saman, P., and Benno, Y. (1999). Classification and characterization of lactic acid bacteria isolated from the intestines of common carp and freshwater prawns. J. Gen. Appl. Microbiol. 45, 177–184. doi: 10.2323/jgam.45.177
Chen, L., Qu, H., Bai, S., Yan, L., You, M., Gou, W., et al. (2020b). Effect of wet sea buckthorn pomace utilized as an additive on silage fermentation profile and bacterial community composition of alfalfa. Bioresour. Technol. 314, 123773. doi: 10.1016/j.biortech.2020.123773
Chen, L. Y., Bai, S. Q., You, M. H., Xiao, B. X., Li, P., Cai, Y. M., et al. (2020a). Effect of a low temperature tolerant lactic acid bacteria inoculant on the fermentation quality and bacterial community of oat round bale silage. Anim. Feed Sci. Technol. 269, 114669. doi: 10.1016/j.anifeedsci.2020.114669
Cheng, Q., Chen, Y., Bai, S., Chen, L., You, M., Zhang, K., et al. (2021). Study on the bacterial community structure and fermentation characteristics of fresh and ensiled paper mulberry. Anim. Sci. J. 92, e13656. doi: 10.1111/asj.13656
Guo, G., Yuan, X. J., Li, L. X., Wen, A. Y., and Shao, T. (2014). Effects of fibrolytic enzymes, molasses and lactic acid bacteria on fermentation quality of mixed silage of corn and hulless-barely straw in the Tibetan Plateau. Grassl. Sci. 60, 240–246. doi: 10.1111/grs.12060
Guyader, J., Baron, V. S., and Beauchemin, K. A. (2018). Corn forage yield and quality for silage in short growing season areas of the Canadian prairies. Agron. Basel 8, 164. doi: 10.3390/agronomy8090164
He, L., Wang, C., Xing, Y., Zhou, W., Pian, R., Chen, X., et al. (2020). Ensiling characteristics, proteolysis and bacterial community of high-moisture corn stalk and stylo silage prepared with Bauhinia variegate flower. Bioresour. Technol. 296, 122336. doi: 10.1016/j.biortech.2019.122336
He, L., Zhou, W., Wang, C., Yang, F., Chen, X., Zhang, Q., et al. (2019). Effect of cellulase and Lactobacillus casei on ensiling characteristics, chemical composition, antioxidant activity, and digestibility of mulberry leaf silage. J. Dairy Sci. 102, 9919–9931. doi: 10.3168/jds.2019-16468
Helander, I. M., and Mattila-Sandholm, T. (2000). Fluorometric assessment of gram-negative bacterial permeabilization. J. Appl. Microbiol. 88, 213–219. doi: 10.1046/j.1365-2672.2000.00971.x
Hu, W., Schmidt, R. J., McDonell, E. E., Klingerman, C. M., and Kung, L. Jr. (2009). The effect of Lactobacillus buchneri 40788 or Lactobacillus plantarum MTD-1 on the fermentation and aerobic stability of corn silages ensiled at two dry matter contents. J. Dairy Sci. 92, 3907–3914. doi: 10.3168/jds.2008-1788
Hu, Z., Ma, D., Niu, H., Chang, J., Yu, J., Tong, Q., et al. (2021). Enzyme additives influence bacterial communities of Medicago sativa silage as determined by Illumina sequencing. AMB Express 11, 5. doi: 10.1186/s13568-020-01158-5
Ke, W. C., Ding, W. R., Xu, D. M., Ding, L. M., Zhang, P., Li, F. D., et al. (2017). Effects of addition of malic or citric acids on fermentation quality and chemical characteristics of alfalfa silage. J. Dairy Sci. 100, 8958–8966. doi: 10.3168/jds.2017-12875
Kung, L., and Shaver, R. (2001). Interpretation and use of silage fermentation analysis reports. Focus Forage 13, 1–5.
Kung, L. Jr., Shaver, R.D., Grant, R.J., and Schmidt, R.J. (2018). Silage review: interpretation of chemical, microbial, and organoleptic components of silages. J. Dairy Sci. 101, 4020–4033. doi: 10.3168/jds.2017-13909
Li, J., Yuan, X., Desta, S. T., Dong, Z., Mugabe, W., Shao, T., et al. (2018). Characterization of Enterococcus faecalis JF85 and Enterococcus faecium Y83 isolated from Tibetan yak (Bos grunniens) for ensiling Pennisetum sinese. Bioresour. Technol. 257, 76–83. doi: 10.1016/j.biortech.2018.02.070
Li, M., Zhou, H., Zi, X., and Cai, Y. (2017). Silage fermentation and ruminal degradation of stylo prepared with lactic acid bacteria and cellulase. Anim. Sci. J. 88, 1531–1537. doi: 10.1111/asj.12795
Li, M., Zi, X., Zhou, H., Lv, R., Tang, J., Cai, Y., et al. (2019). Silage fermentation and ruminal degradation of cassava foliage prepared with microbial additive. AMB Express 9, 180. doi: 10.1186/s13568-019-0906-2
Li, R., Zheng, M., Zheng, M., Cai, R., Cui, X., Wang, Y., et al. (2021). Metagenomic analysis reveals the linkages between bacteria and the functional enzymes responsible for potential ammonia and biogenic amine production in alfalfa silage. J. Appl. Microbiol. 132, 2594–2604. doi: 10.1111/jam.15411
Lim, T. K. (2014). Senna alata. Edible Medicinal And Non-medicinal Plants. Dordrecht: Springer Netherlands. p. 841–59.
Liu, B., Huan, H., Gu, H., Xu, N., Shen, Q., Ding, C., et al. (2019). Dynamics of a microbial community during ensiling and upon aerobic exposure in lactic acid bacteria inoculation-treated and untreated barley silages. Bioresour. Technol. 273, 212–219. doi: 10.1016/j.biortech.2018.10.041
Lv, H., Pian, R., Xing, Y., Zhou, W., Yang, F., Chen, X., et al. (2020). Effects of citric acid on fermentation characteristics and bacterial diversity of Amomum villosum silage. Bioresour. Technol. 307, 123290. doi: 10.1016/j.biortech.2020.123290
Mu, L., Xie, Z., Hu, L., Chen, G., and Zhang, Z. (2020). Cellulase interacts with Lactobacillus plantarum to affect chemical composition, bacterial communities, and aerobic stability in mixed silage of high-moisture amaranth and rice straw. Bioresour. Technol. 315, 123772. doi: 10.1016/j.biortech.2020.123772
Nadeau, E. M., Buxton, D. R., Russell, J. R., Allison, M. J., and Young, J. W. (2000). Enzyme, bacterial inoculant, and formic acid effects on silage composition of orchardgrass and alfalfa. J. Dairy Sci. 83, 1487–1502. doi: 10.3168/jds.S0022-0302(00)75021-1
Ni, K., Wang, Y., Cai, Y., and Pang, H. (2015). Natural lactic acid bacteria population and silage fermentation of whole-crop wheat. Asian Australas. J. Anim. Sci. 28, 1123–1132. doi: 10.5713/ajas.14.0955
Ogunade, I. M., Kim, D. H., Jiang, Y., Weinberg, Z. G., Jeong, K. C., Adesogan, A. T., et al. (2016). Control of Escherichia coli O157:H7 in contaminated alfalfa silage: effects of silage additives. J. Dairy Sci. 99, 4427–4436. doi: 10.3168/jds.2015-10766
Owens, V. N., Albrecht, K. A., Muck, R. E., and Duke, S. H. (1999). Protein degradation and fermentation characteristics of red clover and alfalfa silage harvested with varying levels of total nonstructural carbohydrates. Crop Sci. 39, 1873–1880. doi: 10.2135/cropsci1999.3961873x
Pahlow, G., Muck, R. E., Driehuis, F., Elferink, S. J. W. H. O., and Spoelstra, S. F. (2015). Microbiology of ensiling. Silage science and technology. Agron. Monogr. 42, 31–93. doi: 10.2134/agronmonogr42.c2
Ranjit, N. K., and Kung, L. Jr. (2000). The effect of Lactobacillus buchneri, Lactobacillus plantarum, or a chemical preservative on the fermentation and aerobic stability of corn silage. J. Dairy Sci. 83, 526–535. doi: 10.3168/jds.S0022-0302(00)74912-5
Rauramaa, A., Setäl,ä, J., Moisio, T., Heikkil,ä, T., and Lampila, M. (1987). The effect of inoculants and cellulase on the fermentation and microbiological composition of grass silage: I biochemical changes in the silages. Agric. Food Sci. 59, 361–370. doi: 10.23986/afsci.72269
Schmidt, R. J., and Kung, L. Jr. (2010). The effects of Lactobacillus buchneri with or without a homolactic bacterium on the fermentation and aerobic stability of corn silages made at different locations. J. Dairy Sci. 93, 1616–1624. doi: 10.3168/jds.2009-2555
Sepehri, A., and Sarrafzadeh, M. H. (2019). Activity enhancement of ammonia-oxidizing bacteria and nitrite-oxidizing bacteria in activated sludge process: metabolite reduction and CO2 mitigation intensification process. Appl. Water Sci. 9, 1–2. doi: 10.1007/s13201-019-1017-6
Shao, T., Shimojo, M., Wang, T., and Masuda, Y. (2005). Effect of additives on the fermentation quality and residual mono- and disaccharides compositions of forage oats (Avena sativa L.) and Italian ryegrass (Lolium multiflorum Lam.) silages. Asian Australas. J. Anim. Sci. 18, 1582–1588. doi: 10.5713/ajas.2005.1582
Spoelstra, S. F., Courtin, M. G., and Beers, J. (1988). Acetic acid bacteria can initiate aerobic deterioration of whole crop maize silage. J. Agric. Sci. 3, 127–132. doi: 10.1017/S0021859600082915
Stewart, V. (1988). Nitrate respiration in relation to facultative metabolism in enterobacteria. Microbiol. Rev. 52, 190–232. doi: 10.1128/mr.52.2.190-232.1988
Tian, H., Wang, Y., Liu, Z., Hu, Z., Guo, Y., Deng, M., et al. (2021). Effects of malic acid and sucrose on the fermentation parameters, CNCPS nitrogen fractions, and bacterial community of Moringa oleifera leaves silage. Microorganisms 9, 2102. doi: 10.3390/microorganisms9102102
Van Soest, P. J., Robertson, J. B., and Lewis, B. A. (1991). Methods for dietary fiber, neutral detergent fiber, and nonstarch polysaccharides in relation to animal nutrition. J. Dairy Sci. 74, 3583–3597. doi: 10.3168/jds.S0022-0302(91)78551-2
Wang, B., Sun, Z., and Yu, Z. (2020b). Pectin degradation is an important determinant for alfalfa silage fermentation through the rescheduling of the bacterial community. Microorganisms 8, 488. doi: 10.3390/microorganisms8040488
Wang, C., He, L., Xing, Y., Zhou, W., Yang, F., Chen, X., et al. (2019a). Fermentation quality and microbial community of alfalfa and stylo silage mixed with Moringa oleifera leaves. Bioresour. Technol. 284, 240–247. doi: 10.1016/j.biortech.2019.03.129
Wang, C., Pian, R., Chen, X., Lv, H., Zhou, W., Zhang, Q., et al. (2020a). Beneficial effects of tannic acid on the quality of bacterial communities present in high-moisture mulberry leaf and stylo silage. Front. Microbiol. 11, 586412. doi: 10.3389/fmicb.2020.586412
Wang, L., Zhang, H. J., Zhu, Y. U., Chun-Cheng, X. U., and Sun, Q. Z. (2011). The fermentation quality of mixed silage of Medicago sativa and Roegneria turczaninovii. Pratacult. Sci. 28, 1888–1893.
Wang, Y., Chen, X., Wang, C., He, L., Zhou, W., Yang, F., et al. (2019b). The bacterial community and fermentation quality of mulberry (Morus alba) leaf silage with or without Lactobacillus casei and sucrose. Bioresour. Technol. 293, 122059. doi: 10.1016/j.biortech.2019.122059
Xiang-Lin, L. I., Zhang, X. Y., Tang, Y. G., Feng, H. E., and Zhang, J. Z. (2008). Effect of concentrate-forage ratio in diet on liveweight gain of stall-fed goats. Acta Prataculturae Sinica 17, 85–91. doi: 10.1007/s10499-007-9164-4
Yitbarek, M. B., and Tamir, B. (2014). Silage additives: review. Open J. Appl. Sci. 04, 258–274. doi: 10.4236/ojapps.2014.45026
You, S., Du, S., Ge, G., Wan, T., and Jia, Y. (2021b). Microbial community and fermentation characteristics of native grass prepared without or with isolated lactic acid bacteria on the mongolian plateau. Front. Microbiol. 12, 731770. doi: 10.3389/fmicb.2021.731770
You, S. H., Du, S., Ge, G. T., Wan, T., and Jia, Y. S. (2021a). Selection of lactic acid bacteria from native grass silage and its effects as inoculant on silage fermentation. Agron. J. 113, 3169–3177. doi: 10.1002/agj2.20720
Zadoks, R. N., Griffiths, H. M., Munoz, M. A., Ahlstrom, C., Bennett, G. J., Thomas, E., et al. (2011). Sources of Klebsiella and Raoultella species on dairy farms: be careful where you walk. J. Dairy Sci. 94, 1045–1051. doi: 10.3168/jds.2010-3603
Zhang, Y. C., Li, D. X., Wang, X. K., Lin, Y. L., Zhang, Q., Chen, X. Y., et al. (2019). Fermentation dynamics and diversity of bacterial community in four typical woody forages. Ann. Microbiol. 69, 233–240. doi: 10.1007/s13213-018-1398-z
Zhao, C., Wang, L., Ma, G., Jiang, X., Yang, J., Lv, J., et al. (2021). Cellulase interacts with lactic acid bacteria to affect fermentation quality, microbial community, and ruminal degradability in mixed silage of soybean residue and corn stover. Animals 11, 334. doi: 10.3390/ani11020334
Keywords: Cassia alata, cellulase, Lactiplantibacillus plantarum, silage quality, microbial community
Citation: Xian Z, Wu J, Deng M, Wang M, Tian H, Liu D, Li Y, Liu G, Sun B and Guo Y (2022) Effects of Cellulase and Lactiplantibacillus plantarum on the Fermentation Parameters, Nutrients, and Bacterial Community in Cassia alata Silage. Front. Microbiol. 13:926065. doi: 10.3389/fmicb.2022.926065
Received: 22 April 2022; Accepted: 13 June 2022;
Published: 07 July 2022.
Edited by:
Tian Zhang, Wuhan University of Technology, ChinaReviewed by:
Atte Von Wright, University of Eastern Finland, FinlandCopyright © 2022 Xian, Wu, Deng, Wang, Tian, Liu, Li, Liu, Sun and Guo. This is an open-access article distributed under the terms of the Creative Commons Attribution License (CC BY). The use, distribution or reproduction in other forums is permitted, provided the original author(s) and the copyright owner(s) are credited and that the original publication in this journal is cited, in accordance with accepted academic practice. No use, distribution or reproduction is permitted which does not comply with these terms.
*Correspondence: Yongqing Guo, eW9uZ3FpbmdAc2NhdS5lZHUuY24=
†These authors have contributed equally to this work
Disclaimer: All claims expressed in this article are solely those of the authors and do not necessarily represent those of their affiliated organizations, or those of the publisher, the editors and the reviewers. Any product that may be evaluated in this article or claim that may be made by its manufacturer is not guaranteed or endorsed by the publisher.
Research integrity at Frontiers
Learn more about the work of our research integrity team to safeguard the quality of each article we publish.