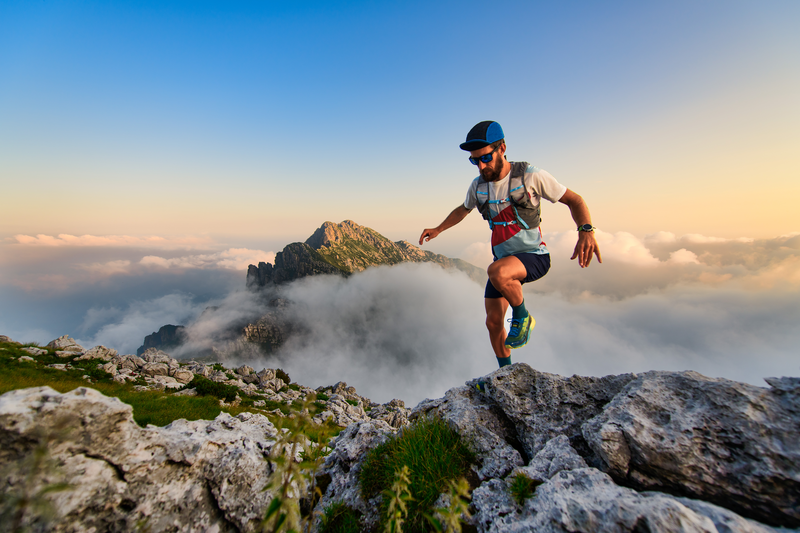
94% of researchers rate our articles as excellent or good
Learn more about the work of our research integrity team to safeguard the quality of each article we publish.
Find out more
REVIEW article
Front. Microbiol. , 15 July 2022
Sec. Food Microbiology
Volume 13 - 2022 | https://doi.org/10.3389/fmicb.2022.922450
This article is part of the Research Topic Antimicrobial Resistant Pathogens in Sustainable Food Systems: How to Prevent, Detect or Control Contamination? View all 7 articles
The fungal decay of fresh fruits and vegetables annually generates substantial global economic losses. The utilization of conventional synthetic fungicides is damaging to the environment and human health. Recently, the biological control of post-harvest fruit and vegetable diseases via antagonistic microorganisms has become an attractive possible substitution for synthetic fungicides. Numerous studies have confirmed the potential of volatile organic compounds (VOCs) for post-harvest disease management. Moreover, VOC emission is a predominant antifungal mechanism of antagonistic microorganisms. As such, it is of great significance to discuss and explore the antifungal mechanisms of microbial VOCs for commercial application. This review summarizes the main sources of microbial VOCs in the post-harvest treatment and control of fruit and vegetable diseases. Recent advances in the elucidation of antifungal VOC mechanisms are emphasized, and the applications of VOCs produced from antagonistic microorganisms are described. Finally, the current prospects and challenges associated with microbial VOCs are considered.
Fruit and vegetable losses are between 35 and 55% of their production volume, depending on the region. Considering that the decay of fruits and vegetables is a consequence of phytopathogen proliferation on their edible parts, various new anti-phytopathogenic strategies are actively being investigated (Leneveu-Jenvrin et al., 2020). Generally, fruits are protected against decay using chemical substances; however, consumer acceptance of such pesticides is decreasing considering their associated environmental pollution and possible harmful health effects. Furthermore, pathogens can develop resistance against pesticides such as carbendazim and diethofencarb (Ocampo-Suarez et al., 2017). SO2 can be used as a fungicide during fruit storage and can inhibit post-harvest pathogen growth in grapes, reduce fruit respiration rate, and maintain fruit quality. However, excess SO2 can cause bleaching of grapes, and the SO2 residue can be detrimental to human health (Considine and Foyer, 2015). Several areas, such as the United States and the European Union, have promoted the “Integrated Pest Management (IPM)” project to reduce and ultimately eliminate chemical pesticide usage (Diaz et al., 2020). Therefore, it is crucial to develop safer, environmentally friendly, and effective methods against pathogenic fungi.
The usage of antagonistic microorganisms in the biological control of post-harvest fruit and vegetable diseases may be a promising substitute for synthetic fungicides. Antagonistic microorganisms inhibit pathogenic growth via competition for nutrients and space, parasitism, antibiosis, host resistance induction, volatile organic compound (VOC) emission, and biofilm formation (Zhang et al., 2020). In particular, VOC emission is a predominant antifungal mechanism of antagonistic microorganisms. Accordingly, Contarino et al. (2019) demonstrated that VOCs produced by Wickerhamomyces anomalus, Metschnikowia pulcherrima, Aureobasidium pullulans, and Saccharomyces cerevisiae effectively inhibited post-harvest pathogenic molds. Moreover, each antagonist can produce a wide variety of VOCs. The main VOCs emitted by biological control yeasts are alcohols (ethanol, 3-methylbutan-1-ol, and 2-phenylethanol) and esters (ethyl acetate and 3-methylbutyl acetate). Volatiles do not require antagonistic microorganisms direct contact with food and are, thus, currently considered potential biofumigants.
VOCs derived from antifungal bacteria, filamentous fungi, and yeasts are used for the control of pathogenic fungi in fruits and vegetables. Herein, we review all reported biological control strategies of VOCs for the management of pathogenic fungi in fruits and vegetables. First, microbial-derived VOCs are discussed in detail, whereafter, recent advances in the elucidation of VOC antifungal mechanisms are emphasized. Finally, the applications of VOCs derived from biological control microbes, and the associated prospects and challenges are reviewed.
The application of yeast as a biological control agent (BCA) has been extensively studied; seeing as they are environmentally friendly, have no negative toxicological impacts, and their large-scale cultivation is effortless and cost-effective (Mari et al., 2016). Moreover, yeast strains, such as Aureobasidium spp., Candida spp., Kloeckera spp., Metschnikowia spp., Pichia spp., Saccharomyces spp., Rhodotorula spp., and Wickerhamomyces spp., have been reported to have antifungal properties (Lemos Junior et al., 2020). Various mechanisms have been postulated to describe these antifungal properties, including enhanced natural host defenses, competition for nutrients, and antifungal VOC production. Among these mechanisms, the production of VOCs may be particularly pertinent to the antifungal properties of yeast (Figure 1A; Table 1).
Figure 1. Molecular classes of major active compounds in volatile organic compounds (VOCs) from yeast (A), bacterial (B), and fungal (C). The active compounds in VOCs exert promising antimicrobial activities in the biological control of plant pathogens.
Volatile organic compounds produced by Pichia spp. reduce the incidence of Monascus purpureus by up to 39.22%, and 2-phenylethanol elicits its antifungal effect on M. purpureus by inducing protein synthesis and DNA damage (Zhang et al., 2021). Moreover, 2-phenylethanol is also the main antifungal VOC produced by Candida intermedia. VOCs produced by C. intermedia can affect protein biosynthesis, proliferative activity, mitochondrial metabolism, and detoxification. Accordingly, C. intermedia-produced VOCs and 2-phenylethanol successfully inhibit Aspergillus carbonarius radial mycelial growth and reduce ochratoxin A (OTA) production (Tilocca et al., 2019). Furthermore, Ruiz-Moyano et al. (2020) found that VOCs produced by Hanseniaspora uvarum effectively controlled the incidence of Botrytis cinerea in strawberries and cherries; the main VOCs identified included acetic acid, octanoic acid, ethyl propanoate, N-propyl acetate, 2-methylpropyl acetate, 2-methylbutyl acetate, furan-2-ylmethyl acetate, benzyl acetate, 2-phenylethyl acetate, and heptan-2-one.
Masoud et al. (2005) demonstrated that, during coffee processing, VOCs produced by Pichia anomala, Pichia kluyveri, and H. uvarum inhibited the growth of Aspergillus ochraceus and prevented the production of OTA. Moreover, the most effective VOC was 2-phenylethyl acetate, which completely inhibited A. ochraceus growth at 48 μg/L headspace. Similarly, VOCs produced by Saccharomyces spp. hampered spore production and mycelial growth of A. carbonarius and A. ochraceus. Among the Culture Collection of Agricultural Microbiology (CCMA) Saccharomyces spp. strains, S. cerevisiae CCMA 0159, 1,299, and 1,302 exhibited the most efficient in vitro inhibition of ochratoxigenic fungi, while also reducing in vivo OTA production to 0.04–10.11 μg/kg (de Souza et al., 2021).
Jaibangyang et al. (2020) identified Candida nivariensis DMKU-CE18 as an effective VOC-producing (mainly pentan-1-ol) yeast against the growth and conidial germination of Aspergillus flavus. Moreover, Jaibangyang et al. (2021) revealed that Kwoniella heveanensis DMKU-CE82-produced VOCs induced conidia structural damage, inhibited mycelia and conidiophore development, and reduced aflatoxin B1 (AFB1) production to less than 20 ppb in A. flavus contaminated corn grains. The major VOCs produced by K. heveanensis DMKU-CE82 were closely matched to 3-methylbutan-1-ol, 2-methylbutan-1-ol, 1,1-dimethylhydrazine, and 3-methylbutanoic acid. Debaryomyces nepalensis-produced VOCs predominantly include 2-phenylethanol and can play important roles in the suppression of Colletotrichum gloeosporioides. This is of great significance, considering that C. gloeosporioides-induced fruit anthracnose results in tremendous economic losses due to the latency of the infection (Zhou et al., 2018).
Volatile organic compounds produced by bacteria have low molecular weights and polarities and can effortlessly diffuse through porous soil structures and over great atmospheric distances (Figure 1B; Table 2). These properties significantly contribute to the potential applications of bacterial VOCs in various environments, including in plantation fields and greenhouses, and during storage (Arrarte et al., 2017). Correspondingly, several studies have demonstrated the potential applications of bacterial VOCs in plant disease management and in post-harvest disease control (Dhouib et al., 2019; Calvo et al., 2020).
The antifungal capability of Bacillus subtilis on post-harvest citrus was first reported in the 1950s (Wilson and Chalutz, 1989). Recently, VOC emissions were identified as the main antifungal mechanism of the Bacillus spp. strains. Massawe et al. (2018) identified eight Bacillus spp.-produced VOCs that reduced sclerotial production and inhibited mycelial growth of Sclerotinia sclerotiorum. Moreover, VOCs emitted by the B. subtilis CL2 strain inhibited the hyphal growth of four pathogenic fungi (Mucor circinelloides LB1, Fusarium arcuatisporum LB5, Alternaria iridiaustralis LB7, and Colletotrichum fioriniae LB8) and significantly reduced the weight loss rate and decay incidence of wolfberry fruits. The main active antifungal substances in these VOCs are butane-2,3-dione and 3-methylbutanoic acid (Ling et al., 2021). VOCs produced by Bacillus pumilus and Bacillus thuringiensis significantly inhibit the in vitro mycelia growth of C. gloeosporioides. Accordingly, the inhibition incidences of inoculated mangos exposed to the VOCs of B. pumilus and B. thuringiensis were 94.3 and 87.6%, respectively (Zheng et al., 2013). VOCs produced by Bacillus velezensis significantly inhibit in vitro and fruit borne B. cinerea, Monilinia fructicola, Monilinia laxa, Penicillium italicum, Penicillium digitatum, and Penicillium expansum growth; particularly M. laxa (66%), M. fructicola (72%), P. italicum (80%), and B. cinerea (100%). These VOCs mainly include nonan-2-one, undecan-2-one, heptan-2-one, butan-1-ol, 3-hydroxybutan-2-one, benzaldehyde, butyl formate, butane-2,3-dione, nonane, and pyrazine (Calvo et al., 2020). Furthermore, the B. velezensis-produced VOCs; tetradecane, 2-phenylacetic acid, benzaldehyde, dec-1-ene, and 2-phenylethanol, also exhibit significant antifungal activity against Verticillium dahliae. In addition, B. velezensis application significantly reduces the incidence of Verticillium wilt by 70.43 ± 7.08% in tomato plants (Dhouib et al., 2019).
Wang et al. (2020) reported that VOCs produced by the antagonistic bacteria, Pseudomonas fluorescens ZX significantly inhibited mycelial growth and conidial germination of P. italicum by 42.14 and 77.86%, respectively. Moreover, the primary active antifungal constituents of these P. fluorescens ZX-produced VOCs included organic acids and sulfur compounds (Wang et al., 2021c).
The VOCs derived from endophytic bacterial strains also exhibit antifungal activity against pathogens. Accordingly, Pseudomonas stutzeri E25 and Stenotrophomonas maltophilia CR71 inhibits B. cinerea growth via VOC emission, with (methyldisulfanyl) methane as the main component (Rojas-Solís et al., 2018). VOCs produced by tomato-derived endophytic bacterial strains, such as Bacillus nakamurai, Bacillus pseudomycoides, Bacillus proteolyticus, B. thuringiensis, Enterobacter asburiae, and Enterobacter cloacae, exhibit antifungal activity against B. cinerea (Chaouachi et al., 2021).
Volatile organic compounds produced by Pseudomonas sp. (No. 3, No. 35), Enterobacter sp. (No. 26, No. 34), Ralstonia sp. (No. 50), Bacillus sp. (No. 62), Arthrobacter sp. (No. 146), Brevibacillus sp. (No. 2–18), and Paenisporosarcina sp. (No. 2–60) exhibits varying inhibitory effects (7.84–100%) on Rhizoctonia solani growth. In particular, Ralstonia sp. completely inhibits the growth of R. solani as a result of VOC production, among which ethyl benzoate, 3-methylbutanoic acid, 2-ethylhexan-1-ol, 3-methylbutan-1-ol, and 6-methylhept-5-en-2-one are confirmed to be toxic to R. solani (Wang et al., 2021a). Additionally, R. solani is also inhibited by VOCs derived from several Streptomyces spp. soil isolates, in which the effective VOC constituents include methyl 2-methylpentanoate and 1,3,5-trichloro-2-methoxy benzene (Cordovez et al., 2015).
Gómez et al. (2021) identified several anti-phytopathogenic marine actinobacteria, including Streptomyces sp. (PNM-149), which exhibited antifungal activity against C. gloeosporioides growth via two major VOC components (methyl 2-aminobenzoate and 1,2,7,7-tetramethylbicyclo[2.2.1]heptan-2-ol). Moreover, Bacillus atrophaeus elicits significant inhibition against various fungal pathogens. The primary inhibitory VOCs produced by B. atrophaeus HAB-5 against C. gloeosporioides included 2-chloroacetic acid, tetradecyl esters, octadecane, and methyl hexadecanoate (Rajaofera et al., 2019). Overall, these studies provide a foundation for the application of antagonistic bacteria in the control of fungal infections.
In addition to yeast and bacteria, several fungal species elicit biological control activities by producing VOCs (Figure 1C; Table 3). Among the antagonistic fungi, the yeast-like fungus A. pullulans is recognized as a propitious post-harvest disease BCA (Di Francesco et al., 2020a). Accordingly, Alternaria alternata and B. cinerea conidia germination and colony growth are suppressed by A. pullulans-derived VOCs, including ethanol and 2-phenylethanol as the key inducers of this inhibitory effect (Di Francesco et al., 2015). Moreover, VOCs derived from A. pullulans L1 and L8 inhibit Monilinia fructigena mycelium growth (70 and 50%, respectively) and M. fructicola conidia germination (85 and 70%, respectively). As the most active compound among the VOCs, 2-phenylethanol displays inhibitory action against all the pathogens on cherry and apricot fruits (Di Francesco et al., 2020b). In addition, VOCs generated by Aureobasidium subglaciale, of which 3-methylbutan-1-ol is the most effective, inhibit B. cinerea mycelial growth (65.4%; Di Francesco et al., 2020a). VOCs produced by A. pullulans, which mainly include ethanol, 2-methylpropan-1-ol, 3-methylbutan-1-ol, and 2-phenylethanol, can increase intracellular reactive oxygen species (ROS) accumulation, lipid peroxidation, and content, leakage, thereby inhibiting B. cinerea growth (Don et al., 2020).
Trichoderma spp. plays an important role as a BCA in a wide variety of plants (Sunpapao et al., 2018; Baiyee et al., 2019). Accordingly, the major Trichoderma spirale T76-1-produced VOC (6-pentylpyran-2-one) suppresses Corynespora cassiicola and Curvularia aeria growth by 41.29 and 42.35%, respectively (Baiyee et al., 2019). Moreover, Trichoderma asperellum T76-14-emitted VOCs, particularly 2-phenylethanol, effectively inhibits Fusarium incarnatum growth (62.5%) and rot after 7 days of incubation (Intana et al., 2021).
Li et al. (2010) reported suppressed P. italicum spore germination, mycelial growth, sporulation, and disease incidence in inoculated citrus in the presence of Streptomyces globisporus JK-1-derived VOCs. Moreover, these VOCs can inhibit B. cinerea growth on media and in inoculated tomatoes (Li et al., 2012).
Limited information exists regarding the molecular and physiological mechanisms by which VOCs control post-harvest diseases. Nevertheless, the main mechanism underlying the antifungal effects of VOCs is the disruption of cell wall and membrane structures, leading to intracellular lysate leakage and oxidative stress induction (Figure 2).
Figure 2. Antifungal mechanism of the VOCs. VOCs damage cell walls and membranes, resulting in changes in the morphology of microbial cells and leakage of cell contents; VOCs disrupt redox balance and increase intracellular ROS level causing membrane lipid peroxidation, mitochondrial dysfunction, and decreased ATP levels.
The shape of microbial cells is maintained by the increased mechanical resistance provided by the cell wall and membrane. The cell wall is composed of three major macromolecules (mannoproteins, β-glucan, and chitin) essential for cell morphology sustainability and protection against mechanical damage. The integrity of fungal cell membranes, which are composed of proteins, phospholipids, and sugars, are vital to the survival of fungi. However, microbial VOCs can damage cell walls and membranes, resulting in microbial morphological changes.
Accordingly, B. cinerea treated with S. globisporus JK-1-derived VOCs exhibit excessive vesiculation, thickened walls, and retracted membranes (Li et al., 2012). Moreover, Trichoderma sp., Phoma sp., and Colletotrichum sp. exposed to Chromobacterium vaccinii-derived VOCs exhibit extensive morphological abnormalities, such as swollen hyphal cells, vacuolar depositions, and cell wall alterations (Ebadzadsahrai et al., 2020). Tahir et al. (2017) reported that benzaldehyde, 1,2-benzothiazol-3-one, and buta-1,3-diene released by Bacillus spp. caused morphological and ultra-structural changes in Ralstonia solanacearum cells. Correspondingly, Wang et al. (2021b) demonstrated inhibition of C. gloeosporioides growth via B. subtilis CF-3 VOC-induced downregulation of gene expression related to cell membrane fluidity, wall integrity, energy metabolism, and the production of cell wall-degrading enzymes. In addition, 2,4-ditert-butylphenol, which is a characteristic VOC of B.subtilis CF-3, elicits similar inhibitory effects on C. gloeosporioides.
Some VOCs directly target fungal cell membranes by increasing membrane permeability and cellular leakage. VOCs, such as organic acids, increase cell membrane fluidity, leading to membrane protein conformational changes, intracellular content leakage, and subsequent fungal cell death. Moreover, the direct insertion of Pseudomonas spp.-produced cis-9-heptadecenoic acid [(Z)-heptadec-9-enoic-acid] in the phospholipid bilayer of cell membranes, and subsequent interaction with fungal cell membranes increases membrane fluidity and eventuates in the death of pathogenic fungi such as B. cinerea (Avis and Belanger, 2001). Furthermore, Bergsson et al. (2001) demonstrated that decanoic acid destroyed Candida albicans cell membranes, resulting in the outflow of cytoplasmic contents and rapid, effective elimination of the pathogen fungi.
Volatile organic compounds also alter fungal membrane permeability via peroxidation of membrane lipids. Accordingly, A. pullulans VOCs may trigger lipid peroxidation and electrolyte leakage in B. cinerea and A. alternata (Yalage Don et al., 2021). Additionally, Psidium guajava exposure to S. cerevisiae VOCs increased the membrane lipid peroxidation plasma membrane permeability (Dalilla et al., 2015). Excessive ROS production alters lipid layer composition and triggers lipid peroxidation via the conversion of unsaturated lipids to polar lipid hydroperoxides (Vazquez et al., 2019). Moreover, extensive lipid peroxidation-induced alterations in cell membrane permeability result in membrane disintegration, free radical chain reactions, and eventual cell death (Massawe et al., 2018).
In summary, cell and organelle membranes are potential VOC targets via membrane damage-induced cell structure deformation and cytoplasmic inclusion of organelle material. Moreover, VOCs may enter fungal cells via hydrogen bonding. Consequently, the forces created during this bonding disturb the aqueous solution of cell membranes and interfere with cellular physiology and functionality.
Volatile organic compounds derived from biological control microbes trigger ROS accumulation and oxidative stress in fungal cells. Excessive ROS accumulation disrupts the redox balance, reacts with cellular macromolecules, such as lipids, proteins, and DNA, and eventuates in cell dysfunction or death.
Massawe et al. (2018) identified four endophytic Bacillus spp. VOCs that strongly induced ROS production in S. sclerotiorum mycelial cells. Insufficient detoxification of ROS by cellular antioxidant defense mechanisms, such as catalase (CAT) and superoxide dismutase (SOD) activities, results in oxidative stress. As such, the increased CAT and SOD activity observed by Fialho et al. (2014) in Guignardia citricarpa mycelia following S. cerevisiae VOC exposure indicated VOC-induced imbalanced fungal redox states.
Fialho et al. (2014) reported that S. cerevisiae CR-1 VOCs inhibited G. citricarpa growth by disrupting the intracellular redox homeostasis and triggering harmful ROS accumulation. Moreover, Xie et al. (2020) reported that the B. subtilis DZSY21 VOC, 3-methylbutyl acetate, strongly induced intracellular ROS accumulation and inhibited mycelia growth and conidial sporulation of Curvularia lunata. Ye et al. (2020) observed significant inhibition of Fusarium oxysporum f. sp. cucumerinum by Corallococcus sp. EGB VOCs, particularly 6-methylheptan-1-ol. Accordingly, ROS accumulation and gradual fungal cell apoptosis occurred following 6-methylheptan-1-ol treatment.
Reactive oxygen species are mainly generated during aerobic respiration via the complex I enzyme of the mitochondrial respiratory chain (Yalage Don et al., 2021). Furthermore, Zhang et al. (2021) demonstrated hypha morphological changes, cell membrane destruction, ergosterol reduction, and significant ROS accumulation in Ceratocystis fimbriata cells following exposure to Pseudomonas chlororaphis subsp. aureofaciens SPS-41 VOCs. Consequentially, oxidative stress-induced mitochondrial dysfunction and decreased ATP levels inhibited the growth of C. fimbriata. Moreover, S. cerevisiae-produced decanoic acid significantly decreases intracellular ATP levels and inhibits B. cinerea growth, possibly via mechanisms related to energy metabolism. Stevens and Hofmeyr (1993) demonstrated the cytoplasmic entry of octanoic acid and decanoic acid through S. cerevisiae membranes and subsequent H+ dissociation, significant cytoplasmic pH decrease, and membrane H+-ATPase activation. Cellular ATP exhaustion occurs during H+ emission, resulting in growth inhibition. Overall, VOC-induced ROS accumulation and oxidative stress lead to pathogenic fungal growth inhibition; however, the complete mechanism of ROS remains to be further elucidated.
Microbial VOCs have been applied in the control of diseases, such as grey mold, green mold, and blue mold, and to reduce toxins such as OTA (Figure 3).
Figure 3. Applications of VOCs. VOCs are applied to inhibit phytopathogenic fungi, such as grey mold, green mold, and blue mold that cause rot in fruit and vegetables; VOCs are also applied to inhibit pathogenic fungi that produce toxins such as OTA.
Grey mold, caused by B. cinerea, is a profound pre- and post-harvest fruit and vegetable disease. More than 200 species of plants, including cucumbers, table grapes, tomatoes, and strawberries, are susceptible to gray mold infection (Huang et al., 2012). Chemical fungicides are utilized in the management of this disease; however, B. cinerea effortlessly develops fungicide resistance due to its high genetic variability, prolific reproduction, and short life cycle (Chaouachi et al., 2021). Recently, research has been aimed at the development of environmentally-friendly control methods against B. cinerea, including BCA utilization. Botrytis cinerea disease incidence and severity on wound-inoculated tomato fruit are inhibited when fumigated with S. globisporus JK-1 (Li et al., 2012). Moreover, the bio-fumigation of apples with A. pullulans L1 and L8 VOCs effectively controlled B. cinerea, Colletotrichum acutatum, P. expansum, P. digitatum, and P. italicum. The lesion diameter of apples inoculated with B. cinerea following A. pullulans L1 and L8 VOC exposure reduced by 88.9 and 94.4%, respectively. Moreover, A. pullulans L1 and L8 VOCs reduce the incidence of blue mold and bitter pit on apples by 73.9 and 44.4%, respectively (Di Francesco et al., 2015). The VOCs produced by M. pulcherrima, W. anomalus, A. pullulans, and S. cerevisiae are highly effective in controlling gray mold-induced decay of grape berries (Parafati et al., 2015). Huang et al. (2011) demonstrated that C. intermedia VOC (cyclooctatetraene and 3-methylbutan-1-ol) exposure significantly reduced the incidence and severity of Botrytis spp. strawberry rot. Moreover, the VOCs derived from Sporidiobolus pararoseus effectively suppressed strawberry gray mold disease under air-tight conditions (Huang et al., 2012). Similarly, the tomato-derived endophytic Enterobacter sp. TR1 VOC (3-methylbutan-1-ol) completely suppresses B. cinerea infection and growth at 0.442 ml/L, whereas Bacillus spp. protects against fungal infection when applied to vegetative cells of tomatoes. VOCs derived from B. velezensis I3 reduce grey mold in grapes by 50%, while those of B. velezensis BUZ-14 decrease brown rot severity in apricots (Chaouachi et al., 2021).
The microbial metabolite, OTA, consists of the amino acid, phenylalanine, linked by an amide bond to a pentaketide dihydroisocoumarin. OTA is the second most predominant mycotoxin found in food and feed products, and is classified as a group 2B carcinogen by the World Health Organization (Farbo et al., 2018). Furthermore, OTAs are predominantly produced by Aspergillus spp. and Penicillium spp. in warm and tropical regions. Candida intermedia 235 and Lachancea thermotolerans 751 significantly inhibit A. carbonarius on grape berries and in vitro, while VOCs produced by non-fermenting (Cyberlindnera jadinii 273 and Candida friedrichii 778) and low-fermenting (C. intermedia 235 and L. thermotolerans 751) yeast strains may prevent in vitro A. carbonarius sporulation. Moreover, C. intermedia 235, L. thermotolerans 751, and C. friedrichii 778 efficiently adsorb artificially spiked OTA from grape juice (Fiori et al., 2014). However, the main antifungal VOC in C. intermedia 253 (2-phenylethanol) only partially mimics the metabolic effects of whole yeast VOCs (Tilocca et al., 2019). Additionally, Saccharomyces spp.-produced VOCs hamper A. carbonarius CCDCA 10608 and A. ochraceus CCDCA 10612 spore production and mycelial growth (de Souza et al., 2021).
Penicillium digitatum (green mold) and P. italicum Wehmer (blue mold) result in significant post-harvest economical losses (Papoutsis et al., 2019). Both P. digitatum and P. italicum are wound pathogens that produce a large amount of airborne spores (Kellerman et al., 2016). The disease incidence of P. expansum on apples in the presence of Candida sake VOCs [mainly 3-methylbutyl hexanoate, 3-methylbutyl pentanoate, 2-methylpropyl hexanoate, and ethyl 4-(4-nitrophenyl)-1,3-thiazole-2-carboxylate] is reduced by 53% and the severity by 20%, indicating that C. sake VOCs are effective P. expansum BCAs in apples. VOCs produced by W. anomalus efficiently reduce P. digitatum infections in mandarin fruits (Parafati et al., 2017). Moreover, Li et al. (2010) reported that blue mold sporulation and disease incidence on citrus were reduced in the presence of the VOCs from S. globisporus JK-1. Accordingly, Wang et al. (2020) reported suppressed citrus blue mold disease incidence and lesion size by VOCs from P. fluorescens. Moreover, the P. fluorescens VOCs (100 μl/L dimethyl disulfide and 10 μl/L dimethyl trisulfide) completely inhibited blue mold on citrus fruits (Wang et al., 2021c).
In the post-harvest stage, VOCs may be regarded as ideal BCAs, considering that their activity does not require direct contact with the pathogen or food. However, to effectively apply these BCAs, their underlying antagonistic and pathogenic mechanisms must first be elucidated, thereby allowing for an understanding of their interactions and biology (Calvo et al., 2020). In the pre-harvest stage, VOCs are mainly used in open-field agricultural and horticultural practices. The major challenge for large-scale VOC application in agricultural and horticultural practices is its volatility (Tilocca et al., 2020). Technical progress from controlled conditions to agricultural systems is required to overcome the current scaling limitations of VOC implementation, thereby formulating more effective and productive applications in the field and during post-harvest management.
To date, a large body of research exists on the utilization of VOCs as pre- and post-harvest BCAs. However, limited studies have reached the commercial development stage and launched commercial products. The main reason for this is the general lack of knowledge associated with VOC mechanisms of action. Moreover, the low solubility of VOCs in water limits its aquatic applications (Kanchiswamy et al., 2015).
Toxicity studies are a requisite for all novel BCAs that reach the market (Ocampo-Suarez et al., 2017). Considering that VOC activity ranges from proximal interactions via water diffusion to distant interactions via air diffusion, the possible hazards of VOCs need to be thoroughly evaluated (Spadaro and Droby, 2016). Some BCAs, such as Pichia kudriavzevii, are known nosocomial pathogens and may cause neonatal deaths (Nagarathnamma et al., 2017). Thus, although numerous studies may be generating valuable information in terms of disease control, the associated data would not be suitable for the practical development of BCAs.
Fruit and vegetable decay results in immense global economic losses and is harmful to human health. Biological control of post-harvest fruit and vegetable diseases by antagonistic microorganisms has been extensively studied, and the post-harvest disease management potential of VOCs has been confirmed; however, successful commercial application of VOCs is yet to be achieved. Therefore, future studies are required to comprehensively elucidate the antifungal mechanisms of VOCs to accommodate the development of antagonistic microorganisms suitable for commercial applications.
XZ: conceptualization, software, visualization, writing—original draft, and writing—review and editing. JZ: investigation, visualization, software, and writing—review and editing. RT: visualization and writing—review and editing. YL: funding acquisition, project administration, and writing—review and editing. All authors contributed to the article and approved the submitted version.
This work was supported by Research start-up funds of Northwest A&F University (Z1090121025), Ningxia Helan Mountain’s East Foothill Wine Experiment and Demonstration Station of Northwest A&F University (750104), and Ningxia Key Research and Development Project (2020BCF01003).
The authors declare that the research was conducted in the absence of any commercial or financial relationships that could be construed as a potential conflict of interest.
All claims expressed in this article are solely those of the authors and do not necessarily represent those of their affiliated organizations, or those of the publisher, the editors and the reviewers. Any product that may be evaluated in this article, or claim that may be made by its manufacturer, is not guaranteed or endorsed by the publisher.
Arrarte, E., Garmendia, G., Rossini, C., Wisniewski, M., and Vero, S. (2017). Volatile organic compounds produced by Antarctic strains of Candida sake play a role in the control of postharvest pathogens of apples. Biol. Control 109, 14–20. doi: 10.1016/j.biocontrol.2017.03.002
Avis, T. J., and Belanger, R. R. (2001). Specificity and mode of action of the antifungal fatty acid cis-9-heptadecenoic acid produced by Pseudozyma flocculosa. Appl. Environ. Microbiol. 67, 956–960. doi: 10.1128/AEM.67.2.956-960.2001
Baiyee, B., Pornsuriya, C., Ito, S.-I., and Sunpapao, A. (2019). Trichoderma spirale T76-1 displays biocontrol activity against leaf spot on lettuce (Lactuca sativa L.) caused by Corynespora cassiicola or Curvularia aeria. Biol. Control 129, 195–200. doi: 10.1016/j.biocontrol.2018.10.018
Bergsson, G., Arnfinnsson, J., Steingrimsson, O., and Thormar, H. (2001). In vitro killing of Candida albicans by fatty acids and monoglycerides. Antimicrob. Agents Chemother. 45, 3209–3212. doi: 10.1128/AAC.45.11.3209-3212.2001
Calvo, H., Mendiara, I., Arias, E., Gracia, A. P., Blanco, D., and Venturini, M. E. (2020). Antifungal activity of the volatile organic compounds produced by Bacillus velezensis strains against postharvest fungal pathogens. Postharvest Biol. Technol. 166, 111208. doi: 10.1016/j.postharvbio.2020.111208
Chaouachi, M., Marzouk, T., Jallouli, S., Elkahoui, S., Gentzbittel, L., Ben, C., et al. (2021). Activity assessment of tomato endophytic bacteria bioactive compounds for the postharvest biocontrol of Botrytis cinerea. Postharvest Biol. Technol. 172:111389. doi: 10.1016/j.postharvbio.2020.111389
Coconubo Guio, L. C., Sinuco León, D. C., and Castellanos Hernández, L. (2020). Fungicidal activity of volatile organic compounds from Paenibacillus bacteria against Colletotrichum gloeosporioides. Rev. Colomb. Quím. 49, 20–25. doi: 10.15446/rev.colomb.quim.v1n49.81996
Considine, M. J., and Foyer, C. H. (2015). Metabolic responses to sulfur dioxide in grapevine (Vitis vinifera L.): photosynthetic tissues and berries. Front. Plant Sci. 6:60. doi: 10.3389/fpls.2015.00060
Contarino, R., Brighina, S., Fallico, B., Cirvilleri, G., Parafati, L., and Restuccia, C. (2019). Volatile organic compounds (VOCs) produced by biocontrol yeasts. Food Microbiol. 82, 70–74. doi: 10.1016/j.fm.2019.01.008
Cordovez, V., Carrion, V. J., Etalo, D. W., Mumm, R., Zhu, H., van Wezel, G. P., et al. (2015). Diversity and functions of volatile organic compounds produced by Streptomyces from a disease-suppressive soil. Front. Microbiol. 6:1081. doi: 10.3389/fmicb.2015.01081
Dalilla, C. R., Mauricio, B. F., Simone, C. B., Silvia, B., and Sergio, F. P. (2015). Antimicrobial activity of volatile organic compounds and their effect on lipid peroxidation and electrolyte loss in Colletotrichum gloeosporioides and Colletotrichum acutatum mycelia. Afr. J. Microbiol. Res. 9, 1527–1535. doi: 10.5897/AJMR2015.7425
de Souza, M. L., Ribeiro, L. S., Miguel, M. G. D. P., Batista, L. R., Schwan, R. F., Medeiros, F. H., et al. (2021). Yeasts prevent ochratoxin A contamination in coffee by displacing Aspergillus carbonarius. Biol. Control 155:104512. doi: 10.1016/j.biocontrol.2020.104512
Dhouib, H., Zouari, I., Ben Abdallah, D., Belbahri, L., Taktak, W., Triki, M. A., et al. (2019). Potential of a novel endophytic Bacillus velezensis in tomato growth promotion and protection against Verticillium wilt disease. Biol. Control 139:104092. doi: 10.1016/j.biocontrol.2019.104092
Di Francesco, A., Di Foggia, M., and Baraldi, E. (2020a). Aureobasidium pullulans volatile organic compounds as alternative postharvest method to control brown rot of stone fruits. Food Microbiol. 87:103395. doi: 10.1016/j.fm.2019.103395
Di Francesco, A., Ugolini, L., Lazzeri, L., and Mari, M. (2015). Production of volatile organic compounds by Aureobasidium pullulans as a potential mechanism of action against postharvest fruit pathogens. Biol. Control 81, 8–14. doi: 10.1016/j.biocontrol.2014.10.004
Di Francesco, A., Zajc, J., Gunde-Cimerman, N., Aprea, E., Gasperi, F., Placi, N., et al. (2020b). Bioactivity of volatile organic compounds by Aureobasidium species against gray mold of tomato and table grape. World J. Microbiol. Biotechnol. 36, 1–11. doi: 10.1007/s11274-020-02947-7
Diaz, M. A., Pereyra, M. M., Picon-Montenegro, E., Meinhardt, F., and Dib, J. R. (2020). Killer yeasts for the biological control of postharvest fungal crop diseases. Microorganisms 8, 1680. doi: 10.3390/microorganisms8111680
Don, S. M. Y., Schmidtke, L. M., Gambetta, J. M., and Steel, C. C. J. R. I. M. (2020). Volatile organic compounds produced by Aureobasidium pullulans induce electrolyte loss and oxidative stress in Botrytis cinerea and Alternaria alternata. Res. Microbiol. 172:103788. doi: 10.1016/j.resmic.2020.10.003
Ebadzadsahrai, G., Higgins Keppler, E. A., Soby, S. D., and Bean, H. D. (2020). Inhibition of fungal growth and induction of a novel volatilome in response to chromobacterium vaccinii volatile organic compounds. Front. Microbiol. 11:1035. doi: 10.3389/fmicb.2020.01035
Farbo, M. G., Urgeghe, P. P., Fiori, S., Marcello, A., Balmas, V., Hassan, U. I. Z., et al. (2018). Effect of yeast volatile organic compounds on ochratoxin A-producing Aspergillus carbonarius and A. ochraceus. Int. J. Food Microbiol. 284, 1–10. doi: 10.1016/j.ijfoodmicro.2018.06.023
Fialho, M., Carvalho, G., Martins, P. F., Azevedo, R. A., and Pascholati, S. F. J. A. J. O. M. R. (2014). Antioxidative response of the fungal plant pathogen Guignardia citricarpa to antimicrobial volatile organic compounds. Afr. J. Microbiol. Res. 8, 2077–2084. doi: 10.5897/AJMR2014.6719
Fiori, S., Urgeghe, P. P., Hammami, W., Razzu, S., Jaoua, S., and Migheli, Q. (2014). Biocontrol activity of four non- and low-fermenting yeast strains against Aspergillus carbonarius and their ability to remove ochratoxin A from grape juice. Int. J. Food Microbiol. 189, 45–50. doi: 10.1016/j.ijfoodmicro.2014.07.020
Gómez, Á. G., Ramos, F. A., and Sinuco, D. C. (2021). Screening of volatile organic compounds from actinobacteria for the control of phytopathogen Colletotrichum gloeosporioides. Biocontrol Sci. Tech. 31, 1067–1079. doi: 10.1080/09583157.2021.1918635
Guo, H., Qin, X., Wu, Y., Yu, W., Liu, J., Xi, Y., et al. (2019). Biocontrol of gray mold of cherry tomatoes with the volatile organic monomer from Hanseniaspora uvarum, trans-Cinnamaldehyde. Food Bioprocess Technol. 12, 1809–1820. doi: 10.1007/s11947-019-02319-6
He, C. N., Ye, W. Q., Zhu, Y. Y., and Zhou, W. W. (2020). Antifungal activity of volatile organic compounds produced by Bacillus methylotrophicus and Bacillus thuringiensis against five common spoilage fungi on loquats. Molecules 25:3360. doi: 10.3390/molecules25153360
Huang, R., Che, H. J., Zhang, J., Yang, L., Jiang, D. H., and Li, G. Q. (2012). Evaluation of Sporidiobolus pararoseus strain YCXT3 as biocontrol agent of Botrytis cinerea on post-harvest strawberry fruits. Biol. Control 62, 53–63. doi: 10.1016/j.biocontrol.2012.02.010
Huang, R., Li, G. Q., Zhang, J., Yang, L., Che, H. J., Jiang, D. H., et al. (2011). Control of postharvest botrytis fruit rot of strawberry by volatile organic compounds of Candida intermedia. Phytopathology 101, 859–869. doi: 10.1094/PHYTO-09-10-0255
Hung, R., Lee, S., and Bennett, J. W. J. F. E. (2013). Arabidopsis thaliana as a model system for testing the effect of Trichoderma volatile organic compounds. Fungal Ecol. 6, 19–26. doi: 10.1016/j.funeco.2012.09.005
Intana, W., Kheawleng, S., and Sunpapao, A. (2021). Trichoderma asperellum T76-14 released volatile organic compounds against postharvest fruit rot in muskmelons (cucumis melo) caused by Fusarium incarnatum. J. Fungi 7:46. doi: 10.3390/jof7010046
Jaibangyang, S., Nasanit, R., and Limtong, S. (2020). Biological control of aflatoxin-producing Aspergillus flavus by volatile organic compound-producing antagonistic yeasts. BioControl 65:387. doi: 10.1007/s10526-020-10001-6
Jaibangyang, S., Nasanit, R., and Limtong, S. (2021). Effects of temperature and relative humidity on Aflatoxin B1 reduction in corn grains and antagonistic activities against Aflatoxin-producing Aspergillus flavus by a volatile organic compound-producing yeast, Kwoniella heveanensis DMKU-CE82. BioControl 66, 433–443. doi: 10.1007/s10526-021-10082-x
Kanchiswamy, C. N., Mainoy, M., and Maffei, M. E. (2015). Chemical diversity of microbial volatiles and their potential for plant growth and productivity. Front. Plant Sci. 6:151. doi: 10.3389/fpls.2015.00151
Kellerman, M., Joubert, J., Erasmus, A., and Fourie, P. H. (2016). The effect of temperature, exposure time and pH on imazalil residue loading and green mould control on citrus through dip application. Postharvest Biol. Technol. 121, 159–164. doi: 10.1016/j.postharvbio.2016.06.014
Lemos Junior, W. J. F., Binati, R. L., Felis, G. E., Slaghenaufi, D., Ugliano, M., and Torriani, S. (2020). Volatile organic compounds from Starmerella bacillaris to control gray mold on apples and modulate cider aroma profile. Food Microbiol. 89:103446. doi: 10.1016/j.fm.2020.103446
Leneveu-Jenvrin, C., Charles, F., Barba, F. J., and Remize, F. (2020). Role of biological control agents and physical treatments in maintaining the quality of fresh and minimally-processed fruit and vegetables. Crit. Rev. Food Sci. Nutr. 60, 2837–2855. doi: 10.1080/10408398.2019.1664979
Li, Q., Ning, P., Zheng, L., Huang, J., Li, G., and Hsiang, T. (2010). Fumigant activity of volatiles of Streptomyces globisporus JK-1 against Penicillium italicum on Citrus microcarpa. Postharvest Biol. Technol. 58, 157–165. doi: 10.1016/j.postharvbio.2010.06.003
Li, Q., Ning, P., Zheng, L., Huang, J., Li, G., and Hsiang, T. (2012). Effects of volatile substances of Streptomyces globisporus JK-1 on control of Botrytis cinerea on tomato fruit. Biol. Control 61, 113–120. doi: 10.1016/j.biocontrol.2011.10.014
Ling, L. J., Zhao, Y. H., Tu, Y. X., Yang, C. Y., Ma, W. X., Feng, S. L., et al. (2021). The inhibitory effect of volatile organic compounds produced by Bacillus subtilis CL2 on pathogenic fungi of wolfberry. J. Basic Microbiol. 61, 110–121. doi: 10.1002/jobm.202000522
Macias-Rubalcava, M. L., Sanchez-Fernandez, R. E., Roque-Flores, G., Lappe-Oliveras, P., and Medina-Romero, Y. M. (2018). Volatile organic compounds from Hypoxylon anthochroum endophytic strains as postharvest mycofumigation alternative for cherry tomatoes. Food Microbiol. 76, 363–373. doi: 10.1016/j.fm.2018.06.014
Mari, M., Bautista-Banos, S., and Sivakumar, D. (2016). Decay control in the postharvest system: role of microbial and plant volatile organic compounds. Postharvest Biol. Technol. 122, 70–81. doi: 10.1016/j.postharvbio.2016.04.014
Masoud, W., Poll, L., and Jakobsen, M. (2005). Influence of volatile compounds produced by yeasts predominant during processing of Coffea arabica in East Africa on growth and ochratoxin A (OTA) production by Aspergillus ochraceus. Yeast 22, 1133–1142. doi: 10.1002/yea.1304
Massawe, V. C., Hanif, A., Farzand, A., Mburu, D. K., Ochola, S. O., Wu, L., et al. (2018). Volatile compounds of Endophytic Bacillus spp. have biocontrol activity against Sclerotinia sclerotiorum. Phytopathology 108, 1373–1385. doi: 10.1094/PHYTO-04-18-0118-R
Morita, T., Tanaka, I., Ryuda, N., Ikari, M., Ueno, D., and Someya, T. (2019). Antifungal spectrum characterization and identification of strong volatile organic compounds produced by Bacillus pumilus TM-R. Heliyon 5:e01817. doi: 10.1016/j.heliyon.2019.e01817
Nagarathnamma, T., Chunchanur, S. K., Rudramurthy, S. M., Vineetha, K. R., Ramamurthy, K., Joseph, J., et al. (2017). Outbreak of Pichia kudriavzevii fungaemia in a neonatal intensive care unit. J. Med. Microbiol. 66, 1759–1764. doi: 10.1099/jmm.0.000645
Ocampo-Suarez, I. B., Lopez, Z., Calderon-Santoyo, M., Ragazzo-Sanchez, J. A., and Knauth, P. (2017). Are biological control agents, isolated from tropical fruits, harmless to potential consumers? Food Chem. Toxicol. 109, 1055–1062. doi: 10.1016/j.fct.2017.05.010
Papoutsis, K., Mathioudakis, M. M., Hasperué, J. H., and Ziogas, V. (2019). Non-chemical treatments for preventing the postharvest fungal rotting of citrus caused by Penicillium digitatum (green mold) and Penicillium italicum (blue mold). Trends Food Sci. Technol. 86, 479–491. doi: 10.1016/j.tifs.2019.02.053
Parafati, L., Vitale, A., Restuccia, C., and Cirvilleri, G. (2015). Biocontrol ability and action mechanism of food-isolated yeast strains against Botrytis cinerea causing post-harvest bunch rot of table grape. Food Microbiol. 47, 85–92. doi: 10.1016/j.fm.2014.11.013
Parafati, L., Vitale, A., Restuccia, C., and Cirvilleri, G. (2017). Performance evaluation of volatile organic compounds by antagonistic yeasts immobilized on hydrogel spheres against gray, green and blue postharvest decays. Food Microbiol. 63, 191–198. doi: 10.1016/j.fm.2016.11.021
Phoka, N., Suwannarach, N., Lumyong, S., Ito, S., Matsui, K., Arikit, S., et al. (2020). Role of volatiles from the endophytic fungus Trichoderma asperelloides PSU-P1 in biocontrol potential and in promoting the plant growth of Arabidopsis thaliana. J. Fungi 6, 341. doi: 10.3390/jof6040341
Rajaofera, M. J. N., Wang, Y., Dahar, G. Y., Jin, P., Fan, L., Xu, L., et al. (2019). Volatile organic compounds of Bacillus atrophaeus HAB-5 inhibit the growth of Colletotrichum gloeosporioides. Pestic. Biochem. Physiol. 156, 170–176. doi: 10.1016/j.pestbp.2019.02.019
Rojas-Solís, D., Zetter-Salmón, E., Contreras-Pérez, M., Rocha-Granados, M. D. C., Macías-Rodríguez, L., and Santoyo, G. (2018). Pseudomonas stutzeri E25 and Stenotrophomonas maltophilia CR71 endophytes produce antifungal volatile organic compounds and exhibit additive plant growth-promoting effects. Biocatal. Agric. Biotechnol. 13, 46–52. doi: 10.1016/j.bcab.2017.11.007
Ruiz-Moyano, S., Hernandez, A., Galvan, A. I., Cordoba, M. G., Casquete, R., Serradilla, M. J., et al. (2020). Selection and application of antifungal VOCs-producing yeasts as biocontrol agents of grey mould in fruits. Food Microbiol. 92:103556. doi: 10.1016/j.fm.2020.103556
Rybakova, D., Rack-Wetzlinger, U., Cernava, T., Schaefer, A., Schmuck, M., and Berg, G. (2017). Aerial warfare: a volatile dialogue between the plant pathogen Verticillium longisporum and its antagonist Paenibacillus polymyxa. Front. Plant Sci. 8:1294. doi: 10.3389/fpls.2017.01294
Song, X. Y., Wang, H., Ren, F., Wang, K., and Strobel, G. J. A. (2019). An endophytic diaporthe apiculatum produces monoterpenes with inhibitory activity against phytopathogenic fungi. Antibiotics 8, 231. doi: 10.3390/antibiotics8040231
Spadaro, D., and Droby, S. (2016). Development of biocontrol products for postharvest diseases of fruit: the importance of elucidating the mechanisms of action of yeast antagonists. Trends Food Sci. Technol. 47, 39–49. doi: 10.1016/j.tifs.2015.11.003
Stevens, S., and Hofmeyr, J. H. S. (1993). Effects of ethanol, octanoic and decanoic acids on fermentation and the passive influx of protons through the plasma-membrane of Saccharomyces-Cerevisiae. Appl. Microbiol. Biotechnol. 38, 656–663.
Sunpapao, A., Chairin, T., and Ito, S. (2018). The biocontrol by Streptomyces and Trichoderma of leaf spot disease caused by Curvularia oryzae in oil palm seedlings. Biol. Control 123, 36–42. doi: 10.1016/j.biocontrol.2018.04.017
Tahir, H. A., Gu, Q., Wu, H., Niu, Y., Huo, R., and Gao, X. (2017). Bacillus volatiles adversely affect the physiology and ultra-structure of Ralstonia solanacearum and induce systemic resistance in tobacco against bacterial wilt. Sci. Rep. 7:40481. doi: 10.1038/srep40481
Tilocca, B., Balmas, V., Hassan, Z. U., Jaoua, S., and Migheli, Q. (2019). A proteomic investigation of Aspergillus carbonarius exposed to yeast volatilome or to its major component 2-phenylethanol reveals major shifts in fungal metabolism. Int. J. Food Microbiol. 306:108265. doi: 10.1016/j.ijfoodmicro.2019.108265
Tilocca, B., Cao, A., and Migheli, Q. (2020). Scent of a killer: microbial volatilome and its role in the biological control of plant pathogens. Front. Microbiol. 11:41. doi: 10.3389/fmicb.2020.00041
Vazquez, J., Grillitsch, K., Daum, G., Mas, A., Beltran, G., and Torija, M. J. (2019). The role of the membrane lipid composition in the oxidative stress tolerance of different wine yeasts. Food Microbiol. 78, 143–154. doi: 10.1016/j.fm.2018.10.001
Wang, E., Liu, X., Si, Z., Li, X., Bi, J., Dong, W., et al. (2021a). Volatile organic compounds from rice Rhizosphere bacteria inhibit growth of the pathogen Rhizoctonia solani. Agriculture 11, 368. doi: 10.3390/agriculture11040368
Wang, Z., Mei, X., Du, M., Chen, K., Jiang, M., Wang, K., et al. (2020). Potential modes of action of Pseudomonas fluorescens ZX during biocontrol of blue mold decay on postharvest citrus. J. Sci. Food Agric. 100, 744–754. doi: 10.1002/jsfa.10079
Wang, K., Qin, Z., Wu, S., Zhao, P., Zhen, C., and Gao, H. (2021b). Antifungal mechanism of volatile organic compounds produced by Bacillus subtilis CF-3 on Colletotrichum gloeosporioides assessed using omics technology. J. Agric. Food Chem. 69, 5267–5278. doi: 10.1021/acs.jafc.1c00640
Wang, C., Wang, Z., Qiao, X., Li, Z., Li, F., Chen, M., et al. (2013). Antifungal activity of volatile organic compounds from Streptomyces alboflavus TD-1. FEMS Microbiol. Lett. 341, 45–51. doi: 10.1111/1574-6968.12088
Wang, Z. R., Zhong, T., Chen, K. W., Du, M. Y., Chen, G. J., Chen, X. H., et al. (2021c). Antifungal activity of volatile organic compounds produced by Pseudomonas fluorescens ZX and potential biocontrol of blue mold decay on postharvest citrus. Food Control 120:107499. doi: 10.1016/j.foodcont.2020.107499
Wilson, C. L., and Chalutz, E. (1989). Postharvest biological-control of Penicillium rots of citrus with antagonistic yeasts and bacteria. Sci. Hortic. 40, 105–112. doi: 10.1016/0304-4238(89)90092-7
Xie, S., Liu, J., Gu, S., Chen, X., Jiang, H., and Ding, T. (2020). Antifungal activity of volatile compounds produced by endophytic Bacillus subtilis DZSY21 against Curvularia lunata. Ann. Microbiol. 70:2. doi: 10.1186/s13213-020-01553-0
Yalage Don, S. M., Schmidtke, L. M., Gambetta, J. M., and Steel, C. C. (2020). Aureobasidium pullulans volatilome identified by a novel, quantitative approach employing SPME-GC-MS, suppressed Botrytis cinerea and Alternaria alternata in vitro. Sci. Rep. 10, 4498. doi: 10.1038/s41598-020-61471-8
Yalage Don, S. M., Schmidtke, L. M., Gambetta, J. M., and Steel, C. C. (2021). Volatile organic compounds produced by Aureobasidium pullulans induce electrolyte loss and oxidative stress in Botrytis cinerea and Alternaria alternata. Res. Microbiol. 172:103788. doi: 10.1016/j.resmic.2020.10.003
Ye, X., Chen, Y., Ma, S., Yuan, S., Wu, Y., Li, Y., et al. (2020). Biocidal effects of volatile organic compounds produced by the myxobacterium Corrallococcus sp. EGB against fungal phytopathogens. Food microbiol 91:103502. doi: 10.1016/j.fm.2020.103502
Zhang, H., Du, H., and Xu, Y. (2021). Volatile organic compounds mediated antifungal activity of Pichia and its effect on the metabolic profiles of fermentation communities. Appl. Environ. Microbiol. 87, e02992–e02920. doi: 10.1128/AEM.02992-20
Zhang, H., Godana, E. A., Sui, Y., Yang, Q., Zhang, X., and Zhao, L. (2020). Biological control as an alternative to synthetic fungicides for the management of grey and blue mould diseases of table grapes: a review. Crit. Rev. Microbiol. 46, 450–462. doi: 10.1080/1040841X.2020.1794793
Zheng, M., Shi, J. Y., Shi, J., Wang, Q. G., and Li, Y. H. (2013). Antimicrobial effects of volatiles produced by two antagonistic Bacillus strains on the anthracnose pathogen in postharvest mangos. Biol. Control 65, 200–206. doi: 10.1016/j.biocontrol.2013.02.004
Zhou, M., Li, P., Wu, S., Zhao, P., and Gao, H. (2019). Bacillus subtilis CF-3 volatile organic compounds inhibit monilinia fructicola growth in peach fruit. Front. Microbiol. 10:1804. doi: 10.3389/fmicb.2019.01804
Keywords: biological control, volatile organic compounds, post-harvest diseases, antifungal mechanism, commercial application
Citation: Zhao X, Zhou J, Tian R and Liu Y (2022) Microbial volatile organic compounds: Antifungal mechanisms, applications, and challenges. Front. Microbiol. 13:922450. doi: 10.3389/fmicb.2022.922450
Received: 18 April 2022; Accepted: 27 June 2022;
Published: 15 July 2022.
Edited by:
Joseph D. Eifert, Virginia Tech, United StatesReviewed by:
Randy Ortiz-Castro, National Council of Science and Technology (CONACYT), MexicoCopyright © 2022 Zhao, Zhou, Tian and Liu. This is an open-access article distributed under the terms of the Creative Commons Attribution License (CC BY). The use, distribution or reproduction in other forums is permitted, provided the original author(s) and the copyright owner(s) are credited and that the original publication in this journal is cited, in accordance with accepted academic practice. No use, distribution or reproduction is permitted which does not comply with these terms.
*Correspondence: Yanlin Liu, eWFubGlubGl1QG53c3VhZi5lZHUuY24=
Disclaimer: All claims expressed in this article are solely those of the authors and do not necessarily represent those of their affiliated organizations, or those of the publisher, the editors and the reviewers. Any product that may be evaluated in this article or claim that may be made by its manufacturer is not guaranteed or endorsed by the publisher.
Research integrity at Frontiers
Learn more about the work of our research integrity team to safeguard the quality of each article we publish.