- 1Department of Plant Protection, Faculty of Agriculture, University of Kurdistan, Sanandaj, Iran
- 2Department of Plant Production and Genetics, Faculty of Agriculture, University of Kurdistan, Sanandaj, Iran
- 3Department of Medical Physiology and Pharmacology, School of Medicine, Kurdistan University of Medical Sciences, Sanandaj, Iran
The volatile organic compounds (VOCs) produced by endophytic bacteria have a significant role in the control of phytopathogens. In this research, the VOCs produced by the endophytic bacteria Streptomyces sp. B86, Pantoea sp. Dez632, Pseudomonas sp. Bt851, and Stenotrophomonas sp. Sh622 isolated from healthy sugar beet (Beta vulgaris) and sea beet (Beta maritima) were evaluated for their effects on the virulence traits of Bacillus pumilus Isf19, the causal agent of harvested sugar beet root rot disease. The gas chromatographymass spectrometry (GC-MS) analysis revealed that B86, Dez632, Bt851, and Sh622 produced 15, 28, 30, and 20 VOCs, respectively, with high quality. All antagonistic endophytic bacteria produced VOCs that significantly reduced soft root symptoms and inhibited the growth of B. pumilus Isf19 at different levels. The VOCs produced by endophytic bacteria significantly reduced swarming, swimming, and twitching motility by B. pumilus Isf19, which are important to pathogenicity. Our results revealed that VOCs produced by Sh622 and Bt851 significantly reduced attachment of B. pumilus Isf19 cells to sugar beetroots, and also all endophytic bacteria tested significantly reduced chemotaxis motility of the pathogen toward root extract. The VOCs produced by Dez632 and Bt851 significantly upregulated the expression levels of defense genes related to soft rot resistance. Induction of PR1 and NBS-LRR2 genes in sugar beetroot slices suggests the involvement of SA and JA pathways, respectively, in the induction of resistance against pathogen attack. Based on our results, the antibacterial VOCs produced by endophytic bacteria investigated in this study can reduce soft rot incidence.
Introduction
Sugar beet (Beta vulgaris L.) is one of the most crops for sugar production all over the world (Trebbi and McGrath, 2004). The amount of sugar beet production exceeds 275 million tons per year worldwide and Iran with a production of approximately 5 × 106 tons per year is in the top twenty sugar beet producing countries (Food and Agriculture Organization [FAO], 2020). The sugar beet can be infected by various bacteria both in the field and in storage. Bacterial root rot disease causes serious losses in sugar beet yield. Usually, sugar beet is subjected to storage providing ideal conditions for microbial growth. Therefore, healthy harvested sugar beet can display rot symptoms during the storage period (Liebe and Varrelmann, 2016). Our storage observations and isolations from the most important sugar beet production areas in Iran revealed that several bacteria might play important roles in causing bacterial root rot. Symptoms include dark-brown to black lesions on taproot. Lesions may be superficially restricted to dark areas on the root. In the advanced stage, the nearly entire root may rot and produce viscous slime. Based on partial nucleotide sequencing of the 16S rRNA gene, one isolate with high sugar beet root rot activity was identified as Bacillus pumilus Isf19.
B. pumilus is a gram-positive, rod-shaped bacterial species found in healthy plant tissues that promote plant growth by enhancing nutrient uptake, atmospheric nitrogen fixation, reducing metal toxicity, and producing antimicrobial substances against various plant pathogenic microorganisms (Yuan and Gao, 2015). Some B. pumilus strains have been previously reported as plant pathogens, including the causal agent of leaf blight of mango trees in Egypt (Galal et al., 2006), the ginger rhizome rot pathogen in China (Peng et al., 2013), Bacillus sp., identified as a possible B. pumilus strain, bacteria associated with leaf and twig dieback of Asian pear trees in China (Li et al., 2009), and the causative agent of Persian oak decline in Iran (Ahmadi et al., 2019).
Necrotrophic bacteria use different strategies employing the production of plant cell wall-degrading enzymes and necrosis-inducing proteins to kill plant tissues (Davidsson et al., 2013). Several plant cell wall-degrading enzymes, including xylanase (Nagar et al., 2012; Subramaniyan, 2012), pectate lyase (Basu et al., 2009), and β-1,4-endoglucanase (Lima et al., 2005), have been isolated from B. pumilus strains that may be related to the mechanisms of pathogenicity. Whole-genome analysis of a ginger rot pathogen B. pumilus GR8 revealed that numerous plant cell wall-degrading enzymes and several proteins involved in the interaction between bacterial pathogen and plant are encoded by the genome of this pathogen (Yuan and Gao, 2015).
Plants are usually associated with different microorganisms; among them, endophytic bacteria that colonize the same ecological niche in plants as plant pathogens might have the potential to suppress the virulence of pathogenic microorganisms (Compant et al., 2005). In sugar beet, the diversity and ecology of the endophytic bacteria have been investigated. Research on endophytic bacteria of sugar beet has included the identification of phyla Proteobacteria, Actinobacteria, and Firmicutes (Wolfgang et al., 2020). Bacterial isolates, such as Pseudomonas sp., Pantoea agglomerans, Microbacterium testaceum, and Subtercola pratensis, have been identified as demonstrating antagonistic potential against various fungal plant pathogens under in vitro conditions (Zachow et al., 2008).
Bacteria have been reported to emit various volatile compounds with significant biological activities on a broad range of plant pathogens (Garbeva and Weisskopf, 2020). In general, volatile compounds are defined as small molecules (<300 Da) of low boiling point with high vapor pressure. These molecules can spread easily between roots and microbes even at distances (Zarraonaindia et al., 2015). The chemical structures of bacterial volatiles are very diverse so that in different groups like small aliphatic, aromatic molecules to large molecules are observed (Schulz and Dickschat, 2007). A few investigations have been reported regarding the antibacterial activity of these volatile compounds (Rajer et al., 2017; Xie et al., 2018). Pseudomonas fluorescens B-4117 and Serratia plymuthica IC1270 produced VOCs that reduced the growth of Agrobacterium tumefaciens and Agrobacterium vitis (Dandurishvili et al., 2011). Volatile organic compounds released by Bacillus amyloliquefaciens SQR-9 and Pseudomonas fluorescens WR-1 can inhibit the growth and virulence traits of Ralstonia solanacearum (Raza et al., 2016a,b). VOCs produced by Bacillus strains caused morphological abnormalities in R. solanacearum cells (Tahir et al., 2017).
Plants generally enhance local or systemic resistance to pathogens or biotic stress. These responses are generally mediated by jasmonic acid (JA) and salicylic acid (SA) pathways which lead to the enhancement of plant defense genes, such as the nucleotide-binding site–leucine-rich repeat (NBS-LRR2) or pathogenesis-related (PR) genes, respectively (Aswani et al., 2022). NBS-LRR gene-mediated disease resistance is among the most important plant defense mechanisms against pathogens (Marone et al., 2013). A previous study revealed that sugar beetroots treated with jasmonic acid have improved resistance to the storage pathogens like Botrytis cinerea, Penicillium claviforme, and Phoma betae (Fugate et al., 2012). Jasmonic acid induces defense responses and upregulated defense genes, including NBS-LRR family, in harvested sugar beetroots (Fugate et al., 2017). Unfortunately, to the best of our knowledge, the roles of NBS-LRR2 gene related to sugar beet soft rot disease resistance have not been investigated. PR1 gene expression is induced in response to a variety of pathogens. Some PR proteins with chitinase and β-1,3-glucanase activity have been identified in sugar beet as a defense reaction to Cercospora beticola (Weltmeier et al., 2011). However, little is known about the impact of B. pumilus as a postharvest root rot agent on the expression of PR genes. Endophytic bacteria can protect their plant hosts against a wide range of pathogens through triggering defense reactions (Pieterse et al., 2012). Previous studies have shown that some endophytic bacteria activate plant defense reactions against biotrophic and necrotrophic plant pathogens. In some pathosystems, induction of defense reactions was mediated by the upregulation of PR genes (Asghari et al., 2020; Portieles et al., 2021).
To the best of our knowledge, there is no research about the effect of VOCs produced by endophytic bacteria on the virulence traits of B. pumilus. Therefore, the objectives of this study were to evaluate the effects of VOCs produced by endophytic bacteria as biocontrol agents on the growth rate, and virulence traits, such as motility, chemotaxis, attachment, and biofilm formation of sugar beet soft rot pathogen B. pumilus. The major VOCs produced by endophytic bacteria against B. pumilus were identified using gas chromatography–mass spectrophotometry (GC-MS). Also, the activity of selected endophytic bacteria to induce defense responses in sugar beet roots against the bacterial pathogen was investigated.
Materials and Methods
Bacterial Strains and Growth Conditions
Bacillus pumilus Isf19 (GenBank Acc. No MZ647525) which exhibited soft rot disease in harvested sugar beetroot, as well as the endophytic bacteria Streptomyces sp. B86 (GenBank Acc. No. MZ647528), Pantoea sp. Dez632 (GenBank Acc. No. MZ647535), Pseudomonas sp. Bt851 (GenBank Acc. No. MZ647537), and Stenotrophomonas sp. Sh622 (GenBank Acc. No. MZ647534) isolated from sugar beet and sea beet plants provided by our laboratory were used in this study. These strains were routinely grown on nutrient agar (NA) medium and stored at 4–6°C as a working stock. All strains were grown in nutrient broth (NB) medium for 24 h at 26–28°C with shaking, and sterile glycerol was added to the final concentration of 20% and then stored at −20°C for long-term storage.
Effect of Volatile Organic Compounds on Soft Rot Development by Bacterial Pathogen
The healthy sugar beetroots were immersed in 0.5% sodium hypochlorite solution for 3 min, rinsed in sterile-distilled water, and allowed to air-dry under sterile conditions. All exterior parts of the root were removed, and then root slices of about 0.5-cm thick and 3–4 cm in diameter were placed in one compartment of divided plates. A hole (5-mm depth) was made in the center of the slice, and 40 μl of the freshly prepared bacterial pathogen suspension (density of about 1 × 108 CFU ml–1) was added. In the other compartment, 40 μl of each endophytic bacteria with a concentration of about 1 × 1011 CFU ml–1 was streaked on the NA medium. The plates were sealed with parafilm and incubated at 30–32°C, and the diameter of soft rotted area was measured for up to 7 days (Strausbaugh and Gillen, 2008). Root slices inoculated with sterile water and only pathogen were used as negative and positive controls, respectively.
Antibacterial Activity of Volatile Organic Compounds Produced by Endophytic Bacteria
The antibacterial activity of VOCs produced by endophytic bacteria against bacterial pathogen was assessed on NA medium using a dual-culture technique. The 24 h growth of the endophytic bacteria (adjusted to the concentration of about 1 × 1011 CFU ml–1) was cultured on one side of the plate, while the opposite side was spot inoculated with 5 μl of the pathogen (1 × 108CFU ml–1). In the control, the pathogen was cultured alone. The plates were then sealed with parafilm and maintained at 28°C for 7 days. The colony numbers of the B. pumilus Isf19 were calculated (Tahir et al., 2017). Three replications were performed for each treatment.
Effect of Volatile Organic Compounds on Swarming, Swimming, and Twitching Motility Behaviors of Bacterial Pathogen
The various motility behaviors of the bacterial pathogen cells exposed to VOCs of endophytic bacteria were assessed using a divided Petri plate. Two microliters of the freshly prepared bacterial pathogen (which was adjusted to the concentration of about 1 × 108CFU ml–1) was spotted on one compartment of the divided plates containing LB medium plus agar (0.3, 0.7, and 1.6%) for swimming, swarming, and twitching motility, respectively. In the other compartment, 20 μl of the endophytic bacterial suspension (concentration of about 1 × 1011 CFU ml–1) was cultured on the NA medium. The plates were incubated at 26–28°C, and the colony diameter of swarming and swimming motility was measured each 12-h interval. The halo diameter of twitching motility was examined after 72 h. Notably, the experiments were done in three replications (Tahir et al., 2017).
Chemotaxis Assay
The overnight growth of the endophytic bacteria was streaked onto one compartment of divided plates containing NA medium. In the other compartment, chemotaxis buffer medium (0.1 mM EDTA, 10 mM K2HPO4, 0.05% glycerol, 5 mM lactic acid, 0.14 μM CaCl2, 0.3 mM (NH4)2SO4, 0.35% agar, and pH 7.2) was prepared (Ordal and Gibson, 1977). Thereafter, 10 mm of the medium was removed and then refilled with 100 μl of root extract of sugar beet (var. Ekbatan). Then, 5 μl of bacterial pathogen cells was spot inoculated at a distance of 5 mm from the hole. The plates were sealed with parafilm and incubated at 28°C. The movement of the bacterial pathogen cells toward the root extract was counted as the CFU ml–1 of the cell. For the preparation of root extract, 2 g of sugar beet roots was homogenized into 20 ml of sterile 0.1 M phosphate buffer, transferred to a sterile 50-ml falcon tube, and incubated for 24 h. Samples were then filtered using a 0.22 μm Millipore membrane filter and stored at −20°C. This experiment was performed in three replicates.
Biofilm Formation Assay
The biofilm formation property of bacterial pathogen cells exposed to VOCs was investigated in polypropylene tubes. Accordingly, twenty microliters of the freshly prepared culture of endophytic bacterial strains (about 1 × 1011CFU ml–1) was cultured onto one compartment of the divided plates containing NA medium. In the other compartment, a microtube containing 150 μl of LB liquid medium plus 1% glucose that was inoculated with 50 μl of the bacterial pathogen (1 × 108 CFU ml–1) was placed vertically. The plates were then sealed with parafilm and maintained at 26–28°C. After 48–72 h, bacterial cells were discarded and 150 μl of 1% crystal violet solution was added to each tube and kept at room temperature for 15 min. The microtubes were then washed twice with sterile water. Subsequently, 2 × 200 μl of 96% ethanol was added to each microtube, the resulting volume was brought to 1 ml with sterile-distilled water, and the absorbance was measured at 540 nm with a spectrophotometer (SPECORD 210, Analytik Jena, Germany). Non-exposed bacterial pathogen cells to VOCs were used as a control. The experiment was conducted in a completely randomized design with three replications (O’Toole and Kolter, 1998).
Sugar Beet Root Attachment Assay
The attachment of bacterial pathogen cells to the root of sugar beet plantlets was assayed after exposure to VOCs produced by endophytic bacteria for 72 h. The roots of forty-day-old sugar beet plantlets (var. Ekbatan) were submerged in 10 ml treated or untreated bacterial pathogen cell suspensions (adjusted to about 1 × 108CFU ml–1) at room temperature and after 3 h rinsed three times with sterile-distilled water. Then, 3–5 mm from the root tips were separated, weighed, and placed individually in 1 ml of sterile-distilled water. After stirring for 5 s, the roots were macerated in 100 μl of sterile water. The obtained suspension was streaked onto NA medium and incubated at 28°C for 48 h, and the CFU ml–1 was counted. The experiment was conducted with three replications.
Effect of Volatile Organic Compounds on Cell Morphology
Scanning electron microscopy (SEM) was used to observe external morphological abnormalities of the bacterial pathogen cells. Bacterial cells with or without exposure to the VOCs of endophytic bacteria for 7 days at 30–32°C were collected into Eppendorf tubes and washed twice with 0.1 M phosphate buffer saline (PBS, pH:7.2). Afterward, the cells were spread onto the aluminum foil placed on the clean slide. After fixation in 2.5% glutaraldehyde solution for 1 h at room temperature, the samples were washed three times with PBS. Serial dehydration was done in ethanol solutions of 35, 50, 75, 90, and 100%, for 5 min, each time followed by 100% ethanol for 1 h. The samples were then conducted by the freeze-drying process at −70°C for 3 h. Finally, the samples were coated with gold, and electron micrographs were taken using a Philips XL30 SEM system (Philips SEM, Netherland).
Gene Expression Analysis of Inoculated Roots
The expression of the PR1 and NBS-LRR2 genes as a marker of salicylic acid (SA) and jasmonic acid (JA) pathways, respectively, was measured by qRT-PCR in the root slices of sugar beet. Treated roots with Dez632 and Bt851 that showed a higher reduction in soft rot development, also roots inoculated with Dez632/Isf19, Bt851/Isf19, and untreated roots (control) were collected after 0, 2, and 7 days, placed in aluminum foil, and stored in a sterile microtube at −40°C until used. For extraction of total RNA, 0.5 g of frozen powdered tissues was macerated into 1 ml of extraction buffer (per TE buffer pH:8.0 of 13 ml saturated phenol, 0.32 M sodium acetate, 0.01 M EDTA, 1% SDS, 1% PVP, and 3% CTAB). The suspension was centrifuged (8,000 rpm, 5 min, 4°C), and the resulting supernatant was transferred into a new tube. The supernatant was mixed with an equal volume of phenol/chloroform/isoamyl alcohol (25/24/1) and centrifuged, and RNA was precipitated with 0.1 volume 3M sodium acetate (pH:5.0) and an equal volume of isopropanol overnight at −20°C. Finally, the suspension was centrifuged at 13,500 rpm for 15 min, and RNA was washed with 70% ethanol, dried, and dissolved in 50 μl of RNase-free-DEPC water. The concentration and purity of RNA were determined using NanoDrop (Thermo Fisher Scientific, United States). DNase treatment was performed using the RNase-free DNase I (Yekta Tajhiz Azma, Iran).
cDNA was amplified from 200 ng of total RNA using the cDNA synthesis Kit (Parstous, Iran) according to the manufacturer’s instructions. Real-time PCR (RT-PCR) was performed with RealQ Plus 2x Master Mix Green (Ampliqon, Denmark) using an AB Applied Biosystem StepOne thermal cycler. PCR reactions were carried out in a 10 μl final volume containing 2x Master Mix Green High ROX, 5 p.m. forward and reverse primers (Table 1), and cDNA. Cycling conditions were 10 min at 95°C, followed by 40 two-step cycles of 15 s at 95°C and 1 min at 60°C. β-actin gene was used as a reference gene for normalization. The specificity of each primer was checked using the method previously described (Schmittgen and Livak, 2008). Relative gene expression was calculated with the following formula (Pfaffl, 2001):
The Ct values were the means of three biological replications and three technical replications.
Identification of Volatile Organic Compounds Produced by Endophytic Bacteria Using Gas Chromatographymass Spectrometry Analysis
To collect the VOCs produced by each endophytic bacteria, a three-compartment plate was used. One compartment, containing NA medium, was streaked with 20 μl (1 × 1011 CFU ml–1) overnight growth of each endophytic bacteria, a second compartment, containing NA medium, was spot inoculated with 5 μl (1 × 108 CFU ml–1) of B. pumilus Isf19, and the third compartment was filled with 0.3 g of sterile activated charcoal to adsorb the VOCs. The same experimental design, including endophytic bacteria and bacterial pathogen without activated charcoal, and also bacterial pathogen alone was used as control. The plates were sealed with parafilm and incubated at 28–30°C for 72 h. The activated charcoal traps were transferred into glass vials, and ethyl acetate (1: 1.25 W/V) was added. The adsorbed VOCs were extracted by shaking for 15 min, followed by centrifugation (5,000 rpm, 15 min), and the supernatants were analyzed by a gas chromatography device connected to a mass spectrometer (Agilent 7890B GC System/5977A MSD, Agilent Technologies, United States).
One microliter of the sample was injected into HP-5 ms column, and the initial column temperature was 50°C, which was increased to 240°C at a rate of 5°C min–1, held for 5 min. The mass spectrometer was operated in the electron ionization mode at 70 eV, with continuous scanning from 50 to 550 m/z. Helium carrier gas with a purity of 99.999%, a 34 psi pressure, and a flow rate of 1 ml min–1 was used. The compounds were identified by comparing their mass spectra with the databases of the device, including the National Institutes of Standards and Technology (NIST) and Wiley databases.
Statistical Analysis
All experiments were conducted in a completely randomized design. To evaluate the significance of the treatments, the data from each experiment were analyzed using analysis of variance (ANOVA), followed by the least-significant difference (LSD) test (P = 0.05), employing SAS ver. 9.1 statistical software (SAS, 2009). Graphs were plotted using the Excel program.
Results
Effect of Volatile Organic Compounds on Soft Rot Disease Development and Their Antibacterial Activity
The results showed that significant differences existed between treatments in the reduction in soft rot development (F = 79.03; P < 0.0001) compared to the control (Table 2). All endophytic bacteria significantly reduced the soft rot symptoms produced by B. pumilus Isf19 at varying levels. VOCs produced by strains Bt851 and Dez632 decreased the symptom development to about 38 and 35%, respectively, followed by Sh622 and B86 with a 15% reduction effect (Figure 1A).

Table 2. Analysis of variance (ANOVA) of swimming, swarming, twitching motility, biofilm production, cell population, chemotaxis, attachment, and soft rot development by B. pumilus Isf19 under the effect of VOCs produced by endophytic bacteria.
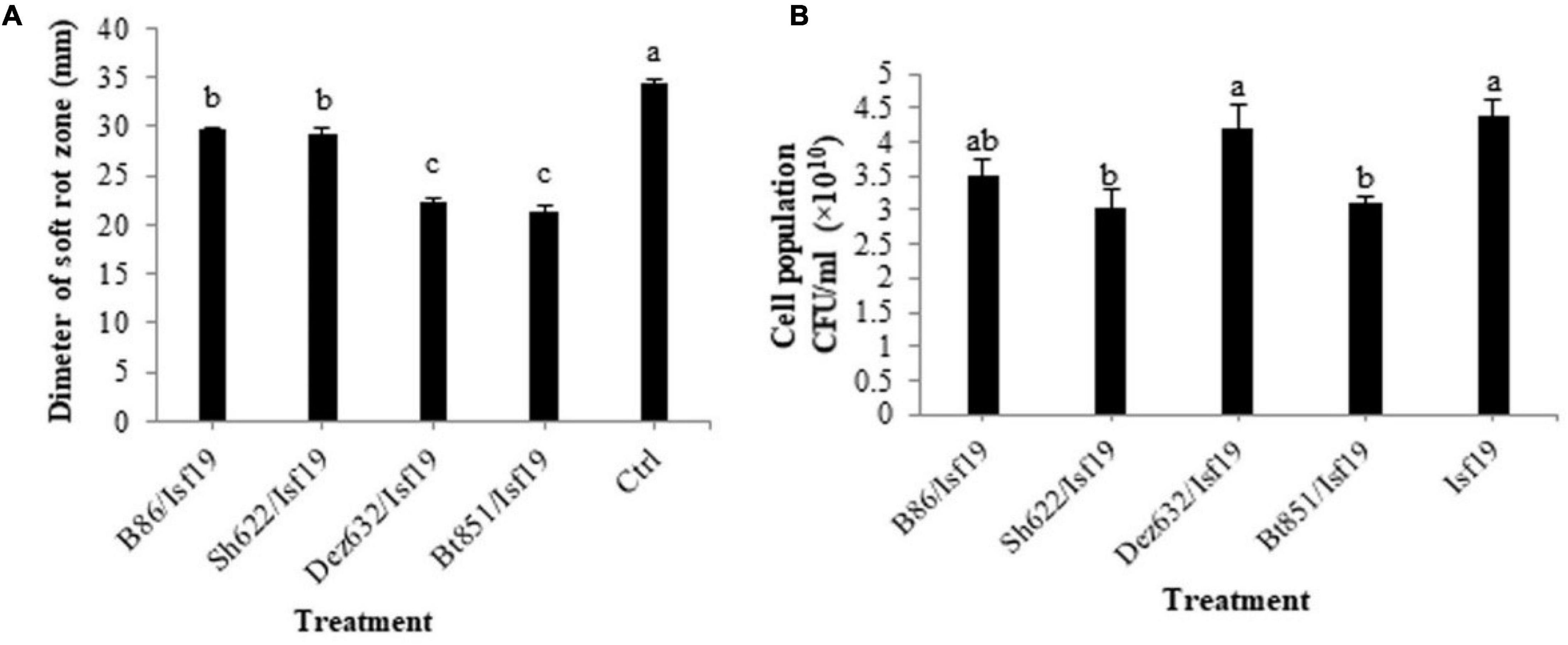
Figure 1. Effects of VOCs produced by Streptomyces sp. B86, Pantoea sp. Dez632, Pseudomonas sp. Bt851, and Stenotrophomonas sp. Sh622 on (A) soft rot development by B. pumilus Isf19 in sugar beetroot slices compared to the non-treated control (Ctrl), and (B) cell population of B. pumilus Isf19. Three replicates were used for each treatment. Error bars indicate the SE of the three replicates. Different letters indicate significant differences (P = 0.05).
According to statistical analysis, significant differences existed between treatments in the reduction in the cell population of Bacillus sp. Isf19 (F = 3.85; P = 0.0304) exposed to VOCs produced by endophytic bacteria compared to the non-exposed control (Table 2). VOCs of Sh622 and Bt851 strains lead to significant cell population inhibition of B. pumilus Isf19 to about 30%, followed by B86 with a 21% reduction effect (Figure 1B).
Effect of Volatile Organic Compounds on the Motility Behaviors of B. pumilus Isf19
According to statistical analysis, significant differences existed between treatments in the swarming (F = 32.28; P < 0.0001), swimming (F = 359.67; P < 0.0001), and twitching (F = 18.00; P = 0.0001) assays (Table 2). Our finding revealed that the swarming motility of B. pumilus Isf19 was significantly inhibited during 72 h exposure to VOCs of endophytic bacterial strains. As illustrated in Figures 2A,B, strains Sh622, B86, and Bt851, with a mean of 25.5, 26.5, and 27.5 mm, respectively, showed the highest inhibition effects of B. pumilus Isf19 cells followed by Dez632 with a mean of 35 mm, as compared to the control with the mean of 53 mm.
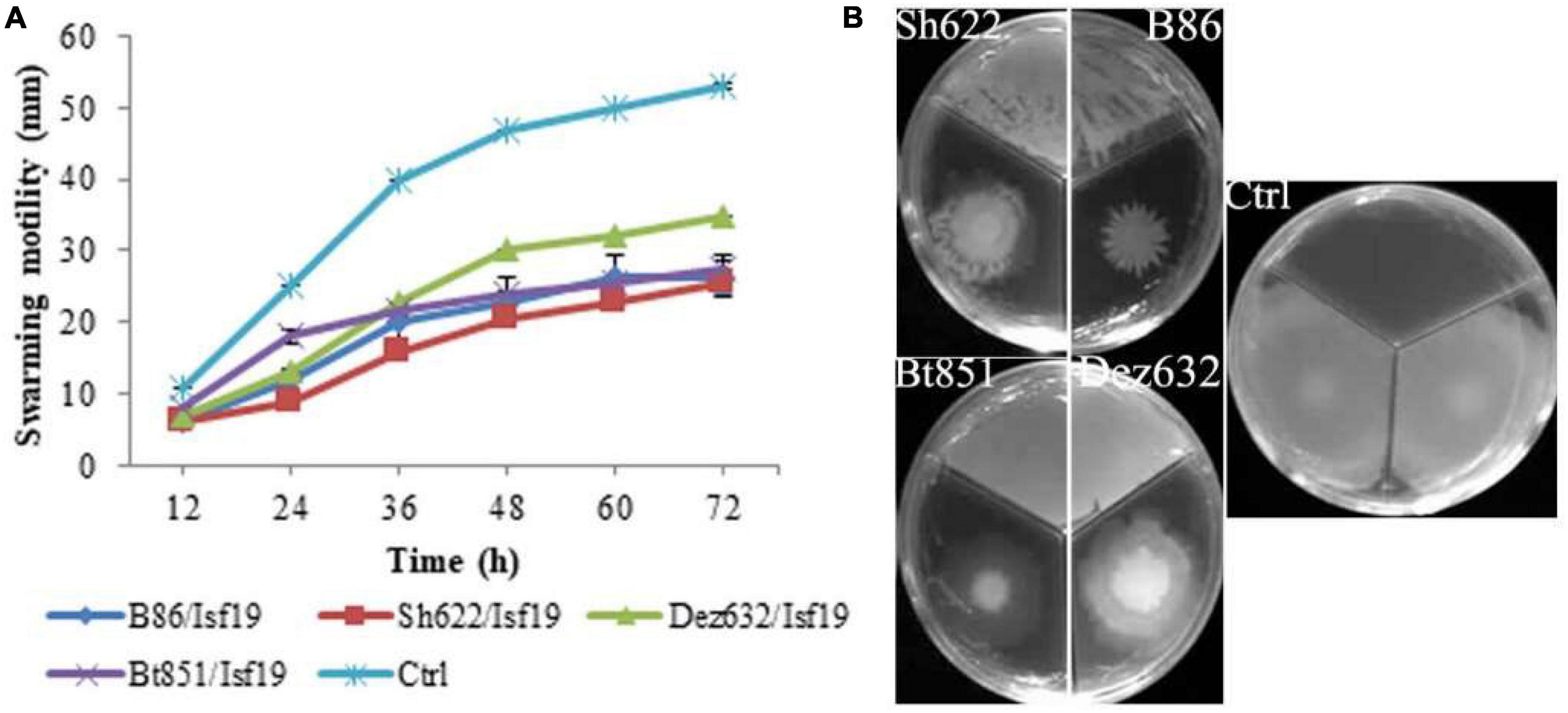
Figure 2. Effects of VOCs produced by Streptomyces sp. B86, Pantoea sp. Dez632, Pseudomonas sp. Bt851, and Stenotrophomonas sp. Sh622 on swarming motility of B. pumilus Isf19 compared to the non-treated control (Ctrl). The diameter of motility zone (A) and representative plate of swarming motility assay (B) were shown. Three replicates were used for each treatment. Error bars indicate the SE of the three replicates. Different letters indicate significant differences (P = 0.05).
The VOCs produced by Dez632 and Sh622 inhibited swimming motility of B. pumilus Isf19 to 12 and 18.5 mm, respectively, as compared to control with 32 mm and had the highest negative effect after 72 h (Figures 3A,B). Similarly, twitching motility was significantly reduced to 7 mm by B86, Sh622, and Bt851 compared to control with 8 mm (Figure 4A). Microscopic examination of the twitching motility exhibited that the circumferential colony edge of B. pumilus Isf19 in the non-exposed control was significantly wider than those exposed with the Dez632 and Bt851 volatiles (Figure 4B).
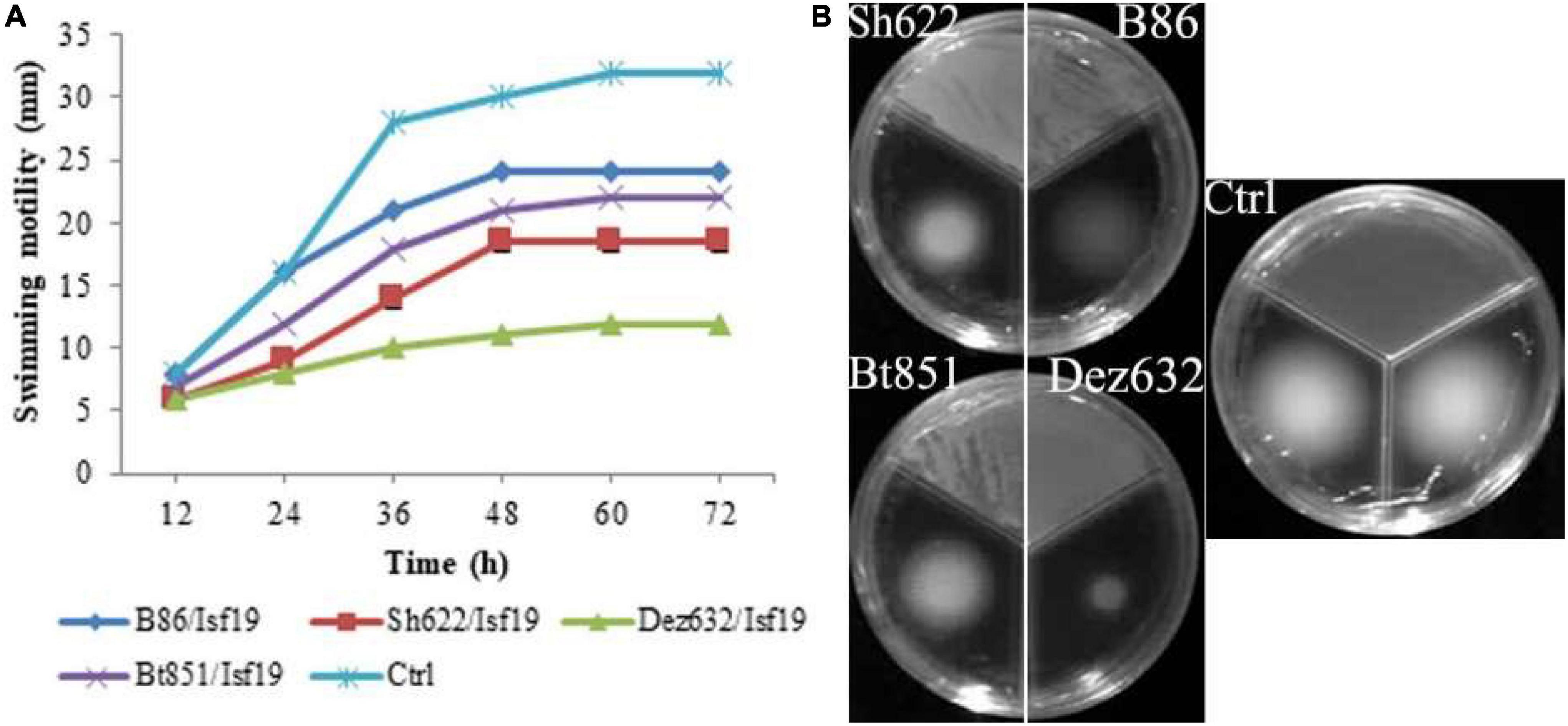
Figure 3. Effects of VOCs produced by Streptomyces sp. B86, Pantoea sp. Dez632, Pseudomonas sp. Bt851, and Stenotrophomonas sp. Sh622 on swimming motility of B. pumilus Isf19 compared to the non-treated control (Ctrl). The diameter of motility zone (A) and representative plate of swarming motility assay (B) were shown. Three replicates were used for each treatment. Error bars indicate the SE of the three replicates. Different letters indicate significant differences (P = 0.05).
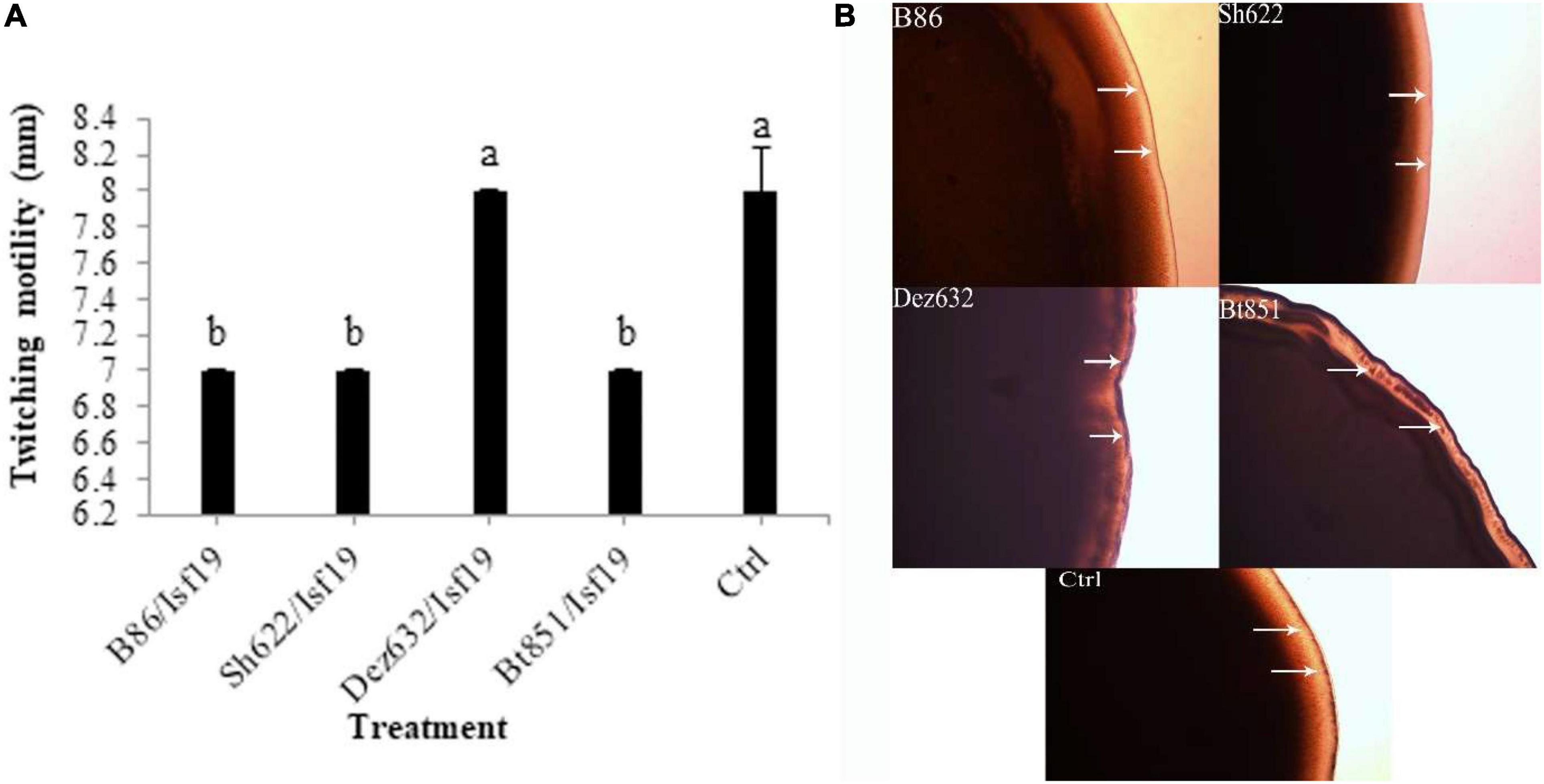
Figure 4. Effects of VOCs produced by Streptomyces sp. B86, Pantoea sp. Dez632, Pseudomonas sp. Bt851, and Stenotrophomonas sp. Sh622 on twitching motility of B. pumilus Isf19 compared to the non-treated control (Ctrl). The diameter of motility zone (A) and representative plate of swarming motility assay (B) were shown. Three replicates were used for each treatment. Error bars indicate the SE of the three replicates. Different letters indicate significant differences (P = 0.05).
Effect of Volatile Organic Compounds of Endophytic Bacteria on Chemotaxis, Biofilm Formation, and Root Attachment
Based on the results of ANOVA analysis, significant differences existed between all treatments in the chemotaxis assay in the number of cells migrated toward the sugar beetroot extract (F = 22.36; P < 0.0001) (Table 2). As shown in Figure 5A, VOCs produced by B86, Bt851, Dez632, and Sh622 strains by about 55, 52, 43, and 38%, respectively, reduced the motility of B. pumilus Isf19 cells toward root extract compared with control.
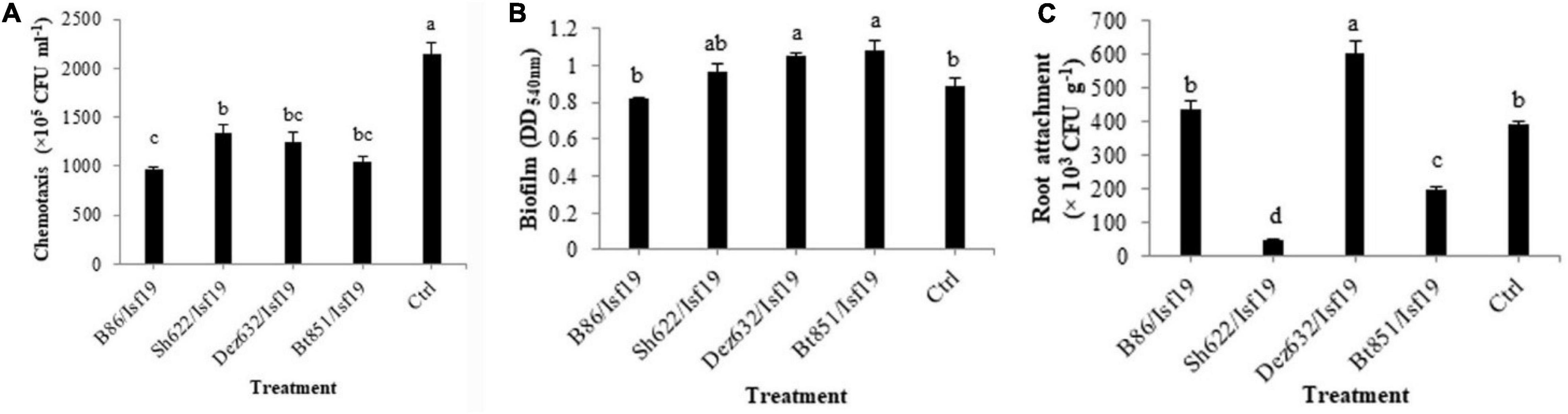
Figure 5. Effects of VOCs produced by Streptomyces sp. B86, Pantoea sp. Dez632, Pseudomonas sp. Bt851, and Stenotrophomonas sp. Sh622 on (A) chemotaxis behavior toward root extract of sugar beet, (B) biofilm formation, and (C) root attachment of B. pumilus Isf19 compared to the non-treated control (Ctrl). Error bars indicate the SE of the three replicates. Different letters indicate significant differences (P = 0.05).
As presented in Table 2, the result of ANOVA showed a significant difference between all treatments in the biofilm formation assay compared with non-treated control (F = 4.31; P = 0.0277). As shown in Figure 5B, no significant difference was observed in the biofilm formation by B. pumilus Isf19 exposed to VOCs of B86 and Sh622. Interestingly, significant increasing effects in biofilm formation were observed after treatment of B. pumilus Isf19 with volatiles of Dez632 and Bt851 strains compared to the control.
The ability of B. pumilus Isf19 to attach to sugar beetroot segments after treatment with endophytic bacterial strains was investigated. Based on the results of ANOVA, significant differences existed between all treatments in the root attachment of treated B. pumilus Isf19 cells (F = 73.40; P < 0.0001). The highest inhibition was related to Sh622 with 88% reduction and then was observed for Bt851 with 50% reduction. Furthermore, our findings showed no significant reduction in the attachment of exposed B. pumilus Isf19 cells to roots by B86 compared with control (Figure 5C). Surprisingly, volatiles of Dez632 increased the root attachment of B. pumilus Isf19 cells.
Scanning Electron Microscopy
SEM analysis revealed that more than 70 and 30% of the B. pumilus Isf19 cell exposure to the VOCs produced by Streptomyces sp. B86 and Stenotrophomonas sp. Sh622 strains, respectively, showed a wide range of morphological abnormalities compared to the non-exposed control (Figure 6). The SEM of the non-treated control showed normal cell shape and growth. By contrast, the B. pumilus Isf19 cells were damaged in the presence of the VOCs.
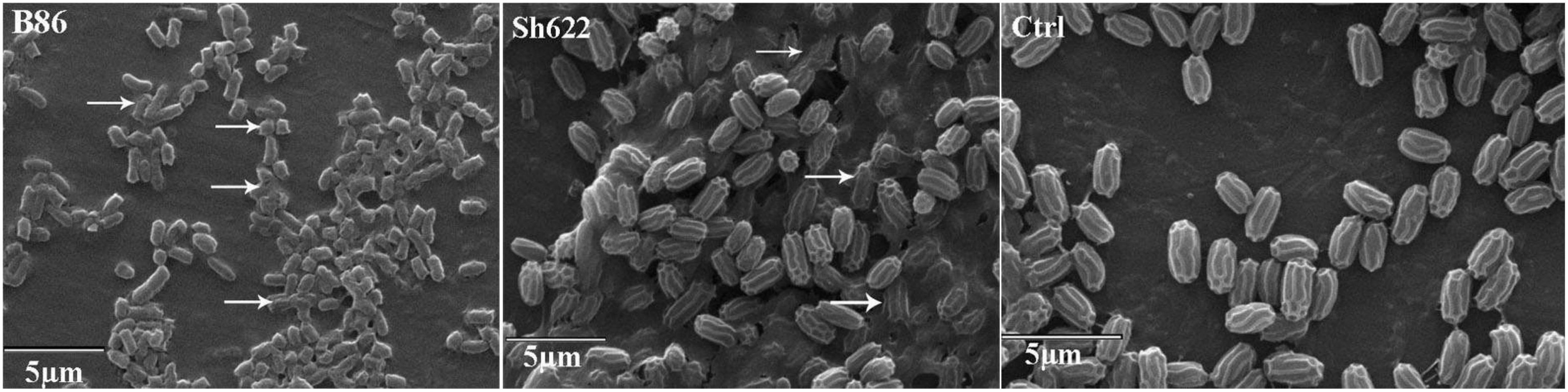
Figure 6. Scanning electron micrographs of Bacillus pumilus Isf19 cells exposed with VOCs produced by Streptomyces sp. B86 and Stenotrophomonas sp. Sh622 strains. Ctrl: non-treated control cells. Arrowheads indicate cell disruption or abnormality.
Induction of Defense Responses in Sugar Beetroots
qRT-PCR was conducted to examine the relative expression levels of PR1 and NBS-LRR2 genes in the non-inoculated sugar beetroot slices (control) and root treated by Dez632, Bt851, and/or B. pumilus Isf19. Results revealed that the expression of the defense gene responsive to salicylic acid (SA), including PR1, did not change in sugar beetroot slices treated with endophytic bacteria and pathogen at day 0, but significant differences were observed between all treatments and control two days after inoculation. Furthermore, on Day 7 after inoculation, significant difference was observed regarding PR1 gene expression level in root slices treated by Dez632 after pathogen challenge (Figure 7A). The expression level of the PR1 gene increased by 8.5- and 18-fold in Dez632/Isf19 treatment than root slices inoculated with pathogen alone and control, respectively.
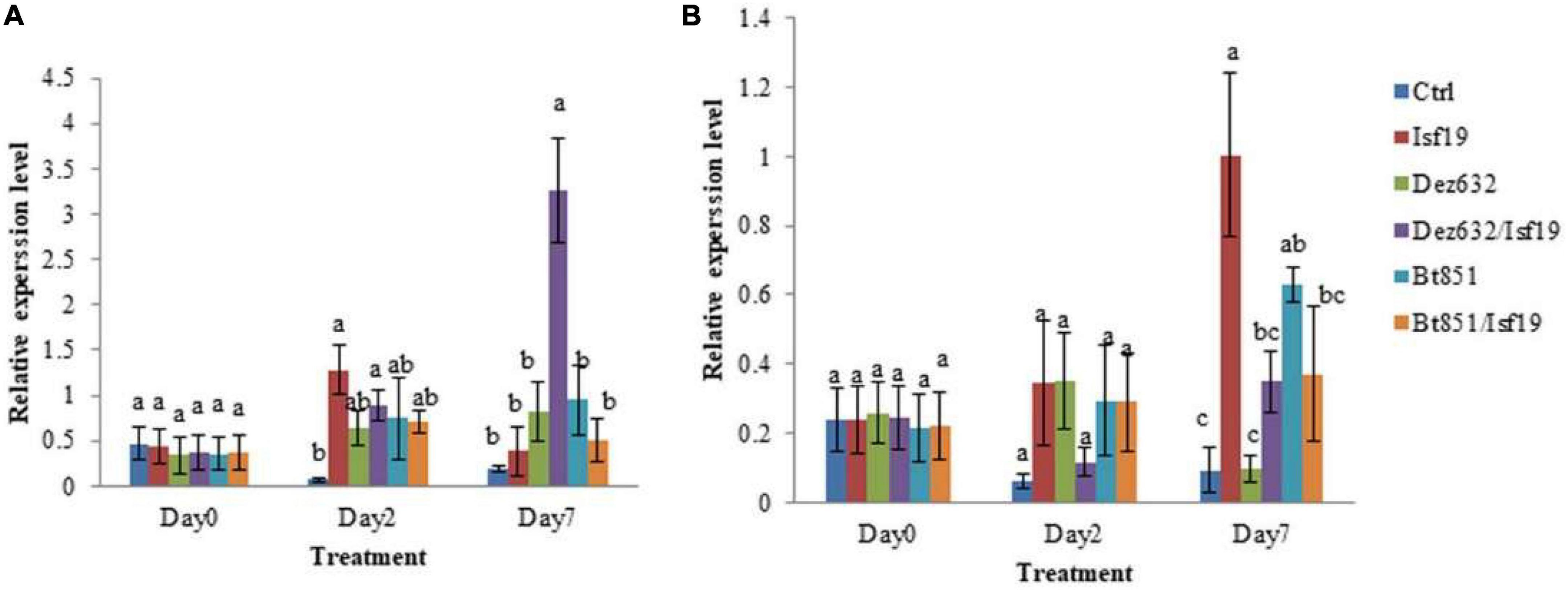
Figure 7. Relative expression levels of PR1 (A) and NBS-LRR2 (B) defense-related genes in the non-inoculated sugar beet root slices (Ctrl), root slices treated by Pantoea sp. Dez632, Pseudomonas sp. Bt851, and/or B. pumilus Isf19. Results represent the means of three replicates. Vertical bars indicate standard errors (SE), and different letters at each day after treatment indicate statistically significant differences between treatments at probability levels of 5%.
In addition, the expression level of the defense gene responsive to jasmonic acid (JA) pathway, including the NBS-LRR2 gene remained unchanged in sugar beetroot slices treated with endophytic bacteria and/or pathogen at Day 0; however, significant differences were observed between all treatments except Dez632/Isf19 compared to the control 2 days after inoculation. Results revealed that on Day 7 after inoculation, significant difference was observed regarding NBS-LRR2 gene expression level in root slices treated by endophytic bacteria and/or pathogen compared to the control (Figure 7B). The exception was treated root with Dez632 alone which remains unchanged. The expression of NBS-LRR2 gene in the root slices inoculated with pathogen alone showed the highest level 7 days after inoculation.
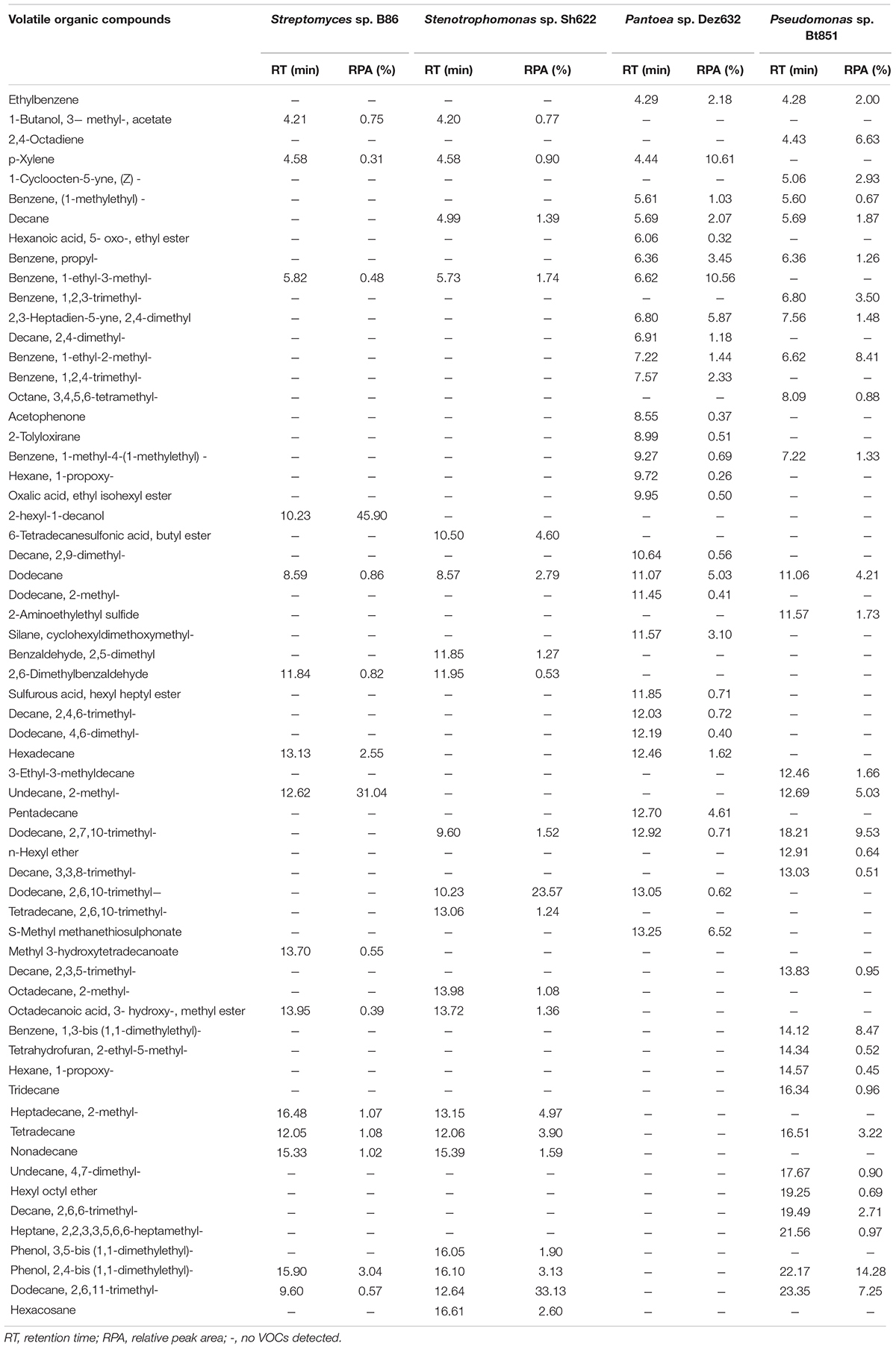
Table 3. Volatile organic compounds produced by B86, Sh622, Dez632, and Bt851 strains against Bacillus pumilus Isf19 and detected by GC–MS analysis.
Identification of Volatile Organic Compounds Produced by Endophytic Bacteria
The GC–MS analysis showed that all endophytic bacteria produced VOCs with various profiles (Table 3). Strains B86, Sh622, Dez632, and Bt851 produced 15, 20, 28, and 30 VOCs, respectively, with high quality. The VOCs dodecane was produced by all bacterial strains tested. The VOCs 1-decanol, 2- hexyl-, methyl 3-hydroxytetradecanoate are specifically produced by strain B86. The main VOC produced by this strain was 1-decanol, 2- hexyl-, and undecane, 2-methyl- with peak areas of 45.90% (RT = 10.23) and 31.04% (RT = 12.62), respectively, in the high quality.
Strain Sh622 specifically produced 6-tetradecanesulfonic acid, butyl ester, benzaldehyde, 2,5-dimethyl, tetradecane, 2,6,10- trimethyl-, octadecane, 2- methyl-, phenol, 3,5-bis (1,1-dimethylethyl)-, and hexacosane volatiles. The main VOCs produced by Sh622 were dodecane, 2,6,10-trimethyl- with a peak area of 23.57% (RT = 10.23) and dodecane, 2,6,11-trimethyl- with a peak area of 33.13% (RT = 12.64).
Only strain Dez632 produced hexanoic acid, 5- oxo-, ethyl ester, decane, 2,4- dimethyl-, benzene, 1,2,4- trimethyl-, acetophenone, 2-tolyloxirane, hexane, 1- propoxy-, oxalic acid, ethyl isohexyl ester, decane, 2,9- dimethyl-, dodecane, 2- methyl-, silane, cyclohexyldimethoxymethyl-, sulfurous acid, hexyl heptyl ester, decane, 2,4,6- trimethyl-, dodecane, 4,6- dimethyl-, pentadecane, and S-methyl methanethiosulfonate as VOCs. The main VOCs produced by this strain were p-xylene and benzene, 1-ethyl-3-methyl- with peak areas of 10.61% (RT = 4.44) and 10.56 (RT = 6.62), respectively.
Strain Bt851 specifically produced VOCs 2,4-octadiyne, 1-cycloocten-5-yne, (Z)-, benzene, 1,2,3- trimethyl-, octane, 3,4,5,6- tetramethyl-, 2-aminoethylethyl sulfide, 3-ethyl-3-methyldecane, n-hexyl ether, decane, 3,3,8- trimethyl-, decane, 2,3,5- trimethyl-, benzene, 1,3-bis (1,1-dimethylethyl)-, tetrahydrofuran, 2-ethyl-5- methyl-, hexane, 1- propoxy-, tridecane, undecane, 4,7- dimethyl-, hexyl octyl ether, decane, 2,6,6- trimethyl-, and heptane, 2,2,3,3,5,6,6-heptamethyl-. The main VOCs produced by Bt851 were phenol, 2,4-bis (1,1-dimethylethyl)- 14.28% (RT = 22.17), followed by dodecane, 2,7,10-trimethyl- 9.53% (RT = 18.21), benzene, 1,3-bis (1,1-dimethylethyl)- 8.47% (RT = 14.12), and benzene, 1-ethyl-2-methyl- 8.41% (RT = 6.62) (Table 3).
Discussion
Due to the importance of extracted sugar from sugar beet, the presence of toxins and chemical residues may endanger people’s health. Therefore, replacing safe methods like biocontrol agents instead with chemical control is a requirement. It is well documented that endophytic bacteria have potential biocontrol activity against plant pathogens. Each plant harbors to a certain degree-specific microbial community, and in some plant–microorganism interactions, the evolutionary relationships have been reported (Bulgarelli et al., 2012; Zachow et al., 2014). Due to the adaptation of endophytic bacteria to sugar beet, such bacteria might provide better effective biocontrol activity. With this hypothesis, endophytic bacteria were isolated from healthy sugar beet and sea beet plants. In this study, we reported that the VOCs produced by some of these endophytic bacteria, including Streptomyces sp. B86, Pantoea sp. Dez632, Pseudomonas sp. Bt851, and Stenotrophomonas sp. Sh622, significantly reduced the virulence traits of B. pumilus Isf19 both in vitro and in situ. The effect of VOCs produced by these biocontrol strains on B. pumilus strain as a pathogen has not been reported before. The VOCs of biocontrol strains have shown antibacterial activity against other plant pathogens, like the VOCs produced by Streptomyces coelicolor and Streptomyces avermitilis, respectively, with a broad-range antibacterial activity (Tetzlaff et al., 2006; Zhao et al., 2008). A plant-associated bacteria, Pseudomonas fluorescens B-4117, emit volatiles that inhibited the growth of Agrobacterium tumefaciens and Agrobacterium vitis (Dandurishvili et al., 2011). Similarly, the VOCs of Pseudomonas fluorescens strain showed bacteriostatic effects on Ralstonia solanacearum (Raza et al., 2016a). Although many studies reported on VOCs produced by Pantoea and Stenotrophomonas strains with antifungal activity (López et al., 2021), only a few studies reported the effects of VOCs on virulence traits of bacterial pathogens (Ghasemi et al., 2021).
Motility and biofilm formation are major adaptive behaviors for Bacillus species in plant tissue colonization and invasion (Houry et al., 2010). In addition, chemotaxis has a major role for plant-associated bacteria, including Bacillus species, whether they are beneficial or pathogenic (Allard-Massicotte et al., 2016). Results presented in our study revealed that VOCs produced by endophytic bacterial strains significantly inhibited motility and chemotaxis behaviors of B. pumilus Isf19. This result is in line with previous reports that show motility behavior of bacterial cells is controlled by chemotaxis (Sourjik and Wingreen, 2011). Surprisingly, no inhibition effects between VOCs-treated and non-treated B. pumilus Isf19 cells were observed in biofilm formation. This finding is in agreement with well documented that there is a motility-to-biofilm transition among bacteria where the inhibition of motility promotes biofilm formation (Guttenplan and Kearns, 2013).
Streptomyces species, as a member of Actinobacteria, are the most taxa found in the rhizosphere of sugar beet plants (Cordovez et al., 2015). Members of the genus Streptomyces produce a wide range of VOCs which are important for the interaction between microorganisms and suppression of phytopathogens (Wan et al., 2008). The VOCs produced by strain Streptomyces sp. B86 significantly reduced motility and chemotaxis behavior of B. pumilus Isf19. In contrast, no significant effects were shown in biofilm formation and attachment. Two main VOCs produced by this strain were 2-hexyl-1-decanol and 2-methyl-undecane. Togashi et al. (2007) reported that 2-hexyl-1-decanol had a bactericidal effect and membrane-damaging activity against Staphylococcus aureus.
Strain Sh622 showed high similarity to Stenotrophomonas sp. BG28 species. Several strains of this genus were reported as effective biocontrol agents against many bacterial plant pathogens, such as Ralstonia solanacearum (Ryan et al., 2009). López et al. (2021) reported endophyte of tomato plants belonging to genera Stenotrophomonas with the antifungal activity which was related to the synthesis of VOCs and soluble compounds. VOCs produced by this strain could significantly inhibit sugar beet root attachment, chemotaxis, and different motility behavior of B. pumilus Isf19. Electron microscopic analysis revealed that bacterial pathogen cells were damaged in the presence of VOCs of Stenotrophomonas sp. Sh622. The main VOCs produced by strain Stenotrophomonas sp. Sh622 were dodecane, 2,6,11-trimethyl and dodecane, 2,3,10-trimethyl. These major compounds may be responsible for antibacterial activity against Isf19. Previous studies have reported the antibacterial activity of these compounds (Rahbar et al., 2012).
Strain Dez632 had high similarity with Pantoea agglomerans species. P. agglomerans has been reported with antagonistic activity against many bacterial and fungal plant pathogens, which is associated with its ability to antibiotic production or other mechanisms (Dutkiewicz et al., 2016). The genome sequencing of plant beneficial P. agglomerans strains indicated the presence of genes involved in the biosynthesis of VOCs that may stimulate plant growth (Shariati et al., 2017). However, there is no information on the involvement of VOCs produced by P. agglomerans strains with antibacterial activity. VOCs produced by these bacteria significantly reduced chemotaxis and swimming motility behaviors of B. pumilus Isf19. The main VOCs produced by this strain were p-xylene and benzene, 1-ethyl-3- methyl-, and S-methyl methanethiosulfonate. These compounds were produced by bacterial species and were shown to efficiently inhibit plant pathogens (Joller et al., 2020).
GC-MS analysis revealed that bacterial strains tested produce VOCs which had various antibacterial activities. Strain Pseudomonas sp. Bt851 significantly reduced chemotaxis, root attachment, and motility but not biofilm formation by B. pumilus Isf19. The main VOCs produced by Bt851 were phenol, 2,4-bis (1,1-dimethylethyl)- synonym 2,4-di-tert-butylphenol. This compound was reported in at least 16 bacterial species (Zhao et al., 2020). 2,4-di-tert-butylphenol with antimicrobial activity has been reported from Pseudomonas fluorescens and Pseudomonas monteilii (Dharni et al., 2014; Ren et al., 2019). Mishra et al. (2020) reported that 2,4,-di-tert-butylphenol isolated from endophytic fungus, Daldinia eschscholtzii, inhibited the quorum sensing and biofilm formation of Pseudomonas aeroginosa.
Pseudomonas and Pantoea genera are widely studied as biocontrol agents. Several species within these genera were described to have the ability to increase defense reaction against different plant pathogens (Weller, 2007; Magnin-Robert et al., 2012). Based on results obtained in this study, Bt851 and Dez632 strains showed higher decreasing effects on soft rot development by B. pumilus Isf19. Therefore, we decided to examine whether this reduction effect is related to the induction of defense response. We analyzed the expression level of PR1 and NBS-LRR2 genes, and markers of the salicylic acid and jasmonic acid pathways, respectively, in sugar beet roots after treatment by Bt851, Dez632, and inoculation with B. pumilus Isf19. The expression level of PR1 and NBS-LRR2 remained unchanged in roots treated only with endophytic bacteria or after challenge with bacterial pathogen at Day 0 compared to the control. These results are consistent with the finding that plant tissues either did not respond or weakly responded to beneficial bacteria to reduce activation of defense responses which might be important to their successful colonization (Zamioudis and Pieterse, 2012). Our results revealed that Pantoea sp. Dez632 and Pseudomonas sp. Bt851 can upregulate the SA-responsive gene PR1 in sugar beetroot slices against B. pumilus Isf19. We also showed that Bt851 can stimulate JA-dependent defense, as indicated by higher expression of the NBS-LRR2 gene upon pathogen challenge, which suggests that at least in part the defense response can be mediated by both SA and JA pathways, activating the expression of PR1 and NBS-LRR2 genes independently against B. pumilus Isf19. Surprisingly, B. pumilus Isf19 could increase the expression of both genes when inoculated into roots. This is in agreement with previous studies that show Bacillus species can activate defense reactions in plant tissues (Poveda et al., 2020).
Conclusion
In conclusion, this is the first report of the effect of VOCs produced by endophytic bacteria isolated from sugar beet and sea beet plants on the growth and virulence traits of B. pumilus as the sugar beet root rot agent. The VOCs can spread over a long distance, make bacteriostatic environment around the plant tissues, and therefore would play an important role to keep pathogen away from the roots. Our results clearly revealed that these endophytic bacteria not only reduce bacterial pathogen growth rate, but also restrict its movement to invade plant roots and/or induce plant resistance. B. pumilus is a soilborne pathogen that mediates postharvest disease; therefore, the application of such endophytic bacteria in soil and/or storage may be a useful method to increase both the quality and quantity of sugar beet roots. Information on the biocontrol mechanisms mediated by VOCs during microbial interactions is important to develop safer methods to control plant disease.
Data Availability Statement
The datasets presented in this study can be found in online repositories. The names of the repository/repositories and accession number(s) can be found below: https://www.ncbi.nlm.nih.gov/genbank/ (MZ647525, MZ647528, MZ647535, MZ647537, and MZ647534).
Author Contributions
SS conducted all the experiments, analyzed the data, and wrote the draft manuscript. BH, BB, and SA assisted and supervised the data. All authors read and approved the manuscript.
Funding
This research work was supported by the University of Kurdistan, Iran.
Conflict of Interest
The authors declare that the research was conducted in the absence of any commercial or financial relationships that could be construed as a potential conflict of interest.
Publisher’s Note
All claims expressed in this article are solely those of the authors and do not necessarily represent those of their affiliated organizations, or those of the publisher, the editors and the reviewers. Any product that may be evaluated in this article, or claim that may be made by its manufacturer, is not guaranteed or endorsed by the publisher.
References
Ahmadi, E., Kowsari, M., Azadfar, D., and Salehi Jouzani, G. (2019). Bacillus pumilus and Stenotrophomonas maltophilia as two potentially causative agents involved in Persian oak decline in Zagros forests (Iran). For. Pathol. 49:e12541. doi: 10.1111/efp.12541
Allard-Massicotte, R., Tessier, L., Lécuyer, F., Lakshmanan, V., Lucier, J. F., Garneau, D., et al. (2016). Bacillus subtilis early colonization of Arabidopsis thaliana roots involves multiple chemotaxis receptors. mBio 7, e1664–e1616. doi: 10.1128/mBio.01664-16
Asghari, S., Harighi, B., Ashengroph, M., Clement, C., Aziz, A., Esmaeel, Q., et al. (2020). Induction of systemic resistance to Agrobacterium tumefaciens by endophytic bacteria in grapevine. Plant Pathol. 69, 827–837. doi: 10.1111/ppa.13175
Aswani, R., Thomas, R., and Radhakrishnan, E. K. (2022). “Induction of plant defense response by endophytic microorganisms,” in Biocontrol Mechanisms of Endophytic Microorganisms, eds E. K. Radhakrishnan, K. Ajay, and R. Aswani (Cambridge: Academic Press), 89–115. doi: 10.1016/B978-0-323-88478-5.00002-X
Basu, S., Saha, M. N., Chattopadhyay, D., and Chakrabarti, K. (2009). Large scale degumming of ramie fibre using a newly isolated Bacillus pumilus DKS1 with high pectate lyase activity. J. Ind. Microbiol. Biotechnol. 36, 239–245. doi: 10.1007/s10295-008-0490-y
Bulgarelli, D., Rott, M., Schlaeppi, K., van Themaat, E. V. L., Ahmadinejad, N., Assenza, F., et al. (2012). Revealing structure and assembly cues for Arabidopsis root-inhabiting bacterial microbiota. Nature 488, 91–95. doi: 10.1038/nature11336
Compant, S., Duffy, B., Nowak, J., Clement, C., and Ait Barka, E. (2005). Use of plant growth-promoting bacteria for biocontrol of plant diseases: principles, mechanisms of action, and future prospects. Appl. Environ. Microbiol. 71, 4951–4959. doi: 10.1128/AEM.71.9.4951-4959.2005
Cordovez, V., Carrion, V. J., Etalo, D. W., Mumm, R., Zhu, H., van Wezel, G. P., et al. (2015). Diversity and functions of volatile organic compounds produced by Streptomyces from a disease-suppressive soil. Front. Microbiol. 6:1081. doi: 10.3389/fmicb.2015.01081
Dandurishvili, N., Toklikishvili, N., Ovadis, M., Eliashvili, P., Giorgobiani, N., Keshelava, R., et al. (2011). Broad-range antagonistic rhizobacteria Pseudomonas fluorescens and Serratia plymuthica suppress Agrobacterium crown gall tumours on tomato plants. J. Appl. Microbiol. 110, 341–352. doi: 10.1111/j.1365-2672.2010.04891.x
Davidsson, P. R., Kariola, T., Niemi, O., and Palva, E. T. (2013). Pathogenicity of and plant immunity to soft rot pectobacteria. Front. Plant Sci. 4:191. doi: 10.3389/fpls.2013.00191
Dharni, S., Sanchita, Maurya, A., Samad, A., Srivastava, S. K., Sharma, A., et al. (2014). Purification, characterization, and in vitro activity of 2,4-Di-tert-butylphenol from Pseudomonas monteilii PsF84: conformational and molecular docking studies. J. Agric. Food Chem. 62, 6138–6146. doi: 10.1021/jf5001138
Dutkiewicz, J., Mackiewicz, B., Lemieszek, M. K., Golec, M., and Milanowski, J. (2016). Pantoea agglomerans: a mysterious bacterium of evil and good. Part IV. Beneficial effects. Ann. Agric. Environ. Med. 23, 206–222. doi: 10.5604/12321966.1203879
Food and Agriculture Organization [FAO] (2020). FAOSTAT, Statistics, Crops Production. Available online at: https://www.fao.org/faostat/en/#data/QCL/visualize
Fugate, K. K., de Oliveira, L. S., Perruzo Ferrareze, J., Bolton, M. D., Deckard, E. L., and Finger, F. L. (2017). Jasmonic acid causes short- and long-term alterations to the transcriptome and the expression of defense genes in sugarbeet roots. Plant Gene 9, 50–63. doi: 10.1016/j.dib.2017.02.008
Fugate, K. K., Ferrareze, J. P., Bolton, M. D., Deckard, E. L., and Campbell, L. G. (2012). Postharvest jasmonic acid treatment of sugarbeet roots reduces rot due to Botrytis cinerea, Penicillium claviforme, and Phoma betae. Post. Biol. Technol. 65, 1–4.
Galal, A. A., El-Bana, A. A., and Janse, J. (2006). Bacillus pumilus, a new pathogen on mango plants. Egypt. J. Phytopathol. 34, 17–29.
Garbeva, P., and Weisskopf, L. (2020). Airborne medicine: bacterial volatiles and their influence on plant health. New Phytol. 226, 32–43. doi: 10.1111/nph.16282
Ghasemi, S., Harighi, B., Mojarrab, M, and Azizi, A. (2021). Response of Pseudomonas tolaasii, the causal agent of mushroom brown blotch disease to the volatile compounds produced by endofungal bacteria. BioControl 66, 421–432. doi: 10.1007/s10526-020-10071-6
Guttenplan, S. B., and Kearns, D. B. (2013). Regulation of flagellar motility during biofilm formation. FEMS Microbiol. Rev. 37, 849–871. doi: 10.1111/1574-6976.12018
Houry, A., Briandet, R., Aymerich, S., and Gohar, M. (2010). Involvement of motility and flagella in Bacillus cereus biofilm formation. Microbiology 156, 1009–1018. doi: 10.1099/mic.0.034827-0
Joller, C., De Vrieze, M., Moradi, A., Fournier, C., Chinchilla, D., L’Haridon, F., et al. (2020). S-methyl Methanethiosulfonate: Promising Late Blight Inhibitor or Broad Range Toxin? Pathogens 9:496. doi: 10.3390/pathogens9060496
Li, B., Qiu, W., Tan, Q. M., Su, T., Fang, Y., and Xie, G. L. (2009). Association of a Bacillus species with leaf and twig dieback of Asian pear (Pyrus pyrifolia) in China. J. Plant Pathol. 91, 705–708. doi: 10.4454/jpp.v91i3.565
Liebe, S., and Varrelmann, M. (2016). Effect of environment and sugar beet genotype on root rot development and pathogen profile during storage. Phytopathology 106, 65–75. doi: 10.1094/PHYTO-07-15-0172-R
Lima, A. O. S., Quecine, M. C., Fungaro, M. H. P., Andreote, F. D., Mac-cheroni, W., Araujo, W. L., et al. (2005). Molecular characterization of a beta-1,4-endoglucanase from an endophytic Bacillus pumilus strain. Appl. Microbiol. Biotech. 68, 57–65. doi: 10.1007/s00253-004-1740-1
López, S. M. Y., Pastorino, G. N., and Balatti, P. A. (2021). Volatile organic compounds profile synthesized and released by endophytes of tomato (Solanum lycopersici L.) and their antagonistic role. Arch. Microbiol. 203, 1383–1397. doi: 10.1007/s00203-020-02136-y
Magnin-Robert, M., Quantinet, D., Couderchet, M., Aziz, A., and Trotel-Aziz, P. (2012). Differential induction of grapevine resistance and defense reactions against Botrytis cinerea by bacterial mixtures in vineyards. BioControl 58, 117–131. doi: 10.1007/s10526-012-9474-y
Marone, D., Russo, M. A., Laidò, G., De Leonardis, A. M., and Mastrangelo, A. M. (2013). Plant nucleotide binding site–leucine-rich repeat (NBS-LRR) genes: active guardians in host defense responses. Int. J. Mol. Sci. 14, 7302–7326. doi: 10.3390/ijms14047302
Mishra, R., Kushveer, J. S., Khan, M. I. K., Pagal, S., Meena, C. K., Murali, A., et al. (2020). 2,4-Di-tert-butylphenol isolated from an endophytic fungus, Daldinia eschscholtzii, reduces virulence and quorum sensing in Pseudomonas aeruginosa. Front. Microbiol. 11:1668. doi: 10.3389/fmicb.2020.01668
Nagar, S., Mittal, A., Kumar, D., and Gupta, V. K. (2012). Production of alkali tolerant cellulase free xylanase in high levels by Bacillus pumilus SV205. Int. J. Biol. Macromol. 50, 414–420. doi: 10.1016/j.ijbiomac.2011.12.026
Ordal, G. W., and Gibson, K. J. (1977). Chemotaxis toward amino acids by Bacillus subtilis. J. Bacteriol. 129, 151–155.
O’Toole, G. A., and Kolter, R. (1998). Initiation of biofilm formation in Pseudomonas fluorescens WCS365 proceeds via multiple, convergent signaling pathways: a genetic analysis. Mol. Microbiol. 28, 449–461. doi: 10.1046/j.1365-2958.1998.00797.x
Peng, Q., Yuan, Y., and Gao, M. (2013). Bacillus pumilus, a novel ginger rhizome rot pathogen in China. Plant Dis. 97, 1308–1315. doi: 10.1094/PDIS-12-12-1178-RE
Pfaffl, M. W. (2001). A new mathematical model for relative quantification in real-time RT-PCR. Nucleic Acids Res. 29, 2003–2007. doi: 10.1093/nar/29.9.e45
Pieterse, C. M. J., van der Does, D., Zamioudis, C., Leon-Reyes, A., and van Wees, S. C. M. (2012). Hormonal modulation of plant immunity. Ann. Rev. Cell Dev. Biol. 28, 489–521. doi: 10.1146/annurev-cellbio-092910-154055
Portieles, R., Xu, H., Yue, Q., Zhao, L., Zhang, D., Du, L., et al. (2021). Heat-killed endophytic bacterium induces robust plant defense responses against important pathogens. Sci. Rep. 11:12182. doi: 10.1038/s41598-021-91837-5
Poveda, J., Barquero, M., and González-Andrés, F. (2020). Insight into the microbiological control strategies against Botrytis cinerea using systemic plant resistance activation. Agronomy 10:1822. doi: 10.3390/agronomy10111822
Rahbar, N., Shafagha, A., and Salimi, F. (2012). Antimicrobial activity and constituents of the hexane extracts from leaf and stem of Origanum vulgare L. sp. viride (Boiss.) Hayek. Growing wild in Northwest Iran. J. Med. Plants Res. 6, 2681–2685.
Rajer, F. U., Wu, H., Xie, Y., Xie, S., Raza, W., Tahir, H. A. S., et al. (2017). Volatile organic compounds produced by a soil-isolate, Bacillus subtilis FA26 induce adverse ultra-structural changes to the cells of Clavibacter michiganensis ssp. sepedonicus, the causal agent of bacterial ring rot of potato. Microbiology 163, 523–530. doi: 10.1099/mic.0.000451
Raza, W., Ling, N., Liu, D., Wei, Z., Huang, Q., and Shen, Q. (2016a). Volatile organic compounds produced by Pseudomonas fluorescens WR-1 restrict the growth and virulence traits of Ralstonia solanacearum. Microbiol. Res. 192, 103–113. doi: 10.1016/j.micres.2016.05.014
Raza, W., Ling, N., Yang, L., Huang, Q., and Shen, Q. (2016b). Response of tomato wilt pathogen Ralstonia solanacearum to the volatile organic compounds produced by a biocontrol strain Bacillus amyloliquefaciens SQR-9. Sci. Rep. 6:24856. doi: 10.1038/srep24856
Ren, J., Wang, J., Karthikeyan, S., Liu, H., and Cai, J. (2019). Natural anti-phytopathogenic fungi compound phenol, 2, 4-bis (1, 1-dimethylethyl) from Pseudomonas fluorescens TL-1. Indian J. Biochem. Biophys. 56, 162–168.
Ryan, R., Monchy, S., Cardinale, M., Taghavi, S., Crossman, L., Avison, M. B., et al. (2009). The versatility and adaptation of bacteria from the genus Stenotrophomonas. Nat. Rev. Microbiol. 7, 514–525. doi: 10.1038/nrmicro2163
Schmidt, J., Dotson, B. R., Schmiderer, L., van Tour, A., Kumar, B., Marttila, S., et al. (2020). Substrate and plant genotype strongly influence the growth and gene expression response to Trichoderma afroharzianum T22 in sugar beet. Plants 9:1005. doi: 10.3390/plants9081005
Schmittgen, T. D., and Livak, K. (2008). Analyzing real-time PCR data by the comparative CT method. Nat. Protoc. 3, 1101–1108. doi: 10.1038/nprot.2008.73
Schulz, S., and Dickschat, J. S. (2007). Bacterial volatiles: the smell of small organisms. Nat. Prod. Rep. 24, 814–842. doi: 10.1039/b507392h
Shariati, V., Malboobi, M. A., Tabrizi, Z., Tavakol, E., Owilia, P., and Safari, M. (2017). Comprehensive genomic analysis of a plant growth-promoting rhizobacterium Pantoea agglomerans strain P5. Sci. Rep. 7:15610. doi: 10.1038/s41598-017-15820-9
Sourjik, V., and Wingreen, N. S. (2011). Responding to chemical gradients: bacterial chemotaxis. Curr. Opin. Cell Biol. 24, 262–268. doi: 10.1016/j.ceb.2011.11.008
Strausbaugh, C. A., and Gillen, A. M. (2008). Bacteria and yeast associated with sugar beet root rot at harvest in the Intermountain West. Plant Dis. 92, 357–363. doi: 10.1094/PDIS-92-3-0357
Subramaniyan, S. (2012). Isolation, purification and characterisation of low molecular weight xylanase from Bacillus pumilus SSP-34. Appl. Biochem. Biotechnol. 166, 1831–1842. doi: 10.1007/s12010-012-9600-4
Tahir, H. A., Gu, Q., Wu, H., Niu, Y., Huo, R., and Gao, X. (2017). Bacillus volatiles adversely affect the physiology and ultra-structure of Ralstonia solanacearum and induce systemic resistance in tobacco against bacterial wilt. Sci. Rep. 7:40481. doi: 10.1038/srep40481
Tetzlaff, C. N., You, Z., Cane, D. E., Takamatsu, S., Omura, S., and Ikeda, H. (2006). A gene cluster for biosynthesis of the sesquiterpenoid antibiotic pentalenolactone in Streptomyces avermitilis. Biochemistry 45, 6179–6186. doi: 10.1021/bi060419n
Togashi, N., Shiraishi, A., Nishizaka, M., Matsuoka, K., Endo, K., Hamashima, H., et al. (2007). Antibacterial activity of long-chain fatty alcohols against Staphylococcus aureus. Molecules 12, 139–148. doi: 10.3390/12020139
Trebbi, D., and McGrath, J. M. (2004). Fluorometric sucrose evaluation for sugar beet. J. Agric. Food Chem. 52, 6862–6867. doi: 10.1021/jf048900c
Wan, M., Li, G., Zhang, J., Jiang, D., and Huang, H. C. (2008). Effect of volatile substances of Streptomyces platensis F-1 on control of plant fungal diseases. Biol. Control 46, 552–559. doi: 10.1016/j.biocontrol.2008.05.015
Weller, D. M. (2007). Pseudomonas biocontrol agents of soilborne pathogens: Looking back over 30 years. Phytopathology 97, 250–256. doi: 10.1094/PHYTO-97-2-0250
Weltmeier, F., Mäser, A., Menze, A., Hennig, S., Schad, M., Breuer, F., et al. (2011). Transcript profiles in sugar beet genotypes uncover timing and strength of defense reactions to Cercospora beticola infection. Mol. Plant Microbe Interact. 24, 758–772. doi: 10.1094/MPMI-08-10-0189
Wolfgang, A., Zachow, C., Müller, H., Grand, A., Temme, N., Tilcher, R., et al. (2020). Understanding the impact of cultivar, seed origin, and substrate on bacterial diversity of the sugar beet rhizosphere and suppression of soil-borne pathogen. Front. Plant Sci. 11:560869. doi: 10.3389/fpls.2020.560869
Xie, A., Zang, H., Wu, H., Rajer, F. U., and Gao, X. (2018). Antibacterial effects of volatile produced by Bacillus strain D13 against Xanthomonas oryzae pv. oryzae. Mol. Plant Pathol. 19, 49–58. doi: 10.1111/mpp.12494
Yuan, Y., and Gao, M. (2015). Genomic analysis of a ginger pathogen Bacillus pumilus providing the understanding to the pathogenesis and the novel control strategy. Sci. Rep. 5:10259. doi: 10.1038/srep10259
Zachow, C., Muller, H., Tilcher, R., and Berg, G. (2014). Differences between the rhizosphere microbiome of Beta vulgaris ssp. maritima-ancestor of all beet crops and modern sugar beets. Front. Microbiol. 5:415. doi: 10.3389/fmicb.2014.00415
Zachow, C., Tilcher, R., and Berg, G. (2008). Sugar beet-associated bacterial and fungal communities show a high indigenous antagonistic potential against plant pathogens. Microb. Ecol. 55, 119–129. doi: 10.1007/s00248-007-9257-7
Zamioudis, C., and Pieterse, C. M. (2012). Modulation of host immunity by beneficial microbes. Mol. Plant Microbe Interact. 25, 139–150. doi: 10.1094/MPMI-06-11-0179
Zarraonaindia, I., Owens, S. M., Weisenhorn, P., West, K., Hampton-Marcell, J., Lax, S., et al. (2015). The soil microbiome influences grapevine-associated microbiota. mBio 24:e02527-14. doi: 10.1128/mBio.02527-14
Zhao, B., Lin, X., Lei, L., Lamb, D. C., Kelly, S. L., Waterman, M. R., et al. (2008). Biosynthesis of the sesquiterpene antibiotic albaflavenone in Streptomyces coelicolor A3(2). J. Biol. Chem. 283, 8183–8189. doi: 10.1074/jbc.M710421200
Keywords: Bacillus pumilus, endophytic bacteria, induce resistance, sugar beet root rot, virulence traits, volatile compounds
Citation: Safara S, Harighi B, Bahramnejad B and Ahmadi S (2022) Antibacterial Activity of Endophytic Bacteria Against Sugar Beet Root Rot Agent by Volatile Organic Compound Production and Induction of Systemic Resistance. Front. Microbiol. 13:921762. doi: 10.3389/fmicb.2022.921762
Received: 16 April 2022; Accepted: 09 May 2022;
Published: 02 June 2022.
Edited by:
Gustavo Cordero-Bueso, University of Cádiz, SpainReviewed by:
Guo-Qiang Wu, Lanzhou University of Technology, ChinaNaser Safaie, Tarbiat Modares University, Iran
Copyright © 2022 Safara, Harighi, Bahramnejad and Ahmadi. This is an open-access article distributed under the terms of the Creative Commons Attribution License (CC BY). The use, distribution or reproduction in other forums is permitted, provided the original author(s) and the copyright owner(s) are credited and that the original publication in this journal is cited, in accordance with accepted academic practice. No use, distribution or reproduction is permitted which does not comply with these terms.
*Correspondence: Behrouz Harighi, bharighi@uok.ac.ir