- 1Key Laboratory of Forest Protection of National Forestry and Grassland Administration, Ecology and Nature Conservation Institute, Chinese Academy of Forestry, Beijing, China
- 2Biological Disaster Control and Prevention Center, National Forestry and Grassland Administration, Shenyang, China
Ophiostomatalean fungi usually facilitate bark beetles to infest tree hosts and seriously endanger the health of coniferous forests. Tomicus pilifer Spessivtsev is a common endemic bark beetle in Asia and primarily threatens Pinus koraiensis. Tomicus species have similar morphology; however, they can be differentiated by their genetic characteristics through phylogenetic analyses. To date, the 28S rDNA sequence of T. pilifer and the diversity of ophiostomatalean fungi associated with T. pilifer have not been reported. In this study, we aimed to clarify the taxonomic status of T. pilifer and identify ophiostomatalean fungi associated with T. pilifer infesting P. koraiensis in northeastern China. In total, 315 ophiostomatalean fungal strains were isolated from 62 adults of T. pilifer and 220 tissue samples from T. pilifer galleries in Jilin Province. Thirty-five representative strains were further identified by comparing their morphological and physiological characteristics and conducting the phylogenetic analysis of ITS, ITS2-LSU, TUB2, and TEF1-α. We identified nine species of ophiostomatalean fungi belonging to four genera, which included six novel species (Ceratocystiopsis changbaiensis sp. nov., Leptographium linjiangense sp. nov., Leptographium qieshaoense sp. nov., Ophiostoma piliferi sp. nov., Ophiostoma tonghuaense sp. nov., and Ophiostoma yaluense sp. nov.), two previously described species (Graphilbum interstitiale and Ophiostoma fuscum), and one undefined specie (Ceratocystiopsis sp. 1). To the best of our knowledge, this is the first report of G. interstitiale and O. fuscum in China and the fungal diversity of ophiostomatalean in T. pilifer. The dominant species were O. piliferi and L. qieshaoense, representing 39.37% and 35.87% of the isolates, respectively. The results of this study provide valuable information on the symbiotic relationship between bark beetles and ophiostomatalean fungi.
Introduction
Synergetic infections of forest insects and microbes are key drivers of deforestation worldwide (Wingfield et al., 2010, 2016; Six and Wingfield, 2011; Linnakoski et al., 2012a). Microbial pathogens and insects can cause more damage synergistically than when acting alone (Raffa et al., 2008). Some well-known examples of synergetic infections that cause major forest disturbances are those of ophiostomatalean fungi and beetles (Grégoire et al., 2015).
The pine shoot beetle, Tomicus Latreille (syn. Blastophagus Eichhoff, Myelophilus Eichhoff, Scolytidae, Coleoptera), includes eight species; some of these kill conifers in Eurasian pine forests (Kirkendall et al., 2008; Li et al., 2010; Lieutier et al., 2015). Tomicus is considered one of the most aggressive bark beetles (Lieutier et al., 2015). During its life cycle, it can invade both sapwood and branches and infest multiple hosts. Currently, seven species have been recorded in China (Wang et al., 2019). Tomicus pilifer Spessivtsev, also called the Korean pine shoot beetle, is endemic to Asia (Russia and China) and primarily lives on Pinus koraiensis (Seib. Ex Succ.). However, it has also been documented on other Asian pines, such as P. armandii, P. tabulaeformis, and P. yunnanensis (Lieutier et al., 2015). In China, T. pilifer is mainly distributed in the Jilin and Gansu provinces, and 90% of the damaged trees are P. koraiensis. Moreover, Tomicus pilifer primarily infests the new shoots of P. koraiensis, which results in the withering of branches and leaves, hindered tree development, and death of seriously damaged trees (Wang et al., 2000).
Tomicus species have highly similar morphology, making it difficult to distinguish them based on morphological traits alone (Lieutier et al., 2015). For example, T. destruens and T. yunnanensis were previously considered to be T. piniperda; however, molecular studies revealed that they are two separate species (Gallego and Galián, 2001; Kerdelhué et al., 2002; Kohlmayr et al., 2002; Duan et al., 2004). Similarly, T. armandii was classified as a new species based on molecular and morphological data (Li et al., 2010). Although 28S rDNA phylogenetic analysis showed a genetically well-characterized clade, including six Tomicus species, it did not include T. pilifer and T. puellus (Li et al., 2010).
Fungi in the order Ophiostomatales (Ascomycota) are referred to as ophiostomatalean fungi. Ophiostomatalean fungi are the most common fungi associated with bark and ambrosia beetles (Wingfield et al., 1993; De Beer and Wingfield, 2013). They have been documented to assist beetles to overcome host defenses (Lieutier et al., 2009; DiGuistini et al., 2011); providing nutrition for beetles to complete their life cycle (Ayres et al., 2000; Lee et al., 2005, 2006; Bleiker and Six, 2007); assisting them in pheromone production; and protecting them from detrimental fungi (Lu et al., 2011; Cale et al., 2019). For example, Leptographium yunnanense is the most virulent fungus associated with T. yunnanensis and it can produce pathogenic toxins after synergetic infections of the host with bark beetles (Liao and Ye, 2004). Moreover, Leptographium wingfieldii is a strong pathogenic fungus associated with T. piniperda and was discovered to be able to kill 87% of Pinus sylvestris seedlings 4 weeks after inoculation (Jankowink, 2006). In addition, Ophiostoma tingens are nutritionally fungal, as the food for the larvae of Tomicus minor (Kirisits, 2004).
Ophiostomatalean fungi associated with Tomicus spp. have been reported earlier (Lieutier et al., 2015; Chang et al., 2017; Wang et al., 2019). A total of 52 ophiostomatalean fungi are associated with Tomicus species (Supplementary Table S1). Tomicus piniperda and T. minor are the most widely distributed species, and the diversity of their associated ophiostomatalean fungi is greater than that of other Tomicus species, with 30 (Jacobs et al., 2003; Zhou et al., 2004; Kim et al., 2005; Jankowiak, 2006; Jankowink, 2006; Lieutier et al., 2015) and 27 (Jankowiak, 2006; Jankowink, 2006; Linnakoski et al., 2010; Wang et al., 2019; Pan et al., 2020b) species located in Eurasia, respectively. A comparative and systematic study was conducted on ophiostomatalean fungi associated with T. yunnanensis and T. brevipilosus. At present, 15 (Zhou et al., 2000, 2013; Chang et al., 2017; Wang et al., 2019; Pan et al., 2020a,b) and 3 (Chang et al., 2017; Wang et al., 2019) species have been reported in Asia, respectively. Ophiostoma minus was documented as the dominant species associated with T. piniperda in Europe and Japan, and with T. yunnanensis in China (Wang et al., 2019). However, its internal transcribed spacer (ITS) region and Bacillus thuringiensis (BT) integrated genes were found to differ, with O. minus strains obtained from T. yunnanensis and T. piniperda being classified into two different clades. In addition, Ophiostoma canum and Ophiostoma brevipilosi were frequently isolated in association with T. minor and T. brevipilosus, respectively (Wang et al., 2019). Tomicus destruens is only distributed in the Mediterranean region of Europe (Lieutier et al., 2015), and only six ophiostomatalean fungi have been sporadically recorded to be associated with it. In addition, three ophiostomatalean fungi have been reported to be associated with T. armandii in China (Yin et al., 2015; Pan et al., 2018, 2020a,b). However, ophiostomatalean fungi associated with T. pilifer and T. puellus have not been reported.
In this study, we elucidated the diversity of ophiostomatalean fungi associated with T. pilifer and its galleries in infested P. koraiensis in northeastern China. Phylogenetic analyses of 28S rDNA datasets were also used to identify the affiliation of T. pilifer with Tomicus spp. This is the first report of ophiostomatalean fungal diversity in T. pilifer, and the results of this study provide a valuable theoretical basis for understanding the mechanisms of T. pilifer infestations.
Materials and methods
Sample collection and fungus isolation
Adult Tomicus pilifer and their bark galleries were sampled in Linjiang (N: 126°55'81″ E: 41°49″53″) and Tonghua (N: 125°45'28″ E: 41°40″34″), Jilin Province, northeast China from June to July 2020. Pinus koraiensis sampled in this study showed signs of being dead or dying. Adult beetles were individually placed in Eppendorf tubes, and their galleries were placed individually in envelope bags. Both beetles and galleries were stored at 5°C until fungal isolation. Each beetle was dismembered directly without superficial disinfection and transferred onto a 2% agar medium (20 g Biolab agar and 1,000 mL deionized water). The galleries were cut into approximately 5 × 5 mm tissue pieces, disinfected with 1.5% sodium hypochlorite (NaClO) for 1 min, rinsed with sterile water three times, and placed in 9-cm Petri dishes with 2% agar medium. All cultures were incubated in humid chambers in the dark at 25°C and inspected every day for mycelial mass. The mycelium apex was used to purify all the strains. Pure strains were transferred onto 2% malt extract agar (MEA; 20 g Biolab malt extract, 20 g Biolab agar, and 1,000 mL deionized water). All strains used in this study were deposited in the culture collection of the Forest Pathology Laboratory of the Chinese Academy of Forestry (CXY). Specimen were deposited in the China Forestry Culture Collection Center (CFCC: part of the National Infrastructure of Microbial Resources; Table 1).
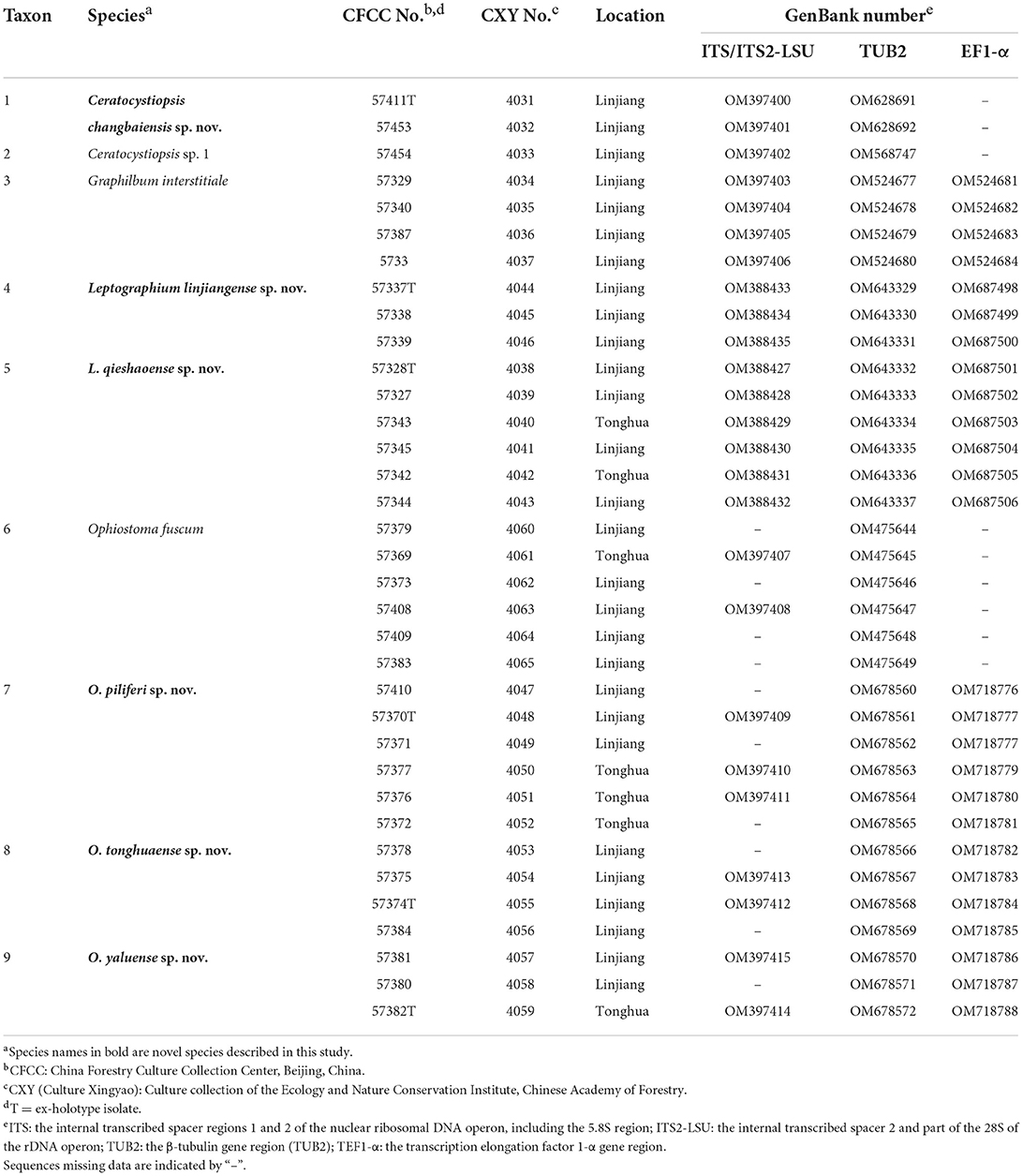
Table 1. Representative strains of ophiostomatalean fungi associated with Tomicus pilifer infesting Pinus koraiensis obtained in northeastern China.
Morphological and physiological characteristics
Beetle specimens were examined using an Olympus SZX16 stereomicroscope (Olympus, Tokyo, Japan). Based on their overall morphology, the beetles were taxonomically identified using the guidelines established by Lieutier et al. (2015). Morphological descriptions were made with strains cultivated on 2% MEA. Pure cultures were incubated for 7–15 days at 25°C in the dark. A Zeiss Axiocam 506 color digital camera (Carl Zeiss Ltd., Munich, Germany) and an Olympus BX51 (Olympus, Center Valley, PA, United States) microscope with differential interference contrast illumination were used to observe the culture morphology and reproductive structures of the fungi. Fifty measurements were repeated for each reproductive structure and are presented as (minimum–) (mean - standard deviation) (mean standard deviation) (–maximum). All data for the type specimens were deposited in MycoBank.
The optimal growth temperatures of the representative strains were determined in the dark at temperatures ranging from 5–35°C at 5°C intervals. A 5-mm-diameter agar plug from an actively growing margin of fungal colonies was set upside down in the center of a 90-mm-diameter 2% MEA plate, with five replicate plates. Colony colors on the surface and reverse side were assessed according to the color charts of Rayner (1970).
DNA extraction, amplification, and sequencing
The mycelia were collected from the actively growing colony margin of each representative strain and transferred to 2-mL Eppendorf tubes. Total genomic DNA of T. pilifer and fungi were extracted and purified using an Invisorb Spin Cell Mini Kit (DP304, Tiangen, Beijing, China) and an Invisorb Spin Plant Mini Kit (DP305, Tiangen), respectively, following the manufacturer's protocol.
The primer pair 28Sscol1/D3R was used to amplify the 28S rDNA of T. pilifer (Li et al., 2010). Four primer pairs were used for fungal nucleotide polymerase chain reaction (PCR) amplification and sequencing. The ITS1-F/ITS4 primer pair (White et al., 1990; Gardes and Bruns, 1993) was used to amplify the internal transcribed spacer (ITS) regions (ITS1 and ITS2, including the 5.8S gene); ITS3/LR3 (White et al., 1990) was used for the internal transcribed spacer 2 and part of the 28S of the rDNA operon (ITS2-LSU); Bt2a/Bt2b (Glass and Donaldson, 1995) was used for the parts of the β-tubulin (TUB2) gene; and EF1F/EF2R (Jacobs et al., 2004) was used to amplify the elongation factor 1-α (TEF1-α) gene region.
The polymerase chain reaction (PCR) mixtures had a volume of 25 μL (10 × EasyTaq Buffer [TianGen], 50 μM dNTPs, 0.1 μM of each primer, 0.75 U Taq DNA polymerase, and 1–10 ng genomic DNA), and the reactions were conducted using a thermocycler (Applied Biosystems, Foster City, CA, USA), following the manufacturer's instructions. The PCR conditions were similar to those described in the literature for primer design. PCR products were sequenced by bidirectional sequencing using a CEQ 2000 XL capillary automated sequencer (Beckman Coulter, Brea, CA, USA), and BioEdit v 7.2.0. was used for analyzing splicing patterns.
Phylogenetic analyses
Preliminary BLAST searches of the obtained ITS DNA sequences were performed in the NCBI GenBank database, and closely related authentic sequences were downloaded for further phylogenetic analyses. Alignments of the sequences were performed with MAFFT v. 7 (http://mafft.cbrc.jp/alignment/server/) using the iterative refinement method (FFT-NS-i strategy with a 200 PAM/k = 2 scoring matrix) and edited manually using MEGA v. 7.0.26. Maximum likelihood (ML) and Bayesian inference (BI) methods were implemented. Gaps were treated as the fifth character.
ML phylogenetic analyses were performed using RAxML v. 7.0.3, (Stamatakis, 2006) with the GTR-GAMMA model. Approximately 1,000 bootstrap replicates were conducted to assess the overall reliability of tree topology and bootstrap values. BI phylogenetic analyses were performed using the Markov chain Monte Carlo (MCMC) algorithm in MrBayes v. 3.1.2 (Ronquist and Huelsenbeck, 2003). Four MCMC chains were run simultaneously from a random starting tree for 5,000,000 generations, and trees were sampled every 100 generations to calculate posterior probabilities. The first 25% of the sampled trees were discarded as burn-in, and the remaining trees were used to construct majority-rule consensus trees. The topology of the phylogenetic trees was subsequently edited using FigTree v.1.4.2 and Adobe Illustrator CS6.
Results
Sample collection and isolation
In this study, 194 and 89 T. pilifer individuals were collected from P. koraiensis in Linjiang and Tonghua, Jilin Province, respectively. A total of 315 ophiostomatalean fungal strains were obtained from 62 adults and 220 tissue pieces of T. pilifer galleries at the two sites. Of these, 256 and 59 ophiostomatalean strains were isolated from T. pilifer and its galleries, respectively (Table 2). Moreover, 237 strains were obtained from 43 adults and 220 tissue pieces at Linjiang, and 78 strains were obtained from 19 adults at Tonghua.
Phylogenetic analyses
The ML and BI phylogenetic methods used in this study obtained concordant topologies with marginal discrepancies in node support values. Three T. pilifer adults were selected as representative bark beetles for the phylogenetic analyses of Tomicus based on 28S rDNA datasets (GenBank Accession No.: TOMP1 = ON042458, TOMP2 = ON042459, TOMP3 = ON042460). The 35 representative strains were separated into four genera (Ceratocystiopsis, Graphilbum, Leptographium, and Ophiostoma) and nine species (Taxa 1–9) based on DNA fragment analyses of ITS, ITS2-LSU, TUB2, and TEF1-α, revealing six new species, two known species, and one undefined species.
For Tomicus, the 28S rDNA sequences of six known pine shoot beetles and T. pilifer were compiled and analyzed (Figure 1). The 28S rDNA phylogenetic tree confirmed that the three T. pilifer sequences formed independent, well-supported clades that were closely related to T. piniperda and T. brevipilosus. The phylogenetic tree also showed that the seven known Tomicus species were divided into two large branches: T. pilifer, T. piniperda, and T. brevipilosus clustered into one branch, and T. armandii, T. destruens, T. minor, and T. yunnanensis clustered into another.
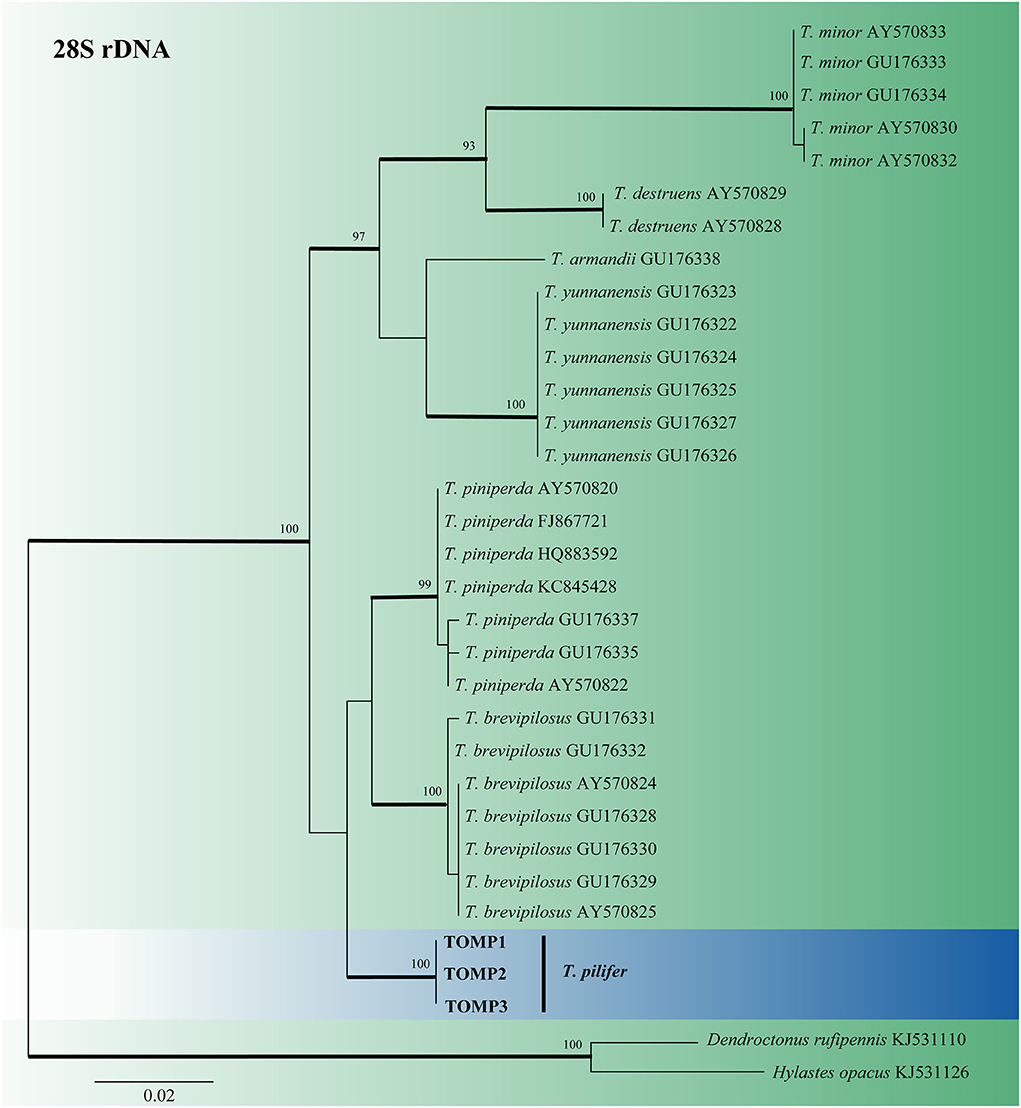
Figure 1. ML tree of Tomicus generated from the 28S rDNA sequence data. Sequences of T. pilifer obtained in this study are presented in bold typeface. The bold branches indicate posterior probability values >0.9. Bootstrap values of ML ≥ 70% are recorded at the nodes. The final alignment of 566 positions, including gaps.
For Ceratocystiopsis, the three representative strains formed two distinct clades (Figures 2, 3) based on the ITS and TUB2 datasets. In the ITS and TUB2 trees, Taxon 1 (consisting of two strains) formed a well-supported clade, closely related to Ceratocystiopsis sp. 2. Taxon 2 (single strain) was closest to, but clearly distinct from, Cop. rollhanseniana. Both ITS and TUB2 phylogenetic analyses confirmed that Taxa 1 and 2 represented undescribed species.
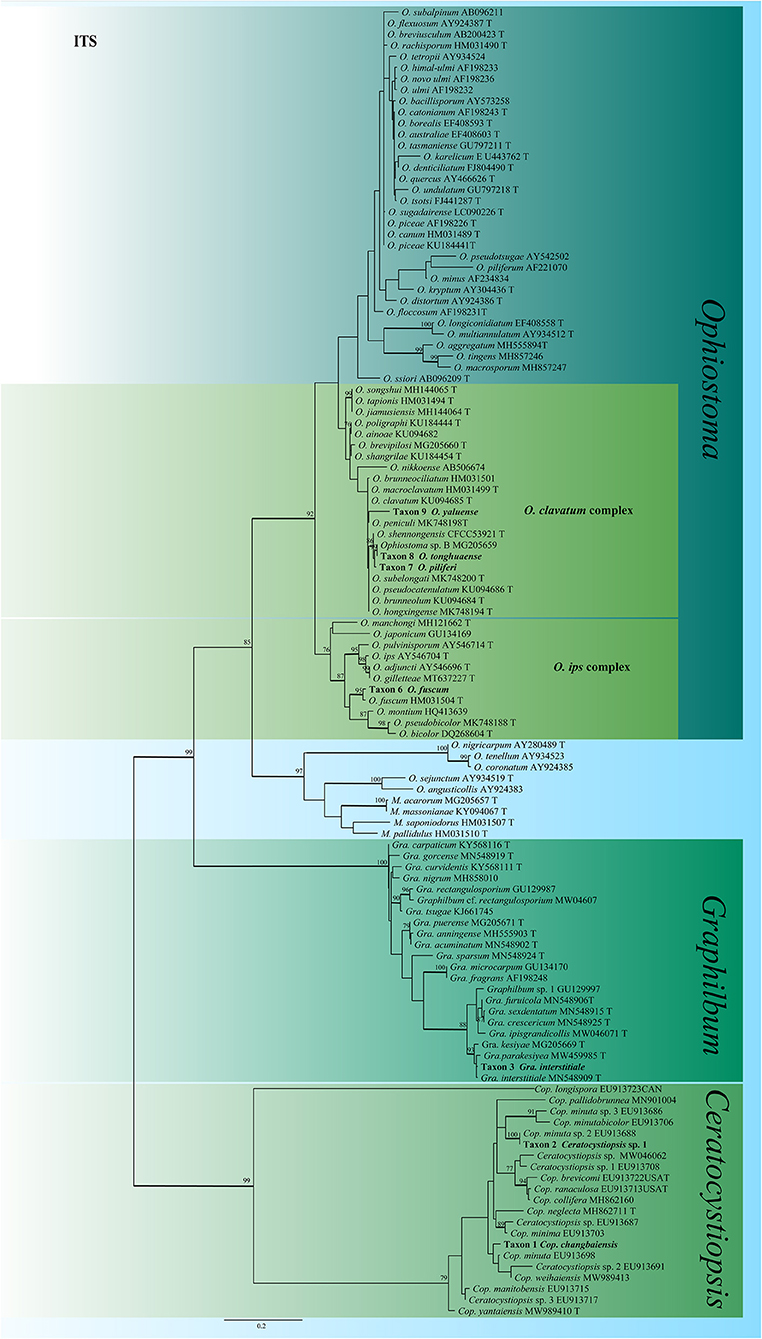
Figure 2. ML tree of Ceratocystiopsis, Graphilbum and Ophiostoma generated from the ITS sequence data. Novel sequences obtained in this study are presented in bold typeface. The bold branches indicate posterior probability values >0.9. Bootstrap values of ML ≥ 70% are recorded at the nodes. T, ex-type strains. The final alignment of 662 positions, including gaps.
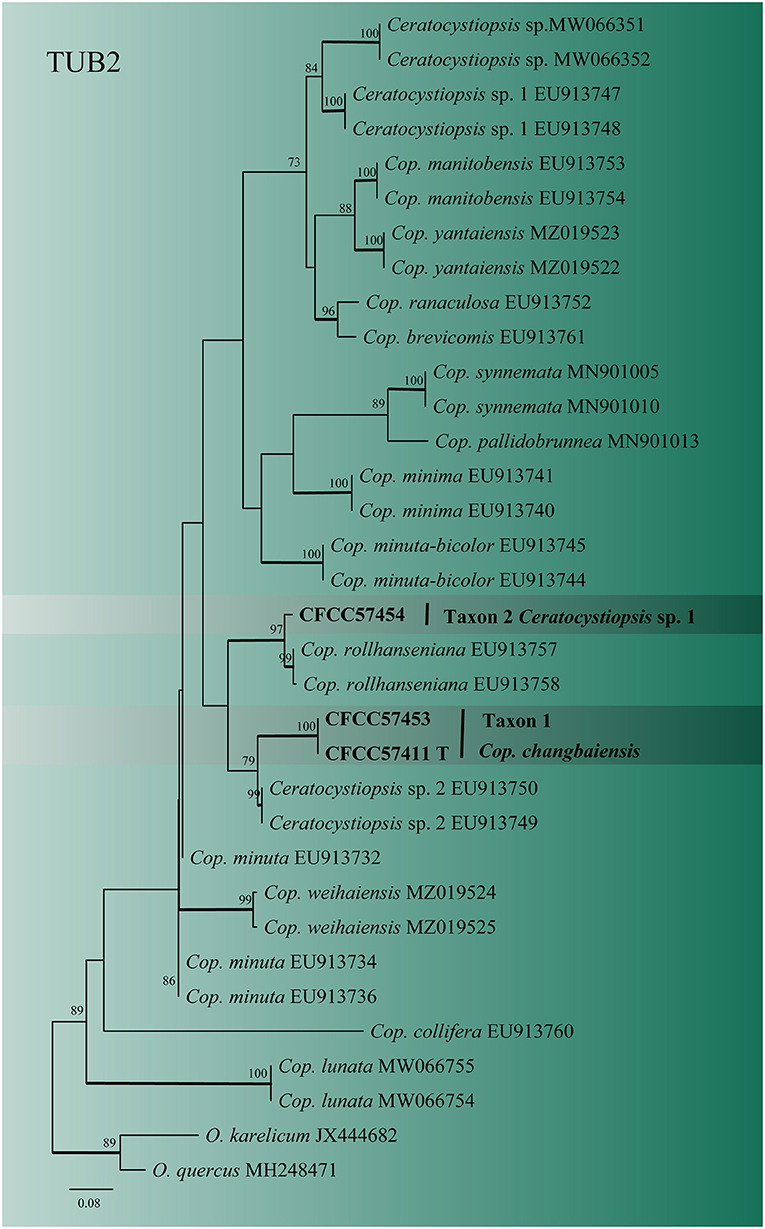
Figure 3. ML tree of Ceratocystiopsis generated from the TUB2 (Taxon 1, 2) sequence data. Novel sequences obtained in this study are presented in bold typeface. The bold branches indicate posterior probability values >0.9. Bootstrap values of ML ≥ 70% are recorded at the nodes. T, ex-type strains. The final alignment of 471 positions, including gaps.
In Graphilbum, the results from ITS, TUB2, and TEF1-α sequences suggested that Taxon 3 (consisting of four strains) emerged as conspecific to Gra. interstitiale (Figure 2 and Supplementary Figures S1, S2).
For the phylogenetic analyses of Leptographium, both the ITS2-LSU, TUB2, TEF1-α and combined (ITS2-LSU + TUB2 + TEF1-α) datasets confirmed that Taxon 4 (consisting of three strains) and Taxon 5 (consisting of six strains) were nested within the L. lundbergii complex (Figures 4, 5). Taxon 4 formed a clade related to, but distinct from, L. shansheni and L. lundbergii and peripheral to the L. lundbergii complex (Figures 4, 5 and Supplementary Figures S3, S4). Taxon 5 formed an independent, well-supported clade with close affinity to L. koreanum and L. pinicola (Figures 4, 5 and Supplementary Figures S3, S4). Thus, Taxa 4 and 5 represented two undescribed Leptographium species.
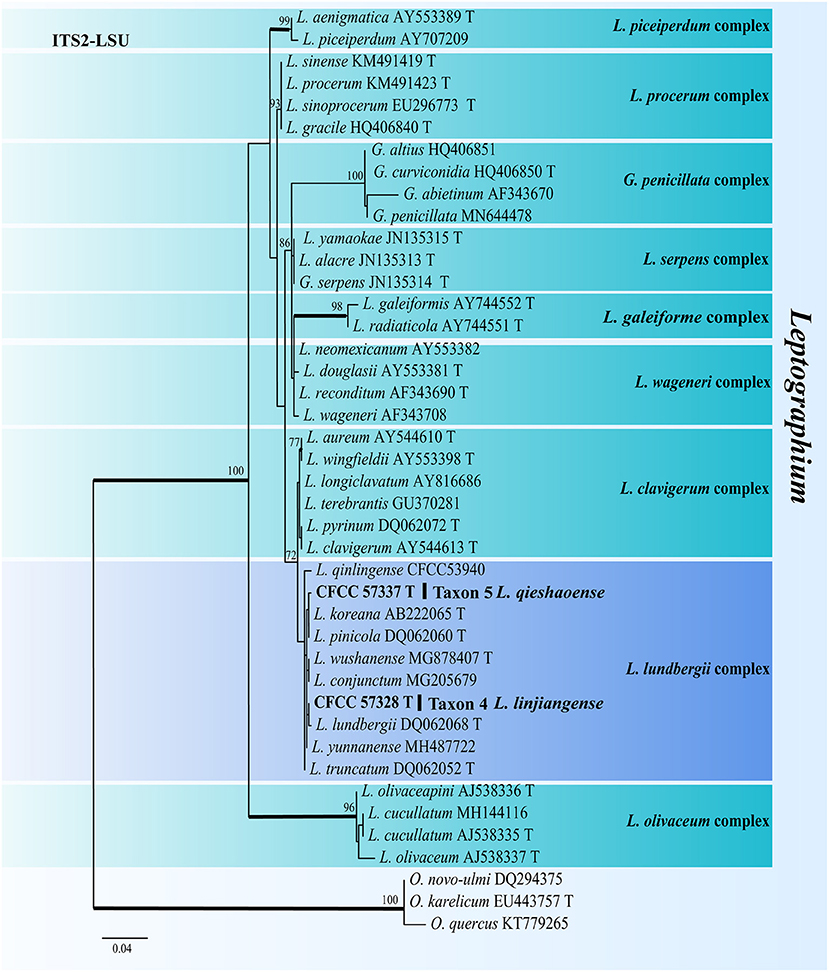
Figure 4. ML tree of Leptographium generated from the ITS2-LSU (Taxon 4, 5) sequence data. Novel sequences obtained in this study are presented in bold typeface. The bold branches indicate posterior probability values >0.9. Bootstrap values of ML ≥ 70% are recorded at the nodes. T, ex-type strains. The final alignment of 597 positions, including gaps.
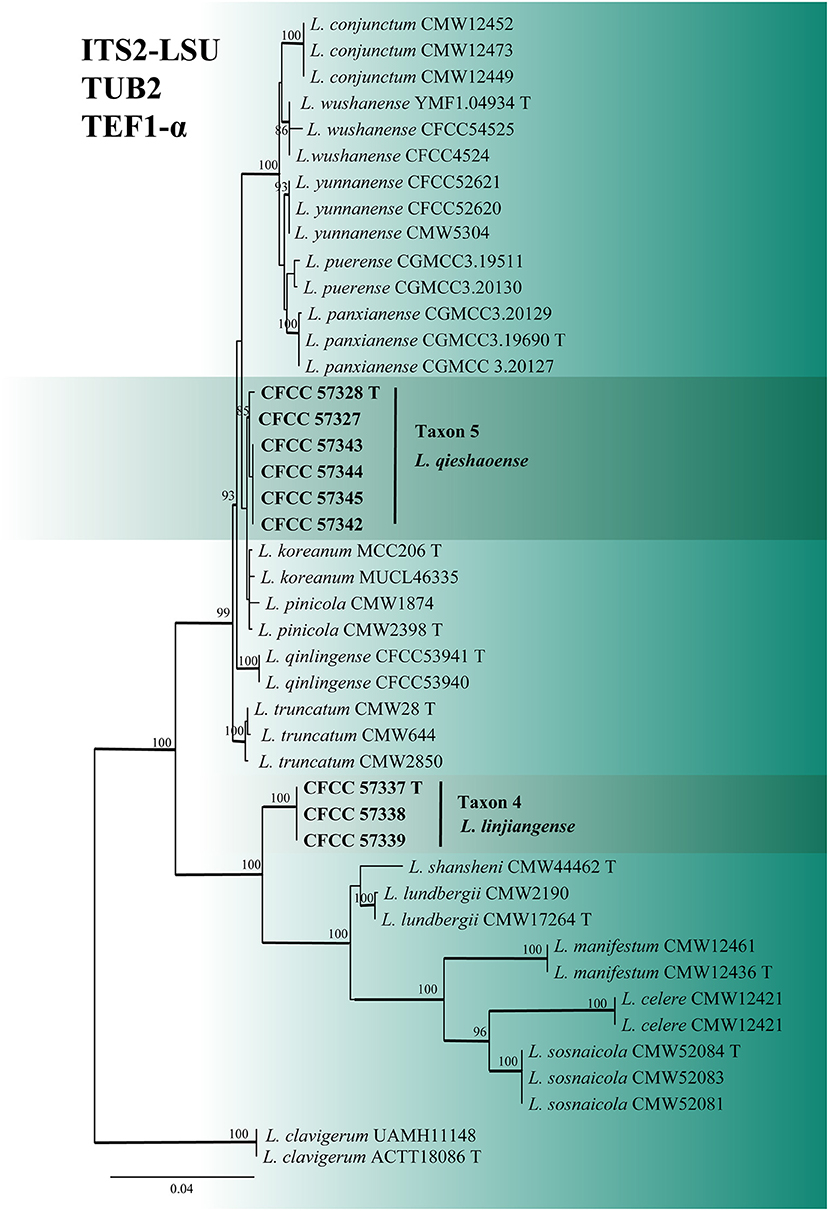
Figure 5. ML tree of L. lundbergii complex generated from the combined (ITS2-LSU + TUB2 + EF1-α) (Taxon 4, 5) sequence data. Novel sequences obtained in this study are presented in bold typeface. The bold branches indicate posterior probability values >0.9. Bootstrap values of ML ≥ 70% are recorded at the nodes. T, ex-type strains. The final alignment of 1597 positions, including gaps.
The identity of the Ophiostoma strains was analyzed using ITS, TUB2, TEF1-α and combined (TUB2 + TEF1-α) datasets. A total of 19 representative strains obtained in this study were separated into four taxa residing in the O. clavatum and O. ips complex (Figure 2). Taxon 6 (consisting of six strains) was nested in the O. ips complex (Figure 2 and Supplementary Figure S7). The ITS and TUB2 dataset analyses confirmed that the taxon was conspecific to O. fuscum. Taxa 7–9 nested within the O. clavatum complex based on ITS, TUB2, TEF1-α and combined (TUB2 + TEF1-α) dataset analyses. Taxon 7 (consisting of six strains) was positioned in the vicinity of O. shennongensis and formed a single, well-supported clade; Taxon 8 (consisting of four strains) together with Ophiostoma sp. B (Chang et al., 2017), formed single clades with high node support values. Taxon 9 (consisting of three strains) formed an independent lineage closely related to O. japonicum, clearly distinct from other species in the O. clavatum complex (Figures 2, 6 and Supplementary Figures S5, S6). These three taxa represented distinct, undescribed species.
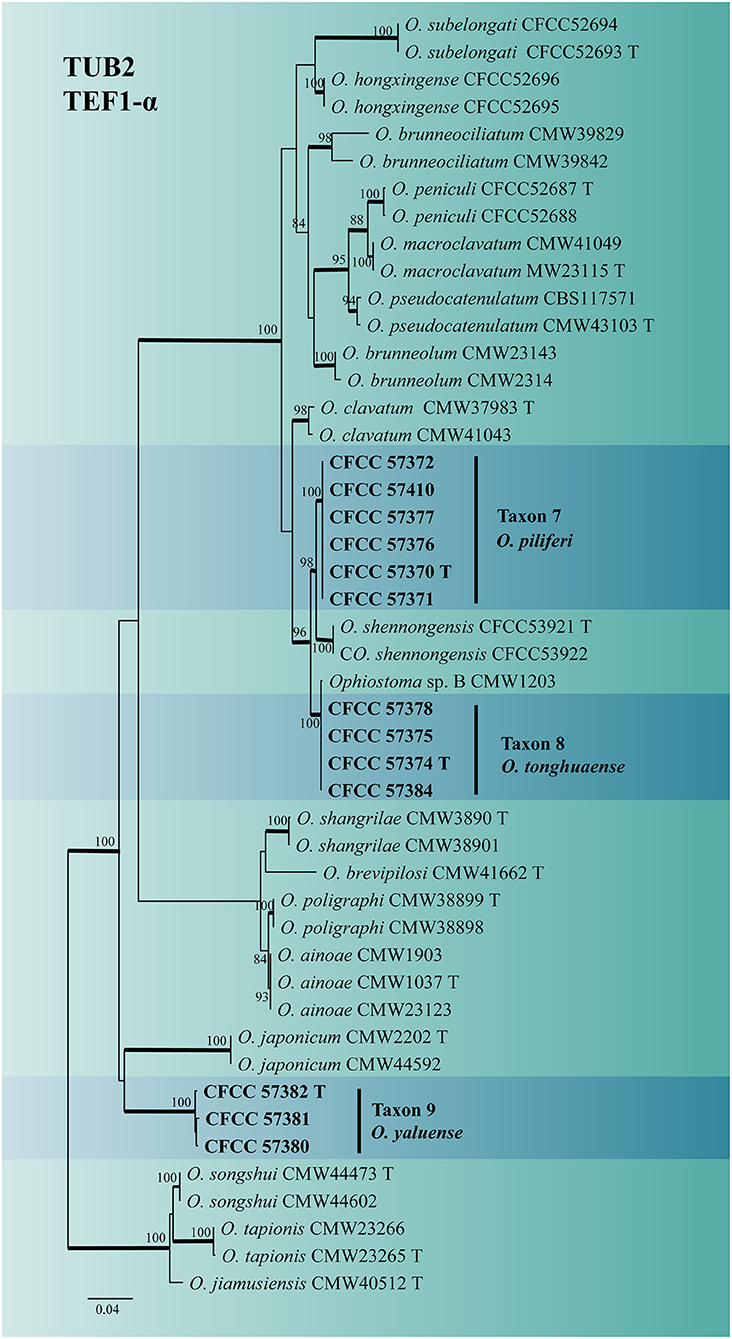
Figure 6. ML tree of O. clavatum complex generated from the combined (TUB2 + EF1-α) (Taxon 7, 8, 9) sequence data. Novel sequences obtained in this study are presented in bold typeface. The bold branches indicate posterior probability values >0.9. Bootstrap values of ML ≥ 70% are recorded at the nodes. T, ex-type strains. The final alignment of 1160 positions, including gaps.
Taxonomy
Based on the phylogenetic analyses, seven of the nine taxa isolated in this study represented undescribed species. However, only one strain was isolated from Taxon 2, and we chose a formal description until more material became available. The remaining six taxa, including one Ceratocystiopsis (Taxon 1), two Leptographium (Taxa 4 and 5), and three Ophiostoma (Taxa 7, 8, and 9) species are described as follows:
Ceratocystiopsis changbaiensis H.M. Wang and Q. Lu, sp. nov.
Mycobank MB843471
Etymology: The name refers to the Changbai Mountain, the mountain on which this species was collected.
Type: China. Jilin Province, Linjiang City, adults of T. pilifer in P. koraiensis. Jun. 2020, H.M. Wang, holotype CXY4031, culture ex-holotype CFCC57411 = CXY4031.
Description: Sexual form: unknown. Asexual forms: Hyalorhinocladiella-like. Conidiophores arise directly from aerial hyphae, simple or loosely branched, macronematous or semi-macronematous, mononematous, the ultimate branched, bearing conidiogenous cells. Conidiogenous cells are solitary, hyaline, think-walled, with rounded apex (3.22–) 7.77 – 19.15 (−28.43) × (0.7–) 0.82 – 1.24 (−1.7) μm. Conidia are hyaline, aseptate, 1-celled, smooth, oval to elliptical (2.36–) 2.73 – 3.41 (−3.94) × (0.66–) 0.73 – 1.35 (−2.95) μm (Figures 7B,C).
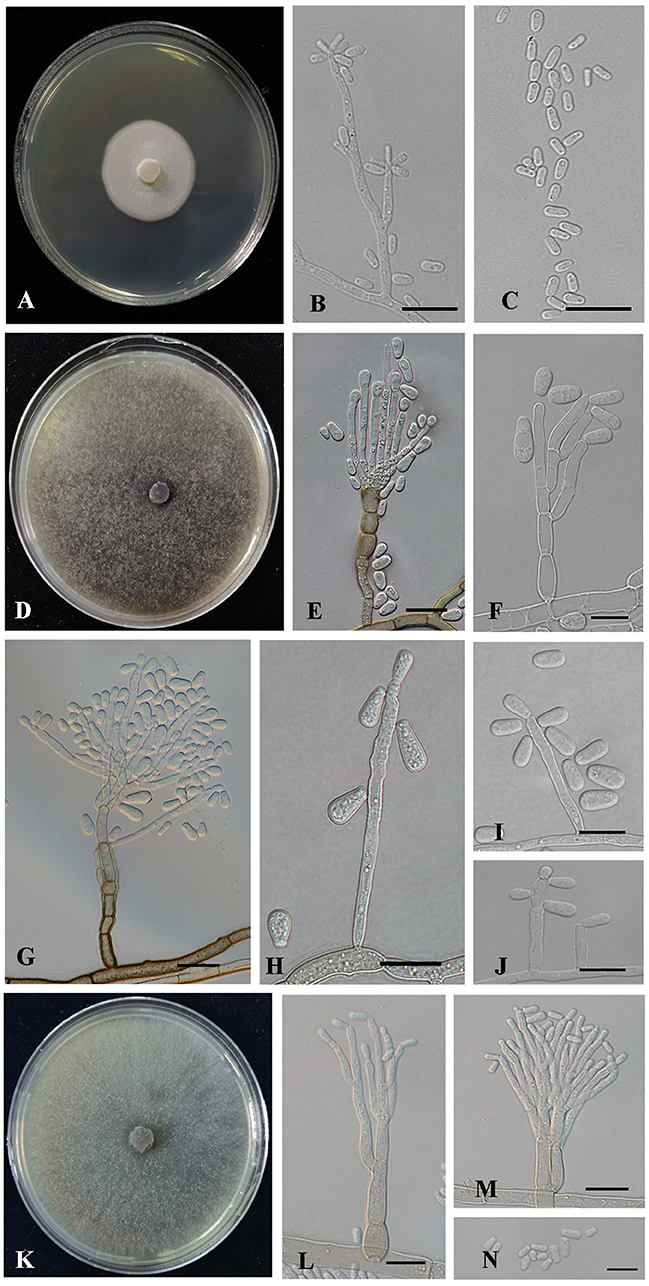
Figure 7. Morphology characteristics of Ceratocystiopsis and Leptographium new species. (A–C) Morphology of Ceratocystiopsis changbaiensis sp. nov (Taxon 1). (A) Cultures on 2% MEA 10 days after inoculation. (B,C) Hyalorhinocladiella-like asexual morph: conidiogenous cells and conidia. (D–J) Morphology of Leptographium linjiangense sp. nov (Taxon 4). (D) Cultures on 2% MEA 10 days after inoculation. (E–G) Leptographium-like asexual morph: conidiogenous cells and conidia. (H–J) Hyalorhinocladiella-like asexual morph: conidiogenous cells and conidia. (K–N) Morphology of Leptographium qieshaoense sp. nov (Taxon 5). (K) Cultures on 2% MEA 10 days after inoculation. (L–N) Leptographium-like asexual morph: conidiogenous cells and conidia. Scale bars: 20 μm (E,G), 10 μm (B,C,F,H–J,L–N).
Culture characteristics: Colonies on 2% MEA at 25°C in the dark, reaching 24.8 mm in diameter in 10 days. The colonies had smooth margins and were hyaline to white in color. Mycelia were compact with sparse aerial mycelia. The optimal growth temperature was 30°C; no growth was observed at 5°C, and litter growth occurred at 35°C (Figure 7A).
Known hosts: P. koraiensis.
Known insect vectors: T. pilifer.
Known distribution: Jilin Province, China.
Additional specimens examined: China. Jilin Province, Linjiang City, adults of T. pilifer in P. koraiensis. Jun. 2020, H.M. Wang, CFCC57453 = CXY4032.
Notes: Ceratocystiopsis changbaiensis was only characterized by a Hyalorhinocladiella-like reproductive structure on 2% MEA. Based on the phylogenetic evidence and morphological features, our strains were identified as novel species. Ceratocystiopsis changbaiensis was phylogenetically close to Ceratocystiopsis sp. 2 (Figures 1, 2). Nevertheless, the vectors and substrates of the two species were different. Ceratocystiopsis changbaiensis was isolated from T. pilifer infesting P. koraiensis in China, and Ceratocystiopsis sp. 2 was isolated from Ips subelongatus infesting Larix kaempferi in Japan (Reid and Hausner, 2010; De Beer and Wingfield, 2013).
Leptographium linjiangense H.M. Wang and Q. Lu, sp. nov.
Mycobank MB843473
Etymology: The name refers to Linjiang City, where this fungus was collected.
Type: China. Jilin Province, Linjiang City, adults of T. pilifer in P. koraiensis. Jun. 2020, H.M. Wang, holotype CXY4044, culture ex-holotype CFCC57337 = CXY4044.
Description: Sexual forms: unknown. Asexual forms: Leptographium-like and Hyalorhinocladiella-like.
Leptographium-like: Conidiophores occur singly without rhizoid-like structures and arise from the superficial mycelium, erect, macronematous, mononematous, (44.39–) 79.14 – 194.62 (−281.51) μm long. The stipes are simple, constricted at septa, cylindrical, olivaceous at the base, 1–7 septate, (6.06–) 21.11 – 78.77 (−120.26) × (3.35–) 4.16 – 8.16 (−11.31) μm. Conidiogenous apparatus with 1–3 series of cylindrical branches. The primary branches are hyaline to pale olivaceous in color, smooth, cylindrical, 2–7 branch, (6.06–) 9.32 – 29.18 (−60.98) × (2.81–) 3.61 – 7.63 (−12.29) μm. Conidiogenous cells are discrete, cylindrical, (12.2–) 17 – 34.04 (−56) × (2.38–) 2.85 – 3.75 (−4.21) μm. Conidia are 1-celled, hyaline, aseptate, globose, and elliptical to drop with truncate bases (3.52–) 5.76 – 10.76 (−14.26) × (2.43–) 2.99 – 4.87 (−6.75) μm.
Hyalorhinocladiella-like: Conidiophores arise directly from aerial hyphae, reduced to conidiogenous cells, macronematous or semi-macronematous, and mononematous. Conidiogenous cells are solitary, simple, hyaline to pale brown in color, aseptate, thin-walled, and variable in length (13.44–) 19.06 – 54.52 (−90.96) × (1.78–) 2.37 – 3.71 (−4.2) μm. Conidia are hyaline, smooth, oval to elliptical with truncate bases, 1-celled, aseptate, (4.93–) 6.29 – 9.39 (−12) × (2.33–) 3.02 – 4.48 (−5.68) μm (Figures 7E–J).
Culture characteristics: Colonies were cultured on 2% MEA at 25°C in the dark, reaching 90 mm in diameter in 7 days. Hyphae were submerged with few aerials, hyaline at first, later becoming dark brown. The mycelia were superficial. The optimal growth temperature was 30°C; no growth was observed at 5°C, and little growth was seen at 35°C (Figure 7D).
Known hosts: P. koraiensis.
Known insect vectors: T. pilifer.
Known distribution: Jilin Province, China.
Additional specimens examined: China. Jilin Province, Linjiang City, adults of T. pilifer in P. koraiensis. Jun. 2020, H.M. Wang, CFCC57338 = CXY4045, CFCC57339 = CXY4046.
Notes: Leptographium linjiangense produced Leptographium-like and Hyalorhinocladiella-like asexual states in vitro. The species was phylogenetically closest to L. shansheni based on TUB2, TEF1-α, and combined (ITS2 + TUB2 + TEF1-α) trees (Figure 4 and Supplementary Figures S3, S4). Leptographium linjiangense produced two asexual states, whereas L. shansheni only produced Leptographium-like asexual state. The stipes and primary branches of the Leptographium-like state between L. linjiangense and L. shansheni differed, as the former is longer than the latter (Stipes: 1–7 septate, 44.39–281.51 μm vs. 1 septate, 9–23 μm. Primary branches: primarily 6.06–60.98 μm vs. mostly 6.5–27.5 μm; Chang et al., 2019). The optimal growth temperatures for L. linjiangense and L. shansheni were 30 and 25°C, respectively, on 2% MEA. Leptographium linjiangense exhibited little growth at 35°C, whereas L. shansheni did not grow at 35°C. Furthermore, L. linjiangense and L. shansheni were associated with T. pilifer and I. typographus, respectively.
Leptographium qieshaoense H.M. Wang and Q. Lu, sp. nov.
Mycobank MB843474
Etymology: The name refers to the Chinese word for pine shoot beetles, “qieshao”.
Type: China. Jilin Province, Linjiang City, adults of T. pilifer in P. koraiensis. Jun. 2020, H.M. Wang, holotype CXY4038, culture ex-holotype CFCC57328 = CXY4038.
Description: Sexual form: unknown. Asexual forms: Leptographium-like. Conidiophores occur singly without rhizoid-like structures and arise from the superficial mycelium, erect, macronematous, mononematous, (43.68–) 52.77 – 97.43 (−166.63) μm long. The stipes are simple, constricted at septa, cylindrical, and pale olivaceous in color at the base, 1–6 septate, (3.89–) 17.44 – 43.68 (−67.33) × (1.94–) 2.69 – 4.53 (−6.73) μm. Conidiogenous apparatus with 2–3 series of cylindrical branches; primary branches hyaline to pale olivaceous, smooth, cylindrical, 2–3 branches, (7.44–) 9.96 – 14.7 (−19.41) × (1.97–) 2.28 – 3.34 (−4.05) μm. Conidiogenous cells are discrete, 1–3 per branch, cylindrical, (9.45–) 10.86 – 17.5 (−23.9) × (0.87–) 1.43 – 2.15 (−2.42) μm. Conidia are 1-celled, hyaline, cylindrical to obovoid with truncate bases, aseptate (3.61–) 4.46 – 6.24 (−7.51) × (1.63–) 1.87 – 2.87 (−3.55) μm (Figures 7L–N).
Culture characteristics: Colonies on 2% MEA at 25°C in the dark, reaching 90 mm in diameter in 7 days, with radial colony margins, initially hyaline, later becoming greenish-olivaceous to olivaceous, with abundant aerial mycelia. The optimal growth temperature was 30°C; no growth was observed at 5°C, and little growth was seen at 35°C (Figure 7K).
Known hosts: P. koraiensis.
Known insect vectors: T. pilifer.
Known distribution: Jilin Province, China.
Additional specimens examined: China. Jilin Province, Linjiang City, T. pilifer adults found in P. koraiensis. Jun. 2020, H.M. Wang, CFCC57327 = CXY4039, CFCC57345 = CXY4041, CFCC57344 = CXY4043; China. Jilin Province, Tonghua City, adults of T. pilifer in P. koraiensis. Jul. 2020, H.M. Wang, CFCC57343 = CXY4040, CFCC57342 = CXY4042.
Notes: Leptographium qieshaoense had a single asexual state and it is Leptographium-like. It was closely related to L. koreanum and L. pinicola based on ITS2-LSU and combined (ITS2 + TUB2 + TEF1-α) phylogenetic analyses (Figures 4, 5). However, L. qieshaoense and L. koreanum differed in their conidia, conidiogenous cells, and stipes of asexual states. The conidiogenous cells of the former were longer than those of the latter (primarily 9.45–23.9 μm vs. mostly 6–11 μm). In contrast, the stipes of L. qieshaoense were shorter than those L. koreanum (3.89–67.33 vs. 35–227 μm), and the conidia size range of L. qieshaoense was shorter than that of L. koreanum (3.61–7.51 μm vs. 3–10 μm; Kim et al., 2005). The conidiogenous cells of L. qieshaoense and L. pinicola were different. The former was shorter than the latter (primarily 9.45–23.9 μm vs. mostly 11–48 μm; Jacobs et al., 2005). Moreover, the optimal temperatures of L. qieshaoense, L. koreanum, and L. pinicola were different, 30, 25, and 25 °C, respectively. The insect vectors, hosts, and collection sites for the three species were different. Leptographium qieshaoense is associated with T. pilifer infesting P. koraiensis in China; L. koreanum is associated with T. piniperda, Hylurgops interstitialis, Hylastes paralleus, and H. plumbeus infesting Pinus densiflora and P. koraiensis in Korea and Japan; L. pinicola is associated with Hylastes infesting P. resinosas and P. densiflora in Canada and Japan (Jacobs et al., 2005; Kim et al., 2005; Masuya et al., 2005).
Ophiostoma piliferi H.M. Wang and Q. Lu, sp. nov.
Mycobank MB843475
Type: China. Jilin Province, Linjiang City, adults of T. pilifer in P. koraiensis. Jun. 2020, H.M. Wang, holotype CXY4048, culture ex-holotype CFCC57370 = CXY4048.
Etymology: The name refers to the bark beetle vector of T. pilifer.
Description: Sexual form: unknown. Asexual forms: Hyalorhinocladiella-like.
Hyalorhinocladiella-like: Conidiophores semi-macronematous, mononematous, arise directly from aerial hyphae, simple or loosely branched, the ultimate branches bearing conidiogenous cells. Conidiogenous cells are hyaline, smooth, thin-walled, aseptate (5.44–) 12.19 – 30.73 (−55.74) × (1. 12–) 1.41 – 2.08 (−3.13) μm. Conidia are hyaline, smooth, 1-celled, clavate to elliptical with pointed bases and rounded apices, aseptate, (2.81–) 4.07 – 5.99 (−8.76) × (1.2–) 1.62 – 2.38 (−3.41) μm (Figures 8B–E).
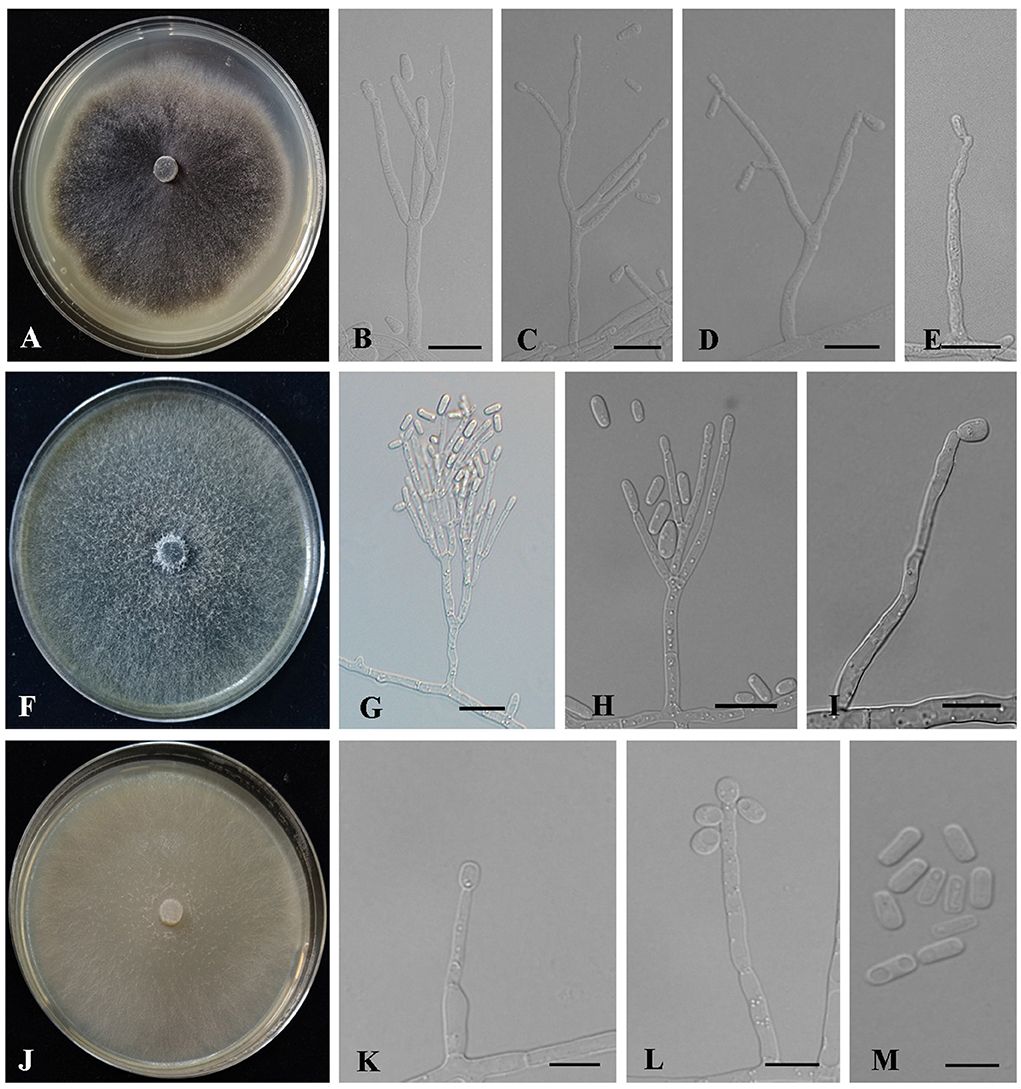
Figure 8. Morphology characteristics of Ophiostoma new species. (A–E) Morphology of Ophiostoma piliferi sp. nov (Taxon 7). (A) Cultures on 2% MEA 10 days after inoculation. (B–E) Hyalorhinocladiella-like asexual morph: conidiogenous cells and conidia. (F–I) Morphology of Ophiostoma tonghuaense sp. nov (Taxon 8). (F) Cultures on 2% MEA 10 days after inoculation. (G,H) Leptographium-like asexual morph: conidiogenous cells and conidia. (I) Hyalorhinocladiella-like asexual morph: conidiogenous cells and conidia. (J–M) Morphology of Ophiostoma tonghuaense sp. nov (Taxon 9). (J) Cultures on 2% MEA 10 days after inoculation. (K–M) Hyalorhinocladiella-like asexual morph: conidiogenous cells and conidia. Scale bars: 20 μm (G); 10 μm (B–E,H–I,K–M).
Culture characteristics: Colonies on 2% MEA at 25°C in the dark, reaching 65 mm in diameter in 10 days, with white hyphae appressed to flocculose, dark brown to black, and anomalous mycelium margins. The optimal growth temperature was 30°C. No growth was observed at 5°C, and little growth was seen at 35°C (Figure 8A).
Known hosts: P. koraiensis.
Known insect vectors: T. pilifer.
Known distribution: Jilin Province, China.
Additional specimens examined: China. Jilin Province, Linjiang City, adults of T. pilifer in P. koraiensis. Jun. 2020, H.M. Wang, CFCC57410 = CXY4047, CFCC57371 = CXY4049; China. Jilin Province, Tonghua City, adults of T. pilifer in P. koraiensis. Jul. 2020, H.M. Wang, CFCC57377 = CXY4050, CFCC57376 = CXY4051, CFCC57372 = CXY4052.
Notes: Ophiostoma piliferi produced Hyalorhinocladiella-like asexual states in 2% MEA. ITS and combined (TUB2 + TEF1-α) phylogenetic analyses showed that O. piliferi is closely related to O. shennongensis, O. tonghuaense, and Ophiostoma sp. 2 (Figures 2, 6). (cf. below for O. tonghuaense).
Ophiostoma tonghuaense H.M. Wang and Q. Lu, sp. nov.
Mycobank MB843477
Type: China, Jilin Province, Tonghua City, adults of T. pilifer in P. koraiensis. Jun. 2020, H.M. Wang, holotype CXY4053, culture ex-holotype CFCC57374 = CXY4055.
Etymology: The name refers to Tonghua City, where this fungus was collected.
Description: Sexual form: unknown. Asexual forms: Leptographium-like and Hyalorhinocladiella-like.
Leptographium-like: Conidiophores occurring singly arise from the superficial mycelium, without rhizoid-like structures, macronematous, mononematous, (28.71–) 42.39 – 93.95 (−142.14) μm long. The stipes are simple, hyaline, not constricted at septa, cylindrical, 1–4 septate, (7.79–) 10.73 – 39.51 (−75.31) × (1.98–) 2.48 – 3.98 (−5.55) μm. Conidiogenous apparatus with 2–3 series of cylindrical branches. The primary branches are cylindrical, hyaline, smooth, 2–3 branches, (6.12–) 9.1 – 18.86 (−27.49) × (1.63–) 2 – 3.36 (−4.84) μm. Conidiogenous cells are discrete, hyaline, smooth, cylindrical, (9.41–) 12.09 – 24.79 (−45.62) × (1.55–) 1.79 – 2.65 (−3.23) μm. Conidia are 1-celled, hyaline, clavate to elliptical, aseptate, (9.41–) 12.09 – 24.79 (−45.62) × (1.55–) 1.79 – 2.65 (−3.23) μm.
Hyalorhinocladiella-like: Conidiophores arise directly from aerial hyphae, solitary or loosely branched, macronematous or semi-macronematous, mononematous, ultimate branched bearing conidiogenous cells. Conidiogenous cells are simple, hyaline to pale brown, aseptate or sparsely septate, thin-walled, and variable in length (12.43–) 13.66 – 56.86 (−81.78) × (1.43–) 1.68 – 3.1 (−3.98) μm. Conidia are hyaline, 1-celled, smooth, aseptate, oval to elliptical with obtuse ends, (2.48–) 3.93 – 6.21 (−7.71) × (1.25–) 1.58 – 2.6 (−3.69) μm (Figures 8G–I).
Culture characteristics: Colonies on 2% MEA at 25°C in the dark, reaching 90 mm in diameter in 5 days. The colony margin was anomalous, with abundant aerial mycelia, flocculose, and hyaline initially, becoming greenish-olivaceous to olivaceous. The optimal growth temperature was 30°C. No growth was observed at 5°C, and litter growth was achieved at 35°C (Figure 8F).
Known hosts: P. koraiensis.
Known insect vectors: T. pilifer.
Known distribution: Jilin Province, China.
Additional specimens examined: China. Jilin Province, Linjiang City, adults of T. pilifer in P. koraiensis. Jun. 2020, H.M. Wang, CFCC57378 = CXY4053, CFCC57375 = CXY4054, CFCC57384 = CXY4056.
Notes: Ophiostoma tonghuaense produced Leptographium-like and Hyalorhinocladiella-like asexual states in 2% MEA. ITS and combined (TUB2 + TEF1-α) phylogenetic analyses showed that O. tonghuaense is grouped with Ophiostoma sp. B (Chang et al., 2017) and is closely related to O. piliferi and O. shennongense (Figures 2, 6). Ophiostoma sp. B was obtained from only one strain isolated from the gallery of an unknown beetle infesting Pinus semaonensis; this species has not been formally described in previous studies (Chang et al., 2017). Therefore, this did not influence the formal description of O. tonghuaense.
Ophiostoma tonghuaense, O. shennongense, and O. piliferi differed in their asexual states. Ophiostoma tonghuaense produces Leptographium-like and Hyalorhinocladiella-like asexual states; O. shennongense and O. piliferi produces a single Hyalorhinocladiella-like asexual state (Figure 8; Wang et al., 2022). Among the three species, the growth rate of O. tonghuaense at 25°C was the fastest (90 mm in diameter in 5 days), followed by O. shennongense (70 mm in diameter in 8 days) and O. piliferi (65 mm in diameter in 10 days).
The culture color of O. tonghuaense, O. piliferi, and O. shennongense on 2% MEA at 25°C was greenish-olivaceous to olivaceous, dark brown to black, and pale olivaceous, respectively. Although the geographic distribution of these three Ophiostoma species overlaps, their hosts and vectors are different. Ophiostoma tonghuaense and O. piliferi are associated with T. pilifer infesting P. koraiensis, whereas O. shennongense is associated with Dendroctonus armandi infesting P. armandii.
Ophiostoma yaluense H.M. Wang and Q. Lu, sp. nov.
Mycobank MB843479
Type: China, Jilin Province, Tonghua City, adults of T. pilifer in P. koraiensis. Jun. 2020, H.M. Wang, holotype CXY4059, culture ex-holotype CFCC57382 = CXY4059.
Etymology: The name refers to the Yalujiang river where this species was collected.
Description: Sexual form: unknown. Asexual forms: Hyalorhinocladiella-like. Conidiophores are solitary branched, arise directly from aerial hyphae, and are reduced to conidiogenous cells, macronematous or semi-macronematous, mononematous, simple, hyaline, aseptate, sparsely septate, thin-walled, and variable in length (7.04–) (16.8) – (62.52) (−98.4) × (1.37–) (1.85) – (2.57) (−2.89) μm. Conidia are aseptate, hyaline, smooth, 1-celled, globose, oval to elliptical, with truncate bases (2.7–) (3.64) – (4.8) (−5.58) × (1.5–) (1.82) – (2.72) (−3.35) μm (Figures 8K–M).
Culture characteristics: Colonies cultured on 2% MEA at 25°C in the dark reached 90 mm in diameter in 9 days. The colonies had smooth margins and were superficial and hyaline in color. The optimal growth temperature was 25°C, and slow growth was observed at 5 and 35°C (Figure 8J).
Known hosts: P. koraiensis.
Known insect vectors: T. pilifer.
Known distribution: Jilin Province, China.
Additional specimens examined: China. Jilin Province, Linjiang City, adults of T. pilifer in P. koraiensis. Jun. 2020, H.M. Wang, CFCC57381 = CXY4057, CFCC57380 = CXY4058.
Notes: Ophiostoma yaluense was found only in an asexual, Hyalorhinocladiella-like state. Phylogenetic analysis of the ITS and combined (TUB2 + TEF1-α) regions revealed that O. yaluense forms a separate lineage with high node support values (Figures 2, 6). Moreover, this species is phylogenetically closely related to O. japonicum based on TUB2 + TEF1-α phylogenetic data; however, the asexual of the two species were different. Ophiostoma japonicum produced Graphilbum-like asexual states (Yamaoka et al., 1997). In addition, vectors and hosts of both species were different. Ophiostoma yaluense was associated with T. pilifer infesting P. koraiensis and O. japonicum was associated with I. typographus japonicus infesting P. jezoensis (Japan) and P. koraiensis (China).
Discussion
Herein, a total of 315 ophiostomatalean fungal strains representing nine taxa were isolated primarily from the adults of T. pilifer and secondarily from their galleries found in infested P. koraiensis trees in northeastern China. The results of this study revealed six new species (Cop. changbaiensis, L. qieshaoense, L. linjiangense, O. piliferi, O. tonghuaense, and O. yaluense), two known species (Gra. interstitiale and O. fuscum), and one undefined species (Ceratocystiopsis sp. 1). Graphilbum interstitiale and O. fuscum have not been previously reported in China. In this study, although the six novel species belonged to three different genera, five of them exhibited optimal growth at 30°C, no growth at 0.5°C, and limited growth at 35°C. Meanwhile, the optimal growth of O. yaluense was recorded at 25°C. In addition, we determined the taxonomic status of T. pilifer based on 28S rDNA phylogenetic analysis (Figure 1), which provides a molecular basis for subsequent studies on the classification of Tomicus species.
The new species identified in this study can be easily distinguished from other known species by integrating phylogenetic analysis, and examining their physiological and morphological characteristics. Tomicus pilifer and ophiostomatalean fungi obtained in this study form a new association of beetle-fungal. Therefore, the following discussion focuses on the association between fungi diversity and beetles.
Ophiostoma piliferi was the most frequently isolated ophiostomatalean fungus associated with T. pilifer in our study (124 out of the 315 strains), accounting for 39.37% (Table 2). Ophiostoma piliferi and two other new species, O. tonghuaense and O. yaluense were classified in the O. clavatum complex (Figure 2). Noteworthily, O. tonghuaense and O. yaluense included 19 and 6 strains, accounting for 6.03% and 1.9% of the total ophiostomatalean fungal strains, respectively.
The fungal diversity of the O. clavatum complex was high, and a total of 22 taxa have been documented, including the three new species obtained in this study (Linnakoski et al., 2016; De beer et al., 2022; Wang et al., 2022; Figure 6 and Supplementary Figures S5, S6). All species of the O. clavatum complex are associated with bark beetles infesting pine or spruce. In addition, multiple new ophiostomatalean fungi of the O. clavatum complex have often been simultaneously isolated from identical bark beetles or hosts. For example, O. jiamusiensis, O. songshui, O. ainoae, and O. brunneolum are associated with I. typographus (Chang et al., 2017). Ophiostoma hongxingense, O. subelongati, and O. peniculi were obtained from I. subelongatus infesting L. gmelinii (Wang et al., 2020). Ophiostoma brevipilosi was the first species in the O. clavatum complex associated with Tomicus (Chang et al., 2017). Subsequently, this study isolated and identified three new species of O. clavatum complex associated with T. pilifer.
In our study, the second most abundant species was L. qieshaoense (113 out of the 315 strains), accounting for 35.87% (Table 2), which forms part of the L. lundbergii complex (Figure 4). In addition, L. linjiangense was grouped in this complex, with a total of 12 strains, accounting for 3.81%. Leptographium lundbergii is a species of Leptographium described in 1927 (Jacobs et al., 2005). The L. lundbergii complex was defined by Jacobs et al. (2005), and is currently composed of 17 taxa, including the two new species obtained in this study (Chang et al., 2019; Pan et al., 2020a,b; De beer et al., 2022; Wang et al., 2022; Figure 5 and Supplementary Figures S3, S4). All species documented in this complex were isolated from conifers (Jacobs et al., 2005; Linnakoski et al., 2012b; Chang et al., 2019).
Regarding the two newly recorded species, Gra. interstitiale was first isolated from H. interstitialis infesting Pinus sylvestris in the far east of Russia (Jankowiak et al., 2020), whereas O. fuscum was first obtained from I. typographus and Pityogenes chalcographus infesting P. abies in Russia (Linnakoski et al., 2010). In this study, 26 and 9 strains of Gra. interstitiale and O. fuscum were collected, accounting for 8.25% and 3.81% of the strains, respectively. Despite the small number of strains, Gra. interstitiale was the third most abundant ophiostomatalean fungus collected (Table 2). These two newly recorded species were first reported to be associated with Tomicus. Many new and newly recorded species were obtained in this study, indicating that a large number of ophiostomatalean fungi associated with T. pilifer are yet to be identified.
Thus far, 61 ophiostomatalean fungi (including the nine species obtained in this study) were reported to be associated with seven Tomicus species, among which T. piniperda had the highest diversity of ophiostomatalean fungi, followed by T. minor, T. yunnanense, T. pilifer, and T. destruens, whereas T. brevipilosus and T. armandii had the lowest diversity with only three species (Table 1 and Supplementary Table S1). This phenomenon may be explained by the wider distribution of T. piniperda and T. minor as compared with other Tomicus species, spanning various climatic regions and diverse hosts (Masuya et al., 1999a,b; Jacobs and Wingfield, 2001; Jacobs et al., 2003; Jankowiak, 2006; Jankowink, 2006; Linnakoski et al., 2010). In addition, the study on ophiostomatalean fungi associated T. piniperda and T. minor began earlier, and the samples analyzed were obtained from a larger area (Mathiesen-Kaarik, 1953; Masuya et al., 1999a,b). Therefore, the documented ophiostomatalean fungi associated T. piniperda and T. minor had the highest diversity among the seven beetles. In contrast, the other Tomicus species were found to only have a few associated ophiostomatalean fungi.
An increasing number of studies have shown that ophiostomatalean fungal symbiose with bark and ambrosia beetles (Biedermann and Vega, 2020). This study determined the diversity of ophiostomatalean fungi associated with T. pilifer-infested P. koraiensis tissues in northeastern China. Fungal classification is the basis for the research on fungal function. Therefore, our findings provide basic knowledge for understanding the relationship between bark beetles and ophiostomatalean fungi and aid in designing strategies for the management of Tomicus.
Data availability statement
The datasets presented in this study can be found in online repositories. The names of the repository/repositories and accession number(s) can be found in the article/Supplementary material.
Author contributions
HW designed the study, analyzed the data and contributed to experiment design. HW and FY collected the samples. HW and CL performed DNA extraction and PCR amplification. QL and HW wrote the manuscript. QL and D-HY reviewed and approved the final manuscript. All authors have read and agreed to the published version of the manuscript. All authors contributed to the article and approved the submitted version.
Funding
This study was supported by the National Natural Science Foundation of China (Project No. 32071769).
Acknowledgments
We would like to thank Dan Wang from Jilin Province Forest Pest Control Station and Lin Ma from Linjiang Forestry and Grassland Administration for their help in sample collection.
Conflict of interest
The authors declare that the research was conducted in the absence of any commercial or financial relationships that could be construed as a potential conflict of interest.
Publisher's note
All claims expressed in this article are solely those of the authors and do not necessarily represent those of their affiliated organizations, or those of the publisher, the editors and the reviewers. Any product that may be evaluated in this article, or claim that may be made by its manufacturer, is not guaranteed or endorsed by the publisher.
Supplementary material
The Supplementary Material for this article can be found online at: https://www.frontiersin.org/articles/10.3389/fmicb.2022.919302/full#supplementary-material
Supplementary Table S1. Diversity of ophiostomatalean fungi associated with Tomicus spp.
Supplementary Table S2. Reference sequences which are involved in phylogenetic tree in this study.
Supplementary Figure S1. ML tree of Graphilbum generated from the TUB2 sequence data. Novel sequences obtained in this study are presented in bold typeface. The bold branches indicate posterior probability values >0.9. Bootstrap values of ML ≥ 70% are recorded at the nodes. T, ex-type strains. The final alignment of 501 positions, including gaps.
Supplementary Figure S2. ML tree of Graphilbum generated from the EF1-α sequence data. Novel sequences obtained in this study are presented in bold typeface. The bold branches indicate posterior probability values >0.9. Bootstrap values of ML ≥ 70% are recorded at the nodes. T, ex-type strains. The final alignment of 704 positions, including gaps.
Supplementary Figure S3. ML tree of L. lundbergii complex generated from the TUB2 (Taxon 4, 5) sequence data. Novel sequences obtained in this study are presented in bold typeface. The bold branches indicate posterior probability values >0.9. Bootstrap values of ML ≥ 70% are recorded at the nodes. T, ex-type strains. The final alignment of 369 positions, including gaps.
Supplementary Figure S4. ML tree of L. lundbergii complex generated from the EF1-α (Taxon 4, 5) sequence data. Novel sequences obtained in this study are presented in bold typeface. The bold branches indicate posterior probability values >0.9. Bootstrap values of ML ≥ 70% are recorded at the nodes. T, ex-type strains. The final alignment of 635 positions, including gaps.
Supplementary Figure S5. ML tree of O. clavatum complex generated from the TUB2 (Taxon 7, 8, 9) sequence data. Novel sequences obtained in this study are presented in bold typeface. The bold branches indicate posterior probability values >0.9. Bootstrap values of ML ≥ 70% are recorded at the nodes. T, ex-type strains. The final alignment of 388 positions, including gaps.
Supplementary Figure S6. ML tree of O. clavatum complex generated from the EF1-α (Taxon 7, 8, 9) sequence data. Novel sequences obtained in this study are presented in bold typeface. The bold branches indicate posterior probability values >0.9. Bootstrap values of ML ≥ 70% are recorded at the nodes. T, ex-type strains. The final alignment of 793 positions, including gaps.
Supplementary Figure S7. ML tree of O. ips complex generated from the TUB2 (Taxon 6) sequence data. Novel sequences obtained in this study are presented in bold typeface. The bold branches indicate posterior probability values >0.9. Bootstrap values of ML ≥ 70% are recorded at the nodes. T, ex-type strains. The final alignment of 414 positions, including gaps.
References
Ayres, M. P., Wilkens, R. T., Ruel, J. J., Lombardero, M. J., and Vallery, E. (2000). Nitrogen budgets of phloem-feeding bark beetles with and without symbiotic fungi. Ecology 81, 2198–2210. doi: 10.1890/0012-9658(2000)081[2198:NBOPFB]2.0.CO;2
Biedermann, P. H. W., and Vega, F. E. (2020). Ecology and evolution of insect–fungus mutualisms. Annu. Rev. Entomol. 5, 431–455. doi: 10.1146/annurev-ento-011019-024910
Bleiker, K. P., and Six, D. L. (2007). Dietary benefits of fungal associates to an eruptive herbivore: potential implications of multiple associates on host population dynamics. Environ. Entomol. 36, 1384–1396. doi: 10.1093/ee/36.6.1384
Cale, J. A., Ding, R., Wang, F., Rajabzadeh, R., and Erbilgin, N. (2019). Ophiostomatoid fungi can emit the bark beetle pheromone verbenone and other semiochemicals in media amended with various pine chemicals and beetle-released compounds. Fungal Ecol. 39, 285–295. doi: 10.1016/j.funeco.2019.01.003
Chang, R., Duong, T. A., Taerum, S. J., Wingfield, M. J., Zhou, X., and De Beer, Z. W. (2017). Ophiostomatoid fungi associated with conifer-infesting beetles and their phoretic mites in Yunnan, China. MycoKeys 28, 19–64. doi: 10.3897/mycokeys.28.21758
Chang, R., Duong, T. A., Taerum, S. J., Wingfield, M. J., Zhou, X., Yin, M., et al. (2019). Ophiostomatoid fungi associated with the spruce bark beetle Ips typographus, including 11 new species from China. Persoonia Mol. Phylo. Evol. Fungi 42, 50–74. doi: 10.3767/persoonia.2019.42.03
De beer, Z. W., Procter, M., Wingfield, M. J., Marincowitz, S., and Duong, T. A. (2022). Generic boundaries in the Ophiostomatales reconsidered and revised. Stud. Mycol. 101, 57–120. doi: 10.3114/sim.2022.101.02
De Beer, Z. W., and Wingfield, M. J. (2013). “Emerging lineages in the Ophiostomatales,” in The Ophiostomatoid Fungi: Expanding Frontiers, eds Z. W. De Beer and M. J. Wingfield (Utrecht: CBS), 21–46.
DiGuistini, S., Wang, Y., Liao, N. Y., Taylor, G., Tanguay, P., Feau, N., et al. (2011). Genome and transcriptome analyses of the mountain pine beetle-fungal symbiont Grosmannia clavigera, a lodgepole pine pathogen. PNAS 108, 2504–2509. doi: 10.1073/pnas.1011289108
Duan, Y., Kerdelhue, C., Ye, H., and Lieutier, F. (2004). Genetic study of the forest pest Tomicus piniperda (Col., Scolytinae) in Yunnan Province (China) compared to Europe: new insights for the systematics and evolution of the genus Tomicus. Heredity 93, 416–422. doi: 10.1038/sj.hdy.6800518
Gallego, D., and Galián, J. (2001). The internal transcribed spacers (ITS1 and ITS2) of the rDNA differentiates the bark beetle forest pests Tomicus destruens and T. piniperda. Insect Mol. Biol. 10, 415–420. doi: 10.1046/j.0962-1075.2001.00279.x
Gardes, M., and Bruns, T. D. (1993). ITS primers with enhanced specificity for basidiomycetes-application to the identification of mycorrhizae and rusts. Mol. Ecol. 2, 113–118. doi: 10.1111/j.1365-294X.1993.tb00005.x
Glass, N. L., and Donaldson, G. C. (1995). Development of primer sets designed for use with the PCR to amplify conserved genes from filamentous ascomycetes. Appl. Environ. Microb. 61, 1323–1330. doi: 10.1128/aem.61.4.1323-1330.1995
Grégoire, J. C., Raffa, K. F., and Lindgren, B. S. (2015). “Economics and politics of bark beetles,” in Bark Beetles: Biology and Ecology of Native and Invasive Species, eds F. E. Vega, and R. W. Hofstetter (San Diego: Academic Press), 585–613. doi: 10.1016/B978-0-12-417156-5.00015-0
Jacobs, K., Bergdahl, D. R., Wingfield, M. J., Halik, S., Seifert, K. A., Bright, D. E., et al. (2004). Leptographium wingfieldii introduced into North America and found associated with exotic Tomicus piniperda and native bark beetles. Mycol. Res. 108, 411–418. doi: 10.1017/S0953756204009748
Jacobs, K., Kirisits, T., and Wingfield, M. J. (2003). Taxonomic re-evaluation of three related species of Graphium, based on morphology, ecology and phylogeny. Mycologia 95, 714–727. doi: 10.1080/15572536.2004.11833075
Jacobs, K., Solheim, H., Wingfield, B. D., and Wingfield, M. J. (2005). Taxonomic re-evaluation of Leptographium lundbergii based on DNA sequence comparisons and morphology. Mycol. Res. 109(Pt 10), 1149–1161. doi: 10.1017/S0953756205003618
Jacobs, K., and Wingfield, M. J. (2001). Leptographium Species: Tree Pathogens, Insect Associates, and Agents of Blue Stain. St. Paul: American Phytopathological Society Press.
Jankowiak, R. (2006). Fungi associated with Tomicus piniperda in Poland and assessment of their virulence using Scots pine seedlings. Ann. For. Sci. 63, 801–808. doi: 10.1051/forest:2006063
Jankowiak, R., Solheim, H., Bilański, P., Marincowitz, S., and Wingfield, M. J. (2020). Seven new species of Graphilbum from conifers in Norway, Poland, and Russia. Mycologia 112, 1240–1262. doi: 10.1080/00275514.2020.1778375
Jankowink, R. (2006). Fungi associated with Tomicus minor on Pinus sylvestris in Poland and their succession into the sapwood of beetle-infested windblown trees. Can. J. For. Res. 38, 2579–2588. doi: 10.1139/X08-101
Kerdelhué, C., Roux-Morabito, G., Forichon, J., Chambon, J. M., Robert, E., and Lieutier, F. (2002). Population genetic structure of Tomicus piniperda L. (Curculionidae: Scolytinae) on different pine species and validation of T. destruens (Woll.). Mol. Ecol. 11, 483–494. doi: 10.1046/j.0962-1083.2002.01460.x
Kim, J. J., Lim, Y. W., Breuil, C., Wingfield, M. J., Zhou, X. D., and Kim, G. H. (2005). A new Leptographium species associated with Tomicus piniperda infesting pine logs in Korea. Mycol. Res. 109, 275–284. doi: 10.1017/S0953756204002060
Kirisits, T. (2004). “Fungal associates of European bark beetles with special emphasis on the ophiostomatoid fungi,” in Bark and Wood Boring Insects in Living Trees in Europe, A Synthesis, eds F. Lieutier, K. R. Day, A. Battisti, J. C. Grégoire, and H. F. Evans HF (The Netherlands: Kluwer Academic Publishers), 181–235. doi: 10.1007/978-1-4020-2241-8_10
Kirkendall, L. R., Faccoli, M., and Ye, H. (2008). Description of the Yunnan shoot borer, Tomicus yunnanensis Kirkendall and Faccoli sp. n. (Curculionidae, Scolytinae), an unusually aggressive pine shoot beetle from southern China, with a key to the species of Tomicus. Zootaxa 1819, 25–39. doi: 10.11646/zootaxa.1819.1.2
Kohlmayr, B., Riegler, M., Wegensteiner, R., and Stauffer, C. (2002). Morphological and genetic identification of the three pine pests of the genus Tomicus (Coleoptera, Scolytidae) in Europe. Agr. For. Entomol. 4, 151–157. doi: 10.1046/j.1461-9563.2002.00139.x
Lee, S., Kim, J. J., and Breuil, C. (2005). Leptographium longiclavatum sp. nov., a new species associated with the mountain pine beetle, Dendroctonus ponderosae. Mycol. Res. 109, 1162–1170. doi: 10.1017/S0953756205003588
Lee, S., Kim, J. J., and Breuil, C. (2006). Diversity of fungi associated with mountain pine beetle, Dendroctonus ponderosae, and infested lodgepole pines in British Columbia. Fungal Diver. 22, 91–105.
Li, X., Zhang, Z., Wang, H. B., Wu, W., Cao, P., and Zhang, P. Y. (2010). Tomicus armandii Li and Zhang (Curculionidae, Scolytinae), a new species from China. Zootaxa 2572, 57–64. doi: 10.11646/zootaxa.2572.1.4
Liao, Z. Y., and Ye, H. (2004). Action mechanisms of phytotoxin produced by Leptographium yunnanense associated with Tomicus. For. Pest Dis. 23, 20–22.
Lieutier, F., Langstrom, B., and Faccoli, M. (2015). “The genus Tomicus,” in Bark Beetles: Biology and Ecology of Native and Invasive Species, eds F. E. Vega, and R. W. Hofstetter (Amsterdam: Elsevier), 371–426. doi: 10.1016/B978-0-12-417156-5.00010-1
Lieutier, F., Yart, A., and Salle, A. (2009). Stimulation of tree defenses by Ophiostomatoid fungi can explain attack success of bark beetles on conifers. Ann. For. Sci. 66, 801–822. doi: 10.1051/forest/2009066
Linnakoski, R., De Beer, Z. W., Ahtiainen, J., Sidorov, E., Niemelä, P., Pappinen, A., et al. (2010). Ophiostoma spp. associated with pine-and spruce-infesting bark beetles in Finland and Russia. Persoonia Mol. Phylo. Evolut. Fungi 25, 72–93. doi: 10.3767/003158510X550845
Linnakoski, R., de Beer, Z. W., Duong, T. A., Niemelä, P., Pappinen, A., and Wingfield, M. J. (2012b). Grosmannia and Leptographium spp. associated with conifer-infesting bark beetles in Finland and Russia, including Leptographium taigense sp. nov. Antonie van Leeuwenhoek 102, 375–399. doi: 10.1007/s10482-012-9747-6
Linnakoski, R., de Beer, Z. W., Niemelä, P., and Wingfield, M. J. (2012a). Associations of conifer-infesting bark beetles and fungi in fennoscandia. Insects 3, 200–227. doi: 10.3390/insects3010200
Linnakoski, R., Jankowiak, R., Villari, C., Kirisits, T., Solheim, H., De Beer, Z. W., et al. (2016). The Ophiostoma clavatum species complex: a newly defined group in the Ophiostomatales including three novel taxa. Antonie van Leeuwenhoek 109, 987–1018. doi: 10.1007/s10482-016-0700-y
Lu, M., Wingfield, M. J., Gillette, N., and Sun, J. H. (2011). Do novel genotypes drive the success of an invasive bark beetle-fungus complex? Implications for potential reinvasion. Ecology 92, 2013–2019. doi: 10.1890/11-0687.1
Masuya, H., Kaneko, S., Yamaoka, Y., and Osawa, M. (1999b). Comparisons of ophiostomatoid fungi associated with Tomicus piniperda and T. minor in Japanese red pine. J. For. Res. 4, 131–135.
Masuya, H., Kaneko, S., Yamaura, Y., and Yamaoka, Y. (1999a). Ophiostomatoid fungi isolated from Japanese red pine and their relationships with bark beetles. Mycoscience 50, 212–223.
Masuya, H., Kim, J. J., Wingfield, M. J., Yamaoka, Y., Kaneko, S., Breuil, C., et al. (2005). Discovery and description of a teleomorph for Leptographium koreanum. Mycotaxon 94, 159–173.
Mathiesen-Kaarik, A. (1953). Eine Ubersicht uber die gewohnlichsten mit Borkenkafern assoziierten Blauepilze in Schweden und einige fur Schweden neue Blauepilze. Meddelanden fran Statens Skogforskningsinstitut 43, 1–74.
Pan, Y., Lu, J., Zhou, X. D., Chen, P., Zhang, H., and Ye, H. (2020a). Leptographium wushanense sp. nov., associated with Tomicus armandii on Pinus armandii in Southwestern China. Mycoscience 61, 43–48. doi: 10.1016/j.myc.2018.10.003
Pan, Y., Lu, J., Zhou, X. D., Yu, Z. F., Chen, P., Wang, J., et al. (2020b). Two new species of Leptographium associated with Tomicus spp. infesting Pinus spp. in Southwestern China. Int. J. Syst. Evolut. Microbiol. 70, 4798–4807. doi: 10.1099/ijsem.0.004349
Pan, Y., Zhao, T., Krokene, P., Yu, Z. F., Qiao, J., Lu, M., et al. (2018). Bark beetle-associated blue-stain fungi increase antioxidant enzyme activities and monoterpene concentrations in Pinus yunnanensis. Front. Plant Sci. 9, 1731. doi: 10.3389/fpls.2018.01731
Raffa, K. F., Aukema, B. H., Bentz, B. J., Carroll, A. L., Hicke, J. A., Turner, M. G., et al. (2008). Cross-scale drivers of natural disturbances prone to anthropogenic amplification: the dynamics of bark beetle eruptions. Bioscience 58, 501–517. doi: 10.1641/B580607
Reid, J., and Hausner, G. (2010). The epitypification of Ophiostoma minutum, now Ceratocystiopsis minuta. Mycotaxon 113, 463–474. doi: 10.5248/113.463
Ronquist, F., and Huelsenbeck, J. P. (2003). MrBayes 3: Bayesian phylogenetic inference under mixed models. Bioinformatics 19, 1572–1574. doi: 10.1093/bioinformatics/btg180
Six, D. L., and Wingfield, M. J. (2011). The role of phytopathogenicity in bark beetle-fungus symbioses: a challenge to the classic paradigm. Ann. Rev. Entomol. 56, 255–272. doi: 10.1146/annurev-ento-120709-144839
Stamatakis, A. (2006). RAxML-VI-HPC: maximum likelihood-based phylogenetic analyses with thousands of taxa and mixed models. Bioinformatics 22, 2688–2690. doi: 10.1093/bioinformatics/btl446
Wang, H., Wang, T., Liu, Y., Zeng, F., Zhang, H., Lu, Q., et al. (2022). Diversity of Ophiostomatoid fungi associated with Dendroctonus armandi infesting Pinus armandii in Western China. J. Fungi 8, 214. doi: 10.3390/jof8030214
Wang, H. M., Wang, Z., Liu, F., Wu, C. X., Zhang, S. F., Kong, X. B., et al. (2019). Differential patterns of ophiostomatoid fungal communities associated with three sympatric Tomicus species infesting pines in south-western China, with a description of four new species. MycoKeys 50, 93–133. doi: 10.3897/mycokeys.50.32653
Wang, Y. C., Zhao, B., Wang, T. K., Li, C., Ma, L., Li, G., et al. (2000). Study on occurring regulation and control method of Tomicus pilifer. Jilin For. Sci. Technol. 29, 10–11. doi: 10.16115/j.cnki.issn.1005-7129.2000.05.004
Wang, Z., Liu, Y., Wang, H., Meng, X., Liu, X., Decock, C., et al. (2020). Ophiostomatoid fungi associated with Ips subelongatus, including eight new species from northeastern China. IMA Fungus 11, 1–29. doi: 10.1186/s43008-019-0025-3
White, T. J., Bruns, T., Lee, S., and Taylor, J. (1990). “Amplification and direct sequencing of fungal ribosomal RNA genes for phylogenetics,” in PCR Protocols: A Guide to Methods and Applications, eds I. M. A. Gelfand, D. H. Sninsky, and T. J. White (San Diego: Academic Press), 18315–322.
Wingfield, M. J., Eifert, K. A., and Webber, J. F. (1993). Ceratocystis and Ophiostoma: Taxonomy, Ecology, and Pathogenicity. St. Paul, Minnesota: American Phytopathological Society, APS Press.
Wingfield, M. J., Garnas, J. R., Hajek, A., Hurley, B. P., de Beer, Z. W., and Taerum, S. J. (2016). Novel and co-evolved associations between insects and microorganisms as drivers of forest pestilence. Biol. Invas. 18, 1045–1056. doi: 10.1007/s10530-016-1084-7
Wingfield, M. J., Slippers, B., and Wingfield, B. D. (2010). Novel associations between pathogens, insects and tree species threaten world forests. N. Zeal. J. For. Sci. 40, S95–S104. Available online at: https://www.scionresearch.com/nzjfs
Yamaoka, Y., Wingfield, M. J., Takahashi, I., and Solheim, H. (1997). Ophiostomatoid fungi associated with the spruce bark beetle Ips typographus f. aponicus in Japan. Mycol. Res. 101, 1215–1227. doi: 10.1017/S0953756297003924
Yin, M., Duong, T. A., Wingfield, M. J., Zhou, X., and De Beer, Z. W. (2015). Taxonomy and phylogeny of the Leptographium procerum complex, including Leptographium sinense. sp. nov. and Leptographium longiconidiophorum. sp. nov. Antonie van Leeuwenhoek. 107, 547–563. doi: 10.1007/s10482-014-0351-9
Zhou, X., de Beer, Z. W., Harrington, T. C., McNew, D., Kirisits, T., and Wingfield, M. J. (2004). Epitypification of Ophiostoma galeiforme and phylogeny of species in the O. galeiforme complex. Mycologia 96, 1306–1315. doi: 10.1080/15572536.2005.11832880
Zhou, X. D., de Beer, Z. W., and Wingfield, M. J. (2013). “Ophiostomatoid fungi associated with conifer infecting bark beetles in China,” in CBS-KNAW Fungal Biodiversity Centre, Ophiostomatoid Fungi: Expanding Frontiers, eds K. A. Seifert, Z. W. de Beer, and M. J. Wingfield (Utrecht: CBS), 91–98.
Keywords: Ceratocystiopsis, Graphilbum, Leptographium, Ophiostoma, taxonomy
Citation: Wang H, Liu C, Yue F, Yan D-H and Lu Q (2022) Identification of ophiostomatalean fungi associated with Tomicus pilifer infesting Pinus koraiensis in Northeastern China. Front. Microbiol. 13:919302. doi: 10.3389/fmicb.2022.919302
Received: 13 April 2022; Accepted: 04 July 2022;
Published: 02 September 2022.
Edited by:
Chunwang Xiao, Minzu University of China, ChinaReviewed by:
XuDong Zhou, Zhejiang Agriculture and Forestry University, ChinaYue Pan, Yunnan University, China
Runlei Chang, Shandong Normal University, China
Copyright © 2022 Wang, Liu, Yue, Yan and Lu. This is an open-access article distributed under the terms of the Creative Commons Attribution License (CC BY). The use, distribution or reproduction in other forums is permitted, provided the original author(s) and the copyright owner(s) are credited and that the original publication in this journal is cited, in accordance with accepted academic practice. No use, distribution or reproduction is permitted which does not comply with these terms.
*Correspondence: Quan Lu, bHVxdWFuJiN4MDAwNDA7Y2FmLmFjLmNu