- 1Instituto de Ecología Regional (IER), Universidad Nacional de Tucumán (UNT)–Consejo Nacional de Investigaciones Científicas y Técnicas (CONICET), Tucumán, Argentina
- 2Facultad de Ciencias Naturales e Instituto Miguel Lillo, Universidad Nacional de Tucumán (UNT), Tucumán, Argentina
- 3Department of Microbiology and Medical Zoology, School of Medicine, University of Puerto Rico, Medical Sciences Campus, San Juan, Puerto Rico
- 4Planta Piloto de Procesos Industriales Microbiológicos (PROIMI-CONICET), Tucumán, Argentina
- 5Fundación Miguel Lillo (FML), Tucumán, Argentina
- 6Centro Científico Tecnológico (CCT-NOA SUR), Consejo Nacional de Investigaciones Científicas y Técnicas (CONICET), Tucumán, Argentina
- 7INSIBIO (CONICET - UNT), Instituto de Química Biológica “Dr. Bernabé Bloj”, Facultad de Bioquímica, Química y Farmacia, Universidad Nacional de Tucumán, Tucumán, Argentina
Plastic production has been increasing exponentially in the last 60 years, but plastic disposal is out of control, resulting in the pollution of all ecosystems on Earth. Finding alternative environmentally sustainable choices, such as biodegradation by insects and their associated gut microbiota, is crucial, however we have only begun to characterize these ecosystems. Some bacteria and one fungus have been previously identified in the gut of Greater Wax Moth larvae (Galleria mellonella L., Lepidoptera, Pyralidae) located mainly in the Northern hemisphere. The aim of this study was to describe changes in the gut microbiota associated with the consumption of polyethylene and polystyrene by the Greater Wax Moth in Argentina, considering both bacteria and fungi. Larvae were fed polyethylene, polystyrene and beeswax as control for 7 days. Next generation sequencing revealed changes in the bacterial gut microbiome of the wax moth larvae at the phyla and genus levels, with an increase in two Pseudomonas strains. The fungal communities showed no differences in composition between diets, only changing in relative abundance. This is the first report of both bacterial and fungal communities associated with a plastivore insect. The results are promising and call for more studies concerning a potential multi-kingdom synergy in the plastic biodegradation process.
Introduction
The massive production of plastic started only 50–60 years ago (i.e., 1960) and recent quantifications estimate 360 million metric tons (Mt) in 2018, with an average annual growth rate of 8.6% (1.7 million tons; PlasticsEurope, 2019). Most items used by humans are made of this inexpensive, flexible and long-lasting material. Its’ unregulated production, use, extremely rapid disposal, and low rates of recycling have resulted, though, in plastic waste accumulation in every biome on Earth, including marine, freshwater, and terrestrial habitats (Rochman, 2018; Bucci et al., 2019; Malizia and Monmany-Garzia, 2019). Next to climate change, it is fast becoming the most important environmental issue of our time. Due to the multiple effects of plastic pollution on the health of ecosystems, solutions toward mitigating these consequences are being sought at different levels of socio-ecological organization (Rochman, 2018; Przemieniecki et al., 2019).
Recently explored solutions for plastic pollution mitigation include plastic biodegradation. Microplastics (i.e., <5 mm) represent a new substrate for distinct microbial colonization and biofilm formation, called the “Plastisphere” (Zettler et al., 2013; McCormick et al., 2014), suggesting that many of these organisms are able to degrade the material. Biodegradation effects on ubiquitous polyolefins such as polyethylene (PE) and polystyrene (PS), may be exerted, in fact, by bacteria, and fungi (or associated enzymes) isolated from the open environment and from the guts of insects (Yang et al., 2015a,b; Bombelli et al., 2017; Brandon et al., 2018; Wang et al., 2020; Woo et al., 2020) and other animals (Leonov and Tiunov, 2020; Song et al., 2020). Concerning insect gut microbiota, a few studies showed evidence of plastic biodegradation mostly by bacteria associated with coleopteran and moth species. For example, Plodia interpunctella (Lepidoptera, Pyralidae) degraded polyethylene by the action of at least two gut bacteria: Enterobacter asburiae and Bacillus sp. (Yang et al., 2014). When examined at the community level, the gut microbiota of insects fed with plastic diets showed changes in structure and composition, as indicated by the relative abundance and identity of the species, respectively (Przemieniecki et al., 2019; Cassone et al., 2020; Lou et al., 2020). For instance, Przemieniecki et al. (2019) reported that gut bacterial communities of Tenebrio molitor larvae fed with oat and cellulose diets, had similar composition, while those individuals fed with polyethylene formed another taxonomic group, and those with a polystyrene diet had the highest dissimilarity in composition.
Some gut bacteria of the Greater Wax Moth, Galleria mellonella (Lepidoptera), a voracious ‘plastivore’, consuming and degrading polyethylene (Bombelli et al., 2017; LeMoine et al., 2020) and polystyrene (Lou et al., 2020), have been identified. High abundances of the bacteria Bacillus spp., Serratia spp., Acinetobacter spp., Enterobacter sp. D1, Enterococcus sp., and Massilia sp. have been recorded in individuals consuming these materials (Ren et al., 2019; Cassone et al., 2020; Lou et al., 2020; Jiang et al., 2021). The phyla Firmicutes and Proteobacteria have been pointed out as part of the core gut bacteria of the Greater Wax Moth, given their predominance in the guts of larvae under nine different feeding conditions (Lou et al., 2020). Most studies examining these changes of gut microbiota at the community level only considered bacteria, while the analysis of fungal species and their co-occurrence with bacteria have been largely unexplored. Only one G. mellonella’s gut fungus, Aspergillus flavus, has been reported participating in the degradation of polyethylene (Zhang et al., 2020). But, the identity of the wax moth’s core gut fungi remains unknown. The combined processes of gut bacteria and fungi have been suggested as an opportunity for insects to deal with challenging diets (Mason et al., 2019). Given the important role of fungus in plastic biodegradation (Gautam et al., 2007; Shah et al., 2008), we need to include fungal communities in the description of G. mellonella’s gut microbiome consuming plastic in order to detect interactions and synergies with bacteria that have remained hidden.
Galleria mellonella is a cosmopolitan species (Kwadha et al., 2017) that has evolved in harsh habitats and developed the ability to digest variable diets during the larval stage, including honey, beeswax, and the skin of bee pupae (Jindra and Sehna, 1989). This ability suggests the combined action of gut bacteria and fungi in plastic degradation that may involve complicated interactions. The moth’s gut microbiota, though, did not have a clear role in this degradation when lab-reared larvae were examined (Kong et al., 2019). Given that the existence of a global resident gut microbiota has been questioned for several Lepidopteran species (Hammer et al., 2017), a first step to assess the importance of gut microbiomes in plastic biodegradation is to detect to what degree the gut bacteria and fungi of the Greater Wax Moth larvae consuming plastics change in comparison to natural diets. Assessments should include wax moth individuals from understudied regions of the world, where knowledge on the subject is limited, and unknown but key microorganisms may exist.
The aim of this study was to describe changes in the gut microbiome associated with the consumption of polyethylene and polystyrene by the Greater Wax Moth, Galleria mellonella L. (Lepidoptera, Pyralidae) in Argentina. Specifically, we aimed to: (1) analyze the composition, diversity, and structure of bacteria and fungi gut communities when the host was exposed to diets based on PE and PS, (2) identify biomarkers for further experiments on plastic degradation, and (3) identify associations between bacteria and fungi occurrence under these diets.
Materials and Methods
Microbial Communities’ Molecular Analyses
Galleria mellonella larvae were acquired at the CEMUBIO (Centro Multiplicador de Biocontroladores Nativos, National Institute of Agricultural Technology - INTA), in Río Negro, Argentina, where they were fed a sterilized artificial diet based on essential nutrients and proteins. The larvae were kept under this artificial diet until the experiments. Larvae with a clear color (i.e., healthy aspect) in the instars three to four were fed with polyethylene, polystyrene, and beeswax for 7 days, at 30°C and in absence of light. This time interval was determined based on our own previous trials in which longer intervals led to pupation and consequent loss of the digestive tubes. Similar feeding intervals have been used in previous studies to examine changes in gut microbiota in response to diet (e.g., Broderick et al., 2004; Ma et al., 2021). Four larvae were put per Petri dish with one 4 cm-side square of any of the three materials and the design was repeated five times for each diet (n = 20 per treatment). After 7 days, two larvae were randomly selected from each Petri dish and their body surfaces disinfected with alcohol 70% for 1 min. Then, the larvae were dissected to obtain their digestive tubes (n = 10 per treatment). The entire guts were used for analysis, including their contents. The 30 digestive tubes were individually placed in empty sterile Eppendorf’s at −80°C until DNA extraction.
Total DNA was extracted using the DNeasy Blood & Tissue Kit (QIAGEN) and at least 10 ng of the obtained DNA per larvae was quantified on a DeNovix DS-11 microvolume spectrophotometer (Supporting data and figures).1 This DNA was conserved in TAE buffer at −80°C and used for rDNA 16S amplification and sequencing.
16S-rRNA and ITS-2 Gene Amplifications and Read Processing
DNA from the 30 digestive tubes were normalized to 4 nm during l6S library prep. The V4 region of the 16S ribosomal RNA gene (~291 bp) was amplified using universal bacterial primers: 515F (5’GTGCCAGCMGCCGCGGTAA3’) and 806R (5’GGACTACHVGGGTWTCTAAT3’; Caporaso et al., 2012) and the ITS-2 gene was amplified with primers ITS9-FW and ITS4-RV with amplicon sizes ranging from 240 to 460 bp as described by de Souza et al. (2016). Raw read pre-processing of demultiplexed files was done with a Phred offset of 33, and default parameters (QIITA, Gonzalez et al., 2018). Reads were trimmed to 250 bp. 16S rRNA genes were classified using the SILVA database (Quast et al., 2013), while ITS reads were classified using the UNITE 7.0 taxonomic database (Nilsson et al., 2018) both with a minimum similarity threshold of 97%. OTU picking was performed using a closed reference approach. The species table (biom file) was downloaded from QIITA for downstream analyses using R and a locally run version of QIIME (Caporaso et al., 2010). Singletons (OTUs with less than three reads), sequences matching chloroplasts, mitochondria, those matching eukaryotes, and taxonomically unassigned sequences were removed from downstream analyses.
Rarefaction was done at a level of 95,035 reads for 16S rRNA and 95,043 reads for ITS (Table 1).
Reads are publicly available and deposited in QIITA project ID 12844 with its associated metadata, as well as in EBI accession number ERP135546.
Community Analysis of Diversity
Beta Diversity Measured as Bray–Curtis Dissimilarity
Quantification of compositional dissimilarity between sample groups was done using pairwise Bray-Curtis index (Bray and Curtis, 1957). Global differences in bacterial and fungal communities were visualized using Non-Metric Multidimensional Scaling (NMDS). Statistical significance between sample groups was assessed using the PERMANOVA test (Anderson, 2001). Additionally, permutational multivariate analysis of variance using distance matrices (adonis permutation test) between different groups (plastic treatments) with a calculated value of p based on the Bray-Curtis distance table which was used to generate the plots. These tests were done using the python script compare_categories for each specific test in QIIME with the distance matrix as the input file and 999 permutations.
Alpha Diversity, Taxonomic Plots and Discriminant Taxa Analyses
Alpha diversity measures of Chao 1 (richness) and Shannon (diversity) were plotted as boxplots using ggplot2 (Wickham, 2009; R Development Core Team, 2020). For alpha diversity statistical tests, we used nonparametric t-tests with Monte Carlo permutations to determine the value of p. Barplots revealing phyla and genus were computed using QIIME (Caporaso et al., 2010).
Biomarker discriminant taxa analyses were performed with the LEfSe algorithm (Segata et al., 2011) for multi-class comparison. In order to reduce false discovery rates, FDR values of p = <0.01 were considered for significant differences—these analyses were performed using the MicrobiomeAnalyst pipeline (Dhariwal et al., 2017).
OTU Ubiquity dot plots were built relating OTU relative abundances with OTU proportions among samples (ubiquity). The 16S rRNA and ITS OTU tables and the metadata files were modified into ubiquity matrices containing the OTU ubiquity (proportion of samples in which it is represented), and the OTU relative abundance, through the integration of multiple R programs such as phyloseq (McMurdie and Holmes, 2013), vegan (Oksanen et al., 2008), reshape (Wickham, 2007) and phytools (Revell, 2012). Dot plots were built with ggplot2 (Wickham, 2009).
Ubiquity and Co-occurrence Analysis Plots
To measure co-occurrence between fungi and bacteria across the different plastic treatments, we calculated the Dice index using previously described methods and scripts (Godoy-Vitorino et al., 2018). The Dice index measures similarity between samples (Dice, 1945), with values that range between 0 (no co-occurrence) to 1 (maximum co-occurrence).
Results
During the experiments we noted some differences in the general condition of the larvae under the different diets. The most notorious was behavioral; the feeding activity of larvae in polyethylene and polystyrene-based diets was high during the first 2 days and almost absent in the last day, while larvae fed on beeswax showed a constantly intermediate feeding activity along the time interval.
Structure and Diversity of Bacterial and Fungal Communities
Beta diversity (i.e., structure) comparisons of gut bacterial communities, based on OTUs and using NMDS ordination, confirmed that there were differences in composition between bacterial communities in larvae fed with beeswax and communities in larvae fed with plastics (p = 0.02) as seen by the majority of beeswax samples to the left of Axis 1 (Figure 1A). In terms of the alpha diversity, the Chao richness index for the bacterial biota showed no differences among treatments, except for a slightly higher richness of gut bacteria in moths consuming polyethylene compared to beeswax (p = 0.052; Figure 1B), but no differences were found for Shannon diversity (Figure 1C).
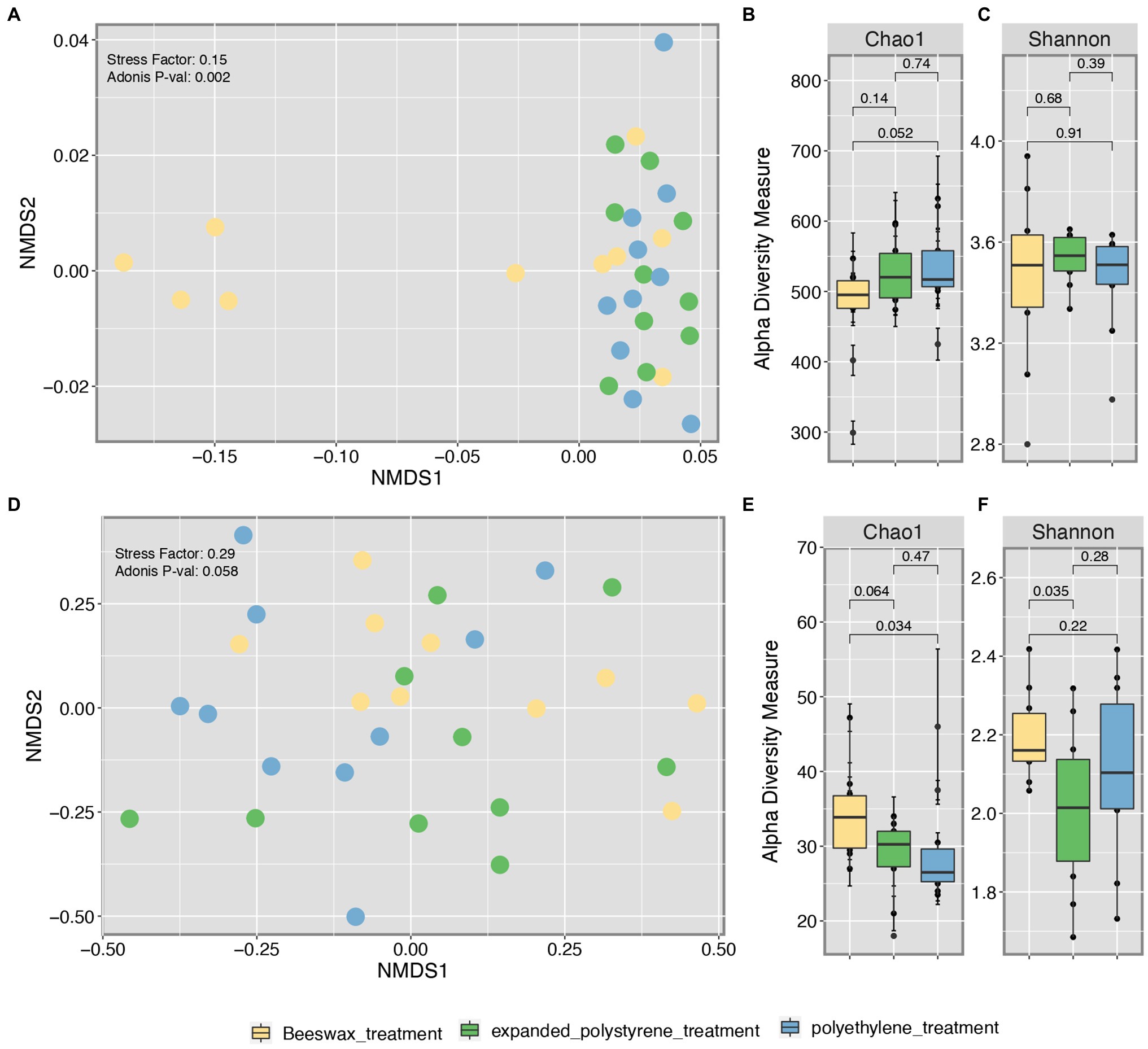
Figure 1. Beta diversity (NMDS) of bacterial (A) and fungal (D) communities. Species Richness based on Shannon (B) and Chao diversity indexes for bacterial (B,C) and fungal (E,F) communities with statistical significance value calculated through Wilcoxon pairwise test.
As for the fungal microbiota, beta diversity comparisons of composition showed marginal to no differences among treatments (p = 0.058), with a majority of samples from plastic eaten moths to the left of Axis 1 (Figure 1D). However, in terms of richness (Chao1) and diversity (Shannon) we found significant differences, with the gut from beeswax consumers being significantly richer than those consuming polyethylene (p = 0.034) and marginally higher than expanded polystyrene (p = 0.064). Expanded polystyrene samples were the ones with the least diversity compared to beeswax (p = 0.035), and no differences were found in the fungal diversity among the plastic consumers (Figures 1E,F).
Composition of Bacterial and Fungi Communities
At the bacterial phyla level, Proteobacteria were the most common across the three treatments, with a relative abundance of 72.2%, followed by Firmicutes (mean = 14.9%) and Bacteroidetes (mean = 7.9%; Figure 2A; Supplementary Table S1). Fusobacteria (mean = 1.5%) were found almost exclusively in the beeswax treatment, while Fibrobacteria (0.1%) was only present in the two plastic treatments (Figure 2A). At the genus level, Pseudomonas and Lactobacillus were the most common, representing, on average, 56.5 and 5.2%, respectively (Figure 2B; Supplementary Table S2). These genera were found in a lower proportion in the beeswax diet in contrast to the plastic treatments; as per the ubiquity plot (Figure 3), the relative abundance of Pseudomonas sp., and P Citronellolis increased from a ~ 5 to 10% in plastic consumers. Members of Neisseriaceae (mean 3.8% of the total), Pasteurella (1.8%), Actinobacillus (1.3%), Alloprevotella (1.1%), Leptotrichia (0.5%), Aggregatibacter (0.4%), Porphyromonas (0.3%), Gemella (0.3%), Carnobacterium (0.2%), Donghicola (0.1%), Eikenella (0.1%) were present in the beeswax diet and absent in plastic treatments. In contrast, Bifidobacterium, Butyricimonas, Alistipes, Fibrobacter, Marinococcus, Beijerinckiaceae, Bradyrhizobium, Rhizobium, Roseovarius, Sphingomonas, Enhydrobacter were found in the plastic treatments but not in beeswax and in a proportion of 0.1% (Figure 2B).
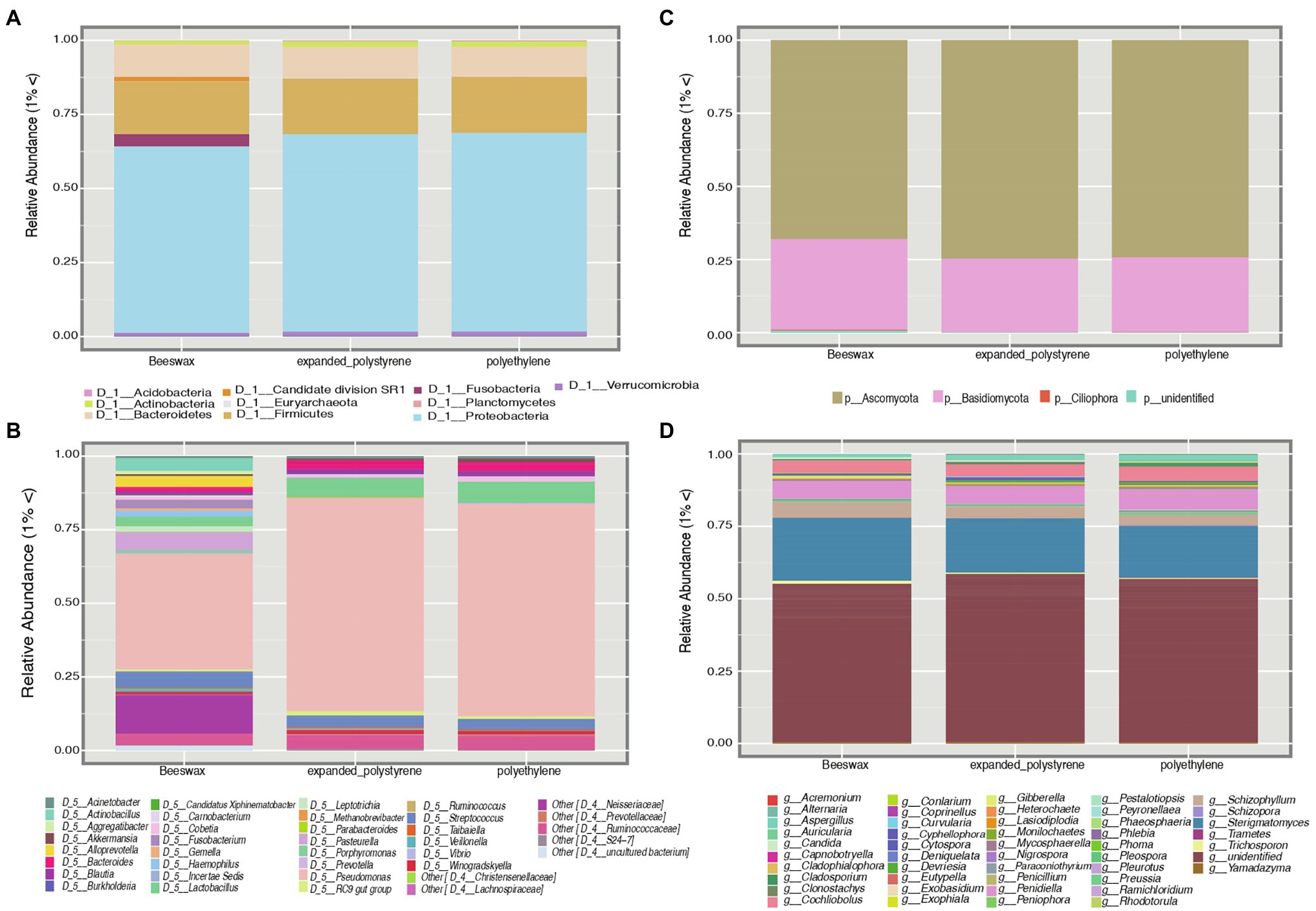
Figure 2. Composed panel depicting taxonomic plots of bacterial diversity at the phyla level (A) and genus level (B). Plots corresponding to fungal diversity at the phyla (C) and genus level (D). The legend shows the taxa with more than 1% of abundance.
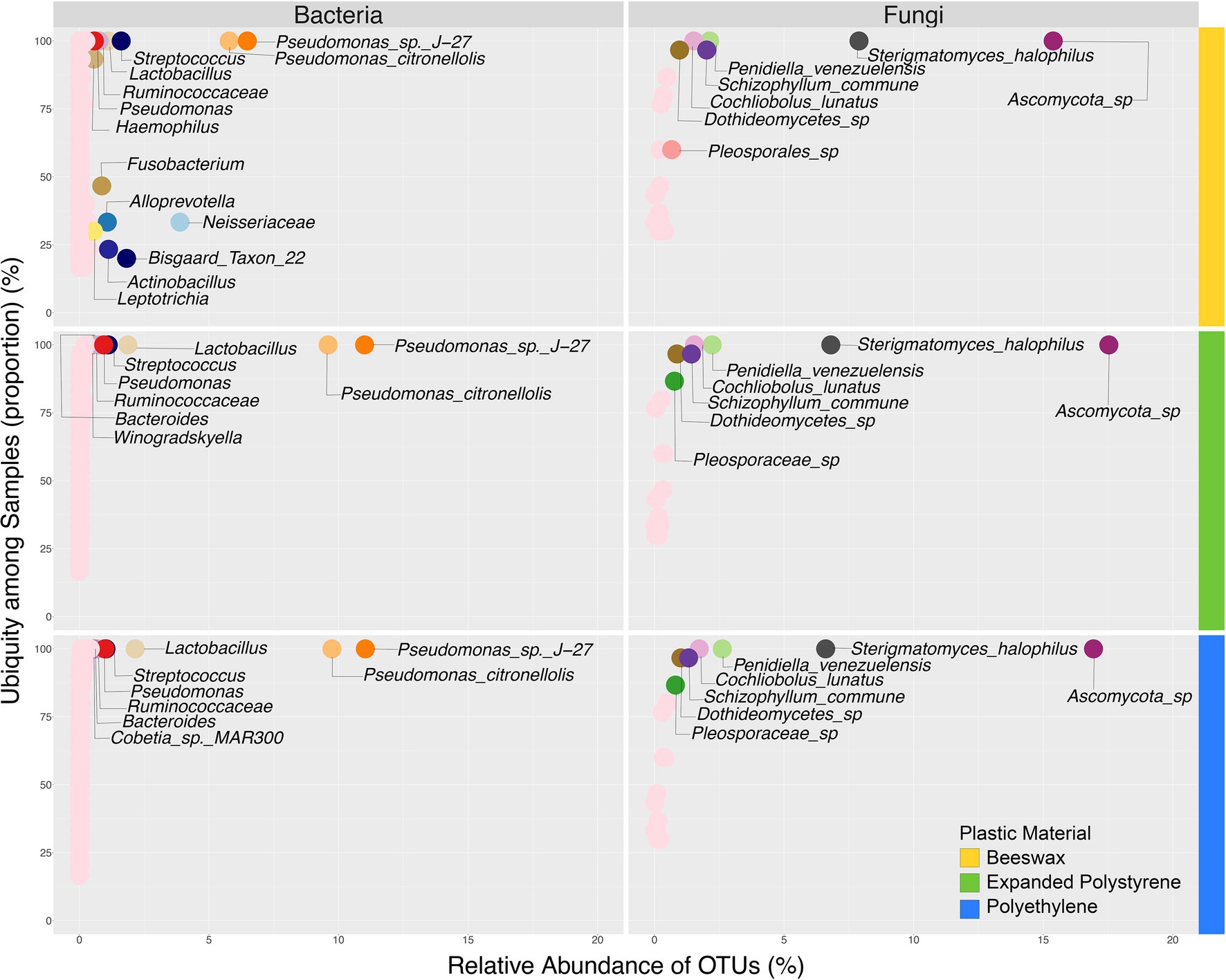
Figure 3. Ubiquity analysis of both bacterial and fungal biomes comparing relative abundance against prevalence in samples based on plastic material used for treatment.
Considering fungi, at the phylum level only two groups were found, Ascomycota (mean = 72.1%) and Basidiomycota (mean = 27.9%; Figure 2C; Supplementary Table S3). Ascomycota occurred above the average in plastic treatments, while Basidiomycota was present above average in the beeswax treatment. At the genus level, an unidentified Ascomycota group was, on average, the most common (49.6%), the second most represented genus was Sterigmatomyces (mean = 21.4%) followed by Penidiella (mean = 7%). These genera were found in all three treatments (Figures 2D, 3). Some genera with low representation (< 5%), such as Schizophyllum and Trichosporon, although present in all three treatments, were more common in the beeswax diet. An undefined group of Capnodiales (Ascomycota), showed percentages <0.1%, and were observed only in plastic treatments.
Biomarker Tests
Bacterial taxa that best characterized each treatment, and changed according to the larval diet are shown in Figure 4. Pseudomonas sp. (p = 0.0276), Pseudomonas citronellolis and Lactobacillus (p = 0.0816) were significantly more abundant in the plastic treatments compared to beeswax.
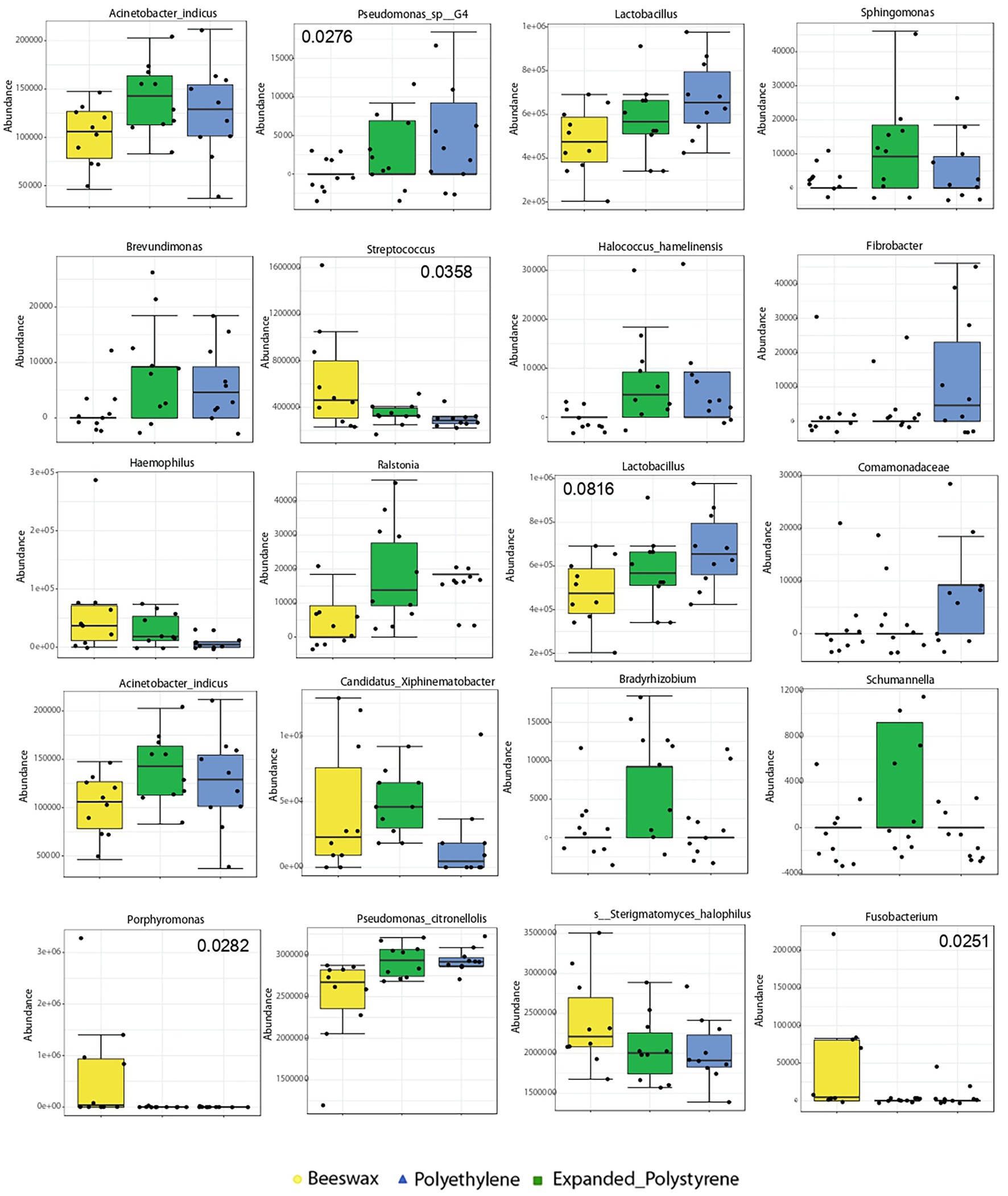
Figure 4. Biomarker discriminant taxa analyses of bacterial communities according to the treatment, via LEfSe (Linear discriminant analysis Effect Size) algorithm, including only OTUs present in at least 50% of the samples. Values of p are in some taxa added to the plot.
Among Proteobacteria, the Comamonadaceae family as well as the genera Pseudomonas sp. G4, Sphingomonas, Halococcus, Brevundimonas and Bradhyrhizobium were present in plastic-fed larvae. Other bacterial groups inhabiting the guts of plastic-fed larvae were found, such as members of Schumannella belonging to the phylum Actinobacteria and populations of the genus Fibrobacter belonging to the Fibrobacteres phylum. The genus Schumannela was exclusively related to a diet based on polystyrene and Fibrobacter was exclusively related to a diet based on polyethylene. On the contrary, the phylum Fusobacteria was exclusively found in beeswax-fed larvae (p = 0.0251). Additionally, Streptococcus (p = 0.0358), Porphyromonas (p = 0.0282) were dominant in beeswax (Figure 4).
Results of the biomarker discriminant taxa analyses (LEfSe) of relevant fungal groups are shown in Figure 5. Analysis showed that polystyrene consumers had a high prevalence of Ascomycota (p = 0.0188) as dominant taxa.
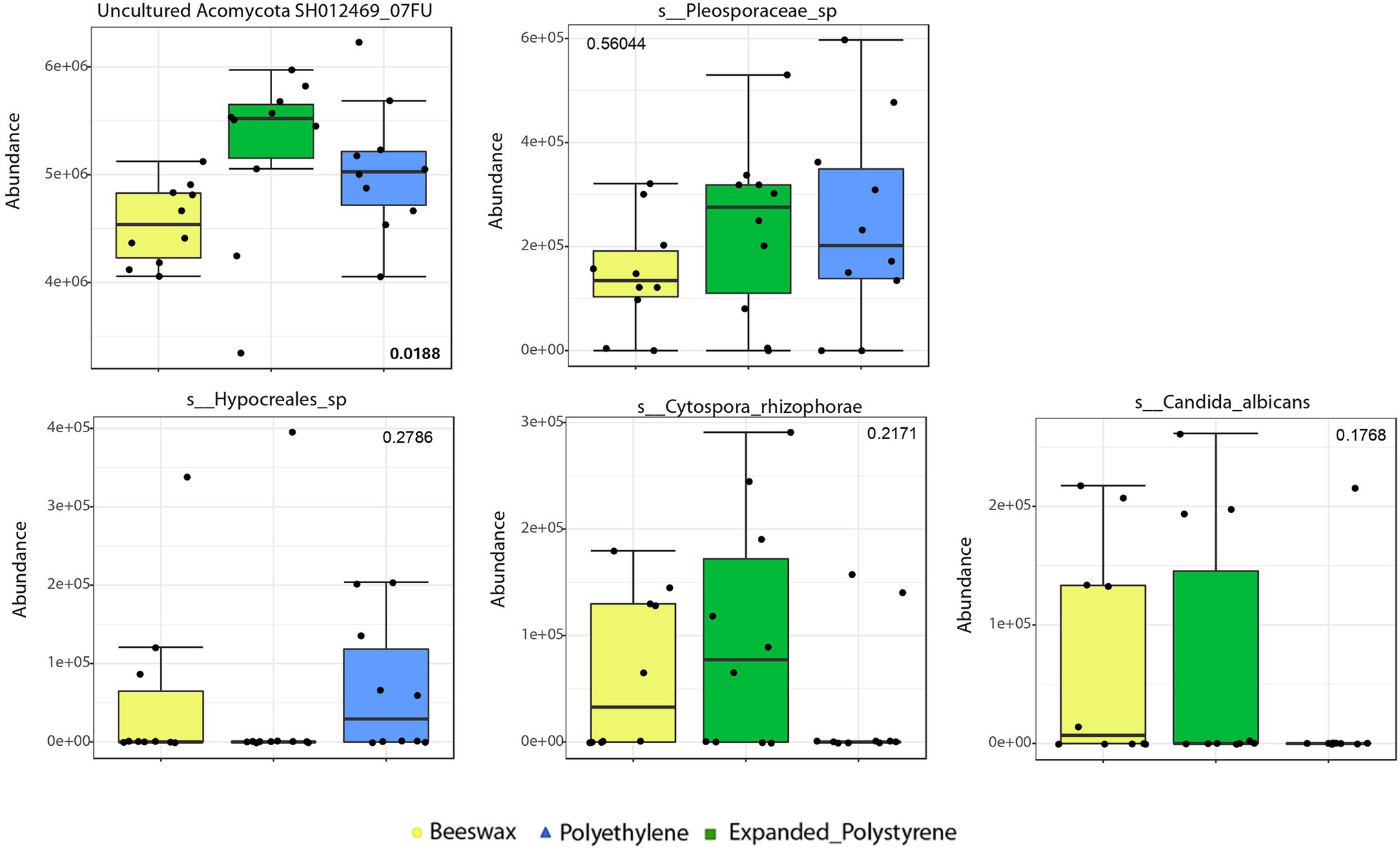
Figure 5. Biomarker discriminant taxa analyses of fungal communities according to the treatment, via LEfSe, including only OTUs present in at least 50% of the samples. Taxa shown here correspond to genus level or to the lowest unclassified taxa. values of p are in some taxa added to the plot.
Co-occurrence Analysis
The larval diets influenced the co-occurrence of species composing of the wax moth gut microbiota. The associations between bacteria and fungi were more diverse in the beeswax diet (Figure 6A), with a number of bacterial and fungal taxa disappearing in the plastic treatments, such as members of Neisseriaceae, Streptococcus, Fusobacterium, Alloprevotella, Actinobacillus, an uncultured Proteobacteria, and Schizophylum commune (Basidiomycota) (Figures 6B C). Nevertheless, we found a group of both bacteria and fungi that co-occurred in all diets: Pseudomonas sp. J27 and P. citronellolis, Ascomycota sp., Penidiella venezuelensis (Ascomycota) and Sterigmatomyces halophilus (Basidiomycota). These five OTUs co-occurred in all treatments and individuals. Between plastics, the polyethylene treatment included a fungal taxon that was absent in the expanded polystyrene diet, Cochliobolus lunatus (Ascomycota).
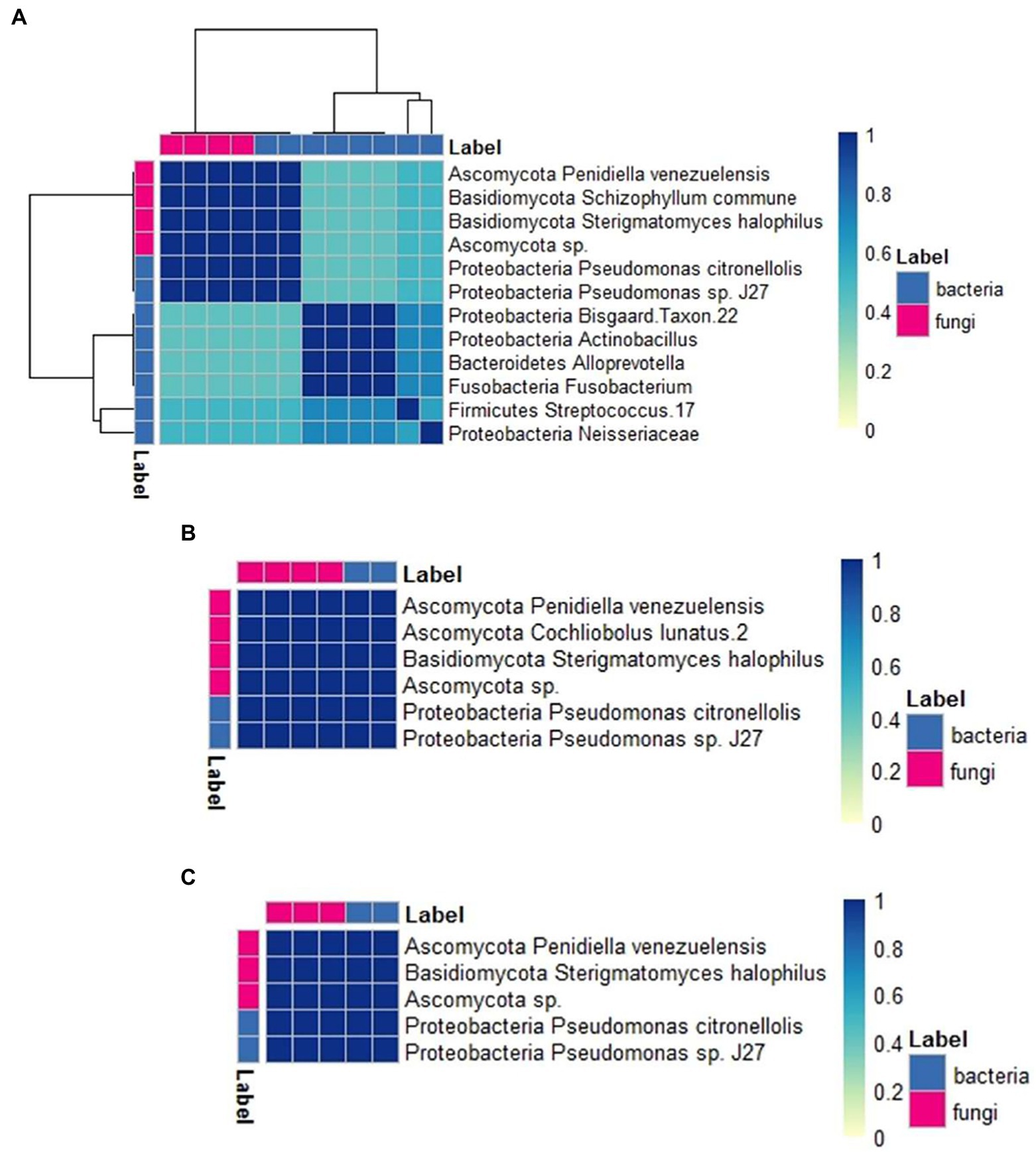
Figure 6. Co-occurrence analysis heat map showing the degree of association between bacteria and fungi taxa across diets, scored using the Dice index. Clustering obtained from Euclidean distances between taxa. (A): beeswax, (B): polyethylene, (C): expanded polystyrene.
Discussion
This is the first multi-kingdom description of the Greater Wax Moth’s gut microbiome associated with the consumption of polyethylene and polystyrene. Gut bacterial and fungal communities of wax moth larvae in Argentina responded differently to the various diets. There were differences in structure and composition between bacterial communities in larvae consuming beeswax and bacterial communities in larvae consuming PE and PS, while fungal communities showed differences mostly in richness and diversity. In addition, we identified both bacterial and fungal biomarkers that may be useful in future experiments and identified co-occurrence of bacteria and fungi that suggest further research avenues.
The response of wax moth gut microbiota to diet was different in bacteria and fungi. Bacterial composition differed between beeswax- and plastic-based diets; particularly, a dominance of Streptococcus, Porphyromonas or Fusobacteria was observed in the beeswax diet. This result agrees with previous reports on G. mellonella and other insects’ gut communities of individuals consuming plastics (Przemieniecki et al., 2019; Cassone et al., 2020) and suggests that plastics, a low-quality resource, changes gut bacterial communities. A possible simplification of communities caused by a plastic-based diet may have ecological consequences for the larvae. Alpha diversity, in contrast, was not different among bacterial communities under the three diets. An opposite result has been reported by Cassone et al. (2020) in Canada, which highlights the importance of screening individuals from different geographic locations to understand gut microbiota-plastic interactions. In comparison to bacteria, fungal communities did not differ in composition among diets however significant differences were found in richness and diversity. This suggests that bacteria communities were more sensitive to changes in diet than fungal communities—only changing in fungal relative abundance—in our study, which in turn may suggest a more active role of bacteria in plastic decomposition, in comparison to fungi.
In our study, abundance of different Pseudomonas populations (Pseudomonadales), Bradyrhizobium (Rhizobiales) and Comamonadaceae (Burkholderiales) populations in the gut of plastic-fed larvae was noticeable. According to other studies, most bacteria that enter into symbiotic relationships with insects belong to the orders Burkholderiales, Pseudomonadales, Rhizobiales, Xanthomonadales (Proteobacteria phylum) and Verrucomicrobiales (Verrucomicrobia phylum; Russell et al., 2009; Sapountzis et al., 2015). In contrast to our study, in which we found Comamonadaceae associated with a polyethylene diet in wax moths, Peydaei et al. (2021) reported this association in polystyrene fed wax moths. In accordance to our results, Cassone et al. (2020) found a dominance of the Proteobacteria phylum in wax moth larvae consuming plastics; but the genera they identified were different from those in this study, except for Pseudomonas. Species of the genus Pseudomonas are among the most cited degraders for a wide range of plastics (Espinosa et al., 2020; Cf et al., 2021); they have also been touted for the bioremediation of crude oil, simple hydrocarbons, naphthalene, toluene and other hydrophobic polymers (Wilkes and Aristilde, 2017). The extent to which plastics are biodegraded depends on both the structural arrangement of the polymer and the type of Pseudomonas strain. Consequently, to isolate new and more degrading-efficient Pseudomonas strains continues to be the focus of worldwide screening programs. Sphingomonas, detected in our study, are also well-known degraders (Coppotelli et al., 2010). At the same time, populations of the nitrogen-fixing bacteria Bradyrhizobium were certainly the most biologically informative group associated with the polystyrene diet. This bacterium could promote indirect re-assimilation of NH3 and atmospheric nitrogen to promote insect survival in nitrogen-deficient environments. Previous evidence on insects other than G. mellonella demonstrated that Proteobacteria and selected species of Actinobacteria supply insects with food by the production of amino acids and are predominant in the digestive tract (Hongoh et al., 2005, 2008; Desai and Brune, 2012). Proteobacteria which were exclusively related to polyethylene-diet such as Fibrobacter in our study have been major bacterial degraders of lignocellulosic biomass in the herbivore gut. Novel lineages are also present in other anoxic environments where cellulose degradation occurs, such as termite gut (Cragg et al., 2015). Considering that there is a growing interest in degradation of plastics materials using microorganisms capable of degrading lignin, starch, and cellulose (Mehmood et al., 2016), the presence of Fibrobacter in the gut of larvae fed polyethylene seems quite outstanding. The role of microbial hydrolase and oxidoreductase found in lignocellulosic degraders is well-known and is potentially useful for plastics degradation (Danso et al., 2019). The predominance of Fibrobacter sp. as well as Pseudomonas strains in the gut of larvae fed polyethylene and/or polystyrene could indicate that these bacteria have mechanisms to break down plastic polymers that are difficult to decompose.
The biomarker discriminant taxa analysis allowed detecting a major difference in predominant bacterial groups according to the treatment. The microbiota found in beeswax-fed larvae differed most considerably from the remaining bacterial groups since Fusobacteria were exclusively associated with this diet. Fusobacterium species are considered opportunistic pathogens in humans and other animals in which they compose the normal microbiota in the gastrointestinal tracts (Spector, 2009). Populations of Fusobacteria were found inhabiting the intestinal tract of T. molitor infected and uninfected with Hymenolepis diminuta (Fredensborg et al., 2020) as well as in the gut of the brown planthopper (Ojha and Zhang, 2019).
In relation to fungi, major changes in gut community composition under different diets were not observed in our study; in general, we found significant differences in richness and diversity (relative abundance), mostly Ascomycota characterized the guts of plastic-fed larvae, while Basidiomycota characterized the guts of beeswax-fed larvae. Common symbiotic gut fungi in insects are trichomycetes sensu lato (lineages within the Zygomycota; Cafaro, 2002; Valle and Stoianova, 2020), yeasts (Ascomycota; Malassigné et al., 2021), and Basidiomycota (Grigorescu et al., 2018). The genera we found in the guts of larvae in all three treatments were an unidentified Ascomycota group, followed by Sterigmatomyces (Basidiomycota), and Penidiella (Ascomycota), respectively. In accordance with our results, Zhang et al. (2020) identified Ascomycota in the guts of wax moth larvae consuming plastics, though Aspergillus flavus was not detected in our study. An undefined group of Capnodiales was present in low abundances only in the plastic treatments and this group differs from that of Aspergillus (Eurotiales). Species found in our study are not frequently reported in the literature regarding mechanical destruction with possible subsequent decomposition by microorganisms (Cf et al., 2021). Similarly to bacterial communities’ patterns, fungal species may differ geographically; but, we suggest that species that were present in all three treatments should be taken as candidate species when describing the core microbiota of G. mellonella.
Our co-occurrence analysis suggested associations between bacteria and fungi and their response to different diets in G. mellonella larvae for the first time. This approach takes into account synergic effects that could arise from the joint metabolic activities of bacteria and fungi in relation to the environment (e.g., Cucini et al., 2020). It has been previously used to describe multi-kingdom ensembles and their behavior under different conditions, in insects of economic importance (Guo et al., 2020), in ruminants (Pitta et al., 2016), and in humans (Godoy-Vitorino et al., 2018). In our study, it helped to identify five taxa that co-occurred in all treatments and individuals, and thus may be participating in core processes. This study also helped to evaluate the hypothesis that states that the dominance of some bacteria in gut communities may facilitate the entrance of entomopathogenic fungi (e.g., Gichuhi et al., 2020), which may have entered from the environment as a result of a depression of the immunological system driven by a low-quality diet such as plastics (e.g., Unckless et al., 2015). But this pattern was not supported by our data. The interactions between bacteria and fungi might play a key role in the plastic biodegradation process, and we showed a first step in identifying these associations using larvae in Argentina.
Our study is the first one to simultaneously identify bacterial and fungal communities associated with the gut of the Greater Wax Moth larvae fed with the two most common plastics: polyethylene (PE) and polystyrene (PS). In this sense, our results are promising in that it sheds light on possible alternatives of plastic pollution mitigation. However, the overproduction of plastic, especially single-use plastics, and its excessive discarding highlight the urgent need for responsible consumption by the entire society.
Data Availability Statement
Reads are publicly available and deposited in QIITA project ID 12844 with its associated metadata, as well as in EBI accession number ERP135546.
Author Contributions
JRB, EM, AG-C, AM, RC, AC, ACM-G, and FG-V contributed to the study conception and design. Material preparation was performed by JRB, EM, AG-C, MO, RC, AC, and ACM-G. Data collection and DNA sequencing were performed by BV-C and FG-V. Data analysis was performed by JRB, BV-C, EM, AG-C, AA, AM, MO, RC, ACM-G, and FG-V. The first draft of the manuscript was written by all authors. Financial resources were searched by EM, AG-C, AM, RC, AC, ACM-G, and FG-V. All authors contributed to the article and approved the submitted version.
Funding
Support for DNA sequencing and publication fees was provided by the PR-INBRE program BiRC core (NIH/NIGMS award number P20 GM103475), Agencia Nacional de Promoción Científica y Tecnológica (PICT2017-Ð833) from Argentina supported larvae acquisition, diet experiments, and DNA extraction costs. Secretaria de Estado e Innovación y Desarrollo Tecnológico from Tucumán, Argentina supported the costs of experiments.
Conflict of Interest
The authors declare that the research was conducted in the absence of any commercial or financial relationships that could be construed as a potential conflict of interest.
Publisher’s Note
All claims expressed in this article are solely those of the authors and do not necessarily represent those of their affiliated organizations, or those of the publisher, the editors and the reviewers. Any product that may be evaluated in this article, or claim that may be made by its manufacturer, is not guaranteed or endorsed by the publisher.
Acknowledgments
Silvina Garrido (INTA Alto Valle, Santa Cruz) donated the larvae reared at the Centro Multiplicador de Biocontroladores Nativos. Secretaría de Estado de Innovación y Desarrollo Tecnológico (SIDETEC 18) and Agencia Nacional de Promoción Científica y Tecnológica (PICT 2017-0833) funded part of this study. Additional support was provided by the PR-INBRE program BiRC core (NIH/NIGMS award number P20 GM103475).
Supplementary Material
The Supplementary Material for this article can be found online at: https://www.frontiersin.org/articles/10.3389/fmicb.2022.918861/full#supplementary-material
Footnotes
1. ^https://www.qiagen.com/zh-us/products/discovery-and-translational-research/dna-rna-purification/dna-purification/genomic-dna/dneasy-blood-and-tissue-kit/
References
Anderson, M. J. (2001). Permutation tests for univariate or multivariate analysis of variance and regression. Can. J. Fish. Aquat. Sci. 58, 626–639. doi: 10.1139/f01-004
Bombelli, P., Howe, C. J., and Bertocchini, F. (2017). Polyethylene bio-degradation by caterpillars of the wax moth galleria mellonella. Curr. Biol. 27, 292–293. doi: 10.1016/j.cub.2017.02.060
Brandon, A. M., Gao, S. H., Tian, R., Ning, D., Yang, S. S., Zhou, J., et al. (2018). Biodegradation of polyethylene and plastic mixtures in mealworms (larvae of Tenebrio molitor) and effects on the gut microbiome. Environ. Sci. Technol. 52, 6526–6533. doi: 10.1021/acs.est.8b02301
Bray, J. R., and Curtis, J. T. (1957). An ordination of the upland forest communities of southern Wisconsin. Ecol. Monogr. 27, 325–349. doi: 10.2307/1942268
Broderick, N. A., Raffa, K. F., Goodman, R. M., and Handelsman, J. (2004). Census of the bacterial community of the gypsy moth larval midgut by using culturing and culture-independent methods. Appl. Environ. Microbiol. 70, 293–300. doi: 10.1128/AEM.70.1.293-300.2004
Bucci, K., Tulio, M., and Rochman, C. M. (2019). What is known and unknown about the effects of plastic pollution: a meta-analysis and systematic review. Ecol. Appl. 30:e02044. doi: 10.1002/eap.2044
Cafaro, M. J. (2002). Species richness patterns in symbiotic gut fungi (Trichomycetes). Fungal Divers. 9, 47–56.
Caporaso, J. G., Kuczynski, J., Stombaugh, J., Bittinger, K., Bushman, F. D., Costello, E. K., et al. (2010). QIIME allows analysis of high-throughput community sequencing data. Nat. Methods 7, 335–336. doi: 10.1038/nmeth.f.303
Caporaso, J., Lauber, C. L., Walters, W. A., Berg-Lyons, D., Huntley, J., Fierer, N., et al. (2012). Ultra-high-throughput microbial community analysis on the Illumina HiSeq and MiSeq platforms. ISME J. 6, 1621–1624. doi: 10.1038/ismej.2012.8
Cassone, B. J., Grove, H. C., Elebute, O., Villanueva, S. M., and LeMoine, C. M. (2020). Role of the intestinal microbiome in low-density polyethylene degradation by caterpillar larvae of the greater wax moth, Galleria mellonella. Proc. R. Soc. B 287:20200112. doi: 10.1098/rspb.2020.0112
Cf, S. F., Rebello, S., Mathachan Aneesh, E., Sindhu, R., Binod, P., Singh, S., et al. (2021). Bioprospecting of gut microflora for plastic biodegradation. Bioengineered 12, 1040–1053. doi: 10.1080/21655979.2021.1902173
Coppotelli, B. M., Ibarrolaza, A., Dias, R. L., Del Panno, M. T., Berthe-Corti, L., and Morelli, I. S. (2010). Study of the degradation activity and the strategies to promote the bioavailability of phenanthrene by Sphingomonas paucimobilis strain 20006FA. Microb. Ecol. 59, 266–276. doi: 10.1007/s00248-009-9563-3
Cragg, S. M., Beckham, G. T., Bruce, N. C., Bugg, T. D. H., Distel, D. L., Dupree, P., et al. (2015). Lignocellulose degradation mechanisms across the tree of life. Curr. Opin. Chem. Biol. 29, 108–119. doi: 10.1016/j.cbpa.2015.10.018
Cucini, C., Leo, C., Vitale, M., Frati, F., Carapelli, A., and Nardi, F. (2020). Bacterial and fungal diversity in the gut of polystyrene-fed Alphitobius diaperinus (Insecta: Coleoptera). Animal Gene 17:200109. doi: 10.1016/j.angen.2020.200109
Danso, D., Chow, J., and Streita, W. R. (2019). Plastics: environmental and biotechnological perspectives on microbial degradation. Appl. Environ. Microbiol. 85:9519. doi: 10.1128/AEM.0109519
de Souza, R. S. C., Okura, V. K., Armanhi, J. S. L., Jorrín, B., Lozano, N., da Silva, M. J., et al. (2016). Unlocking the bacterial and fungal communities assemblages of sugarcane microbiome. Sci. Rep. 6:28774. doi: 10.1038/srep28774
Desai, M. S., and Brune, A. (2012). Bacteroidales ectosymbionts of gut flagellates shape the nitrogen-fixing community in dry-wood termites. ISME J. 6, 1302–1313. doi: 10.1038/ismej.2011.194
Dhariwal, A., Chong, J., Habib, S., King, I. L., Agellon, L. B., and Xia, J. (2017). MicrobiomeAnalyst: a web-based tool for comprehensive statistical, visual and meta-analysis of microbiome data. Nucleic Acids Res. 45, W180–W188. doi: 10.1093/nar/gkx295
Dice, L. R. (1945). Measures of the amount of ecologic association between species. Ecology 26, 297–302. doi: 10.2307/1932409
Espinosa, Cárdenas, Blanco, Andrea Colina, Schmidgall, Tabea, Atanasoff-Kardjalieff, Anna Katharina, Kappelmeyer, Uwe, Tischler, Dirk, et al. (2020). Toward biorecycling: isolation of a soil bacterium That grows on a polyurethane oligomer and monomer. Front. Microbiol. 11:404. doi: 10.3389/fmicb.2020.00404
Fredensborg, B. L., Fossdalí Kalvali, I. F., Johannesen, T. B., Stensvold, C. R., Nielsen, H. V., and Kapel, C. M. O. (2020). Parasites modulate the gut-microbiome in insects: A proof-of-concept study. PLoS One 15:e0227561. doi: 10.1371/journal.pone
Gautam, R., Bassi, A. S., and Yanful, E. K. (2007). A review of biodegradation of synthetic plastic and foams. Appl. Biochem. Biotechnol. 141, 85–108. doi: 10.1007/s12010-007-9212-6
Gichuhi, J., Khamis, F., Van den Berg, J., Mohamed, S., Ekesi, S., and Herren, J. K. (2020). Influence of inoculated gut bacteria on the development of Bactrocera dorsalis and on its susceptibility to the entomopathogenic fungus, Metarhizium anisopliae. BMC Microbiol. 20, 1–11. doi: 10.1186/s12866-020-02015-y
Godoy-Vitorino, F., Romaguera, J., Zhao, C., Vargas-Robles, D., Ortiz-Morales, G., Vázquez-Sánchez, F., et al. (2018). Cervicovaginal fungi and bacteria associated with cervical intraepithelial neoplasia and high-risk human papillomavirus infections in a hispanic population. Front. Microbiol. 9:2533. doi: 10.3389/fmicb.2018.02533
Gonzalez, A., Navas-Molina, J. A., Kosciolek, T., McDonald, D., Vázquez-Baeza, Y., Ackermann, G., et al. (2018). Qiita: rapid, web-enabled microbiome meta-analysis. Nat. Methods 15, 796–798. doi: 10.1038/s41592-018-0141-9
Grigorescu, A. S., Renoz, F., Sabri, A., Foray, V., Hance, T., and Thonart, P. (2018). Accessing the hidden microbial diversity of aphids: an illustration of how culture-dependent methods can be used to decipher the insect microbiota. Microb. Ecol. 75, 1035–1048. doi: 10.1007/s00248-017-1092-x
Guo, L. X., et al. (2020). Diversity and co-occurrence patterns of microbial communities in the fertilized eggs of Thitarodes insect, the implication on the occurrence of chinese Cordyceps (preprint). doi: 10.21203/rs.3.rs-24686/v1
Hammer, T. J., Janzenc, D. H., Hallwachsc, W., Jaffed, S. P., and Fierera, N. (2017). Caterpillars lack a resident gut microbiome. PNAS 114, 9641–9646. doi: 10.1073/pnas.1707186114
Hongoh, Y., Deevong, P., Inoue, T., Moriya, S., Trakulnaleamsai, S., Ohkuma, M., et al. (2005). Intra- and interspecific comparisons of bacterial diversity and community structure support coevolution of gut microbiota and termite host. Appl. Environ. Microbiol. 71, 6590–6599. doi: 10.1128/AEM.71
Hongoh, Y., Sharma, V. K., Prakash, T., Noda, S., Taylor, T. D., Kudo, T., et al. (2008). Complete genome of the uncultured termite group 1 bacteria in a single host protist cell. PNAS 105, 5555–5560. doi: 10.1073/pnas.0801389105
Jiang, S., Su, T., Zhao, J., and Wang, Z. (2021). Isolation, identification, and characterization of polystyrene-degrading bacteria from the gut of galleria mellonella (Lepidoptera: Pyralidae) larvae. Front. Bioeng. Biotechnol. 9:736062. doi: 10.3389/fbioe.2021.736062
Jindra, M., and Sehna, F. (1989). Larval growth, food consumption and utilization of dietary protein and energy in galleria mellonella. J. Insect Physiol. 35, 719–724. doi: 10.1016/0022-1910(89)90091-7
Kong, H. G., Kim, H. H., Chung, J. H., Jun, J. H., Lee, S., Kim, H. M., et al. (2019). The galleria mellonella hologenome supports microbiota-independent metabolism of long-chain hydrocarbon beeswax. Cell Rep. 26, 2451–2464.e5. doi: 10.1016/j.celrep.2019.02.018
Kwadha, C. A., Ong’amo, G. O., Ndegwa, P. N., Raina, S. K., and Fombong, A. T. (2017). The biology and control of the greater wax moth, Galleria mellonella. Insects 8:61. doi: 10.3390/insects8020061
LeMoine, C. M., Grove, H. C., Smith, C. M., and Cassone, B. J. (2020). A very hungry caterpillar: polyethylene metabolism and lipid homeostasis in larvae of the greater wax moth (galleria mellonella). Environ. Sci. Technol. 54, 14706–14715. doi: 10.1021/acs.est.0c04386
Leonov, V. D., and Tiunov, A. V. (2020). Interaction of invertebrates and synthetic polymers in soil: a review. Russ. J. Ecol. 51, 503–517. doi: 10.1134/S1067413620060041
Lou, Y., et al. (2020). Bio-degradation of polyethylene and polystyrene by greater wax moth larvae (galleria mellonella L.) and the effect of co-diet supplementation on the core gut microbiome. Environ. Sci. Technol. 54, 2821–2831. doi: 10.1021/acs.est.9b07044
Ma, Q., Cui, Y., Chu, X., Li, G., Yang, M., Wang, R., et al. (2021). Gut bacterial communities of Lymantria xylina and their associations with host development and diet. Microorganisms 9:1860. doi: 10.3390/microorganisms9091860
Malassigné, S., Minard, G., Vallon, L., Martin, E., Valiente Moro, C., and Luis, P. (2021). Diversity and functions of yeast communities associated with insects. Microorganisms 9:1552. doi: 10.3390/microorganisms9081552
Malizia, A., and Monmany-Garzia, A. C. (2019). Terrestrial ecologists should stop ignoring plastic pollution in the Anthropocene time. Sci. Total Environ. 668, 1025–1029. doi: 10.1016/j.scitotenv.2019.03.044
Mason, C. J., Jones, A. G., and Felton, G. W. (2019). Co-option of microbial associates by insects and their impact on plant–folivore interactions. Plant Cell Environ. 42, 1078–1086. doi: 10.1111/pce.13430
McCormick, A., Hoellein, T. J., Mason, S. A., Schluep, J., and Kelly, J. (2014). Microplastic is an abundant and distinct microbial habitat in an urban river. Environ. Sci. Technol. 48, 11863–11871. doi: 10.1021/es503610r
McMurdie, P. J., and Holmes, S. (2013). Phyloseq: an R package for reproducible interactive analysis and graphics of microbiome census data. PLoS One 8:e61217. doi: 10.1371/journal.pone.0061217
Mehmood, C. T., Qazi, I. A., Hashmi, I., Bhargava, S., and Deepa, S. (2016). Biodegradation of low density polyethylene (LDPE) modified with dye sensitized titania and starch blend using Stenotrophomonas pavanii. Int. Biodeterior. 113, 276–286. doi: 10.1016/j.ibiod.2016.01.025
Nilsson, R. H., Larsson, K. H., Taylor, A. F. S., Bengtsson-Palme, J., Jeppesen, T. S., Schigel, D., et al. (2018). The UNITE database for molecular identification of fungi: handling dark taxa and parallel taxonomic classifications. Nucleic Acids Res. 47, D259–D264. doi: 10.1093/nar/gky1022
Ojha, A., and Zhang, W. (2019). A comparative study of microbial community and dynamics of Asaia in the brown planthopper from susceptible and resistant rice varieties. BMC Microbiol. 19:139. doi: 10.1186/s12866-019-1512-9
Oksanen, J., et al. (2008). vegan: Community Ecology Package. R package version 1.17-2. Available at: http://cran.r-project.org/package=vegan, available in: vegan.r-forge.r-project.org.
Peydaei, A., Bagheri, H., Gurevich, L., de Jonge, N., and Nielsen, J. L. (2021). Mastication of polyolefins alters the microbial composition in galleria mellonella. Environ. Pollut. 280:116877. doi: 10.1016/j.envpol.2021.116877
Pitta, D. W., Pinchak, W. E., Indugu, N., Vecchiarelli, B., Sinha, R., and Fulford, J. D. (2016). Metagenomic analysis of the rumen microbiome of steers with wheat-induced frothy bloat. Front. Microbiol. 7:689. doi: 10.3389/fmicb.2016.00689
PlasticsEurope (2019). Plastics - the Facts 2019: an Analysis of European plastic Production, demand and Waste data. Plast. Eur. Brussels, Belgium.
Przemieniecki, S. W., Kosewska, A., Ciesielski, S., and Kosewska, O. (2019). Changes in the gut microbiome and enzymatic profile of Tenebrio molitor larvae biodegrading cellulose, polyethylene and polystyrene waste. Environ. Pollut. 256:113265. doi: 10.1016/j.envpol.2019.113265
Quast, C., Pruesse, E., Yilmaz, P., Gerken, J., Schweer, T., Yarza, P., et al. (2013). The SILVA ribosomal RNA gene database project: improved data processing and web-based tools. Nucleic Acids Res. 41, D590–D596. doi: 10.1093/nar/gks1219
R Development Core Team (2020). R: A Language and Environment for Statistical Computing. Vienna: R Foundation for Statistical Computing.
Ren, L., Men, L., Zhang, Z., Guan, F., Tian, J., Wang, B., et al. (2019). Biodegradation of polyethylene by Enterobacter Sp. D1 from the guts of wax moth galleria mellonella. Int. J. Environ. Res. Public Health 16:1941. doi: 10.3390/ijerph16111941
Revell, L. J. (2012). Phytools: An R package for phylogenetic comparative biology (and other things). Methods Ecol. Evol. 3, 217–223. doi: 10.1111/j.2041-210X.2011.00169.x
Rochman, C. M. (2018). Microplastics research—from sink to source. Science 360, 28–29. doi: 10.1126/science.aar7734
Russell, J. A., Moreau, C. S., Goldman-Huertas, B., Fujiwara, M., Lohman, D. J., and Pierce, N. E. (2009). Bacterial gut symbionts are tightly linked with the evolution of herbivory in ants. PNAS. USA. 106, 21236–21241. doi: 10.1073/pnas.0907926106
Sapountzis, P., Zhukova, M., Hansen, L. H., Sørensen, S. J., Schiøtt, M., and Boomsma, J. J. (2015). Acromyrmex leaf-cutting ants have simple gut microbiota with nitrogen-fixing potential. Appl. Environ. Microbiol. 81, 5527–5537. doi: 10.1128/AEM.00961-15
Segata, N., Izard, J., Waldron, L., Gevers, D., Miropolsky, L., Garrett, W. S., et al. (2011). Metagenomic biomarker discovery and explanation. Genome Biol. 12:R60. doi: 10.1186/gb-2011-12-6-r60
Shah, A. A., Hasan, F., Hameed, A., and Ahmed, S. (2008). Biological degradation of plastics: a comprehensive review. Biotechnol. Adv. 26, 246–265. doi: 10.1016/j.biotechadv.2007.12.005
Song, Y., Qiu, R., Hu, J., Li, X., Zhang, X., Chen, Y., et al. (2020). Biodegradation and disintegration of expanded polystyrene by land snails Achatina fulica. Sci. Total Environ. 746:141289. doi: 10.1016/j.scitotenv.2020.141289
Spector, M. P. (2009). Metabolism, Central (Intermediary). In Encyclopedia of Microbiology (pp. 242–264). Netherlands: Elsevier Inc.
Unckless, R. L., Rottschaefer, S. M., and Lazzaro, B. P. (2015). The complex contributions of genetics and nutrition to immunity in Drosophila melanogaster. PLoS Genet. 11:e1005030. doi: 10.1371/journal.pgen.1005030
Valle, L. G., and Stoianova, D. (2020). First record of Harpellales, Orphellales (Kickxellomycotina) and Amoebidiales (Mesomycetozoea) from Bulgaria, including a new species of Glotzia. MycoKeys 67, 55–80. doi: 10.3897/mycokeys.67.52055
Wang, Z., Xin, X., Shi, X., and Zhang, Y. (2020). A polystyrene-degrading Acinetobacter bacterium isolated from the larvae of Tribolium castaneum. Sci. Total Environ. 726:138564. doi: 10.1016/j.scitotenv.2020.138564
Wickham, H. (2007). Reshaping data with the reshape package. J. Stat. Softw. 21, 1–20. doi: 10.18637/jss.v021.i12
Wilkes, R. A., and Aristilde, L. (2017). Degradation and metabolism of synthetic plastics and associated products by Pseudomonas sp.: capabilities and challenges. J. Appl. Microbiol. 123, 582–593. doi: 10.1111/jam.13472
Woo, S., Song, I., and Cha, H. J. (2020). Fast and facile biodegradation of polystyrene by the gut microbial flora of Plesiophthalmus davidis larvae. Appl. Environ. Microbiol. 86, e01361–e01320. doi: 10.1128/AEM.01361-20
Yang, J., Yang, Y., Wu, W.-M., Zhao, J., and Jiang, L. (2014). Evidence of polyethylene biodegradation by bacterial strains from the guts of plastic-eating waxworms. Environ. Sci. Technol. 48, 13776–13784. doi: 10.1021/es504038a
Yang, Y., Yang, J., Wu, W. M., Zhao, J., Song, Y., Gao, L., et al. (2015a). Biodegradation and mineralization of polystyrene by plastic-eating mealworms: part 1. chemical and physical characterization and isotopic tests. Environ. Sci. Technol. 49, 12080–12086. doi: 10.1021/acs.est.5b02661
Yang, Y., Yang, J., Wu, W. M., Zhao, J., Song, Y., Gao, L., et al. (2015b). Biodegradation and mineralization of polystyrene by plastic-eating mealworms: part 2. role of gut microorganisms. Environ. Sci. Technol. 49, 12087–12093. doi: 10.1021/acs.est.5b02663
Zettler, E. R., Mincer, T. J., and Amaral-Zettler, L. A. (2013). Life in the “plastisphere”: microbial communities on plastic marine debris. Environ. Sci. Technol. 47, 7137–7146. doi: 10.1021/es401288x
Keywords: plastic pollution, insect gut, plastivore, Argentina, bacteria, fungi
Citation: Ruiz Barrionuevo JM, Vilanova-Cuevas B, Alvarez A, Martín E, Malizia A, Galindo-Cardona A, de Cristóbal RE, Occhionero MA, Chalup A, Monmany-Garzia AC and Godoy-Vitorino F (2022) The Bacterial and Fungal Gut Microbiota of the Greater Wax Moth, Galleria mellonella L. Consuming Polyethylene and Polystyrene. Front. Microbiol. 13:918861. doi: 10.3389/fmicb.2022.918861
Edited by:
Jose Luis Ramirez, United States Department of Agriculture (USDA), United StatesReviewed by:
Zhanyong Wang, Shenyang Agricultural University, ChinaOlga Yaroslavtseva, Institute of Systematics and Ecology of Animals (RAS), Russia
Krishnendu Mukherjee, University Hospital Münster, Germany
Copyright © 2022 Ruiz Barrionuevo, Vilanova-Cuevas, Alvarez, Martín, Malizia, Galindo-Cardona, de Cristóbal, Occhionero, Chalup, Monmany-Garzia and Godoy-Vitorino. This is an open-access article distributed under the terms of the Creative Commons Attribution License (CC BY). The use, distribution or reproduction in other forums is permitted, provided the original author(s) and the copyright owner(s) are credited and that the original publication in this journal is cited, in accordance with accepted academic practice. No use, distribution or reproduction is permitted which does not comply with these terms.
*Correspondence: A. Carolina Monmany-Garzia, acmonmany@gmail.com; Filipa Godoy-Vitorino, filipa.godoy@upr.edu