- Guangdong Provincial Engineering Laboratory of Biomass High Value Utilization, Institute of Biological and Medical Engineering, Guangdong Academy of Sciences, Guangzhou, China
Keratin-containing wastes become pollution to the environment if they are not treated properly. On the other hand, these wastes can be converted into value-added products applicable to many fields. Organic fertilizers and biofertilizers are important for sustainable agriculture by providing nutrients to enhance the growth speed of the plant and production. Keratin-containing wastes, therefore, will be an important resource to produce organic fertilizers. Many microorganisms exhibit capabilities to degrade keratins making them attractive to convert keratin-containing wastes into valuable products. In this review, the progress in microbial degradation of keratins is summarized. In addition, perspectives in converting keratin into bio- and organic fertilizers for agriculture are described. With proper treatment, feather wastes which are rich in keratin can be converted into high-value fertilizers to serve as nutrients for plants, reduce environmental pressure and improve the quality of the soil for sustainable agriculture.
Introduction
Modern agriculture can be considered as farming with improved technologies and strategies to increase food production and reduce the consumption of resources and energy. It brings in large quantities of high-quality and safe food to support and improve everyday life (Singh et al., 2021; Ranjha et al., 2022). The impact of modern agriculture on the environment is of great concern due to its effect on the soil, water, and our living environment (Figure 1). The high efficiency of food products results in loss of nutrients in the soil, which in turn will affect the yields of the crops in the future. The application of fertilizers and other chemicals such as pesticides will contaminate soil and groundwater, giving rise to threats to the environment (Humbert et al., 2016; Vejan et al., 2021; Martins-Gomes et al., 2022). Therefore, it is necessary to reduce the environmental pressure and recover the soil nutrient using suitable strategies (Zhuang et al., 2019; Tan et al., 2022). Sustainable agriculture was proposed to provide high-quality food for human beings without damaging the natural environment (Figure 1). Technologies, novel agriculture-related products, and strategies are explored to meet the requirement of sustainable agriculture by reducing soil erosion, relieving contamination of groundwater due to the application of artificial chemicals and excess amounts of fertilizers and recovering the eco-system by reducing the damages to the environment through bioremediation and applications of suitable fertilizers (Raliya et al., 2018; Ali et al., 2021; Rajput et al., 2021).
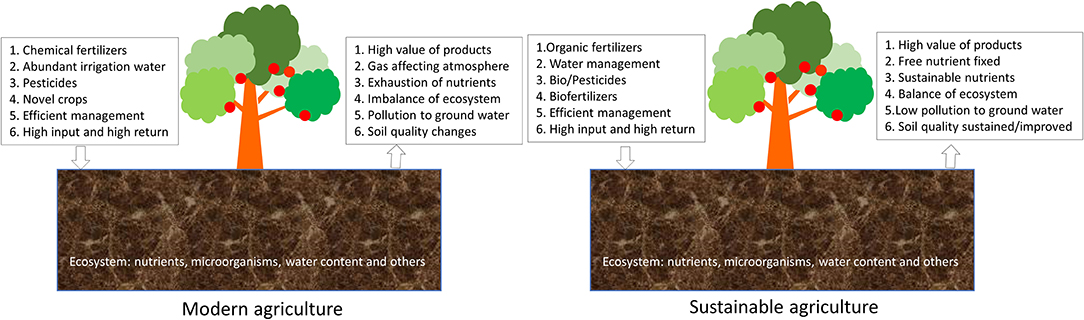
Figure 1. Modern agriculture and sustainable agriculture. The difference of these two types of agriculture is included.
Fertilizers for Agriculture
Fertilizers are usually rich in nutrients such as nitrogen, phosphorus, and potassium and can be prepared through different strategies (Figure 2) (Nazir et al., 2021; Ngo et al., 2021). Fertilizers can be classified into different types based on their components such as mineral fertilizers and methods in manufacture such as organic, inorganic fertilizers, and biofertilizers (Hirel et al., 2011; Liu et al., 2021; Urmi et al., 2022). The application of fertilizer improves the life of human beings while it also causes environmental problems (Herridge et al., 2008; Hirel et al., 2011; Zilio et al., 2022). Chemically synthesized fertilizers have been widely used in agriculture because of their high purity, easy application, and fast absorption by the plants (Hirel et al., 2011). With the wide application of fertilizers, their impact on the ecosystem such as atmosphere, soil microorganisms, and groundwater in soil has been highly recognized (Liu et al., 2021; Guo et al., 2022; Lisowska et al., 2022; Maeda, 2022). For example, the application of synthesized nitrogen-containing fertilizer can supply the nutrient for fast growth of crops while excess amount of nitrogen can be converted into nitrogen gas which can be released to the atmosphere to serve as one of the greenhouse gases causing global warming (Gao et al., 2022; Islam et al., 2022; Mikhael et al., 2022). Unutilized components from the fertilizers can be released into the groundwater to cause contamination. Therefore, suitable strategies are required to remove the contamination in the groundwater (Burow et al., 2010; Petzet and Cornel, 2011; Gu et al., 2013; Harris et al., 2022).
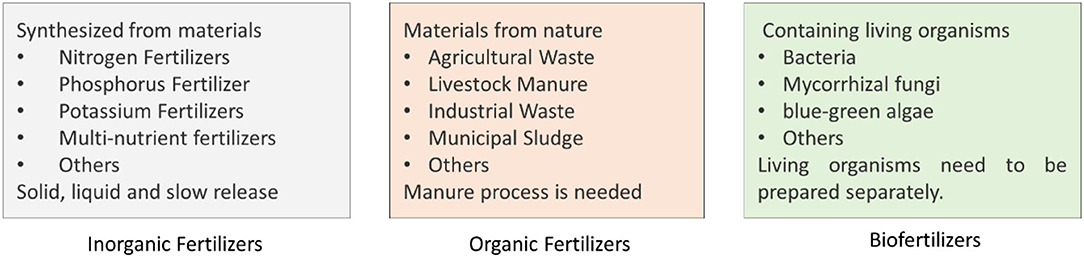
Figure 2. Three types of fertilizers. Three types of fertilizers based on different criteria are shown. Biofertilizers will be playing important roles in sustainable agriculture due to the advantages to sustain the ecosystem of soil and improving growth of the plants.
Biofertilizers in Agriculture
To avoid the potential threats caused by the overuse of chemical fertilizers to the ecosystem, organic fertilizers and biofertilizers have been recognized as an important element in sustainable agriculture (Abdel-Hamid et al., 2021; Kumar et al., 2022b; Yang et al., 2022). The combination of these fertilizers with chemical fertilizers can provide nutrients for plants and reduce the threat of chemical fertilizers (Kumar et al., 2022b). At the same time, these fertilizers can improve the environment of soil and solubilize the elements such as nitrogen and phosphate in soil to avoid loss of nutrients and contamination of the environment (Nosheen et al., 2022). The addition of microorganisms in the fertilizers will be very useful for balancing soil microorganisms, which will also be critical for plant growth and sustaining the ecosystem (Young and Ritz, 2000; van der Heijden et al., 2008; Saleem et al., 2019). Although both organic fertilizers and biofertilizers can be produced from animal and plant sources, biofertilizers contain microorganisms important for plant growth or the soil environment. Despite their differences, organic fertilizers can be mixed with biofertilizers to increase their efficiency (Al-Amri, 2021). Therefore, it is an economic way to produce biofertilizers using animal or plant wastes that are rich in carbon, nitrogen, and other elements. The resulting biofertilizer will contain both nutrients for the growth of plants and microorganisms that can be helpful for the ecosystem of soil. Keratin is a fibrous protein and presents in many wastes such as feathers from the poultry industry, representing a class of resource that can be converted into value-added chemicals. Feathers contain over 90% keratin and occupy 5–7% total weight of chicken (Adav et al., 2018; Li, 2019). Feathers have become one of the pollutants to the environment if they are not properly processed. Feathers can be converted into different materials used in different fields (Barone et al., 2005; Dias et al., 2010; Li, 2019; Tamreihao et al., 2019).
In this review, the application of feather-a keratin-containing waste in the generation of biofertilizers for sustainable agriculture is described. Feathers from the poultry industry will serve as a low-cost resource for producing fertilizers for agriculture, which overcomes the challenges in modern agriculture. With the inclusion of certain microorganisms, feather keratin can be converted into biofertilizers which contain nutrients for plant growth and microorganisms important for the soil ecosystem.
Keratin
Structure and Composition of Keratin
Keratin is a class of protein and presents in feathers, hair, hooves, wool, and nails (McKittrick et al., 2012; Chilakamarry et al., 2021). Keratin is a highly stable protein because of its high content of cysteine residues to form disulfide bonds and the presence of hydrogen bonds and hydrophobic interactions to stabilize its structure (Vidmar and Vodovnik, 2018). Fibrous keratin is not soluble even in some organic solvents, water, diluted acids, and alkaline solutions, making them resistant to normal proteases such as pepsin and trypsin (Vidmar and Vodovnik, 2018). Keratin can be classified into α-keratin and β-keratin according to the secondary structures of the protein chains (Fraser and Parry, 2008, 2011; Lange et al., 2016). The types and contents of amino acids affect the structures and properties of keratin (Qiu et al., 2020). The polypeptide chains in keratin are packed into a more complicated structure through inter- and intra-residue disulfide bonds via cysteine residues, hydrogen bonds, and hydrophobic interactions (Vidmar and Vodovnik, 2018). Based on the content of disulfide bonds, keratin is classified into soft and hard keratins. Feathers are hard keratins, and the main component of its keratins is mainly β-keratins. It has been noted that feathers also contain both α- and β-keratins. Like some proteins in human bodies, keratin can also be modified after translation such as phosphorylation, sumoylation, and glycosylation. Modification of keratin can play a role to affect its structure and stability (Snider and Omary, 2014). Like cellulose, lignin and hemicellulose are abundant in nature, keratin represents a class of renewable organic polymers (Lange et al., 2016; Bealer et al., 2020) and represents a valuable source for providing nutrients for both animals and plants (Pettett et al., 2004; Gurav and Jadhav, 2013; Bhari et al., 2021).
Keratin Waste-Feather
Feathers are one of the common byproducts in poultry industry. Due to increase of human population and higher demand for chicken-derived food, the yield of feather is increasing annually (Verma et al., 2017; da Silva, 2018). It is estimated that over 2 million tons of feathers generated from the poultry industry globally and proper treatment is needed to handle this byproduct to avoid generation of toxic reagents to the environment (Tamreihao et al., 2019). Feathers can be processed into some starting materials using different strategies to serve as a starting material, which will eliminate their impact on the environment. As feathers from poultry industry are always mixed with water and other wastes (Tamreihao et al., 2019), they provide an environment for the growth of many pathogenic microorganisms or contain some toxic compounds to the environment (Franke-Whittle and Insam, 2013; Dréano et al., 2021). In addition, the presence of microorganisms in feather waste could result in the releasing of pollutants such as nitrous oxide, ammonia, and hydrogen sulfide that can be released into the atmosphere (Tesfaye et al., 2017). Physical processing such as incineration and controlled landfilling will result in waste of such a large amount of organic substance (Tesfaye et al., 2017). Another way to treat feathers is to convert them as a material for making clothes, decorations, medical devices, fertilizers, dusters, bedding materials, and feedstocks using a fast and simple procedure such as washing and chemical treatment (Papadopoulos et al., 1986). Feathers can be converted into animal feeds through treatment with chemicals and steam pressure cooking method, but all these processes require a large amount of energy and the nutrients in feathers are not well maintained (Papadopoulos, 1989; Latshaw et al., 1994; Wang and Parsons, 1997). Converting feather waste into value-added products beneficial to the society using an economic strategy is of great interest to many researchers (Kang et al., 2018; Jain et al., 2016). Although chemical hydrolysis of feathers can produce soluble proteins and amino acids, it requires significant energies and impacts the quality of the product such as proteins and amino acids (Kim et al., 2001; Joardar and Rahman, 2018; Li, 2019). Accumulated studies have shown that microbial degradation of feathers is feasible and quite a number of microorganisms that are able to degrade keratin or keratin-containing wastes have been identified (Calin et al., 2017; Qu et al., 2018; Vidmar and Vodovnik, 2018; Bohacz and Korniłłowicz-Kowalska, 2019). The available studies suggested that it is feasible to convert feathers into value-added products such as biofertilizers through microorganisms, which provides a strategy to make full use of keratin from these wastes and generate low-cost products for sustainable agriculture (Tamreihao et al., 2019).
Microbial Degradation of Keratin
Keratin Degradation by Microorganisms
Keratin is resistant to normal proteases and not soluble in water while there is no accumulation of keratin wastes in nature, which is due to the contribution of microorganisms (Williams and Shih, 1989). Indeed, bacteria, actinomycetes, and fungi are shown to have the capability to degrade keratin (Calin et al., 2017; Bohacz and Korniłłowicz-Kowalska, 2019). Many researchers have isolated keratin-degrading microorganisms from different environments and proved that these microorganisms can be utilized to convert keratinous wastes into value-added products (de Menezes et al., 2021; Nnolim and Nwodo, 2021). These isolated microorganisms exhibited their potential to degrade keratin and keratin-containing wastes such as feathers into soluble fractions and amino acids (Callegaro et al., 2018; Shanmugasundaram et al., 2018; Bohacz, 2019; Tamreihao et al., 2019; Chaudhary et al., 2021). Fermentation conditions have been explored to obtain better keratin degradation efficiency (Rajput and Gupta, 2013). Both submerged and solid-state fermentation conditions were investigated to explore the optimal conditions for keratin degradation (De Azeredo et al., 2006). Mutagenesis induced by various ways has been explored to improve the efficiency. For example, ethyl methanesulfonate was applied to a bacterium Bacillus subtilis LFB-FIOCRUZ 1266. The resulting strains exhibited higher feather degradation efficiency with an improved rate of 15% over the wild-type strain (de Paiva et al., 2018).
Keratinases
Due to the insoluble property of keratin, enzymes secreted outside of the cells will play a major role in degradation (Williams and Shih, 1989; da Silva, 2018; Tamreihao et al., 2019; de Menezes et al., 2021). Because of the stable structure of keratin, normal proteases cannot degrade the substrate (Kasperova et al., 2013). Therefore, the degradation of keratin should include breaking the disulfide bonds followed by peptide degradation by proteases (Ramnani et al., 2005; Korniłłowicz-Kowalska and Bohacz, 2011). Although keratin degradation is a complicated step, these two steps including disulfide bond breakage by enzymes or reducing reagents and proteolysis of the peptides are indispensable. Other mechanisms such as mechanical destruction by fungi may also be included in keratin degradation (Korniłłowicz-Kowalska and Bohacz, 2011). As the disulfide bonds link polypeptides to form fibrous structures, breaking the disulfide bonds may be the first step in microbial degradation (Kasperova et al., 2013; Lange et al., 2016; Mercer and Stewart, 2018; Flückger et al., 2020). This step can be catalyzed by the produced inorganic sulfite and disulfide reductase (Korniłłowicz-Kowalska and Bohacz, 2011). Some disulfide reductases involved in keratin degradation have been isolated from microorganisms and characterized (Kasperova et al., 2013; Navone and Speight, 2018). Keratinases are another component for breaking the poly-peptides into shorter peptides and amino acids (Qiu et al., 2020). As a protease usually prefers to a cleavage site formed by specific residues, different types of proteases are needed to degrade keratin into amino acids completely (Qiu et al., 2020). Diverse proteases from different microorganisms have been identified with the molecular weights at the range from 20 to 130 kDa (Kanoksilapatham and Intagun, 2017; Sharma and Devi, 2018; Pinski et al., 2020; Qiu et al., 2020) and preference to various pHs, temperatures, and substrates (Tatineni et al., 2007; Tiwary and Gupta, 2010b; Rai and Mukherjee, 2011; Khodayari and Kafilzadeh, 2018; Arokiyaraj et al., 2019; Nnolim et al., 2020a; Almahasheer et al., 2022). Even the structures of the keratinases within the same protease family exhibit different folding and contain different structural elements (Figure 3).
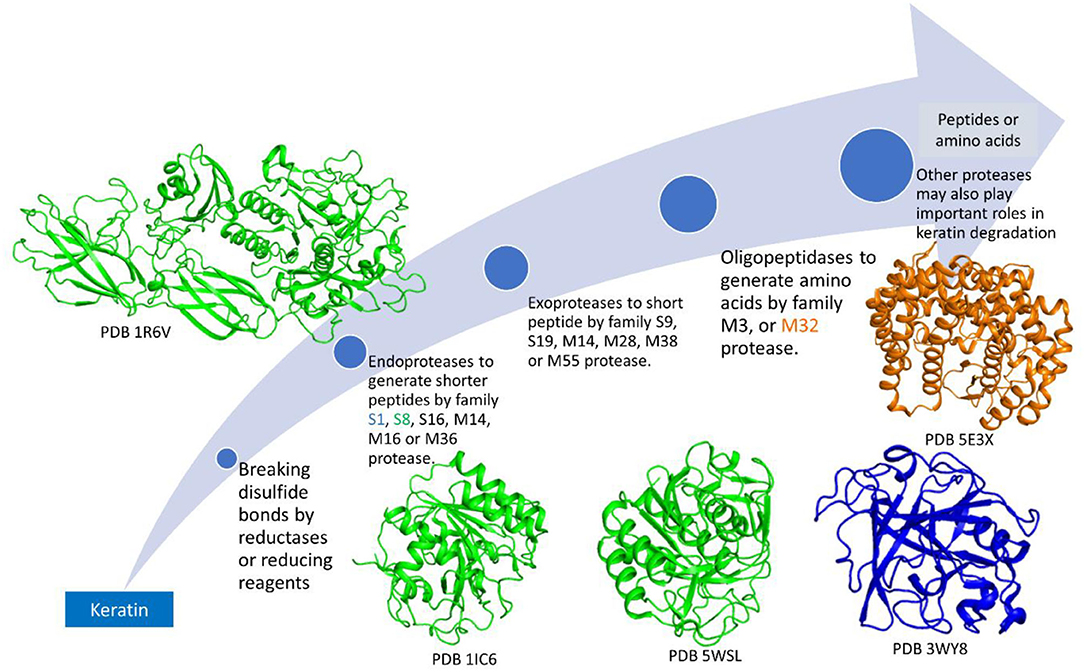
Figure 3. Microbial enzymes involved in keratin degradation. There are at least 14 different protease families identified in microorganisms. These proteases play different roles in keratin degradation. Keratinases among the same family may have different structures. The structures from protein databank (PDB) are shown with the access numbers indicated in the figure.
Recombinant keratinases can be obtained when the sequences are available while the selection of host cells and expression systems are critical because the target protein may not be easily overexpressed (Descamps et al., 2003; liu et al., 2013b; Fang et al., 2014; Yong et al., 2020; Parinayawanich et al., 2021; Yahaya et al., 2021). This method is very useful to obtain keratinases with high purify and study the enzymes from pathogenic microorganisms (Muhammed et al., 2021). Enzymatic characterization and mutagenesis can be readily studied using recombinant proteins (Zhang et al., 2020). Recombinant bacterial keratinases can be produced in Escherichia coli (E. coli) (Tiwary and Gupta, 2010a). Interestingly, the yield of the recombinant keratinase in expression systems such as E. coli may not be higher than that from its native source. To increase the yield of recombinant protein multiple copies of kerA gene were inserted into the chromosome of expression bacterial cells (Wang et al., 2004). The amino acid sequences of most keratinases are available, and the structures of several keratinases have been determined, which makes it possible to perform rational protein design for improving the activity and thermal stability of keratinases. Indeed, site-directed mutagenesis (N122Y, N217S, A193P, and N160C) was used to improve the keratinase of Bacillus licheniformis BBE11 (liu et al., 2013a). It is shown that point mutation N122Y in keratinase increased the enzymatic activity with an approximately 5.6-fold (liu et al., 2013a). As the N- and C-termini of a protease may play a regulatory role in the enzymatic activity, swapping these regions with similar domains of enzymes from other bacteria can also result in an enzyme with improved activity and stability (Fang et al., 2016; Peng et al., 2021).
Extensive studies have been performed to explore the strains, enzymes, and conditions required for the degradation of keratin and keratin-containing wastes such as feathers (Onifade et al., 1998; Gupta and Ramnani, 2006; Brandelli et al., 2010; Chaturvedi et al., 2014; Sahni et al., 2015; Sharma and Gupta, 2016; Vidmar and Vodovnik, 2018). It has been noted that most studies have been focused on a single strain, one enzyme using one substrate in the assay, and one processing condition. Keratin degradation is a complicated step that requires multiple strains or multiple enzymes in nature. A more comprehensive analytical strategy is needed to achieve an efficient degradation. Bacterial consortia might be of great interest to process keratin-containing wastes efficiently while more careful studies are needed to identify microorganisms required. Due to the amount of keratin in nature, it is not surprising that microbial keratinases would have industrial and biotechnological applications (Table 1) (Onifade et al., 1998; Gupta and Ramnani, 2006; Rai et al., 2009; Syed et al., 2009; Brandelli et al., 2010; Sahni et al., 2015; Kanoksilapatham and Intagun, 2017; Gegeckas et al., 2018; Vidmar and Vodovnik, 2018). Keratin is rich in carbon, nitrogen, and sulfur which can be converted into diverse products (McKittrick et al., 2012; Wang et al., 2016). Modern agriculture requires certain types of fertilizers that can recover the loss of nutrients in soil and sustain the microbial ecosystem for the growth of plants and fixing/solubilizing nutrients in soil. Keratin-containing wastes such as feathers and its degradation bacteria will be a source for producing biofertilizers with a combination of the merits from both organic fertilizer and biofertilizers for sustainable agriculture.
Converting Keratin to Biofertilizers
Keratin degrading microorganisms and the related enzymes have been summarized (Adav et al., 2018; Li, 2019, 2021; Hassan et al., 2020a; Qiu et al., 2020). Keratin and keratin-containing wastes such as feathers are a valuable source for carbon, nitrogen, and sulfur because of the components of polypeptides in the wastes (Pettett et al., 2004; Gurav and Jadhav, 2013; Qiu et al., 2020) (Figure 4). During the growth of microorganisms, other nutrients such as phosphate will be taken from the medium for energy synthesis and building up cell organelles such as cell membrane. Keratin-containing wastes will play a role in sustainable agriculture by providing value-added products (da Silva, 2018; Shestakova et al., 2021). The keratin hydrolysate influences the plant growth because of the peptides and amino acids which play versatile roles in plants (Bhari et al., 2021). These products have been shown to have an impact on the stimulation of carbon and nitrogen metabolism, which may result in the difference in seeds germination, seedling growth, and activation of the plant proton pump (Calin et al., 2019). Peptides in the keratin hydrolysate may exhibit hormonal activities. These products can also play a role in stimulating plant growth through their indirect effect on nutrient update and usage (Raguraj et al., 2022). The amino acids in keratin hydrolysate will have an impact on the rhizospheric microbes by serving as a nitrogen source, which in turn will affect the action of the microorganism on the root of the plant (Moe, 2013). Therefore, hydrolysate derived from keratin wastes is a promising biofertilizer. Studies have shown that microbial keratin hydrolysate can serve as a biofertilizer to promote plant growth (Table 2). Addition of hydrolysate such as 5 g feather in 250 g soil and 1 kg in 10 kg soil enhanced plant growth (Kumari and Kumar, 2020). Biofertilizers derived from keratin-containing wastes can be obtained through the following mechanisms. First, the polyproteins in keratin can be converted into amino acids to serve as nitrogen sources for plant growth (Geisseler et al., 2021). Second, the microorganisms used in waste treatment will be another source of nutrients for the plant growth. The microbial products of feathers can be used as a fertilizer to improve the growth of plants (Gurav et al., 2020; Ortiz and Sansinenea, 2021). Third, the microorganisms can also play a role in absorbing the excess inorganic nutrient in soil released from synthesized fertilizers to prevent nutrient loss (Maitra et al., 2022). Lastly, mixing other microorganisms used in biofertilizers with microbial-treated keratin wastes will result in a new fertilizer with the properties of both organic fertilizers and biofertilizers (Mitter et al., 2019). An ideal microorganism is the one used in biofertilizers with the capability to degrade keratins.

Figure 4. Keratin and its potential to be converted into biofertilizers. The source of keratin and its conversion to biofertilizers are listed. Keratin-containing wastes, structures of keratin, methods for keratin treatment, keratin products, and application of biofertilizers are indicated.
Unlike organic and inorganic fertilizers rich in nutrients, biofertilizers contain living microbes that play important roles in promoting the growth of crops through enlarging the supply of essential nutrients absorbable by the crops (Jiménez-Mejía et al., 2022; Maitra et al., 2022). These living organisms that are helpful for plant growth usually include bacteria, blue-green algae, and mycorrhizal fungi (Kumar et al., 2022b) and many types of biofertilizers are commercially available. These organisms can help extracting minerals from organic materials or turning nutrients from one type of forms to another such as nitrogen fixation (Pirttilä et al., 2021; Kumar et al., 2022b) (Figure 4). The following types of microorganisms are commonly used in biofertilizers. Rhizobium is one of the symbiotic nitrogen-fixing bacteria used in biofertilizers and able to fix nitrogen for growth of the plants (Gopalakrishnan et al., 2015; Mendoza-Suárez et al., 2021). Azospirillum live at the root of the plants serving as nitrogen-fixing bacteria without developing an intimate relationship with plants (Berg, 2009). Some organisms such as cyanobacteria have a symbiotic association with the plants can also be used in biofertilizers (Bhattacharjee et al., 2008; Bononi et al., 2020). Due to the association of this type of organisms with plants, potential challenges may occur during the preparation or storage of the biofertilizers. Other bacteria whose growth and living are not relying on the plants such as free-living nitrogen-fixing bacteria are of great interest in keratin-derived biofertilizers (Lucy et al., 2004; Deka et al., 2020).
The organisms used in keratin degradation have different mechanisms of action from those applied in biofertilizers (Korniłłowicz-Kowalska and Bohacz, 2011; Ortiz and Sansinenea, 2021; Sypka et al., 2021). Theoretically, the presence of microbial keratin hydrolysate in soil will play a role in the ecosystem because the growth of microorganisms also results in nitrogen-fixing and absorption of phosphate and potassium from the soil (Kumar et al., 2022b). Microbial keratin hydrolysate containing mixtures of microorganisms and products can be considered as a form of biofertilizers because of the presence of living organisms and nutrients. To achieve higher benefit to the ecosystem, the following strategies will be useful. First, the selection of suitable keratin-degrading organisms in wastes treatment should be considered. Some microorganisms such as Bacillus subtilis can degrade keratin and play a role in phosphate solubilization to enhance plant nutrient uptake (Santoyo et al., 2012; Jeevana Lakshmi et al., 2013; Chaturvedi et al., 2014; Gupta et al., 2017; de Paiva et al., 2018; Kumar et al., 2022b). This type of organisms will be the ideal candidate for converting keratin-containing wastes into biofertilizers. To obtain such organisms, samples used for strain isolation of keratin degrading microorganisms should be obtained from soil or other environment where the organisms beneficial for growth of plant can survive (Jagadeesan et al., 2020). Second, mixing multiple organisms during the processing of keratin-containing wastes may improve the effect of biofertilizer on plant growth (Zahra et al., 2020). Two classes of organisms are important. One class of organisms can degrade keratin and the other will be beneficial for plant growth and the soil ecosystem. The later class of organisms can be mixed with microbial keratin hydrolysate to generate a fertilizer with the function of both organic and biofertilizers (Sabir et al., 2021). Lastly, in the microbial treatment of keratin-containing wastes such as feathers, mixing the processed wastes with other wastes such as cellulose and organisms can be considered (Brandelli et al., 2015; Bohacz, 2019; Patinvoh et al., 2020), which might provide a more complicated system for both plant and organism growth.
Perspectives
With the development of economics and the increment of global population, the demand for food and meat such as chicken is increasing. Feathers production from the poultry industry is growing rapidly and this keratin-containing waste can be a threat to the environment and cause a waste of carbon and nitrogen sources if it is not treated properly (Pahua-Ramos et al., 2017; Kowalczyk et al., 2018). The quality of soil needs to be sustained when the quality and quantity of food production are enhanced in modern agriculture. To maintain sustainable agriculture, it is essential to reduce the usage of inorganic fertilizers and balance the ecosystem in soil by application of new fertilizers (Young and Ritz, 2000; Orr et al., 2011; Liu et al., 2021). Organic fertilizers and biofertilizers are indispensable for sustainable agriculture (Hernández-Fernández et al., 2021; Wang et al., 2021; Kumar et al., 2022a). Converting keratin-containing wastes into biofertilizers can overcome some disadvantages of organic fertilizers such as toxicity to plants and soil under certain conditions, and low nutrient content of organic mulches.
To produce biofertilizers using keratin-containing materials, microbial treatment of keratins is feasible (Tamreihao et al., 2019; Nnolim et al., 2020b; Izydorczyk et al., 2022). The strains used in treatment need to be identified or selected from strain libraries. The selection of microbial consortia is of great interest while more studies are still needed to identify or build up a suitable system for keratin degradation. Breaking the disulfide bonds in keratin to release the polypeptides is a critical step to release soluble proteins that can be readily used by many organisms (Gurung et al., 2018; Sharma and Devi, 2018; Qiu et al., 2020; Nnolim and Nwodo, 2021). Studies on the detailed components involved in keratin degradation are still required. With the development of analytical methods such as mass spectrometry and wide application of genome sequencing, the degradation of keratin can be analyzed in more details (Khodayari and Kafilzadeh, 2018; Navone and Speight, 2018; Fang et al., 2019; Hamiche et al., 2019; Hassan et al., 2020b; Yahaya et al., 2021). The addition of organisms suitable for the growth of plants to keratin degradation product will result in a high-quality biofertilizer to provide nutrients for the growth of plant, sustain the microorganism in the soil and reduce the usage of pesticides (Figure 5). The organisms used in conventional biofertilizers can be screened from the soil or purchased from commercial resources, which can be applied in keratin treatment or mixed with microbial-treated keratins to produce biofertilizers with multiple functions to contribute to the sustainable agriculture (Bano et al., 2021; Kumar et al., 2021; Lee et al., 2021; Rios-Galicia et al., 2021; Seenivasagan and Babalola, 2021).
In summary, keratin-containing waste is rich in amino acids serving as a valuable resource for the growth of plants. With proper treatment, wastes such as feathers can be converted into biofertilizers to play a role in sustainable agriculture. With a proper microbial treatment of feathers and the addition of other organisms, feathers will be converted into biofertilizers applied to sustainable agriculture.
Author Contributions
The author confirms being the sole contributor of this work and has approved it for publication.
Funding
This research was supported by funds from the Hundred-Talent Program (Grant Numbers: 2020GDASYL-20200102010 and 2020GDASYL-20200102009) and GDAS' Project of Science and Technology Development (2022GDASZH-2022010110), Guangdong Academy of Sciences, China.
Conflict of Interest
The author declares that the research was conducted in the absence of any commercial or financial relationships that could be construed as a potential conflict of interest.
Publisher's Note
All claims expressed in this article are solely those of the authors and do not necessarily represent those of their affiliated organizations, or those of the publisher, the editors and the reviewers. Any product that may be evaluated in this article, or claim that may be made by its manufacturer, is not guaranteed or endorsed by the publisher.
Acknowledgments
The author appreciates the support from Guangdong Academy of Sciences.
References
Abdel-fattah, A. M., Nashy, E. L. S. H.A., Sabiel, E.-T. A., Hussien, M. M., and Attia, A. S. (2015). Novel keratinolytic activity of cyberlindnera fabianii nrc3 aza as a plant growth promoting agent (PGPA). Int. J. Appl. Sci. 3, 609–618. doi: 10.3126/ijasbt.v3i4.13683
Abdel-Hamid, M. S., Fouda, A., El-Ela, H. K. A., El-Ghamry, A. A., and Hassan, S. E.-D. (2021). Plant growth-promoting properties of bacterial endophytes isolated from roots of Thymus vulgaris L. and investigate their role as biofertilizers to enhance the essential oil contents. Biomol. Concepts. 12, 175–196. doi: 10.1515/bmc-2021-0019
Adav, S. S., Subbaiaih, R. S., Kerk, S. K., Lee, A. Y., Lai, H. Y., Ng, K. W., et al. (2018). Studies on the proteome of human hair - identification of histones and deamidated keratins. Sci. Rep. 8, 1599. doi: 10.1038/s41598-018-20041-9
Adetunji, C. O., Makanjuola, O. R., Arowora, K. A., Afolayan, S. S., and Adetunji, J. B. (2012). Production and Application of Keratin-Based Organic Fertilizer from Microbially Hydrolyzed Feathers to cowpea. Int. J. Sci. Eng. Res. 3. Available online at: https://www.ijser.org/paper/Production-and-Application-of-KeratinBased-Organic-Fertilizer-from-Microbially-Hydrolyzed-Feathers-to-cowpea.html
Al-Amri, S. M. (2021). Response of growth, essential oil composition, endogenous hormones and microbial activity of Mentha piperita to some organic and biofertilizers agents. Saudi J. Biol. Sci. 28, 5435–5441. doi: 10.1016/j.sjbs.2021.06.094
Ali, S. S., Al-Tohamy, R., Koutra, E., Moawad, M. S., Kornaros, M., Mustafa, A. M., et al. (2021). Nanobiotechnological advancements in agriculture and food industry: Applications, nanotoxicity, and future perspectives. Sci. Total Environ. 792, 148359. doi: 10.1016/j.scitotenv.2021.148359
Almahasheer, A. A., Mahmoud, A., El-Komy, H., Alqosaibi, A. I., Aktar, S., AbdulAzeez, S., et al. (2022). Novel feather degrading keratinases from Bacillus cereus group: biochemical, genetic and bioinformatics analysis. Microorganisms. 10, 93. doi: 10.3390/microorganisms10010093
Arokiyaraj, S., Varghese, R., Ali Ahmed, B., Duraipandiyan, V., and Al-Dhabi, N. A. (2019). Optimizing the fermentation conditions and enhanced production of keratinase from Bacillus cereus isolated from halophilic environment. Saudi J. Biol. Sci. 26, 378–381. doi: 10.1016/j.sjbs.2018.10.011
Bano, S., Wu, X., and Zhang, X. (2021). Towards sustainable agriculture: rhizosphere microbiome engineering. Appl. Microbiol. Biotechnol. 105, 7141–7160. doi: 10.1007/s00253-021-11555-w
Barone, J. R., Schmidt, W. F., and Liebner, C. F. E. (2005). Thermally processed keratin films. J. Appl. Polym. Sci. 97, 1644–1651. doi: 10.1002/app.21901
Bealer, E. J., Onissema-Karimu, S., Rivera-Galletti, A., Francis, M., Wilkowski, J., Salas-de la Cruz, D., et al. (2020). Protein–polysaccharide composite materials: fabrication and applications. Polymers. 12, 464. doi: 10.3390/polym12020464
Berg, G. (2009). Plant–microbe interactions promoting plant growth and health: perspectives for controlled use of microorganisms in agriculture. Appl. Microbiol. Biotechnol. 84, 11–18. doi: 10.1007/s00253-009-2092-7
Bhange, K., Chaturvedi, V., and Bhatt, R. (2016). Ameliorating effects of chicken feathers in plant growth promotion activity by a keratinolytic strain of Bacillus subtilis PF1. Biores. Biopro. 3, 13. doi: 10.1186/s40643-016-0091-y
Bhari, R., Kaur, M., and Sarup Singh, R. (2021). Chicken feather waste hydrolysate as a superior biofertilizer in agroindustry. Curr. Microbiol. doi: 10.1007/s00284-021-02491-z
Bhattacharjee, R. B., Singh, A., and Mukhopadhyay, S. N. (2008). Use of nitrogen-fixing bacteria as biofertiliser for non-legumes: prospects and challenges. Appl. Microbiol. Biotechnol. 80, 199–209. doi: 10.1007/s00253-008-1567-2
Bohacz, J. (2019). Changes in mineral forms of nitrogen and sulfur and enzymatic activities during composting of lignocellulosic waste and chicken feathers. Environ. Sci. Pollut. Res. 26, 10333–42. doi: 10.1007/s11356-019-04453-2
Bohacz, J., and Korniłłowicz-Kowalska, T. (2019). Fungal diversity and keratinolytic activity of fungi from lignocellulosic composts with chicken feathers. Proc. Biochem. 10, 2717. doi: 10.1016/j.procbio.2019.02.012
Bononi, L., Chiaramonte, J. B., Pansa, C. C., Moitinho, M. A., and Melo, I. S. (2020). Phosphorus-solubilizing Trichoderma spp. from Amazon soils improve soybean plant growth. Sci. Rep. 10, 2858. doi: 10.1038/s41598-020-59793-8
Bose, A., Pathan, S., Pathak, K., and Keharia, H. (2014). Keratinolytic protease production by bacillus amyloliquefaciens 6b using feather meal as substrate and application of feather hydrolysate as organic nitrogen input for agricultural soil. Waste Biomass Valorization. 5, 595–605. doi: 10.1007/s12649-013-9272-5
Brandelli, A., Daroit, D. J., and Riffel, A. (2010). Biochemical features of microbial keratinases and their production and applications. Appl. Microbiol. Biotechnol. 85, 1735–1750. doi: 10.1007/s00253-009-2398-5
Brandelli, A., Sala, L., and Kalil, S. J. (2015). Microbial enzymes for bioconversion of poultry waste into added-value products. Food Res. Int. 73, 3–12. doi: 10.1016/j.foodres.2015.01.015
Burow, K. R., Nolan, B. T., Rupert, M. G., and Dubrovsky, N. M. (2010). Nitrate in groundwater of the United States, 1991–2003. Environ. Sci. Technol. 44, 4988–4997. doi: 10.1021/es100546y
Calin, M., Constantinescu-Aruxandei, D., Alexandrescu, E., Raut, I., Doni, M. B., Arsene, M.-L., et al. (2017). Degradation of keratin substrates by keratinolytic fungi. Electron. J. Biotechnol. 28, 101–112. doi: 10.1016/j.ejbt.2017.05.007
Calin, M., Raut, I., Arsene, M. L., Capra, L., Gurban, A. M., Doni, M., et al. (2019). Applications of fungal strains with keratin-degrading and plant growth promoting characteristics. Agronomy. 9, 543. doi: 10.3390/agronomy9090543
Callegaro, K., Welter, N., and Daroit, D. J. (2018). Feathers as bioresource: microbial conversion into bioactive protein hydrolysates. Proc. Biochem. 75, 1–9. doi: 10.1016/j.procbio.2018.09.002
Chaturvedi, V., Bhange, K., Bhatt, R., and Verma, P. (2014). Production of kertinases using chicken feathers as substrate by a novel multifunctional strain of Pseudomonas stutzeri and its dehairing application. Biocatal. Agric. Biotechnol. 3, 167–174. doi: 10.1016/j.bcab.2013.08.005
Chaudhary, L., Siddiqui, M. H., Vimal, A., and Bhargava, P. (2021). Biological degradation of keratin by microbial keratinase for effective waste management and potent industrial applications. Curr. Protein Pept. Sci. doi: 10.2174/1389203722666210215151952
Chilakamarry, C. R., Mahmood, S., Saffe, S. N. B. M., Arifin, M. A. B., Gupta, A., Sikkandar, M. Y., et al. (2021). Extraction and application of keratin from natural resources: a review. Biotech. 11, 220. doi: 10.1007/s13205-021-02734-7
da Silva, R. R. (2018). Keratinases as an alternative method designed to solve keratin disposal on the environment: its relevance on agricultural and environmental chemistry. J. Agric. Food Chem. 66, 7219–7221. doi: 10.1021/acs.jafc.8b03152
De Azeredo, L. A. I., De Lima, M. B., Coelho, R. R. R., and Freire, D. M. G. (2006). Thermophilic protease production by Streptomyces sp. 594 in submerged and solid-state fermentations using feather meal. J. Appl. Microbiol. 100, 641–647. doi: 10.1111/j.1365-2672.2005.02791.x
de Menezes, C. L. A., Santos, R. D. C., Santos, M. V., Boscolo, M., da Silva, R., Gomes, E., et al. (2021). Industrial sustainability of microbial keratinases: production and potential applications. World J. Microbiol. Biotechnol. 37, 86. doi: 10.1007/s11274-021-03052-z
de Paiva, D. P., de Oliveira, S. S. A., Mazotto, A. M., Vermelho, A. B., and de Oliveira, S. S. (2018). Keratinolytic activity of Bacillus subtilis LFB-FIOCRUZ 1266 enhanced by whole-cell mutagenesis. Biotech. 9, 2. doi: 10.1007/s13205-018-1527-1
Deka, D., Sonowal, S., Chikkaputtaiah, C., and Velmurugan, N. (2020). Symbiotic associations: key factors that determine physiology and lipid accumulation in oleaginous microorganisms. Front. Microbiol. 11. doi: 10.3389/fmicb.2020.555312
Descamps, F., Brouta, F., Vermout, S., Monod, M., Losson, B., and Mignon, B. (2003). Recombinant expression and antigenic properties of a 31.5-kDa keratinolytic subtilisin-like serine protease from Microsporum canis. FEMS Microbiol. Immunol. 38, 29–34. doi: 10.1016/S0928-8244(03)00101-9
Dias, G. J., Peplow, P. V., McLaughlin, A., Teixeira, F., and Kelly, R. J. (2010). Biocompatibility and osseointegration of reconstituted keratin in an ovine model. J. Biomed. Mater Res. A. 92A, 513–520. doi: 10.1002/jbm.a.32394
Dréano, E., Laurentie, M., Hurtaud-Pessel, D., and Mompelat, S. (2021). Multi–class analysis of 30 antimicrobial residues in poultry feathers by liquid chromatography tandem mass spectrometry. Food Additives Contaminants A. 38, 1701–1716. doi: 10.1080/19440049.2021.1942561
Fang, Z., Sha, C., Peng, Z., Zhang, J., and Du, G. (2019). Protein engineering to enhance keratinolytic protease activity and excretion in Escherichia coli and its scale-up fermentation for high extracellular yield. Enzyme Microb. Technol. 121, 37–44. doi: 10.1016/j.enzmictec.2018.11.003
Fang, Z., Zhang, J., Liu, B., Du, G., and Chen, J. (2016). Enhancement of the catalytic efficiency and thermostability of Stenotrophomonas sp. keratinase KerSMD by domain exchange with KerSMF. Microbial Biotechnol. 9, 35–46. doi: 10.1111/1751-7915.12300
Fang, Z., Zhang, J., Liu, B., Jiang, L., Du, G., and Chen, J. (2014). Cloning, heterologous expression and characterization of two keratinases from Stenotrophomonas maltophilia BBE11-1. Proc. Biochem. 49, 647–654. doi: 10.1016/j.procbio.2014.01.009
Flückger, S., Igareta, N. V., and Seebeck, F. P. (2020). Convergent Evolution of Fungal Cysteine Dioxygenases. Chembiochem. 21, 3082–3086. doi: 10.1002/cbic.202000317
Franke-Whittle, I. H., and Insam, H. (2013). Treatment alternatives of slaughterhouse wastes, and their effect on the inactivation of different pathogens: a review. Crit. Rev. Microbiol. 39, 139–151. doi: 10.3109/1040841X.2012.694410
Fraser, R. D. B., and Parry, D. A. D. (2008). Molecular packing in the feather keratin filament. J. Struct. Biol. 162, 1–13. doi: 10.1016/j.jsb.2008.01.011
Fraser, R. D. B., and Parry, D. A. D. (2011). The structural basis of the filament-matrix texture in the avian/reptilian group of hard β-keratins. J. Struct. Biol. 173, 391–405. doi: 10.1016/j.jsb.2010.09.020
Gao, H., Tian, H., Zhang, Z., and Xia, X. (2022). Warming-induced greenhouse gas fluxes from global croplands modified by agricultural practices: a meta-analysis. Sci. Total Environ. 153288. doi: 10.1016/j.scitotenv.2022.153288
Gegeckas, A., Šimkute, A., Gudiukaite, R., and Citavičius, D. J. (2018). Characterization and application of keratinolytic paptidases from Bacillus spp. Int. J. Biol. Macromol. 113, 1206–1213. doi: 10.1016/j.ijbiomac.2018.03.046
Geisseler, D., Smith, R., Cahn, M., and Muramoto, J. (2021). Nitrogen mineralization from organic fertilizers and composts: literature survey and model fitting. J. Environ. Qual. 50, 1325–1338. doi: 10.1002/jeq2.20295
Gopalakrishnan, S., Sathya, A., Vijayabharathi, R., Varshney, R. K., Gowda, C. L. L., and Krishnamurthy, L. (2015). Plant growth promoting rhizobia: challenges and opportunities. Biotech 5, 355–377. doi: 10.1007/s13205-014-0241-x
Gu, B., Ge, Y., Chang, S. X., Luo, W., and Chang, J. (2013). Nitrate in groundwater of China: Sources and driving forces. Global Environm. Change. 23, 1112–1121. doi: 10.1016/j.gloenvcha.2013.05.004
Guo, Z., Lv, L., Liu, D., He, X., Wang, W., Feng, Y., et al. (2022). A global meta-analysis of animal manure application and soil microbial ecology based on random control treatments. PLoS ONE. 17, e0262139. doi: 10.1371/journal.pone.0262139
Gupta, R., and Ramnani, P. (2006). Microbial keratinases and their prospective applications: an overview. Appl. Microbiol. Biotechnol. 70, 21. doi: 10.1007/s00253-005-0239-8
Gupta, S., Tewatia, P., Misri, J., and Singh, R. (2017). Molecular modeling of cloned bacillus subtilis keratinase and its insinuation in psoriasis treatment using docking studies. Indian J. Microbiol. 57, 485–491. doi: 10.1007/s12088-017-0677-x
Gurav, R., Nalavade, V., Aware, C., Vyavahare, G., Bhatia, S. K., Yang, Y.-H., et al. (2020). Microbial degradation of poultry feather biomass in a constructed bioreactor and application of hydrolysate as bioenhancer to vegetable crops. Environ. Sci. Pollut. Res. 27, 2027–2035. doi: 10.1007/s11356-019-06536-6
Gurav, R. G., and Jadhav, J. P. (2013). A novel source of biofertilizer from feather biomass for banana cultivation. Environ. Sci. Pollut. Res. 20, 4532–4539. doi: 10.1007/s11356-012-1405-z
Gurung, S. K., Adhikari, M., Kim, S. W., Bazie, S., Kim, H. S., Lee, H. G., et al. (2018). Discovery of two chrysosporium species with keratinolytic activity from field soil in Korea. Mycobiology 46, 260–268. doi: 10.1080/12298093.2018.1514732
Hamiche, S., Mechri, S., Khelouia, L., Annane, R., El Hattab, M., Badis, A., et al. (2019). Purification and biochemical characterization of two keratinases from Bacillus amyloliquefaciens S13 isolated from marine brown alga Zonaria tournefortii with potential keratin-biodegradation and hide-unhairing activities. Int. J. Biol. Macromol. 122, 758–769. doi: 10.1016/j.ijbiomac.2018.10.174
Harris, S. J., Cendón, D. I., Hankin, S. I., Peterson, M. A., Xiao, S., and Kelly, B. F. J. (2022). Isotopic evidence for nitrate sources and controls on denitrification in groundwater beneath an irrigated agricultural district. Sci. Total Environ. 817, 152606. doi: 10.1016/j.scitotenv.2021.152606
Hassan, M. A., Abol-Fotouh, D., Omer, A. M., Tamer, T. M., and Abbas, E. (2020a). Comprehensive insights into microbial keratinases and their implication in various biotechnological and industrial sectors: A review. Int. J. Biol. Macromol. 154, 567–583. doi: 10.1016/j.ijbiomac.2020.03.116
Hassan, M. A., Taha, T. H., Hamad, G. M., Hashem, M., Alamri, S., and Mostafa, Y. S. (2020b). Biochemical characterisation and application of keratinase from Bacillus thuringiensis MT1 to enable valorisation of hair wastes through biosynthesis of vitamin B-complex. Int. J. Biol. Macromol. 153, 561–572. doi: 10.1016/j.ijbiomac.2020.03.032
Hernández-Fernández, M., Cordero-Bueso, G., Ruiz-Muñoz, M., and Cantoral, J. M. (2021). Culturable yeasts as biofertilizers and biopesticides for a sustainable agriculture: a comprehensive review. Plants. 10, 822. doi: 10.3390/plants10050822
Herridge, D. F., Peoples, M. B., and Boddey, R. M. (2008). Global inputs of biological nitrogen fixation in agricultural systems. Plant Soil. 311, 1–18. doi: 10.1007/s11104-008-9668-3
Hirel, B., Tétu, T., Lea, P. J., and Dubois, F. (2011). Improving nitrogen use efficiency in crops for sustainable agriculture. Sustainability. 3, 1452–1485. doi: 10.3390/su3091452
Humbert, J.-Y., Dwyer, J. M., Andrey, A., and Arlettaz, R. (2016). Impacts of nitrogen addition on plant biodiversity in mountain grasslands depend on dose, application duration and climate: a systematic review. Glob. Chang. Biol. 22, 110–120. doi: 10.1111/gcb.12986
Islam, S. M. M., Gaihre, Y. K., Islam, M. R., Ahmed, M. N., Akter, M., Singh, U., et al. (2022). Mitigating greenhouse gas emissions from irrigated rice cultivation through improved fertilizer and water management. J. Environ. Manage. 307, 114520. doi: 10.1016/j.jenvman.2022.114520
Izydorczyk, G., Mikula, K., Skrzypczak, D., Witek-Krowiak, A., Mironiuk, M., Furman, K., et al. (2022). Valorization of poultry slaughterhouse waste for fertilizer purposes as an alternative for thermal utilization methods. J. Hazard. Mater. 424, 127328. doi: 10.1016/j.jhazmat.2021.127328
Jagadeesan, Y., Meenakshisundaram, S., Saravanan, V., and Balaiah, A. (2020). Sustainable production, biochemical and molecular characterization of thermo-and-solvent stable alkaline serine keratinase from novel Bacillus pumilus AR57 for promising poultry solid waste management. Int. J. Biol. Macromol. 163, 135–146. doi: 10.1016/j.ijbiomac.2020.06.219
Jain, R., Jain, A., Rawat, N., Nair, M., and Gumashta, R. (2016). Feather hydrolysate from Streptomyces sampsonii GS 1322: a potential low cost soil amendment. J. Biosci. Bioeng. 121, 672–677. doi: 10.1016/j.jbiosc.2015.11.003
Jeevana Lakshmi, P., Kumari Chitturi, C. M., and Lakshmi, V. V. (2013). Efficient degradation of feather by keratinase producing Bacillus sp. Int. J. Microbiol. 2013, 7. doi: 10.1155/2013/608321
Jiménez-Mejía, R., Medina-Estrada, R. I., Carballar-Hernández, S., Orozco-Mosqueda, M. d. C, Santoyo, G., et al. (2022). Teamwork to survive in hostile soils: use of plant growth-promoting bacteria to ameliorate soil salinity stress in crops. Microorganisms. 10, 150. doi: 10.3390/microorganisms10010150
Joardar, J. C., and Rahman, M. M. (2018). Poultry feather waste management and effects on plant growth. Int. J. Recycl. Org. Waste Agric. 7, 183–188. doi: 10.1007/s40093-018-0204-z
Kang, D., Herschend, J., Al-Soud, W. A., Mortensen, M. S., Gonzalo, M., Jacquiod, S., et al. (2018). Enrichment and characterization of an environmental microbial consortium displaying efficient keratinolytic activity. Bioresour. Technol. 270, 303–310. doi: 10.1016/j.biortech.2018.09.006
Kanoksilapatham, W., and Intagun, W. (2017). A review: biodegradation and applications of keratin degrading microorganisms and keratinolytic enzymes, focusing on thermophiles and thermostable serine proteases. Am. J. Appl. Sci. 14, 1016–1023. doi: 10.3844/ajassp.2017.1016.1023
Kasperova, A., Kunert, J., and Raska, M. (2013). The possible role of dermatophyte cysteine dioxygenase in keratin degradation. Medical Mycol. 51, 449–454. doi: 10.3109/13693786.2013.794310
Kaur, M., Bhari, R., and Singh, R. S. (2021). Chicken feather waste-derived protein hydrolysate as a potential biostimulant for cultivation of mung beans. Biologia. 76, 1807–1815. doi: 10.1007/s11756-021-00724-x
Khodayari, S., and Kafilzadeh, F. (2018). Separating keratinase producer bacteria from the soil of poultry farms and optimization of the conditions for maximum enzyme production. The Eur. J. Exp. Biol. 8, 35. doi: 10.21767/2248-9215.100076
Kim, J. M., Lim, W. J., and Suh, H. J. (2001). Feather-degrading Bacillus species from poultry waste. Proc. Biochem. 37, 287–291. doi: 10.1016/S0032-9592(01)00206-0
Kim, J. M. K. U., Choi, Y. M. S. C., and Suh, H. J. K. U. (2005). Preparation of feather digests as fertilizer with Bacillus pumilis KHS-1. J. Microbiol. Biotechnol. 15, 427–476.
Korniłłowicz-Kowalska, T., and Bohacz, J. (2011). Biodegradation of keratin waste: theory and practical aspects. Waste Manag. 31, 1689–1701. doi: 10.1016/j.wasman.2011.03.024
Kowalczyk, P., Mahdi-Oraibi, S., Misiewicz, A., Gabzdyl, N., Miskiewicz, A., and Szparecki, G. (2018). Feather-degrading bacteria: their biochemical and genetic characteristics. Arab J Sci Eng. 43, 33–41. doi: 10.1007/s13369-017-2700-2
Kumar, A., Jha, M. N., Singh, D., Pathak, D., and Rajawat, M. V. S. (2021). Prospecting catabolic diversity of microbial strains for developing microbial consortia and their synergistic effect on Lentil (Lens esculenta) growth, yield and iron biofortification. Arch. Microbiol. 203, 4913–4928. doi: 10.1007/s00203-021-02446-9
Kumar, A., Verma, L. M., Sharma, S., and Singh, N. (2022a). Overview on agricultural potentials of biogas slurry (BGS): applications, challenges, and solutions. Biomass Conversion and Biorefinery. doi: 10.1007/s13399-021-02215-0
Kumar, J., Sharma, A., Kumar, P., and Kushwaha, R. (2017). Enhancement of soil nutrition using fermented feather and their efficacy on seed germination. Int. J. Pure App. Biosci 5, 92–98. doi: 10.18782/2320-7051.2429
Kumar, S., Diksha, S. S. S., and Kumar, R. (2022b). Biofertilizers: An ecofriendly technology for nutrient recycling and environmental sustainability. CRMICR. 3, 100094. doi: 10.1016/j.crmicr.2021.100094
Kumari, M., and Kumar, J. (2020). Chicken feather waste degradation by Alternaria tenuissima and its application on plant growth. J. Appl. Nat. Sci. 12, 411–414. doi: 10.31018/jans.v12i3.2345
Lange, L., Huang, Y., and Busk, P. K. (2016). Microbial decomposition of keratin in nature-a new hypothesis of industrial relevance. Appl. Microbiol. Biotechnol. 100, 2083–2096. doi: 10.1007/s00253-015-7262-1
Latshaw, J. D., Musharaf, N., and Retrum, R. (1994). Processing of feather meal to maximize its nutritional value for poultry. Anim. Feed Sci. Technol. 47, 179–188. doi: 10.1016/0377-8401(94)90122-8
Lee, S.-K., Lur, H.-S., and Liu, C.-T. (2021). From lab to farm: elucidating the beneficial roles of photosynthetic bacteria in sustainable agriculture. Microorganisms. 9, 2453. doi: 10.3390/microorganisms9122453
Li, Q. (2019). Progress in microbial degradation of feather waste. Front. Microbiol. 10, 2717. doi: 10.3389/fmicb.2019.02717
Li, Q. (2021). Structure, Application, and Biochemistry of Microbial Keratinases. Front. Microbiol. 12. doi: 10.3389/fmicb.2021.674345
Lisowska, A., Filipek-Mazur, B., Sołtys, J., Niemiec, M., Gorczyca, O., Bar-Michalczyk, D., et al. (2022). Preparation, characterization of granulated sulfur fertilizers and their effects on a sandy soils. Materials. 15, 612. doi: 10.3390/ma15020612
liu, B., Zhang, J., Fang, Z., Gu, L., Liao, X., Du, G., et al. (2013a). Enhanced thermostability of keratinase by computational design and empirical mutation. J. Ind. Microbiol. Biotechnol. 40, 697–704. doi: 10.1007/s10295-013-1268-4
liu, B., Zhang, J., Li, B., Liao, X., Du, G., and Chen, J. (2013b). Expression and characterization of extreme alkaline, oxidation-resistant keratinase from Bacillus licheniformis in recombinant Bacillus subtilis WB600 expression system and its application in wool fiber processing. World J. Microbiol. Biotechnol. 29, 825–832. doi: 10.1007/s11274-012-1237-5
Liu, Y., Lv, Z., Hou, H., Lan, X., Ji, J., and Liu, X. (2021). Long-term effects of combination of organic and inorganic fertilizer on soil properties and microorganisms in a Quaternary Red Clay. PLoS ONE. 16, e0261387. doi: 10.1371/journal.pone.0261387
Lucy, M., Reed, E., and Glick, B. R. (2004). Applications of free living plant growth-promoting rhizobacteria. Antonie Van Leeuwenhoek. 86, 1–25. doi: 10.1023/B:ANTO.0000024903.10757.6e
Maeda, I. (2022). Potential of phototrophic purple nonsulfur bacteria to fix nitrogen in rice fields. Microorganisms. 10, 28. doi: 10.3390/microorganisms10010028
Maitra, S., Brestic, M., Bhadra, P., Shankar, T., Praharaj, S., Palai, J. B., et al. (2022). Bioinoculants—natural biological resources for sustainable plant production. Microorganisms. 10, 51. doi: 10.3390/microorganisms10010051
Martins-Gomes, C., Silva, T. L., Andreani, T., and Silva, A. M. (2022). Glyphosate vs. glyphosate-based herbicides exposure: a review on their toxicity. J. Xenobiot. 12, 21–40. doi: 10.3390/jox12010003
McKittrick, J., Chen, P. Y., Bodde, S. G., Yang, W., Novitskaya, E. E., and Meyers, M. A. (2012). The structure, functions, and mechanical properties of keratin. JOM. 64, 449–468. doi: 10.1007/s11837-012-0302-8
Mendoza-Suárez, M., Andersen, S. U., Poole, P. S., and Sánchez-Cañizares, C. (2021). Competition, Nodule Occupancy, and Persistence of Inoculant Strains: Key Factors in the Rhizobium-Legume Symbioses. Front. Plant Sci. 12. doi: 10.3389/fpls.2021.690567
Mercer, D. K., and Stewart, C. S. (2018). Keratin hydrolysis by dermatophytes. Medical Mycol. 57, 13–22. doi: 10.1093/mmy/myx160
Mikhael, J. E. R., Wang, J. J., Dodla, S., Scaglia, G., and Dattamudi, S. (2022). Effects of biochar and N-stabilizers on greenhouse gas emissions from a subtropical pasture field applied with organic and inorganic nitrogen fertilizers. J. Environ. Manage. 306, 114423. doi: 10.1016/j.jenvman.2021.114423
Mitter, B., Brader, G., Pfaffenbichler, N., and Sessitsch, A. (2019). Next generation microbiome applications for crop production — limitations and the need of knowledge-based solutions. Curr. Opin. Microbiol. 49, 59–65. doi: 10.1016/j.mib.2019.10.006
Moe, L. A. (2013). Amino acids in the rhizosphere: From plants to microbes. Am. J. Bot. 100, 1692–1705. doi: 10.3732/ajb.1300033
Muhammed, N. S., Hussin, N., Lim, A. S., Jonet, M. A., Mohamad, S. E., and Jamaluddin, H. (2021). Recombinant production and characterization of an extracellular subtilisin-like serine protease from acinetobacter baumannii of fermented food origin. Protein J. doi: 10.1007/s10930-021-09986-5
Navone, L., and Speight, R. (2018). Understanding the dynamics of keratin weakening and hydrolysis by proteases. PLoS ONE. 13, e0202608. doi: 10.1371/journal.pone.0202608
Nazir, S., Zaman, Q. u, Abbasi, A., Komal, N., Riaz, U., et al. (2021). Bioresource nutrient recycling in the rice–wheat cropping system: cornerstone of organic agriculture. Plants. 10, 2323. doi: 10.3390/plants10112323
Ngo, H. T. T., Watts-Williams, S. J., Panagaris, A., Baird, R., McLaughlin, M. J., and Cavagnaro, T. R. (2021). Development of an organomineral fertiliser formulation that improves tomato growth and sustains arbuscular mycorrhizal colonisation. Sci. Total Environ. 151977. doi: 10.1016/j.scitotenv.2021.151977
Nnolim, N. E., Mpaka, L., Okoh, A. I., and Nwodo, U. U. (2020a). Biochemical and molecular characterization of a thermostable alkaline metallo-keratinase from Bacillus sp. Nnolim-K1. Microorganisms. 8, 1304. doi: 10.3390/microorganisms8091304
Nnolim, N. E., and Nwodo, U. U. (2021). Microbial keratinase and the bio-economy: a three-decade meta-analysis of research exploit. AMB Express. 11, 12. doi: 10.1186/s13568-020-01155-8
Nnolim, N. E., Udenigwe, C. C., Okoh, A. I., and Nwodo, U. U. (2020b). Microbial keratinase: next generation green catalyst and prospective applications. Front. Microbiol. 11, 580164. doi: 10.3389/fmicb.2020.580164
Nosheen, A., Yasmin, H., Naz, R., Keyani, R., Mumtaz, S., Hussain, S. B., et al. (2022). Phosphate solubilizing bacteria enhanced growth, oil yield, antioxidant properties and biodiesel quality of Kasumbha. Saudi J. Biol. Sci. 29, 43–52. doi: 10.1016/j.sjbs.2021.09.068
Onifade, A. A., Al-Sane, N. A., Al-Musallam, A. A., and Al-Zarban, S. (1998). A review: Potentials for biotechnological applications of keratin-degrading microorganisms and their enzymes for nutritional improvement of feathers and other keratins as livestock feed resources. Bioresour. Technol. 66, 1–11. doi: 10.1016/S0960-8524(98)00033-9
Orr, C. H., James, A., Leifert, C., Cooper, J. M., and Cummings, S. P. (2011). Diversity and activity of free-living nitrogen-fixing bacteria and total bacteria in organic and conventionally managed soils. Appl. Environ. Microbiol. 77, 911–919. doi: 10.1128/AEM.01250-10
Ortiz, A., and Sansinenea, E. (2021). Recent advancements for microorganisms and their natural compounds useful in agriculture. Appl. Microbiol. Biotechnol. 105, 891–897. doi: 10.1007/s00253-020-11030-y
Pahua-Ramos, M. E., Hernández-Melchor, D. J., Camacho-Pérez, B., and Quezada-Cruz, M. (2017). Degradation of chicken feathers: a review. Biotechnol. Ind. J. 13, 658–666. https://www.tsijournals.com/articles/degradation-of-chicken-feathers-a-review-13509.html
Papadopoulos, M. C. (1989). Effect of processing on high-protein feedstuffs: a review. Biological Wastes. 29, 123–138. doi: 10.1016/0269-7483(89)90092-X
Papadopoulos, M. C., El Boushy, A. R., Roodbeen, A. E., and Ketelaars, E. H. (1986). Effects of processing time and moisture content on amino acid composition and nitrogen characteristics of feather meal. Anim. Feed Sci. Technol. 14, 279–290. doi: 10.1016/0377-8401(86)90100-8
Parinayawanich, S., Sittipol, D., Ajingi, Y. S., Rodpan, S., Pattanapanyasat, K., and Jonguraj, N. (2021). Application of recombinant hyperthermostable keratinase for degradation of chicken feather waste. Biocatal. Agric. Biotechnol. 36, 102146. doi: 10.1016/j.bcab.2021.102146
Patinvoh, R. J., Lundin, M., Taherzadeh, M. J., and Sárvári Horváth, I. (2020). Dry Anaerobic Co-Digestion of Citrus Wastes with Keratin and Lignocellulosic Wastes: Batch And Continuous Processes. Waste Biomass Valorization. 11, 423–434. doi: 10.1007/s12649-018-0447-y
Paul, T., Halder, S. K., Das, A., Bera, S., Maity, C., Mandal, A., et al. (2013). Exploitation of chicken feather waste as a plant growth promoting agent using keratinase producing novel isolate Paenibacillus woosongensis TKB2. Biocatal. Agric. Biotechnol. 2, 50–57. doi: 10.1016/j.bcab.2012.10.001
Peng, Z., Zhang, J., Song, Y., Guo, R., Du, G., and Chen, J. (2021). Engineered pro-peptide enhances the catalytic activity of keratinase to improve the conversion ability of feather waste. Biotechnol. Bioeng. doi: 10.1002/bit.27771
Pettett, L. M., Ipek, K., and ouml ke, D. (2004). Development of an Environmentally Friendly Biofertilizer with Keratin Degrading and Antibiotic Producing Actinomycetes. Actinomycetologica 18, 34–42. doi: 10.3209/saj.18_34
Petzet, S., and Cornel, P. (2011). Towards a complete recycling of phosphorus in wastewater treatment – options in Germany. Water Sci. Technol. 64, 29–35. doi: 10.2166/wst.2011.540
Pinski, A., Zur, J., Hasterok, R., and Hupert-Kocurek, K. (2020). Comparative Genomics of Stenotrophomonas maltophilia and Stenotrophomonas rhizophila Revealed Characteristic Features of Both Species. Int. J. Mol. Sci. 21, 4922. doi: 10.3390/ijms21144922
Pirttilä, A. M., Mohammad Parast Tabas, H., Baruah, N., and Koskimäki, J. J. (2021). Biofertilizers and biocontrol agents for agriculture: how to identify and develop new potent microbial strains and traits. Microorganisms 9, 817. doi: 10.3390/microorganisms9040817
Qiu, J., Wilkens, C., Barrett, K., and Meyer, A. S. (2020). Microbial enzymes catalyzing keratin degradation: classification, structure, function. Biotechnol. Adv. 44, 107607. doi: 10.1016/j.biotechadv.2020.107607
Qu, F., Chen, Q., Ding, Y., Liu, Z., Zhao, Y., Zhang, X., et al. (2018). Isolation of a feather-degrading strain of bacterium from spider gut and the purification and identification of its three key enzymes. Mol. Biol. Rep. 45, 1681–1689. doi: 10.1007/s11033-018-4311-8
Raguraj, S., Kasim, S., Md Jaafar, N., and Nazli, M. H. (2022). Growth of tea nursery plants as influenced by different rates of protein hydrolysate derived from chicken feathers. Agronomy 12, 299. doi: 10.3390/agronomy12020299.
Rai, S. K., Konwarh, R., and Mukherjee, A. K. (2009). Purification, characterization and biotechnological application of an alkaline β-keratinase produced by Bacillus subtilis RM-01 in solid-state fermentation using chicken-feather as substrate. Biochem. Eng. J. 45, 218–225. doi: 10.1016/j.bej.2009.04.001
Rai, S. K., and Mukherjee, A. K. (2011). Optimization of production of an oxidant and detergent-stable alkaline β-keratinase from Brevibacillus sp. strain AS-S10-II: Application of enzyme in laundry detergent formulations and in leather industry. Biochem. Eng. J. 54, 47–56. doi: 10.1016/j.bej.2011.01.007
Rajput, R., and Gupta, R. (2013). Thermostable keratinase from Bacillus pumilus KS12: Production, chitin crosslinking and degradation of Sup35NM aggregates. Bioresour. Technol. 133, 118–126. doi: 10.1016/j.biortech.2013.01.091
Rajput, V. D., Singh, A., Minkina, T., Rawat, S., Mandzhieva, S., Sushkova, S., et al. (2021). Nano-enabled products: challenges and opportunities for sustainable agriculture. Plants. 10, 2727. doi: 10.3390/plants10122727
Raliya, R., Saharan, V., Dimkpa, C., and Biswas, P. (2018). Nanofertilizer for Precision and Sustainable Agriculture: Current State and Future Perspectives. J. Agric. Food Chem. 66, 6487–6503. doi: 10.1021/acs.jafc.7b02178
Ramnani, P., Singh, R., and Gupta, R. (2005). Keratinolytic potential of Bacillus licheniformis RG1: structural and biochemical mechanism of feather degradation. Can. J. Microbiol. 51, 191–196. doi: 10.1139/w04-123
Ranjha, M. M. A. N., Shafique, B., Khalid, W., Nadeem, H. R., Mueen-ud-Din, G., and Khalid, M. Z. (2022). Applications of biotechnology in food and agriculture: a mini-review. Proc. Natl. Acad. Sci. India Sect. B Biol Sci. 92, 11–15. doi: 10.1007/s40011-021-01320-4
Rios-Galicia, B., Villagómez-Garfias, C., De la Vega-Camarillo, E., Guerra-Camacho, J. E., Medina-Jaritz, N., Arteaga-Garibay, R. I., et al. (2021). The Mexican giant maize of Jala landrace harbour plant-growth-promoting rhizospheric and endophytic bacteria. Biotech. 11, 447. doi: 10.1007/s13205-021-02983-6
Sabir, M. S., Shahzadi, F., Ali, F., Shakeela, Q., Niaz, Z., and Ahmed, S. (2021). Comparative effect of fertilization practices on soil microbial diversity and activity: an overview. Curr. Microbiol. 78, 3644–3655. doi: 10.1007/s00284-021-02634-2
Sahni, N., Sahota, P. P., and Gupta, P. U. (2015). Bacterial keratinases and their prospective applications: a review. Int. J. Curr. Microbiol. 4, 768–783.
Saleem, M., Hu, J., and Jousset, A. (2019). More than the sum of its parts: microbiome biodiversity as a driver of plant growth and soil health. Annu. Rev. Ecol. Evol. Syst. 50, 145–168. doi: 10.1146/annurev-ecolsys-110617-062605
Santoyo, G., Orozco-Mosqueda, M. d. C, and Govindappa, M. (2012). Mechanisms of biocontrol and plant growth-promoting activity in soil bacterial species of Bacillus and Pseudomonas: a review. Biocontrol Sci. Technol. 22, 855–872. doi: 10.1080/09583157.2012.694413
Saravanan, K., and Dhurai, B. (2012). Exploration on the amino acid content and morphological structure in chicken feather fiber. J. Text. Appar. Technol. Manag. 7. https://ojs.cnr.ncsu.edu/index.php/JTATM/article/viewFile/1829/1406#:~:text=An%20attempt%20has%20been%20made,feather%20partially%20hygroscopic%20in%20nature
Seenivasagan, R., and Babalola, O. O. (2021). Utilization of microbial consortia as biofertilizers and biopesticides for the production of feasible agricultural product. Biology. 10, 1111. doi: 10.3390/biology10111111
Shanmugasundaram, O. L., Syed Zameer Ahmed, K., Sujatha, K., Ponnmurugan, P., Srivastava, A., Ramesh, R., et al. (2018). Fabrication and characterization of chicken feather keratin/polysaccharides blended polymer coated nonwoven dressing materials for wound healing applications. Mater. Sci. Eng. C 92, 26–33. doi: 10.1016/j.msec.2018.06.020
Sharma, R., and Devi, S. (2018). Versatility and commercial status of microbial keratinases: a review. Rev. Environ. Sci. Biotechnol. 17, 19–45. doi: 10.1007/s11157-017-9454-x
Sharma, S., and Gupta, A. (2016). Sustainable management of keratin waste biomass: applications and future perspectives. Braz. Arch. Biol. Technol. 59. doi: 10.1590/1678-4324-2016150684
Shestakova, A., Timorshina, S., and Osmolovskiy, A. (2021). Biodegradation of keratin-rich husbandry waste as a path to sustainable agriculture. Sustainability. 13, 8691. doi: 10.3390/su13168691
Singh, H., Sharma, A., Bhardwaj, S. K., Arya, S. K., Bhardwaj, N., and Khatri, M. (2021). Recent advances in the applications of nano-agrochemicals for sustainable agricultural development. Environm. Sci. 23, 213–239. doi: 10.1039/D0EM00404A
Sivakumar, T., Shankar, T., Pandian, V., and Subramanian, R. (2012). Plant growth promoting activity of nickel tolerant Bacillus cereus TS1. J. Agri. Technol. 8, 2099–2111.
Snider, N. T., and Omary, M. B. (2014). Post-translational modifications of intermediate filament proteins: mechanisms and functions. Nat. Rev. Molecular Cell Biol. 15, 163. doi: 10.1038/nrm3753
Sobucki, L., Ramos, R. F., Gubiani, E., Brunetto, G., Kaiser, D. R., and Daroit, D. J. (2019). Feather hydrolysate as a promising nitrogen-rich fertilizer for greenhouse lettuce cultivation. Int. J. Recycl. Org. Waste Agric. 8, 493–499. doi: 10.1007/s40093-019-0281-7
Syed, D. G., Lee, J. C., Li, W.-J., Kim, C.-J., and Agasar, D. (2009). Production, characterization and application of keratinase from Streptomyces gulbargensis. Bioresour. Technol. 100, 1868–1871. doi: 10.1016/j.biortech.2008.09.047
Sypka, M., Jodłowska, I., and Białkowska, A. M. (2021). Keratinases as versatile enzymatic tools for sustainable development. Biomolecules 11, 1900. doi: 10.3390/biom11121900
Tamreihao, K., Devi, L. J., Khunjamayum, R., Mukherjee, S., Ashem, R. S., and Ningthoujam, D. S. (2017). Biofertilizing potential of feather hydrolysate produced by indigenous keratinolytic Amycolatopsis sp. MBRL 40 for rice cultivation under field conditions. Biocatal. Agric. Biotechnol. 10, 317–320. doi: 10.1016/j.bcab.2017.04.010
Tamreihao, K., Mukherjee, S., Khunjamayum, R., Devi, L. J., Asem, R. S., and Ningthoujam, D. S. (2019). Feather degradation by keratinolytic bacteria and biofertilizing potential for sustainable agricultural production. J. Basic Microbiol. 59, 4–13. doi: 10.1002/jobm.201800434
Tan, Y., Wang, J., He, Y., Yu, X., Chen, S., Penttinen, P., et al. (2022). Organic fertilizers shape soil microbial communities and increase soil amino acid metabolites content in a blueberry orchard. Microb. Ecol. doi: 10.1007/s00248-022-01960-7
Tatineni, R., Doddapaneni, K. K., Potumarthi, R. C., and Mangamoori, L. N. (2007). Optimization of keratinase production and enzyme activity using response surface methodology with streptomyces sp7. Appl. Biochem. Biotechnol. 141, 187. doi: 10.1007/BF02729061
Tesfaye, T., Sithole, B., and Ramjugernath, D. (2017). Valorisation of chicken feathers: a review on recycling and recovery route—current status and future prospects. Clean Technol. Environm. Policy. 19, 2363–2378. doi: 10.1007/s10098-017-1443-9
Tiwary, E., and Gupta, R. (2010a). Extracellular expression of keratinase from bacillus licheniformis ER-15 in Escherichia coli. J. Agric. Food Chem. 58, 8380–8385. doi: 10.1021/jf100803g
Tiwary, E., and Gupta, R. (2010b). Medium optimization for a novel 58kDa dimeric keratinase from Bacillus licheniformis ER-15: Biochemical characterization and application in feather degradation and dehairing of hides. Bioresour. Technol. 101, 6103–6110. doi: 10.1016/j.biortech.2010.02.090
Urmi, T. A., Rahman, M. M., Islam, M. M., Islam, M. A., Jahan, N. A., Mia, M. A. B., et al. (2022). Integrated nutrient management for rice yield, soil fertility, and carbon sequestration. Plants. 11, 138. doi: 10.3390/plants11010138
van der Heijden, M. G., Bardgett, R. D., and van Straalen, N. M. (2008). The unseen majority: soil microbes as drivers of plant diversity and productivity in terrestrial ecosystems. Ecol. Lett. 11, 296–310. doi: 10.1111/j.1461-0248.2007.01139.x
Vejan, P., Khadiran, T., Abdullah, R., and Ahmad, N. (2021). Controlled release fertilizer: A review on developments, applications and potential in agriculture. J. Controlled Release. 339, 321–334. doi: 10.1016/j.jconrel.2021.10.003
Verma, A., Singh, H., Anwar, S., Chattopadhyay, A., Tiwari, K. K., Kaur, S., et al. (2017). Microbial keratinases: industrial enzymes with waste management potential. Crit. Rev. Biotechnol. 37, 476–491. doi: 10.1080/07388551.2016.1185388
Vidmar, B., and Vodovnik, M. (2018). Microbial keratinases: enzymes with promising biotechnological applications. Food Technol. Biotechnol. 56, 312–328. doi: 10.17113/ftb.56.03.18.5658
Wang, B., Yang, W., McKittrick, J., and Meyers, M. A. (2016). Keratin: Structure, mechanical properties, occurrence in biological organisms, and efforts at bioinspiration. Prog. Mater. Sci. 76, 229–318. doi: 10.1016/j.pmatsci.2015.06.001
Wang, J.-J., Rojanatavorn, K., and Shih, J. C. H. (2004). Increased production of Bacillus keratinase by chromosomal integration of multiple copies of the kerA gene. Biotechnol. Bioeng. 87, 459–464. doi: 10.1002/bit.20145
Wang, X., and Parsons, C. (1997). Effect of processing systems on protein quality of feather meals and hog hair meals. Poult. Sci. 76, 491–496. doi: 10.1093/ps/76.3.491
Wang, Y., Peng, S., Hua, Q., Qiu, C., Wu, P., Liu, X., et al. (2021). The long-term effects of using phosphate-solubilizing bacteria and photosynthetic bacteria as biofertilizers on peanut yield and soil bacteria community. Front. Microbiol. 12. doi: 10.3389/fmicb.2021.693535
Williams, C. M., and Shih, J. C. H. (1989). Enumeration of some microbial groups in thermophilic poultry waste digesters and enrichment of a feather-degrading culture. J. Appl. Bacteriol. 67, 25–35. doi: 10.1111/j.1365-2672.1989.tb04951.x
Yahaya, R. S. R., Normi, Y. M., Phang, L. Y., Ahmad, S. A., Abdullah, J. O., and Sabri, S. (2021). Molecular strategies to increase keratinase production in heterologous expression systems for industrial applications. Appl. Microbiol. Biotechnol. doi: 10.1007/s00253-021-11321-y
Yang, L.-Y., Zhou, S.-Y.-D., Lin, C.-S., Huang, X.-R., Neilson, R., and Yang, X.-R. (2022). Effects of biofertilizer on soil microbial diversity and antibiotic resistance genes. Sci. Total Environ. 820, 153170. doi: 10.1016/j.scitotenv.2022.153170
Yong, B., Fei, X., Shao, H., Xu, P., Hu, Y., Ni, W., et al. (2020). Recombinant expression and biochemical characterization of a novel keratinase BsKER71 from feather degrading bacterium Bacillus subtilis S1-4. AMB Express 10, 9. doi: 10.1186/s13568-019-0939-6
Young, I. M., and Ritz, K. (2000). Tillage, habitat space and function of soil microbes. Soil and Tillage Research. 53, 201–213. doi: 10.1016/S0167-1987(99)00106-3
Zahra, T., Hamedi, J., and Mahdigholi, K. (2020). Endophytic actinobacteria of a halophytic desert plant Pteropyrum olivieri: promising growth enhancers of sunflower. Biotech. 10, 514. doi: 10.1007/s13205-020-02507-8
Zhang, R.-X., Gong, J.-S., Su, C., Qin, J., Li, H., Li, H., et al. (2020). Recombinant expression and molecular engineering of the keratinase from Brevibacillus parabrevis for dehairing performance. J. Biotechnol. 320, 57–65. doi: 10.1016/j.jbiotec.2020.06.016
Zhuang, M., Lam, S. K., Zhang, J., Li, H., Shan, N., Yuan, Y., et al. (2019). Effect of full substituting compound fertilizer with different organic manure on reactive nitrogen losses and crop productivity in intensive vegetable production system of China. J. Environ. Manage. 243, 381–384. doi: 10.1016/j.jenvman.2019.05.026
Zilio, M., Pigoli, A., Rizzi, B., Herrera, A., Tambone, F., Geromel, G., et al. (2022). Using highly stabilized digestate and digestate-derived ammonium sulphate to replace synthetic fertilizers: The effects on soil, environment, and crop production. Sci. Total Environ. 815, 152919. doi: 10.1016/j.scitotenv.2022.152919
Keywords: keratin, feather degradation, fertilizers, protein, value-added chemicals, keratinase
Citation: Li Q (2022) Perspectives on Converting Keratin-Containing Wastes Into Biofertilizers for Sustainable Agriculture. Front. Microbiol. 13:918262. doi: 10.3389/fmicb.2022.918262
Received: 12 April 2022; Accepted: 23 May 2022;
Published: 20 June 2022.
Edited by:
Ranjit Gurav, Konkuk University, South KoreaReviewed by:
Niloofar Eslahi, Islamic Azad University, IranSandeep Kumar Singh, Centre of Bio-Medical Research (CBMR), India
Copyright © 2022 Li. This is an open-access article distributed under the terms of the Creative Commons Attribution License (CC BY). The use, distribution or reproduction in other forums is permitted, provided the original author(s) and the copyright owner(s) are credited and that the original publication in this journal is cited, in accordance with accepted academic practice. No use, distribution or reproduction is permitted which does not comply with these terms.
*Correspondence: Qingxin Li, cWluZ3hpbl9saUBvdXRsb29rLmNvbQ==