- 1School of Pharmacy, Lanzhou University, Lanzhou, China
- 2Collaborative Innovation Center for Northwestern Chinese Medicine, Lanzhou University, Lanzhou, China
- 3School of Chemistry and Chemical Engineering, Lanzhou University, Lanzhou, China
Peganum harmala L. is a perennial herb of the Tribulus family and its aerial parts and seeds can be used as medicine in the traditional medicine of China. However, the differences in chemical components and antibacterial activity between different parts have not been reported. In this study, the chemical composition of the different parts of P. harmala was characterized by high-performance liquid chromatography (HPLC) and headspace-solid phase microextraction-gas chromatography-mass spectrometry (HS-SPME-GC-MS). The antimicrobial activities of the different parts and some isolated components were also carried out on 12 bacterial strains and phytopathogenic fungi. The HPLC results revealed that the contents of harmine and harmaline in the seeds were higher than that in the aerial parts. A total of 94 volatile organic compounds (VOCs) were tentatively identified by HS-SPME-GC-MS for the first time. The major components were methyl hexadecanoate, p-xylene, octane, (Z)-9-octadecanoate, ethylbenzene, methyl octadecanoate, ethyl hexadecanoate, and methyl tetradecanoate. At the concentration of 800 μg·mL−1, the methanol extracts of seeds showed stronger antimicrobial activities with a wide antimicrobial spectrum, inhibiting Escherichia coli (ATCC 24433), Xanthomonas oryzae (ACCC 11602), and Xanthomonas axonopodis with inhibitory rates of more than 90%. Furthermore, harmine and harmaline showed better antibacterial activities against all the bacteria. These findings indicated that alkaloids from P. harmala could account for antimicrobial activity, which could be used as lead molecules in the development of new antimicrobial drugs.
Introduction
Peganum harmala L. is widely distributed in the arid areas of Northwest China, and the aerial parts and seeds of P. harmala can be used as medicine in the traditional medicine of Uygur, Kazak, Mongolian, and Tibetan, which possesses neuroprotective, anticancer, antiviral, larvicidal, hepatoprotective activity, antioxidant and anti-inflammatory, antitussive, expectorant, and bronchodilating effects (Li et al., 2017). It has been reported that the main compounds of P. harmala were alkaloids, such as β-carboline and quinazolone alkaloids and their derivatives (Jalali et al., 2021). However, the differences in chemical components between different parts have not been reported. High-performance liquid chromatography (HPLC) has the characteristics of high sensitivity, high separation efficiency, easy operation, and good repeatability (Sahu et al., 2018; Martinez-Navarro et al., 2021). Gas chromatography-mass spectrometry (GC-MS) combined with solid-phase microextraction (SPME) provides a more environmentally friendly, simple, and accurate method for the study of volatile components, which are widely used in the detection of volatile organic compounds (VOCs) in plants (Feng et al., 2022). The crushed leaves and stems of P. harmala have a disagreeable odor (Xu et al., 2008). However, the VOCs of P. harmala has not been reported using HS-SPME-GC-MS, and its volatile components in the aerial parts are still poorly understood. To explore the chemical constituents of P. harmala L., its aerial parts and seeds were analyzed by HPLC and HS-SPME-GC-MS.
Natural products derived from plants have significant efficacy with antibacterial activity, particularly against pathogens that could be used to overcome drug resistance (Ye et al., 2020). For example, it has been demonstrated that P. harmala has antimicrobial activities, effectively inhibiting the growth of Staphylococcus aureus (DSM 25693), Bacillus cereus (DSM 4313), Bacillus cereus (DSM4384), Escherichia coli (DMS 857), and Pseudomonas aeruginosa (ATCC 50071) (Azzazy et al., 2021). But P. harmala has not been reported for potential antifungal activities against phytopathogenic fungi.
In the present study, to clarify the chemicals of different parts of P. harmala, the HPLC method was established for the simultaneous determination of harmine, harmaline, vasicine, and vasicinone in its aerial parts and seeds. HS-SPME-GC-MS technology was also used to determine the differences of VOCs in different parts of P. harmala. Furthermore, extracts from different parts of P. harmala were assayed against 12 bacterial strains and phytopathogenic fungi. To our knowledge, this is the first time to report the HPLC, HS-SPME-GC-MS, and antimicrobial activity results of different parts of P. harmala.
Materials and Methods
Materials and Reagents
Fresh aerial parts of P. harmala L. were collected and dried at room temperature in October 2020 from Pakistan and Gansu of China. Dried seeds were collected from Xinjiang of China. The plants were identified by Dr. Zhigang Yang, and the voucher specimen (No. 202010002) was stored in the School of Pharmacy at Lanzhou University.
Ethanol, methanol, ethyl acetate, dichloromethane, and chloroform were purchased from Tianjin Jiatong Chemical reagent Co., Ltd., Dimethylsulfoxide (DMSO) was obtained from Sigma-Aldrich (USA); Acetonitrile was purchased from Merck (Germany).
Extraction and Separation
The seeds of P. harmala (9.0 kg) were extracted under reflux with 70% ethanol for three times. The extracts (1.6 kg) were suspended in 5% HCl, then the acidic mixture was successively extracted with dichloromethane and the aqueous layer was alkalinized to pH 9 with NaOH solution, followed by exhaustive extraction with dichloromethane to obtain crude alkaloids (273 g). The crude alkaloids were subjected to silica gel column chromatography, eluting with CH2Cl2:MeOH (100:0-0:100), to obtain nine fractions (Fr.A-I) (Wang et al., 2017). Fr.E was separated on Al2O3 chromatography and eluted by chloroform and methanol to obtain compound 1 (1.4 g). Fr.F was separated on silica gel chromatography, eluting with CH2Cl2:MeOH, and obtained compound 2 (0.1 g) by recrystallization in MeOH. Compound 3 (1.4 g) was obtained from Fr.B via recrystallization in MeOH. Fr.B was separated on silica gel chromatography, eluting with dichloromethane and acetone, and obtained compound 4 (0.1 g). Their structures were elucidated based on nuclear magnetic resonance (NMR). The NMR experiments were performed on a Bruker AVANCE AV III-400 instrument (Bruker, Switzerland, 100 MHz for 13C and 400 MHz for 1H) with tetramethylsilane (TMS) as an internal reference.
HPLC Chromatographic Conditions
Preparation of Standard Compound Solutions
The vasicine (3.53 mg), vasicinone (1.54 mg), harmine (6.66 mg), and harmaline (5.90 mg) were dissolved in methanol and configured at different gradient concentrations.
Preparation of Samples Solutions
In total, 0.25 g powders of different parts from P. harmala were extracted by ultrasonic treatment with 25 ml methanol for 30 min at 30°C using an ultrasonic cleaner (Model KQ5200E, Kunshan ultrasonic instrument Co. LTD, China). Then, the sample was filtered by a membrane filter (0.22 μm) for HPLC analysis.
HPLC Analysis
EasySep-3030 liquid chromatography (Shanghai Tongwei Company) included an EasySep3030 UV detector, AS-2000 automatic sampler, and EasySep3030 binary pump. The column was C18 (4.6 × 250 mm, 5 μm, Shanghai Tongwei), and the mobile phase A was acetonitrile and mobile phase B was 0.6% glacial acetic acid solution containing 0.088 mol/L ammonium acetate. The HPLC elution conditions were operated as follows: 0–7 min, 10% A; 7.1–25 min, 19% A; 25.1–37 min, 27% A; 37.1–45 min, 27–40% A; and 45.1–60 min, 100–10% A. The injection volume was 10 μl and eluted at 1.0 ml/min, the column temperature was kept at 30°C, and the detection wavelength was 265 nm (Wen et al., 2012).
HS-SPME-GC-MS Conditions
Isolation and Concentration of GC-MS
In total, 1 g powder of samples was transferred immediately to a 20-ml head-space vial (Agilent, Palo Alto, CA, USA), containing NaCl saturated solution. The vials were sealed using crimp-top caps with TFE-silicone headspace septa (Agilent). At the time of SPME analysis, each vial was placed at 100°C for 5 min, then a 120-μm divinylbenzene/carboxen/polydimethylsilioxane fiber (Agilent) was exposed to the headspace of the sample for 15 min at 250°C.
GC-MS Conditions
After sampling, desorption of the VOCs from the fiber coating was carried out in the injection port of the GC-MS (Agilent; Model 8890, 5977B) at 250°C for 5 min in the splitless mode. The GC was equipped with a 30 m × 0.25 mm × 0.25 μm DB-5MS (5% phenyl-polymethylsiloxane) capillary column. Helium was used as the carrier gas at a linear velocity of 1.2 ml/min. The injector temperature was kept at 250°C and the detector at 280°C. The oven temperature was programmed from 40°C (3.5 min), increasing at 10°C/min to 100°C, at 7°C/min to 180°C, and at 25°C/min to 280°C, and held for 5 min. Mass spectra were recorded in electron impact (EI) ionization mode at 70 eV, using the full scan mode. The quadrupole mass detector, ion source, and transfer line temperatures were set at 150, 230, and 280°C, respectively. Mass spectra were scanned in the range m/z 50 to 500 amu at 1 s intervals. Identification of volatile compounds was achieved by comparing the mass spectra with the data system library (NIST) and linear retention index. The concentration of each compound in the P. harmala was calculated by the internal standard method.
Determination of Antibacterial Activity
The petroleum ether and methanol extracts were prepared as follows: 0.50 g powder of seeds and the aerial parts were refluxed with 100 ml petroleum ether and methanol three times. After filtration, concentration, and evaporation, the petroleum ether and methanol extract were dried and then obtained for antibacterial activity tests. E. coli (ATCC 25922), Staphylococcus aureus (Newman), X. oryzae (ACCC 11602), X. axonopodis, and Pectobacterium atroseptica (ACCC 19901) were provided by the Institute of Plant Protection, Gansu Academy of Agricultural Science. The strains were removed and underlined on NB solid medium and incubated at 28°C until single colonies grew. Colonies on solid medium were picked for agricultural bacterial NB liquid medium at 28°C and 180 rpm and shaken to grow. Strains in the log growth phase were diluted to about 106 CFU/ml. The samples were separately dissolved in dimethyl sulfoxide (DMSO) and then added to the liquid medium with a concentration of 1,600 μg/ml (0.5% DMSO). Before inoculating each well with 50 μl of bacterial suspension, a 2-fold series of NB dilutions of 50 μl of extracts and alkaloids were prepared in a 96-well plate (final concentrations of extracts were 800, 400, 200, 100, and 50 μg/ml; final concentrations of alkaloids were 100, 50, 25, and 12.5 μg/ml). A 100-μl suspension of the same concentration containing the same volume of DMSO was used as a control. The plates were then incubated at 28°C for 24 to 48 h and the optical density at 600 nm (OD600) was measured. The OD600 of 100 μl of NB solution containing the same concentration of DMSO or compounds was measured for correction. The inhibition rate was calculated using the following formula (Ferreira-Santos et al., 2021):
Where C is the corrected turbidity values of control and T is the corrected turbidity values of bacterial growth treated with compounds. The MIC values were defined as the concentration of the compound that inhibited bacterial growth by, at least, 90% (the MIC90).
Determination of Antifungal Activity
Seven pathogenic fungi, Candida albicans (ATCC 24433), Rhizoctonia solani, Sclerotinia sclerotiorum, Botrytis cinerea, Fusarium graminearum, Magnaporthe oryzae, and Phytophthora capsici were obtained from the Institute of Plant Protection, Gansu Academy of Agricultural Science. The fungi were cultured in potato dextrose agar medium (PDA) at 25°C for 3–6 days to obtain new mycelia for the antifungal assay. The mycelium growth inhibition method was used to evaluate the antifungal activity of P. harmala. First, the petroleum ether, methanol extracts, and alkaloids were dissolved in DMSO and then added to the PDA medium to obtain sterile media of different concentrations. Then, a 5-mm agar plug of each fungal strain was obtained from a 3-day-old PDA culture, which was inoculated in the middle of PDA plates containing samples. The same amount of DMSO (0.5%) was added to the sterile medium as a blank control. Petri dishes were incubated at 25°C, and the mycelial growth diameter was measured using the cross-bracketing method until the fungal growth of the blank control had completely covered the Petri dishes. The inhibitory rates were calculated according to the following formula (Yan et al., 2021):
Where C and T are the average colony diameters of the mycelium of the blank control and treatment, respectively.
Statistical Analysis
All test data were calculated for the means and SDs using the SPSS 22.0 software, one-way ANOVA was employed for the significant differences test, and the means were compared by Duncan's tests at P < 0.05 and P < 0.01.
Results and Discussion
NMR Data of the Isolated Alkaloids
Compound 1 was obtained as pale yellow prismatic crystallization and showed purple fluorescence at 365 nm. 1H-NMR (400 MHz DMSO-d6) δppm: 11.45 (1H, s, NH), 8.17 (1H, d, J =5.3 Hz), 8.06 (1H, d, J =8.6 Hz), 7.82 (1H, d, J =5.3 Hz), 7.03 (1H, d, J =2.0 Hz), 6.87 (1H, dd, J =8.6, 2.0 Hz), 3.88 (3H, s, OCH3), and 2.74 (3H, s, CH3); 13C-NMR (100 MHz DMSO-d6) δppm: 142.4 (C-1), 138.1 (C-3), 112.4 (C-4), 123.1 (C-5), 109.5 (C-6), 160.6 (C-7), 95.1 (C-8), 135.0 (C-10), 127.7 (C-11), 115.3 (C-12), 141.7 (C-13), 55.8 (OCH3), and 20.8 (CH3). Thus, the structure of compound 1 was determined as harmaline by comparing the NMR data with the literature (Benarous et al., 2015).
Compound 2 was obtained as yellow sheet crystallization and showed bright blue fluorescence at 365 nm. 1H-NMR (400 MHz DMSO-d6) δppm: 11.16 (1H, s, NH), 7.43 (1H, d, J = 8.7 Hz), 6.86 (1H, d, J = 1.8 Hz), 6.71 (1H, dd, J = 8.7, 2.2 Hz), 3.79 (3H, s, OCH3), 3.65 (2H, t, J = 8.8 Hz), 2.70 (3H, t, 8.4 Hz), and 2.25 (3H, s, CH3); 13C-NMR (100 MHz DMSO-d6) δppm: 157.7 (C-1), 48.0 (C-3), 19.6 (C-4), 119.9 (C-5), 110.7 (C-6), 157.3 (C-7), 95.1 (C-8), 129.0 (C-10), 120.8 (C-11), 115.2 (C-12), 138.1 (C-13), 55.6 (OCH3), and 22.4 (CH3). Therefore, the structure of compound 2 was elucidated as harmine by comparing the NMR data with the literature (Mukhtar et al., 2002).
Compound 3 was obtained as white amorphous powder. 1H-NMR (400 MHz CDCl3) δppm: 11.42 (1H, s, NH), 8.16 (1H, d, J = 5.2 Hz), 7.80 (1H, d, J = 5.2 Hz), 8.05 (1H, d, J = 8.8 Hz), 6.84 (1H, dd, J = 8.8, 2.0 Hz), 7.01 (1H, d, J = 2.0 Hz), 3.87 (3H, s, –OCH3), and 2.72 (3H, s, –CH3); 13C-NMR (100 MHz CdCl3) δ ppm: 160.0 (C-1), 137.7 (C-3), 111.9 (C-4), 122.6 (C-5), 109.0 (C-6), 141.9 (C-7), 94.6(C-8), 141.2 (C-10), 127.2 (C-11), 114.8 (C-12), 134.5 (C-13), 55.3 (OCH3), and 20.3 (CH3). Therefore, the structure of compound 3 was determined as vasicine by comparing the NMR data with the literature (Wang et al., 2015).
Compound 4 was obtained as a white amorphous powder. 1H-NMR (400 MHz DMSO-d6) δppm: 7.78 (1H, dd, J = 8.2, 1.1 Hz), 7.84 (1H, m), 7.53 (1H, m), 8. 16 (1H, dd, J = 8.1, 1.5 Hz), 4.99 (1H, t, J = 4.0 Hz), 4.11 (1H, m), 3.89 (1H, m), and 1.98 (2H, m); 13C-NMR (100 MHz DMSO-d6) δppm: 159.9 (C-2), 160.6 (C-4), 134.2 (C-5), 120.6 (C-5'), 125.8 (C-6), 127.0 (C-7), 126.35 (C-8), 149.1 (C-8'), 71.3 (C-9), 29.6 (C-10), and 43.0 (C-11). Therefore, the structure of compound 4 was determined as vasicinone by comparing the NMR data with the literature (Wang et al., 2015). Their structures are shown in Figure 1.
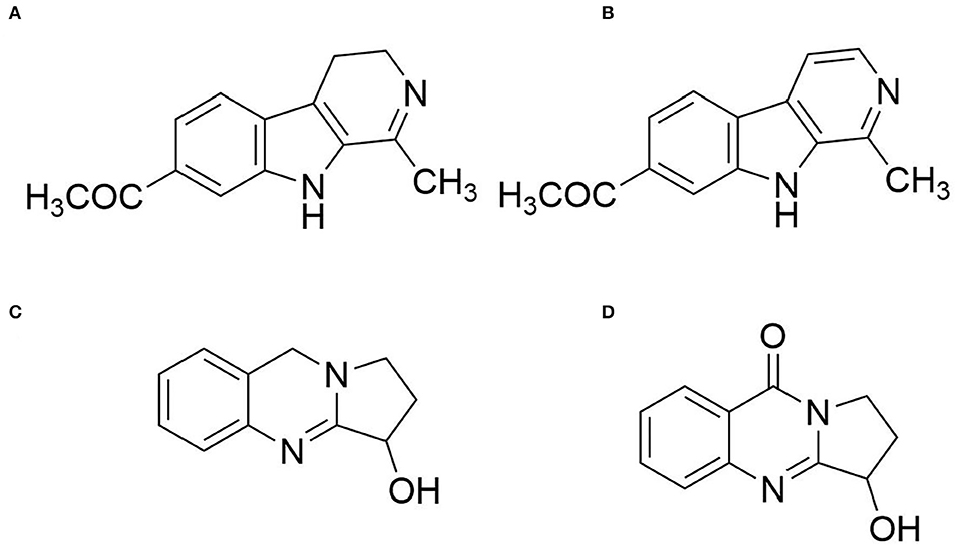
Figure 1. Structures of the major alkaloids found in the seeds of P. harmala. (A) Compound 1 (harmine); (B) Compound 2 (harmaline); (C) Compound 3 (vasicine); and (D) Compound 4 (vasicinone).
HPLC Method Validation
Precision
The sample (No. S1) was injected six times according to the chromatographic conditions mentioned in the section HPLC analysis. The RSD values of the relative retention time of vasicine, vasicinone, harmaline, and harmine were 0.26, 0.13, 0.33, and 0.35%, respectively; the RSD of relative peak area were 1.12, 1.34, 0.82, and 0.86%, respectively.
Stability
The sample (No. S1) was analyzed by the aforementioned chromatography conditions after 0, 2, 4, 6, 12, and 24 h of storage. The RSD value of the relative retention time of vasicine, vasicinone, harmaline, and harmine were 0.33, 0.16, 0.33, and 0.35%, respectively; The RSD values of the relative peak area were 1.03, 2.13, 0.90, and 1.02%, respectively.
Repeatability
The sample (No. S1) was extracted five times in parallel by the method mentioned in Section Preparation of Samples Solutions and measured by chromatography in the aforementioned Section HPLC Analysis. The RSD values of relative retention time of vasicine, vasicinone, harmaline, and harmine were 0.22, 0.09, 0.15, and 0.15%, respectively; the RSD of relative peak area were 0.15, 0.50, 0.22, and 0.30%, respectively.
Linear Relationship
According to the chromatographic conditions mentioned under Section HPLC Analysis, the different mass concentrations were the transverse coordinate (X) and the peak area as the vertical ordinate (Y), and the results are shown in Table 1.

Table 1. Linear regression equations, linear range, and correlation coefficients for the four alkaloids.
Results of the HPLC Analysis
The sample solution was prepared according to Section HPLC Chromatographic Conditions and analyzed according to the chromatographic conditions of Section HPLC Chromatographic Conditions. The chromatogram of the standard compounds is shown in Figure 2 and the HPLC chromatogram of batch 10 samples is shown in Figure 3. The contents of each index component were calculated, and the results are shown in Figure 4. The contents of vasicine, vasicinone, harmaline, and harmine in seeds were 21.36–35.27 μg·g−1, 5.18–13.66 μg·g−1, 88.05–472.23 μg·g−1, and 136.41–650.12 μg·g−1, respectively. The contents of vasicine and vasicinone in the aerial parts were 12.99–60.46 μg·g−1 and 2.15–8.18 μg·g−1, respectively, and the harmaline and harmine were not detected in the aerial parts.
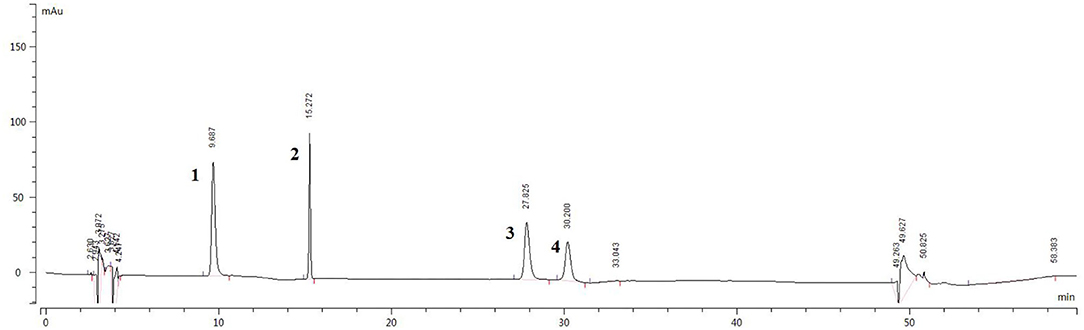
Figure 2. HPLC chromatogram of the standard compounds. 1. Vasicine, 2. Vasicinone, 3. Harmaline, and 4. Harmine.
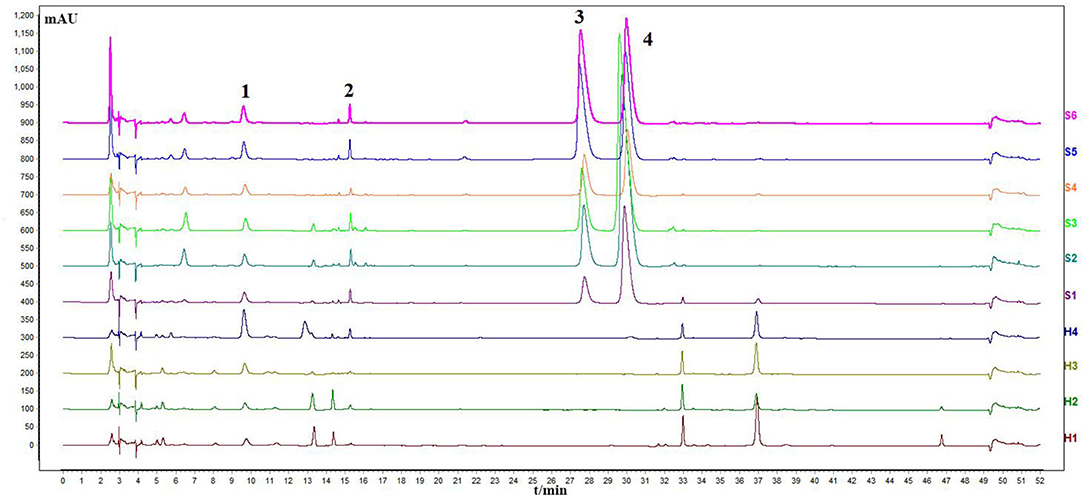
Figure 3. Chromatogram of HPLC content determination of P. harmala L. S1–S6, the seeds; H1–H4, the aerial parts.
Results of the HS-SPME-GC-MS Analysis
Based on the NIST database, the metabolites of the samples were qualitatively quantified by mass spectrometry, and the compounds with a matching degree above 80 points were selected. The mass spectrometry files were opened with MassHunter quantitative software for the integration and correction of chromatographic peaks. A total of 94 VOCs were detected by HS-SPME-GC-MS, and the metabolite numbers, values, and corresponding metabolites names of some metabolites detected in this experiment are shown in Table 2. These consisted of five alcohols, eight aromatic hydrocarbons, seven aldehydes, five acids, 17 terpenoids, 12 hydrocarbons, and 24 esters. The clustering heat map of all metabolites by classification and content is shown in Figure 5. The main volatile components in the whole herbs from Pakistan were 1,2-Benzenedicarboxylic acid, bis(2-methylpropyl) ester, Hexadecanoic acid, methyl ester, methyl salicylate, 3-methyl-4-isopropyl phenol, and propofol. The main volatile components in the whole herbs from Gansu were bis (2-methylpropyl) 1, 2-phthalate, methyl hexadecate, (Z, Z)-1,8,11, 14-heptadiene, p-xylene, ethyl hexadecanoate, butyl heptadiate-4-butyl phthalate, methyl tetradecanoate, and propofol. The main volatile components in the seed samples from Xinjiang were methyl hexadecanoate, p-xylene, octane, (Z)-9-octadecanoate, ethylbenzene, methyl octadecanoate, ethyl hexadecanoate, and methyl tetradecanoate (Table 2). Four alkaloids, caffeine, pentyl furan, isoquinoline, and 2-ethyl-furan were also detected by GC-MS.
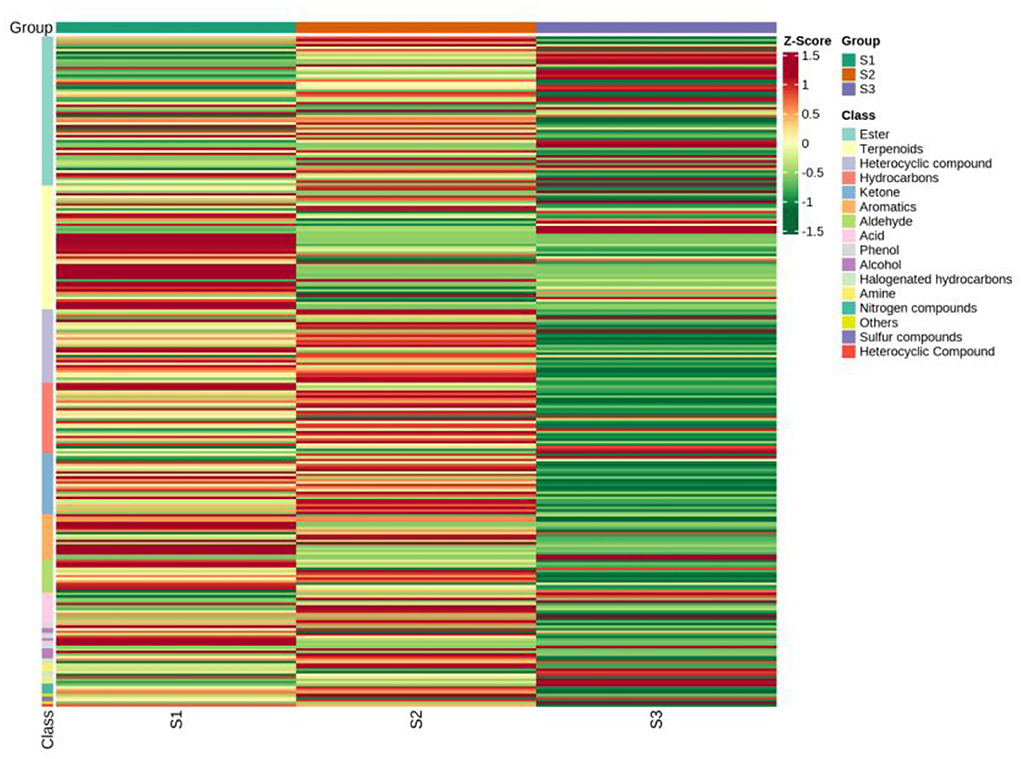
Figure 5. Clustering heat map of all metabolites by classification and content by GC-MS. S1, the whole herbs in Pakistan; S2, the whole herbs in Lanzhou; S3, the seeds in Xinjiang.
Antimicrobial Activity
Results of Antibacterial Activity
The petroleum ether extract and methanol extract of P. harmala showed broad-spectrum antibacterial activity against all the tested bacteria, as shown in Table 3. At the concentration of 800 μg·ml−1, the methanol extract of seeds displayed the highest bioactivity against E. coli ATCC 25922 with an inhibition rate of more than 90% and the petroleum ether extract of seeds showed slight inhibition against Staphylococcus aureus. The methanol and petroleum ether extracts of the aerial parts were only slightly against Candida albicans ATCC 24433 with inhibition rates of 20.08 and 15.07%, respectively.
The methanol extract of seeds displayed the highest bioactivity against X. oryzae, and the inhibition rate was 92.61% at 800 μg·ml−1. The petroleum ether extract of seeds and the aerial parts and the methanol extract of seeds displayed moderate bioactivity against X. oryzae, and the inhibition rates were from 48.05 to 68.68% at 800 μg·ml−1.
Citrus canker is the most serious Citrus bacterial disease caused by the gram-positive X. axonopodis; it can affect plant growth, fruit quality, and yield, which caused great economic losses to the citrus industry (Sun et al., 2019). In the present study, the methanol extract of seeds was effective against X. axonopodis, the inhibition rates were 67.42 and 100% at 50 and 800 μg·ml−1, respectively. The petroleum ether extract of seeds was slightly against X. axonopodis with an inhibition rate of 27.86% at 800 μg·ml−1.
As shown in Table 3, methanol extract of seeds exhibited moderate antimicrobial activity against P. atroseptica ACCC 19901, and the inhibition rate was 47.34% at 800 μg·ml−1. Petroleum ether extract of seeds and the aerial parts and methanol extract of seeds also exhibited slight antimicrobial activity against P. atroseptica ACCC 19901, and the inhibition rates were 32.15, 31.25, and 24.49%, respectively.
Antifungal Activity of P. harmala Extracts
The antifungal activities of P. harmala extract against pathogenic fungi are shown in Table 4. At the concentration of 500 μg·ml−1, the methanol extract of seeds effectively inhibited seven fungi of Rhizoctonia solani, Sclerotinia sclerotiorum, Botrytis cinerea, Fusarium graminearum, Magnaporthe oryzae, Phytophthora capsici, and Candida albicans ATCC 24433, with inhibition rates of 50.33, 64.32, 76.32, 52.98, 42.57, 55.58, and 47.47%, respectively. The petroleum ether extract of seeds weakly inhibited Rhizoctonia solani and Magnaporthe oryzae, with inhibition rates of 10.88 and 10.88%, respectively. The methanol and parts petroleum extract of aerial parts only weakly suppressed Fusarium graminearum and Magnaporthe oryzae, with inhibition rates of 10.89% and 10.7%, and 12.08% and 16.57%, respectively. The methanol extracts of seeds showed stronger antifungal activities with a wide antifungal spectrum. Our results showed that the aerial parts and seed extracts have broad-spectrum antibacterial activities against agricultural pathogenic bacteria, which may be related to its alkaloids (Adnan et al., 2019; Zhang et al., 2020), but its specific active components and its antibacterial mechanism need to be further studied.
Furthermore, we evaluated the antimicrobial activity of four alkaloids isolated from P. harmala, including Rhizoctonia solani, Sclerotinia sclerotiorum, Magnaporthe oryzae, Fusarium graminearum, and Phytophthora capsici, and found that the growth of all five strains was inhibited in the presence of alkaloids (Tables 3, 4), while harmine and harmaline showed broad-spectrum antibacterial activities against X. oryzae (ACCC 11602), E. coli ATCC 25922, and Staphylococcus aureus (Newman). These findings indicated that alkaloids could account for antimicrobial activity. Furthermore, harmine and harmaline showed better antibacterial activities, which could be used as lead molecules in the development of new antimicrobial drugs. In the future study, we are going to select harmine and harmaline as lead compounds, and a series of derivatives are supposed to design and synthesize to evaluate their antifungal activities and preliminary antifungal mechanism.
Conclusion
In conclusion, the aerial parts and seeds of Peganum harmala L showed a large difference in the content of four alkaloids. Harmine and harmaline were mainly found in seeds, while the aerial parts mainly contained vasicine and vasicinone. A total of 94 metabolites were detected by HS-SPME-GC-MS technology for the first time. The VOCs of P. harmala mainly consisted of hydrocarbons, acids, ketones, aromatic hydrocarbons, esters, and heterocyclic compounds. In addition, the extracts of the aerial parts and seeds showed antibacterial activities against 12 bacterial strains and fungi. This study demonstrates that the alkaloids from P. harmala are a potentially non-toxic and ecofriendly botanical fungicide for the management of fungi.
Data Availability Statement
The original contributions presented in the study are included in the article/Supplementary Material, further inquiries can be directed to the corresponding author/s.
Author Contributions
Methodology, conceptualization, investigation, and writing—original draft preparation: NW. Supervision, writing—review and editing, and project administration: ZY. Investigation and formal analysis: JA and ZZ. Writing—review and visualization: YL and JF. All authors have read and agreed to the published version of the manuscript.
Funding
This work was supported by the Key Project of NMPA Key Laboratory for Quality Control of Traditional Chinese Medicine (2020GSMPA-KL11), the Natural Science Foundation of Gansu Province (20JR5RA311 and 20JR5RA216), the Key Program for International S&T Cooperation Projects of Gansu Province (18YF1WA115), and the Fundamental Research Funds for the Central Universities (lzujbky-2021-kb40 and lzujbky-2020-46).
Conflict of Interest
The authors declare that the research was conducted in the absence of any commercial or financial relationships that could be construed as a potential conflict of interest.
Publisher's Note
All claims expressed in this article are solely those of the authors and do not necessarily represent those of their affiliated organizations, or those of the publisher, the editors and the reviewers. Any product that may be evaluated in this article, or claim that may be made by its manufacturer, is not guaranteed or endorsed by the publisher.
Supplementary Material
The Supplementary Material for this article can be found online at: https://www.frontiersin.org/articles/10.3389/fmicb.2022.916371/full#supplementary-material
References
Adnan, M., Ali, S., Sheikh, K., and Amber, R. (2019). Review on antibacterial activity of Himalayan medicinal plants traditionally used to treat pneumonia and tuberculosis. J. Pharm. Pharmacol. 71, 1599–1625. doi: 10.1111/jphp.13156
Azzazy, H. M. E. S., Fahmy, S. A., Mahdy, N. K., Meselhy, M. R., and Bakowsky, U. (2021). Chitosan-coated PLGA nanoparticles loaded with peganum harmala alkaloids with promising antibacterial and wound healing activities. Nanomaterials 11, 2438. doi: 10.3390/nano11092438
Benarous, K., Bombarda, I., Iriepa, I., Moraleda, I., Gaetan, H., Linani, A., et al. (2015). Harmaline and hispidin from Peganum harmala and Inonotus hispidus with binding affinity to Candida rugosa lipase: in silico and in vitro studies. Bioorg. Chem. 62, 1–7. doi: 10.1016/j.bioorg.2015.06.005
Feng, X. Y., Wang, H. W., Wang, Z. R., Huang, P. M., and Kan, J. Q. (2022). Discrimination and characterization of the volatile organic compounds in eight kinds of huajiao with geographical indication of China using electronic nose, HS-GC-IMS and HS-SPME-GC-MS. Food Chem. 375, 131671. doi: 10.1016/j.foodchem.2021.131671
Ferreira-Santos, P., Badim, H., Salvador, A. C., Silvestre, A. J. D., Santos, S. A. O., Rocha, S. M., et al. (2021). Chemical characterization of Sambucus nigra L. flowers aqueous extract and its biological implications. Biomolecules 11, 1222. doi: 10.3390/biom11081222
Jalali, A., Dabaghian, F., and Zarshenas, M. M. (2021). Alkaloids of Peganum harmala: anticancer biomarkers with promising outcomes. Curr. Pharm. Des. 27, 185–196. doi: 10.2174/1381612826666201125103941
Li, S., Cheng, X., and Wang, C. (2017). A review on traditional uses, phytochemistry, pharmacology, pharmacokinetics and toxicology of the genus Peganum. J. Ethnopharmacol. 203, 127–162. doi: 10.1016/j.jep.2017.03.049
Martinez-Navarro, E. M., Cebrian-Tarancon, C., Moratalla-Lopez, N., Lorenzo, C., Alonso, G. L., and Salinas, R. M. (2021). Development and validation of an HPLC-DAD method for determination of oleuropein and other bioactive compounds in olive leaf by-products. J. Sci. Food Agric. 101, 1447–1453. doi: 10.1002/jsfa.10758
Mukhtar, N., Iqbal, K., Anis, I., and Malik, A. (2002). Sphingolipids from Conyza canadensis. Phytochemistry 61, 1005–1008. doi: 10.1016/S0031-9422(02)00385-0
Sahu, P. K., Ramisetti, N. R., Cecchi, T., Swain, S., Patro, C. S., and Panda, J. (2018). An overview of experimental designs in HPLC method development and validation. J. Pharm. Biomed. Anal. 147, 590–611. doi: 10.1016/j.jpba.2017.05.006
Sun, L., Nasrullah, K.e, F., Nie, Z., Wang, P., and Xu, J. (2019). Citrus genetic engineering for disease resistance: past, present and future. Int. J. Mol. Sci. 20, 5256. doi: 10.3390/ijms20215256
Wang, C. H., Zeng, H., Wang, Y. H., Li, C., Cheng, J., Ye, Z. J., et al. (2015). Antitumor quinazoline alkaloids from the seeds of Peganum harmala. J. Asian Nat. Prod. Res. 17, 595–600. doi: 10.1080/10286020.2015.1042373
Wang, K. B., Li, D. H., Bao, Y., Cao, F., Wang, W. J., Lin, C., et al. (2017). Structurally diverse alkaloids from the seeds of Peganum harmala. J. Nat. Prod. 80, 551–559. doi: 10.1021/acs.jnatprod.6b01146
Wen, F. F., Zheng, L. M., Li, X. J., Li, Y., Zhang, L., Cheng, X. M., et al. (2012). Research on quality standards of herbs of Peganum harmala. Zhongguo Zhong Yao Za Zhi 37, 2971–2976. doi: 10.4268/cjcmm20121929
Xu, X. P., BU, R., and Chen, X. H. (2008). Investigation on distribution and medicinal prospect of Peganum in our province and northwest of China. J. Shaanxi Coll. Trad. Chin. Med. 31, 70–71.
Yan, Y. F., Wu, T. L., Du, S. S., Wu, Z. R., Hu, Y. M., Zhang, Z. J., et al. (2021). The antifungal mechanism of isoxanthohumol from Humulus lupulus Linn. Int. J. Mol. Sci. 22, 10853. doi: 10.3390/ijms221910853
Ye, L., Zhang, J., Xiao, W., and Liu, S. (2020). Efficacy and mechanism of actions of natural antimicrobial drugs. Pharmacol. Ther. 216, 107671. doi: 10.1016/j.pharmthera.2020.107671
Keywords: alkaloids, HS-SPME-GC-MS, volatile organic compounds, antimicrobial activity, Peganum harmala L
Citation: Wang N, An J, Zhang Z, Liu Y, Fang J and Yang Z (2022) The Antimicrobial Activity and Characterization of Bioactive Compounds in Peganum harmala L. Based on HPLC and HS-SPME-GC-MS. Front. Microbiol. 13:916371. doi: 10.3389/fmicb.2022.916371
Received: 09 April 2022; Accepted: 22 June 2022;
Published: 19 July 2022.
Edited by:
Maria Jorge Campos, Polytechnic Institute of Leiria, PortugalReviewed by:
Tao Feng, Shanghai Institute of Technology, ChinaGuiqiang He, Southwest University of Science and Technology, China
Copyright © 2022 Wang, An, Zhang, Liu, Fang and Yang. This is an open-access article distributed under the terms of the Creative Commons Attribution License (CC BY). The use, distribution or reproduction in other forums is permitted, provided the original author(s) and the copyright owner(s) are credited and that the original publication in this journal is cited, in accordance with accepted academic practice. No use, distribution or reproduction is permitted which does not comply with these terms.
*Correspondence: Zhigang Yang, yangzg@lzu.edu.cn