- 1Biocontrol Engineering Research Center of Crop Disease and Pest, Yunnan University, Kunming, China
- 2Yunnan Engineering Research Center of Microbial Agents, Yunnan University, Kunming, China
- 3School of Life Science, Yunnan University, Kunming, China
- 4Kunming Branch of Yunnan Tobacco Company, Kunming, China
- 5School of Agriculture, Yunnan University, Kunming, China
- 6School of Ecology and Environmental Science, Yunnan University, Kunming, China
- 7Department of Microbiology, PSGVP Mandal's S I Patil Arts, G B Patel Science and STKVS Commerce College, Shahada, India
- 8Hainan Key Laboratory for Sustainable Utilization of Tropical Bioresource, College of Tropical Crops, Hainan University, Haikou, China
- 9Department of Agronomy, The University of Haripur, Haripur, Pakistan
Root-knot nematode (Meloidogyne incognita) is the most widespread nematode affecting Solanaceae crops. Due to the lack of effective measures to control this nematode, its management can be achieved, using biocontrol agents. This study investigated in vitro efficacy of the antagonistic bacterial strain J211 isolated from tobacco rhizosphere soil against M. incognita, and further assessed its role in controlling nematodes, both in pot and field trials. Phylogenetic analysis of the 16S rRNA gene sequence of strain J211 assigned to Burkholderia arboris. Culture filtrates B. arboris J211 exhibited anematicidal activity against the second-stage juveniles (J2s) of M. incognita, with a 96.6% mortality after 24 h exposure. Inoculation of J211 in tobacco roots significantly reduced the root galling caused by M. incognita, both in pot and field trials. Meanwhile, plant growth-promoting (PGP) traits results showed that J211 had outstanding IAA-producing activity, and the IAA production reached 66.60 mg L−1. In the field study, B. arboris J211 also promoted tobacco growth and increase flue-cured tobacco yield by 8.7–24.3%. Overall, B. arboris J211 as a high-yielding IAA nematicidal strain effectively controlled M. incognita and improved tobacco yield making it a promising alternative bionematocide.
Introduction
Plant-parasitic nematodes, such as root-knot nematodes (RKNs), cause over $100 billion in annual crop losses, worldwide (Elling, 2013). Meloidogyne spp. is considered the most damaging nematodes in the world (Jones et al., 2013). A remarkable stage in the life cycle of the RKNs is the second-stage juveniles (J2s) which is the stage that has the capacity to move through soil and infect plants (Topalovi et al., 2019). RKNs severely reduce crop production by absorbing nutrients from host plants for self-reproduction (Williamson, 1999). These nematodes are a broad host range of over 2,000 plant species (Sasser, 1980), including vegetables, beans, grains, grass shrubs, fruit trees, and industrial crops (Bagheri et al., 2014). In particular, M. incognita, known as southern root-knot nematode, causes the most devastating root-knot diseases (Akhyani et al., 1984).
Over the past decades, several traditional strategies, including rotation, resistance breeding, and application of nematicidal agents, were used to control nematodes (Oka et al., 2012; Ralmi et al., 2016; Makunde et al., 2018). Due to monoculture or rotation with plant species that are also hosts (Nyczepir and Thomas, 2009), coupled with the difficulty of cultivating disease-resistant varieties (Sadeghi et al., 2021), RKNs control still remains extremely difficult. Control of RKNs has been mostly achieved through the use of nematicides, such as fumigants, carbamates, and organophosphates (Karavina and Mandumbu, 2012), and despite their effectiveness, nematicides are highly toxic to the natural environment and human health, that is why their use being often banned (Sharma and Sharma, 2016). During the last years, more emphasis has been given to nematode management on green and environment-friendly alternative strategies (Zhai et al., 2019).
Currently, biological control has shown an eco-friendly approach to reducing nematode damage (Cheng et al., 2017). Microorganisms are living biological agents that produce bioactive molecules and have the ability to suppress, antagonize and control nematodes in most cultivated fields (Chelinho et al., 2017). Various antagonistic bacterial and fungal species have been isolated for biocontrol of RKNs (Xiang et al., 2017; Du et al., 2020). For example, previous studies have demonstrated the biological control capability of bacterial isolates of the genera Pseudomonas spp. (Ali et al., 2002), Bacillus thuringiensis (Yu et al., 2015), Pasteurella penetrans (Kariuki and Dickson, 2007) and Purpureocillium lilacinum (Kiewnick and Sikora, 2003). Abbasi et al. (2013) used Bacillus spp. to reduce nematode infestation of eggplants (Solanum melongena). Pretreatment of tomato seeds with Streptomyces hydrogenans DH-16 culture supernatant reduced nematode infestation and promoted tomato seedlings' growth (Sharma et al., 2020). Purpureocillium lilacinum AUMC 10149 is used to control the root-knot nematode M. incognita infestation of tomatoes (Isaac et al., 2021). Microorganisms, the natural enemies of nematodes, inhibit nematode disease through multiple pathways: the production of toxins, antibiotics, crystal proteins, and nematicidal substances (Zhang and Mo, 2006). A cyclic dipeptide Cyclo (L-Pro-L-Leu) isolated from the metabolite of Pseudomonas simiae MB751 has activity against the J2s of M. incognita (Sun et al., 2021). M. incognita is synergistically controlled by the crystal proteins Cry6Aa and Cry55Aa produced by Bacillus thuringiensis (Peng et al., 2011). Cheng et al. (2017) revealed that 11 volatile organic compounds produced by Paenibacillus polymyxa KM2501-1 control M. incognita through multiple strategies.
Although significant progress has been made in the use of microorganisms to control RKNs in recent years, microbial root competence was considered to be a key prerequisite for successful biocontrol (Maurer et al., 2013). For this reason, new nematicidal strains with long-term survival should be isolated to control RKNs under field conditions. Plant rhizosphere soil surrounds a variety of bacteria that promote plant growth by dissolving phosphates, producing siderophores and plant growth regulators. This bacterium is called plant growth-promoting rhizobium (PGPR). PGPR is also considered a potential alternative option for controlling RKNs (Groover et al., 2020). Many studies indicated the antagonistic ability of PGPR toward RKNs, including genera Bacillus, Serratia, Pseudomonas, and Burkholderia (Gray and Smith, 2005). The rhizosphere bacterium Pseudoxanthomonas japonensis ZKB-2 showed strong nematostatic activity against M. incognita on tomatoes (Lycopersicon esculentum) (Hu et al., 2018). Pseudomonas aeruginosa and Burkholderia gladioli are used to control M. incognita on tomatoes, remarkably reducing root galls and promoting plant growth (Khanna et al., 2019). El-Aal et al. (2021) reported that mixed application of Serratia spp. and Pseudomonas spp. controlled M. incognita and promoted the growth of sponge gourd (Luffa aegyptiaca). Bacillus cereus Bc-cm103 isolated from cucumber (Cucumis sativus) rhizosphere completely killed the J2s of M. incognita within 12 h, and significantly reduced the infection of the nematode to cucumber root (Yin et al., 2021). Even though many microbial agents have been used to control root-knot nematodes, the activity and stability of biological agents are still affected by environmental factors, such as soil texture, moisture, and temperature.
Considering the harm of chemicals to the environment and the instability of biological agents in the current application of nematode control, it is necessary to screen an environment-friendly and sustainable method of nematode control. The objective of the present study was to evaluate the antagonistic bacterial strain J211, isolated from tobacco rhizosphere soil, for RKNs biocontrol potential on tobacco. Specifically, the purposes were to assess the nematicidal activity of strain J211 on the viability of M. incognita in vitro and to evaluate the efficacy of the strain as a biocontrol agent under pot and field conditions.
Materials and Methods
Isolation of Rhizospheric Bacteria
We followed the method described by Huang et al. (2014) with modifications to isolate the rhizospheric bacteria. Rhizosphere soil in which healthy tobacco plants grew was collected from areas infested with tobacco root-knot nematode disease. Tobacco rhizosphere soils were collected from Anning County, Yunnan Province, China (102°21′16″E, 24°56′20″N). Soil samples (1 g) were suspended in 10 mL of sterile distilled water and mixed on a table concentrator for 10 min. The soil samples were serially diluted (up to 10−7-fold), plated on a nutrient agar (NA) medium, and incubated at 30 ± 2°C for 2 to 3 days. Bacterial colonies growing on the plates were isolated for further study based on color and morphological characteristics.
Meloidogyne incognita Population
The second-stage juveniles (J2s) of M. incognita were obtained from a pure population isolated in Fumin county, Yunnan province, China from tobacco (Nicotiana tabacum var. Hongda) roots. Eggs were extracted from roots according to Hussey and Barker (1973) and hatched in sterile water at 25–28°C for 72 h to obtain freshly hatched J2s. The collected J2s suspension was diluted with sterile water to a concentration of 5,000 per milliliter. Only freshly hatched J2s of M. incognita were used in the experiments.
Toxicity Tests of Bacterial Broth Culture Filtrates
The isolated bacterial strains were screened using in vitro nematicidal assay, for which purpose, they were transferred to a NA medium plate. After activation on the NA plate at 30°C, purified single colonies were picked using a sterile inoculating loop and placed in a 500 ml conical flask containing 300 ml nutrient broth (NB). The isolated strains were grown in NB at 30°C for 3 days with shaking at 180 rpm and filtered through a 0.22 μm sterile filter unit to yield the culture filtrate. Three mL of bacterial broth culture filtrates were added to 35 mm Petri dishes, then adding suspension containing ~100 J2s in 20 μL sterile water. The controls were sterile NB with 100 μg mL−1 rifampin added to the Petri dishes. The number of dead nematodes was recorded after 12, 24, and 36 h. Viability was established using the hydroxide technique (Chen and Dickson, 2000), and nematodes without mobility are considered dead. The mortality using the Abbott (1925) formula: relative mortality (%) = [(mortality in treatment—mortality in negative control)/(1—mortality in negative control)] × 100. All treatments were conducted with five replicates and the experiment was repeated twice.
Identification of the Bacterial Strain
The DNA of J211 was extracted by Bacterial Genomic DNA Extraction Kit (TaKaRa, Japan). The 16S rRNA gene was amplified using universal primers 27F and 1492R. The PCR products were separated on agarose gel (1%) by electrophoresis, purified with the Agarose Gel DNA Extraction Kit (TaKaRa, Japan) according to the manufacturer's instructions, and sequenced using the ABI3730xl platform (Beijing Tsingke Biological Technology Co., Ltd, China). The sequence of J211 was submitted to the NCBI GenBank database and accession numbers were obtained. The acquired 16S rRNA sequence of J211 was analyzed by using the EzBioCloud database. Other sequences of related taxa were selected from the EzBioCloud database. The Multiple Sequence Alignment was done using MEGA version 7. Phylogenetic tree of 16S rRNA gene sequences using MEGA version 7 with distance options according to the Kimura two-parameter model (Kimura, 1980) and the neighbor-joining (NJ) method, and 1,000 bootstrap replications.
Biochemical Characterization of J211
The strain J211 was further characterized based on its biochemical characteristics as per Bergey's Manual of Systematic Bacteriology (Holt et al., 1994). Routine biochemical tests like Gram staining, spore staining, catalase activity, starch hydrolysis, gelatin hydrolysis, indole test, nitrate reduction, Methyl Red (M. R.) test, Voges-Proskauer (V. P.) test, and H2S production were performed for J211.
Assessment of Plant Growth-Promoting (PGP) Traits of J211
IAA Production
Indole-3-Acetic Acid (IAA) production of J211 in the culture broth was determined by the colorimetric method as described by Tang and Bonner (1948) with minor modifications. J211 was grown in NB and incubated at 28 ± 2°C in an orbital incubator shaker at 180 rpm for 144 h. The cells in samples were harvested by centrifugation at 10,000 rpm for 10 min. Then, 4 ml of Salkowaski reagent (50 mL 35% perchloric acid mixed with 1 ml of 0.5% FeCl3) were added to 2 ml of supernatant and incubated for 30 min at room temperature in dark for the development of color, and the absorbance was measured at OD 530 nm. The concentration of IAA present in supernatant was calculated using a standard curve of IAA (Gordon and Weber, 1951).
Phosphate Solubilization
The strain J211 to be tested was inoculated into the Monkina inorganic phosphorus liquid medium at a dose of 1% and incubated at 28 ± 2°C and 180 rpm for 7 days. Then, 2 ml of the bacterial suspension were then centrifuged to obtain the supernatant. Dissolved phosphorus content was then quantified according to the antimony molybdenum anti-colorimetric method (Wu et al., 2012).
Siderophore Production
Quantitative analysis of siderophore was performed by CAS shuttle assay (Schwyn and Neilands, 1987). J211 was inoculated in a sterile siderophore inducing medium (Alexander and Zuberer, 1991) and incubated at 28 ± 2°C in an orbital incubator shaker at 180 rpm. After 7days, 1 mL supernatant of the culture was mixed with 1 mL of CAS reagent and 20 μL of Shuttle Solution (0.2 M 5-sulfosalicyclic acid). After 20 min of incubation at room temperature, absorbance was read at 630 nm. Siderophore Unit (%) was calculated using the following formula: Siderophore Unit (%) = (Ar-As)/Ar × 100, where, Ar absorbance of reference at 630 nm, As absorbance of the sample at OD 630 nm.
Pot Experiment
Tobacco var. Hongda is extremely susceptible to root-knot nematodes, and for this reason, it was used in the assay. The potting mix (pH 6.5–7) consisted of peat and a small amount of soil amended with 0.3% (w/v) of a water-soluble fertilizer (Haoyunzhixing®, Haoshiji Chemical Industry Co., Sichuan, China). Three days before transplanting, each pot was inoculated with 3,000 J2s of M. incognita. Then five-leaf stage tobacco plants were transplanted into the pots (15cm diam, 13cm depth), one for each pot. J211 was inoculated in NB at 30°C for 3 days with shaking at 180 rpm, and then the fermentation broth was diluted with sterile water to adjust to 1 × 109 CFU ml−1. The experiment was split into four treatments, including (i) untreated control with 300 mL water, (ii) 1 g of commercially available Purpureocillium lilacinum powder (Xinlonghui®, Xinlong Biotechnology Co., Jiangxi, China) was diluted 300-fold with water, and 300 ml of the suspension was inoculated on the roots of tobacco plants, (iii) inoculated with 300 ml culture of J211 adjusted to 1 × 109 CFU ml−1, and (iv) 1 g of commercially available P. lilacinum powder was diluted 300-fold with adjusted J211 culture (1 × 109 CFU ml−1), and 300 ml of the mixed suspension was inoculated on the roots of tobacco plants. Five replicated pots were used for each treatment. Each experiment was conducted twice, wherein plants were maintained at 25–28°C and relative humidity between 60 and 70% for 8 weeks.
Plant height was measured from five plants per treatment after 8 weeks. The root galling index (GI) was determined using a 0 to 10 rating system (Barker et al., 1986), where 0 = no galls and 10 = 90–100% of roots galled. Egg masses were stained in aqueous phloxine B and enumerated under a dissecting microscope (Dickson and Struble, 1965).
Field Experiment
In April 2019, field trials were conducted in tobacco fields at Fumin county, Kunming, China (102°61′45″E, 25°47′34″N). The field has been in conventional tobacco products for many years and was infested with M. incognita (510 ± 23 nematodes per 100 g soil). The soil type was sandy clay with 38.9 g kg−1 of organic matter. The available nitrogen (AN), phosphorous (AP), and potassium (AK) contents were 153.6 mg kg−1, 68.7 mg kg−1, and 512 mg kg−1 in the field, respectively, and pH was 5.37. Potassium sulfate (150 kg ha−1) and N-P-K compound fertilizer (600 kg ha−1) were applied as basal fertilizers.
Tobacco var. Hongda was also used in the field trials of five-leaf stage plants. Five treatments were applied: (i) a 12,000-fold dilution of fluopyram (Lufta®, Bayer Crop Science, Beijing, China), (ii) a 2,000-fold dilution of fosthiazate (Sunchungtan®, Farm Hannong Co., Seoul, Korea), (iii) a 10-fold dilution of Burkholderia arboris J211 broth culture(J211 was inoculated in 5 L NB at 30 °C, 180 rpm for 7 days with a concentration of about 2.6 × 1011CFU ml−1), and (iv) a 300-fold dilution P. lilacinum (Xinlonghui®, Xinlong Biotechnology Co., Jiangxi, China), the application of (v) water was the control. 300 mL aliquot of each treatment was poured into the soil around the tobacco roots four times every 15 days. The experiment was conducted as a completely randomized block design with three replicates, each replicated plot consisted of a single row, 25 m long, with 50 tobacco plots under the same management.
To assess tobacco growth after inoculating with J211, agronomic characters, including height, stem girth, effective leaves, and leaf area, were investigated on ten random plants per plot at the topping stage (75 days after inoculation with J211). Furthermore, flue-cured tobacco yields were recorded according to the National Standard (GB 2635-1992). The disease index and control efficacy were recorded after harvest according to the National Standard (GB/T 23222−2008). Root-knot severity was rated on a standard scale from 0 to 9: 0, no symptoms; 1, less than a quarter of the roots had a few root-knots; 3, one quarter to one-third of the roots have a few root-knots; 5, one third to one-half of the roots have root-knots; 7, more than one-half of the roots have root-knots; 9, all roots are covered with root-knots. The disease index (DI) and control efficacy were calculated using the following formulas:
Data Analysis
Data were analyzed by one-way ANOVA, and the means of the treatments were separated by Duncan's multiple-range test (P < 0.05) using SPSS software (version 23 for Windows; SPSS, Chicago, IL, USA).
Results
Identification of Bacterial Strain and Its in vitro Nematicidal Activity
We isolated and purified 17 strains of bacteria from tobacco rhizosphere soil, and in vitro nematicidal assays showed that the fermentation supernatants of 4 strains had toxic effects on M. incognita J2s (data not shown). In particular, the isolatedJ211 showed high killing activity against M. incognita J2s. Testing in vitro displayed that the J211 culture filtrates exhibited highly nematocidal activity against J2s of M. incognita, with mortality close to 96.6% 24 h and 100% after 36 h (Figure 1). The colony of J211 was round, white, and non-transparent, with a smooth surface (Figure 2A). The 16S rRNA gene sequence of J211 was deposited at the NCBI GenBank database with accession MT879598. J211 was identified to the species level by using the 16S rRNA gene sequence to construct an NJ phylogenetic tree, the 16S rRNA sequence of J211 from other selected species (Figure 2B). The tree showed that J211 was located at the same branch as Burkholderiaarboris R-24201, with a 99% similarity to B. arboris.
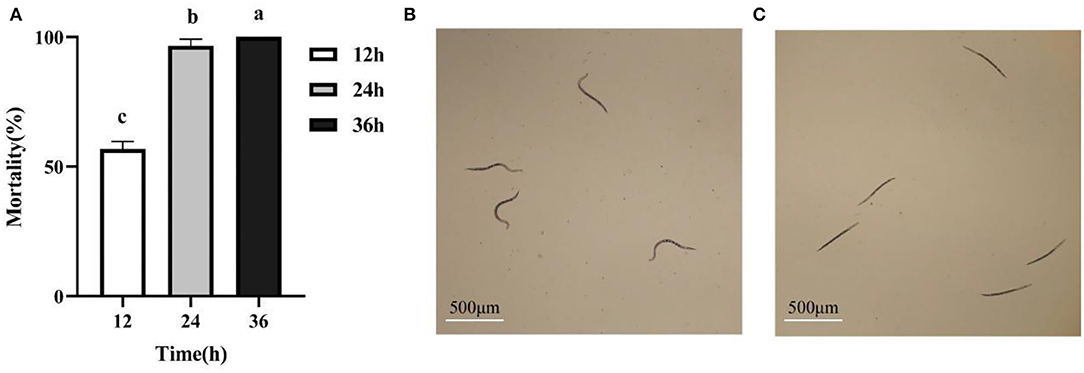
Figure 1. The nematocidal activity of the culture filtrates from J211 against the J2s of M. incognita at different times. Values are means ± standard deviation of two runs with five replicates each (A). Relationships among means were analyzed by one-way ANOVA and Duncan's multiple-range test (P < 0.05). Means with the same letter did not differ significantly. Nematodes activity shows control, curved are vigorous (B); the antagonistic stiff died and have no vitality (C) after 24 h. Bar: 500 μm.
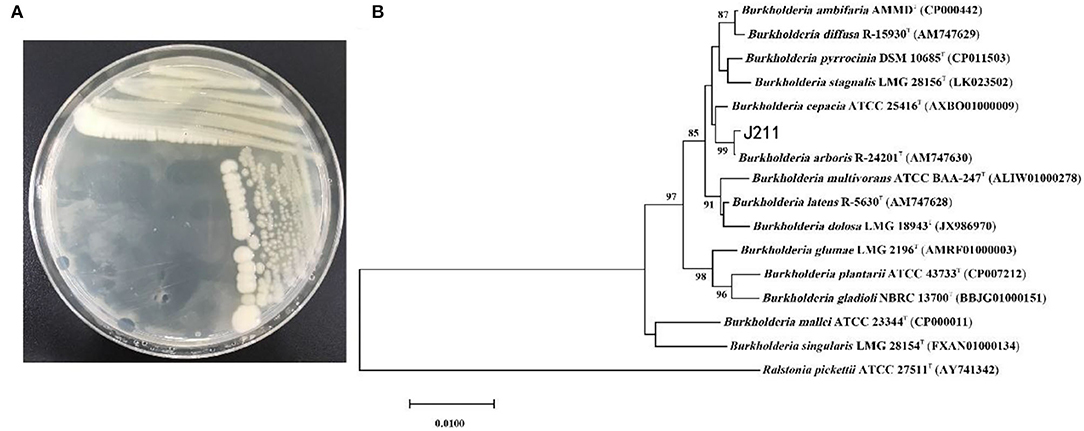
Figure 2. Colony morphology and phylogenetic tree of strain J211. Colony morphology of J211 on NA medium (A). Phylogenetic tree constructed with J211 and other type strains of related species in Burkholderia based on16s rRNA gene sequences (B). The bootstrap consensus tree, inferred from 1,000 replicates, was reconstructed using the neighbor-joining (NJ) method based on the general time-reversible model.
Biochemical Characterization and Plant Growth-Promoting (PGP) Traits of J211
The biochemical characteristics of J211 were determined according to Gram staining, spore staining, catalase test, starch hydrolysis, gelatin hydrolysis, indole test, nitrate reduction, and methyl red (M. R.), Voges-Proskauer (V. P.) and H2S production (Table 1). J211 had a strong ability to produce IAA, with the production amount reaching 66.60 mg L−1 (Table 1). Quantitative analysis showed that J211 could dissolve inorganic phosphorus, with the dissolved amount reaching 3.41 mg L−1 (Table 1). J211 showed a low siderophore production efficiency of only 0.19% (Table 1).
Effect of J211 for Control of M. incognita in Pot Trials
To evaluate the efficacy of J211 against M. incognita under potted conditions, we conducted two rounds of tobacco pot experiments. Plant height, galling index (GI), and the number of egg masses were analyzed after 8 weeks (Table 2). All nematicidal treatments, including P. lilacinum or J211, greatly decreased GI and egg masses compared to the untreated control. Due to the severe infestation of root-knot nematodes, the plant height of control was significantly lower than other treatments. In the treatment of inoculated with P. lilacinum, J211, and combined P. lilacinum and J211, insignificant differences were observed for height and GI, in the pot trials. Combined inoculation of P. lilacinum and J211 significantly reduced the number of egg masses compared to their respective inoculations alone.

Table 2. Effects of J211 on the growth of plants and the formation of root-knots in tobacco roots caused by M. incognita in the pot trial.
Control of Tobacco Root-Knot in Field Experiments
In the field trial, the role of different treatments to nematodes was evaluated after tobacco harvest. The roots of the untreated control were severely damaged after nematode infestation, and the root knots were thick (Figure 3E). However, four other nematicidal measures significantly reduced disease symptoms at the root of tobacco (Figures 3A–D). Moreover, the broth culture of J211 at a 10-fold dilution (DI = 91.1), prominently decreased the DI of tobacco roots by 57.8 compared to untreated control (DI = 33.3) (Figure 4). No difference appeared in the tobacco DI after J211 treatment with the chemical agents fluopyram and fosthiazate. Nematicidal applications significantly increased tobacco height compared to untreated control. After chemical and biological agents treatment, caused by M. incognita was inhibited. The control effects were 70% (fluopyram), 56.4% (fosthiazate), 64.3% (J211), and 42.4% (P. lilacinum), respectively. Particularly, the application of J211 displayed a better effect on the promotion of tobacco height and stem girth, with an increase of effective leaves and leaf area, in comparison to chemical nematicides and P.lilacinum(Table 3). At harvest, the tobacco yield was greater in the J211 treatment than in other treatments (Table 3).
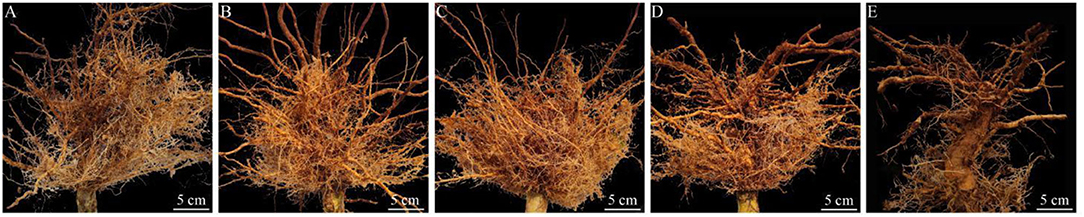
Figure 3. Root symptoms of tobacco with different treatments after harvest. Fluopyram (A), Fosthiazate (B), J211 (C), P. lilacinum (D), Control (E). Bar: 5 cm.
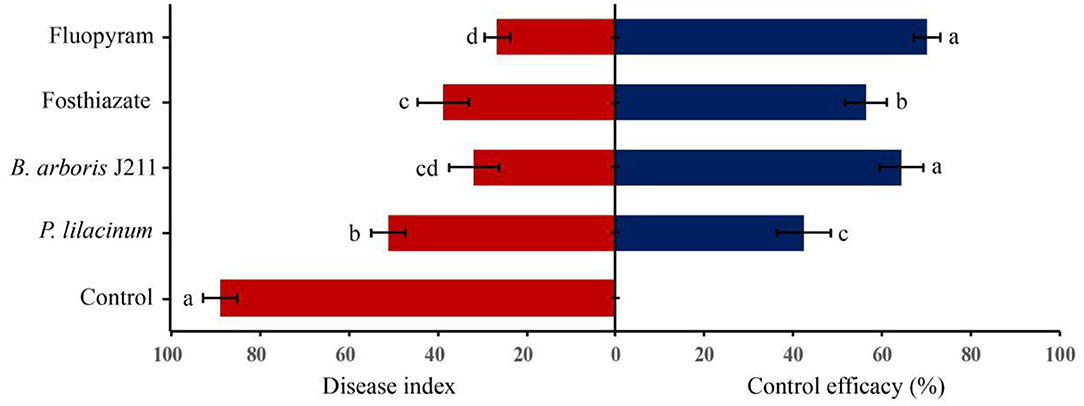
Figure 4. Effect of chemical and biological nematicides against tobacco RKNs in the field. Bars represent the mean ± standard deviation of three replicates (10 tobacco plants per replicate). Bars with the same letters are not significantly different (P > 0.05) based on Duncan's multiple-range tests.
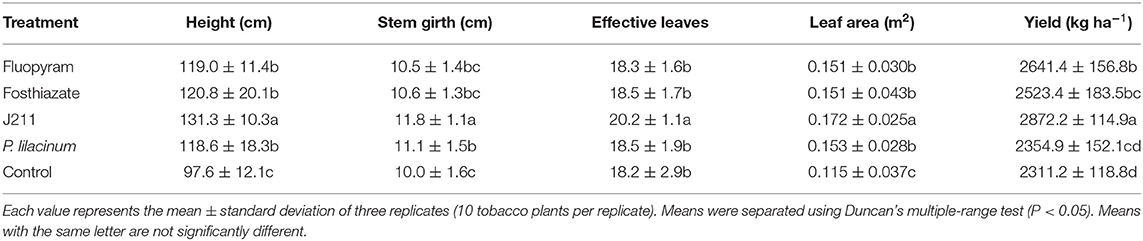
Table 3. Effects of different treatments on agronomic characteristics, yield, and control efficacy of M. incognita in tobacco field assay.
Discussion
Root-knot nematodes are severe threats to world agriculture. The application of bacteria especially PGPR, and biological control of RKNs have drawn increasing attention (Siddiqui and Futai, 2009; Xiang et al., 2017). In vitro tests from this study indicated J211 a high mortality of M. incognita J2s. Phylogenetic analysis assigned the strain J211 to B. arboris. To our knowledge, this is the first report of antagonistic activity shown by a strain of B. arboris against M. incognita.
Both the pot and field trials demonstrated the potential of B. arboris J211 as a biological nematicide for the management of RKNs in tobacco cultivars. The use of biocontrol bacteria for the management of nematodes is difficult due to inefficient bacterial colonization (Liu et al., 2020). It is stated that PGPR has a strong ability to survive in and colonize rhizosphere soil (Saharan and Nehra, 2011). High control efficacy was obtained by drenching the soil with rhizosphere isolates bacteriaJ211to likely ensure colonization, and drastically reduced RKNs incidence. In pot experiments, biocontrol treatments, including inoculation with J211, P. lilacinum, and mixed inoculation of J211 and P. lilacinum, significantly reduced nematode-induced root damage compared to controls. The results of the pot experiment showed that the three biocontrol treatments had no significant difference in root galling index (GI) caused by nematodes. To evaluate the efficacy of J211 in controlling nematodes under field conditions, we compared the effects of chemical and biocontrol fungi treatments. The application of J211 reduced the root damage with a nematode control efficacy similar to fluopyram or even greater than that of fosthiazate and P. lilacinumin in the field experiment. P. lilacinum, an egg-parasitic fungus with the ability to infect and destroy nematode eggs, has become a commercial biological agent for nematode control (Mendoza et al., 2004; Baidoo et al., 2017). Indeed, the stability of biologics is affected by a variety of environmental factors. P. lilacinum was significantly less effective than J211 in controlling nematodes under field conditions. The possible explanation is that J211 isolated from tobacco rhizosphere soil is more suitable to colonize tobacco rhizosphere soil to play its role. The present study revealed the biocontrol potential of J211 in controlling M. incognita. PGPR mediated plant resistance toward RKNs is provided by the production of various antagonistic compounds, lytic enzymes, toxins, and antibiotics that inhibit the nematode proliferation or directly kill them (Cetintas et al., 2018). In this study, we observed that the effect of J2s completely killed by J211 fermentation filtrate within 36 h may be somewhat similar to that reported by Köthe et al. (2003) that Burkholderia cepacia culture filtrate containing extracellular toxin killed Caenorhabditis elegans within 24 h. However, the specific mechanism of action of J211 against M. incognita is not understood.
Furthermore, we measured the PGP traits of J211 and found that the ability to dissolve phosphate and produce siderophore was not outstanding, but noted that J211 was a high-yielding strain of IAA with a yield of up to 66.6 mg L−1. Tobacco inoculated with J211 gave rise to an 8.7–24.3% flue-cured tobacco yield increase in this study. On the one hand, J211 reduced nematode damage to tobacco roots, and on the other hand, it may be related to J211, which produces IAA activity, and promoted root growth. J211 had a similar nematode control effect as the chemical agent fluopyram, but J211 promoted the growth of lateral roots of tobacco more obviously (Figure 3C). PGPR has been described as being able to fortify plant development by regulating plant hormones, such as auxins, gibberellins, cytokinin, and ethylene (Tahir et al., 2017). Auxin is a phytohormone that regulates most plant processes. Though endogenous auxin synthesis occurs in plants, it also depends on the external supply of auxin which can be fulfilled by PGPR isolates that occur in the rhizosphere of the plant (Patten and Glick, 2002). Islam et al. (2015) reported that cucumber rhizosphere isolates, including Pseudomonas stutzeri, Bacillus subtilis, Stenotrophomonas maltophilia, and Bacillus amyloliquefaciens, produced IAA levels as high as 26.78−51.28 mg L−1. Treatment of these PGPR strains significantly promoted cucumber growth. In addition, the B. arboris CSRS12 isolated by Singh et al. (2020) promoted mung bean (Vigna radiata) lateral root growth by solubilizing phosphate and siderophores. This is different from the underlying mechanism that B. arboris J211 promotes tobacco plant growth through high-yield IAA observed in this study.
Unlike chemical nematicides, bacterial agents probably create no harm to humans, animals, and the environment (Rahman et al., 2018; Vurukonda et al., 2018). J211 can be safely and continuously used during crop cultivation to control nematodes and has excellent IAA producing activity, which significantly promoted the growth of tobacco plants and improved the yield of flue-cured tobacco. PGPR produces bioactive substances in the rhizosphere to resist pathogens and promote plant growth. PGPR have a distinct advantage over the obligate nematode parasite Pasteuria penetrans as potential biocontrol agents because they can establish in the rhizosphere independently of the nematode population. In conclusion, the utilization of PGPR is treated as an environmentally sound and promising method for the management of RKNs and increases crop yields through both direct and indirect mechanisms (Lugtenberg and Kamilova, 2009; Vejan et al., 2016).
Conclusion
In this study, the strain of Burkholderia arborisJ211 was isolated from the rhizosphere soil of tobacco plants. The nematicidal assay test demonstrated the high nematicidal activity of J211 fermentation metabolites within 24 h. Inoculation with J211 significantly reduced infestation of tobacco roots by M. incognita in pot and field experiments. Moreover, J211 is a high-yielding strain of IAA, and the yield of tobacco plants treated with J211 was significantly increased. It is, however, necessary to find how J211 acts against RKNs, and the active substance involved, to develop a potential bionematicide from this isolate.
Data Availability Statement
The raw data supporting the conclusions of this article will be made available by the authors, without undue reservation.
Author Contributions
YC and SC designed the experiments. RZ and JO designed and carried out the experiments and wrote the manuscript. XX, JL, JS, and DZ participated and analyzed the data from the experiments. MR, GD, SF, and RS reviewed the manuscript. All authors contributed to the article and approved the submitted version.
Funding
This work was financially supported by the Key Special Project of Science and Technology of the Yunnan Branch of China National Tobacco Corporation (Contract No. 2021530000241031).
Conflict of Interest
JO, XX, and JL are the employers of Yunnan Tobacco Company.
The remaining authors declare that this research was conducted in the absence of any commercial or financial relationships that could be construed as a potential conflict of interest.
Publisher's Note
All claims expressed in this article are solely those of the authors and do not necessarily represent those of their affiliated organizations, or those of the publisher, the editors and the reviewers. Any product that may be evaluated in this article, or claim that may be made by its manufacturer, is not guaranteed or endorsed by the publisher.
References
Abbasi, M. W., Ahmed, N., Zaki, M. J., Shuakat, S. S., and Khan, D. (2013). Potential of Bacillus species against Meloidogyne javanica parasitizing eggplant (Solanum melongena L.) and induced biochemical changes. Plant Soil 375, 159–173. doi: 10.1007/s11104-013-1931-6
Abbott, W. S. (1925). A method of computing the effectiveness of an insecticide. http://www.letpub.com.cn/index.php?journalid=4433&page=journalapp&view=detail J. Econ. Entomol. 18, 265–267.
Akhyani, A., Modjtahedi, H., and Naderi, A. (1984). Species and physiological races of root-knot nematodes in Iran. Iran. J. Plant Pathol. 20, 57–70.
Alexander, D. B., and Zuberer, D. A. (1991). Use of chrome azurol S reagents to evaluate siderophore production by rhizosphere bacteria. Biol. Fert. Soils 12, 39–45. doi: 10.1007/BF00369386
Ali, N. I., Siddiqui, I. A., Shaukat, S. S., and Zaki, M. J. (2002). Nematicidal activity of some strains of Pseudomonas spp. Soil Biol. Biochem. 34, 1051–1058. doi: 10.1016/S0038-0717(02)00029-9
Bagheri, N., Ahmadzadeh, M., and Heydari, R. (2014). Effects of Pseudomonas fluorescens strain utpf5 on the mobility, mortality and hatching of root-knot nematode Meloidogyne javanica. Arch. Phytopathol. Plant Prot. 47, 744–752. doi: 10.1080/03235408.2013.820868
Baidoo, R., Mengistu, T., Mcsorley, R., Stamps, R. H., and Crow, W. T. (2017). Management of root-knot nematode (Meloidogyne incognita) on Pittosporum tobira under greenhouse, field, and on-farm conditions in Florida. J. Nematol. 49, 133–139. doi: 10.21307/jofnem-2017-057
Barker, K. R., Townshend, J. L., Bird, G. W., Thomason, I. J., and Dickson, D. W. (1986). “Determining nematode population responses to control agents,” in Methods for Evaluating Pesticides for Control of Plant Pathogens, ed Hickey, K. D. (St. Paul, MN: APS Press), p. 283–296.
Cetintas, R., Kusek, M., and Fateh, S. A. (2018). Effect of some plant growth-promoting rhizobacteria strains on root-knot nematode, Meloidogyne incognita, on tomatoes. Egypt. J. Biol. Pest Control 28, 7. doi: 10.1186/s41938-017-0008-x
Chelinho, S., Maleita, C. M. N., Francisco, R., Braga, M. E. M., Cunha, M. J. M. D. D., Abrantes, I., et al. (2017). Toxicity of the bionematicide 1,4-naphthoquinone on non-target soil organisms. Chemosphere 181, 579–588. doi: 10.1016/j.chemosphere.2017.04.092
Chen, S. Y., and Dickson, D. W. (2000). A technique for determining live second-stage juveniles of Heteroderaglycines. J. Nematol. 32, 117–121.
Cheng, W., Yang, J., Nie, Q., Huang, D., Yu, C., Zheng, L., et al. (2017). Volatile organic compounds from Paenibacillus polymyxa KM2501-1 control Meloidogyne incognita by multiple strategies. Sci. Rep. 7, 16213. doi: 10.1038/s41598-017-16631-8
Dickson, D. W., and Struble, F. B. (1965). A sieving-staining technique for extraction of eggmasses of Meloidogyne incognita from soil. Phytopathology 55, 497.
Du, B., Xu, Y., Dong, H., Li, Y., and Wang, J. (2020). Phanerochaete chrysosporiumstrain B-22, a nematophagous fungus parasitizing Meloidogyne incognita. PLoS One 15, e0216688. doi: 10.1371/journal.pone.0216688
El-Aal, E. M. A., Shahen, M., Sayed, S., Kesba, H., Ansari, M. J., El-Ashry, R. M., et al. (2021). In vivo and in vitro management of meloidogyne incognita (tylenchida: heteroderidae) using rhizosphere bacteria, pseudomonas spp. and Serratia spp. compared with oxamyl. Saudi J. Biol. Sci. 28, 4876–4883. doi: 10.1016/j.sjbs.2021.06.078
Elling, A. A. (2013). Major emerging problems with minor Meloidogyne species. Phytopathology 103, 1092–1102. doi: 10.1094/PHYTO-01-13-0019-RVW
Gordon, S. A., and Weber, R. P. (1951). Colorimetric estimation of inodole3-acetic acid. Plant Physiol. 26, 192–195. doi: 10.1104/pp.26.1.192
Gray, E. J., and Smith, D. L. (2005). Intracellular and extracellular PGPR: commonalities and distinctions in the plant-bacterium signaling processes. http://www.letpub.com.cn/index.php?journalid=7547&page=journalapp&view=detail Soil Biol. Biochem. 37, 395–412. doi: 10.1016/j.soilbio.2004.08.030
Groover, W., Held, D., Lawrence, K., and Carson, K. (2020). Plant growth-promoting rhizobacteria: a novel management strategy for Meloidogyne incognita on turf grass. Pest Manag. Sci. 76, 3127–3138. doi: 10.1002/ps.5867
Holt, J. G., Krieg, N. R., Sneath, P., Staley, J., and Williams, S. (1994). Bergey's Manual of Determinative Bacteriology. Baltimore: Williams and Wilkins. p. 7879.
Hu, Y., Li, J., Li, J., Zhang, F., Wang, J., Mo, M., et al. (2018). Biocontrol efficacy of Pseudoxanthomonas japonensis against Meloidogyne incognita and its nematostatic metabolites. FEMS microbiol. Lett. 19, 287. doi: 10.1093/femsle/fny287
Huang, Y., Ma, L., Fang, D. H., Xi, J. Q., Zhu, M. L., Mo, M. H., et al. (2014). Isolation and characterisation of rhizosphere bacteria active against Meloidogyne incognita, Phytophthora nicotianae and the root knot-black shank complex in tobacco. Pest Manag. Sci. 71, 415–422. doi: 10.1002/ps.3820
Hussey, R. S., and Barker, K. R. (1973). A comparison of methods collecting inocula of Meloidogyne spp. including a new technique. Plant Dis. Rep. 57, 1025–1028. doi: 10.1007/BF00010711
Isaac, G. S., Elderiny, M. M., and Taha, R. G. (2021). Efficacy of Purpureocillium lilacinum AUMC 10149 as biocontrol agent against root-knot nematode Meloidogyne incognita infecting tomato plant. Braz. J. Biol. 84, e253451. doi: 10.1590/1519-6984.253451
Islam, S., Akanda, A. M., Prova, A., Islam, M. T., and Hossain, M. M. (2015). Isolation and identification of plant growth promoting rhizobacteria from cucumber rhizosphere and their effect on plant growth promotion and disease suppression. Front. Microbiol. 6, 1360. doi: 10.3389/fmicb.2015.01360
Jones, J. T., Haegeman, A., Danchin, E. G. J., Guar, H. S., Helder, J., Jones, M. G. K., et al. (2013). Top 10 plant parasitic nematodes in molecular plant pathology. http://www.letpub.com.cn/index.php?journalid=5983&page=journalapp&view=detail Mol. Plant Pathol. 14, 946–961. doi: 10.1111/mpp.12057
Karavina, C., and Mandumbu, R. (2012). Phytoparasitic nematode management post-methyl bromide: Where to for Zimbabwe? J. Agr. Technol. 8, 1141–1160.
Kariuki, G. M., and Dickson, D. W. (2007). Transfer and development of Pasteuria penetrans. J. Nematol. 39, 55–61.
Khanna, K., Sharma, A., Ohri, P., Bhardwaj, R., Allah, E. F. A., Hashem, A., et al. (2019). Impact of plant growth promoting rhizobacteria in the orchestration of Lycopersicon esculentum Mill. resistance to plant parasitic nematodes: a metabolomic approach to evaluate defense responses under field conditions. Biomolecules 9, 676. doi: 10.3390/biom9110676
Kiewnick, S., and Sikora, R. A. (2003). Efficacy of Paecilomyces lilacinus (strain 251) for the control of root-knot nematodes. Commun. Agric. Appl. Biol. Sci. 68, 123–128.
Kimura, M. A. (1980). simple method for estimating evolutionary rates of base substitutions through comparative studies of nucleotide sequences. http://www.letpub.com.cn/index.php?journalid=4842&page=journalapp&view=detail J. Mol. Evol. 16,111–120. doi: 10.1007/BF01731581
Köthe, M., Antl, M., Huber, B., Stoecker, K., and Eberl, L. (2003). Killing of Caenorhabditis elegans by Burkholderia cepacia is controlled by the cep quorum-sensing system. Cell. Microbiol. 5, 343–351. doi: 10.1046/j.1462-5822.2003.00280.x
Liu, G., lin, X., Xu, S., Liu, G., Liu, F., and Mu, W (2020). Screening, identification and application of soil bacteria with nematicidal activity against root knot nematode (Meloidogyne incognita) on tomato. Pest Manag. Sci. 76, 2217–2224. doi: 10.1002/ps.5759
Lugtenberg, B., and Kamilova, F. (2009). Plant-growth-promoting rhizobacteria. http://www.letpub.com.cn/index.php?journalid=675&page=journalapp&view=detail Annu. Rev. Microbiol. 63, 541–556. doi: 10.1146/annurev.micro.62.081307.162918
Makunde, P. T., Dimbi, S., and Mahere, T. S. (2018). Leguminous crops as an alternative rotation with tobacco to control Meloidogyne javanica. J. Entomol. Nematol. 10, 1–5. doi: 10.5897/JEN2017.0192
Maurer, K. A., Zachow, C., Seefelder, S., and Berg, G. (2013). Initial steps towards biocontrol in hops: successful colonization and plant growth promotion by four bacterial biocontrol agents. Agrono. 3, 583–594. doi: 10.3390/agronomy3040583
Mendoza, A., Sikora, R. A., and Kiewnick, S. (2004). Efficacy of Paecilomyces lilacinus (strain 251) for the control of Radopholus similis in banana. Commun. Agric. Appl. Biol. Sci. 69, 365–372.
Nyczepir, A. P., and Thomas, S. H. (2009). “Current and future management strategies in intensive crop production systems,” in Root-Knot Nematode, eds Perry, R. N., Mones, M., Starr, J. (Wallingford: CAB International), p. 412–435.
Oka, Y., Shuker, S., and Tkachi, N. (2012). Systemic nematicidal activity of fluensulfone against the root knot nematode Meloidogyne incognita on pepper. Pest Manag. Sci. 68, 268–275. doi: 10.1002/ps.2256
Patten, C. L., and Glick, B. R. (2002). Role of Pseudomonas putida indole acetic acid in development of the host plant root system. Appl. Environ. Microbiol. 68, 3795–3801. doi: 10.1128/AEM.68.8.3795-3801.2002
Peng, D., Chai, L., Wang, F., Zhang, F., Ruan, L., and Sun, M. (2011). Synergistic activity between Bacillus thuringiensis Cry6aa and Cry55aa toxins against Meloidogyne incognita. Microb. Biotechnol. 4, 794–798. doi: 10.1111/j.1751-7915.2011.00295.x
Rahman, S. F. S. A., Singh, E., Pieterse, C. M. J., and Schenk, P. M. (2018). Emerging microbial biocontrol strategies for plant pathogens. Plant Sci. 267, 102–111. doi: 10.1016/j.plantsci.2017.11.012
Ralmi, N. H. A. A., Khandaker, M. M., and Nashriyah, M. (2016). Occurrence and control of root knot nematode in crops: a review. Aust. J. Crop Sci. 10, 1649–1654.
Sadeghi, L., Jamali, S., Naderpour, M., Asareh, M. H., Lahiji, H. S., and Naghavi, M. R. (2021). Reactions of selected bean cultivars and accessions to Iranian populations of Meloidogyne javanica and race of M. incognita. Crop Prot. 140, 105433. doi: 10.1016/j.cropro.2020.105433
Saharan, B. S., and Nehra, V. (2011). Plant growth promoting rhizobacteria: a critical review. Life Sci. Med. Res. 21, 195–230.
Sasser, J. N. (1980). Root-knot nematodes: a global menace to crop production. Plant Dis. 64, 36–41.
Schwyn, B., and Neilands, J. B. (1987). Universal chemical assay for the detection and determination of siderophores. Anal. Biochem. 160, 47–56. doi: 10.1016/0003-2697(87)90612-9
Sharma, I. P., and Sharma, A. K. (2016). Physiological and biochemical changes in tomato cultivar PT-3 with dual inoculation of mycorrhiza and PGPR against root-knot nematode. Symbiosis 71, 175–183. doi: 10.1007/s13199-016-0423-x
Sharma, N., Khanna, K., Manhas, R. K., Bhardwaj, R., Ohri, P., Alkahtani, J., et al. (2020). Insights into the role of Streptomyces hydrogenans as the plant growth promoter, photosynthetic pigment enhancer and biocontrol agent against Meloidogyne incognita in Solanum lycopersicum Seedlings. Plant (Basel) 9, 1109. doi: 10.3390/plants9091109
Siddiqui, Z. A., and Futai, K. (2009). Biocontrol of Meloidogyne incognita on tomato using antagonistic fungi, plant growth promoting rhizobacteria and cattle manure. Pest Manag. Sci. 65, 943–948. doi: 10.1002/ps.1777
Singh, T. B., Sahai, V., Goyal, D., Prasad, M., Yadav, A., Shrivastav, P., et al. (2020). Identification, characterization and evaluation of multifaceted traits of plant growth promoting rhizobacteria from soil for sustainable approach to agriculture. Curr. Microbiol. 77, 3633–3642. doi: 10.1007/s00284-020-02165-2
Sun, X., Zhang, R., Ding, M., Liu, Y., and Li, L. (2021). Biocontrol of the root-knot nematode Meloidogyne incognita by a nematicidal bacterium Pseudomonas simiae MB751 with cyclic dipeptide. Pest Manag. Sci. 77, 4365–4374. doi: 10.1002/ps.6470
Tahir, H. A. S., Gu, Q., Wu, H., Raza, W., Safdar, A., Huang, Z., et al. (2017). Effect of volatile compounds produced by Ralstonia solanacearum on plant growth promoting and systemic resistance inducing potential of Bacillus volatiles. BMC Plant Biol. 17, 133. doi: 10.1186/s12870-017-1083-6
Tang, Y. W., and Bonner, J. (1948). The enzymatic inactivation of indole acetic acid. II. The physiology of the enzyme. Am. J. Bot. 35, 570–578. doi: 10.2307/2438053
Topalovi, O., Elhady, A., Hallmann, J., Richert-Pggeler, K. R., and Heuer, H. (2019). Bacteria isolated from the cuticle of plant-parasitic nematodes attached to and antagonized the root-knot nematode Meloidogyne hapla. Sci. Rep. 9, 11477. doi: 10.1038/s41598-019-47942-7
Vejan, P., Abdullah, R., Khadiran, T., Ismail, S., and Boyce, A. N. (2016). Role of plant growth promoting rhizobacteria in agricultural sustainability-a review. Molecules 21, 573. doi: 10.3390/molecules21050573
Vurukonda, S. S. K. P., Giovanardi, D., and Stefani, E. (2018). Plant growth promoting and biocontrol activity of Streptomyces spp. as endophytes. http://www.letpub.com.cn/index.php?journalid=3812&page=journalapp&view=detail Int. J. Mol. Sci. 19, 952. doi: 10.3390/ijms19040952
Williamson, V. M. (1999). Plant nematode resistance genes. Curr. Opin. Plant Biol. 2, 327–331. doi: 10.1016/S1369-5266(99)80057-0
Wu, F., Wan, J. H. C., Wu, S., and Wong, M. (2012). Effects of earthworms and plant growth-promoting rhizobacteria (PGPR) on availability of nitrogen, phosphorus, and potassium in soil. J Plant Nutr. Soil Sci. 175, 423–433. doi: 10.1002/jpln.201100022
Xiang, N., Lawrence, K. S., Kloepper, J. W., Donald, P. A., Mcinroy, J. A., and Lawrence, G. W. (2017). Biological control of Meloidogyne incognita by spore-forming plant growth-promoting rhizobacteria on cotton. Plant Dis. 101, 774–784. doi: 10.1094/PDIS-09-16-1369-RE
Yin, N., Zhao, J., Liu, R., Li, Y., Ling, J., Yang, Y., et al. (2021). Biocontrol efficacy of Bacillus cereus strain Bc-cm103 against Meloidogyne incognita. Plant Dis. 105, 2061–2070. doi: 10.1094/PDIS-03-20-0648-RE
Yu, Z., Xiong, J., Zhou, Q., Luo, H., Hu, S., Xia, L., et al. (2015). The diverse nematicidal properties and biocontrol efficacy of Bacillus thuringiensis Cry6A against the root-knot nematode Meloidogyne hapla. J. Invertebr. Pathol. 125, 73–80. doi: 10.1016/j.jip.2014.12.011
Zhai, Y., Shao, Z., Cai, M., Zheng, L., Li, G., Yu, Z., et al. (2019). Cyclo (l-Pro–l-Leu) of Pseudomonas putida MCCC 1A00316 isolated from antarctic soil: identification and characterization of activity against Meloidogyne incognita. Molecules 24, 768. doi: 10.3390/molecules24040768
Keywords: nematode, Meloidogyne incognita, plant growth-promoting rhizosphere (PGPR), biocontrol, tobacco
Citation: Zhang R, Ouyang J, Xu X, Li J, Rehman M, Deng G, Shu J, Zhao D, Chen S, Sayyed RZ, Fahad S and Chen Y (2022) Nematicidal Activity of Burkholderia arboris J211 Against Meloidogyne incognita on Tobacco. Front. Microbiol. 13:915546. doi: 10.3389/fmicb.2022.915546
Received: 08 April 2022; Accepted: 11 May 2022;
Published: 10 June 2022.
Edited by:
Anukool Vaishnav, Agroscope, SwitzerlandReviewed by:
Sushil K. Sharma, National Institute of Biotic Stress Management, IndiaShailendra Singh, National Bureau of Agriculturally Important Microorganisms (ICAR), India
Udai B. Singh, National Bureau of Agriculturally Important Microorganisms (ICAR), India
Copyright © 2022 Zhang, Ouyang, Xu, Li, Rehman, Deng, Shu, Zhao, Chen, Sayyed, Fahad and Chen. This is an open-access article distributed under the terms of the Creative Commons Attribution License (CC BY). The use, distribution or reproduction in other forums is permitted, provided the original author(s) and the copyright owner(s) are credited and that the original publication in this journal is cited, in accordance with accepted academic practice. No use, distribution or reproduction is permitted which does not comply with these terms.
*Correspondence: Suiyun Chen, chensuiyun@ynu.edu.cn; Shah Fahad, shah_fahad80@yahoo.com; Yaqiong Chen, 312547613@qq.com
†These authors have contributed equally to this work