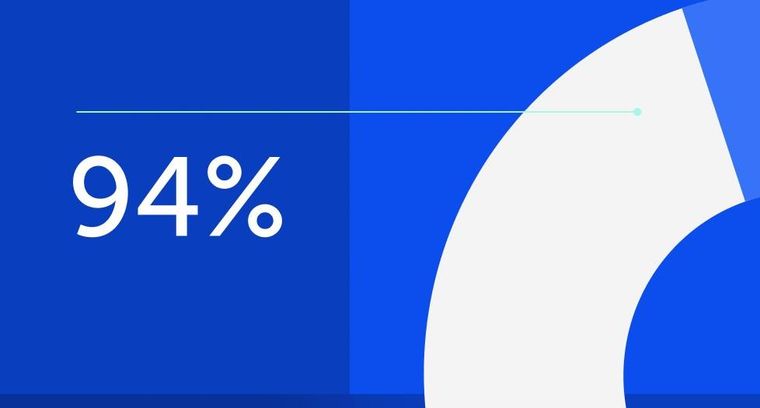
94% of researchers rate our articles as excellent or good
Learn more about the work of our research integrity team to safeguard the quality of each article we publish.
Find out more
ORIGINAL RESEARCH article
Front. Microbiol., 09 June 2022
Sec. Terrestrial Microbiology
Volume 13 - 2022 | https://doi.org/10.3389/fmicb.2022.914627
Arbuscular mycorrhizal (AM) fungi have promising applications in low-quality farmlands all over the world, but research on their responses to conventional and organic farming systems in low-quality soil is limited. We hypothesized that the colonization activity and community diversity of AM fungi in conventional farming systems may not be lower than in organic farming on low-quality farmlands where beneficial symbiosis is required. We collected soil and maize root samples from medium to low fertility farmlands with conventional or organic farming systems in western Jilin Province, China. The colonization percentage and intensity, taxonomic and phylogenetic diversity, community composition of soil AM fungi, and soil factors were detected and compared between the two farming systems. The colonization intensity and operational taxonomic unit (OTU) taxonomic diversity on conventional farms were higher than on organic farms. Glomus was the most common genus on conventional farms, whereas Paraglomus and Glomus were the most common on organic farms. We also found a simpler AM fungal network structure with lower OTU phylogenetic diversity on conventional farms. Our findings suggested that though the conventional farming system resulted in different compositions and simpler structures of soil AM fungal community, there are potential diverse OTU resources currently present on conventional farms. This work has potential impacts on understanding the influence of different farming systems on soil AM fungi in low-quality farmlands and the development of efficient mycorrhizal inoculant production.
Soil biology is a key determinant of the effectiveness of soil nutrient resources and plays an important role in sustainable agriculture (Bender and van der Heijden, 2015). Arbuscular mycorrhizal (AM) fungi are of interest for their contribution to nutrient uptake by plants (Govindarajulu et al., 2005; Davison et al., 2015), especially in nutrient supply deficit or environmental adversities (Akiyama et al., 2005; Begum et al., 2019). Compared to other types of ecosystems, farmland usually has a higher soil nutrient content, but still requires large amounts of fertilizer inputs each year for high yields. Higher soil nutrients can weaken the mutually beneficial symbiosis formed between AM fungi and plant roots (Scullion et al., 1998; Liu et al., 2019) and decrease the colonization intensity and diversity of AM fungi (van Geel et al., 2017; Zeng et al., 2021). Therefore, several previous studies have shown that AM fungi have limited benefits for agricultural production, especially in high-yielding farmlands (Ryan and Graham, 2002, 2018). However, AM fungi are still potentially important for the large areas of low-quality agricultural land that are widely distributed worldwide. The adverse environmental conditions, such as drought or salinity, usually occur on low-quality lands (Bi et al., 2018), while mutualistic symbiosis can enhance plant growth by improving soil nutrient and water use efficiency (Begum et al., 2019). Since AM fungi have promising applications in low-quality farmland, related studies in low-quality soil conditions, such as the effects of conventional and organic farming systems on AM fungal communities, should receive more attention (Sale et al., 2015; Roy et al., 2017).
Conventional agriculture is a farming system commonly practiced throughout the world that uses large quantities of chemical fertilizers, biocides, high-yielding crop varieties, tillage, and managed irrigated systems to maximize crop yields. In practice, the application rate of chemical fertilizers has met and sometimes exceeded the needs of crop growth (Tang et al., 2008). Long-term application of chemical fertilizers can reduce spore density (Wu et al., 2011; Lin et al., 2020), species richness (Sheng et al., 2013), diversity (Oehl et al., 2004), and alter the community structure of AM fungi in agriculture soils (He et al., 2016). On the contrary, organic farming using organic amendments such as animal manure has grown rapidly in the last few decades due to the growing problems of chemical fertilizers damage to soil organisms, nutrient disorders, and environmental pollution (Yue et al., 2016). The high organic carbon content in organic amendments can promote the mycelial proliferation of AM fungi (Hart and Reader, 2002), thus increasing the inoculation capacity of AM fungi on plant roots. Therefore, some studies found that colonization activity (Qin et al., 2015; Jiang Y. J. et al., 2020) and AM fungal diversity (Bhadalung et al., 2005; Lin et al., 2012) were higher on organic farms than on conventional farms. However, we hypothesize that these effects of conventional and organic farming systems on soil AM fungi may be different in low-quality farmlands for the following reasons.
Firstly, the nutrient concentrations and release rates of chemical fertilizers applied in conventional farming systems differ from those of organic amendments applied in organic farming (Niedziński et al., 2021). When chemical fertilizers are added to the soil, AM fungi may be suppressed because the environmental nutrient content is increased by the rapid release of high nutrient concentration (Xiang et al., 2016). And the increased nutrient content in the soils in response to fertilization also reduces the mycorrhizal dependency of the crop plants. However, when soil nutrients of low-quality farmlands fall back to their original levels due to uptake by plants or loss by leaching, plant-AM fungal symbiosis may again become established widely on conventional farms. Unlike chemical fertilizers, the relatively low nutrient concentrations and slow-release rates of organic amendments applied on organic farms do not appear to inhibit the inoculation of plant roots by AM fungi. However, the negative effects of sustained nutrient release on the symbiosis should not be overlooked.
Secondly, because chemical fertilizers contain almost only one form of the nutrient, for example, the same chemical form of N, P, or K regardless of brand and manufacturer, it creates a stable environmental filter for soil organisms year after year. Therefore, it is widely believed that AM fungal diversity on conventional farms subsequently declines as some species, genera, or families are filtered out (Lin et al., 2012). However, due to polymorphism of ribosomal DNA (heterokaryosis) in individual spores of AM fungi (Pawlowska and Taylor, 2004), high genetic diversity has been found in spores and extrametrical hyphae (Bever and Wang, 2005; Tisserant et al., 2013). This suggests that even though the diversity of families, genera, or species declines, the diversity of smaller taxonomic units, such as operational taxonomic unit (OTU), may not (Rosendahl, 2008). Recent studies using high-throughput sequencing methods have also confirmed high OTU diversity in AM fungal communities in conventional farm soil (Chen et al., 2014).
Based on these two reasons, we hypothesized that the colonization activity and OTU diversity of AM fungal community in conventional farming systems will not necessarily be lower than in organic farming, especially on low-quality farmlands where beneficial symbiosis is required. To test our hypothesis, we collected soil and maize root samples from low-quality (medium-low grade farmlands in China, please see details below) maize farms in western Jilin Province, China, under conventional and organic farming management, respectively. Firstly, we assessed whether soil AM fungi activities (percentage and intensity of colonization to roots and spore density in the soil) were lower on conventional farms than on organic farms, as is commonly believed. Genus composition, taxonomy, and phylogenetic OTU diversity indices of AM fungal communities were then compared to determine whether conventional farming systems decreased AM fungal diversity on low-quality farmlands. Community composition is also a major factor influencing community function. Therefore, we created OTU networks of AM fungal communities and analyzed them with structural parameters to determine the composition of AM fungal communities on conventional and organic farms, and whether there were significant differences in their compositional characteristics. Finally, we analyzed whether the activity, diversity, and community composition of AM fungi were related to soil factors and the relative influence of each factor. This study contributes to the understanding of the effects of conventional and organic farming systems on the soil AM fungi in low-quality farmlands and promotes the rational application of AM fungal fertilizers in agro-ecosystems to restore and maintain the ecological functions of farm soils.
Jilin Province is a major maize producing area in China and belongs to the temperate maize belt of the world. This study was conducted in the semi-arid plain of western Jilin Province (Figure 1). The area has a temperate continental monsoon climate with dry springs, an average annual temperature of 4–6°C, and average annual precipitation of 350–500 mm, with precipitation concentrated in July and August (Li et al., 2005). The soil is sandy loam (Typic Hapludolls) with high salinity and low soil nutrient content (Zhu, 1994; Yang et al., 2014). According to the nutrient grading criteria of the second soil census in China (Shi et al., 2004; Yang et al., 2014), the soil fertility grades of the farmlands at our survey sites were all medium-low. After 2 years of field trips from 2019 to 2020, fifteen sites in six counties were finally identified as sampling areas for this study (Supplementary Table 1). Each site had both conventional and organic farmlands planted with maize, i.e., large areas of conventional farmland and small areas of organically farmed maize fields. A pair of sampling areas, one conventional (Con) maize field and one organic (Org) maize field, were randomly selected at each site, away from the road. Thus, a total of 15 sites with both farming systems were included in this study, for a total of 30 sampling areas.
All the conventional farmlands we investigated are under a continuous maize monoculture cropping system. In the past 4 years, the conventional tillage (rotary plowing and residue removal) in the study area was gradually replaced by conservation tillage (ridge tillage and residue retention) as recommended by the government (The Ministry of Agriculture and Rural Affairs of China, 2020). Chemical compound fertilizers and herbicides were used in conventional farmlands every year. The fertilizer applied in organic farming is generally based on animal manure and no herbicides were applied. Weeds were controlled manually in organic farmlands at the beginning of the growing season and then suppressed by the canopy of maize. Organic farmlands have been under organic farming for at least 5 years, and some of them are more than 30 years (Supplementary Table 1). According to our field survey, the maize varieties planted on conventional and organic farmlands not only varied from place to place but also from year to year. Basically, the type of corn on organic farms is waxy corn while dent corn is common on conventional farms in our sampling area.
All soil and root samples from the maize fields were collected in late August 2020 (reproductive stage of maize). Three sampling spots were randomly selected in each sampling field, with a distance of about 5–10 m between each sampling spot. After removing approximately 2 cm of topsoil, a soil volume of 20 cm × 20 cm × 30 cm was dug at a horizontal distance of around 15 cm from the maize stalk, and the fine roots of maize were picked out. The soil from the three sampling spots was mixed thoroughly, and then about 2 kg of fresh soil was randomly collected and stored in plastic sealed bags (soil sample I). About 10 g of fresh soil was randomly selected into plastic sealed bags and placed in an icebox (soil sample II). Fine maize roots from the three sampling spots were mixed and stored in plastic bottles containing formalin–acetic acid–alcohol (FAA) fixative. All soil and root samples were then transported back to the laboratory. Impurities such as plant and animal residues were removed from soil sample I and air-dried indoors to measure AM spore density and soil parameters. Soil sample II was stored in a refrigerator at −80°C for 3 days and then sent to Majorbio Co., Ltd., for DNA extraction and sequencing of AM fungi. FAA-fixed roots were used to measure the percentage and intensity of AM fungal colonization.
The soil sample I was air-dried and sieved through a 40-mesh sieve (<0.425 mm). Soil pH was measured in a soil-water slurry (1:5, w/v) using a pH meter (Mettler-Toledo pH reader, Switzerland). The potassium dichromate method was used to determine the organic carbon content of the soil (Lu, 1999). The soil’s total nitrogen content was determined using the Kjeldahl method (Lu, 1999). The soil hydrolysable nitrogen was measured by alkaline hydrolysis diffusion (Lu, 1999). The total and available phosphorus contents were determined spectrophotometrically by a continuous flow analyzer (SAN + +, Skalar, Breda, Holland).
The FAA-fixed roots were washed with 10% KOH solution at 90°C for 60 min, acidified with 1% HCl for 30 min, and then cut into approximately 1 cm root segments. The root segments were soaked in 0.05% (v) trypan blue solution and then placed on a hot plate at 90°C for 30 min (Koske and Gemma, 1989). The stained roots were mounted on microscope slides for assessment of AMF colonization following the method of Trouvelot et al. (1986). The percentage of colonization (frequency of the mycorrhiza in the root system) and colonization intensity (intensity of the mycorrhizal colonization in the root system) were then calculated (Trouvelot et al., 1986).
AMF spores were isolated from 20 g of randomly divided dry soil sample I by wet sieving and gradient centrifugation (Gerdemann and Nicolson, 1963). The spore density (total number of spores in 20 g of dry soil) was determined by the number of spores counted under a stereomicroscope (Daniels and Skipper, 1982). Only spores with complete shape structure were selected for counting.
The total DNA of 0.5 g soil samples was extracted according to the manufacturer’s instruction using a E.Z.N.A.® soil (Omega Bio-Tek, Norcross, GA, United States). The primers AML1F/AML2R were used to amplify the V4-V5 hypervariable regions of the fungal 18S rRNA gene in a thermocycler PCR system (Axygen Biosciences, Union City, CA, United States). The second amplification used the AMF specific primer set AMV4.5NF/AMDGR. The PCR reactions were conducted using the following program: 3 min of denaturation at 95°C, 27 cycles of 30 s at 95°C, 30 s for annealing at 55°C, and 45 s for elongation at 72°C, and a final extension at 72°C for 10 min. PCR reactions were performed in a triplicate 20 μL mixture containing 4 μL of 5 × FastPfu Buffer, 2 μL of 2.5 mM dNTPs, 0.8 μL of each primer (5 μM), 0.4 μL of FastPfu Polymerase and 10 ng of template DNA.
Raw FASTQ files were demultiplexed, quality-filtered by Trimmomatic, and merged by FLASH with the following criteria: (i) The reads were truncated at any site receiving an average quality score < 20 over a 50 bp sliding window. (ii) Primers were exactly matched allowing 2 nucleotide mismatching and reads containing ambiguous bases were removed. (iii) Sequences whose overlap was longer than 10 bp were merged according to their overlap sequence. Operational taxonomic units (OTUs) were clustered with a 97% similarity cutoff using UPARSE (version 7.11) and chimeric sequences were identified and removed using UCHIME. The taxonomic identities of the representative sequences were checked against the Maarjam081 AMF database online (2 Accession to cite for these SRA data: PRJNA819886).
Four OTU diversity indices of AM fungal communities were calculated, including the Chao1 index, the Shannon diversity index, the Simpson evenness index, and the phylogenetic diversity index (Caporaso et al., 2010). The conventional and organic farms are matched sample pairs, therefore paired t-tests were performed using SPSS 25.0 software (IBM) to analyze whether soil parameters, mycorrhizal colonization, spore density, and diversity indices of AM fungi differed between conventional and organic farming systems. Normality and homogeneity of the distribution of residuals were verified and log or arcsine transformations were performed when necessary.
To detect differences in soil AM fungal community structure between conventional and organic maize farms, non-metric multidimensional scaling (NMDS) was performed using the R package “vegan” based on Bray–Curtis dissimilarities of the compositional data matrix. Differences in Bray-Curtis dissimilarity between the two farming systems were also Monte Carlo permutation tested for significance.
Using the “SpiecEasi” package in R software, OTU network maps of soil AM fungi from conventional and organic farming maize fields were constructed separately. Several parameters characterizing the networks were calculated. OTUs with relative abundance > 0.05% were selected for SparCC correlation analysis, and correlation networks were constructed by screening for significant (P < 0.01) and strong (| r| > 0.3) correlations (Kim et al., 2020). Network visualization was performed on the Gephi (v.0.9.2) platform3 and colored by family classification.
To investigate whether AM fungal colonization, spore density, and diversity indices were related to soil factors, correlation heat maps were drawn in two farming systems based on the Spearman correlation coefficients between five soil factors (pH, contents of organic matter, total and available nitrogen, total and available phosphorus) and the above indicators. Using the “gbmplus” package (R v.3.6.3) with 500 trees for boosting (De’ath, 2007), an Aggregated Boosted Tree (ABT) analysis was conducted to quantify the effect of five soil factors on the OTUs composition of AM fungal communities.
The percentage of plant root colonization was less variable (86.67–100%), but colonization intensity (1.86–44.5%) and spore density (25–103 spores/20 g soil) were highly variable across all sampling areas including conventional and organic farms (Figure 2). Percentage of colonization (t = 1.733, P = 0.105) and spore density (t = −0.822, P = 0.425) were not different between the two farming systems (Figures 2A,C), but colonization intensity was different (t = 2.159, P = 0.049, Figure 2B).
Figure 2. The percentage of colonization (A), colonization intensity (B) of roots, and soil spore density (C) on conventional (Con) and organic (Org) maize farms. The * represents the difference between two farming systems is significant at P = 0.05 level.
A total of 171 OTUs of AM fungi were detected in all samples, of which 107 and 125 OTUs were detected on conventional and organic farms, respectively. The diversity of soil AM fungal community was different between the two farming systems. The OTU Shannon diversity and Chao1 index of AM fungal communities were higher on conventional farms than on organic farms (Figures 3A,C), while the difference in Simpson evenness index was not significant (Figure 3B). The OTU phylogenetic diversity index (PD) was lower for AM fungal communities on conventional farms than on organic farms (Figure 3D).
Figure 3. The OTU diversity indices of AM fungal communities on conventional (Con) and organic (Org) maize farms including Shannon diversity index (A), Simpson evenness index (B), Chao 1 index (C), and phylogenetic diversity index (D). The *** represents the difference between the two farming systems is significant at P = 0.001 level.
For maize fields in the conventional farming system, 64.79% of the AM fungi OTUs belonged to the family Glomeraceae (including 41.8% Glomus, 14.08% Septoglomus, 8.68% Funneliformis, and 0.23% Kamienskia), 13.88% to Paraglomeraceae (Paraglomus), 9.7% to Claroideoglomeraceae (Claroideoglomus), and 5.45% to Diversisporaceae (Diversispora). Other families, such as Archaeosporaceae (Archaeospora), Geosiphonaceae (Geosiphon), Gigasporaceae (Scutellospora), Ambisporaceae (Ambispora), and Diversisporaceae (Redeckera), represented less than 10% of the total OTUs (Figure 4).
Figure 4. The genus composition of soil AM fungal communities on conventional (Con) and organic (Org) maize farms.
For maize fields in the organic farming system, 54.73% of the AMF OTUs belonged to the family Glomeraceae (including 25.63% Glomus, 18.04% Septoglomus, 11.06% Funneliformis), 27.34% to Paraglomeraceae (Paraglomus). Other families (each was less than 5%), including Ambisporaceae (Ambispora), Archaeosporaceae (Archaeospora), Claroideoglomeraceae (Claroideoglomus), Diversisporaceae (Diversispora, Redeckera), Geosiphonaceae (Geosiphon), and Gigasporaceae (Scutellospora) were about 18% in total (Figure 4).
Based on the results of the non-metric multidimensional scaling analysis, Bray-Curtis dissimilarity was different between the two farming systems, indicating that the OTU composition of soil AM fungal community differed between conventional and organic farms (Figure 5).
Figure 5. Non-metric multidimensional scaling ordinations (A) of AM fungal communities on conventional (blue) and organic (yellow) farms based upon their OTU composition derived from Bray-Curtis distances (B). The *** represents the difference between the two farming systems is significant at P = 0.001 level.
We found that the soil AM fungal network on both conventional (modularity of 0.814) and organic farms (modularity of 0.724) had a modular structure (>0.4) (Table 1). The nodal OTUs with high connectivity were predominantly from the Glomeraceae and Diversisporaceae families in the conventional farming system while from the Glomeraceae and Paraglomeraceae in the organic farming system (Figure 6). Furthermore, the key taxon of the Glomeraceae family included completely different OTUs components on conventional and organic farms, respectively.
Table 1. The network attributes of AM fungal community composition on conventional (Con) and organic (Org) maize fields.
Figure 6. Network diagram of AM fungal community on conventional (A) and organic (B) maize farms. The size of each node is proportional to the number of connections (degree).
The soil AM fungal network of organic farms was more complex because it had more nodes and links, higher average connectivity, graph density, and average clustering coefficients (Table 1 and Figure 6). Some negative connections between nodes appeared in the network of conventional farms (21.59% of total links) and organic farms (12.14% of total links).
Except for soil pH, the soil organic matter, total nitrogen, total phosphorus, available nitrogen and phosphorus contents were higher on organic farms (Supplementary Table 2). In particular, the difference in soil available phosphorus content between the two farming systems was about eight-fold.
The correlations between soil factors and soil AM fungi (colonization, spore density, and diversity) were different in the two farming systems (Figure 7). In general, soil factors were correlated with AM fungal diversity on conventional farms, while they were hardly significant on organic farms. On conventional farms, all four diversity indices of soil AM fungi increased with increasing soil nutrients (especially phosphorus) content and decreasing soil pH (Figure 7A). Only the phylogenetic diversity index was negatively correlated with soil pH on organic farms (Figure 7B). In all farming systems, neither the percentage nor the intensity of root colonization was correlated with soil factors. On organic farms, the spore density was negatively correlated with total and available soil phosphorus content (Figure 7B).
Figure 7. The correlation between soil factors and indices of AM fungal communities on conventional (A) and organic (B) maize farms. The *, **, and *** represents the correlation is significant at P = 0.05, 0.01, and 0.001 level, respectively.
On both conventional and organic farms, total soil phosphorus and available phosphorus content were the most important factors affecting soil AM fungal community composition (Figure 8). In addition to phosphorus, total soil N content on conventional farms and soil pH on organic farms also influenced the community composition of AM fungi in maize fields (Figure 8).
Figure 8. The aggregated boosted tree (ABT) analysis showed the relative influence of each soil factor on AM fungal community composition on conventional (A) and organic (B) maize farms.
There is a generally accepted opinion that conventional farming systems inhibit AM fungal colonization, while organic farming systems enhance AM fungal colonization to plant roots (Cely et al., 2016; Jiang Y. J. et al., 2020). However, in the present study, we have found the opposite result in that colonization intensity was higher on conventional farms (Figure 2B). Furthermore, soil nutrients have been verified in many previous studies as the main factor influencing AM fungal colonization and reproduction (Johnson, 2010; Begum et al., 2019). But our study showed that although the soil nutrient content was higher on organic farms than on conventional farms (Supplementary Table 2), the percentage and intensity of colonization and spore density were independent of almost all the soil factors we measured (Figure 7). The different results found in this study may be due to the adaptation of the AM fungal taxa to the nutrient levels in the low-quality of soil in the area.
According to the nutrient grading criteria of the second soil census in China (Zhu, 1994; Shi et al., 2004), all soil fertility classes in the area were medium-low. Regardless of whether the input nutrients are chemical or organic fertilizers, soil nutrient content can only increase over a certain period and within a limited soil space (Cavagnaro et al., 2015; Yang et al., 2017). In this study, almost all roots sampled from conventional and organic farms were colonized by AM fungi (Figure 2A), suggesting that crop plants may rely on the cooperation of AM fungi for additional resources. This is consistent with the idea that plant-AM fungi symbiosis is easily established under low nutrient conditions (Graham and Eissenstat, 1994; Lekberg et al., 2021). In addition, the nutrient content of conventional field soils is more likely to fall back to the original low-quality level due to crop uptake of nutrients released rapidly from chemical fertilizers or nutrient loss by leaching (Bender and van der Heijden, 2015). The nutrient content of conventional field soils during a later growth season (late August) was lower than that in organic field soils, which might contribute to the increased colonization intensity on conventional farms in this study.
Therefore, background soil fertility levels, as well as other environmental factors (e.g., salinity, drought, etc.), should be considered when we assess the effects of conventional and organic farming systems on soil AM fungi (Franke-Snyder et al., 2001; Boomsma and Vyn, 2008). Because their strong effects on AM fungi may interfere with our correct understanding of the beneficial and detrimental effects of different farming systems on the ecological functions of AM fungi and/or other soil organisms.
Conventional and organic farming systems not only differently affect the establishment of the plant-AM fungi symbiosis, but also result in different diversity of soil fungal communities (Sale et al., 2015; Roy et al., 2017). It is generally accepted that the diversity of soil AM fungal communities at the family or genus level is often decreased when farmland is subjected to high levels of chemical fertilizers, herbicides, and mechanized tillage under conventional farming management (Zeng et al., 2021). Furthermore, the most common taxon of AM fungal community in such farming systems tends to be Glomus, a more disturbance-tolerant and widely distributed genus (Daniell et al., 2001; Hart and Reader, 2002). Our study is consistent with this (Figure 4). However, although fewer families and genera were found on conventional farms, their OTU diversity was higher than on organic farms (Figures 3A,C).
Soil fertility was low to medium on both conventional and organic farms (Zhu, 1994; Shi et al., 2004), but soil nutrient levels were higher on organic farms than on conventional farms, especially for available phosphorus content (Supplementary Table 2). Livestock manure is the main component of organic fertilizer in the region, which contains high phosphorus content (Liu et al., 2019). The phosphorus content is an important factor in establishing symbiosis and AM fungal diversity (Xiao et al., 2019). Usually, the increase in AM fungal diversity with increasing soil phosphorus happens in low fertile soils (Johnson et al., 2003), till a threshold level beyond which phosphorus negatively affects AM fungi (Kiers et al., 2011; He et al., 2016). Relatively adequate P availability in nutrient-poor soils may reduce plant dependence on AM fungi, which in turn may reduce carbohydrate supply to fungi from the root system (Jakobsen et al., 1992; Kiers et al., 2011). Thus, higher soil nutrient levels, especially P content, on organic farms may be one of the reasons for their lower OTU diversity.
On the other hand, since different nucleotypes carrying different genetic information may rapidly diverge (Angelard et al., 2014), the nucleotypes adapted to conventional farm soil conditions (stable soil disturbance, drought, and salinity) then subsequently accumulate and occupy the ecological niches left by those that were filtered out (long-term studies are needed to ascertain this). This allows an AM fungus to undergo rapid genotypic change by altering the relative frequencies of different nucleotypes in response to environmental change and results in high functional diversity (Munkvold et al., 2004; Kruger et al., 2012). Therefore, although only relatively few families were detected on conventional farms, the OTU diversity of AM fungi was high (Figures 3, 4). This has an important implication for mycorrhizal inoculant production and application, as the AM fungal OTUs currently present in low-quality or stressed agricultural fields may be potential and valuable resources. Compared to common commercial mycorrhizal agents, native strains screened from local environments are more effective for plant-fungi symbiosis (Chenchouni et al., 2020; Wu et al., 2021). In addition, fungal inoculants using native strains may be more beneficial in maintaining or restoring stable soil micro-food webs.
As some microbial groups are more sensitive to environmental disturbances than others (Bhadalung et al., 2005; Qin et al., 2015), not only the diversity of soil AM fungi, but also the community structure may be altered by agricultural management on conventional farmland (Bhadalung et al., 2005; Chen et al., 2014). Glomus is often considered to be the most common fungal genus in agricultural soils (Berthrong et al., 2013). Our data showed that Glomus comprised 41.8% of the AM fungal community on conventional farms, higher than the 25.63% on organic farms (Figure 4). Conventional commercial farmland is subjected to more mechanized tillage than organic farmland, and some of the reproductive characteristics of Glomus, such as the ability to reproduce not only through spores but also through mycelial fragments (Biermann and Linderman, 1983), mitigate to some extent the negative effects of frequent tillage on the survival of AM fungi. These reproductive properties and the widely known stress tolerance give Glomus an advantage in AM fungal communities that suffer from various agricultural disturbances (Hijri et al., 2006; Brito et al., 2012).
In contrast to the decreasing proportion of Glomus in soil AM fungal community, Paraglomus increased on organic farms and even became the most common genus in some sample sites (Figure 4). Several studies have found that the genus occurs mainly on organic rather than conventional agricultural soils (Gosling et al., 2014). Less disturbing agricultural management and higher soil phosphorus levels may have contributed in part to the increase of Paraglomus on organic farmland (Jiang S. et al., 2020). The dominant family in conventional and organic farming systems was the same, i.e., Glomeraceae.
Network analysis further suggested that the dominant taxon Glomeraceae was also a keystone taxon in the network structure of soil fungi, regardless of the farming system. Keystone taxon has a unique position in microbial communities and their absence or alteration has a significant impact on community structure and function (Banerjee et al., 2018). Although Glomeraceae is the key taxon of the fungal network in both farming systems, this family included completely different OTUs components on conventional and organic farms, respectively (Figure 6). This meant that the genetic composition of the dominant Glomeraceae was completely different, which may lead to different functions of the soil AM fungal community in these two farming systems. Furthermore, the conventional farm management has led to a simpler network structure of soil AM fungal communities and weaker interactions between OTU nodes. The higher percentage of negative links on conventional farms may imply more competitive interactions or niche differentiation among OTUs (Deng et al., 2016). Despite the high OTU diversity of soil AM fungal communities on conventional farms, the simpler network structure may reduce their functions and resistance to environmental changes compared to organic farms.
Comparing the differences in soil AM fungal community composition between conventional and organic farms is important for both agriculture production and restoration of degraded arable soil. We found a higher colonization intensity of maize roots by AM fungi and higher OTU taxonomic diversity on conventional farms compared to organic farms, which was contrary to what had been found. This suggests that the background soil fertility level should be considered when assessing the effects of conventional and organic farming systems on soil AM fungi. Though conventional farming systems resulted in different compositions and simpler structures of soil AM fungal community, there are potential diverse OTU resources currently present on conventional farms, which might be valuable for efficient mycorrhizal inoculant production. The AM fungal strains screened from conventional farms may be potential strains for creating more efficient biofertilizers for industrial farmland applications, while strains from organic farms may be a potential resource for degraded arable land fauna recovery or sustainable agriculture.
The datasets presented in this study can be found in online repositories. The names of the repository/repositories and accession number(s) can be found in the article/Supplementary Material.
PW and YZ contributed to the study’s conception and design. JC and YW performed the material preparation and data collection. JC, JL, and YY performed the data analyses. JC and JL wrote the first draft of the manuscript. All authors commented on previous versions of the manuscript, read, and approved the final manuscript.
This work was supported by grants from the Science and Technology Development Program of Jilin Province (20200402012NC) and the National Natural Science Foundation of China (31770475).
The authors declare that the research was conducted in the absence of any commercial or financial relationships that could be construed as a potential conflict of interest.
All claims expressed in this article are solely those of the authors and do not necessarily represent those of their affiliated organizations, or those of the publisher, the editors and the reviewers. Any product that may be evaluated in this article, or claim that may be made by its manufacturer, is not guaranteed or endorsed by the publisher.
We thank Lei Wang, Qian Xiao, and Luanwen Zhao for their help with the field and laboratory work.
The Supplementary Material for this article can be found online at: https://www.frontiersin.org/articles/10.3389/fmicb.2022.914627/full#supplementary-material
Akiyama, K., Matsuzaki, K., and Hayashi, H. (2005). Plant sesquiterpenes induce hyphal branching in arbuscular mycorrhizal fungi. Nature 435, 824–827. doi: 10.1038/nature03608
Angelard, C., Tanner, C. J., Fontanillas, P., Niculita-Hirzel, H., Masclaux, F., and Sanders, I. R. (2014). Rapid genotypic change and plasticity in arbuscular mycorrhizal fungi is caused by a host shift and enhanced by segregation. ISME J. 8, 284–294. doi: 10.1038/ismej.2013.154
Banerjee, S., Schlaeppi, K., and van der Heijden, M. G. A. (2018). Keystone taxa as drivers of microbiome structure and functioning. Nat. Rev. Microbiol. 16, 567–576. doi: 10.1038/s41579-018-0024-1
Begum, N., Qin, C., Ahanger, M. A., Raza, S., Khan, M. I., Ashraf, M., et al. (2019). Role of arbuscular mycorrhizal fungi in plant growth regulation: implications in abiotic stress tolerance. Front. Plant Sci. 10:1068. doi: 10.3389/fpls.2019.01068
Bender, S. F., and van der Heijden, M. G. A. (2015). Soil biota enhance agricultural sustainability by improving crop yield, nutrient uptake and reducing nitrogen leaching losses. J. Appl. Ecol. 52, 228–239. doi: 10.1111/1365-2664.12351
Berthrong, S. T., Buckley, D. H., and Drinkwater, L. E. (2013). Agricultural management and labile carbon additions affect soil microbial community structure and interact with carbon and nitrogen cycling. Microb. Ecol. 66, 158–170. doi: 10.1007/s00248-013-0225-0
Bever, J., and Wang, M. (2005). Hyphal fusion and multigenomic structure. Nature 433, E3–E4. doi: 10.1038/nature03294
Bhadalung, N. N., Suwanarit, A., Dell, B., Nopamornbodi, O., Thamchaipenet, A., and Rungchuang, J. (2005). Effects of long-term NP-fertilization on abundance and diversity of arbuscular mycorrhizal fungi under a maize cropping system. Plant Soil 270, 371–382. doi: 10.1007/s11104-004-1829-4
Bi, Y., Qiu, L., Zhakypbek, Y., Jiang, B., Cai, Y., and Sun, H. (2018). Combination of plastic film mulching and AMF inoculation promotes maize growth, yield and water use efficiency in the semiarid region of Northwest China. Agric. Water Manag. 201, 278–286. doi: 10.1016/j.agwat.2017.12.008
Biermann, B., and Linderman, R. (1983). Use of vesicular-arbuscular mycorrhizal roots, intraradical vesicles and extraradical vesicles as inoculum. New Phytologist 95, 97–105. doi: 10.1111/j.1469-8137.1983.tb03472.x
Boomsma, C. R., and Vyn, T. J. (2008). Maize drought tolerance: potential improvements through arbuscular mycorrhizal symbiosis? Field Crops Res. 108, 14–31. doi: 10.1016/j.fcr.2008.03.002
Brito, I., Goss, M. J., de Carvalho, M., Chatagnier, O., and van Tuinen, D. (2012). Impact of tillage system on arbuscular mycorrhiza fungal communities in the soil under Mediterranean conditions. Soil Tillage Res. 121, 63–67. doi: 10.1016/j.still.2012.01.012
Caporaso, J. G., Kuczynski, J., Stombaugh, J., Bittinger, K., Bushman, F. D., Costello, E. K., et al. (2010). QIIME allows analysis of high-throughput community sequencing data. Nat. Methods 7, 335–336. doi: 10.1038/nmeth.f.303
Cavagnaro, T. R., Bender, S. F., Asghari, H. R., and van der Heijden, M. G. A. (2015). The role of arbuscular mycorrhizas in reducing soil nutrient loss. Trends Plant Sci. 20, 283–290. doi: 10.1016/j.tplants.2015.03.004
Cely, M. V. T., de Oliveira, A. G., de Freitas, V. F., de Luca, M. B., Barazetti, A. R., dos Santos, I. M. O., et al. (2016). Inoculant of arbuscular mycorrhizal fungi (Rhizophagus clarus) increase yield of soybean and cotton under field conditions. Front. Microbiol. 7:720. doi: 10.3389/fmicb.2016.00720
Chen, Y. L., Zhang, X., Ye, J. S., Han, H. Y., Wan, S. Q., and Chen, B. D. (2014). Six-year fertilization modifies the biodiversity of arbuscular mycorrhizal fungi in a temperate steppe in Inner Mongolia. Soil Biol. Biochem. 69, 371–381. doi: 10.1016/j.soilbio.2013.11.020
Chenchouni, H., Mekahlia, M. N., and Beddiar, A. (2020). Effect of inoculation with native and commercial arbuscular mycorrhizal fungi on growth and mycorrhizal colonization of olive (Olea europaea L.). Sci. Hortic. 261:108969. doi: 10.1016/j.scienta.2019.108969
Daniell, T. J., Husband, R., Fitter, A. H., and Young, J. P. W. (2001). Molecular diversity of arbuscular mycorrhizal fungi colonising arable crops. FEMS Microbiol. Ecol. 36, 203–209. doi: 10.1016/s0168-6496(01)00134-9
Daniels, B. A., and Skipper, H. D. (1982). Methods for the Recovery and Quantitative Estimation of Propagules From Soil. St. Paul: American Phytopathological Society.
Davison, J., Moora, M., Opik, M., Adholeya, A., Ainsaar, L., Ba, A., et al. (2015). Global assessment of arbuscular mycorrhizal fungus diversity reveals very low endemism. Science 349, 970–973. doi: 10.1126/science.aab1161
De’ath, G. (2007). Boosted trees for ecological modeling and prediction. Ecology 88, 243–251. doi: 10.1890/0012-9658(2007)88[243:btfema]2.0.co;2
Deng, Y., Zhang, P., Qin, Y., Tu, Q., Yang, Y., He, Z., et al. (2016). Network succession reveals the importance of competition in response to emulsified vegetable oil amendment for uranium bioremediation. Environ. Microbiol. 18, 205–218. doi: 10.1111/1462-2920.12981
Franke-Snyder, M., Douds, D. D., Galvez, L., Phillips, J. G., Wagoner, P., Drinkwater, L., et al. (2001). Diversity of communities of arbuscular mycorrhizal (AM) fungi present in conventional versus low-input agricultural sites in eastern Pennsylvania, USA. Appl. Soil Ecol. 16, 35–48. doi: 10.1016/s0929-1393(00)00100-1
Gerdemann, J., and Nicolson, T. H. (1963). Spores of mycorrhizal Endogone species extracted from soil by wet sieving and decanting. Trans. Br. Mycol. Soc. 46, 235–244. doi: 10.1016/S0007-1536(63)80079-0
Gosling, P., Proctor, M., Jones, J., and Bending, G. D. (2014). Distribution and diversity of Paraglomus spp. in tilled agricultural soils. Mycorrhiza 24, 1–11. doi: 10.1007/s00572-013-0505-z
Govindarajulu, M., Pfeffer, P. E., Jin, H. R., Abubaker, J., Douds, D. D., Allen, J. W., et al. (2005). Nitrogen transfer in the arbuscular mycorrhizal symbiosis. Nature 435, 819–823. doi: 10.1038/nature03610
Graham, J. H., and Eissenstat, D. M. (1994). Host genotype and the formation and function of VA mycorrhizae. Plant Soil 159, 179–185. doi: 10.1007/bf00000107
Hart, M. M., and Reader, R. J. (2002). Taxonomic basis for variation in the colonization strategy of arbuscular mycorrhizal fungi. New Phytol. 153, 335–344. doi: 10.1046/j.0028-646X.2001.00312.x
He, D., Xiang, X., He, J. S., Wang, C., and Chu, H. (2016). Composition of soil fungal community is more sensitive to phosphorus than nitrogen addition in alpine meadow on the Qinghai-Tibetan Plateau. Biol. Fertil. Soils 52, 1059–1072. doi: 10.1007/s00374-016-1142-4
Hijri, I., Sýkorová, Z., Oehl, F., Ineichen, K., Mäder, P., Wiemken, A., et al. (2006). Communities of arbuscular mycorrhizal fungi in arable soils are not necessarily low in diversity. Mol. Ecol. 15, 2277–2289. doi: 10.1111/j.1365-294X.2006.02921.x
Jakobsen, J. I., Abbott, L., and Robson, A. (1992). External hyphae of vesicular-arbuscular mycorrhizal fungi associated with Trifolium subterraneum L. 1. Spread of hyphae and phosphorus inflow into roots. New Phytol. 120, 371–380. doi: 10.1111/j.1469-8137.1992.tb01077.x
Jiang, S., Hu, X., Kang, Y., Xie, C., An, X., Dong, C., et al. (2020). Arbuscular mycorrhizal fungal communities in the rhizospheric soil of litchi and mango orchards as affected by geographic distance, soil properties and manure input. App. Soil Ecol. 152:103593. doi: 10.1016/j.apsoil.2020.103593
Jiang, Y. J., Luan, L., Hu, K. J., Liu, M. Q., Chen, Z. Y., Geisen, S., et al. (2020). Trophic interactions as determinants of the arbuscular mycorrhizal fungal community updates with cascading plant-promoting consequences. Microbiome 8:142. doi: 10.1186/s40168-020-00918-6
Johnson, N. C. (2010). Resource stoichiometry elucidates the structure and function of arbuscular mycorrhizas across scales. New Phytol. 185, 631–647. doi: 10.1111/j.1469-8137.2009.03110.x
Johnson, N. C., Rowland, D. L., Corkidi, L., Egerton-Warburton, L. M., and Allen, E. B. (2003). Nitrogen enrichment alters mycorrhizal allocation at five mesic to semiarid grasslands. Ecology 84, 1895–1908.
Kiers, E. T., Duhamel, M., Beesetty, Y., Mensah, J. A., Franken, O., Verbruggen, E., et al. (2011). Reciprocal rewards stabilize cooperation in the mycorrhizal symbiosis. Science 333, 880–882. doi: 10.1126/science.1208473
Kim, H., Lee, K. K., Jeon, J., Harris, W. A., and Lee, Y. H. (2020). Domestication of Oryza species eco-evolutionarily shapes bacterial and fungal communities in rice seed. Microbiome 8, 1–17. doi: 10.1186/s40168-020-00805-0
Koske, R., and Gemma, J. (1989). A modified procedure for staining roots to detect VA mycorrhizas. Mycol. Res. 92, 486–488. doi: 10.1016/S0953-7562(89)80195-9
Kruger, M., Kruger, C., Walker, C., Stockinger, H., and Schussler, A. (2012). Phylogenetic reference data for systematics and phylotaxonomy of arbuscular mycorrhizal fungi from phylum to species level. New Phytol. 193, 970–984. doi: 10.1111/j.1469-8137.2011.03962.x
Lekberg, Y., Arnillas, C. A., Borer, E. T., Bullington, L. S., Fierer, N., Kennedy, P. G., et al. (2021). Nitrogen and phosphorus fertilization consistently favor pathogenic over mutualistic fungi in grassland soils. Nat. Commun. 12, 1–8. doi: 10.1038/s41467-021-23605-y
Li, Q. S., Willardson, L. S., Deng, W., Li, X. J., and Liu, C. J. (2005). Crop water deficit estimation and irrigation scheduling in western Jilin province, Northeast China. Agric. Water Manag. 71, 47–60. doi: 10.1016/j.agwat.2004.07.003
Lin, C., Wang, Y., Liu, M., Li, Q., Xiao, W., and Song, X. (2020). Effects of nitrogen deposition and phosphorus addition on arbuscular mycorrhizal fungi of Chinese fir (Cunninghamia lanceolata). Sci. Rep. 10:12260. doi: 10.1038/s41598-020-69213-6
Lin, X. G., Feng, Y. Z., Zhang, H. Y., Chen, R. R., Wang, J. H., Zhang, J. B., et al. (2012). Long-term balanced fertilization decreases arbuscular mycorrhizal fungal diversity in an arable soil in north China revealed by 454 pyrosequencing. Environ. Sci. Technol. 46, 5764–5771. doi: 10.1021/es3001695
Liu, W., Zhang, Y. L., Jiang, S. S., Murray, P. J., Liao, L. Q., Li, X. L., et al. (2019). Spatiotemporal differences in the arbuscular mycorrhizal fungi communities in soil and roots in response to long-term organic compost inputs in an intensive agricultural cropping system on the North China Plain. J. Soils Sediments 19, 2520–2533. doi: 10.1007/s11368-019-02244-3
Lu, R. K. (1999). Soil and Agro-Chemical Analytical Methods. Beijing: China Agricultural Science and Technology Press, 146–195.
Munkvold, L., Kjoller, R., Vestberg, M., Rosendahl, S., and Jakobsen, I. (2004). High functional diversity within species of arbuscular mycorrhizal fungi. New Phytol. 164, 357–364. doi: 10.1111/j.1469-8137.2004.01169.x
Niedziński, T., Sierra, M. J., Łabętowicz, J., Noras, K., Cabrales, C., and Millán, R. (2021). Release of nitrogen from granulate mineral and organic fertilizers and its effect on selected chemical parameters of soil. Agronom. Basel 11:1981. doi: 10.3390/agronomy11101981
Oehl, F., Sieverding, E., Mäder, P., Dubois, D., Ineichen, K., Boller, T., et al. (2004). Impact of long-term conventional and organic farming on the diversity of arbuscular mycorrhizal fungi. Oecologia 138, 574–583. doi: 10.1007/s00442-003-1458-2
Pawlowska, T. E., and Taylor, J. W. (2004). Organization of genetic variation in individuals of arbuscular mycorrhizal fungi. Nature 427, 733–737. doi: 10.1038/nature02290
Qin, H., Lu, K., Strong, P. J., Xu, Q., Wu, Q., Xu, Z., et al. (2015). Long-term fertilizer application effects on the soil, root arbuscular mycorrhizal fungi and community composition in rotation agriculture. Appl. Soil Ecol. 89, 35–43. doi: 10.1016/j.apsoil.2015.01.008
Rosendahl, S. (2008). Communities, populations and individuals of arbuscular mycorrhizal fungi. New Phytol. 178, 253–266. doi: 10.1111/j.1469-8137.2008.02378.x
Roy, J., Reichel, R., Brueggemann, N., Hempel, S., and Rillig, M. C. (2017). Succession of arbuscular mycorrhizal fungi along a 52-year agricultural recultivation chronosequence. FEMS Microbiol. Ecol. 93:fix102. doi: 10.1093/femsec/fix102
Ryan, M. H., and Graham, J. H. (2002). Is there a role for arbuscular mycorrhizal fungi in production agriculture? Plant Soil 244, 263–271. doi: 10.1023/a:1020207631893
Ryan, M. H., and Graham, J. H. (2018). Little evidence that farmers should consider abundance or diversity of arbuscular mycorrhizal fungi when managing crops. New Phytol. 220, 1092–1107. doi: 10.1111/nph.15308
Sale, V., Aguilera, P., Laczko, E., Mader, P., Berner, A., Zihlmann, U., et al. (2015). Impact of conservation tillage and organic farming on the diversity of arbuscular mycorrhizal fungi. Soil Biol. Biochem. 84, 38–52. doi: 10.1016/j.soilbio.2015.02.005
Scullion, J., Eason, W. R., and Scott, E. P. (1998). The effectivity of arbuscular mycorrhizal fungi from high input conventional and organic grassland and grass-arable rotations. Plant Soil 204, 243–254. doi: 10.1023/a:1004319325290
Sheng, M., Lalande, R., Hamel, C., and Ziadi, N. (2013). Effect of long-term tillage and mineral phosphorus fertilization on arbuscular mycorrhizal fungi in a humid continental zone of Eastern Canada. Plant Soil 369, 599–613. doi: 10.1007/s11104-013-1585-4
Shi, X., Yu, D., Warner, E., Pan, X., Petersen, G., Gong, Z., et al. (2004). Soil database of 1: 1,000,000 digital soil survey and reference system of the Chinese genetic soil classification system. Soil Surv. Horiz. 45, 129–136. doi: 10.2136/sh2004.4.0129
Tang, X., Li, J., Ma, Y., Hao, X., and Li, X. (2008). Phosphorus efficiency in long-term (15 years) wheat-maize cropping systems with various soil and climate conditions. Field Crop. Res. 108, 231–237. doi: 10.1016/j.fcr.2008.05.007
The Ministry of Agriculture and Rural Affairs of China (2020). Action Plan For Conservation Tillage Of Black Soil In The Northeast China (2020–2025). Available online at: http://www.moa.gov.cn/gk/tzgg_1/tz/202003/t20200318_6339304.htm (accessed March 18, 2020).
Tisserant, E., Malbreil, M., Kuo, A., Kohler, A., Symeonidi, A., Balestrini, R., et al. (2013). Genome of an arbuscular mycorrhizal fungus provides insight into the oldest plant symbiosis. PNAS 110, 20117–20122. doi: 10.1073/pnas.1313452110
Trouvelot, A., Kough, J. L., and Gianinazzi-Pearson, V. (1986). “Mesure du taux de mycorhization VA d’un système radiculaire. Recherche de méthodes d’estimation ayant une signification fonctionnelle,” in Physiological and Genetic Aspects of Mycorrhizae, eds V. Gianinazzi-Pearson and S. Gianinazzi (Paris: INRA), 217–221.
van Geel, M., Verbruggen, E., De Beenhouwer, M., van Rennes, G., Lievens, B., and Honnay, O. (2017). High soil phosphorus levels overrule the potential benefits of organic farming on arbuscular mycorrhizal diversity in northern vineyards. Agric. Ecosyst. Environ. 248, 144–152. doi: 10.1016/j.agee.2017.07.017
Wu, F., Dong, M., Liu, Y., Ma, X., An, L., Young, J. P. W., et al. (2011). Effects of long-term fertilization on AM fungal community structure and Glomalin-related soil protein in the Loess Plateau of China. Plant Soil 342, 233–247. doi: 10.1007/s11104-010-0688-4
Wu, Y. H., Wang, H., Liu, M., Li, B., Chen, X., Ma, Y. T., et al. (2021). Effects of native arbuscular mycorrhizae isolated on root biomass and secondary metabolites of Salvia miltiorrhiza Bge. Front. Plant Sci. 12:617892. doi: 10.3389/fpls.2021.617892
Xiang, X., Gibbons, S. M., He, J. S., Wang, C., He, D., Li, Q., et al. (2016). Rapid response of arbuscular mycorrhizal fungal communities to short-term fertilization in an alpine grassland on the Qinghai-Tibet Plateau. PeerJ 4:e2226. doi: 10.7717/peerj.2226
Xiao, D., Che, R., Liu, X., Tan, Y., Yang, R., Zhang, W., et al. (2019). Arbuscular mycorrhizal fungi abundance was sensitive to nitrogen addition but diversity was sensitive to phosphorus addition in karst ecosystems. Biol. Fertil. Soils 55, 457–469. doi: 10.1007/s00374-019-01362-x
Yang, W., Guo, Y., Wang, X., Chen, C., Hu, Y., Cheng, L., et al. (2017). Temporal variations of soil microbial community under compost addition in black soil of Northeast China. Appl. Soil Ecol. 121, 214–222. doi: 10.1016/j.apsoil.2017.10.005
Yang, Z., Yu, T., Hou, Q., Xia, X., Feng, H., Huang, C., et al. (2014). Geochemical evaluation of land quality in China and its applications. J. Geochem. Explor. 139, 122–135. doi: 10.1016/j.gexplo.2013.07.014
Yue, X., Zhang, J., Shi, A., Yao, S., and Zhang, B. (2016). Manure substitution of mineral fertilizers increased functional stability through changing structure and physiology of microbial communities. Eur. J. Soil Biol. 77, 34–43. doi: 10.1016/j.ejsobi.2016.10.002
Zeng, H. L., Yu, L. L., Liu, P., Wang, Z. G., Chen, Y., and Wang, J. W. (2021). Nitrogen fertilization has a stronger influence than cropping pattern on AMF community in maize/soybean strip intercropping systems. Appl. Soil Ecol. 167:104034. doi: 10.1016/j.apsoil.2021.104034
Keywords: arable soil, chemical fertilizer, colonization intensity, network structure, OTU diversity
Citation: Chen J, Li J, Yang Y, Wang Y, Zhang Y and Wang P (2022) Effects of Conventional and Organic Agriculture on Soil Arbuscular Mycorrhizal Fungal Community in Low-Quality Farmland. Front. Microbiol. 13:914627. doi: 10.3389/fmicb.2022.914627
Received: 11 April 2022; Accepted: 20 May 2022;
Published: 09 June 2022.
Edited by:
Mahaveer P. Sharma, ICAR-Indian Institute of Soybean Research, IndiaReviewed by:
Thangavelu Muthukumar, Bharathiar University, IndiaCopyright © 2022 Chen, Li, Yang, Wang, Zhang and Wang. This is an open-access article distributed under the terms of the Creative Commons Attribution License (CC BY). The use, distribution or reproduction in other forums is permitted, provided the original author(s) and the copyright owner(s) are credited and that the original publication in this journal is cited, in accordance with accepted academic practice. No use, distribution or reproduction is permitted which does not comply with these terms.
*Correspondence: Ping Wang, d2FuZ3A3NDRAbmVudS5lZHUuY24=
†These authors have contributed equally to this work
Disclaimer: All claims expressed in this article are solely those of the authors and do not necessarily represent those of their affiliated organizations, or those of the publisher, the editors and the reviewers. Any product that may be evaluated in this article or claim that may be made by its manufacturer is not guaranteed or endorsed by the publisher.
Research integrity at Frontiers
Learn more about the work of our research integrity team to safeguard the quality of each article we publish.