- 1School of Agriculture and Food Science, University College Dublin, Dublin, Ireland
- 2School of Biology and Environmental Science, University College Dublin, Dublin, Ireland
Fusarium head blight (FHB) is a devastating disease encountered by spring-grown barley. Traditionally, synthetic chemicals have been used to control this disease on small grain cereals. A move toward biological control agents as part of sustainable agriculture is pertinent due to the evolutionary mechanisms employed by fungal diseases to circumvent current protection strategies. This study evaluated the effect of six lactic acid bacteria isolates on the development of FHB under in vitro and glasshouse conditions. The relative expression of Fusarium marker genes and transcription factors under Fusarium infection was examined. Dual-culture assays observed inhibition zones of up to 10 and 17% of total plate area for L. amylovorus FST 2.11 and L. brevis R2Δ, respectively. Detached leaf assays validated the antifungal activity and showed the potential of all test isolates to significantly inhibit sporulation of Fusarium culmorum and Fusarium graminearum strains. Spray inoculation of lactic acid bacteria to barley spikelets prior to Fusarium spore application significantly reduced disease severity for five candidates (P < 0.05) under glasshouse conditions. Mycotoxin analysis revealed the ability of L. amylovorus DSM20552 to significantly reduce deoxynivalenol content in spikelets (P < 0.05). A preliminary gene expression study showed the positive influence of lactic acid bacteria on the expression of important defense-related marker genes and transcription factors upon FHB. These results indicate the potential of lactic acid bacteria to be included as part of an integrated pest management strategy for the management of FHB disease. This strategy will reduce FHB severity and deoxynivalenol (DON) contamination of spring barley, leading to high acceptance in the grain market.
Introduction
Barley (Hordeum vulgare L.) is among the world’s first domesticated land plants that has underpinned the development of present-day civilizations. It is one of the most important food staples considered to be the premium grain used by malting and brewing industries (Ullrich, 2010; CGIAR, 2012; Murphy, 2016). The Irish brewing and distilling sector has shown a growth rate of 4% between 2014 and 2019 with a 22% increase in the number of micro-breweries. The number of distilleries has increased to 38 in 2020 with global sales at 20 million nine-liter cases (Umego and Barry-Ryan, 2022). Therefore, it is imperative that we provide an active concern for world barley production and the threats associated with the escalation of crop losses as we enter an era of uncertainty surrounding food security. FHB is a serious spoilage disease encountered by barley, wheat (Triticum aestivum), and other small grain cereals worldwide (McMullen et al., 1997; Wegulo et al., 2015; Gunupuru et al., 2018). Primary agents of the disease vary across geographical regions with Fusarium graminearum, Fusarium culmorum, Fusarium poae, and Fusarium avenaceum encountered by European cereal growers (Bottalico and Perrone, 2002; Wegulo et al., 2015; Beccari et al., 2017). Fusarium species are also the causal organism of Fusarium foot rot and Fusarium seedling blight of small grain cereals resulting in reduced germination and poor plant establishment leading to intensified yield and quality losses (Brennan et al., 2003; Goswami and Kistler, 2004; Khan et al., 2006; Scherm et al., 2013). Accordingly, barley’s worth as a seed source for malt production for brewing and other food industries declines (Parry et al., 1995; Scherm et al., 2013; Hückelhoven et al., 2018). In barley, Fusaria of the FHB complex secure our attention as they produce a wide range of mycotoxins including: DON, HT-2 toxin, T-2 toxin, aflatoxin, and ochratoxin, known instigators of chronic and acute mycotoxicosis in humans and animals (Placinta et al., 1999; Haidukowski et al., 2005; Nielsen et al., 2014; Bolechová et al., 2015). The consequence of extreme disease outbreaks resulting in an FHB epidemic has been seen with extensive economic and sociological impacts (Jones, 2000; McMullen et al., 2012).
To circumvent additional costs associated with reduced yield, poorer quality, and extra costs of cleaning grain, synthetic fungicides have traditionally been applied to prevent FHB disease outbreaks (Smiley et al., 2005; Blandino et al., 2012; Matny, 2015). However, the efficacy of available fungicides is uncertain due to the evolving nature of Fusarium, changing weather patterns, and the inherent variability of cultivar resistance (Simpson et al., 2001; Magan et al., 2002; Blandino et al., 2012; Talas and McDonald, 2015). Even with the introduction of disease predictive models allowing for optimal timing of fungicide applications, their employment only remains practical for a one-off application at mid-anthesis, with further treatment costs failing to be justified by yield and quality improvements (McMullen et al., 1997; D’Angelo et al., 2014). Extensive field studies on azole-based fungicides to manage FHB and mycotoxin accumulation presented reductions between 40 and 70%, respectively (Paul et al., 2008, 2018; Willyerd et al., 2012; D’Angelo et al., 2014). However, with increscent fungicide applications, over time, gradual resistance among the target organisms is a real possibility (Ferrigo et al., 2016). Notably, the application of the fungicide azoxystrobin 2 days post-artificial inoculation of wheat spikelets with Fusarium spp. resulted in enhanced DON production per unit of pathogen (Simpson et al., 2001). Another study quantified that sub-lethal levels of prothioconazole increased DON production in wheat (Audenaert et al., 2010). Consequently, there has been a renewed focus on the implementation of integrated pest management (IPM) systems as both an economically and ecologically justified means to reduce the risks to human health and the environment (Union, 2009; Pretty and Bharucha, 2015; Cowger et al., 2016).
To date, research has focused on the development of proficient biological control agents (BCAs), with the aim of contriving new commercially applicable FHB antagonists (Wachowska and Głowacka, 2014; Xue et al., 2014; Wang et al., 2015). A potential group of 22 endophytes to control F. graminearum (30–51% inhibition) and F. culmorum (15–53% inhibition) in vitro, including the discovery of three novel endophytic species, i.e., Aureobasidium proteae, Phoma glomeramycota and Sarocladium kiliense, have been reported (Comby et al., 2017). Recently, the mode of action of the known antifungal species Bacillus amyloliquefaciens Y1 has been identified as the antifungal compound cyclo(D-PRO-L-VAL), which was characterized using H1 and C13 nuclear magnetic resonance techniques (Jamal et al., 2017). Furthermore, it has been shown that detoxification of mycotoxin-infused produce, including grain, is possible through the addition of biological agents prior to and during the processing of foodstuffs (Petchkongkaew et al., 2008; Tian et al., 2016). Field trials gave comparable results for reductions in DON accumulation and FHB severity following applications of Bacillus subtilis strain RC218 (Palazzini et al., 2016; Cantoro et al., 2021). Several strains of lactic acid bacteria (LAB) have similarly shown promise through their enhancement of organoleptic properties and ability to mitigate fungal contamination of foodstuffs, most notably their incorporation into starter cultures during malting and the formulation of probiotics (Schnürer and Magnusson, 2005; Kedia et al., 2007; Franco et al., 2011; Rathore et al., 2012; Nakkarach and Withayagiat, 2018). The versatile use potential was further confirmed to reduce mycotoxin accumulation and gushing when applied to barley in situ (Lowe and Arendt, 2004; Peyer et al., 2016). It is considered that their role in fungal inhibition is through the production of organic acids, proteinaceous compounds, and other low molecular weight compounds (Lynch et al., 2016; Siedler et al., 2019). Despite making major progress, the role of many BCAs in the regulation of plant defense responses, mechanistic of LAB-mediated resilience to biotic stresses, viz., FHB disease of barley, remains limited (Miedaner et al., 2018; Gómez-Lama Cabanás et al., 2021).
As a pathogen-associated molecular pattern (PAMP) is conserved molecules among pathogens and PAMP-triggered immunity (PTI) contributes to basal and non-host resistance, the transfer of pattern recognition receptors (PRRs) might confer resistance to current pathogens in crops by recognition of undetected epitopes (Schoonbeek et al., 2015). Hence, expression analysis of selected PRRs, transcription factors, kinase, and R genes was performed on FHB infection in barley to ascertain its role in resistance. Interestingly, silencing of the chitin elicitor-binding protein (CEBiP) orthologous gene in barley increases susceptibility to the fungus Magnaporthe oryzae (Tanaka et al., 2010), suggesting that CEBiP may be involved in chitin perception. TaMPK3, which encodes a MAP kinase, involved in resistance to Mycosphaerella graminicola (Rudd et al., 2008). A wheat pore-forming toxin-like (PFT) homolog of HvPFT gene was highly expressed and regulatory FHB-resistant gene in Sumai 3 (He et al., 2018). The transcription factor HvWRKY23 regulates flavonoid glycoside and hydroxycinnamic acid amide biosynthetic genes in barley to combat FHB (Karre et al., 2019), whereas TaWRKY70 in wheat QTL-2DL regulates downstream metabolite biosynthetic genes to resist Fusarium graminearum infection spread within spike (Kage et al., 2017). Expression of salicylate-responsive pathogenesis-related 1 (HvPR1) was strongly induced by Fusarium graminearum strain Fg-IFA65GFP in barley leaves (Koch et al., 2016). The homolog ubiquitin ligase gene PUB23-like was highly induced in response to Fusarium PAMP treatment in Arabidopsis (Trujillo et al., 2008). The wheat homologs of HvLOX2, HvCOI1, HvICS1, HvPAL1, HvNPR1, and HvNPR3 were reported to have a regulatory role in FHB resistance (Thapa et al., 2018). The wheat homolog of HvCamBP is a particularly good marker due to robust upregulation upon PAMP treatment (Schoonbeek et al., 2015), whereas the CRISPR/Cas9 editing of susceptibility SWEET1 gene provides the potential for the development of FHB-resistant barley (Ahmad et al., 2020). As most of the genes get upregulated upon FHB infection, their selection in gene for expression analysis may reveal a resistance mechanism and can be used as PTI marker genes in barley.
The research evaluated the ability of six LAB isolates to inhibit Fusarium spp. and the Fusarium infection of spring barley through in vitro and glasshouse experiments. In vitro dual-culture assays were followed by detached leaf assays. FHB trials were established under glasshouse conditions, and a gene expression study examined the potential priming capabilities of starter cultures of LAB on the regulation of spring barley defense gene expression.
Materials and Methods
Plant Material
The Spring barley cv. “Sanette” used for in vitro and glasshouse studies was kindly provided by Gold Crop Ltd. (Cluide, Dunleer, Co., Louth, Ireland).
Lactic Acid Bacteria, Fusarium, and Their Culture
LAB isolates (Table 1) were chosen based on their ability to inhibit the growth of F. culmorum strain TMW4.2043 in vitro (Axel et al., 2016), which reported that F. culmorum strain 126 TMW4.2043 growth was inhibited by these selected LABs depending upon the synthesis of antifungal-active acids such as 3-phenyllactic acid, 4-hydroxyphenyllactic acid and 2-hydroxyisocaproic acid in quantities between 0.1 and 360 mg/kg. L. reuteri M13, lacking antifungal activity, was selected as a negative control (Lynch et al., 2016; Peyer et al., 2016). LAB were re-cultured regularly on de Man, Rogosa, and Sharpe (MRS) agar-filled Petri plates (M.R.S AGAR, Oxoid Ltd., Hampshire, United Kingdom) as per Meroth et al. (2003). Cultures were incubated at 30°C (L. amylovorus and L. brevis strains) and 37°C (L. reuteri strains) for 48 h under static conditions, and they were stored short term at 4°C. Single colonies were sub-cultured and used to inoculate 10 ml of de man, Rogosa, and Sharpe broth (MRS broth, Oxoid Ltd., Hampshire, United Kingdom). After a 24-h period, bacteria were sub-cultured at 1% into MRS broth at the respective temperatures for a further 48 h. Growth was observed through the assessment of the optical density of MRS broth at 620 nm (OD620) (Kedia et al., 2007). Fusarium graminearum strain GZ3639 and Fusarium culmorum strain FCF 200 were obtained from the UCD School of Biology and Environmental Science fungal collection (the former kindly provided by Robert Proctor, USDA). Fresh asexual macroconidia of both Fusarium spp. were cultured, and conidia were prepared as per the method outlined by Brennan et al. (2003). Following incubation, cultures were washed, and spores were counted using a haemocytometer (Hycor Biomedical) and adjusted to the 2 × 105 spores/ml using 0.2% Tween 20 (VWR Chemicals, Pennsylvania, United States).
Fungicide Preparation
The fungicide Fandango® (Bayer CropScience Ltd., Dublin, Ireland) was used as a comparative treatment based on its commercial use against foliar, spikelet, and stem base diseases of small grain cereals. It was prepared as per manufacturers’ recommendations at the rate of 1.25 L/ha (Bayer CropScience Ltd., Dublin, Ireland).
Dual-Culture Assay
Antifungal activity of the selected LAB against F. graminearum strain GZ3639 was observed using a modified version of the dual-culture assay (Khan et al., 2006). Potato dextrose agar (PDA; Oxoid Ltd., United Kingdom) plates were inoculated with mycelial plugs (10 mm diameter) from 7-day-old Fusarium cultures placed at the center of each plate. Three sterile 1.0-cm Whatman filter paper disks (Whatman plc, Buckinghamshire, United Kingdom) were equidistantly placed at the perimeter of each plate 26 mm from mycelial plugs. Ten microliter aliquots of LAB (log CFU/ml = 9) were pipetted onto each disk (MRS broth was used as a negative control). Plates were incubated at 21°C ± 1°C in darkness. The antifungal activity was evaluated by measuring the area of the inhibition surrounding individual filter paper disks 168 h post-incubation, using ImageJ software (NIH, United States). A total of three trials were completed. Each trial consisted of three biological replications for each treatment and control.
Detached Leaf Assay
This experiment was a modification of the detached leaf assay (Chen et al., 2006). Four seeds were sown in 4-cm pots containing John Innes Number 2 compost (John Innes Manufacturer Association, Berkshire, United Kingdom) and grown at 20°C ± 4°C under artificial light conditions of 16-h light/8-h dark cycle at 150 μmol m-2 s-1. The relative humidity was maintained at 50% ± 5%. Seedlings were thinned to two per pot 7 days after germination. After 21–25 days, second true leaves, growth stage 12 (Zadoks et al., 1974), were detached. Leaf sections (6 cm) were placed adaxial side up in square Petri dishes on 10% plant nutrient agar (Duchefa, Netherlands), pH 5.7, comprising 0.67 μM benzimidazole (Oxoid Ltd., Hampshire, United Kingdom). Treatments were positioned centrally along the midrib of each leaf section. They consisted of 10 μl containing 1 × 106 F. graminearum strain GZ3639 conidia ml–1 0.02% Tween 20 and 20 μl aliquot of the chosen LAB. The negative control treatment combination was 10 μl of 0.02% (v/v) Tween 20 and 20 μl of MRS broth. The positive fungal control treatment combination was 10 μl of 1 × 106 F. graminearum strain GZ3639 conidia ml–1 0.02% Tween 20 and 20 μl of MRS broth. The fungicide Fandango® was incorporated as a comparative treatment. The same inoculation method and pattern were followed for F. culmorum experiment wherein F. culmorum spores were used. The treated leaves in plates were incubated under the conditions. The number of conidia per leaf section was determined at 5 dpi as per Chen et al. (2006). Starting at 48 hpi, mycelial spread was measured using ImageJ computer software (NIH, United States). Disease severity was assessed per the relative lesion area (RLA) at 48, 72, 96, and 120 h post-inoculation. RLA was calculated using the formula %RLA = 100 × (total area of lesions)/(total leaf area). A total of three replicate trials were completed. Each replicate trial consisted of 12 biological replicates for each treatment and control.
FHB Severity and Mycotoxin Analysis
An adult plant experiment was conducted to establish the effects of LAB on FHB development and mycotoxin accumulation. Four pre-germinated seedlings were transferred at 10-cm spacings into 3-L containers of John Innes Number 3 compost (John Innes Manufacturer Association, Berkshire, United Kingdom). Plants were placed in an unlit, ventilated greenhouse. Natural light levels were maintained under shading engaged at 60,000 lux m−1 and retracted at 50,000 lux m-1. Seedlings were culled to two per container after 20 days. Plants were irrigated three times weekly along with an added liquid feed of 18–18–18 at a dilution of 1:1,000 weekly with a granular feed of 22-5-6 + 2 Mg + TE (4–5 months, Osmocote® Topdress FT, Everris International BV, Netherlands, containing 22% nitrogen, 2.2% phosphorous, 5% potassium, 1.2% magnesium and trace elements) applied 14 days after transplanting and ca.1 week prior to applications of biocontrol agents.
Spray inoculations (4 ml) of LAB isolates at a concentration of log CFU/ml = 9 (onto secondary tillers) were made ca. 24 h prior to mid-anthesis. Control heads were treated with MRS broth. At mid-anthesis [growth stage 65 (Zadoks et al., 1974)], heads were treated with 4 ml of 0.02% (v/v) Tween 20 (control) or 4 ml of 0.02% (v/v) Tween 20 (mock) supplemented with 2 × 105 conidia ml–1 of F. graminearum strain GZ3639. Directly after inoculations, all treated heads were enclosed in resealable bags for 3 days. A total of three trials were completed. Each trial consisted of eight biological replicates for each treatment/control arranged in a randomized block design. Disease severity was estimated by counting the number of infected spikelets per ear and expressing this as a percentage of total spikelet infections at 5, 10, and 14 dpi. Fusarium infection was identified as brown discolored lesions with premature blanching of individual spikelets (McCallum and Tekauz, 2002; McMullen et al., 2012). The treated spikelets were harvested at plant senescence (growth stage 99 (Zadoks et al., 1974). A total of three biological replicates were completed. Each biological replicate consisted of 32 trials for each treatment and control. Spikelets were dried in a desiccator with silica gel beads for 14 days. Spikelets were triturated using a Glen Creston Hammer Mill and sieved through a 1.0-mm mesh sieve. Sieved samples were bulked according to the treatment per replicate. Mycotoxin testing was carried out for DON, aflatoxin, ZEA T-2 toxin, and ochratoxin by© Sciantec Analytical Laboratories using specific R-Biopharm RIDASCREEN® enzyme-linked immunosorbent assay (ELISA) tests. The absorbance value measured at 450 nm with the RIDA®SOFT Win (Art. No. Z9999) software package (R-Biopharm, Darmstadt, Germany) was used to deduce the toxin content of each sample.
Analysis of Defense Gene Expression in Adult Barley Plants
A separate experiment used quantitative reverse transcriptase-polymerase chain reaction (qRT-PCR) analysis to elucidate the effect of LAB isolates on the regulation of important defense genes and transcription factors in flowering barley heads (Table 2). Spikelets were treated with the same LAB, Fusarium, and control treatments detailed above. The treated spikelets were harvested at 12-, 24-, 48-, 72- and 120-h time points post-fungal inoculation. RNA was extracted using Trizol™ reagent according to the manufacturers’ specifications (Thermo Fischer Scientific, Massachusetts, United States) (Chomczynski, 1993). Reverse transcription of total RNA was performed as previously described (Meroth et al., 2003; Walter et al., 2008). Accumulated threshold cycle (Ct) values were obtained by qPCR, and the barley genes HvActin and HvU-61 were used as housekeeping genes to calculate the relative expression of the selected defense genes. The formula described previously (Livak and Schmittgen, 2001) was used to calculate relative gene expression: 2-Δ (Ct target gene—Ct housekeeping gene). This gene expression study was based on an FHB experiment comprised of three biological replicates with 32 trials for each treatment in each biological replicate. Each treatment time point consisted of 3–4 pooled spikelets from two separate plants per replicate. All real-time RT-PCR analyses were performed two times (two cDNAs generated from independent reverse transcriptions from each individual sample of RNA) for all the treatments.
Statistical Analysis
All data from in vitro and glasshouse experiments were analyzed in R v4.0.2 (R Core Team). Normality was tested using the Shapiro–Wilk test. Non-normal data were analyzed by a pairwise Kruskal–Wallis test. Correlations of data were tested using Spearman’s rank correlation coefficient analysis. Data from gene expression studies were analyzed using GraphPad Prism6® (GraphPad Software, San Diego, California, United States).
Results
Assessment of the Inhibitory Effect of Lactic Acid Bacteria Against Fusarium graminearum in Dual Cultures
Dual-culture assays assessed the capacity of six Lactobacillus strains to inhibit the spread of mycelium from Fusarium graminearum GZ3639. The maximum inhibition was provided by L. brevis R2Δ with an average reduction of 17% in mycelial growth (p < 0.05) (Table 3). Both L. amylovorus FST 2.11 and L. amylovorus DSM 20053 significantly reduced mycelial spread compared with the control (P < 0.05). The three remaining Lactobacillus isolates, namely, L. amylovorus DSM 20552, L. reuteri M13 and L. reuteri R29, failed to significantly inhibit mycelial spread compared with the control (p > 0.05). No clear zone of inhibition was observed at the point of contact between the control (MRS broth) and the fungus. In addition to mycelial impedance, it was observed that L. amylovorus FST 2.11 altered hyphal color from cream/light pink to a bright pink/red hue (Figure 1).
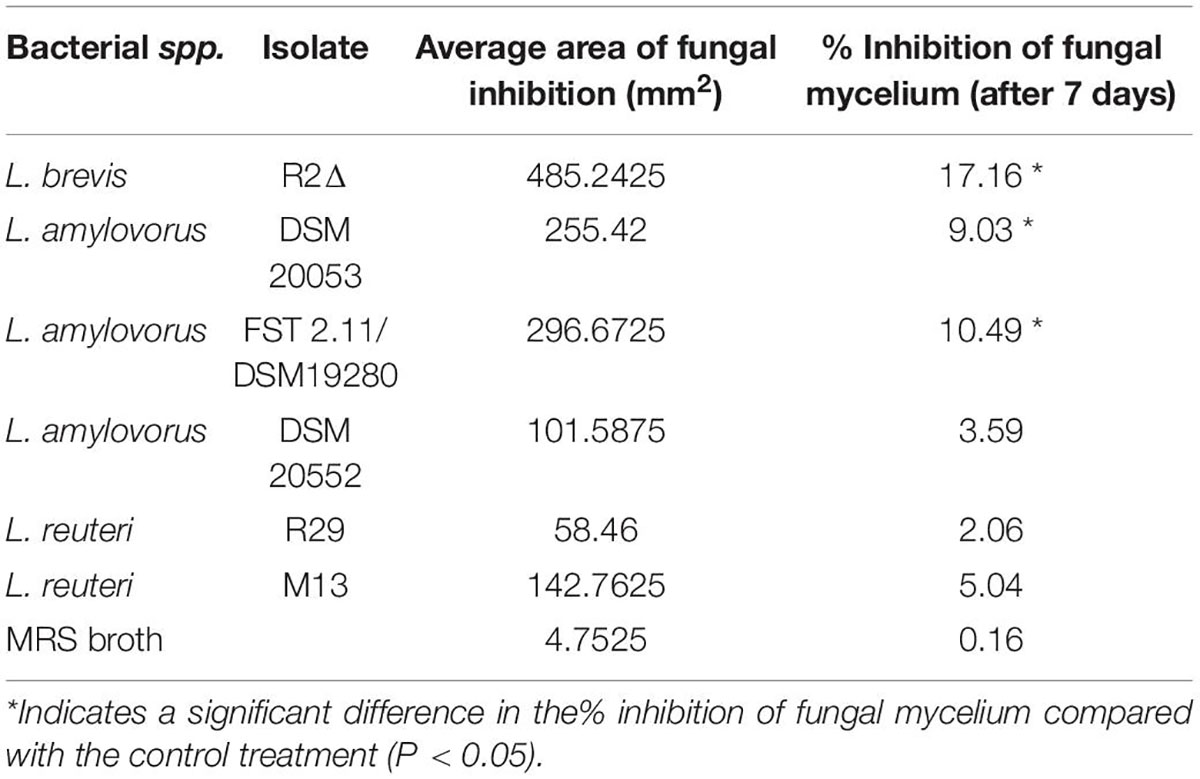
Table 3. Percentage of growth inhibition of F. graminearum by six Lactobacillus species under in vitro dual-culture conditions.
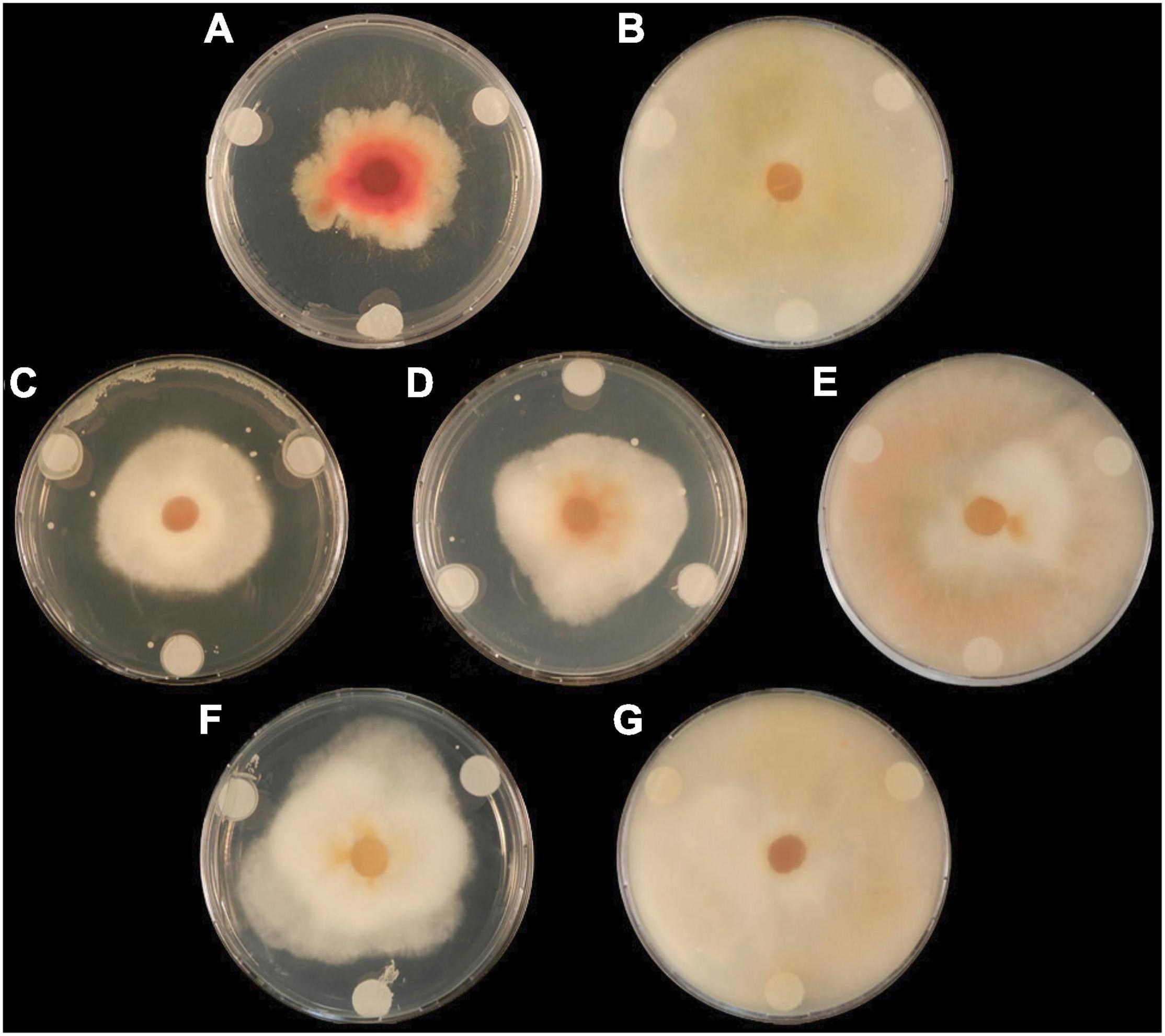
Figure 1. The effect of lactic acid bacteria on radial growth of F. graminearum GZ3639 on potato dextrose agar plates at 7 days. Treatments (A) (L. amylovorus FST 2.11), (C) (L. brevis R2Δ), and (D) (L. amylovorus DSM 20053) displayed clear zones of fungal inhibition compared with the control (P < 0.05), whereas treatments (B) (L. reuteri M13), (E) (L. reuteri R29), and (F) (L. amylovorus DSM 20552) displayed lowered antifungal activity (P > 0.05). Control treatment (G) (MRS broth) displayed no inhibition.
Assessment of the Ability of Lactic Acid Bacteria to Inhibit Fusarium Infection, as Determined Using a Detached Leaf Assay
Based on the mycelial growth inhibition in vitro assay, the selected LAB was assessed in plant for the fungal spread and sporulation suppression of F. graminearum and F. culmorum using a modified leaf detachment assay (Chen et al., 2006). No Fusarium-like lesions appeared on control treatments (MRS broth and Tween 20). On all other treatments, at 120 hours post-inoculation (hpi), all leaves displayed brown necrotic lesions typical of Fusarium infection (Koch et al., 2013). Lesion area on leaves treated with the positive control of both test fungi was significantly larger than that of the negative control at 120 hpi, although these differences were only significant from 72 hpi onward (p < 0.001). The positive control across both Fusarium spp. resulted in the highest average relative lesion area, as compared to all other treatments (Figure 2A). Lesion size was expressed as a percentage of infection to control treatment 10 μl of 0.02% (v/v) Tween 20 and 20 μl of MRS broth. For the leaves treated with LAB in combination with F. culmorum, the most effective isolates minimizing lesion size were L. amylovorus DSM 20053 (24%), L. amylovorus FST 2.11 (26%) and L. brevis R2Δ (32%).
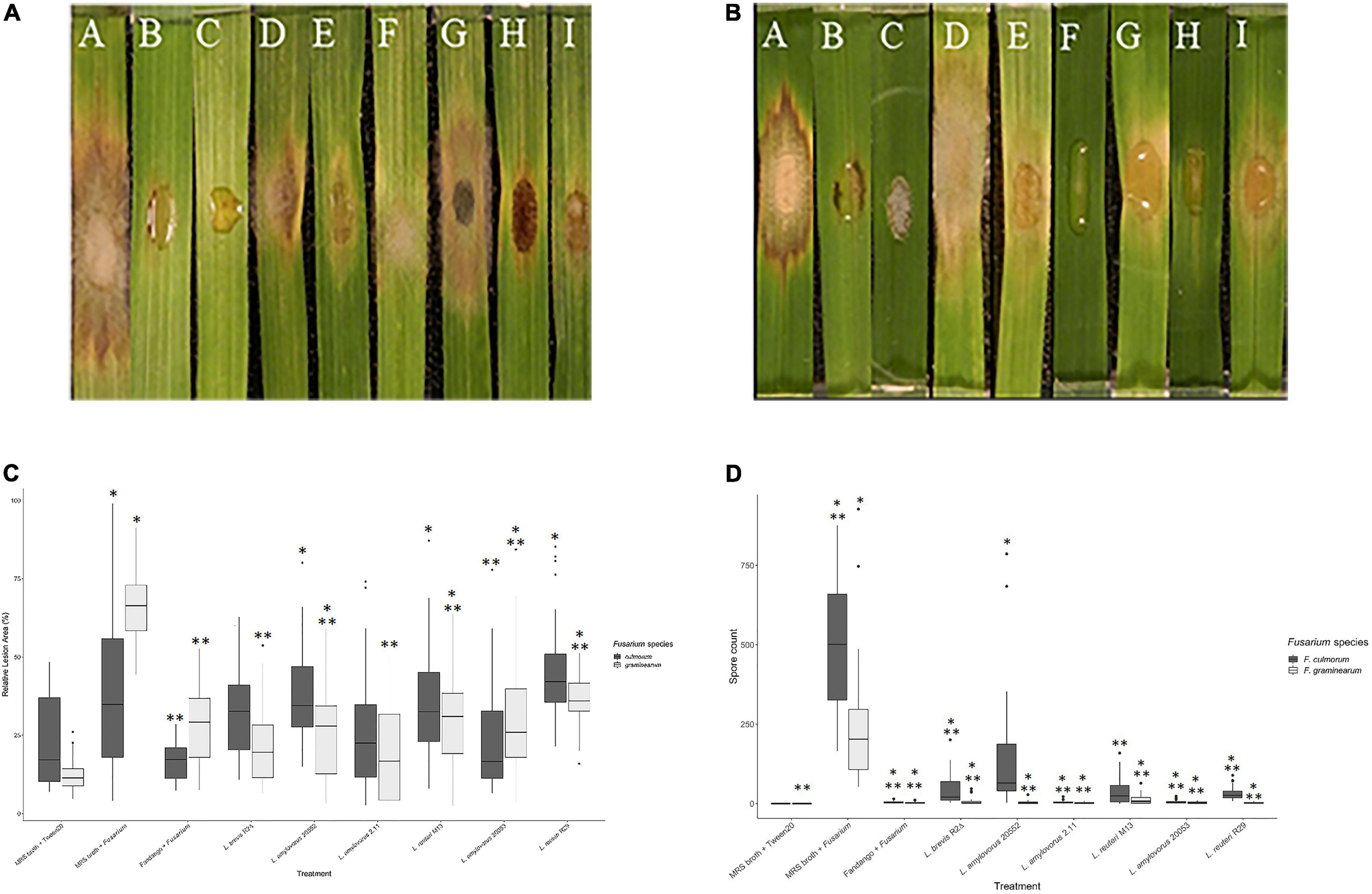
Figure 2. Effect of lactic acid bacteria on the mycelial growth of Fusarium spp. using detached leaf assay. (A) Treatments were applied simultaneously. Disease estimation was calculated by measuring the relative lesion area at 120 h post-inoculation. * Indicates treatments significantly different than the control MRS broth + Tween 20 (F. graminearum). ** Indicates treatments significantly different than the control MRS broth + F. graminearum. (B) Symptomatic diseased lesions on barley leaves. The barley leaves were treated with F. graminearum and controls [(A) MRS broth + F. graminearum, (B) Fandango® + F. graminearum (C) MRS broth + Tween 20]. MRS broth and lactic acid bacteria [(D) L. reuteri R29, (E) L. brevis R2Δ, (F) L. amylovorus FST2.11, (G) L. reuteri M13, (H) L. amylovorus DSM 20053, and (I) L. amylovorus DSM 20552]. The effects of the treatments above were observed 120 h post-inoculation. (C) Symptomatic diseased lesions on barley leaves treated with F. culmorum and controls [(A) MRS broth + F. culmorum, (B) Fandango® + F. culmorum (C) MRS broth + Tween 20]. MRS broth and lactic acid bacteria [(D) L. reuteri R29, (E) L. brevis 2Δ, (F) L. amylovorus FST 2.11, (G) L. reuteri M13, (H) L. amylovorus DSM 20053, and (I) L. amylovorus DSM 20552]. The effects of treatments above observed 120 h post-inoculation. (D) An assessment of LAB treatments on sporulation of Fusarium spp. by detached leaf assay. Conidia production was determined 5 days post-inoculation. *** Indicates treatments significantly different than the control MRS broth + Tween 20 (F. culmorum). **** Indicates treatments significantly different than the control MRS broth + F. culmorum. * Indicates treatments significantly different than the control MRS broth + Tween 20 (F. graminearum). ** Indicates treatments significantly different than the control MRS broth + F. graminearum.
The fungicide Fandango® provided significantly higher levels of disease suppression compared with all LAB isolates (P < 0.001) except L. amylovorus DSM 20552. Against F. graminearum, all LAB isolates significantly reduced diseased lesion area compared with the positive control (MRS + F. graminearum) (P < 0.05). The effect of LAB on sporulation of both Fusarium species was examined. Leaves treated with the positive control resulted in significantly greater spore quantities than those of the negative control (P < 0.001). All LAB isolates displayed an ability to reduce sporulation of F. graminearum compared with the positive control (P < 0.001) (Figure 2D). Leaves treated with Fandango® + Fusarium graminearum resulted in a larger lesioned area than that of the negative control of MRS + Tween 20 (p > 0.05). Leaves inoculated with the test fungus F. graminearum and MRS broth brought about the largest relative lesion area (68%), while L. brevis R2Δ and L. amylovorus DSM 20552 were the LAB that caused the greatest lesion inhibition at 24 and 23%, respectively (Figure 2C). Compared with the positive control, sporulation of F. graminearum saw the biggest reduction by L. amylovorus FST 2.11 with an average spore count of 2.3 per detached leaf (p < 0.001). Subsequent bacteria which significantly reduced sporulation of F. graminearum included L. reuteri R29 and L. amylovorus DSM 20053 with average spore counts of 2.61 and 3.00 per detached leaf, respectively (p < 0.001).
Comparable results were observed for detached leaves co-inoculated with F. culmorum and LAB (Figures 2B,D). The positive treatment resulted in the highest levels of spore production which were significantly higher (504.22) than most LAB treatments (P < 0.001). The exception was L. amylovorus DSM 20552 which did not have a significantly reduced spore count (p > 0.05). LAB isolates with the greatest inhibition of spore count included L. amylovorus FST 2.11 and L. amylovorus DSM 20053 which did not differ significantly when compared with the negative control (p > 0.05). No correlations between treatments were found for LAB co-inoculations against both test fungi.
Evaluation of the Effect of Lactic Acid Bacteria Treatments on FHB Development and Mycotoxin Accumulation Under Greenhouse Conditions
An FHB experiment assessed the impact of L. amylovorus FST 2.11, L. amylovorus DSM 20552, L. amylovorus DSM 20053, L. reuteri M13, L. brevis R2Δ, and L. reuteri R29 (Table 1) on disease development and mycotoxin accumulation in grain. Disease severity scoring was assessed at 5, 10, and 14 dai and used to calculate the area under disease pressure curve (AUDPC) (Jeger and Viljanen-Rollinson, 2001; Legzdina and Buerstmayr, 2004; Legzdina et al., 2013). Disease symptoms were minimal but not completely absent on spikelets treated with Tween 20 (mock). This is likely due to the heightened levels of background infection after unnatural levels of inoculum were applied to the neighboring plants. The majority of LAB treatments significantly reduced FHB severity compared with the positive control of MRS + F. graminearum (Figure 3). The exception was heads treated with L. reuteri R29, which showed no significant reduction compared with the positive control (P > 0.05). A noteworthy observation was the deleterious effect that early inoculations of MRS broth, and MRS broth containing bacterial cultures, had on spike maturation. In some cases, heads treated more than 24 h before mid-anthesis commenced premature cessation.
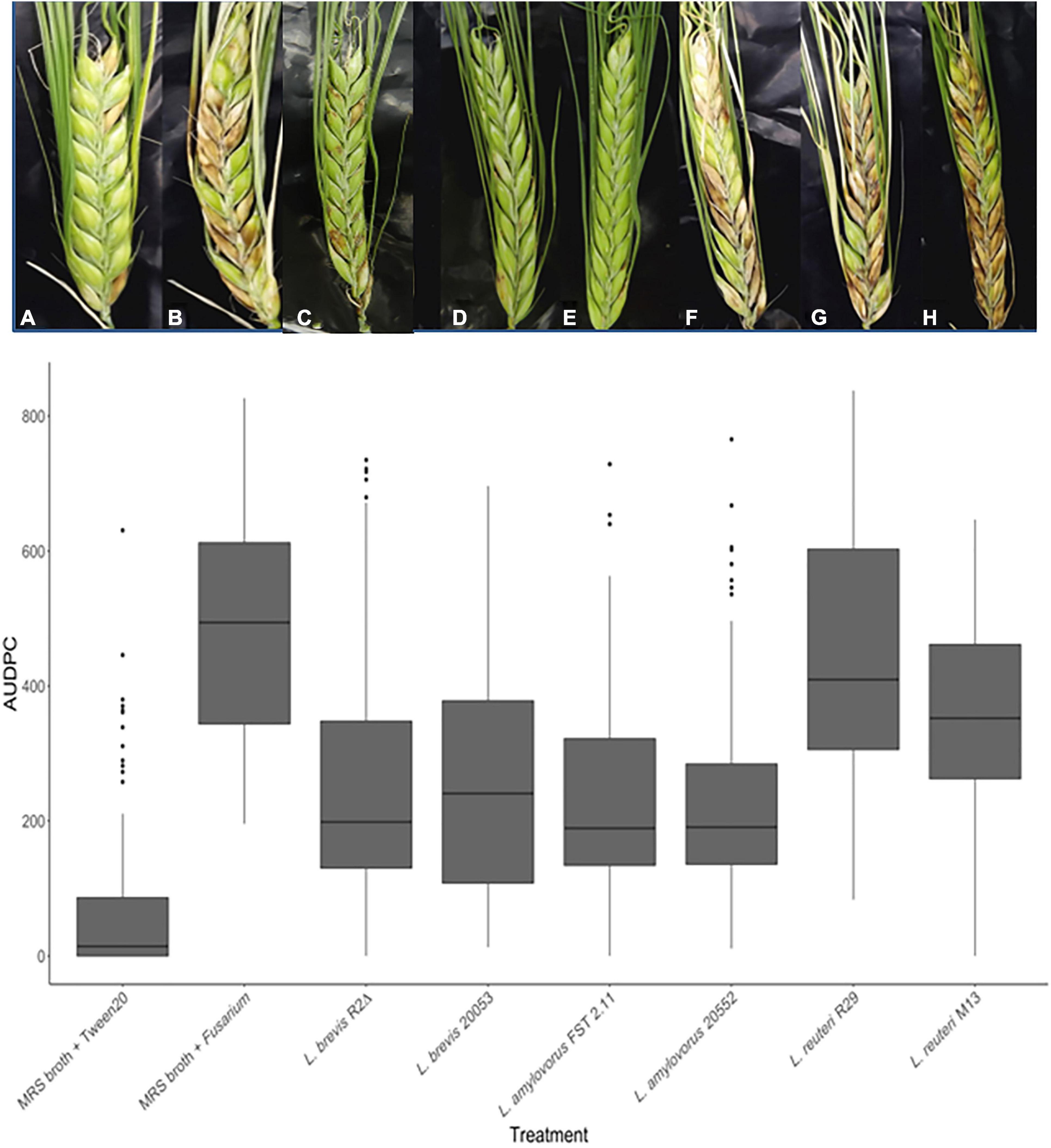
Figure 3. FHB symptoms of spring barley cultivar “Sanette” assessed following LAB and control treatments at 14 days post-inoculation. Chosen spikelets received LAB [(C) (L. amylovorus DSM 20552)], [(D) (L. amylovorus DSM 20053)], [(E) (L. brevis R2Δ)], [(F) (L. amylovorus FST 2.11)], [(G) (L. reuteri M13)], [(H) (L. reuteri R29)] or MRS broth (A,B). Conidia of F. graminearum (B) or Tween 20 (mock) (A) were spray applied at mid-anthesis. Disease severity means were analyzed using the Kruskal–Wallis H test. * Indicates treatments significantly different from the control MRS broth + Tween 20. ** Indicates treatments significantly different than the control MRS broth + F. graminearum/F. culmorum.
Spikelets were analyzed for the mycotoxins DON, aflatoxin, ochratoxin A, T-2 toxin, and zearalenone (Figure 4). Correlation of mycotoxin accumulation was moderate to strong between the three trials (r > 0.55) across all treatments. DON was the highest recorded mycotoxin at 204.4 μg/kg. Ochratoxin A levels were undetectable using ELISA. Mycotoxin accumulation of DON, T-2 toxin, ZEA, and aflatoxin was not significantly different across treatments from three replications (p > 0.05). Although mycotoxin accumulation was generally higher in the samples treated with MRS broth and Fusarium than in samples treated with LAB and Fusarium, no significant differences were found in mycotoxin accumulation between LAB treatments, once P-values were adjusted for multiple comparison testing.
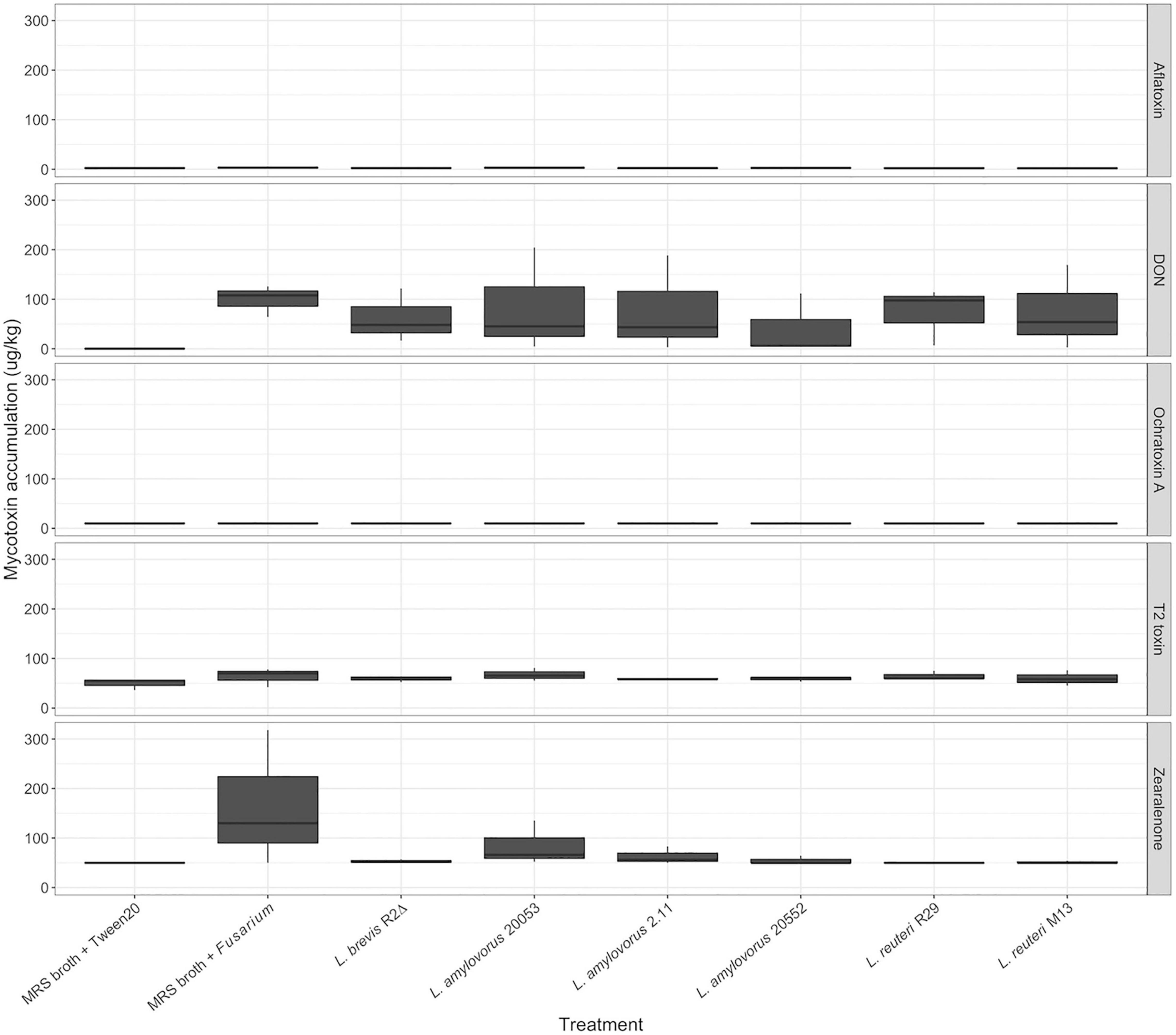
Figure 4. Mycotoxin accumulation in pooled FHB grain samples. Mycotoxin means were separated using the Kruskal–Wallis H test.
The Effect of Lactic Acid Bacteria on Defense Gene Expression in Spikelet Tissue
Quantitative real-time PCR (qRT-PCR) was used to quantitatively determine the effect of LAB isolates on the expression of 11 important defense-related genes (Table 2). All genes were expressed in barley spikelets treated with 0.2% Tween 20 and MRS broth (mock). The target gene expression was quantified relative to that of the barley housekeeping genes HvActin and HvU-61. Responsiveness of defense-related genes following LAB and Fusarium applications showed that different treatments triggered variable expression levels across the time points. One notable trend was the increased quantity of HvPUB23, HvICS1, HvWRKY23, HvActin, HvCAMBP1, and HvCOI1 transcripts from 72 to 120 hpi. Spray inoculations of spikelets with LAB and F. graminearum resulted in no significant changes in the expression of HvSWEET1, HvNPR3, HvPR1, HvLOX2, and HvMPK3 compared with those treated with the positive or negative controls (Figure 5). Levels of mRNA accumulation of HvICS1 were found to progressively increase from 12 hpi to 120 hpi (Figure 6A). Significant differences in HvICS1 expression levels were not noted until 120 hpi where L. amylovorus FST 2.11-treated spikelets had significantly more transcripts as compared to all other LAB treatments and controls (p < 0.05). Significant changes triggered by LAB treatments on the expression of HvCOI1 were noted from 12 hpi (p < 0.05) (Figure 6B). At 120 hpi, all LAB treatments brought about significant changes in levels of HvCOI1 expression compared with the positive control of MRS broth + F. graminearum. Of the six LAB isolates, only L. amylovorus FST 2.11 expressed higher levels of HvCOI1 expression (P < 0.05), the remainder expressing lower levels compared with the positive control at 120 hpi (p < 0.05). Three treatments of L. amylovorus FST 2.11, L. amylovorus DSM 20053, and L. brevis R2Δ did induce HvCOI1 expression compared with the negative control of MRS broth + Tween 20 (p < 0.05), but only L. amylovorus FST 2.11 expressed higher levels of HvCOI1 compared with the positive control treatment (p < 0.05). The remaining LAB treatments all provided lower levels of HvCOI1 expression compared with the negative control, with L. reuteri M13 significantly lower (p ≤ 0.006).
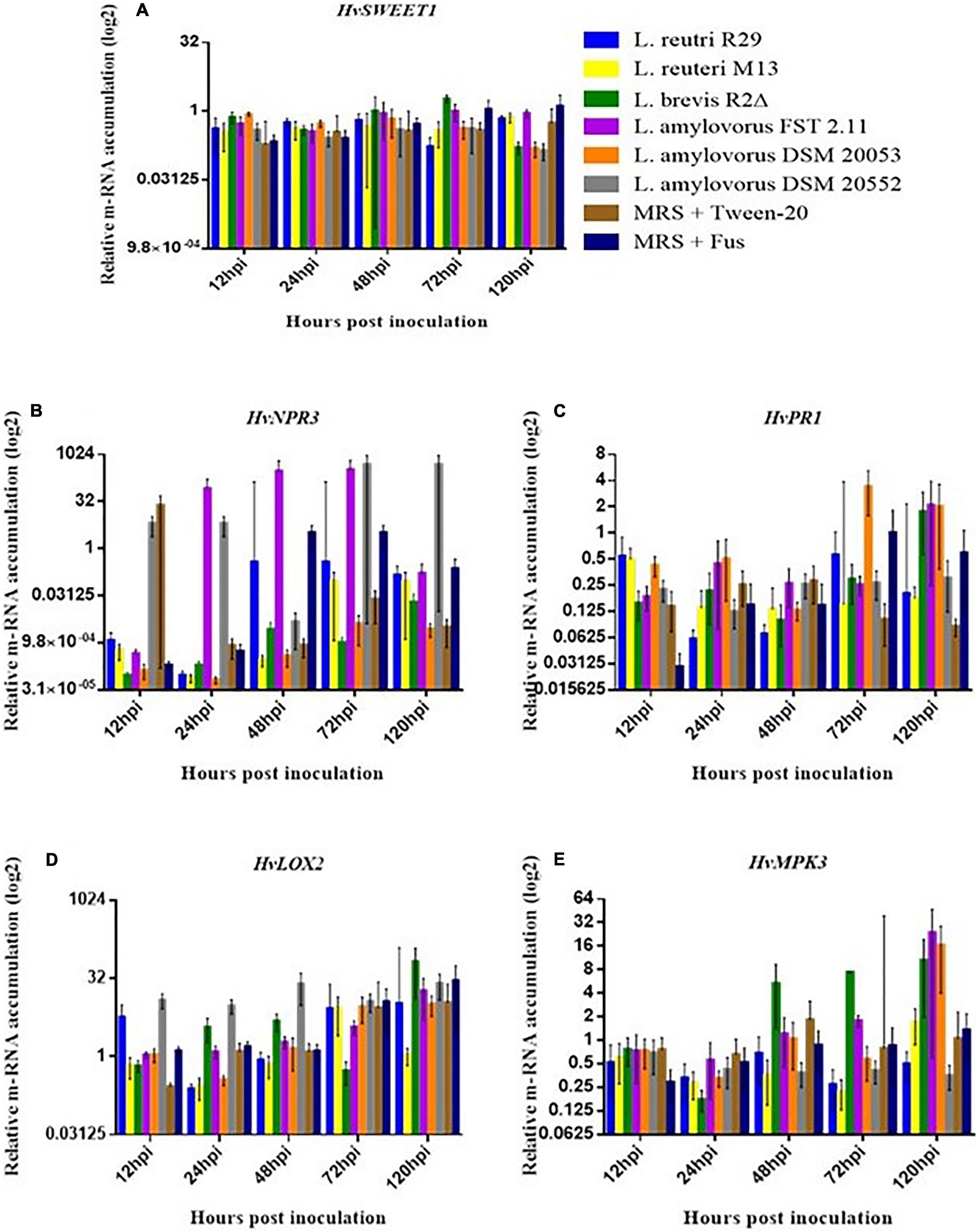
Figure 5. Relative gene expression level in barley head tissue harvested at 12, 24, 48, 72, and 120 hpi of fungal inoculation. (A) HvSEEWT1, (B) HvNPR3, (C) HvPR1, (D) HvLOX2 and (E) HvMPK3. Error bars indicate ± SEM (n = 8). Asterisks show significant differences between expression levels (*P, 0.05).
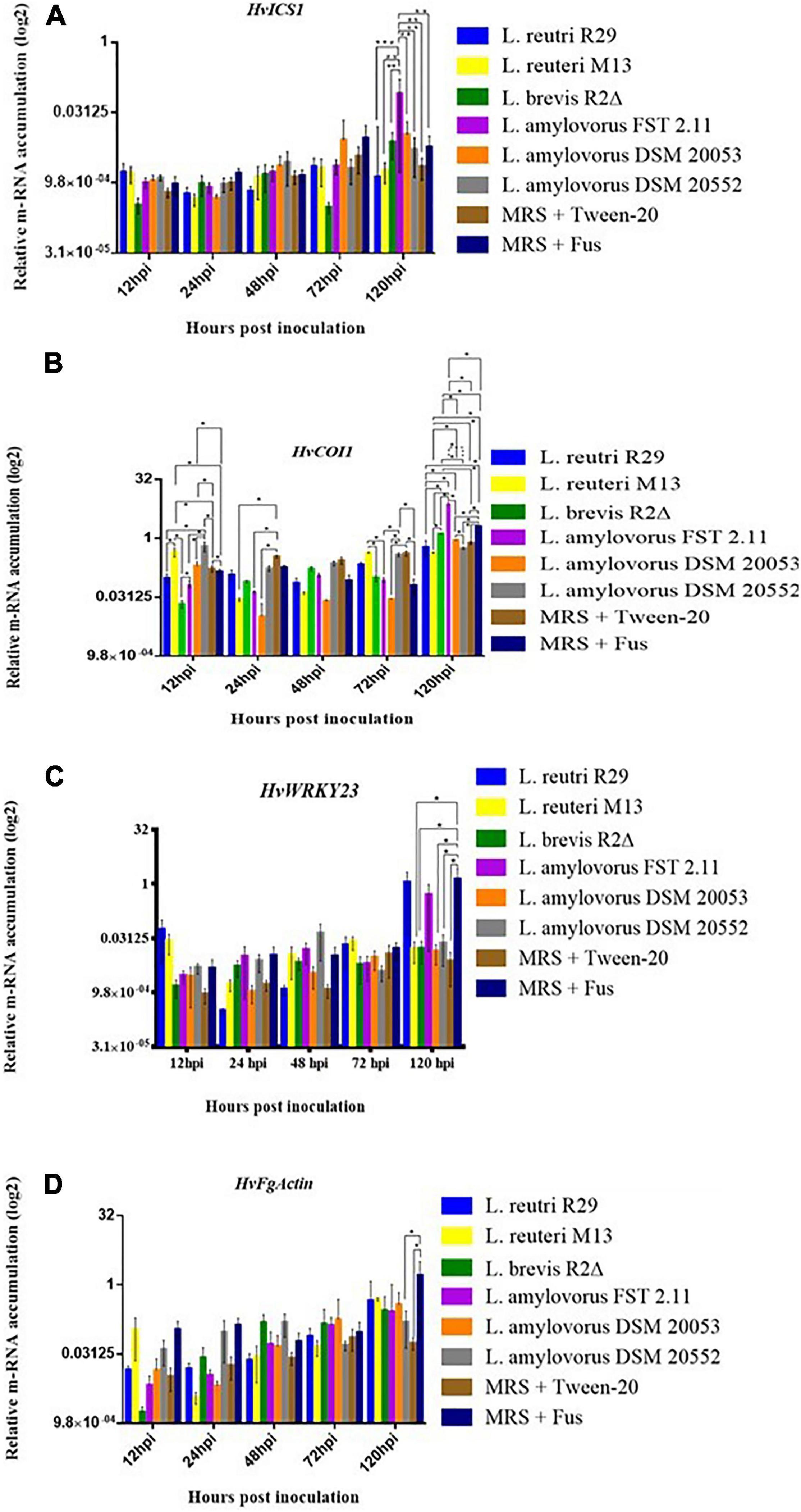
Figure 6. Relative gene expression level in barley head tissue harvested at 12, 24, 48, 72, and 120 hpi of fungal inoculation. (A) HvICS1, (B) HvCOI1, (C) HvWRKY23 and (D) FgActin. Error bars indicate ± SEM (n = 8). Asterisks show significant differences between expression levels (*P, 0.05).
The expression of transcription factor HvWRKY23 from 12 to 120 hpi was inconsistent with induction and downregulation (Figure 6C). From 12 to 72 hpi, no significant effect of treatments was observed. At 120 hpi, a significant reduction in expression levels was noted when the control treatment MRS + F. graminearum was compared with LAB treatments of L. brevis R2Δ, L. amylovorus DSM20053 and L. amylovorus DSM20552 (P < 0.05). At the same time point, expression levels of HvWRKY23 were also significantly greater for spikelets treated with MRS broth + F. graminearum compared with the mock treatment of MRS broth + Tween 20 (P ≤ 0.01). The expression levels of HvPUB23 were examined with significant differences only observed at 120 hpi. Accumulation of mRNA was significantly greater in L. reuteri R29 when compared with L. reuteri M13 (P ≤ 0.014), L. amylovorus DSM 20053 (P ≤ 0.022), L. amylovorus DSM 2055 (P ≤ 0.013), MRS broth + F. graminearum (P ≤ 0.046) and MRS broth + Tween 20 (P ≤ 0.017). Differences in expression levels of L. reuteri R29, L. brevis R2Δ, and L. amylovorus FST 2.11 were not significant (P > 0.05). The relative gene expression of FgActin, monitored as an indicator of fungal activity, revealed a general incline from 12 to 120 hpi (Figure 6D). LAB treatments of L. reuteri M13 and L. reuteri R29 provided the highest levels of FgActin although these differences were not significant (p > 0.05). Two treatments, namely, L. amylovorus DSM 20552 and the mock, were shown to significantly reduce levels of FgActin mRNA accumulation compared with that of MRS broth + F. graminearum-treated heads (p < 0.05).
Discussion
Management of fungal diseases caused by several Fusarium spp. including F. graminearum has remained a ceaseless challenge since the realization of their impact on yield and quality of grain. Indeed, the incessant threat of cool damp weather, particularly at flowering, and increased concern regarding fungicide use to combat the pathogens that cause head blight, foot rot, and seedling blight mean we must reconsider our options (Arendrup et al., 2013; Shi et al., 2020). In addition to yield losses, it is the propensity of many Fusarium spp. to contaminate grain with mycotoxins that impacts the health of humans and animals who consume the affected grain which causes much consternation (Koch et al., 2013; Gallo et al., 2015; Tibola et al., 2016). Reforming our approach to these challenges by providing both economically and environmentally driven alternatives to existing fungicide solutions will allow for sustainably driven tillage systems. Hence, the use of LAB as BCAs to protect crops becomes imminent, as BCAs are cheaper, are environment friendly, leave no toxic residue, and are easy to handle, apply, manufacture and multiply in the soil (Sharma et al., 2013; Mittholiya et al., 2020). There has been a report of in vivo efficacy of LAB as BCAs against Fusarium oxysporum protection in tomato when used as seed treatment (Hamed et al., 2011). Lactobacillus coryniformis subsp. coryniformis strain Si3 was also reported to produce PLA, cyclo(Phe-Pro), cyclo(Phe-4-OH-Pro) in vitro inhibiting Fusarium graminearum, Fusarium culmorum, Fusarium sporotrichioides and Rhodotorula mucilaginosa (Magnusson and Schnürer, 2001). Furthermore, the LAB were found to live in positive dynamic and assembly as epiphyte and endophyte with Origanum vulgare L (Pontonio et al., 2018), which open a door for natural BCAs. Hence, herein the research project to investigate the potential of LAB to be used as BCAs to control FHB in barley was taken up.
Dual-culture assays revealed that three of the tested LABs, namely, L. amylovorus FST 2.11, L. amylovorus DSM 20053, and L. brevis R2Δ, can reduce the mycelial growth of the test fungus. For the majority, these results agree with the antifungal potential of the selected isolates as shown in previous studies (Lynch et al., 2016; Peyer et al., 2016) with the exception of L. reuteri R29 with a diminished antifungal activity. This may be explained by the specificity of L. reuteri R29 to F. culmorum strain TMW4.2043 (Axel et al., 2016); therefore, the antifungal activity of this LAB isolate could be fungi-specific. In addition, it is acknowledged that growth media and their components used in dual-culture assays may alter the antifungal activity of the test organism (Fiddaman and Rossall, 1994; Wang and Liu, 2008). The result of this assay carried out on a PDA medium can be further validated by carrying out the same assay on nutrient-limited media. These Petri dish assays did not examine the specific manner of antifungal activity, although previous work has noted the production of carboxylic acids by L. brevis R2Δ as the basis for the observed antifungal activity (Axel et al., 2016). These experiments agree with the study where L. reuteri M13 provided low to absent levels of fungal inhibition (Lynch et al., 2016). A possible reason for mycelium inhibition by the LAB examined could include the production of secondary metabolites which may directly inhibit mycelium spread as seen in other fungal pathogens (Allioui et al., 2016). Other explanations for mycelium inhibition could be the alteration of the growth media such as a change in pH and thus reducing its affinity to support mycelial growth. Alternatively, the LAB could potentially play a hyperparasitic role in the presence of Fusarium. Direct competition for space may also have led to a reduction in Fusarium growth needed to acquire nutrients from the media.
A modified detached leaf assay was used as an auxiliary study to quantify the antifungal potential of the selected agents while eliminating the effect of the growth media used in the dual-culture assay. The three aforesaid LAB which provided the most effective control of mycelial spread on dual culture presented similarly in the detached leaf assay. The reduction in differences in the antifungal activity between the best (L. brevis R2Δ) and worst (L. reuteri R29) performing isolate could be due to the removal of an artificial growth medium. The difference in the induction of host differences in leaves not seen in dual-culture assay may be due to the variation in the inherent potential of LABs to produce antifungal substances, such as cyclic dipeptides, proteinaceous compounds, organic acids, fatty acids, and reuterin (Crowley et al., 2013; Gajbhiye and Kapadnis, 2016). The biocontrol mechanisms of LAB can vary with strains in vitro but may have different performance in leaf surfaces. This means that all strains of LABs do not have the same potential to circumvent fungal spoilage owing to different capabilities to produce antifungal metabolites (Lačanin et al., 2017; Russo et al., 2017; Sadiq et al., 2019). The biocontrol mechanism of LAB strains is not limited to the antifungal activity, and it can extend up to interaction with fungal mycotoxins, resulting in inactivation or removal through cell wall binding (Blagojev et al., 2012; Ahlberg et al., 2015; Juodeikiene et al., 2018). The in vitro inhibitory mechanism of LAB strains PM411 and TC92 against Pseudomonas syringae pv. actinidiae in kiwifruit, Xanthomonas arboricola pv. pruni in Prunus and Xanthomonas fragariae in strawberry was reported to be pH-lowering effect and the production of lactic acid rather than the production of antifungal activity. However, both strains showed similar survival rates on leaf surfaces (Daranas Boadella et al., 2019). There are reports of LAB having antifungal activity but with variation. Recently, 10 different genotypes of Lactobacillus plantarum confirmed having antifungal activity, but all of them were genetically heterogeneous (Dong et al., 2017). In addition, the presence of naturally occurring saprophytes on detached leaves could have a synergistic effect when co-inoculated with the chosen LAB. Although these experiments did not examine the exact mode of inhibition, as a proxy to further trials they give ample evidence to show potential for in planta investigations. To allow for a clearer understanding of biocontrol agents, test fungus and plant, biofilm investigations along with whole leaf clearing and live–dead staining techniques could be explored (Bruzzese and Hasan, 1983; Bais et al., 2004).
Though there are more in vitro studies examining the potential of biocontrol agents in vivo, there is a need to find biocontrol agents that provide control at levels similar to or exceeding that of commercially available fungicides, and glasshouse studies were established (Palazzini et al., 2007; Matarese et al., 2012). To date, few studies have examined the relationship between LAB-mediated protection against FHB and mycotoxin accumulation (Oliveira et al., 2014; Baffoni et al., 2015; Zhao et al., 2017, 2019). In this study, LAB isolates of L. amylovorus DSM 20552, L. amylovorus DSM 20053, and L. brevis R2Δ provided evidence to be most suited as spray inoculations for the control of FHB in planta and reduction in the levels of mycotoxins on an individual basis. The main reason for these LAB isolates providing FHB resistance is the reduction in DON accumulation, the main virulence factor for Fusarium, inhibiting disease spread within barley heads (Sella et al., 2014; Gunupuru et al., 2017). Synergy studies have shown that when combined, LAB compounds have a higher relative antifungal activity (Niku-Paavola et al., 1999; Ndagano et al., 2011). Future glasshouse studies applying combinations of these isolates would offer more insight as to the potentially deleterious or beneficial effects co-inoculations might have on FHB control and mycotoxin moderation.
Previous studies have clearly shown that biocontrol agents alter plant defense systems upon host–agent contact (Khan et al., 2006; Palazzini et al., 2018; Köhl et al., 2019). The rough exploration of the changes in defense pathways was taken up in this study, as it may prove to be beneficial for rapid in vitro selection of future biocontrol agents (Conn et al., 2008). The gene expression of FgActin showed the increase in mRNA level of Fusarium indicating FHB development with time. But interestingly, L. amylovorus DSM 20552 and the mock were shown to significantly reduce levels of FgActin mRNA accumulation compared with that of MRS broth + F. graminearum-treated heads, suggesting the inhibition of FHB development. The HvICS1 transcript level increment was found progressively from 12 hpi to 120 hpi. This can be related to FHB development with time, and the increase in HvICS1 seems logical as HvICS1 is the important gene for SA (salicylic acid) synthesis and SA signaling has been proven to confer FHB resistance (Makandar et al., 2012; Hao et al., 2018; Thapa et al., 2018). This may suggest that HvICS1 plays a role in SA accumulation upon FHB infection in barley, which, in turn, confers a basal resistance to F. graminearum by modulating the accumulation of H2O2, O–2 and reactive oxygen-associated enzymatic activities (Antalová et al., 2020). The significant changes triggered by LAB treatments on the expression of HvCOI1 at 12 hpi (p < 0.05) do suggest toward the role of LAB in priming barley heads against FHB with time. This is because coronatine-insensitive protein 1(COI1) is a reported receptor of jasmonic acid (JA) signaling and has been known to play a crucial role in FHB resistance in plants (Makandar et al., 2010; Kazan and Gardiner, 2018; Srivastava et al., 2018).
To conclude, this study has explored the effectiveness of Lactobacillus isolates as biocontrol agents in vitro and under glasshouse conditions. Several isolates provide promising evidence with L. brevis R2Δ, L. amylovorus DSM 20552, and L. amylovorus FST 2.11 showing the most promise regarding mycelial spread in vitro, disease severity, and mycotoxin accumulation. Gene expression results add to the burgeoning library recording the underlying interactions between LAB biocontrol agents and their host, resulting in FHB resistance. This work provides a step forward in developing new biological approaches to combat important fungal pathogens during a time when available chemical controls are declining. These results show that LAB control of fungal pathogens is species-specific and caution must be noted with regard to variance in biocontrol properties if the pathogen changes. These LAB can be exploited for their antifungal activity and can be considered useful to control the pathogens and crop improvement. In summary, the results illustrate the potential for a multi-pronged approach to control FHB through direct fungal inhibition, toxin reduction, and host defense pathway induction. This provides an alternative stronghold leading to sustainable agriculture, green control of pathogens, food security, environment friendly, and circular bioeconomy.
Data Availability Statement
The original contributions presented in this study are included in the article/Supplementary Material, further inquiries can be directed to the corresponding author.
Author Contributions
JB obtained funding for the project. MB, GT, and JB designed all the experiments. JB and GT provided guidance during the experiment implementation. MB and GT carried out all the experiments. FD and JB reviewed the final manuscript. All authors contributed to the article and approved the submitted version.
Funding
We thank the Irish Department of Agriculture, Food, and Marine for funding provided under the Research Stimulus Fund (RSF) (Grant No. 11SF 317).
Conflict of Interest
The authors declare that the research was conducted in the absence of any commercial or financial relationships that could be construed as a potential conflict of interest.
Publisher’s Note
All claims expressed in this article are solely those of the authors and do not necessarily represent those of their affiliated organizations, or those of the publisher, the editors and the reviewers. Any product that may be evaluated in this article, or claim that may be made by its manufacturer, is not guaranteed or endorsed by the publisher.
Acknowledgments
We would like to thank the assistance of Elke Arendt and Claudia Axel, University College Cork, for the provision of the culture strains for laboratory, glasshouse, and field trials.
Supplementary Material
The Supplementary Material for this article can be found online at: https://www.frontiersin.org/articles/10.3389/fmicb.2022.912632/full#supplementary-material
References
Ahlberg, S. H., Joutsjoki, V., and Korhonen, H. J. (2015). Potential of lactic acid bacteria in aflatoxin risk mitigation. Int. J. Food Microbiol. 207, 87–102. doi: 10.1016/j.ijfoodmicro.2015.04.042
Ahmad, S., Wei, X., Sheng, Z., Hu, P., and Tang, S. (2020). CRISPR/Cas9 for development of disease resistance in plants: recent progress, limitations and future prospects. Brief. Funct. Genomics 19, 26–39. doi: 10.1093/bfgp/elz041
Ali, S. S., Gunupuru, L. R., Kumar, G., Khan, M., Scofield, S., Nicholson, P., et al. (2014). Plant disease resistance is augmented in uzu barley lines modified in the brassinosteroid receptor BRI1. BMC Plant Biol. 14:227. doi: 10.1186/s12870-014-0227-1
Allioui, N., Siah, A., Brinis, L., Reignault, P., and Halama, P. (2016). Identification of Qol fungicide-resistant genotypes of the wheat pathogen Zymoseptoria tritici in Algeria. Phytopathol. Mediterr. 55, 89–97.
Antalová, Z., Bleša, D., Martinek, P., and Matušinsky, P. (2020). Transcriptional analysis of wheat seedlings inoculated with Fusarium culmorum under continual exposure to disease defence inductors. PLoS One 15:e0224413. doi: 10.1371/journal.pone.0224413
Arendrup, M., Dzajic, E., Jensen, R., Johansen, H., Kjaldgaard, P., Knudsen, J., et al. (2013). Epidemiological changes with potential implication for antifungal prescription recommendations for fungaemia: data from a nationwide fungaemia surveillance programme. Clin. Microbiol. Infect. 19, e343–e353. doi: 10.1111/1469-0691.12212
Audenaert, K., Callewaert, E., Höfte, M., De Saeger, S., and Haesaert, G. (2010). Hydrogen peroxide induced by the fungicide prothioconazole triggers deoxynivalenol (DON) production by Fusarium graminearum. BMC Microbiol. 10:112. doi: 10.1186/1471-2180-10-112
Axel, C., Brosnan, B., Zannini, E., Peyer, L. C., Furey, A., Coffey, A., et al. (2016). Antifungal activities of three different Lactobacillus species and their production of antifungal carboxylic acids in wheat sourdough. Appl. Microbiol. Biotechnol. 100, 1701–1711. doi: 10.1007/s00253-015-7051-x
Baffoni, L., Gaggia, F., Dalanaj, N., Prodi, A., Nipoti, P., Pisi, A., et al. (2015). Microbial inoculants for the biocontrol of Fusarium spp. in durum wheat. BMC Microbiol. 15:242. doi: 10.1186/s12866-015-0573-7
Bais, H. P., Fall, R., and Vivanco, J. M. (2004). Biocontrol of Bacillus subtilis against infection of Arabidopsis roots by Pseudomonas syringae is facilitated by biofilm formation and surfactin production. Plant Physiol. 134, 307–319. doi: 10.1104/pp.103.028712
Beccari, G., Prodi, A., Tini, F., Bonciarelli, U., Onofri, A., Oueslati, S., et al. (2017). Changes in the Fusarium head blight complex of malting barley in a three-year field experiment in Italy. Toxins 9:120. doi: 10.3390/toxins9040120
Blagojev, N., Škrinjar, M., Vesković-Moraèanin, S., and Šošo, V. (2012). Control of mould growth and mycotoxin production by lactic acid bacteria metabolites. Rom. Biotechnol. Lett. 17, 7219–7226.
Blandino, M., Haidukowski, M., Pascale, M., Plizzari, L., Scudellari, D., and Reyneri, A. (2012). Integrated strategies for the control of Fusarium head blight and deoxynivalenol contamination in winter wheat. Field Crops Res. 133, 139–149. doi: 10.1016/j.fcr.2012.04.004
Bolechová, M., Benešová, K., Běláková, S., Čáslavský, J., Pospíchalová, M., and Mikulíková, R. (2015). Determination of seventeen mycotoxins in barley and malt in the Czech Republic. Food Control 47, 108–113. doi: 10.1016/j.foodcont.2014.06.045
Bottalico, A., and Perrone, G. (2002). Toxigenic Fusarium species and mycotoxins associated with head blight in small-grain cereals in Europe. Eur. J. Plant Pathol. 108, 611–624. doi: 10.1023/A:1020635214971
Brennan, J., Fagan, B., Van Maanen, A., Cooke, B., and Doohan, F. (2003). Studies on in vitro growth and pathogenicity of European Fusarium fungi. Eur. J. Plant Pathol. 109, 577–587. doi: 10.1023/A:1024712415326
Brown, N. A., Bass, C., Baldwin, T. K., Chen, H., Massot, F., Carion, P. W., et al. (2011). Characterisation of the Fusarium graminearum-wheat floral interaction. J. Pathog. 2011:626345. doi: 10.4061/2011/626345
Bruzzese, E., and Hasan, S. (1983). A whole leaf clearing and staining technique for host specificity studies of rust fungi. Plant Pathol. 32, 335–338. doi: 10.1111/j.1365-3059.1983.tb02841.x
Cantoro, R., Palazzini, J. M., Yerkovich, N., Miralles, D. J., and Chulze, S. N. (2021). Bacillus velezensis RC 218 as a biocontrol agent against Fusarium graminearum: effect on penetration, growth and TRI5 expression in wheat spikes. BioControl 66, 259–270. doi: 10.1007/s10526-020-10062-7
Chen, X., Steed, A., Harden, C., and Nicholson, P. (2006). Characterization of Arabidopsis thaliana–Fusarium graminearum interactions and identification of variation in resistance among ecotypes. Mol. Plant Pathol. 7, 391–403. doi: 10.1111/j.1364-3703.2006.00349.x
Chomczynski, P. (1993). A reagent for the single-step simultaneous isolation of RNA, DNA and proteins from cell and tissue samples. Biotechniques 15, 532–534, 536–537.
Comby, M., Gacoin, M., Robineau, M., Rabenoelina, F., Ptas, S., Dupont, J., et al. (2017). Screening of wheat endophytes as biological control agents against Fusarium head blight using two different in vitro tests. Microbiol. Res. 202, 11–20. doi: 10.1016/j.micres.2017.04.014
Conn, V., Walker, A., and Franco, C. (2008). Endophytic actinobacteria induce defense pathways in Arabidopsis thaliana. Mol. Plant Microbe Interact. 21, 208–218. doi: 10.1094/MPMI-21-2-0208
Cowger, C., Weisz, R., Arellano, C., and Murphy, P. (2016). Profitability of integrated management of Fusarium head blight in North Carolina winter wheat. Phytopathology 106, 814–823. doi: 10.1094/PHYTO-10-15-0263-R
Crowley, S., Mahony, J., and Van Sinderen, D. (2013). Current perspectives on antifungal lactic acid bacteria as natural bio-preservatives. Trends Food Sci. Technol. 33, 93–109. doi: 10.1016/j.tifs.2013.07.004
D’Angelo, D., Bradley, C., Ames, K., Willyerd, K., Madden, L., and Paul, P. (2014). Efficacy of fungicide applications during and after anthesis against Fusarium head blight and deoxynivalenol in soft red winter wheat. Plant Dis. 98, 1387–1397. doi: 10.1094/PDIS-01-14-0091-RE
Daranas Boadella, N., Roselló Prados, G., Cabrefiga Olamendi, J., Donati, I., Francés Ortega, J., Badosa Romañó, E., et al. (2019). Biological control of bacterial plant diseases with Lactobacillus plantarum strains selected for their broad-spectrum activity. Ann. Appl. Biol. 174, 92–105. doi: 10.1111/aab.12476
Dempsey, D. M. A., Vlot, A. C., Wildermuth, M. C., and Klessig, D. F. (2011). Salicylic acid biosynthesis and metabolism. Arabidopsis Book 9:e0156. doi: 10.1199/tab.0156
Dong, A.-R., Lo, R., Bansal, N., and Turner, M. S. (2017). A genetic diversity study of antifungal Lactobacillus plantarum isolates. Food Control 72, 83–89. doi: 10.1016/j.foodcont.2016.07.026
Ferdous, J., Li, Y., Reid, N., Langridge, P., Shi, B.-J., and Tricker, P. J. (2015). Identification of reference genes for quantitative expression analysis of microRNAs and mRNAs in barley under various stress conditions. PLoS One 10:e0118503. doi: 10.1371/journal.pone.0118503
Ferrigo, D., Raiola, A., and Causin, R. (2016). Fusarium toxins in cereals: occurrence, legislation, factors promoting the appearance and their management. Molecules 21:627. doi: 10.3390/molecules21050627
Fiddaman, P., and Rossall, S. (1994). Effect of substrate on the production of antifungal volatiles from Bacillus subtilis. J. Appl. Bacteriol. 76, 395–405. doi: 10.1111/j.1365-2672.1994.tb01646.x
Franco, T., Garcia, S., Hirooka, E., Ono, Y., and Dos Santos, J. (2011). Lactic acid bacteria in the inhibition of Fusarium graminearum and deoxynivalenol detoxification. J. Appl. Microbiol. 111, 739–748. doi: 10.1111/j.1365-2672.2011.05074.x
Gajbhiye, M. H., and Kapadnis, B. P. (2016). Antifungal-activity-producing lactic acid bacteria as biocontrol agents in plants. Biocontrol Sci. Technol. 26, 1451–1470. doi: 10.1080/09583157.2016.1213793
Gallo, A., Giuberti, G., Frisvad, J. C., Bertuzzi, T., and Nielsen, K. F. (2015). Review on mycotoxin issues in ruminants: occurrence in forages, effects of mycotoxin ingestion on health status and animal performance and practical strategies to counteract their negative effects. Toxins 7, 3057–3111. doi: 10.3390/toxins7083057
Gómez-Lama Cabanás, C., Fernández-González, A. J., Cardoni, M., Valverde-Corredor, A., López-Cepero, J., Fernández-López, M., et al. (2021). The banana root endophytome: differences between mother plants and suckers and evaluation of selected bacteria to control Fusarium oxysporum f.sp. cubense. J. Fungi 7:194. doi: 10.3390/jof7030194
Goswami, R. S., and Kistler, H. C. (2004). Heading for disaster: Fusarium graminearum on cereal crops. Mol. Plant Pathol. 5, 515–525. doi: 10.1111/j.1364-3703.2004.00252.x
Gunupuru, L., Perochon, A., and Doohan, F. (2017). Deoxynivalenol resistance as a component of FHB resistance. Trop. Plant Pathol. 42, 175–183. doi: 10.1007/s40858-017-0147-3
Gunupuru, L. R., Arunachalam, C., Malla, K. B., Kahla, A., Perochon, A., Jia, J., et al. (2018). A wheat cytochrome P450 enhances both resistance to deoxynivalenol and grain yield. PLoS One 13:e0204992. doi: 10.1371/journal.pone.0204992
Haidukowski, M., Pascale, M., Perrone, G., Pancaldi, D., Campagna, C., and Visconti, A. (2005). Effect of fungicides on the development of Fusarium head blight, yield and deoxynivalenol accumulation in wheat inoculated under field conditions with Fusarium graminearum and Fusarium culmorum. J. Sci. Food Agric. 85, 191–198. doi: 10.1002/jsfa.1965
Hamed, H. A., Moustafa, Y. A., and Abdel-Aziz, S. M. (2011). In vivo efficacy of lactic acid bacteria in biological control against Fusarium oxysporum for protection of tomato plant. Life Sci. J. 8, 462–468.
Hao, Q., Wang, W., Han, X., Wu, J., Lyu, B., Chen, F., et al. (2018). Isochorismate-based salicylic acid biosynthesis confers basal resistance to Fusarium graminearum in barley. Mol. Plant Pathol. 19, 1995–2010. doi: 10.1111/mpp.12675
He, Y., Zhang, X., Zhang, Y., Ahmad, D., Wu, L., Jiang, P., et al. (2018). Molecular characterization and expression of PFT, an FHB resistance gene at the Fhb1 QTL in wheat. Phytopathology 108, 730–736. doi: 10.1094/PHYTO-11-17-0383-R
Hückelhoven, R., Hofer, K., Coleman, A., and Heß, M. (2018). Fusarium infection of malting barley has to be managed over the entire value chain. J. Plant Dis. Protect. 125, 1–4. doi: 10.1007/s41348-017-0101-0
Jamal, Q., Cho, J.-Y., Moon, J.-H., and Kim, K. Y. (2017). Purification and antifungal characterization of cyclo (D-Pro-L-Val) from Bacillus amyloliquefaciens Y1 against Fusarium graminearum to control head blight in wheat. Biocatal. Agric. Biotechnol. 10, 141–147. doi: 10.1016/j.bcab.2017.01.003
Jeger, M., and Viljanen-Rollinson, S. (2001). The use of the area under the disease-progress curve (AUDPC) to assess quantitative disease resistance in crop cultivars. Theor. Appl. Genet. 102, 32–40. doi: 10.1007/s001220051615
Jones, R. (2000). Assessments of Fusarium head blight of wheat and barley in response to fungicide treatment. Plant Dis. 84, 1021–1030. doi: 10.1094/PDIS.2000.84.9.1021
Juodeikiene, G., Bartkiene, E., Cernauskas, D., Cizeikiene, D., Zadeike, D., Lele, V., et al. (2018). Antifungal activity of lactic acid bacteria and their application for Fusarium mycotoxin reduction in malting wheat grains. Lwt 89, 307–314. doi: 10.1016/j.lwt.2017.10.061
Kage, U., Yogendra, K. N., and Kushalappa, A. C. (2017). TaWRKY70 transcription factor in wheat QTL-2DL regulates downstream metabolite biosynthetic genes to resist Fusarium graminearum infection spread within spike. Sci. Rep. 7:42596. doi: 10.1038/srep42596
Karre, S., Kumar, A., Yogendra, K., Kage, U., Kushalappa, A., and Charron, J.-B. (2019). HvWRKY23 regulates flavonoid glycoside and hydroxycinnamic acid amide biosynthetic genes in barley to combat Fusarium head blight. Plant Mol. Biol. 100, 591–605. doi: 10.1007/s11103-019-00882-2
Kazan, K., and Gardiner, D. M. (2018). Transcriptomics of cereal–Fusarium graminearum interactions: what we have learned so far. Mol. Plant Pathol. 19, 764–778. doi: 10.1111/mpp.12561
Kedia, G., Wang, R., Patel, H., and Pandiella, S. S. (2007). Use of mixed cultures for the fermentation of cereal-based substrates with potential probiotic properties. Process Biochem. 42, 65–70.
Khan, M. R., Fischer, S., Egan, D., and Doohan, F. M. (2006). Biological control of Fusarium seedling blight disease of wheat and barley. Phytopathology 96, 386–394. doi: 10.1094/PHYTO-96-0386
Koch, A., Biedenkopf, D., Furch, A., Weber, L., Rossbach, O., Abdellatef, E., et al. (2016). An RNAi-based control of Fusarium graminearum infections through spraying of long dsRNAs involves a plant passage and is controlled by the fungal silencing machinery. PLoS Pathog. 12:e1005901. doi: 10.1371/journal.ppat.1005901
Koch, A., Kumar, N., Weber, L., Keller, H., Imani, J., and Kogel, K.-H. (2013). Host-induced gene silencing of cytochrome P450 lanosterol C14α-demethylase–encoding genes confers strong resistance to Fusarium species. Proc. Natl. Acad. Sci. U.S.A. 110, 19324–19329. doi: 10.1073/pnas.1306373110
Köhl, J., Kolnaar, R., and Ravensberg, W. J. (2019). Mode of action of microbial biological control agents against plant diseases: relevance beyond efficacy. Front. Plant Sci. 10:845. doi: 10.3389/fpls.2019.00845
Lačanin, I., Mounier, J., Pawtowski, A., Dušková, M., Kameník, J., and Karpíšková, R. (2017). Assessment of the antifungal activity of Lactobacillus and Pediococcus spp. for use as bioprotective cultures in dairy products. World J. Microbiol. Biotechnol. 33:188. doi: 10.1007/s11274-017-2354-y
Legzdina, L., Bleidere, M., Usele, G., Vilcane, D., Beinarovica, I., Mezaka, I., et al. (2013). “Phenotypic evaluation of spring barley RIL mapping populations for pre-harvest sprouting, Fusarium head blight and β-glucans,” in Advance in Barley Sciences, eds G. Zhang, C. Li, and X. Liu (Dordrecht: Springer), 441–452.
Legzdina, L., and Buerstmayr, H. (2004). Comparison of infection with Fusarium head blight and accumulation of mycotoxins in grain of hulless and covered barley. J. Cereal Sci. 40, 61–67.
Livak, K. J., and Schmittgen, T. D. (2001). Analysis of relative gene expression data using real-time quantitative PCR and the 2−ΔΔCT method. Methods 25, 402–408. doi: 10.1006/meth.2001.1262
Lowe, D. P., and Arendt, E. K. (2004). The use and effects of lactic acid bacteria in malting and brewing with their relationships to antifungal activity, mycotoxins and gushing: a review. J. Inst. Brew. 110, 163–180. doi: 10.1002/j.2050-0416.2004.tb00199.x
Lynch, K., Zannini, E., Guo, J., Axel, C., Arendt, E., Kildea, S., et al. (2016). Control of Zymoseptoria tritici cause of septoria tritici blotch of wheat using antifungal Lactobacillus strains. J. Appl. Microbiol. 121, 485–494. doi: 10.1111/jam.13171
Magan, N., Hope, R., Colleate, A., and Baxter, E. (2002). Relationship between growth and mycotoxin production by Fusarium species, biocides and environment. Eur. J. Plant Pathol. 108, 685–690. doi: 10.1023/A:1020618728175
Magnusson, J., and Schnürer, J. (2001). Lactobacillus coryniformis subsp. coryniformis strain Si3 produces a broad-spectrum proteinaceous antifungal compound. Appl. Environ. Microbiol. 67, 1–5. doi: 10.1128/AEM.67.1.1-5.2001
Makandar, R., Nalam, V., Chaturvedi, R., Jeannotte, R., Sparks, A. A., and Shah, J. (2010). Involvement of salicylate and jasmonate signaling pathways in Arabidopsis interaction with Fusarium graminearum. Mol. Plant Microbe Interact. 23, 861–870. doi: 10.1094/MPMI-23-7-0861
Makandar, R., Nalam, V. J., Lee, H., Trick, H. N., Dong, Y., and Shah, J. (2012). Salicylic acid regulates basal resistance to Fusarium head blight in wheat. Mol. Plant Microbe Interact. 25, 431–439. doi: 10.1094/MPMI-09-11-0232
Matarese, F., Sarrocco, S., Gruber, S., Seidl-Seiboth, V., and Vannacci, G. (2012). Biocontrol of Fusarium head blight: interactions between Trichoderma and mycotoxigenic Fusarium. Microbiology 158, 98–106. doi: 10.1099/mic.0.052639-0
Matny, O. (2015). Fusarium head blight and crown rot on wheat & barley: losses and health risks. Adv. Plants Agric. Res. 2:00039. doi: 10.15406/apar.2015.02.00039
McCallum, B., and Tekauz, A. (2002). Influence of inoculation method and growth stage on fusarium head blight in barley. Can. J. Plant Pathol. 24, 77–80. doi: 10.1080/07060660109506976
McMullen, M., Bergstrom, G., De Wolf, E., Dill-Macky, R., Hershman, D., Shaner, G., et al. (2012). A unified effort to fight an enemy of wheat and barley: Fusarium head blight. Plant Dis. 96, 1712–1728. doi: 10.1094/PDIS-03-12-0291-FE
McMullen, M., Jones, R., and Gallenberg, D. (1997). Scab of wheat and barley: a re-emerging disease of devastating impact. Plant Dis. 81, 1340–1348. doi: 10.1094/PDIS.1997.81.12.1340
Meroth, C. B., Walter, J., Hertel, C., Brandt, M. J., and Hammes, W. P. (2003). Monitoring the bacterial population dynamics in sourdough fermentation processes by using PCR-denaturing gradient gel electrophoresis. Appl. Environ. Microbiol. 69, 475–482. doi: 10.1128/AEM.69.1.475-482.2003
Miedaner, T., Schmid, J. E., Flath, K., Koch, S., Jacobi, A., Ebmeyer, E., et al. (2018). A multiple disease test for field-based phenotyping of resistances to Fusarium head blight, yellow rust and stem rust in wheat. Eur. J. Plant Pathol. 151, 451–461. doi: 10.1007/s10658-017-1386-3
Mittholiya, S., Chittora, D., and Sharma, K. (2020). “Role of lactic acid bacteria as biological control agent,” in Innovative Research on Science, Humanities, Engineering & Management, eds R. Shyam and R. K. Kaswan (Paris: RFI), 21.
Murphy, B. R. (2016). Unlocking the Potential of Endophytes in Cereal Crops. Doctoral thesis. Dublin: Trinity College of Dublin.
Nakkarach, A., and Withayagiat, U. (2018). Comparison of synbiotic beverages produced from riceberry malt extract using selected free and encapsulated probiotic lactic acid bacteria. Agric. Nat. Resour. 52, 467–476. doi: 10.1016/j.anres.2018.11.013
Ndagano, D., Lamoureux, T., Dortu, C., Vandermoten, S., and Thonart, P. (2011). Antifungal activity of 2 lactic acid bacteria of the Weissella genus isolated from food. J. Food Sci. 76, M305–M311. doi: 10.1111/j.1750-3841.2011.02257.x
Nielsen, L., Cook, D., Edwards, S., and Ray, R. (2014). The prevalence and impact of Fusarium head blight pathogens and mycotoxins on malting barley quality in UK. Int. J. Food Microbiol. 179, 38–49. doi: 10.1016/j.ijfoodmicro.2014.03.023
Niku-Paavola, M. L., Laitila, A., Mattila-Sandholm, T., and Haikara, A. (1999). New types of antimicrobial compounds produced by Lactobacillus plantarum. J. Appl. Microbiol. 86, 29–35. doi: 10.1046/j.1365-2672.1999.00632.x
Oliveira, P. M., Zannini, E., and Arendt, E. K. (2014). Cereal fungal infection, mycotoxins, and lactic acid bacteria mediated bioprotection: from crop farming to cereal products. Food Microbiol. 37, 78–95. doi: 10.1016/j.fm.2013.06.003
Palazzini, J., Roncallo, P., Cantoro, R., Chiotta, M., Yerkovich, N., Palacios, S., et al. (2018). Biocontrol of Fusarium graminearum sensu stricto, reduction of deoxynivalenol accumulation and phytohormone induction by two selected antagonists. Toxins 10:88. doi: 10.3390/toxins10020088
Palazzini, J. M., Dunlap, C. A., Bowman, M. J., and Chulze, S. N. (2016). Bacillus velezensis RC 218 as a biocontrol agent to reduce Fusarium head blight and deoxynivalenol accumulation: genome sequencing and secondary metabolite cluster profiles. Microbiol. Res. 192, 30–36. doi: 10.1016/j.micres.2016.06.002
Palazzini, J. M., Ramirez, M. L., Torres, A. M., and Chulze, S. N. (2007). Potential biocontrol agents for Fusarium head blight and deoxynivalenol production in wheat. Crop Prot. 26, 1702–1710. doi: 10.1016/j.cropro.2007.03.004
Parry, D., Jenkinson, P., and Mcleod, L. (1995). Fusarium ear blight (scab) in small grain cereals—a review. Plant Pathol. 44, 207–238. doi: 10.1111/j.1365-3059.1995.tb02773.x
Paul, P., Lipps, P., Hershman, D., Mcmullen, M., Draper, M., and Madden, L. (2008). Efficacy of triazole-based fungicides for Fusarium head blight and deoxynivalenol control in wheat: a multivariate meta-analysis. Phytopathology 98, 999–1011. doi: 10.1094/PHYTO-98-9-0999
Paul, P. A., Bradley, C. A., Madden, L. V., Dalla Lana, F., Bergstrom, G. C., Dill-Macky, R., et al. (2018). Effects of pre-and postanthesis applications of demethylation inhibitor fungicides on Fusarium head blight and deoxynivalenol in spring and winter wheat. Plant Dis. 102, 2500–2510. doi: 10.1094/PDIS-03-18-0466-RE
Petchkongkaew, A., Taillandier, P., Gasaluck, P., and Lebrihi, A. (2008). Isolation of Bacillus spp. from Thai fermented soybean (Thua-nao): screening for aflatoxin B1 and ochratoxin A detoxification. J. Appl. Microbiol. 104, 1495–1502. doi: 10.1111/j.1365-2672.2007.03700.x
Peyer, L. C., Axel, C., Lynch, K. M., Zannini, E., Jacob, F., and Arendt, E. K. (2016). Inhibition of Fusarium culmorum by carboxylic acids released from lactic acid bacteria in a barley malt substrate. Food Control 69, 227–236. doi: 10.1016/j.foodcont.2016.05.010
Placinta, C., D’mello, J. F., and Macdonald, A. (1999). A review of worldwide contamination of cereal grains and animal feed with Fusarium mycotoxins. Anim. Feed Sci. Technol. 78, 21–37. doi: 10.1016/S0377-8401(98)00278-8
Pontonio, E., Di Cagno, R., Tarraf, W., Filannino, P., De Mastro, G., and Gobbetti, M. (2018). Dynamic and assembly of epiphyte and endophyte lactic acid bacteria during the life cycle of Origanum vulgare L. Front. Microbiol. 9:1372. doi: 10.3389/fmicb.2018.01372
Pretty, J., and Bharucha, Z. P. (2015). Integrated pest management for sustainable intensification of agriculture in Asia and Africa. Insects 6, 152–182. doi: 10.3390/insects6010152
Rathore, S., Salmerón, I., and Pandiella, S. S. (2012). Production of potentially probiotic beverages using single and mixed cereal substrates fermented with lactic acid bacteria cultures. Food Microbiol. 30, 239–244. doi: 10.1016/j.fm.2011.09.001
Rudd, J. J., Keon, J., and Hammond-Kosack, K. E. (2008). The wheat mitogen-activated protein kinases TaMPK3 and TaMPK6 are differentially regulated at multiple levels during compatible disease interactions with Mycosphaerella graminicola. Plant Physiol. 147, 802–815. doi: 10.1104/pp.108.119511
Russo, P., Arena, M. P., Fiocco, D., Capozzi, V., Drider, D., and Spano, G. (2017). Lactobacillus plantarum with broad antifungal activity: a promising approach to increase safety and shelf-life of cereal-based products. Int. J. Food Microbiol. 247, 48–54. doi: 10.1016/j.ijfoodmicro.2016.04.027
Sadiq, F. A., Yan, B., Tian, F., Zhao, J., Zhang, H., and Chen, W. (2019). Lactic acid bacteria as antifungal and anti-mycotoxigenic agents: a comprehensive review. Compr. Rev. Food Sci. Food Saf. 18, 1403–1436. doi: 10.1111/1541-4337.12481
Scherm, B., Balmas, V., Spanu, F., Pani, G., Delogu, G., Pasquali, M., et al. (2013). Fusarium culmorum: causal agent of foot and root rot and head blight on wheat. Mol. Plant Pathol. 14, 323–341. doi: 10.1111/mpp.12011
Schnürer, J., and Magnusson, J. (2005). Antifungal lactic acid bacteria as biopreservatives. Trends Food Sci. Technol. 16, 70–78.
Schoonbeek, H. J., Wang, H. H., Stefanato, F. L., Craze, M., Bowden, S., Wallington, E., et al. (2015). Arabidopsis EF-Tu receptor enhances bacterial disease resistance in transgenic wheat. New Phytol. 206, 606–613. doi: 10.1111/nph.13356
Sella, L., Gazzetti, K., Castiglioni, C., Schäfer, W., and Favaron, F. (2014). Fusarium graminearum possesses virulence factors common to Fusarium head blight of wheat and seedling rot of soybean but differing in their impact on disease severity. Phytopathology 104, 1201–1207. doi: 10.1094/PHYTO-12-13-0355-R
Sharma, A., Diwevidi, V., Singh, S., Pawar, K. K., Jerman, M., Singh, L., et al. (2013). Biological control and its important in agriculture. Int. J. Biotechnol. Bioeng. Res. 4, 175–180.
Shi, S., Zhao, J., Pu, L., Sun, D., Han, D., Li, C., et al. (2020). Identification of new sources of resistance to crown rot and Fusarium head blight in Wheat. Plant Dis. 104, 1979–1985. doi: 10.1094/PDIS-10-19-2254-RE
Siedler, S., Balti, R., and Neves, A. R. (2019). Bioprotective mechanisms of lactic acid bacteria against fungal spoilage of food. Curr. Opin. Biotechnol. 56, 138–146.
Simpson, D. R., Weston, G. E., Turner, J. A., Jennings, P., and Nicholson, P. (2001). Differential control of head blight pathogens of wheat by fungicides and consequences for mycotoxin contamination of grain. Eur. J. Plant Pathol. 107, 421–431.
Smiley, R. W., Gourlie, J. A., Easley, S. A., and Patterson, L.-M. (2005). Pathogenicity of fungi associated with the wheat crown rot complex in Oregon and Washington. Plant Dis. 89, 949–957. doi: 10.1094/PD-89-0949
Srivastava, A. K., Orosa, B., Singh, P., Cummins, I., Walsh, C., Zhang, C., et al. (2018). SUMO suppresses the activity of the jasmonic acid receptor CORONATINE INSENSITIVE1. Plant Cell 30, 2099–2115. doi: 10.1105/tpc.18.00036
Talas, F., and McDonald, B. A. (2015). Genome-wide analysis of Fusarium graminearum field populations reveals hotspots of recombination. BMC Genomics 16:996. doi: 10.1186/s12864-015-2166-0
Tanaka, S., Ichikawa, A., Yamada, K., Tsuji, G., Nishiuchi, T., Mori, M., et al. (2010). HvCEBiP, a gene homologous to rice chitin receptor CEBiP, contributes to basal resistance of barley to Magnaporthe oryzae. BMC Plant Biol. 10:288. doi: 10.1186/1471-2229-10-288
Thapa, G., Gunupuru, L. R., Hehir, J. G., Kahla, A., Mullins, E., and Doohan, F. M. (2018). A pathogen-responsive leucine rich receptor like kinase contributes to Fusarium resistance in cereals. Front. Plant Sci. 9:867. doi: 10.3389/fpls.2018.00867
Tian, Y., Tan, Y., Liu, N., Liao, Y., Sun, C., Wang, S., et al. (2016). Functional agents to biologically control deoxynivalenol contamination in cereal grains. Front. Microbiol. 7:395. doi: 10.3389/fmicb.2016.00395
Tibola, C. S., Fernandes, J. M. C., and Guarienti, E. M. (2016). Effect of cleaning, sorting and milling processes in wheat mycotoxin content. Food Control 60, 174–179. doi: 10.4315/0362-028X-47.6.489
Trujillo, M., Ichimura, K., Casais, C., and Shirasu, K. (2008). Negative regulation of PAMP-triggered immunity by an E3 ubiquitin ligase triplet in Arabidopsis. Curr. Biol. 18, 1396–1401. doi: 10.1016/j.cub.2008.07.085
Umego, E. C., and Barry-Ryan, C. (2022). Overview of the Irish brewing and distilling sector: processing inputs supply and quality requirements. BrewingScience 75, 9–16.
Union, E. (2009). Regulation (EC) No 1107/2009 of the European parliament and of the council of 21 October 2009 concerning the placing of plant protection products on the market and repealing council directives 79/117/EEC and 91/414/EEC. Off. J. Eur. Union 52, 1–50.
Wachowska, U., and Głowacka, K. (2014). Antagonistic interactions between Aureobasidium pullulans and Fusarium culmorum, a fungal pathogen of winter wheat. BioControl 59, 635–645.
Walter, S., Brennan, J. M., Arunachalam, C., Ansari, K. I., Hu, X., Khan, M. R., et al. (2008). Components of the gene network associated with genotype-dependent response of wheat to the Fusarium mycotoxin deoxynivalenol. Funct. Integr. Genomics 8, 421–427. doi: 10.1007/s10142-008-0089-4
Wang, L.-Y., Xie, Y.-S., Cui, Y.-Y., Xu, J., He, W., Chen, H.-G., et al. (2015). Conjunctively screening of biocontrol agents (BCAs) against fusarium root rot and fusarium head blight caused by Fusarium graminearum. Microbiol. Res. 177, 34–42. doi: 10.1016/j.micres.2015.05.005
Wang, Z.-W., and Liu, X.-L. (2008). Medium optimization for antifungal active substances production from a newly isolated Paenibacillus sp. using response surface methodology. Bioresour. Technol. 99, 8245–8251. doi: 10.1016/j.biortech.2008.03.039
Wegulo, S. N., Baenziger, P. S., Nopsa, J. H., Bockus, W. W., and Hallen-Adams, H. (2015). Management of Fusarium head blight of wheat and barley. Crop Prot. 73, 100–107.
Willyerd, K., Li, C., Madden, L., Bradley, C., Bergstrom, G., Sweets, L., et al. (2012). Efficacy and stability of integrating fungicide and cultivar resistance to manage Fusarium head blight and deoxynivalenol in wheat. Plant Dis. 96, 957–967.
Xue, A. G., Chen, Y., Voldeng, H. D., Fedak, G., Savard, M. E., Längle, T., et al. (2014). Concentration and cultivar effects on efficacy of CLO-1 biofungicide in controlling Fusarium head blight of wheat. Biol. Control 73, 2–7.
Zadoks, J. C., Chang, T. T., and Konzak, C. F. (1974). A decimal code for the growth stages of cereals. Weed Res. 14, 415–421.
Zhang, Y., Cheng, Y. T., Qu, N., Zhao, Q., Bi, D., and Li, X. (2006). Negative regulation of defense responses in Arabidopsis by two NPR1 paralogs. Plant J. 48, 647–656. doi: 10.1111/j.1365-313X.2006.02903.x
Zhao, H., Vegi, A., and Wolf-Hall, C. (2017). Screening of lactic acid bacteria for anti-Fusarium activity and optimization of incubation conditions. J. Food Prot. 80, 1648–1656. doi: 10.4315/0362-028X.JFP-17-100
Keywords: biological control, Fusarium head blight, lactic acid bacteria, barley (Hordeum vulgare L.), pathogenesis-related (PR) genes
Citation: Byrne MB, Thapa G, Doohan FM and Burke JI (2022) Lactic Acid Bacteria as Potential Biocontrol Agents for Fusarium Head Blight Disease of Spring Barley. Front. Microbiol. 13:912632. doi: 10.3389/fmicb.2022.912632
Received: 04 April 2022; Accepted: 07 June 2022;
Published: 22 July 2022.
Edited by:
Xiaodan Wang, China Agricultural University, ChinaReviewed by:
Fuguo Xing, Institute of Food Science and Technology (CAAS), ChinaM. Y. Sreenivasa, University of Mysore, India
Copyright © 2022 Byrne, Thapa, Doohan and Burke. This is an open-access article distributed under the terms of the Creative Commons Attribution License (CC BY). The use, distribution or reproduction in other forums is permitted, provided the original author(s) and the copyright owner(s) are credited and that the original publication in this journal is cited, in accordance with accepted academic practice. No use, distribution or reproduction is permitted which does not comply with these terms.
*Correspondence: James I. Burke, amltbXkuYnVya2VAdWNkLmll