- School of Marine Science, Ningbo University, Ningbo, China
Freshwater gastropods are widely distributed and play an important role in aquatic ecosystems. Symbiotic microorganisms represented by gut microbes can affect the physiological and biochemical activities of their hosts. However, few studies have investigated the response of the gut microbial community of snails to environmental stress. In this study, the dynamics of the gut microbiota of the gastropod Bellamya aeruginosa were tracked to explore their responses in terms of their composition and function to cyanobacterial bloom. Differences in gut microbial community structures during periods of non-cyanobacterial bloom and cyanobacterial bloom were determined. Results showed that the alpha diversity of the gut microbiota exposed to cyanobacterial bloom was lower than that of the gut microbiota exposed to non-cyanobacterial bloom. The main genera differentiating the two periods were Faecalibacterium, Subdoligranulum, Ralstonia, and Pelomonas. Microcystins (MCs) and water temperature (WT) were the primary factors influencing the gut microbial community of B. aeruginosa; between them, the influence of MCs was greater than that of WT. Fourteen pathways (level 2) were notably different between the two periods. The pathways of carbohydrate metabolism, immune system, environmental adaptation, and xenobiotics biodegradation and metabolism in these differential pathways exhibited a strong linear regression relationship with MCs and WT. Changes in the functions of the gut microbiota may help B. aeruginosa meet its immunity and energy needs during cyanobacterial bloom stress. These results provide key information for understanding the response pattern of freshwater snail intestinal flora to cyanobacterial blooms and reveal the underlying environmental adaptation mechanism of gastropods from the perspective of intestinal flora.
Introduction
With the sustained intensification of anthropogenic activities, global climate warming and eutrophication have provoked the widespread occurrence of cyanobacterial blooms in various water bodies worldwide (Paerl, 2018). The increasing frequency, magnitude, and duration of cyanobacterial blooms not only seriously disrupt aquatic ecosystem functions but also pose a major threat to the survival of aquatic animals through the release of cyanotoxins (Huisman et al., 2018). Owing to the high spatial overlap between the cyanobacterial scum accumulation zones and the main activity areas of gastropods during cyanobacterial blooms, and because the major bloom season also coincides with the breeding season of gastropods (Lance et al., 2010), the interactions between cyanobacterial blooms and freshwater snails are inevitable.
Cyanotoxins, represented by microcystins (MCs), may accumulate in the various tissues of snails through intestinal (consumption) or gill/skin (absorption) epithelia (Massey et al., 2018), resulting in a series of adverse reactions in gastropods (Bownik, 2016). Numerous studies have investigated the response of freshwater snails to cyanobacterial blooms and their toxins from multiple layers and perspectives, including biochemistry and histopathology (Zhu et al., 2011), transcriptomics (Qiao et al., 2018a), metabolomics (Yang M. J. et al., 2022), development (Lei et al., 2017), fecundity (Qiao et al., 2018b), feeding ecology (Qiu et al., 2017), and community dynamics (Gérard and Lance, 2019) and structure (Gérard et al., 2009). However, almost all of these studies have focused on the snails themselves and have failed to consider the dynamics and functions of their symbiotic microbes when exposed to cyanobacterial blooms.
With the rapid development of high-throughput sequencing technology, we can effectively and comprehensively gain insights into the composition and dynamics of symbiotic bacteria associated with gastropods, especially gut microbiota (Hu et al., 2018; Li et al., 2019; Hao et al., 2020). Gut microbes not only provide various key digestive enzymes for the snail host (Brendelberger, 1997; Ito et al., 2019) but also strongly affect many physiological processes, such as uric acid oxidation (Van Horn et al., 2012), cold hardiness (Nicolai et al., 2015), and amino acid and energy metabolism (Grandiosa et al., 2018), which were once thought to depend only on the gastropod host genome. Some gut microbes can even adhere to intestinal mucosa and stimulate immune response that is mediated by the regulation of the expression of Toll-like receptors in snail intestinal mucosa (Dushku et al., 2019). Moreover, unlike the largely static host genome, the symbiotic microbiome is highly plastic and can rapidly respond to changes in host diet or environmental conditions through changes in community composition, mutations, exchange of genetic material with bacteria from the environment, or changes in gene expression (Shapira, 2016), as well as enhance host adaptation to the fast and drastic environmental changes by influencing various physiological activities of the host (Gilbert et al., 2015). Thus, the gut microbiota is an important source of metabolic flexibility for the host, and it might be a key, yet understudied, factor in tolerance and adaptation to environmental stress (Macke et al., 2017).
Bellamya aeruginosa is an indigenous freshwater snail in China. It mainly lives in the littoral zones of rivers and lakes and plays a key role in aquatic ecosystems as an important link between primary producers and secondary consumers (Gong et al., 2009). Owing to its high sensitivity to environmental toxins and strong enrichment ability of pollutants, it is considered as an ideal indicator of ecosystem health, and it has been recommended as a model organism for freshwater ecotoxicology studies (Ma et al., 2010). As far as we know, the composition and dynamic characteristics of the gut microbiota of Bellamya have not been described nor have their response pattern and mechanism to environmental stress been reported. Characterizing the responses of gut microbiota to cyanobacterial bloom will help us understand how changing environmental conditions can influence symbiotic microbes and reveal the mechanisms of gastropod-microbiome interactions. The aim of this study was to determine how the intestinal bacterial community and its function change during the snail host exposure to toxic cyanobacterial bloom. We investigated monthly variation in the gut microbiota of B. aeruginosa in a water body experiencing cyanobacterial bloom during the activity period of Bellamya to address this question and test the following hypotheses: (1) the composition and function of gut microbiota are expected to be notably different between periods of non-cyanobacterial bloom and cyanobacterial bloom; (2) the dynamic responses of the gut microbiota in B. aeruginosa are related to environmental conditions and the degree of MCs accumulation.
Materials and Methods
Investigation Site and Sample Collection
B. aeruginosa individuals were collected by hand picking from the littoral zone of a eutrophic shallow pond (ca. 0.35 ha, ca. 1.5 m deep, 29.91°N, 121.64°E) at Ningbo, eastern China. Although the pond has undergone several ecological remediations (dredging and macrophyte restoration), cyanobacteria-dominant (mainly Microcystis spp.) algal blooms still frequently occur. Snail samples (three individuals) and water samples were collected every month during the activity period of Bellamya (from March to November 2020). Then, the snail samples were individually placed in a plastic container with sterile water and transported to the laboratory. After scrubbing their surface with 70% ethanol, their shells were carefully crushed, and then their intestines and hepatopancreases were dissected for gut microbiota studies and MCs content analysis, respectively.
Environmental Analysis
Water temperature (WT), pH, and dissolved oxygen (DO) were recorded in situ by using a multi-probe (YSI 6000, YSI Inc., Yellow Springs, USA). Secchi depth (SD) was estimated by a 0.2 m diameter black-white Secchi disk. Levels of nitrite (NO2-N), nitrate (NO3-N), ammonium (NH4-N), orthophosphate (PO4-P), total nitrogen (TN) and total phosphorus (TP) were analyzed following standard methods by using an automated spectrophotometer (Smart-Chem 200 Discrete Analyzer, Westco Scientific Instruments, Brookfield, USA). Concentration of chlorophyll a (Chl-a) was measured via the hot ethanol method (Chen, 2006).
Phytoplankton Analysis
Phytoplankton sample (ca. 250 mL) was obtained from the pond each month and immediately fixed with 1% Lugol's iodine solution. Taxa were counted in sedimentation chambers (Hydro-Bios Apparatebau GmbH Kiel, Germany) by using an inverted microscope (CK2, Olympus Corporation, Tokyo, Japan) according to quantitative Utermöhl method (Utermöhl, 1958). Phytoplankton biomass was calculated volumetrically through the OptiCount software (SequentiX, Klein Raden, Germany). The specific density of phytoplankton cells was assumed to be 1 g cm−3 (wet weight).
Quantification of MCs
The hepatopancreases were weighed before and after lyophilization. Microcystin extraction was conducted as previously described by Yang W. et al. (2022). MCs concentrations in the hepatopancreases were determined by the immunoassay method by using an ELISA test kit (sensitivity: 0.1 μg/L) with a detection range of 0.1–2 μg/L microcystin (Beacon Analytical Systems, Portland, ME, USA) following the manufacturers' instructions. The Beacon Microcystin Plate Kit was not available for the identification of microcystin variants. Thus, MCs concentration was expressed as the equivalent of MC-LR.
Recoveries of MCs extracted and matrix effects were determined by spiking with MC-LR standard (3 μg/g dry weight, purity > 98%; MedChemExpress, Monmouth Junction, NJ, USA) (Zhang et al., 2016). The response was compared with 100% methanol spiked with the same amount. The efficiency of MCs extraction was 91.6%. Matrix effects were negligible for the hepatopancreases of the snails (4.3% of differences between matrix and methanol results). Owing to the high recoveries of MCs extracted and the low matrix effects of the assay methods, the data on MCs contents in the present study were the actual determined data, without any additional calculation using recoveries and matrix effects.
DNA Extraction, Amplification, and Pyrosequencing
Snail intestinal genomic DNA was extracted from individual snails following the standard DNA extraction procedure with a DNA extraction kit (MinkaGene Bacterial DNA Kit) in accordance with the manufacturer's instructions. PCR amplification of the 16S rRNA gene V3–V4 variable regions was performed using the bacterial universal primers 338 F (5′-ACTCCTACGGGAGGCAGCA-3′) and 806 R (5′-GGACTACHVGGGTWTCTAAT-3′) under the following conditions: 95°C for 3 min, followed by 28 cycles of 95°C for 30 s, 50°C for 30 s, and 72°C for 45 s; with a final extension step at 72°C for 10 min. PCR analysis was performed in triplicate for each sample. The PCR amplicons were purified and quantified with a PCR fragment purification kit (Takara, Japan) and a Quant-iT Pico Green dsDNA Quantification Kit (Invitrogen, Carlsbad, CA, USA), respectively. The equimolar PCR products from each sample were combined into equimolar ratios for paired-end library preparation and sequenced on an Illumina MiSeq Platform (Illumina, San Diego, CA, USA).
Processing of Original Sequencing Data
Paired-end sequence reads were merged using USEARCH (version 11). The paired reads were merged into the Fastq mergepairs function and filtered with a “maxee” value of 1.0. Unique sequence reads were obtained using the fastx_uniques function, and then noise reduction was performed using the unoise3 (unoise_alpha = 4, minsize = 6) algorithm to correct errors. The remaining sequences were then screened to remove chimeras and clustered into zero-radius operational taxonomic units (ZOTUs) with 97% similarity or more, after which all quality-filtered reads were mapped to these ZOTUs (Edgar, 2016). The representative sequences for each ZOTU were assigned to taxonomic groups by using the RDP classifier within the SILVA database (16s_v138) clustered at 99% similarity.
Data Analysis
ZOTUs with a relative abundance higher than 0.001% of all ZOTUs were included for further analysis. The gut microbes data were Hellinger transformed, and the environmental factors were normalized before analysis.
The Bray-Curtis dissimilarity of gut microbial community composition was calculated to build the matrix to identify the relationships among samples by using the function vegdist in the “vegan” package. Non-metric multidimensional scaling (NMDS) was implemented to evaluate the overall differences in gut microbial community among different months by using the function metaMDS in the “vegan” package. An analysis of similarity (ANOSIM) was performed to determine the significance of differences in gut microbial community composition between periods of non-cyanobacterial and cyanobacterial blooms by using the function anosim in the “vegan” package. Alpha diversity indices, including Shannon-Wiener index, Simpson index, Pilou's evenness, and richness, were calculated using the R package “vegan.” Student's t-test was used to identify significant differences in the alpha diversity indices between the two periods. The top 10 taxa in terms of abundance at the phylum and class levels were selected for creating stack maps in the “ggplot2” package. Furthermore, bacterial taxa differentially represented between the two periods at the species or higher taxonomic levels (depending on taxa annotation) were identified by performing linear discriminant analysis (LDA) coupled with effect size (LEfSe) (http://huttenhower.sph.harvard.edu/galaxy) (Segata et al., 2011). The environmental factors that have a significant effect on the gut microbial community were assessed by performing a forward selection model with Monte Carlo permutation tests by using the “packfor” package. Multicollinearity among variables was assessed by calculating variance inflation factors (VIF) by using the vif.cca function in the “vegan” package. Then, redundancy analysis (RDA) was conducted to analyze the relationship between the selected factors and the gut microbial community composition. Variance partitioning analysis (VPA) was performed to quantify the fractions of variation explained by the different forward-selected environmental variables by using the varpart function in the “vegan” package with 999 permutation test. PICRUSt2 (https://github.com/picrust/picrust2) was used to obtain information at different pathway levels (levels 1–3) compared with Kyoto Encyclopedia of Genes and Genomes (KEGG) database. The level 2 KEGG pathways were compared between the two periods based on t-test (P < 0.05) and visualized in heatmap via the “pheatmap” package. Pearson correlation and linear regression between the differential KEGG pathways and main influencing factors were analyzed via the “corrplot” package.
All statistical analyses were performed in R environment (https://www.r-project.org/) unless otherwise mentioned.
Results
Phytoplankton Community and Environmental Parameters
A total of 52 species of phytoplankton belonging to seven phyla were identified across all samples during the study period. Among these species, there were 25 species of Chlorophyta, 9 species of Cyanophyta, 8 species of Bacillariophyta, 5 species of Euglenophyta, 2 species of Pyrrophyta, 2 species of Cryptophyta, and 1 species of Chrysophyta. Although surface cyanobacteria biomass decreased in July because of the heavy rainfall brought by a typhoon, severe cyanobacterial blooms were observed that begun in May and continued into November (Supplementary Figure 1). Colonial Microcystis spp. (mainly Microcystis aeruginosa) with an abundance over 107 cells/L were the predominant species in the cyanobacterial bloom. Accordingly, March-April was determined as the non-cyanobacterial bloom (NCB) period and May-November as the cyanobacterial bloom (CB) period.
The environmental parameters showed clear temporal variations (Supplementary Table 1). WT gradually increased from March, peaked in August (29.3°C), and then decreased. The pH ranged from 6.23 to 8.51 throughout the sampling period, with the maximal value in August and the lowest value in October. DO and SD varied from 1.49 to 9.14 mg/L and from 0.17 to 0.71 m, respectively. In general, the variations of NH4-N, NO2-N, NO3-N, and TN all initially decreased and then increased, and their minimal value of 0.04, 0.02, 0.28, and 0.52 mg/L, respectively, was observed in June and July. The trends of phosphate (PO4-P) and total phosphorus (TP) were similar, with variations ranging from 0.05 to 0.15 mg/L and 0.06 to 0.83 mg/L, respectively.
Composition of the Gut Microbial Community of B. aeruginosa in the Two Periods
A total of 2,489 ZOTUs belonging to 25 phyla and 311 genera were detected across the samples. Among these ZOTUs, 893 (35.9%) were shared by two distinct periods, 875 (35.2%) and 719 (28.9%) were unique in samples from the NCB and CB periods, respectively (Supplementary Figure 2). At the phylum level, Proteobacteria and Firmicutes were the two most dominant phyla in the gut of B. aeruginosa (Figure 1A), with an overall relative abundance of 58.8 and 26.3%, respectively. The relative abundance of Proteobacteria was lower during the NCB period but higher during the CB period. The opposite trend was observed in Firmicutes. Moreover, the relative abundance of Bacteroidota was higher during the NCB period than that during the CB period. At the class level, Gammaproteobacteria (49.8%) and Bacilli (19.9%) were the dominant classes, followed by Alphaproteobacteria (8.9%), Clostridia (6.3%), and Bacteroidia (5.4%). As shown in Figure 1B, the relative abundance of Gammaproteobacteria was lower but that of Clostridia was higher during the NCB period than those during the CB period.
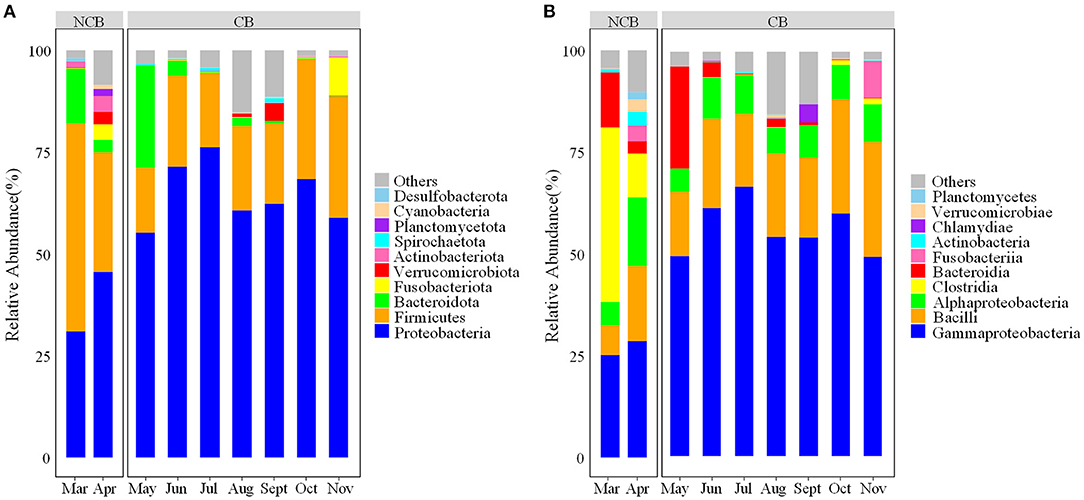
Figure 1. Composition of the bacterial community in the gut of B. aeruginosa snails (A) at the phylum level; (B) at the class level. NCB, non-cyanobacterial bloom; CB, cyanobacterial bloom.
NMDS was used to determine the similarity of the gut microbial community of B. aeruginosa in different months (Figure 2). The stress value was 0.09, indicating that the ordination pattern was acceptable and reliable. NMDS indicated that the snail samples clustered into two groups that corresponded to the NCB and CB periods. ANOSIM revealed significant differences between the two periods (P < 0.01, r = 0.884). The alpha diversity of the gut microbial community of B. aeruginosa was assessed. The four alpha diversity indices (Shannon-Wiener index, Simpson index, Pilou's evenness, and richness) were significantly higher during the NCB period than those during the CB period (P < 0.05), indicating that the bacterial richness and microbial diversity in the gut of B. aeruginosa decreased with the occurrence of cyanobacterial bloom (Figure 3). Microorganisms that were differentially represented in the two periods were identified by performing LEfSe on taxa with an LDA score > 2. Firmicutes (including the family [Eubacterium]_coprostanoligenes_group and the genus Faecalibacterium, Subdoligranulum), Fusobacteria (including the genus Cetobacterium), and Proteobacteria (including the family Rhodobacteraceae and the genus Rhizobiales_Incertae_Sedis_uncultured, Escherichia-Shigella) were more abundant during the NCB period than during the CB period (P < 0.05). Proteobacteria (including the genus Ralstonia, Pelomonas, Acinetobacter, and Lysobacter) were more abundant during the CB period than during the NCB period (Figure 4).
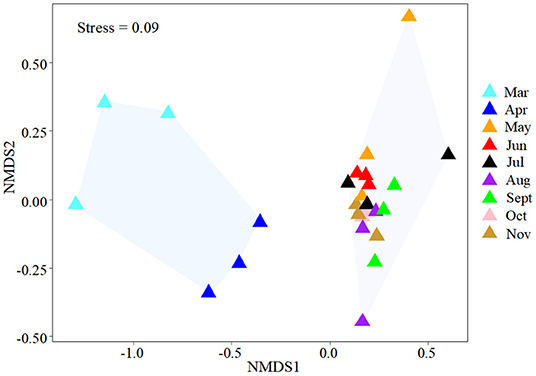
Figure 2. NMDS plot used to visualize the dissimilarities of the gut microbial communities of snails in different months.
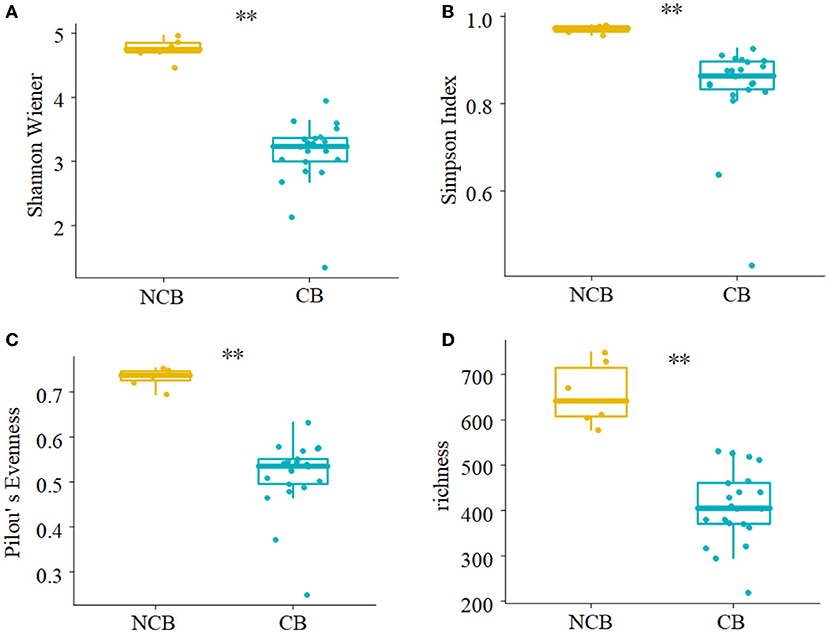
Figure 3. Comparison of alpha diversity indices of gut microbiota between non-cyanobacterial bloom (NCB) and cyanobacterial bloom (CB) periods. (A) Shannon-Wiener index, (B) Simpson index, (C) Pilou's evenness, (D) richness. **P < 0.01.
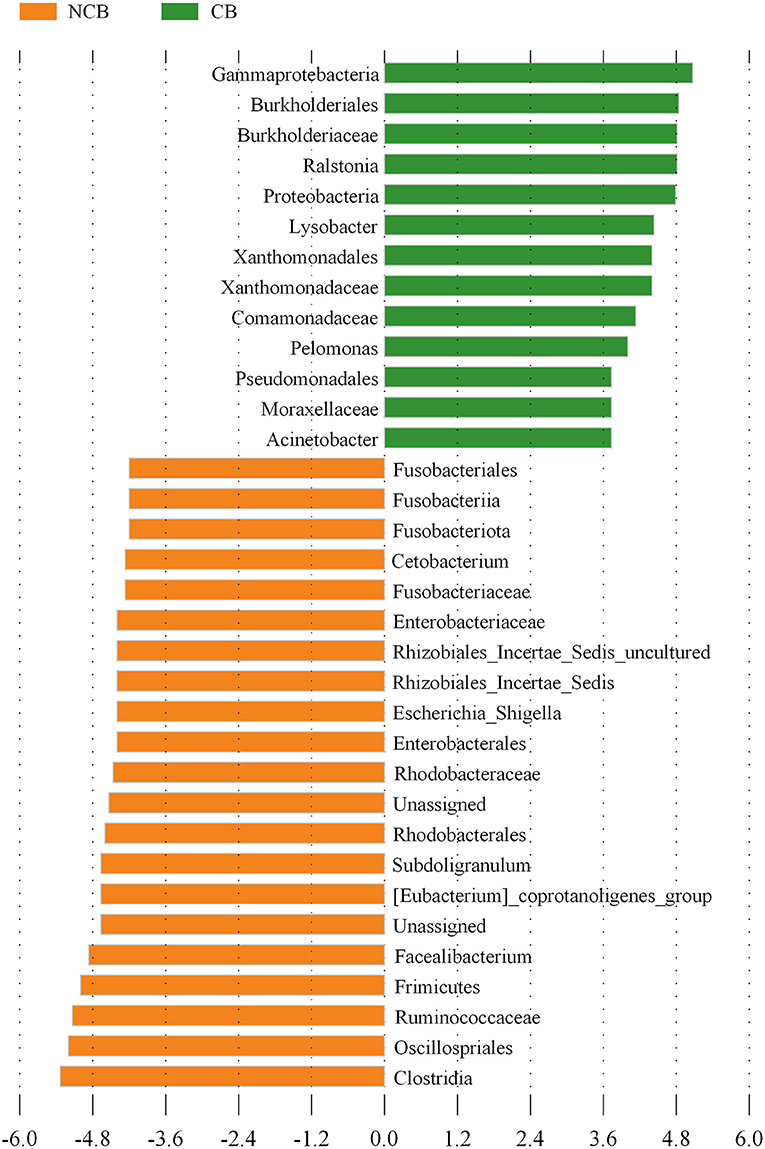
Figure 4. Bacterial taxa differentially represented between the non-cyanobacterial bloom (NCB) period and the cyanobacterial bloom (CB) period, as determined by LEfSe by using the default parameters.
Potential Relationships Between the Gut Microbial Community and Environmental Factors
Using the forward selection procedure, MCs (F = 4.508, P < 0.001) and WT (F = 1.989, P = 0.023) were selected as explanatory factors for gut microbial community variations. The relationship between gut microbial community and significant environmental factors was revealed by the RDA model. The first and second axes of the RDA model explained 70.3% and 29.7% of the variations, respectively (Figure 5). VPA revealed that the MCs alone effects explained 11.5% of the variations (P < 0.01), which was higher than that of the WT alone effects (6.3%, P < 0.05). The shared effects of MCs and WT factors explained 7.3% of the variations. The unexplained proportion was 74.9% (Figure 6). In addition, the abundant discriminant taxa of the two periods exhibited a certain correlation with the environmental variables. The abundant discriminant taxa during the NCB period exhibited negative correlations with MCs in tissues, WT, and Chl-a. The abundant discriminant taxa during the CB period were strongly correlated with WT, MCs, DO, and TP. For example, Ralstonia showed a positive correlation with WT, and Lysobacter displayed a positive correlation with TP (Figure 7).
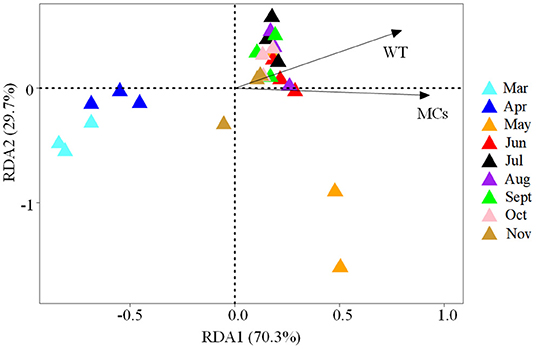
Figure 5. The biplot of the first two axes of the RDA analysis for the significant environmental factors associated with gut microbial community variation.
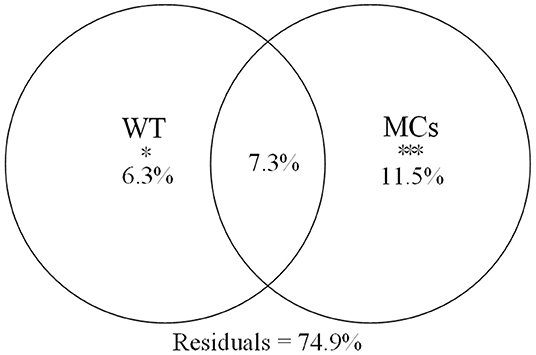
Figure 6. Variance partitioning plot of gut microbial community of B. aeruginosa. *P < 0.05, ***P < 0.001.
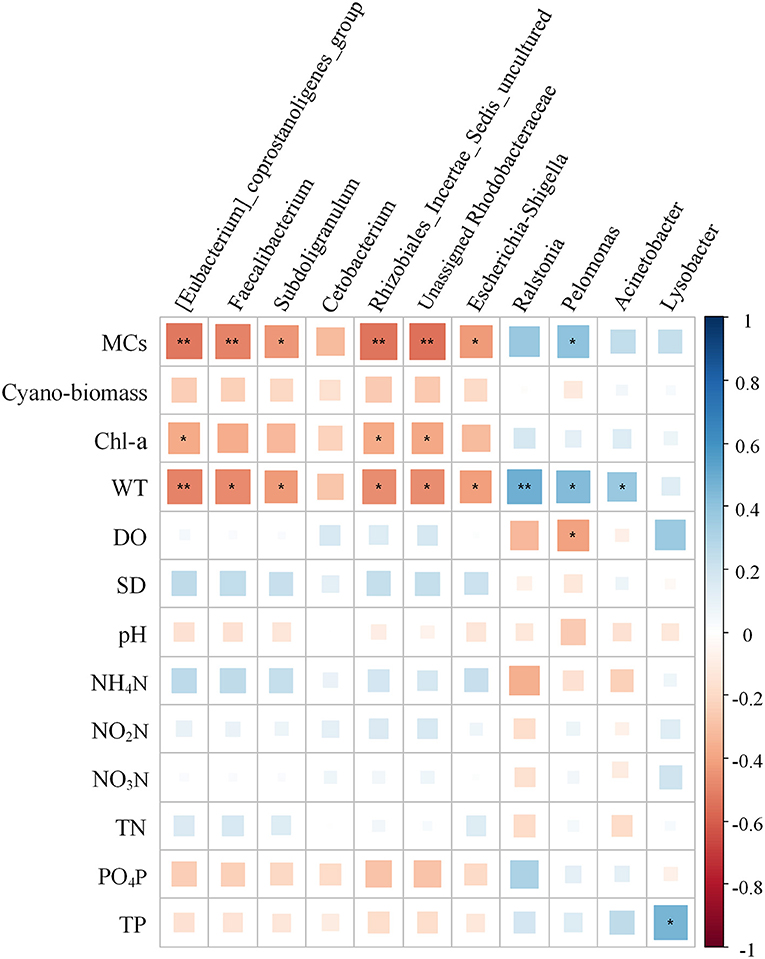
Figure 7. Relationship between the abundant discriminant taxa and environmental factors. The significance is noted as follows: **P < 0.01; *P < 0.05; and “blank,” not significant. Cyano-biomass: Cyanobacteria biomass.
Prediction and Comparison of Gut Microbial Community Functions in the Two Periods
Functional metabolic pathways of the gut microbial community 16S rRNA gene sequences were predicted by PICRUSt2. The mean Nearest Sequenced Taxon Index value was 0.10 ± 0.06. The level 1 KEGG pathway indicated a high abundance of genes related to metabolic pathways, environmental information processing, and genetic information processing (Supplementary Table 2). Nine pathways (e.g., carbohydrate metabolism and immune system) were more abundant during the NCB period, whereas five pathways (e.g., environmental adaptation and xenobiotics biodegradation and metabolism) were more abundant during the CB period (P < 0.05) (Figure 8). The results of significant regression analysis of the differential pathways and the two main influencing factors (MCs and WT) are shown in Figure 9. The pathways of carbohydrate metabolism, immune system, environmental adaptation, and xenobiotics biodegradation and metabolism showed a significant linear regression relationship with MCs and WT.
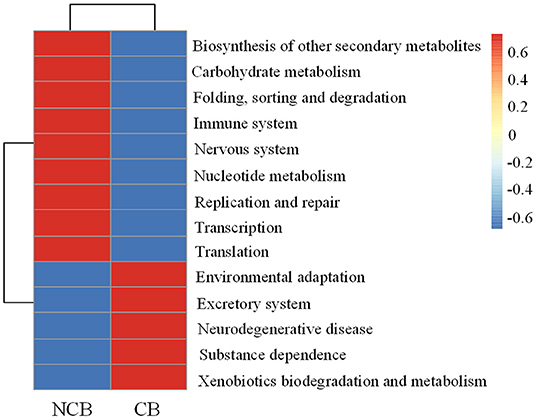
Figure 8. Differences in KEGG functions in the B. aeruginosa gut microbial community during non-cyanobacterial bloom (NCB) and cyanobacterial bloom (CB) periods (level 2). (P < 0.05).
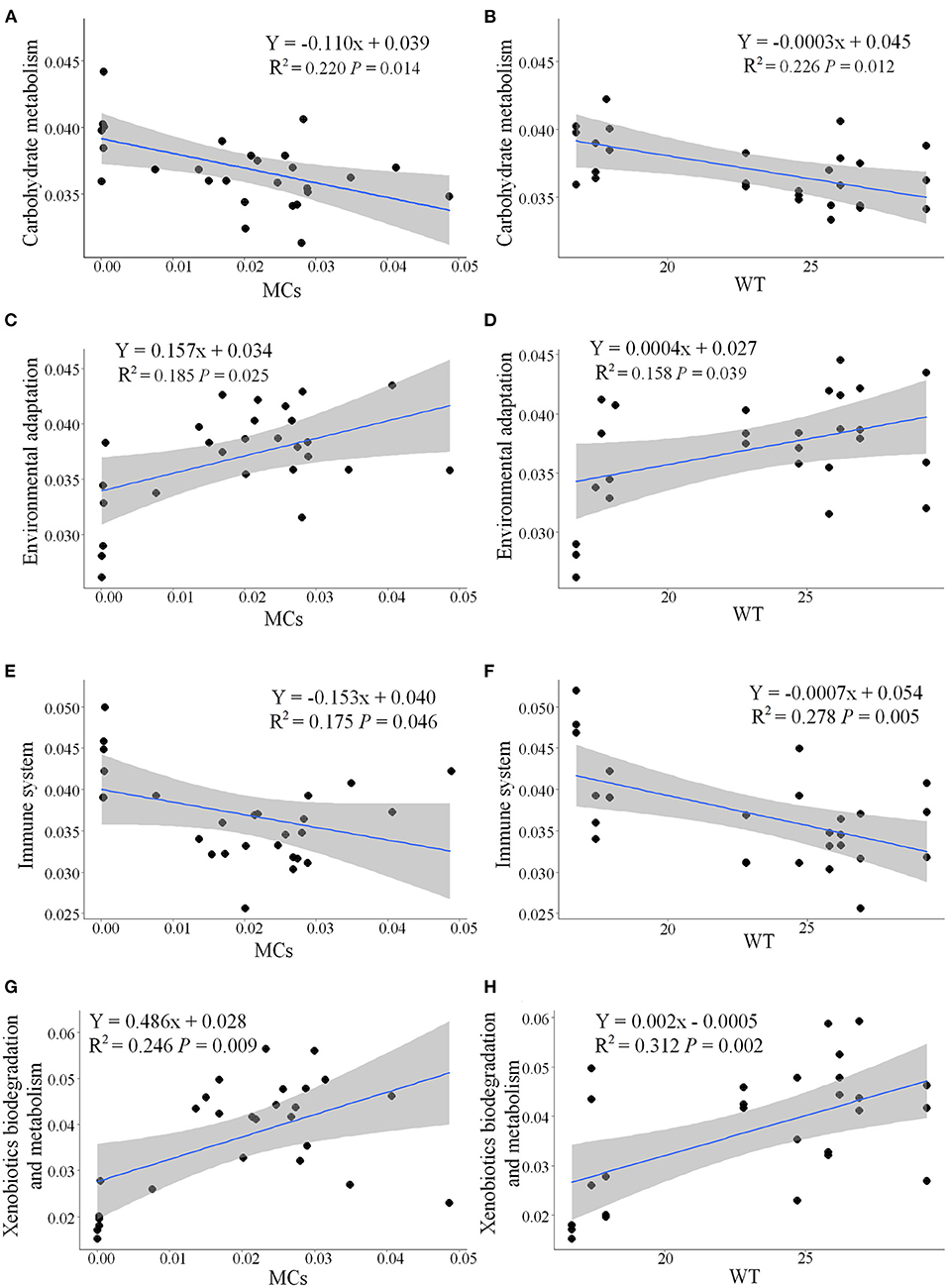
Figure 9. Linear relationships among the pathways carbohydrate metabolism (A,B), environmental adaptation (C,D), immune system (E,F), and xenobiotics biodegradation and metabolism (G,H) and changes in MCs and WT. The R2 values represent correlation coefficient, P-values represent significant differences (P < 0.05). The gray-shaded area represents a 95% confidence interval.
Discussion
Changes in and Influencing Factors of the Gut Microbiota Composition of B. aeruginosa Exposed to Cyanobacterial Bloom
The main gut microbial community composition of B. aeruginosa was similar to that of other aquatic snails, such as Oncomelania hupensis (Hao et al., 2020) and Pomacea canaliculata (Li et al., 2019). Proteobacteria, Firmicutes, and Bacteroidota constitute the prominent phyla in the intestines of freshwater gastropods. Although the gut microbiota compositions of different species of aquatic snails are relatively similar at the phylum level, notable differences may still be observed at other taxonomic levels. These differences are also common in the gut microbiota from different temporal (Hu et al., 2018) or spatial (Li et al., 2019) samples. In our case, the taxonomic composition and the alpha diversity of the gut microbiota of B. aeruginosa were variable between the NCB and CB periods (Figure 2). These results were consistent with previous field observations that the gut microbiota of aquatic snails could respond to different environmental conditions (Yang et al., 2019; Hao et al., 2020). Although no study has reported on the response of gut microbiota of gastropods to cyanobacterial blooms, the impacts of bloom-forming cyanobacteria and their toxins on intestinal flora have been confirmed in fish (Duperron et al., 2019; Qian et al., 2019) and cladocerans (Macke et al., 2017). The gut bacterial composition and diversity of aquatic gastropods can be determined by multiple factors, including environmental variation (Yang et al., 2019) and diet (Ito et al., 2019). Considering that these snails were all collected from the same habitat in the same location in this study, environmental fluctuations associated with blooms thus may have been the major driver that shaped the gut microbiota of Bellamya. Compared with other physicochemical factors of the habitat, MCs and WT were the main influencing factors on the gut microbial community of B. aeruginosa, and the contribution of MCs was greater than that of WT. This result suggested that the gut microbiome of B. aeruginosa can rapidly respond to changes in host environmental conditions, especially toxin accumulation in tissues, through changes in community composition.
Some indoor experiments have shown that temperature change determines the gut microbiota composition and function of aquatic animals (e.g., Li et al., 2018). However, unlike direct testing of the impacts of temperature under controlled experimental conditions, field studies cannot readily identify the associations between temperature and gut microbiota (Sepulveda and Moeller, 2020). Although multicollinearity among variables was eliminated through statistical approach in this study, and due to the fact that WT often covaries with other environmental variables (Sepulveda and Moeller, 2020), WT likely reflects the dynamics of unobserved or difficult-to-quantify variables, such as changes in food availability or sources. As a facultative suspension feeder, B. aeruginosa tend to obtain food by filter-feeding when sufficient food materials (such as edible algae) are present in the water column, and the presence of large concentrations of toxic cyanobacteria may result in a high percentage of food materials (such as detritus) being collected by scraping (Qiu et al., 2017). Since blooms usually occur during hot seasons, changes in food sources caused by the blooms frequently co-occurred with changes in water temperature. This may explain why the contribution of interaction between WT and MCs was also high, even higher than that of WT alone and the presence of such a high proportion of unexplained residuals.
Responses of Potential Functions of Gut Microbiota of B. aeruginosa to Cyanobacterial Bloom
Faecalibacterium and Subdoligranulum (both belonging to the family Ruminococcaceae) are considered to be able to produce diverse organic acids and short chain fatty acids (Flint et al., 2012; Miquel et al., 2013), which help to enhance anti-inflammatory effects and improve the immunity of the hosts (Lopez-Siles et al., 2017). However, the relative abundance of these bacteria was higher during the NCB period than that during the CB period. Regression analysis revealed that the underlying metabolic functions related to immune system were negatively correlated with the increase of MCs content (Figure 9E), suggesting that the occurrence of cyanobacterial blooms weakened the host immunity mediated by the gut bacteria. Meanwhile, the decrease in carbohydrate metabolism related to MCs also reflected the inhibition of energy metabolism. However, the pathways related to environmental adaptation and xenobiotics biodegradation and metabolism were upregulated during the CB period, suggesting that the potential for host-microbial synergistic detoxification was increasing. Aquatic animals display similar responses to environmental stress, such as heavy metals (Yan et al., 2020) and chemical pollutants (Milan et al., 2019).
During cyanobacterial blooms, the major food source of B. aeruginosa changes to detritus (Qiu et al., 2017), which is believed to be mainly composed of allochthonous resources from terrestrial plants in small and shallow water bodies (Holgerson et al., 2016). Although terrigenous detritus usually consists of cellulose and lignin that most macroinvertebrates are unable to digest and assimilate, studies have found that the gut bacteria of freshwater snails can help their host degrade these indigestible substances (e.g., Brendelberger, 1997). We also found that the abundance of bacteria with similar functions substantially increased after the occurrence of cyanobacterial bloom. As plant pathogens, Ralstonia can secrete various plant cell wall-degrading enzymes (Poueymiro and Genin, 2009), and their increased abundance may help the snail host to adapt to changes in food source. Furthermore, some previous studies indicated that remarkable changes in the abundance of Rhodobacteraceae are associated with the switching of food or energy sources during metamorphosis in aquatic invertebrates (Zhang et al., 2020; Yang M. J. et al., 2022). In our results, the changes in these bacteria at different periods further reflected the response characteristics of the gut microbiota assembly of B. aeruginosa to food changes.
Conclusion
Proteobacteria and Firmicutes were the prominent phyla of gut microbes of B. aeruginosa. The composition and relative abundance of the gut microbes were affected by environmental conditions, especially those related to toxic cyanobacteria. The alpha diversity of the gut microbiota of B. aeruginosa exposed to cyanobacterial bloom was lower than that in non-cyanobacterial bloom. The potential functions of the gut microbiome could help the snail meet its immunity and energy needs during cyanobacterial bloom stress. These results not only provide key information for revealing the response mechanism of freshwater snails to algal blooms but also helps clarify the biological adaptation mechanism of gastropod host from the perspective of intestinal flora.
Data Availability Statement
The data presented in the study are deposited in the NCBI repository, accession number PRJNA822529.
Author Contributions
TL: methodology, formal analysis, and writing – original draft. JZ: conceptualization, supervision, writing – review, editing, and funding acquisition. XY: investigation. WY: validation, writing – review, and editing. ZZ: writing – review and editing. All authors contributed to the article and approved the submitted version.
Funding
This work was supported by National Natural Science Foundation of China (42077219), Hangzhou Municipal Agriculture and Social Development Project (2020ZDSJ0697), and Fundamental Research Funds for the Provincial Universities of Zhejiang (SJLY2020011).
Conflict of Interest
The authors declare that the research was conducted in the absence of any commercial or financial relationships that could be construed as a potential conflict of interest.
Publisher's Note
All claims expressed in this article are solely those of the authors and do not necessarily represent those of their affiliated organizations, or those of the publisher, the editors and the reviewers. Any product that may be evaluated in this article, or claim that may be made by its manufacturer, is not guaranteed or endorsed by the publisher.
Supplementary Material
The Supplementary Material for this article can be found online at: https://www.frontiersin.org/articles/10.3389/fmicb.2022.906278/full#supplementary-material
References
Bownik, A. (2016). Harmful algae: effects of cyanobacterial cyclic peptides on aquatic invertebrates-a short review. Toxicon. 124, 26–35. doi: 10.1016/j.toxicon.2016.10.017
Brendelberger, H. (1997). Bacteria and digestive enzymes in the alimentary tract of Radix peregra (Gastropoda, Lymnaeidae). Limnol. Oceanogr. 42, 1635–1638. doi: 10.4319/lo.1997.42.7.1635
Chen, W. M. (2006). Discussion on possible error for phytoplankton chlorophyll-α concentration analysis using hot-ethanol extraction method. J. Lake Sci. 18, 550–552. doi: 10.18307/2006.0519
Duperron, S., Halary, S., Habiballah, M., Gallet, A., Huet, H., Duval, C., et al. (2019). Response of fish gut microbiota to toxin-containing cyanobacterial extracts: a microcosm study on the medaka (Oryzias latipes). Environ. Sci. Technol. Lett. 6, 341–347. doi: 10.1021/acs.estlett.9b00297
Dushku, E., Ioannou, A., Staikou, A., and Yiangou, M. (2019). Probiotic properties and immunomodulatory activity of gastrointestinal tract commensal bacterial strains isolated from the edible farmed snail Cornu aspersum maxima. Fish Shellfish Immunol. 92, 792–801. doi: 10.1016/j.fsi.2019.06.061
Edgar, R. C. (2016). UNOISE2: improved error-correction for Illumina 16S and ITS amplicon sequencing. BioRxiv. 081257. doi: 10.1101/081257
Flint, H. J., Scott, K. P., Louis, P., and Duncan, S. H. (2012). The role of the gut microbiota in nutrition and health. Nat. Rev. Gastro. Hepat. 9, 577–589. doi: 10.1038/nrgastro.2012.156
Gérard, C., and Lance, E. (2019). Decline of freshwater gastropods exposed to recurrent interacting stressors implying cyanobacterial proliferations and droughts. Aquat. Ecol. 53, 79–96. doi: 10.1007/s10452-019-09674-8
Gérard, C., Poullain, V., Lance, E., Acou, A., Brient, L., and Carpentier, A. (2009). Influence of toxic cyanobacteria on community structure and microcystin accumulation of freshwater molluscs. Environ. Pollut. 157, 609–617. doi: 10.1016/j.envpol.2008.08.017
Gilbert, S. F., Bosch, T. C., and Ledón-Rettig, C. (2015). Eco-Evo-Devo: developmental symbiosis and developmental plasticity as evolutionary agents. Nat. Rev. Genet.16, 611–622. doi: 10.1038/nrg3982
Gong, Z. J., Li, Y. L., and Xie, P. (2009). Population dynamics and production of bellamya aeruginosa (reeve) (mollusca: viviparidae) in lake donghu, wuhan. J. Lake Sci. 21, 401–407. doi: 10.18307/2009.0314
Grandiosa, R., Mérien, F., Young, T., Van Nguyen, T., Gutierrez, N., Kitundu, E., et al. (2018). Multi-strain probiotics enhance immune responsiveness and alters metabolic profiles in the New Zealand black-footed abalone (Haliotis iris). Fish Shellfish Immunol. 82, 330–338. doi: 10.1016/j.fsi.2018.08.034
Hao, Y., Guan, W., Wu, H., Li, L., Abe, E. M., Xue, J., et al. (2020). Intestinal microbiome profiles in Oncomelania hupensis in mainland China. Acta Trop. 201, 105202. doi: 10.1016/j.actatropica.2019.105202
Holgerson, M. A., Post, D. M., and Skelly, D. K. (2016). Reconciling the role of terrestrial leaves in pond food webs: a whole-ecosystem experiment. Ecology 97, 1771–1782. doi: 10.1890/15-1848.1
Hu, Z., Chen, X., Chang, J., Yu, J., Tong, Q., Li, S., et al. (2018). Compositional and predicted functional analysis of the gut microbiota of Radix auricularia (Linnaeus) via high-throughput Illumina sequencing. PeerJ. 6, e5537. doi: 10.7717/peerj.5537
Huisman, J., Codd, G. A., Paerl, H. W., Ibelings, B. W., Verspagen, J. M., and Visser, P. M. (2018). Cyanobacterial blooms. Nat. Rev. Microbiol. 16, 471–483. doi: 10.1038/s41579-018-0040-1
Ito, M., Watanabe, K., Maruyama, T., Mori, T., Niwa, K., Chow, S., et al. (2019). Enrichment of bacteria and alginate lyase genes potentially involved in brown alga degradation in the gut of marine gastropods. Sci. Rep. 9, 1–13. doi: 10.1038/s41598-018-38356-y
Lance, E., Brient, L., Carpentier, A., Acou, A., Marion, L., Gérard, C., et al. (2010). Impact of toxic cyanobacteria on gastropods and microcystin accumulation in a eutrophic lake (Grand-Lieu, France) with special reference to Physa (= Physella) acuta. Sci. Total Environ. 408, 3560–3568. doi: 10.1016/j.scitotenv.2010.04.050
Lei, K., Qiao, F., Liu, Q., Wei, Z., An, L., Qi, H., et al. (2017). Preliminary evidence for snail deformation from a Eutrophic lake. Environ. Toxicol. Pharmacol. 53, 219–226. doi: 10.1016/j.etap.2017.06.019
Li, L. H., Lv, S., Lu, Y., Bi, D. Q., Guo, Y. H., Wu, J. T., et al. (2019). Spatial structure of the microbiome in the gut of Pomacea canaliculata. BMC Microbiol. 19, 1–9. doi: 10.1186/s12866-019-1661-x
Li, Y. F., Yang, N., Liang, X., Yoshida, A., Osatomi, K., Power, D., et al. (2018). Elevated seawater temperatures decrease microbial diversity in the gut of Mytilus coruscus. Front. Physiol. 9, 839. doi: 10.3389/fphys.2018.00839
Lopez-Siles, M., Duncan, S. H., Garcia-Gil, L. J., and Martinez-Medina, M. (2017). Faecalibacterium prausnitzii: from microbiology to diagnostics and prognostics. ISME J. 11, 841–852. doi: 10.1038/ismej.2016.176
Ma, T., Gong, S., Zhou, K., Zhu, C., Deng, K., Luo, Q., et al. (2010). Laboratory culture of the freshwater benthic gastropod Bellamya aeruginosa (Reeve) and its utility as a test species for sediment toxicity. J. Environ. Sci. 22, 304–313. doi: 10.1016/S1001-0742(09)60109-1
Macke, E., Callens, M., De Meester, L., and Decaestecker, E. (2017). Host-genotype dependent gut microbiota drives zooplankton tolerance to toxic cyanobacteria. Nat. Commun. 8, 1–13. doi: 10.1038/s41467-017-01714-x
Massey, I. Y., Yang, F., Ding, Z., Yang, S., Guo, J., Al-Osman, M., et al. (2018). Exposure routes and health effects of microcystins on animals and humans: a mini-review. Toxicon. 151, 156–162. doi: 10.1016/j.toxicon.2018.07.010
Milan, M., Smits, M., Dalla Rovere, G., Iori, S., Zampieri, A., Carraro, L., et al. (2019). Host-microbiota interactions shed light on mortality events in the striped venus clam Chamelea gallina. Mol. Ecol. 28, 4486–4499. doi: 10.1111/mec.15227
Miquel, S., Martin, R., Rossi, O., Bermúdez-Humarán, L. G., Chatel, J. M., Sokol, H., et al. (2013). Faecalibacterium prausnitzii and human intestinal health. Curr. Opin. Microbiol. 16, 255–261. doi: 10.1016/j.mib.2013.06.003
Nicolai, A., Rouland-Lefèvre, C., Ansart, A., Filser, J., Lenz, R., Pando, A., et al. (2015). Inter-population differences and seasonal dynamic of the bacterial gut community in the endangered land snail Helix pomatia (Gastropoda: Helicidae). Malacologia 59, 177–190. doi: 10.4002/040.059.0101
Paerl, H. W. (2018). Mitigating toxic planktonic cyanobacterial blooms in aquatic ecosystems facing increasing anthropogenic and climatic pressures. Toxins 10, 76. doi: 10.3390/toxins10020076
Poueymiro, M., and Genin, S. (2009). Secreted proteins from Ralstonia solanacearum: a hundred tricks to kill a plant. Curr. Opin. Microbiol. 12, 44–52 doi: 10.1016/j.mib.2008.11.008
Qian, H., Zhang, M., Liu, G., Lu, T., Sun, L., and Pan, X. (2019). Effects of different concentrations of Microcystis aeruginosa on the intestinal microbiota and immunity of zebrafish (Danio rerio). Chemosphere 214, 579–586. doi: 10.1016/j.chemosphere.2018.09.156
Qiao, F., Lei, K., Han, X., Wei, Z., Zhao, X., An, L., et al. (2018b). No impacts of microcystins on wild freshwater snail Bellamya Aeruginosa fecundity from a eutrophic lake. Environ. Toxicol. Pharmacol. 60, 165–168. doi: 10.1016/j.etap.2018.04.024
Qiao, F., Lei, K., Li, Z., Wei, Z., Liu, Q., Yang, L., et al. (2018a). Transcriptomic responses of the freshwater snail (Parafossarulus striatulus) following dietary exposure to cyanobacteria. Sci Total Environ. 624, 153–161. doi: 10.1016/j.scitotenv.2017.12.112
Qiu, H., Lu, K., Zheng, Z., Wang, J., and Zhu, J. (2017). Blooms of toxic cyanobacteria cause the gastropod Bellamya aeruginosa to shift its diet from planktic to benthic material. Int. Rev. Hydrobiol. 102, 90–99. doi: 10.1002/iroh.201601859
Segata, N., Izard, J., Waldron, L., Gevers, D., Miropolsky, L., Garrett, W. S., et al. (2011). Metagenomic biomarker discovery and explanation. Genome Biol. 12, 1–18. doi: 10.1186/gb-2011-12-6-r60
Sepulveda, J., and Moeller, A. H. (2020). The effects of temperature on animal gut microbiomes. Front. Microbiol. 11, 384. doi: 10.3389/fmicb.2020.00384
Shapira, M. (2016). Gut microbiotas and host evolution: scaling up symbiosis. Trends Ecol. Evol. 31, 539–549. doi: 10.1016/j.tree.2016.03.006
Utermöhl, H. (1958). Zur Vervollkommnung der quantitativen Phytoplankton-methodik. Mitt. Int. Verein. Limnol. 9, 1–38. doi: 10.1080/05384680.1958.11904091
Van Horn, D. J., Garcia, J. R., Loker, E. S., Mitchell, K. R., Mkoji, G. M., Adema, C. M., et al. (2012). Complex intestinal bacterial communities in three species of planorbid snails. J. Molluscan Stud. 78, 74–80. doi: 10.1093/mollus/eyr038
Yan, W., Hamid, N., Deng, S., Jia, P. P., and Pei, D. S. (2020). Individual and combined toxicogenetic effects of microplastics and heavy metals (Cd, Pb, and Zn) perturb gut microbiota homeostasis and gonadal development in marine medaka (Oryzias melastigma). J. Hazard. Mater. 397, 122795. doi: 10.1016/j.jhazmat.2020.122795
Yang, M. J., Song, H., Feng, J., Yu, Z. L., Shi, P., Liang, J., et al. (2022). Symbiotic microbiome and metabolism profiles reveal the effects of induction by oysters on the metamorphosis of the carnivorous gastropod Rapana venosa. Comput. Struct. Biotechnol. J. 20, 1–14. doi: 10.1016/j.csbj.2021.11.041
Yang, M. J., Song, H., Sun, L. N., Yu, Z. L., Hu, Z., Wang, X. L., et al. (2019). Effect of temperature on the microflora community composition in the digestive tract of the veined rapa whelk (Rapana venosa) revealed by 16S rRNA gene sequencing. Genom. Proteom. 29, 145–153. doi: 10.1016/j.cbd.2018.10.006
Yang, W., Ye, Y., Lu, K., Zheng, Z., and Zhu, J. (2022). NMR-based metabolomic responses of freshwater gastropod Bellamya aeruginosa to MC-producing and non MC-producing Microcystis aeruginosa. J. Oceanol. Limnol. 40, 260–272. doi: 10.1007/s00343-021-0336-2
Zhang, J., Xie, Z., and Wang, Z. (2016). Oxidative stress responses and toxin accumulation in the freshwater snail Radix swinhoei (Gastropoda, Pulmonata) exposed to microcystin-LR. Environ. Sci. Pollut R. 23, 1353–1361. doi: 10.1007/s11356-015-5366-x
Zhang, M., Chen, H., Liu, L., Xu, L., Wang, X., Chang, L., et al. (2020). The changes in the frog gut microbiome and its putative oxygen-related phenotypes accompanying the development of gastrointestinal complexity and dietary shift. Front. Microbiol. 11, 162. doi: 10.3389/fmicb.2020.00162
Keywords: Bellamya aeruginosa, gut microbiota, cyanobacterial bloom, microcystins, PICRUSt2
Citation: Lyu T, Zhu J, Yang X, Yang W and Zheng Z (2022) Responses of Gut Microbial Community Composition and Function of the Freshwater Gastropod Bellamya aeruginosa to Cyanobacterial Bloom. Front. Microbiol. 13:906278. doi: 10.3389/fmicb.2022.906278
Received: 28 March 2022; Accepted: 20 April 2022;
Published: 13 May 2022.
Edited by:
Qingyun Yan, Sun Yat-sen University, ChinaReviewed by:
Qiuhua Li, Guizhou Normal University, ChinaQinfeng Gao, Ocean University of China, China
Copyright © 2022 Lyu, Zhu, Yang, Yang and Zheng. This is an open-access article distributed under the terms of the Creative Commons Attribution License (CC BY). The use, distribution or reproduction in other forums is permitted, provided the original author(s) and the copyright owner(s) are credited and that the original publication in this journal is cited, in accordance with accepted academic practice. No use, distribution or reproduction is permitted which does not comply with these terms.
*Correspondence: Jinyong Zhu, zhujinyong@nbu.edu.cn