- 1Department of Respiratory and Critical Care Medicine, Tianjin Medical University General Hospital, Tianjin, China
- 2Department of Respiratory and Critical Care Medicine, The Affiliated Hospital of Inner Mongolia Medical University, Hohhot, China
- 3Department of Respiratory and Critical Care Medicine, Shandong Second Provincial General Hospital, Jinan, China
- 4Institute of Infectious Diseases, The Second Hospital of Tianjin Medical University, Tianjin, China
In this study, we explored the clinical value of next-generation metagenome sequencing (mNGS) using bronchoalveolar lavage fluid (BALF) samples from patients with acid-fast staining (AFS) sputum smear-negative pulmonary tuberculosis (PTB) and non-tuberculous mycobacterial pulmonary disease (NTM-PD). Data corresponding to hospitalized patients with pulmonary infection admitted to the hospital between July 2018 and July 2021, who were finally diagnosed with AFS sputum smear-negative PTB and NTM-PD, were retrospectively analyzed. Bronchoscopy data as well as mNGS, Xpert, AFS (BALF analysis), and T-SPOT (blood) data, were extracted from medical records. Thereafter, the diagnostic performances of these methods with respect to PTB and NTM-PD were compared. Seventy-one patients with PTB and 23 with NTM-PD were included in the study. The sensitivities of mNGS, Xpert, T-SPOT, and AFS for the diagnosis of PTB were 94.4% (67/71), 85.9% (61/71), 64.8% (46/71), and 28.2% (20/71), respectively, and the diagnostic sensitivity of mNGS combined with Xpert was the highest (97.2%, 67/71). The specificity of Xpert was 100%, while those of AFS and T-SPOT were 73.9% (17/23) and 91.3% (21/23), respectively. Further, the 23 patients with NTM-PD could be identified using mNGS, and in the population with immunosuppression, the sensitivities of mNGS, Xpert, T-SPOT, and AFS were 93.5% (29/31), 80.6% (25/31), 48.4% (15/31), and 32.3% (10/31), respectively, and the diagnostic sensitivity of mNGS combined with Xpert was the highest (100%, 31/31). The specificities of Xpert and T-SPOT in this regard were both 100%, while that of AFS was 40% (2/5). Furthermore, using mNGS, all the NTM samples could be identified. Thus, the analysis of BALF samples using mNGS has a high accuracy in the differential diagnosis of MTB and NTM. Further, mNGS combined with Xpert can improve the detection of MTB, especially in AFS sputum smear-negative samples from patients with compromised immune states or poor responses to empirical antibiotics.
Introduction
Tuberculosis (TB), classified as pulmonary tuberculosis (PTB) or extrapulmonary tuberculosis, is an infectious disease that is caused by the Mycobacterium tuberculosis complex (MTBC). Specifically, PTB accounts for approximately 80% of all TB cases (WHO, 2021), and globally, the number of TB cases in 2020 was approximately 10 million, including 5.8 million new cases. Within this same year, TB also accounted for 1.5 million deaths. A greater proportion of patients with TB are adults (approximately 90%), with 8% of the total number of cases globally registered in China (WHO, 2021). Accurate and timely diagnosis of PTB is not only the basis for a successful treatment but also is key to improving the prognosis of patients and preventing the spread of the disease.
Presently, PTB diagnosis methods primarily include acid-fast staining (AFS), Mycobacterium tuberculosis culturing, and nucleic acid amplification (Xpert). The specificity of the culture method for the diagnosis of PTB is >99%. However, its sensitivity is approximately 80% (Lee et al., 2019). Additionally, it is time consuming (2–8 weeks), and this impedes early diagnosis (Pai et al., 2016a,b; Batyrshina and Schwartz, 2019). In contrast, AFS sputum smear microscopy is a fast and widely used TB screening method. However, its sensitivity is only approximately 50–60% (Young et al., 2008; Deshwal et al., 2019). In this regard, some authors have proposed that AFS using bronchoalveolar lavage fluid (BALF) samples can be performed in patients with negative sputum smears; however, the sensitivity of this test still needs to be improved (Shi et al., 2020; Liu et al., 2021). Further, whether using sputum or BALF smears, the major challenge associated with TB diagnosis using AFS is that a non-tuberculous mycobacteria (NTM) infection can be mistaken for an Mycobacterium tuberculosis (MTB) infection, resulting in patients receiving incorrect treatment. Xpert MTB/RIF assay (Cepheid, Sunnyvale, CA, United States) has been recognized by the World Health Organization (WHO) for preliminary diagnosis of suspected TB cases. However, even though its diagnostic specificity for PTB is >98%, its sensitivity for sputum smear-negative PTB is only 60%. Additionally, it does not offer the possibility of diagnosing NTM infections (Lee et al., 2019, 2020, CDC, 2019). Therefore, in clinical practice, it is challenging to distinguish MTB infections from NTM infections.
Metagenomic next-generation sequencing (mNGS) is a rapid diagnostic tool that differs from traditional microbial culture methods. mNGS identifies the possible pathogens through sequencing of nucleic acids in clinical samples, constructing biological reference databases, and rigorous sequence read mapping. This greatly improves the diagnostic ability (Doan et al., 2016; Chiu and Miller, 2019). Currently, mNGS is used for the diagnosis of various infection-related pathogens, including respiratory tract infections (Langelier et al., 2018; Xie et al., 2019), blood infections (Long et al., 2016), abscesses (Zhang et al., 2019), meningitis, and encephalitis (Guan et al., 2016; Zhao et al., 2020). mNGS can help detect intracellular pathogens and mycobacteria families (Zhang et al., 2019; Shi et al., 2020). However, only a few reports have described its use in the diagnosis of suspected PTB. The subjects included in the previous studies were AFS sputum smear-positive patients with normal immune function (Brown et al., 2015; Votintseva et al., 2017; Doyle et al., 2018; Zhou et al., 2019). To the best of our knowledge, there are only a few studies that evaluated the diagnostic value of mNGS in AFS sputum smear-negative PTB patients (Brown et al., 2015; Votintseva et al., 2017; Doyle et al., 2018; Zhou et al., 2019). We aimed to improve the diagnosis of PTB in immunocompromised patients using the mNGS approach. Conversely, whole-genome sequencing (WGS) has been used to identify Mycobacterium abscessus (Bryant et al., 2013; Sassi and Drancourt, 2014; Harris et al., 2015) and Mycobacterium avium (Operario et al., 2019), and few case reports (He et al., 2020; Taur et al., 2021) and cases (Huang et al., 2019) have described NTM determination using mNGS. More importantly, there is no previous study on the identification of MTB and NTM in patients with unexplained pulmonary infection with AFS negative sputum smear using mNGS. PTB and NTM-PD are similar in terms of clinical presentation; there have been cases of NTM-PD misdiagnosed as PTB (Maiga et al., 2012; Shahraki et al., 2015). However, there are significant differences between the two strains in treatment results and prognosis. Therefore, it is particularly important to identify NTM-PD in patients with suspected PTB during clinical diagnosis and treatment. However, in previous studies on the application value of mNGS in the diagnosis of suspected PTB, the control group was predominantly characterized by a common pathogen and was rarely identified with NTM infection. (Brown et al., 2015; Votintseva et al., 2017; Doyle et al., 2018; Zhou et al., 2019). In addition, there is no definitive conclusion on the ability of mNGS to distinguish NTM from MTB infection.
Thus, we conducted a single-center retrospective study involving hospitalized patients with suspected PTB and non-tuberculous mycobacterial pulmonary disease (NTM-PD) who were sputum smear-negative based on AFS, aiming to clarify the clinical value of mNGS in the diagnosis of AFS sputum smear-negative PTB and NTM-PD.
Materials and Methods
Research Design and Participants
This retrospective survey was approved by the Ethics Committee for trial registration (approval number ChiCTR1900023727) of the Tianjin Medical University General Hospital. In total, 120 patients was included in this retrospective study. The inclusion criteria were as follows: (1) patients hospitalized at the Tianjin Medical University General Hospital from July 2018 to July 2021; (2) patients with AFS-negative sputum smear and suspected PTB; (3) hospitalized patients who were eventually diagnosed with active PTB and NTM-PD; and (4) patients who underwent BALF acid-fast staining, mNGS, Xpert, and blood T-SPOT test during hospitalization.
The criteria for determining suspected PTB were as follows: (1) preliminary diagnosis based on pneumonia signs or symptoms (i.e., fever, new or increased cough, new or increased purulent sputum, chest pain, and new or increased dyspnea); (2) imaging analysis showing new infiltration and consolidation, or other lesions at the time of admission, with the new lesions not attributable to other causes (Qu and Cao, 2016); and (3) at least one antibiotic treatment administered before admission.
Diagnostic Criteria
Mycobacterium lung diseases include PTB and NTM-PD. The diagnosis of active PTB was based on the WHO TB treatment guidelines (fourth edition) and the Chinese Tuberculosis Clinical Treatment Guidelines (2017 edition) (Committee, 2010, Electron, 2018), while the diagnosis of NTM-PD was based on the consensus of experts on the diagnosis and treatment of NTM-PD in China and the 2020 guidelines for the diagnosis and treatment of ATS/ERS/ESCMID/IDSA (Haworth et al., 2017; Association, 2020; Daley et al., 2020).
Patients were classified as immunocompromised if they met any of the following criteria (Pan et al., 2019): (1) chemotherapy or neutropenia at <1,000 cells per μL within the past 28 days; (2) corticosteroid treatment at ≥20 mg per day for 14 days; (3) immunosuppressive therapy after organ or stem cell transplantation; and (4) human immunodeficiency virus (HIV) infection.
Specimen Collection and Processing
Experienced doctors collected BALF samples and lung biopsy specimens through bronchoscopy according to standard operating procedures (Meyer et al., 2012). During bronchoscopy, the surgeon recorded complications such as bleeding, fatal hemoptysis, arrhythmia, and death. Within 2 h after collection, lung biopsy specimens were sent to the histopathology laboratory and then processed according to standard procedures (Zhao et al., 2021). The remaining lung biopsy specimens were stored at −80°C for mNGS. Part of the BALF was used for Xpert, MTB, and microscopic smears and part was used for fungal and bacterial culture. The remaining BALF specimens were stored at −80°C for mNGS.
Next-Generation Metagenome Sequencing
Alveolar lavage fluid samples (5 ml) and biopsy specimens (200 mg) were collected. After enrichment through centrifugation at 12,000 × g, at 4°C, for 10 min, 400 μl of the treated samples were mixed with glass beads and lysed using BioPrep-24 (TIANGEN BIOTECH, Beijing, China). The microbial DNA was extracted and purified using a magnetic bead method (MAGEN BIOTECH, Guangzhou, China). The concentration of the extracted nucleic acids was measured using a Qubit 4 (Thermo Fisher Scientific) fluorometer. The extracted nucleic acids were used to construct the metagenomic library using NextEra XT DNA Library Prep Kit (Illumina, San Diego, CA, United States), according to the manufacturer’s instructions. In brief, the DNA was fragmented, followed by terminal repair and primer ligation. Finally, the nucleic acids were amplified to construct a library that meets the sequencing requirements (library size: ∼300 bp). The quality of the library was evaluated using a Qubit 4 fluorometer (Thermo Fisher Scientific) and agarose gel electrophoresis. The qualified libraries with different index junction sequences were mixed at equal concentrations. High-throughput sequencing was performed using the Illumina NextSeq 550 DX sequencing platform (sequencing strategy: SE75). During trimming, the low-quality bases, and short sequences (<35 bp) were removed, the remaining clean reads were compared with the human genome reference sequence (version: GRCh38), and Bowtie2 (BWA) was used to remove human-related reads. The remaining reads were further compared with the pathogenic microorganism database1, and the detected sequence number (reads) for pathogenic microorganisms was obtained. According to the interpretation standard of pathogen pathogenicity: (1) Mycobacterium tuberculosis was considered positive when at least one read was aligned to the reference genome at the species or genus level and (2) NTM was defined as positive when the mapping reading (genus or species level) was at the top 10 of the bacterial list; (3) for bacteria other than mycobacteria, viruses, and parasites, the top 10 bacteria in each category were selected, and suspicious pathogens were reported in combination with clinical characteristics (Miao et al., 2018).
Statistical Analysis
Continuous variables corresponding to the two groups were compared using an independent sample t-test or Mann–Whitney U test, and the classified treatments were compared using the chi-square test or the Fisher exact probability method. All statistical analyses were performed using SPSS software v25.0 (IBM, Armonk, NY, United States) and GraphPad Prism 8.0, and statistical significance was defined as p < 0.05.
Results
Baseline Information of Subjects
As shown in Figure 1, between July 2018 and July 2021, 120 hospitalized patients with undetermined causes of pulmonary infection were selected and finally diagnosed with PTB or NTM-PD. Notably, all the patients were AFS sputum smear-negative. However, 26 patients without BALF sample analysis data based on mNGS or Xpert were excluded. Thus, 94 cases (71 PTB cases and 23 NTM-PD cases) were ultimately selected.
As shown in Table 1, the NTM-PD group, which predominantly consisted of female patients (17/23, 73.9%), showed a higher incidence of structural lung disease (14/23, 60.9%), especially bronchiectasis with non-cystic fibrosis than the PTB group (9/71, 39.1%). Further, the imaging results showed a higher incidence of bronchiectasis with non-cystic fibrosis (9/23, 39.1%) and emphysema (4/23, 17.4%) in the NTM-PD group than in the PTB group (p > 0.05). However, there were no significant differences between them with respect to age, symptoms, immune dysfunction, imaging features, histopathology, CRP, PCT, ferritin, white blood cell count, and albumin level. Additionally, 36 cases (38.3%), including 31 in the PTB group and 5 in the NTM-PD group, were immunocompromised to some extent.
Comparison of the Detection Performances of Next-Generation Metagenome Sequencing, Xpert, Acid-Fast Staining (Bronchoalveolar Lavage Fluid Samples), and T-SPOT (Sera Samples) to Distinguish Mycobacterium tuberculosis From Non-tuberculous Mycobacteria
As shown in Table 2 and Figures 2, 3A, out of the 71 patients with PTB, based on the analysis of BALF samples, 67 cases were detected using mNGS, resulting in a sensitivity of 94.4%, followed by Xpert, with a sensitivity of 85.9% (61/71), which was significantly higher than that of the conventional methods T-SPOT 64.8% (46/71) and AFS 28.2% (20/71), and the difference was statistically significant (p < 0.001). However, there was no significant difference in sensitivity between mNGS and Xpert (p > 0.05). When the two rapid detection methods mNGS and Xpert were combined, 69 cases of MTB were detected with the highest sensitivity (97.2%), which was statistically significant compared with that of the conventional method (p < 0.001). Notably, for the NTM-PD group, the specificity of Xpert was 100%, implying that all the patients with NTM-PD were Xpert-negative. However, the specificities of AFS and T-SPOT were 73.9% (17/23) and 91.3% (21/23), respectively. These results indicated that neither AFS only nor T-SPOT only can be used to diagnose PTB. Further, mNGS showed the ability to recognize both MTB and NTM, indicating its superior diagnostic performance relative to Xpert, AFS, and T-SPOT. Notably, the 23 patients with NTM-PD in this study could all be identified using mNGS, indicating that the specificity of mNGS was the same as that of Xpert. The specificity was statistically significant compared with that of conventional methods (p < 0.001).
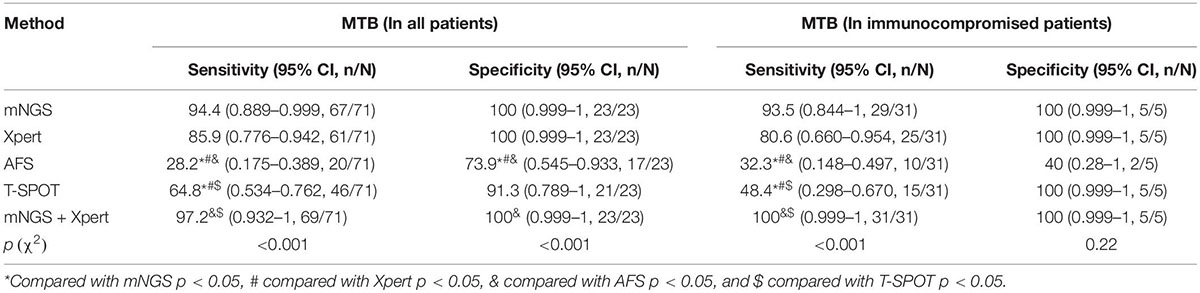
Table 2. Comparison of the detection performances of mNGS, Xpert, AFS (BALF samples), and T-SPOT (sera samples) in all patients with Mycobacterium tuberculosis infection and immunocompromised patients.
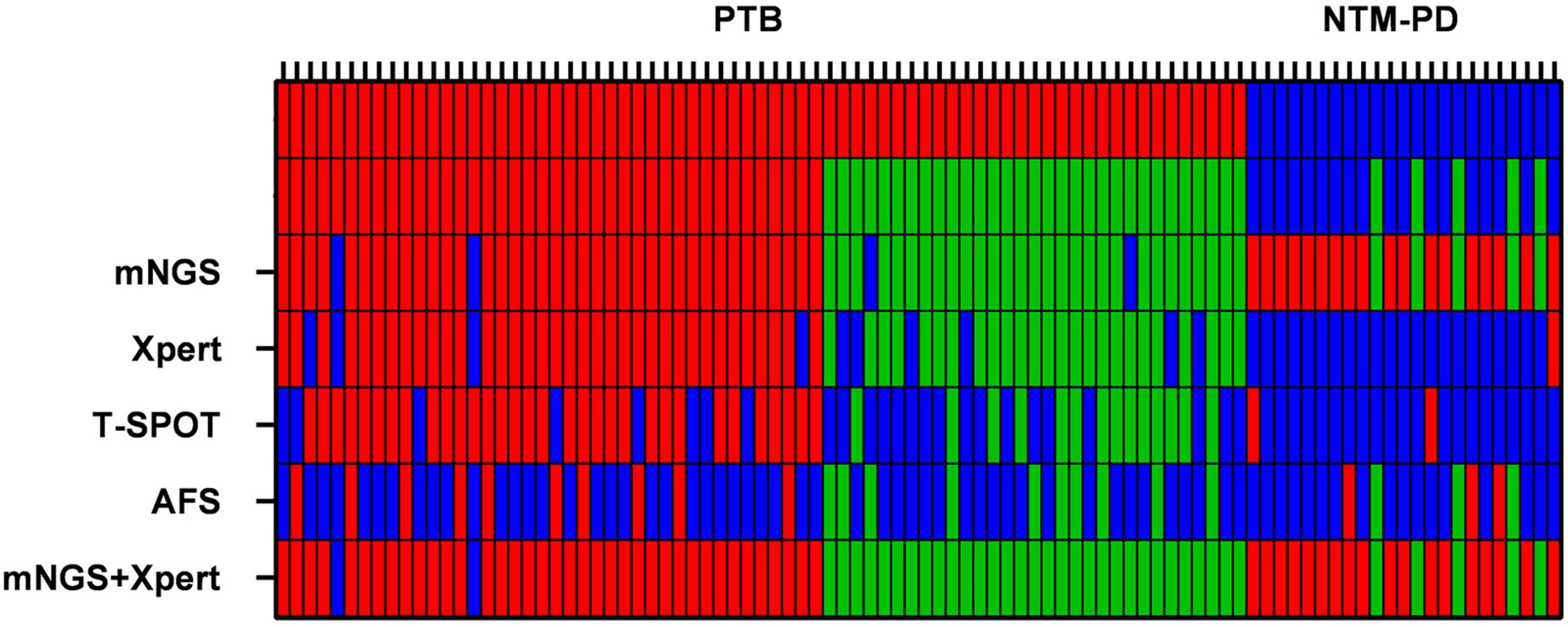
Figure 2. Heat maps indicating the performances of mNGS, Xpert, AFS (BALF samples), and T-SPOT (sera samples) in the diagnosis of MTB and NTM. The red bars indicate that MTB and NTM were detected correctly. The green bars indicate that MTB and NTM were detected correctly in immunocompromised patients.
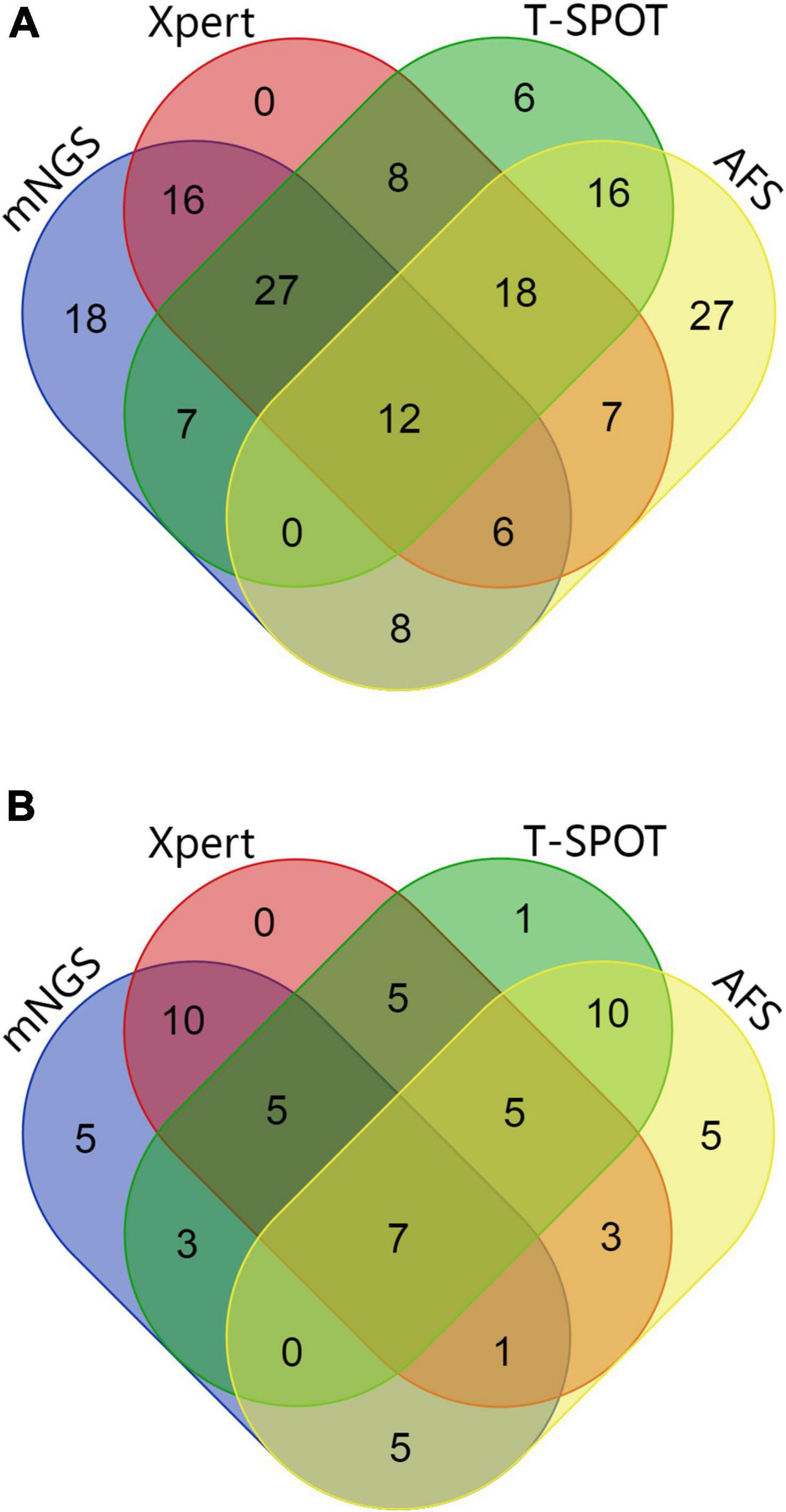
Figure 3. (A) Wayne diagram showing the evaluation of the performances of mNGS, Xpert, AFS (BALF samples), and T-SPOT (sera samples) with respect to the detection of MTB and NTM. (B) Wayne diagram showing the evaluation of the performances of mNGS, Xpert, AFS (BALF samples), and T-SPOT (sera samples) in immunocompromised patients for the detection of MTB and NTM.
Immunocompromised hosts are prone to disseminated infection, which increases mortality rate, after PTB or NTM-PD (Ratnatunga et al., 2020). Given that the non-specific symptoms, signs, and imaging findings corresponding to TB often overlap with those of other pulmonary bacterial infections, the use of conventional antibiotics leads to a low diagnosis performance for the traditional diagnostic methods, leading to prolonged disease and poor therapeutic effects (Mukhopadhyay and Gal, 2010; Nachiappan et al., 2017). mNGS is a molecular method that can be used to detect pathogen DNA. Therefore, we performed subgroup analysis and selected individuals with low immunity for this study. As shown in Table 1, 36 immunocompromised patients were included in this study. Among them, 16 were treated with corticosteroids at doses ≥20 mg/day for connective tissue disease and other diseases for more than 14 days, 9 had neutropenia (<1,000 cells per μL) due to hematological malignancies, 10 received immunosuppressive therapy after hematopoietic stem cell transplantation, and 2 with lung malignant tumors received maintenance chemotherapy within 28 days.
Additionally, as shown in Table 2 and Figures 2, 3B, based on BALF analysis, 29 cases of MTB were detected using mNGS, which showed a diagnostic sensitivity of 93.5%, followed by Xpert, with a sensitivity of 80.6% (25/31), while the sensitivities of T-SPOT and AFS were 48.4% (15/31) and 32.3% (10/31), respectively. Notably, the diagnostic sensitivities of Xpert and T-SPOT were reduced in this subgroup. However, the sensitivity of mNGS was not significantly different from that of Xpert (p > 0.05). After mNGS and Xpert were combined, all MTB cases were detected, and the diagnostic sensitivity (100%) was the highest, which was statistically significant compared with that of the conventional method (p < 0.001). This indicates that the combination of mNGS and Xpert can significantly improve the diagnostic sensitivity for PTB in immunocompromised populations. For the NTM-PD groups, the specificities of Xpert and T-SPOT were both 100%, and the specificity of AFS was 40% (2/5). These findings indicated that AFS only and T-SPOT only cannot be used for the diagnosis of PTB in immunocompromised patients. It is worth noting that there were five patients with NTM-PD in this subgroup, and they all could be identified using mNGS.
Our findings also indicated that using BALF samples, MTB was not detected in 4 out of the 71 patients with PTB using mNGS; however, when mNGS was performed using bronchoscopic lung biopsy samples, MTB could be diagnosed in these 4 patients.
Identification of Non-tuberculous Mycobacteria Strains and Mixed Infection in Bronchoalveolar Lavage Fluid Samples Using Next-Generation Metagenome Sequencing
The strains from the 23 patients in the NTM-PD group in this study were identified using mNGS. Among the patients, there were 12 cases of Mycobacterium intracellulare, five cases of Mycobacterium avium, two cases of Mycobacterium kansasii, two cases of Mycobacterium abscessus, one case of Mycobacterium xenopi, and 1 case of Mycobacterium scrofulaceum.
As shown in Figures 4, 5, mNGS could also identify other mixed-pathogen infections (including bacteria, fungi, and viruses). Among the 94 patients included in our study, 50 cases were complicated by other pathogenic bacterial infections, and most of these cases were complicated with immunosuppression and structural lung disease. Further, we compared mNGS with routine clinical detection methods (BALF bacterial and fungal culture, bacterial and fungal microscopic smears, serum Aspergillus IgG antibody, GM test, Cryptococcus antigen detection, respiratory syncytial virus antibody, Cytomegalovirus antibody, adenovirus antibody, EB virus antibody, and human herpesvirus type I and type II antibodies). All common bacteria, including Haemophilus parainfluenzae, Klebsiella pneumoniae, Streptococcus pneumoniae, Pseudomonas aeruginosa, Acinetobacter baumannii, and Stenotrophomonas maltophilia, were detected by mNGS, with the sensitivity being significantly higher than that of BALF routine bacterial culture and microscopic smear examinations (34.5%, 10/29). Further, six cases, all of which were detected using mNGS, were complicated by fungal infection (Aspergillus spp.). One case of Aspergillus fumigatus infection was detected using the BALF fungal culture method, and three cases were positive based on fungal serum immunoassay. Furthermore, mNGS could also detect one case of Pneumocystis carinii, one case of Legionella pneumophila, one case of St. George Nocardia, and three cases of the genus Actinomyces spp., which could not be detected using the conventional pathogen detection methods. Ten patients with viral infections, including Circovirus, Cytomegalovirus, parainfluenza virus, and polyomavirus, were also detected using mNGS. Three cases of Cytomegalovirus antibody (IgM and IgG) and one case of EB virus antibody (IgM) were detected using the traditional etiological methods, while others were not detected.
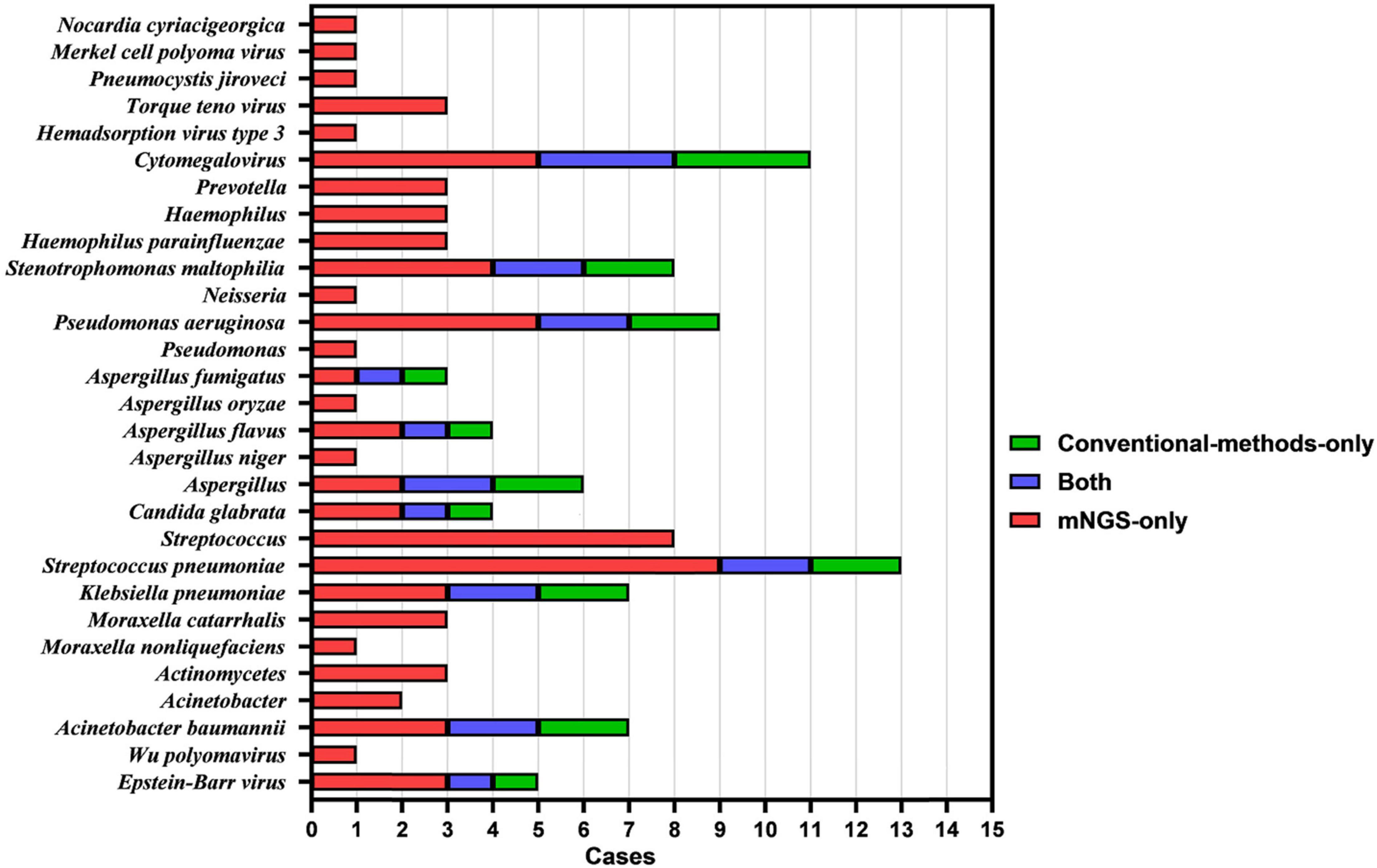
Figure 4. Histogram showing the results of mNGS and conventional methods in identifying mixed infection in BALF specimens of patients with PTB and NTM-PD.
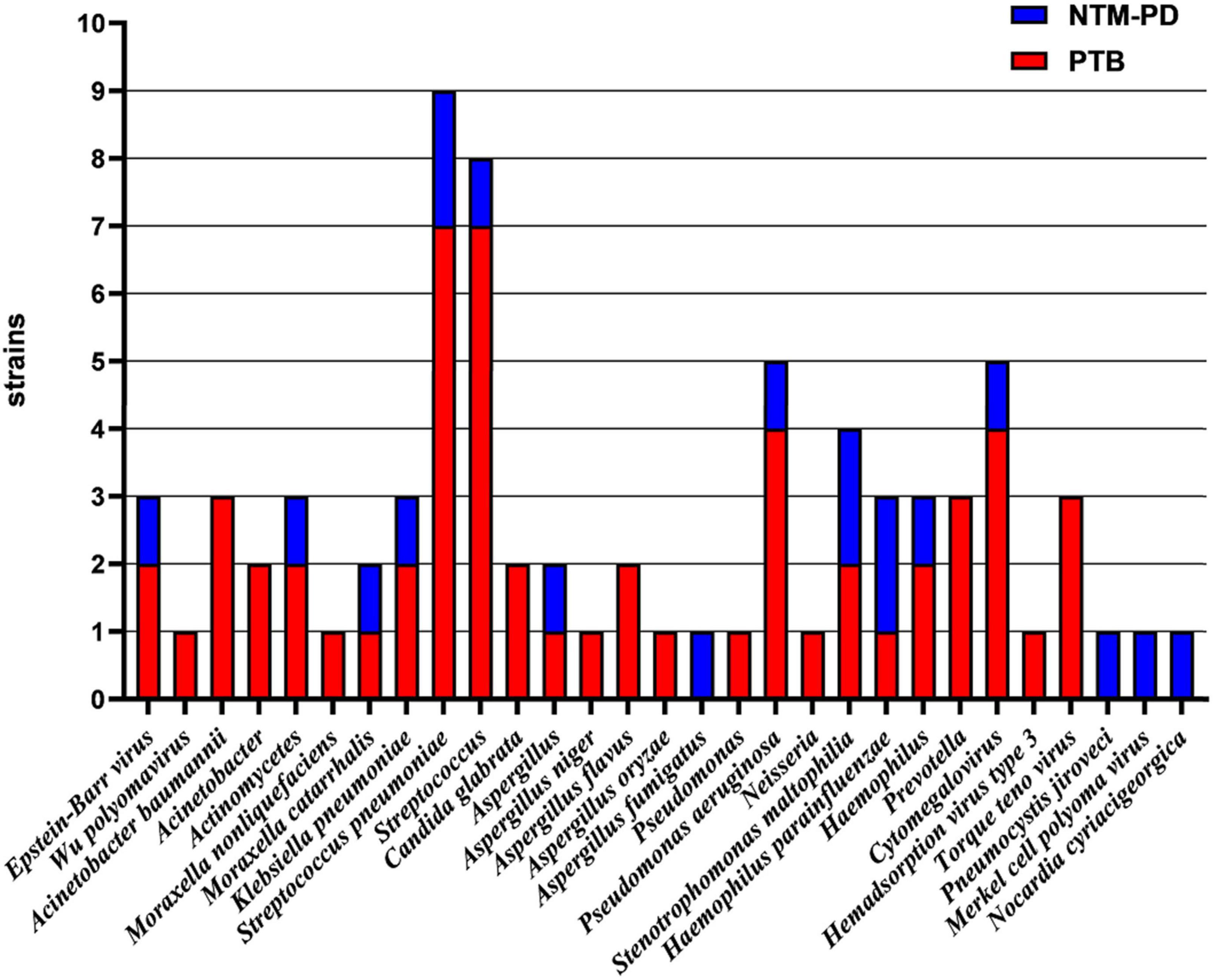
Figure 5. Identification of strains in BALF samples from patients with PTB and NTM-PD complicated with co-infection using mNGS.
We also found that 44% (n = 22) of PTB patients with co-infection were complicated with one microbial infection, 22% (n = 11) with two microbial infections, 10% (n = 5) with three microbial infections, and 2% (n = 1) with five microbial infections. Among NTM-PD patients with co-infection, the proportions of patients co-infected with one, two, three, and four microorganisms were 10% (n = 5), 6% (n = 3), 4% (n = 2), and 2% (n = 1), respectively.
Discussion
In this study, we comprehensively evaluated the performance of mNGS for the etiological diagnosis of suspected PTB patients with negative sputum smear. In the diagnosis of PTB, the diagnostic sensitivity of mNGS (94.4%) was higher than those of Xpert (85.9%) and the conventional diagnostic methods T-SPOT (64.8%) and AFS (28.2%). The diagnostic sensitivity of mNGS combined with Xpert (97.2%) was the highest, suggesting that the combination of mNGS and Xpert can improve the diagnosis of PTB, which is consistent with other reports (Zhou et al., 2019; Shi et al., 2020; Liu et al., 2021). However, the sensitivity of mNGS in this study was not significantly different from that of Xpert. Thus, mNGS is not superior to Xpert in the detection of MTB, consistent with the conclusion of other studies (Zhou et al., 2019; Shi et al., 2020). However, compared with these three detection methods, mNGS has a prominent advantage in that it can also identify NTM, which is difficult for culture-based methods to detect, because some NTM species require a time-consuming procedure to detect and some are difficult to culture (Kim et al., 2018). Since our patients used antibiotics before they were enrolled, we believe that mNGS has a higher accuracy than conventional diagnostic methods in detecting MTB and NTM in BALF specimens. Therefore, its use in the diagnosis of TB using BALF samples is a reliable method to distinguish MTB from NTM.
The importance of our study lies in the fact that this patient population lacks specific clinical symptoms, and their imaging features can be easily mistaken for pulmonary infection. Thus, patients with compromised immune function are confused with the original diseases or are considered to be infected with multiple pathogens if the clinical features of TB change. This can lead to missed diagnoses, misdiagnoses, and increased TB-related deaths (Miller et al., 2000; Liam et al., 2006). However, we found that the diagnostic sensitivity of Xpert and T-SPOT was also decreased in the immunocompromised subgroups, and Xpert was prone to false negative results, consistent with other studies (Lee et al., 2019, 2020). The combination of mNGS and Xpert can significantly improve the diagnostic sensitivity for PTB in immunocompromised populations, which has not been mentioned in other studies on the detection of Mycobacterium tuberculosis by mNGS and Xpert.
Studies have shown that the clinical manifestations of PTB and NTM-PD are similar and that they can have common CT manifestations in imaging, including tree bud-like signs, bronchiectasis, and cavities; moreover, necrotizing granulomas can represent histopathological features of both diseases (Mukhopadhyay, 2011; Nachiappan et al., 2017), which are consistent with the results of our study. However, in the present study, NTM was detected using mNGS, revealing the feasibility and unique advantages of mNGS in distinguishing MTB from NTM. The risk of NTM-PD was significantly higher (P < 0.05) in patients with structural pulmonary disease (14/23, 60.9%), especially bronchiectasis without cystic fibrosis (9/23, 39.1%), compared to that for PTB; this was consistent with the results of previous study (Cowman et al., 2019). Our study included 14 structural lung diseases in 23 patients with NTM-PD. For patients with structural or inflammatory lung diseases, such as non-cystic fibrobronchiectasis, chronic obstructive pulmonary disease, or interstitial lung disease, the accurate diagnosis of NTM infection is critical because these emerging pathogens can lead to an accelerated decline in lung function and fatal complications (Floto et al., 2016).
Additionally, it has been reported that patients with impaired immune function and structural lung disease are more susceptible to fungal and other microbial infections after PTB and NTM-PD (Wu and Holland, 2015; Zeng et al., 2020). Specifically, when an immune-impaired host has a mixed infection of pulmonary infiltration, the successful diagnosis of co-existing opportunistic infections using conventional diagnostic methods is challenging (Pan et al., 2019). Further, the use of antibiotics decreases the diagnostic rate of conventional diagnostic methods to a low level. In our study, 46% of the patients with co-infection were infected with more than two types of microorganisms. Our findings also indicated that the analysis of BALF samples using mNGS can lead to the simultaneous detection of mixed infections by bacteria, fungi, viruses, and mycobacteria, which is consistent with other reports (Pan et al., 2019; Xie et al., 2019; Zhao et al., 2021). The results of our study suggested that mNGS is a good diagnostic method for patients with pulmonary infections who have poor responses to empirical antibiotics and are AFS sputum smear-negative. mNGS also offers the possibility to identify not only MTB and NTM but also other mixed infections. Further, it is also suitable for application in immunocompromised patients with complex infections. This not only indicates an improvement in diagnostic ability in immunocompromised people with acute lower respiratory tract infections (LRTI) but also can help clinicians evaluate patients more comprehensively and prescribe a more effective treatment in time.
In our study, MTB was not detected by mNGS in four BALF specimens, although it was discovered in the four bronchoscopic lung biopsies from these patients. It has been reported that the diagnostic rate of mNGS in lung biopsy is higher than that in BALF (Mondoni et al., 2017), probably because the bacterial load in diseased tissue is higher than that in the sputum and BALF. These results suggest that we can use mNGS to analyze lung tissues from patients who are highly suspected of PTB, but whose mNGS results are negative based on the analysis of BALF.
Although some encouraging results were obtained in this study, some limitations were also noted. First, due to the relatively high cost, the widespread clinical application of mNGS may be under restrictions (Lee and Pai, 2017; Lee et al., 2019). Second, diagnosis of infections using invasive techniques, such as bronchoalveolar lavage, may lead to further respiratory deterioration in patients with hypoxemia (Bauer et al., 2019; Azoulay et al., 2020), thus the collection of BALF samples is limited. Third, the sample size was relatively small, as this was a single-center retrospective study. Therefore, a multicenter prospective study with a larger sample size is necessary to further confirm these findings.
In summary, the findings of this study indicate that the analysis of BALF samples using mNGS has a high accuracy in the differential diagnosis of MTB and NTM. Further, mNGS combined with Xpert can improve the detection of MTB, especially in AFS sputum smear-negative samples from patients with compromised immune states or poor responses to empirical antibiotics. Therefore, we believe that mNGS is a powerful tool for identifying pathogenic microorganisms, and further investigations are required on this aspect.
Data Availability Statement
The data presented in the study are deposited in the NCBI and National Genomics Data Center repository, accession numbers: PRJNA847681, PRJNA848420, and PRJCA010004, respectively.
Ethics Statement
The studies involving human participants were reviewed and approved by the Ethics Review Committee of Tianjin Medical University General Hospital. Written informed consent to participate in this study was provided by the participants’ legal guardian/next of kin.
Author Contributions
YW and JF contributed to the research design and revision of the manuscript. PX and KY drafted the research protocol, analyzed the results, and drafted the manuscript. LY, ZW, and FJ contributed to the data analysis and data deposition in an acceptable repository. All authors approved the submitted version and agreed to be responsible for all aspects.
Funding
This research was supported by the National Natural Science Foundation of China (81970083, 81270144, 81570084, and 30800507 to JF), National Key Technology R&D Program of China (2015BAI12B00 to JF), Natural Science Foundation of Inner Mongolia Autonomous Region of China (2021MS0801 to PX), Research Program of Science and Technology at Universities of Inner Mongolia Autonomous Region (NJZY21584 to PX), Medical Program of Inner Mongolia Autonomous Region (202202198 to PX), Educational Reform Program of Inner Mongolia University (NYJXGG2021085 to PX), and General Program of Inner Mongolia University [YKD2020KJBW(LH)007 to PX].
Conflict of Interest
The authors declare that the research was conducted in the absence of any commercial or financial relationships that could be construed as a potential conflict of interest.
Publisher’s Note
All claims expressed in this article are solely those of the authors and do not necessarily represent those of their affiliated organizations, or those of the publisher, the editors and the reviewers. Any product that may be evaluated in this article, or claim that may be made by its manufacturer, is not guaranteed or endorsed by the publisher.
Acknowledgments
We would like to thank Zhide Hu for providing useful inspiration, suggestions, and technical guidance.
Footnotes
References
Association, T. B. O. C. M. (2020). Guidelines for the Diagnosis and Treatment of Nontuberculous Mycobacterial Diseases (2020 edition) [J]. Chin. J. Tuberculosis Respir. Dis. 43, 918–946. doi: 10.3760/cma.j.cn112147-20200508-00570
Azoulay, E., Russell, L., Van de Louw, A., Metaxa, V., Bauer, P., Povoa, P., et al. (2020). Diagnosis of severe respiratory infections in immunocompromised patients. Intensive Care Med. 46, 298–314. doi: 10.1007/s00134-019-05906-5
Batyrshina, Y. R., and Schwartz, Y. S. (2019). Modeling of Mycobacterium tuberculosis dormancy in bacterial cultures. Tuberculosis 117, 7–17. doi: 10.1016/j.tube.2019.05.005
Bauer, P. R., Chevret, S., Yadav, H., Mehta, S., Pickkers, P., Bukan, R. B., et al. (2019). Diagnosis and outcome of acute respiratory failure in immunocompromised patients after bronchoscopy. Eur. Respir. J. 54:1802442. doi: 10.1183/13993003.02442-2018
Brown, A. C., Bryant, J. M., Einer-Jensen, K., Holdstock, J., Houniet, D. T., Chan, J. Z., et al. (2015). Rapid whole-genome sequencing of Mycobacterium tuberculosis isolates directly from clinical samples. J. Clin. Microbiol. 53, 2230–2237. doi: 10.1128/JCM.00486-15
Bryant, J. M., Grogono, D. M., Greaves, D., Foweraker, J., Roddick, I., Inns, T., et al. (2013). Whole-genome sequencing to identify transmission of Mycobacterium abscessus between patients with cystic fibrosis: a retrospective cohort study. Lancet 381, 1551–1560. doi: 10.1016/S0140-6736(13)60632-7
Chiu, C. Y., and Miller, S. A. (2019). Clinical metagenomics. Nat. Rev. Genet. 20, 341–355. doi: 10.1038/s41576-019-0113-7
Committee, W. G. A. B. T. G. R. (2010). WHO Guidelines Approved by the Guidelines Review Committee. Treatment of Tuberculosis: Guidelines. Geneva: World Health Organization.
Cowman, S., van Ingen, J., Griffith, D. E., and Loebinger, M. R. (2019). Non-tuberculous mycobacterial pulmonary disease. Eur. Respir. J. 1:54. doi: 10.1183/13993003.00250-2019
Daley, C. L., Iaccarino, J. M., Lange, C., Cambau, E., Wallace, R. J., Andrejak, C., et al. (2020). Treatment of nontuberculous mycobacterial pulmonary disease: an official ATS/ERS/ESCMID/IDSA clinical practice guideline. Eur. Respir. J. 56:2020. doi: 10.1183/13993003.00535-2020
Deshwal, H., Avasarala, S. K., Ghosh, S., and Mehta, A. C. (2019). Forbearance with bronchoscopy: a review of gratuitous indications. Chest 155, 834–847. doi: 10.1016/j.chest.2018.08.1035
Doan, T., Wilson, M. R., Crawford, E. D., Chow, E. D., Khan, L. M., Knopp, K. A., et al. (2016). Illuminating uveitis: metagenomic deep sequencing identifies common and rare pathogens. Genome Med. 8:90. doi: 10.1186/s13073-016-0344-6
Doyle, R. M., Burgess, C., Williams, R., Gorton, R., Booth, H., Brown, J., et al. (2018). Direct whole-genome sequencing of sputum accurately identifies drug-resistant Mycobacterium tuberculosis Faster than MGIT culture sequencing. J. Clin. Microbiol. 56:18. doi: 10.1128/JCM.00666-18
Electron (2018). Diagnostic criteria for pulmonary tuberculosis (WS 288–2017). J. Emerg. Infect. Dis. 3, 59–61. doi: 10.19871/j.cnki.xfcrbzz.2018.01.017
Floto, R. A., Olivier, K. N., Saiman, L., Daley, C. L., Herrmann, J., Nick, J. A., et al. (2016). US Cystic Fibrosis Foundation and European Cystic Fibrosis Society consensus recommendations for the management of non-tuberculous mycobacteria in individuals with cystic fibrosis. Thorax 71, (Suppl. 1), i1–i22. doi: 10.1136/thoraxjnl-2015-207360
Guan, H., Shen, A., Lv, X., Yang, X., Ren, H., Zhao, Y., et al. (2016). Detection of virus in CSF from the cases with meningoencephalitis by next-generation sequencing. J. Neurovirol. 22, 240–245. doi: 10.1007/s13365-015-0390-7
Harris, K. A., Underwood, A., Kenna, D. T., Brooks, A., Kavaliunaite, E., Kapatai, G., et al. (2015). Whole-genome sequencing and epidemiological analysis do not provide evidence for cross-transmission of mycobacterium abscessus in a cohort of pediatric cystic fibrosis patients. Clin. Infect. Dis. 60, 1007–1016. doi: 10.1093/cid/ciu967
Haworth, C. S., Banks, J., Capstick, T., Fisher, A. J., Gorsuch, T., Laurenson, I. F., et al. (2017). British Thoracic Society guidelines for the management of non-tuberculous mycobacterial pulmonary disease (NTM-PD). Thorax 72, (Suppl. 2), ii1–ii64. doi: 10.1136/thoraxjnl-2017-210927
He, Y., Gong, Z., Zhao, X., Zhang, D., and Zhang, Z. (2020). Comprehensive determination of Mycobacterium tuberculosis and nontuberculous mycobacteria from targeted capture sequencing. Front. Cell. Infect. Microbiol. 10:449. doi: 10.3389/fcimb.2020.00449
Huang, Z., Zhang, C., Fang, X., Li, W., Zhang, C., Zhang, W., et al. (2019). Identification of musculoskeletal infection with non-tuberculous mycobacterium using metagenomic sequencing. J. Infectol. 78, 158–169. doi: 10.1016/j.jinf.2018.10.002
Kim, J. U., Ryu, D. S., Cha, C. H., and Park, S. H. (2018). Paradigm for diagnosing mycobacterial disease: Direct detection and differentiation of Mycobacterium tuberculosis complex and non-tuberculous mycobacteria in clinical specimens using multiplex real-time PCR. J. Clin. Pathol. 71, 774–780. doi: 10.1136/jclinpath-2017-204945
Langelier, C., Zinter, M. S., Kalantar, K., Yanik, G. A., Christenson, S., O’Donovan, B., et al. (2018). Metagenomic sequencing detects respiratory pathogens in hematopoietic cellular transplant patients. Am. J. Respir. Crit. Care Med. 197, 524–528. doi: 10.1164/rccm.201706-1097LE
Lee, H. S., Kee, S. J., Shin, J. H., Kwon, Y. S., Chun, S., Lee, J. H., et al. (2019). Xpert MTB/RIF assay as a substitute for smear microscopy in an intermediate-burden setting. Am. J. Respir. Crit. Care Med. 199, 784–794. doi: 10.1164/rccm.201804-0654OC
Lee, R. S., and Pai, M. (2017). Real-time sequencing of Mycobacterium tuberculosis: are we there yet? J. Clin. Microbiol. 55, 1249–1254. doi: 10.1128/JCM.00358-17
Lee, S. W., Kang, Y. A., Jin, C. E., Kim, H. C., Noh, G. S., Lee, H. J., et al. (2020). Gene-based diagnosis of tuberculosis with a new-generation pathogen enrichment technique. Eur. Respir. J. 55:2019. doi: 10.1183/13993003.01885-2019
Liam, C. K., Pang, Y. K., and Poosparajah, S. (2006). Pulmonary tuberculosis presenting as community-acquired pneumonia. Respirology 11, 786–792. doi: 10.1111/j.1440-1843.2006.00947.x
Liu, X., Chen, Y., Ouyang, H., Liu, J., Luo, X., Huang, Y., et al. (2021). Tuberculosis Diagnosis by metagenomic Next-generation Sequencing on bronchoalveolar lavage Fluid: a cross-sectional analysis. Int. J. Infect. Dis. 104, 50–57. doi: 10.1016/j.ijid.2020.12.063
Long, Y., Zhang, Y., Gong, Y., Sun, R., Su, L., Lin, X., et al. (2016). Diagnosis of sepsis with cell-free DNA by next-generation sequencing technology in ICU patients. Arch. Med. Res. 47, 365–371. doi: 10.1016/j.arcmed.2016.08.004
Maiga, M., Siddiqui, S., Diallo, S., Diarra, B., Traoré, B., Shea, Y. R., et al. (2012). Failure to recognize nontuberculous mycobacteria leads to misdiagnosis of chronic pulmonary tuberculosis. PLoS One 7:e36902. doi: 10.1371/journal.pone.0036902
Meyer, K. C., Raghu, G., Baughman, R. P., Brown, K. K., Costabel, U., du Bois, R. M., et al. (2012). An official American Thoracic Society clinical practice guideline: The clinical utility of bronchoalveolar lavage cellular analysis in interstitial lung disease. Am. J. Respir. Crit. Care Med. 185, 1004–1014. doi: 10.1164/rccm.201202-0320ST
Miao, Q., Ma, Y., Wang, Q., Pan, J., Zhang, Y., Jin, W., et al. (2018). Microbiological diagnostic performance of metagenomic next-generation sequencing when applied to clinical practice. Clin. Infect. Dis. 67, S231–S240. doi: 10.1093/cid/ciy693
Miller, L. G., Asch, S. M., Yu, E. I., Knowles, L., Gelberg, L., and Davidson, P. (2000). A population-based survey of tuberculosis symptoms: how atypical are atypical presentations? Clin. Infect. Dis. 30, 293–299. doi: 10.1086/313651
Mondoni, M., Repossi, A., Carlucci, P., Centanni, S., and Sotgiu, G. (2017). Bronchoscopic techniques in the management of patients with tuberculosis. Int. J. Infect. Dis. 64, 27–37. doi: 10.1016/j.ijid.2017.08.008
Mukhopadhyay, S., and Gal, A. A. (2010). Granulomatous lung disease: an approach to the differential diagnosis. Arch. Pathol. Lab. Med. 134, 667–690. doi: 10.5858/134.5.667
Mukhopadhyay, S. (2011). Role of histology in the diagnosis of infectious causes of granulomatous lung disease. Curr. Opin. Pulm. Med. 17, 189–196. doi: 10.1097/MCP.0b013e3283447bef
Nachiappan, A. C., Rahbar, K., Shi, X., Guy, E. S., Mortani Barbosa, E. J., Shroff, G. S., et al. (2017). Pulmonary tuberculosis: role of radiology in diagnosis and management. Radiographics 37, 52–72. doi: 10.1148/rg.2017160032
Operario, D. J., Pholwat, S., Koeppel, A. F., Prorock, A., Bao, Y., Sol-Church, K., et al. (2019). Mycobacterium avium complex diversity within lung disease, as revealed by whole-genome sequencing. Am. J. Respir. Crit. Care Med. 200, 393–396. doi: 10.1164/rccm.201903-0669LE
Pai, M., Behr, M. A., Dowdy, D., Dheda, K., Divangahi, M., Boehme, C. C., et al. (2016a). Tuberculosis. Nat. Rev. Dis. Primers. 2:16076. doi: 10.1038/nrdp.2016.76
Pai, M., Nicol, M. P., and Boehme, C. C. (2016b). Tuberculosis Diagnostics: State of the art and future directions. Microbiol. Spectr. 4:2016. doi: 10.1128/microbiolspec.TBTB2-0019-2016
Pan, T., Tan, R., Qu, H., Weng, X., Liu, Z., Li, M., et al. (2019). Next-generation sequencing of the BALF in the diagnosis of community-acquired pneumonia in immunocompromised patients. J. Infect. 79, 61–74. doi: 10.1016/j.jinf.2018.11.005
Qu, J. M., and Cao, B. (2016). Chinese adult community-acquired pneumonia diagnosis and treatment guidelines. Chin. J. Tuberc. Respir. Dis. 39, 253–279. doi: 10.3760/cma.j.issn.1001-0939.2016.04.005
Ratnatunga, C. N., Lutzky, V. P., Kupz, A., Doolan, D. L., Reid, D. W., Field, M., et al. (2020). The rise of non-tuberculosis mycobacterial lung disease. Front. Immunol. 11:303. doi: 10.3389/fimmu.2020.00303
Sassi, M., and Drancourt, M. (2014). Genome analysis reveals three genomospecies in Mycobacterium abscessus. BMC Genomics 15:359. doi: 10.1186/1471-2164-15-359
Shahraki, A. H., Heidarieh, P., Bostanabad, S. Z., Khosravi, A. D., Hashemzadeh, M., Khandan, S., et al. (2015). “Multidrug-resistant tuberculosis” may be nontuberculous mycobacteria. Eur. J. Intern. Med. 26, 279–284. doi: 10.1016/j.ejim.2015.03.001
Shi, C. L., Han, P., Tang, P. J., Chen, M. M., Ye, Z. J., Wu, M. Y., et al. (2020). Clinical metagenomic sequencing for diagnosis of pulmonary tuberculosis. J. Infect. 81, 567–574. doi: 10.1016/j.jinf.2020.08.004
Taur, P. D., Gowri, V., Pandrowala, A. A., Iyengar, V. V., Chougule, A., Golwala, Z., et al. (2021). Clinical and molecular findings in Mendelian susceptibility to mycobacterial diseases: experience from India. Front. Immunol. 12:631298. doi: 10.3389/fimmu.2021.631298
Votintseva, A. A., Bradley, P., Pankhurst, L., Del Ojo, Elias, C., Loose, M., et al. (2017). Same-day diagnostic and surveillance data for tuberculosis via whole-genome sequencing of direct respiratory samples. J. Clin. Microbiol. 55, 1285–1298. doi: 10.1128/JCM.02483-16
Wu, U. I., and Holland, S. M. (2015). Host susceptibility to non-tuberculous mycobacterial infections. Lancet Infect. Dis. 15, 968–980. doi: 10.1016/S1473-3099(15)00089-4
Xie, Y., Du, J., Jin, W., Teng, X., Cheng, R., Huang, P., et al. (2019). Next generation sequencing for diagnosis of severe pneumonia: China, 2010–2018. J. Infect. 78, 158–169. doi: 10.1016/j.jinf.2018.09.004
Young, D. B., Perkins, M. D., Duncan, K., and Barry, C. E. (2008). Confronting the scientific obstacles to global control of tuberculosis. J. Clin. Invest. 118, 1255–1265. doi: 10.1172/JCI34614
Zeng, Q. Z., Zhang, Y. Y., Wu, Y. J., Zhang, Z. Y., Zhang, J. N., Fu, H. X., et al. (2020). Frequency, Risk Factors, and Outcome of Active tuberculosis following Allogeneic hematopoietic Stem Cell Transplantation. Biol. Blood Marrow Transplant. 26, 1203–1209. doi: 10.1016/j.bbmt.2020.02.018
Zhang, H. C., Ai, J. W., Cui, P., Zhu, Y. M., Hong-Long, W., Li, Y. J., et al. (2019). Incremental value of metagenomic next generation sequencing for the diagnosis of suspected focal infection in adults. J. Infect. 79, 419–425. doi: 10.1016/j.jinf.2019.08.012
Zhao, M., Tang, K., Liu, F., Zhou, W., Fan, J., Yan, G., et al. (2020). Metagenomic next-generation sequencing improves diagnosis of osteoarticular infections from abscess specimens: A multicenter retrospective study. Front. Microbiol. 11:2034. doi: 10.3389/fmicb.2020.02034
Zhao, Z., Song, J., Yang, C., Yang, L., Chen, J., Li, X., et al. (2021). Prevalence of fungal and bacterial co-infection in pulmonary fungal infections: A metagenomic next generation sequencing-based study. Front. Cell. Infect. Microbiol. 11:749905. doi: 10.3389/fcimb.2021.749905
Zhou, X., Wu, H., Ruan, Q., Jiang, N., Chen, X., Shen, Y., et al. (2019). Clinical evaluation of diagnosis efficacy of active Mycobacterium tuberculosis complex infection via metagenomic next-generation sequencing of direct clinical samples. Front. Cell. Infect. Microbiol. 9:351. doi: 10.3389/fcimb.2019.00351
Keywords: bronchoalveolar lavage fluid, pulmonary tuberculosis, next-generation metagenome sequencing, diagnostic value, non-tuberculous mycobacteria
Citation: Xu P, Yang K, Yang L, Wang Z, Jin F, Wang Y and Feng J (2022) Next-Generation Metagenome Sequencing Shows Superior Diagnostic Performance in Acid-Fast Staining Sputum Smear-Negative Pulmonary Tuberculosis and Non-tuberculous Mycobacterial Pulmonary Disease. Front. Microbiol. 13:898195. doi: 10.3389/fmicb.2022.898195
Received: 26 March 2022; Accepted: 07 June 2022;
Published: 01 July 2022.
Edited by:
Xin Zhou, Stanford University, United StatesReviewed by:
Mandira Varma Basil, University of Delhi, IndiaYinzhou Zhu, 10x Genomics, United States
Copyright © 2022 Xu, Yang, Yang, Wang, Jin, Wang and Feng. This is an open-access article distributed under the terms of the Creative Commons Attribution License (CC BY). The use, distribution or reproduction in other forums is permitted, provided the original author(s) and the copyright owner(s) are credited and that the original publication in this journal is cited, in accordance with accepted academic practice. No use, distribution or reproduction is permitted which does not comply with these terms.
*Correspondence: Yubao Wang, yubaowang2020@hotmail.com; Jing Feng, zyyhxkfj@126.com
†These authors have contributed equally to this work and share first authorship