- 1Institute of Applied Genomics, Fuzhou University, Fuzhou, China
- 2College of Biological Science and Engineering, Fuzhou University, Fuzhou, China
As the market size of the cosmetics industry increases, the safety and effectiveness of new products face higher requirements. The marine environment selects for species of micro-organisms with metabolic pathways and adaptation mechanisms different from those of terrestrial organisms, resulting in their natural products exhibiting unique structures, high diversity, and significant biological activities. Natural products are usually safe and non-polluting. Therefore, considerable effort has been devoted to searching for cosmetic ingredients that are effective, safe, and natural for marine micro-organisms. However, marine micro-organisms can be difficult, or impossible, to culture because of their special environmental requirements. Metagenomics technology can help to solve this problem. Moreover, using marine species to produce more green and environmentally friendly products through biotransformation has become a new choice for cosmetic manufacturers. In this study, the natural products of marine micro-organisms are reviewed and evaluated with respect to various cosmetic applications.
Introduction
The skin is the largest organ of the human body and plays an important protective role (Resende et al., 2021). As humans age, their skin becomes thinner and loses its original elasticity and moisturizing ability. Aged skin is dry, flabby, wrinkled, and increasingly fragile (Wang et al., 2015; Resende et al., 2021). Because skin has prolonged contact with the outside world, external factors, such as UV radiation, dust, and chemical reagents, can reduce skin’s antioxidant capacity and accelerate its aging rate (Resende et al., 2021). Skin care is essential for maintaining its appearance and health, but it also strengthens the barrier function of the skin (Bedoux et al., 2014). The concept of skin care is well known. With the idea of skincare gaining popularity, many antiaging creams, moisturizers, and sunscreens are on the market. However, the majority of cosmetics sold are composed of synthetic chemicals, which may have harmful side effects (Morais et al., 2021). Parabens, the most widely used preservatives in cosmetics, have been reported to mimic the effects of estrogen, increase the risk of breast cancer in women, and influence the development of malignant melanoma (Kerdudo et al., 2016). The California Department of Environment has found that chemicals in cosmetics and personal care products are associated with birth defects in male reproductive organs, reduced sperm count, and altered pregnancy outcomes in animal experiments (Barrett, 2005). Although the study did not show the same effect in humans, it was met with serious skepticism when it was published. Safety-conscious consumers are no longer looking for skin cosmetics that only provide fragrance and temporary adornment, but are willing to pay more for cosmetics containing natural ingredients that are more beneficial to the skin (Draelos, 2018). It is estimated that the global market value of natural cosmetics will reach USD 54.5 billion by 2027 (Thiyagarasaiyar et al., 2020). With the continuous increase in consumer demand and the expansion of the cosmetics market, it is necessary to develop a large number of active natural substances. Interestingly, the ocean attracts developers because of its unique environment (high pressure, high salt, and low temperature). Shu Uemura’s first addition of seawater to skin-care products has led researchers to explore the use of marine natural products in cosmetics (Martins et al., 2014).
Finding novel active natural compounds is the main target of developing new cosmetics. The oceans cover more than 70% of the Earth’s surface. They are the largest habitat on Earth and are home to 90% of all living organisms (Gomez et al., 2009; Dash et al., 2012). Therefore, marine micro-organisms are considered important potential sources of active natural products. Some bioactive compounds from marine micro-organisms have antitumor activity (Rehman et al., 2020), anti-inflammatory activity (Toledo et al., 2014), and antibacterial activity (Nalini et al., 2018). The natural products of marine microbes have received great attention in the cosmetics industry. However, few active compounds derived from marine micro-organisms have been used in the cosmetics industry. In this paper, we reviewed the application and possible mechanism of active substances derived from marine micro-organisms in sun protection, whitening, moisturizing, anti-aging, repair, etc. (Supplementary Table 1). In addition, some possible difficulties and solutions of natural products in the cosmetics industry derived from marine micro-organisms are discussed to provide a reference for the cosmetics industry.
Sunscreen
Part of the UV radiation emitted by the sun is absorbed by oxygen molecules in the stratosphere (UVC, 100–290 nm), part is absorbed by the ozone layer (UVB, 290–320 nm), and the remainder of UVA radiation (UVA, 320–400 nm) and a small part of UVB are transmitted to the ground (Battistin et al., 2020). Basking in the sun can help the conversion of 7-dehydrocholesterol into vitamin D in human skin, but long-term exposure to UV light causes mutagenic and non-specific damage to the epidermis. UV-induced reactive oxygen species (ROS) can alter genetic material and inhibit the production of extracellular matrix proteins. This can contribute to the loss of skin elasticity, skin photoaging, actinic keratosis, and skin cancer (Guillerme et al., 2017; Battistin et al., 2020; He et al., 2021).
Sunscreens are a sun protection products that help reduce the damage caused by UV rays, but some sunscreens lack light stability, irritate the skin, and cause allergic reactions (Greenspoon et al., 2013). In addition, these compounds can also affect marine life (Downs et al., 2015) and the marine environment (Sang and Leung, 2016; Chaves Lopes et al., 2020) when discharged into the ocean with sewage. Therefore, the cosmetics market is constantly looking for new environmentally friendly UV-resistant molecules to change this situation. Lower eukaryotes, such as marine microalgae, have evolved mechanisms to synthesize secondary metabolites unrelated to their growth and reproduction, but most of these metabolites can interact with UV light to coordinate cell functions (Kostyuk et al., 2018). This led to widespread concern in the cosmetics market. Many marine microbial sources of mycosporine-like amino acids (MAAs) can absorb UV rays ranging from 310 to 360 nm. They are colorless, water-soluble, and low molecular weight compounds composed of cyclohexanone or cyclohexanol imine chromophores with the nitrogen substituent of an amino acid or its imino alcohol, which inhabit fungi, algae, cyanobacteria, and other marine organisms. MAAs are also considered a potential source of environmentally friendly sunscreen ingredients with good anti-UV activity (Sinha et al., 2007; Sun et al., 2020; Nedeljka, 2021). People’s interest in MAAs has increased in recent years. MAAs are distributed in the cytoplasm of fungal cells and can also be released into extracellular colony mucus, enhancing UV protection for themselves and possibly for other community members (Llewellyn and Airs, 2010). Kogej et al. (2006) found two different UV-absorbable mycosporine-glutaminol-glucosides (Figure 1) and three unidentified UV-absorbable compounds in fungi in glaciers and high-salt waters, which they considered to be MAAs. These are natural sources of MAAs, which will have a good market value if they can be further applied to cosmetics. Some sunscreens with MAAs as the main active ingredient have been marketed globally, such as Helionori® and Helioguard365® (Chrapusta et al., 2017). However, some microbes are difficult to grow in laboratory conditions because of environmental constraints, which impede access to these metabolites. Fortunately, the current sequencing technology is very advanced, and people can obtain the relevant gene cluster information of the metabolites of MAAs produced by marine micro-organisms based on metagenomic sequencing technology, obtain the genes through the chemical synthesis in vitro, and then transform them into Escherichia coli for production using genetic engineering. Miyamoto et al. (2014) found gene clusters for MAAs synthesis in Actinosynnema mirum DSM 43827 and Pseudonocardia P1. These genes were not expressed or rarely expressed, in laboratory culture conditions. However, silenced MAAs gene clusters were expressed in the Streptomyces sp. avermitilis SUKA22 strain after genetic engineering. This result encourages the cosmetics industry to obtain these natural active products and helps accelerate the innovation and development of the cosmetics industry.
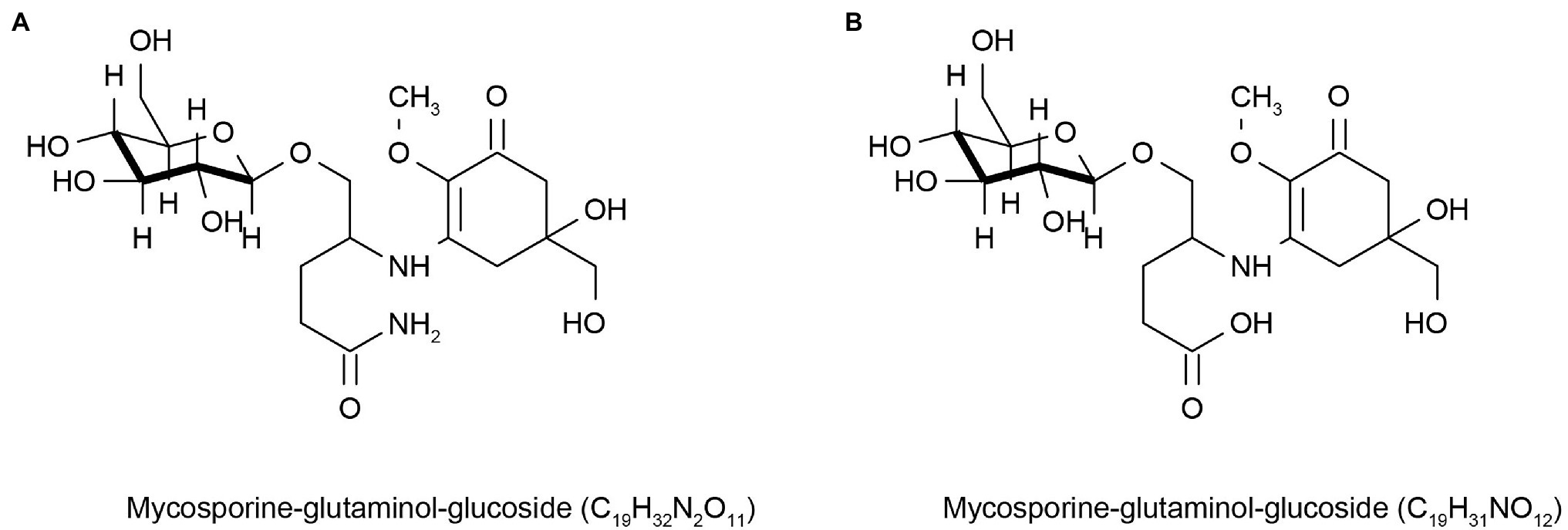
Figure 1. Reprinted with the kind permission of Environmental Chemistry publications. Chemical structures of Mycosporine-glutaminol-glucoside (A,B) isolated from fungi hypersaline waters and polar glacial ice. Adapted from Kogej et al. (2006).
Moreover, carotenoids, such as astaxanthin, zeaxanthin, lycopene, and β-carotene are the most common pigments in nature and have biological functions such as light capture and photoprotection (Vílchez et al., 2011). Microalgae are important sources of carotenoids, fatty acids, and amino acids (Ambati et al., 2018). Haematococcus lacustris (formerly Haematococcus pluvialis; Chlorophyta) can accumulate astaxanthin under high salinity, high temperatures, and light stress (Naguib, 2000). This microalga has the highest astaxanthin yield and has great application potential (Kuang et al., 2019). Astaxanthin has a higher antioxidant activity than lutein, lycopene, β-carotene, and other carotenoids (Naguib, 2000). The antioxidant activity of astaxanthin is 10 times that of zeaxanthin, lutein, and β-carotene (Matsuno and Miki, 1990). This is due to the carbonyl functional groups in the ionone ring of astaxanthin (Liu and Osawa, 2007). Due to its strong antioxidant activity, astaxanthin has been added as its active ingredient in some cosmetics brands around the world, such as Geisha Astaxanthin Refining Serum, Home Facial Pro Astaxanthin Essence, and Kose Becky Firming Essence (Kuang et al., 2019). However, astaxanthin is unstable and prone to oxidation and discoloration, so better technologies are needed to develop and expand astaxanthin applications. Zeaxanthin is an important factor in the photoprotection mechanism of Nannochloropsis oculata (Shen et al., 2011). Microalgal-derived fucoxanthin protects against sunburn (Matsui et al., 2016). Lycopene neutralizes oxygen free radicals and is a powerful natural antioxidant with the potential to be used as a sunscreen ingredient (Mourelle et al., 2017). Hashtroudi and colleagues isolated strains that produce carotenoids from Iranian terrestrial and aquatic ecosystems. Anabaena (Cyanobacteria) was the first time a strain with the highest natural lycopene production was identified (Hashtroudi et al., 2013). In addition to the microalgae, scytonemin in the outer sheath of cyanobacteria mainly absorbs UVA radiation, while MAAs are keys to protecting against UVB radiation. At low MAA-content conditions, the carotenoids in cells are rapidly synthesized and bind to the outer membrane to act as photoprotection (Ehling-Schulz et al., 1997). Scytonemin is a unique natural product that consists of indole and phenolic subunit dimers. It can be used in sunscreens due to its anti-UV and antioxidant effects (Mourelle et al., 2017; Stoyneva-Gärtner et al., 2020). Additionally, cyanobacteria have high carotenoid potential (Mezzomo and Ferreira, 2016). All in all, carotenoids produced by cyanobacteria and microalgae have great potential for use in cosmetics.
Marine heterotrophic bacteria and fungi are also potentially important sources of carotenoids. Phaffia, Rhodozyma, and Xanthophyllomyces produce large amounts of astaxanthin (Ambati et al., 2014). Two rare, monocyclic carotenoids, (3R, 2’S)-myxol (Figure 2A) and (3R)-saproxanthin (Figure 2B), were found in a new bacterium species from the family Flavobacteriaceae isolated in Okinawa, Japan. Compared to zeaxanthin and β-carotene, saproxanthin and myxol showed stronger antioxidant activity (Shindo et al., 2007). Zhang et al. (2008) isolated a new benzodiazepine alkaloid, cyclosporine I (Figure 3A), from the metabolites of the marine fungus Exophiala. This compound had a structure similar to cyclosporine C and G (Figures 3B,C) from the same source and had good anti-UVA activity. Compared with microalgae, the amount of carotenoid produced by marine fungi and bacteria is small, but fungi and bacteria are easy to culture and rapidly reproduce. Thus, they can be modified using genome sequencing and genetic engineering to improve carotenoid production (Galasso et al., 2017). These findings suggest that there may be other exploitable substances in marine micro-organisms with anti-UV activity.
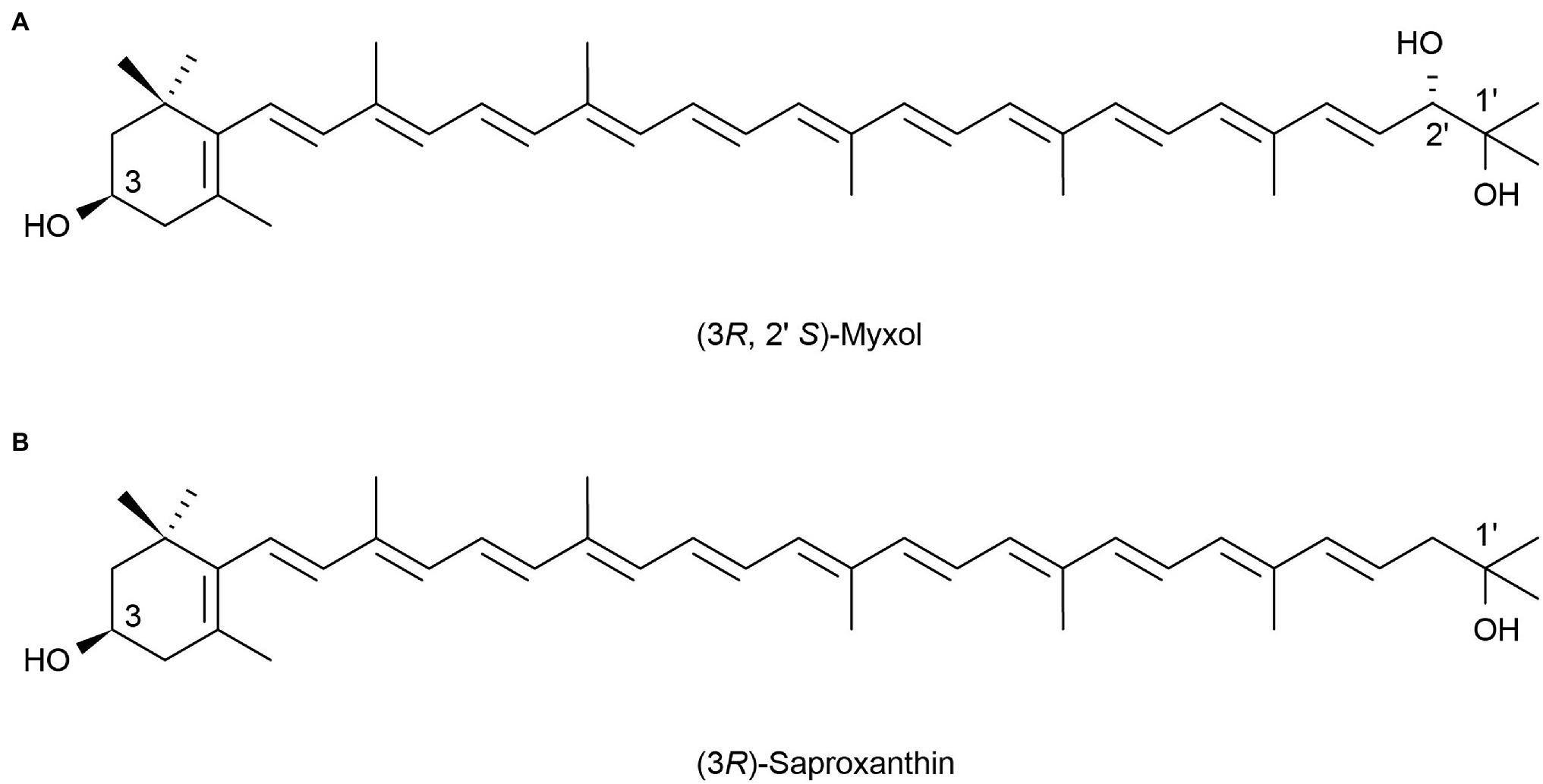
Figure 2. Reprinted with the kind permission of Applied Microbiology and Biotechnology publications. Chemical structures of two rare monocyclic carotenoids (3R, 2’S)-Myxol (A) and (3R)-Saproxanthin (B). Adapted from Shindo et al. (2007).
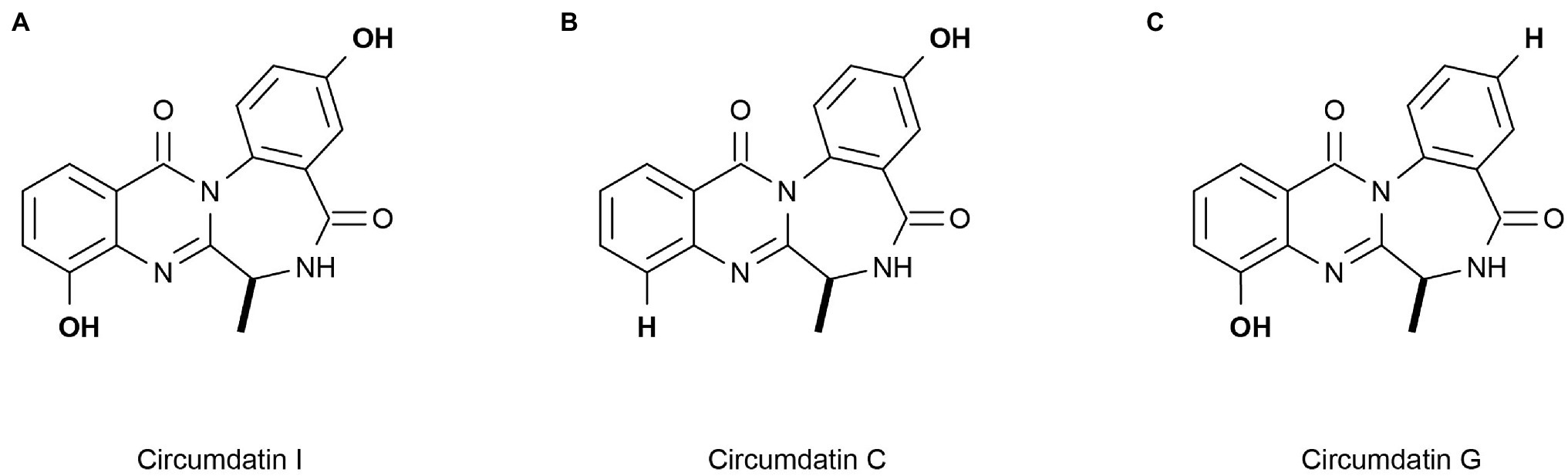
Figure 3. Reprinted with the kind permission of The Journal of Antibiotics publications. Chemical structures of Circumdatin I (A), C (B), and G (C). Adapted from Zhang et al. (2008).
Natural ingredients can provide protection through the direct absorption of UV rays and protect skin from UV damage through antioxidant action (He et al., 2021). Many antioxidants derived from marine organisms have been studied for their UV protection. Two compounds, a golmaenone of the diketopiperazine alkaloid and neoechinulin A of related alkaloids, were isolated from the marine fungus Aspergillus sp. They showed the activity of scavenging free radicals against 1, 1-diphenyl 2-picrylhydrazyl (DPPH) and showed better anti-UV activity than oxybenzone in sunscreen (Li et al., 2004). Most lipophilic vitamins also have strong antioxidant activity (Lourenço-Lopes et al., 2020). Tocopherol (vitamin E) is only synthesized by photosynthetic organisms and has the same antioxidant activity as carotenoids. Tocopherol was studied in 130 cultured microalgae and cyanobacteria [including 118 microalgal strains from four phylogenetic lineages (Chlorophyta, Streptophyta, Heterokontophyta, and Rhodophyta) and 12 cyanobacterial strains] by Mudimu et al. (2017). They found that α-tocopherol was most abundant, while β-tocopherol and γ-tocopherol were present in some algae but in lower amounts. Among them, the α-tocopherol production of Chlorophyta was higher than that of Rhodophyta, which could be a natural source of α-tocopherol (Mudimu et al., 2017). Sivakumar et al. (2014) discovered a new strain (Stichococcus bacillaris strain siva2011) that produces natural RRR-α-tocopherol and could be commercialized. Leya et al. (2009) found two strains of Raphidonema from snow and permafrost substrates, which were good α-tocopherol producers (Leya et al., 2009). Shanuja et al. (2018) extracted pigment from a marine Aspergillus nidulans and found that it had structural similarity to 5, 6-dihydroxyindole-2-carboxylic acid (DHICA), the precursor of melanin. It can reduce ROS generation after UV irradiation and has the potential for use in sunscreen formulations.
Moreover, some other natural molecules have anti-UV activity, such as phenolic substances. Phenolic compounds are produced during the production of ROS and are considered stress-induced compounds with protective mechanisms against UV radiation and anti-reactive oxygen (Bedoux et al., 2014). Phenols also play an important role in the antioxidant mechanism of cells and research on phenols in marine algae has been conducted (Stoyneva-Gärtner et al., 2020). Haoujar and colleagues tested four microalgae: Phaeodactylum tricornutum (Bacillariophyta), Microchloropsis gaditana (formerly Nannochloropsis gaditana; Ochrophyta, Eustigmatophyceae), Nannochloris sp., and Tetraselmis (Chlorophyta), and found that the antioxidant capacity of microalgae was positively correlated with the content of phenolic substances. Phaeodactylum tricornutum had the highest phenolic content and the best antioxidant activity among the four microalgae. These results indicate that P. tricornutum is a potential source for developing novel antioxidant substances (Haoujar et al., 2019). Duval et al. (1999) isolated Chlorella sp. (Chlorophyta) from a snow microalgal community at King George Island and showed that the total content of phenols in Chlorella increased under UVA and UVC irradiation. This confirms that phenols contained in microalgae act as antioxidants when stimulated by UV light (Haoujar et al., 2019). Although algae are rich in phenolic compounds, it has been found that the content of phenolic compounds in algae extracts also depends on the algae collection site and extraction method (Mateos et al., 2020). This requires conducting topographic analysis and selecting extraction methods before development to maximize the use of phenolic substances in algae.
Whitening Effect
Skin, consisting of the epidermis and dermis, plays a protective role in the human body. The epidermis is the outermost layer of the skin and is mainly composed of melanocytes and keratinocytes. Melanocytes provide melanin to keratinocytes through the dendritic transfer of melanin bodies so that keratinocytes can form melanin caps and reduce UV-induced DNA damage to the epidermis (Costin and Hearing, 2007). One’s skin color is mainly determined by the amount, type, and distribution of melanin in the skin (Dessinioti et al., 2009). There are two types of melanin, eumelanin (dark brown insoluble polymers) and non-melanin (reddish sulfur-containing polymers). Tyrosinase is an important enzyme in melanin synthesis (Alves et al., 2020). Under its catalysis, tyrosine can be converted into dopaquinone, which is finally converted into melanin through a series of complex processes (Dessinioti et al., 2009; Agrawal et al., 2018). Marine organisms are important sources of compounds that can inhibit tyrosinase. The kojic acid produced by the fungi Altenaria sp. isolated from the surface of marine Ulva lactuca (Chlorophyta) has been shown to have tyrosinase activity (Li et al., 2003). Li et al. (2005) isolated Myrothecium sp. (Fungi) strain MFA58 from marine green algae. Two cyclopentenone compounds, designated as myrothenones A and B, were found in this strain. Only myrothenone A showed tyrosinase inhibitor activity and its activity was greater than that of kojic acid, which is currently used in sunscreen products. Tsuchiya et al. (2008) found that homothallin II produced by marine Trichoderma viride (Fungi) may inhibit tyrosinase activity by competing for the copper ion active site. Wu et al. (2013) isolated two new sesquiterpene compounds, 1β, 5α, 6α, 14-tetraacetoxy-9α-benzoyloxy-7βH-eudesman-2β, 11-diol and 4α, 5α-diacetoxy-9α-benzoyloxy-7βH-eudesman-1β, 2β, 11, 14-tetraol, from the marine fungus Pestalotiopsis sp. strain Z233, which also showed tyrosinase inhibitory activity. The Micromonospora sp. strain SH-89, which is symbiotic with sponges, also showed significant inhibitory activity against tyrosinase, as described by Said Hassane et al. (2020). Some substances produced by marine organisms that inhibit tyrosinase activity have been used commercially, but other substances (hydroquinone) have been banned in all European countries as they threaten human health (Burger et al., 2016). Therefore, there is a constant search for new, active whitening molecules. Marine micro-organisms such as microalgae and bacteria can also produce active substances with whitening functions. Astaxanthin, produced by marine yeast, has been found to protect skin from age spots (Corinaldesi et al., 2017). Astaxanthin and zeaxanthin produced by microalgae from Nannochloropsis oculata (Ochrophyta, Eustigmatophyceae) or H. lacustris (Chlorophyta) have antityrosinase activity (Balasubramaniam et al., 2021). Some whitening active substances derived from marine micro-organisms have also been discovered. A N-acyl dehydrotyrosine derivative derived from Thalassotalea sp. (Bacteria) strain PP2-459 isolated from crustaceans can act as a tyrosinase inhibitor superior to commercial kojic acid and arbutin (Deering et al., 2016). Cell extracts with the activity of cell-free tyrosinase were produced in a Pseudomonas sp. isolated from the waters off Ganghwa Island, South Korea. An extract of dichloromethane from the secondary metabolite of these new bacteria can reduce the melanin deposition in cultured skin and zebrafish. This extract has a whitening effect and could be used as a novel whitening ingredient (Kang et al., 2011). Kim et al. (2017) isolated the Bacillus strain SCO 147 from Gwangyang Bay, South Korea. The (−)-4-hydroxysattabacin metabolite from its crude extract had an anti-melanogenic effect in a human epidermal model and they identified it as a new natural melanin reducing agent (Kim et al., 2017). Khan et al. (2021) found that cydromicin (1), a secondary metabolite produced by the Tolypocladium sp. (Fungi) strain SCSIO 40433 isolated from arctic glacial sediments, also showed tyrosinase inhibitor activity. It follows that polar fungi are also potential sources of natural active substances. Pseudoalteromone A (1), a ubiquinone derivative produced by APmarine002 and RoA-050 strains of Pseudoalteromonas sp. (Bacteria) of marine origin, can inhibit tyrosinase activity by inhibiting melanin-producing gene expression. The whitening effect of the substance was evaluated by using a 3D pigment epidermis model, and it was confirmed that the substance had whitening and brightening effect, which provided a new source of active molecules for whitening products in cosmetics (Lim et al., 2021).
Moisturizing Effect
The main cell type of the dermis is the fibroblast, which is embedded in collagen, elastic fibers, and an extracellular matrix composed of glycoprotein, hyaluronic acid, glycosaminoglycan, and other mixtures such as water and salts to form a gel. Glycosaminoglycan (GAG) is a proteoglycan that stores most of the water in the skin (Costin and Hearing, 2007; Bedoux et al., 2014). Water is essential for the skin to function properly. The influence of external factors can promote skin aging and skin aging destroys its barrier function. This makes it more fragile, and it gradually loses its natural elasticity and moisturizing function (Pimentel et al., 2017). Keratinocytes in the skin’s epidermis contain natural moisturizing factors (NMF), which are natural hygroscopic compounds, such as urea, polysaccharides, amino acids, and minerals (Brunt and Burgess, 2018; Pimentel et al., 2017). The lipids between the cells of the skin’s cuticle are also moisturizing, lining up to form a barrier to prevent water loss (Verdier-Sévrain and Bonté, 2007).
Marine organisms produce moisturizing molecules such as fatty acids and polysaccharides, which are commonly used in cosmetics. Algae-derived omega-6 polyunsaturated fatty acids, especially C-18 linoleic acid and gamma-linolenic acid, such as those found in marine microalgae, can be added to the oil in water emulsions to moisturize skin (Guillerme et al., 2017). A lack of unsaturated fatty acids has been reported to cause dermatitis and skin dehydration (Ziboh et al., 2000; Kim et al., 2008). Marine micro-organisms are important producers of unsaturated fatty acids. A strain of Vibrio cyclitrophicus isolated from the ocean has been reported to produce eicosapentaenoic acid (EPA; Abd Elrazak et al., 2013). According to Kim et al. (2008), Nanochloropsis sp. can produce EPA. Cladophora glomerata, a filamentous green alga of marine origin, was described as containing saturated fatty acids (C16:0) and unsaturated fatty acids (C16:1) (N-7) and (C18:1) (N-3), which can be used as an active moisturizing agent to prevent skin moisture loss (Couteau and Coiffard, 2020). The thickness of the cell wall of algae and the mucus layer formed by polysaccharides contained in the cell wall explain how they keep the cells hydrated (Stoyneva-Gärtner et al., 2020). Polysaccharides and oligosaccharides can be hydrogen-bonded to keratin to retain moisture (Bedoux et al., 2014). Wang et al. (2013) tested the moisturizing ability of polysaccharides extracted from five kinds of algae and found that polysaccharide DL extracted from Phaeophyceae had a good moisturizing effect, and its moisturizing performance was better than that of hyaluronic acid (HA). This indicated that seaweed polysaccharides could be used as an additive for moisturizing cosmetics. Nostoc commune moisturizing serum can be used to moisturize, whiten, and be non-greasy for the skin (Stoyneva-Gärtner et al., 2020).
In addition to the moisturizing function of seaweed polysaccharides, the extracellular polysaccharide (EPS) of marine bacteria also has moisturizing potential. The EPS of the Polaribacter sp. SM1127, isolated from Arctic kelp, had a good moisturizing ability that was superior to HA in cosmetics. This EPS has a significant protective effect on human dermal fibroblasts at low temperatures and can be used as a moisturizing ingredient in cosmetics (Sun et al., 2015). Phyllobacterium sp. 921F can produce a large amount of EPSs, and its water absorption and retention ability are better than collagen, chitosan, and glycerol (Li et al., 2015). There are few examples of bacterial exopolysaccharides used in cosmetics, so discovering these exopolysaccharides provides new moisturizing molecules for cosmetic formulations (Brunt and Burgess, 2018). A new strain of Pseudoalteromonas sp. has been isolated from polar regions. Intracellular extracts of the strains of RefirMAR® by BIOALVO have been applied to RefirMAR®, a cosmetic that is a good hydration agent (Martins et al., 2014). EPS HYD657, extracted via the fermentation of Alteromonas macleodii subsp. fijiensis biovar deepsane, is used in Abyssine® cosmetics (Martins et al., 2014). Chlorella extracts have been described to promote collagen synthesis and reduce wrinkles and appear to have value for skin-care products (Wang et al., 2015).
Antiaging Effect
The aging process of the skin is affected by both internal factors and the external environment (Ganceviciene et al., 2012). Internal factor changes are mainly related to age, while external environmental stressors include high temperature, smoke, pollutants, and UV radiation (Zhang and Duan, 2018). Skin aging is mainly due to a reduction in collagen, elastic fibers, and hyaluronic acid and is manifested by wrinkles, dryness, loss of elasticity, sagging, and rough appearance (Brunt and Burgess, 2018). In the aging process of the skin, cells suffer from oxidative stress and lose the ability to regulate ROS (Bedoux et al., 2014). Fortunately, many substances from marine organisms effectively combat oxidative damage to cells and prevent skin aging. Fucoxanthin extracted from brown seaweed has been shown to protect keratinocytes from oxidative damage (Zheng et al., 2013). A glycosaminoglycan extracellular polysaccharide (HE 800) was discovered from the deep-sea bacteria Vibrio diabolicus, which can facilitate skin regeneration (Courtois et al., 2014). Alteromonas fermentation extract is an EPS produced by extremophiles living in deep-sea hydrothermal vents. The fermentation extracts of Alteromonas can reduce MDA (the end product of lipid peroxidation), chelate cadmium, and lead and form a protective film on the body surface (Borel et al., 2017). It was combined with carnosine, a sodium hyaluronate cross-polymer, and a tripeptide to create a new beauty cream, and the product was shown to improve facial contours and skin quality (Garre et al., 2017). This formulation has good tolerance and is an excellent antiaging product.
Marine micro-organisms contain many high-quality functional proteins and bioactive natural peptides with diverse molecular structures (Xia et al., 2021). For example, marine peptides derived from proteolytic products of Navicula salinicola (formerly Navicula incerta; Bacillariophyta) microalgae inhibit the activity of gamma-glutamate transferase (GGT), thereby reducing oxidative damage and delaying cell senescence (Kang et al., 2012). Similarly, peptides from Chlorella can reduce the expression of matrix metalloproteinase-1 (MMP-1) in human skin fibroblasts, thereby reducing the breakdown of collagen and delaying aging (Chen et al., 2011). We can use biotechnology to find more of these products from the ocean. A typical example of this is the research by Said Hassane et al. (2020), who studied microbial diversity from the marine sponge Scopalina hapalia using metagenomics and found that the microbial secondary metabolites had biological activity against seven targets associated with cell senescence. These were elastase, tyrosinase, catalase, sirtuin 1, cyclin-dependent kinase 7 (CDK7), fyn kinase, and proteasome. These data showed the potential of marine microbes to produce antiaging compounds.
Repair Function
The skin is the first barrier of the immune system, and its most important function is to separate the internal environment from the external environment. The skin barrier inhibits water loss and prevents harmful substances from entering (Proksch et al., 2009). The skin barrier mainly includes the cuticle, which contains protein-rich cells, keratinocytes, and lipid-rich cells. These lipids include cholesterol, free fatty acids, and ceramides (sphingolipids; Elias and Friend, 1975). When the skin barrier is damaged, the skin becomes dry and dehydrated. As a result, it is more easily invaded by external germs and irritants, which trigger inflammatory reactions and other symptoms (Proksch et al., 2009). Thus, skin repair is an important part of beauty care (Figure 4).
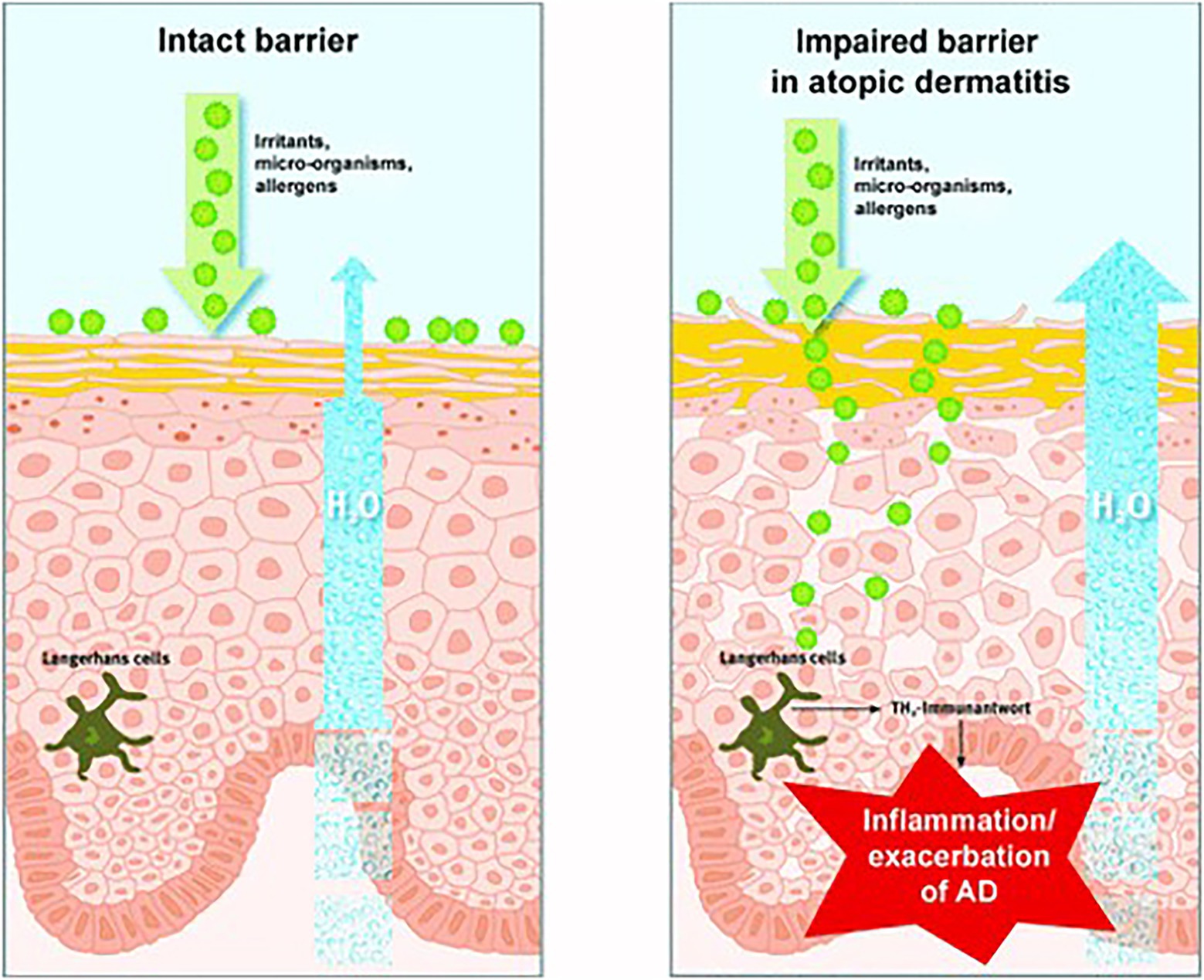
Figure 4. Reprinted with the kind permission of Journal der Deutschen Dermatologischen Gesellschaft publications (Proksch et al., 2009). The skin barrier is normal (left) and impaired (right). When the skin barrier is compromised, water loss increases, and environmental toxins can penetrate the skin, triggering irritation, allergic reactions, inflammation, or exacerbation of specific eczema.
To deal with these problems, first, we can promote wound repair, that is, the growth or protection of skin fibrocytes. Zhang (2019) found that the EPS of marine bacteria Polaribacter sp. SM1127 can increase fibrocytes, promote the healing of skin wounds in mice, and reduce skin injury caused by low temperatures. In addition, the EPS of Polaribacter sp. SM1127 can also reduce lactate dehydrogenase (LDH) and ROS, increase glutathione (GSH) content, reduce superoxide dismutase (SOD) enzyme activity, and maintain cell activity and integrity to resist UV radiation damage. Letsiou et al. (2020) isolated Aspergillus chevalieri TC2-S6 from sponges (Axinella) and cultured it in potato dextrose broth (PDB) to obtain a component named ACCB from the culture medium. ACCB is mainly tetrahydroauroglaucin and flavoglaucin, and is capable of protecting human fibroblasts under oxidative stress conditions.
Second, we can protect the keratinocytes from damage by moisturizing. Long et al. (2010) cloned a new endo-type β-agarase gene agaA from the marine bacteria Agarivorans sp. LQ48 and expressed it in E. coli. This agaA enzyme (AgaA) had strong acid and alkali resistance. AgaA can produce DP6 (neoagarohexaose) and DP4 (neoagarotetraose) by hydrolysis from agarose, and these can be used as skin moisturizers. Collagen is a structural skin protein commonly used as the active ingredient in moisturizers (Brunt and Burgess, 2018). Swatschek et al. (2002) extracted collagen from kidney-shaped cartilage sponges and compared the effects of sponge-derived collagen with existing collagen in human skin. Marine collagen increased skin lipids, although both had similar moisturizing effects. We believe that marine collagen has a superior repair function because the added lipids help the skin hold moisture better.
In addition, we can also use substances with antioxidant effects to protect and repair skin cells, strengthen the vitality and connectivity of skin cells, enhance their immune capacity, and reduce free radical damage to skin cells. Squalene is an antioxidant, and hydrogenated squalane is used in cosmetics (Stoyneva-Gärtner et al., 2020). Squalene can also be added to moisturizers as an emollient that is quickly absorbed by the skin (Stoyneva-Gärtner et al., 2020). The HS-399 strain of Aurantiochytrium acetophilum sp. (a thraustochytrid) was isolated from a mangrove swamp in Biscayne Bay, Florida, United States, producing squalene and lipids (Ganuza et al., 2019). Moreover, bioactive indole derivatives were isolated from the marine sponge Rhopaloeides odorabile and its derived fungus Hyrtios sp., and DPPH (a stable free radical) was used to test its antioxidant capacity (Longeon et al., 2011); its antioxidant capacity was similar to that of Trolox. Compound 9 in the indole derivatives had no cytotoxicity and was suitable for skin repair. Raveendran et al. (2013) extracted mauran (MR), a highly polyanionic sulfated EPS from Halomonas maura, and showed that MR induces antioxidant properties by preventing the production of LPO (lipid peroxidation) and free radicals. It does not affect the production and function of the body’s own antioxidants, such as GSH (which normally functions as an antioxidant in cells), GR (which is essential for GSH production), and GPx (glutathione peroxidase). Li et al. (2011) isolated an aromatic polyketone compound from the sponge-derived fungus Aspergillus versicolor. Through the control experiment using standard antioxidants, including butylated hydroxyanisole (BHA), butylated hydroxytoluene (BHT), tertiarybutylhydroquinone (TBHQ), and ascorbic acid (VC), its excellent antioxidant performance was proven. On the other hand, lipase can also be used to produce whitening antioxidant products. For example, Sang (2017) screened microbial strains producing ferulic acid esterase (FAES1) from seawater. Ferulic acid is a phenolic acid with a strong antioxidant capacity and scavenging effect on free radicals. In addition, skin fibroblasts can be protected by enhancing lipid cell differentiation and promoting adiponectin synthesis, such as docosahexaenoic acid (DHA). DHA is usually obtained from marine fish oil (Lee et al., 2020), but due to environmental pollution, fish oil-derived DHA has become unreliable, and marine microbial DHA, produced by Thraustochytrium sp. 26185, can be used as a substitute. Meesapyodsuk and Qiu (2016) provided a partial solution to the biosynthesis mechanism of DHA. DHA has moisturizing and antiaging functions as well as tanning under certain conditions (Martini, 2017).
The above ideas provide a method for skin repair, but how to effectively combine these functional molecules to produce new skin-repair products still needs to be supported by experiments.
Skin Lipid Conditioning
The average rate of sebum production in normal human skin is about 1 mg/10 cm2 every 3 h (Hong et al., 2020). If the sebum secretion of human skin exceeds 1.5 mg/10 cm2 every 3 h, caused by high secretion of the sebaceous glands, then the skin becomes oily. However, a sebum secretion rate as low as 0.5 mg/10 cm2 per 3 h in human skin will result in dry skin (Endly and Miller, 2017). Excessive oil secretion may affect the microbial environment of the face, leading to acne (Youn, 2010). In addition, this can lead to enlarged facial pores and a greasy facial appearance (Hong et al., 2020). Therefore, it is particularly important to clean the face. Lipase can catalyze the hydrolysis of insoluble triglycerides into glycerol diesters and glycerol monomers (Yvergnaux, 2017) to produce cleansing facial oil. Compared with chemical reagents, the enzymes are milder and less irritating, and many of these commercial products now have enzymes added (Liu et al., 2016). Lipase comes from a variety of sources, including animals, plants, and microorganisms. Significantly, microorganisms have many advantages, such as easy cultivation, short generation cycles, and resistance to external environmental conditions (Hasan et al., 2006). Marine micro-organisms can generate many lipases that can withstand various conditions due to their unique environment, better meeting the needs of commercial production and practice requirements, so they are favored by people. For example, Su et al. (2015) obtained alkaline-resistant lipase (LipA) from a metagenomic library of the sponge Ircinia sp. Yuan et al. (2016) expressed a MAS1 gene derived from the marine Streptomyces strain W007 in Pichia pastoris and proved that MAS1 is a heat-resistant and alkali-resistant lipase. Nishihara et al. (2008) isolated a bacterium HFKI0020 from the intestinal contents of marine fish that could produce phospholipase A1 at low temperatures. The skin barrier of patients with dry skin tends to be itchy, red in color, scaly, and easily injured (Tončić et al., 2018). Lipase can be used to produce simple lipids for dry skin tonings, such as myristyl myristate (Figure 5A) and cetyl-ricinoleate (Figure 5B; Metzger and Bornscheuer, 2006). In addition, collagen is not absorbed by the skin, but binds to water through hydration and attaches to the skin, thereby maintaining moisture (Swatschek et al., 2002). Swatschek et al. (2002) collected the Chondrosia reniformis Nardo sponge from the Aegean Sea and extracted collagen, proving that this sea-derived collagen has a good moisturizing ability and can increase skin lipids. These lipids can treat dry skin instead of traditional collagen products.
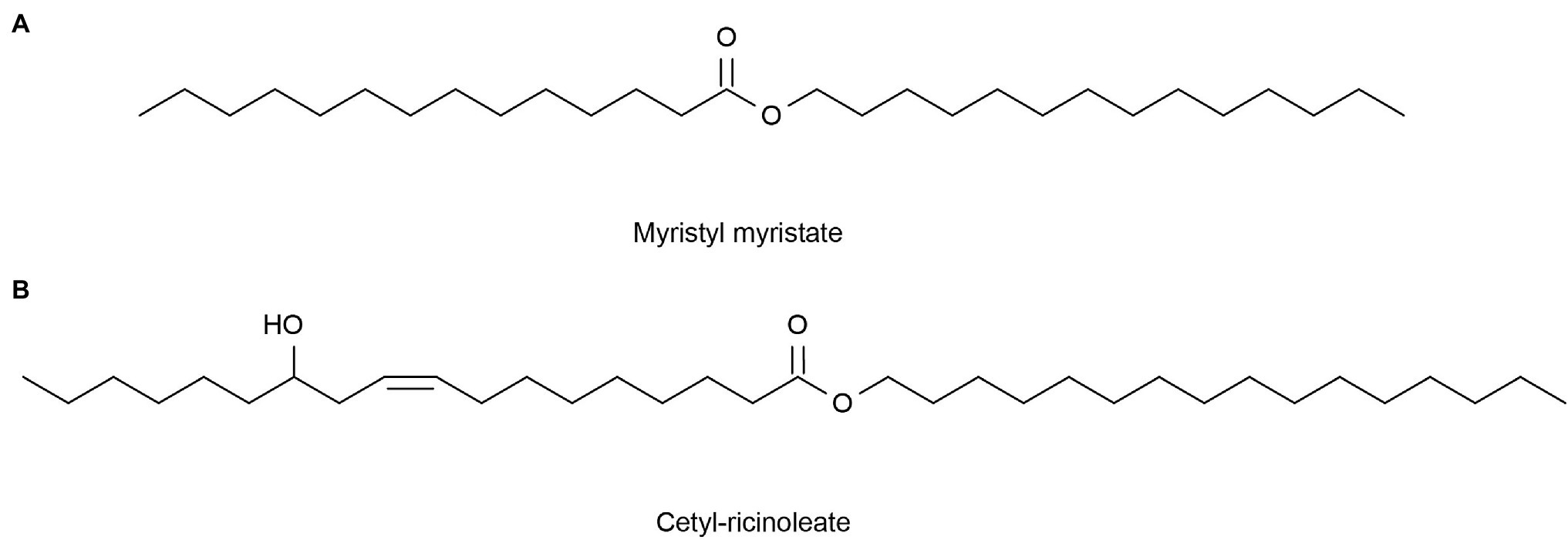
Figure 5. Reprinted with the kind permission of Applied Microbiology and Biotechnology publications (Metzger and Bornscheuer, 2006). Chemical structures of Myristyl myristate (A) and Cetyl-ricinoleate (B) are produced by lipase-catalyzed esterification.
Aromatic Substances
Fragrances are aromatic substances that can be perceived by smell. Olfactory cells on the mucous membrane inside the nasal cavity can accept the stimulation of odor molecules and produce olfactory stimuli. The smell of perfume passes the nasal cavity and stimulates the olfactory cell, the olfactory center that transmits the fragrance to the cerebrum, thereby forming olfactory sensations and bringing joyful experiences (Silva Teixeira et al., 2015). Fragrances are generally short-chain fatty acids or alcohol esters (Macedo et al., 2003), that are pleasing to people and are often used in cosmetic products. On the one hand, the natural volatile aromas of some marine microbes could be used as a new source of perfume; on the other hand, known perfume ingredients can be produced through biological transformation. These methods are greener and more environmentally friendly than those used in the traditional chemical industry. Most compounds with volatile fragrances belong to terpenes (Riad et al., 2021). Synechococcus PCC 6911 can reduce Geranial (1) into Geraniol (2), and Synechococcus PCC 6716 transforms (−)-Menthone ((−)-4) into (−)-Menthol ((−)-5), which are important components of perfumes’ essential oils (Jϋttner and Hans, 1986). These needed spices can also be produced by the catalysis of natural enzymes, such as acetic acid and cinnamic acid. Wang (2016) screened lipase L-1, which can synthesize cinnamic acid acetate with the highest conversion rate from the genome of the deep-sea micro-organism Streptomyces sp. SCSIO 13580. Recombinant enzymes designed for specific substrates are more efficient than traditional tools for biotransformations, such as micro-organisms. Fu et al. (2012) achieved recombinant expression of ene-reductase from Synechococcus sp. PCC 7942 and obtained a new ene-reductase that can efficiently convert (R)-(−)-carvone to (2R, 5R)-dihydrocarvone. Additional aromas from marine microbes are likely to be discovered in the future (Figure 6).
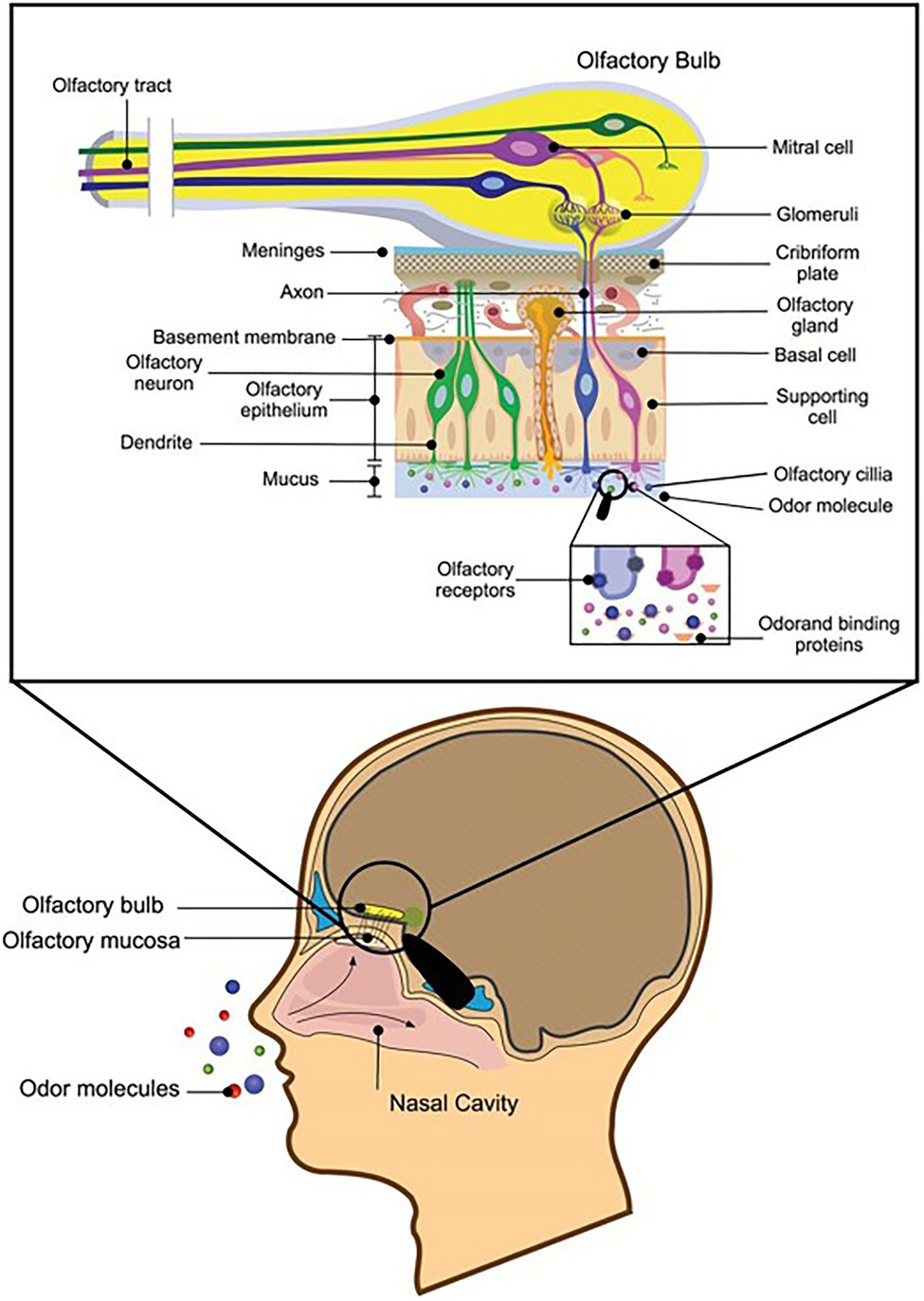
Figure 6. Reprinted with the kind permission of Chemical Senses publications (Silva Teixeira et al., 2015). Schematic representation of a sagittal section through the human head with a section of the olfactory nervous system depicted in greater detail. Air transmits odor molecules to olfactory mucosa, combines with odor transporter proteins to form complexes, and transports them to olfactory receptors on olfactory cilia. After a series of signal transductions, they are transmitted to the olfactory tract to produce olfactory sensations.
Pigment Substances
Beautiful colors provide sensory enjoyment and are found in nail polish, lipstick, and other cosmetics. Industrial pigments are usually synthesized from benzene, toluene, and other chemical reagents, so they can be toxic and unacceptable to many people (Kalra et al., 2020). Natural pigments are widely used in products due to their safety and stability. They are represented by anthocyanins, carotenoids, and chlorophyll (Kalra et al., 2020). Marine microbes are considered sources of inexpensive, novel, stable, and safe biological pigments, so many relevant studies have been reported. Du et al. (2008) obtained two pigments from Aspergillus glaucus isolated from marine sediments around mangrove roots in Fujian Province, China. These were named yellow pigment (+) variecolorquinones A and red pigment aspergiolide B. Dhale and Vijay-Raj (2009) isolated Penicillium sp. NIOM-02 from marine sediments in Miramar (India). The removal of the DPPH radical and the production of red dye were achieved simultaneously. When it was cultured on malt extract agar (MEA) plates, the red pigment secreted by the fungus occurred around its colony. This showed that the red dye has good water solubility. Penicillium bilaii (MST-MF667) was isolated from the Huon Estuary, Tasmania, and subjected to chemical analysis. Analysis revealed two yellow pigments including (−)-2, 3-dihydrocitromycetin and (−)-2, 3-dihydrocitromycin (Capon et al., 2007). Ganesh Kumar et al. (2013) isolated the hMGM-7 [MTCC 11712] strain from the surface of Hypnea musciformis (Rhodophyta) and found that it could produce acid-resistant melanin.
In addition, some invertebrates in the ocean, such as sponges and corals, are very colorful and these bright colors may be related to the photosynthetic pigments of symbiotic micro-organisms. Xu et al. (2008) isolated Aspergillus sp. from coral reefs in Manado, Indonesia, and obtained the red pigment bostrycin and two new yellow hexahydroanthrones, named tetrahydrobostrycin and 1-deoxytetrahydrobostrycin. Yellow compounds were isolated from Eurotium cristatum, a fungus from a sponge Mycale sp., which contains 2-(2′, 3-epoxy-1′, 3′-heptadienyl)-6-hydroxy-5-(3-methyl-2-butenyl) benzaldehyde and 1, 8-dihydroxy-6-methoxy-3-methyl-9, 10-anthracenedione (physcion). Phycocyanin produced by thermophilic cyanobacteria can be used to make eye shadows (Bermejo et al., 2003). Phycocyanin from Spirulina has been used as a colorant in eye shadow by Ink Chemicals in Japan (Ryu et al., 2015; Pangestuti et al., 2020; Yarkent et al., 2020). Pink and purple pigments in cosmetics can also be formulated from natural pigments extracted from red microalgae (Arad and Yaron, 1992). Biotechnically, obtained R-phycoerythrin has been used in colored creams and cosmetics as a natural dye instead (Bedoux et al., 2014). Antioxidant phycoerythrobilins from Arthrospira/Spirulina (Cyanobacteria) and Porphyridium (Rhodophyta) can be used in lipstick and eyeliner (Hamed, 2016). In summary, pigments derived from marine micro-organisms are now widely used in the cosmetics industry.
Others
The size and largely unexplored nature of the ocean often produce surprising discoveries. The complex and diverse ocean environment leads to metabolic pathways and adaptation mechanisms of marine micro-organisms that are completely different from those of terrestrial organisms. These pathways generate various natural products with unique structures, great diversity, and significant biological activities (Zhang et al., 2021). Jin et al. (2020) used AGAR biodegradation bacteria Flammeovirga pacifica WPAGA1 isolated from deep-sea locations to prepare algal oligosaccharide (AOS). AOS inhibited dihydrotrophil (DHT) to prolong the hair growth period of mice and might be used to prevent androgenetic alopecia (AGA). Peng et al. (2006) isolated the bacterial strain A4B-17 belonging to microspheres from sea squirts in the coastal waters of Palau. A4B-17 can generate alkyl esters of 4HBA (4-hydroxybenzoate), which can effectively prevent the growth of yeast, mold, and Gram-positive bacteria. This indicated it could possibly be used as a cosmetic preservative. The compounds extracted from microalgae can be used as the main components of cosmetics, but they can also be used as cosmetic stabilizers, dyes, thickeners, and other auxiliary materials depending on their different characteristics (Ryu et al., 2015; Wang et al., 2015). The rich pigments of microalgae can also be used in deodorants, antioxidants, creams, cleansers, and other products. They can also be used as deodorants because of their ability to mask odors. Vitamins produced by green unicellular Chlorella can promote hair growth by treating dandruff (Stoyneva-Gärtner et al., 2020). Seaweed polysaccharides are also widely used. Cationic polysaccharides derived from seaweed, such as chitosan, are very useful film-forming agents and are widely used in hair damage care and gel fixing products due to their unique advantage of binding tightly to the proteins (negative charges) found on human skin and hair (Kanlayavattanakul and Lourith, 2015). Chitosan can be used in dental products, such as toothpaste and chewing gum (Rinaudo, 2006). Non-ionic polysaccharides such as hydroxymethylcellulose can be used in nail products such as film formers and thickeners (Kanlayavattanakul and Lourith, 2015). Polysaccharides extracted from algae, such as D-glucose, D-mannose, D-galactose, and D-glucuronic acid, have been considered excipients in cosmetics due to their viscosity (Kim et al., 2008). Seaweed-derived tocopherol can be used in baby wipes, eyebrow growth serums, beard creams, and hair shampoo (Stoyneva-Gärtner et al., 2020). These versatile and strange natural products draw the scientist’s mind. The ocean’s treasures are almost endless, and they are waiting to be discovered.
Conclusion
This review covers the recent research and applications of natural products from marine micro-organisms in cosmetics, including marine bacteria, fungi, microalgae, and other micro-organisms. Starting from functional aspects, the mechanism and potential of natural compounds such as phenols, polysaccharides, vitamins, enzymes, proteins, and peptides in the field of cosmetics are summarized. As mentioned above, naturally active substances extracted from marine microbes have fewer skin side effects than chemically synthesized substances and can reduce skin damage while maintaining beauty. However, the exact mechanisms by which most of these natural compounds act on the skin have not been fully studied. We believe that it is necessary to further study these compounds to determine their mechanisms of action, preferably through clinical trials or in vitro cell tests to investigate the absorption and sensitization of these active substances on different skin types. This not only helps to improve the quality of cosmetics but also helps different groups of people to better choose cosmetics to protect the rights and interests of consumers and promote the benign development of the cosmetics market.
However, relatively little development of marine resources has been made to date. The unique environment of the ocean produces rich microbial resources and includes marine sediments, the symbiotic micro-organisms of invertebrates, and the intestinal micro-organisms of marine fish. The environment of these micro-organisms is very different from that of terrestrial organisms, endowing them with unique metabolic pathways and adaptation mechanisms. Adaptation to the marine environment also makes organisms unable to survive outside of this environment and difficult to culture. This is a great obstacle to developing and utilizing new molecules and enzymes, but metagenomic technology may help in this regard. Metagenomics can extract DNA from the whole environment to obtain the genome of unculturable micro-organisms in the whole environment and has been considered a good method to utilize the natural active substances of unculturable micro-organisms (Martins et al., 2014). Said Hassane et al. (2020) used metagenomic technology to discover metabolites with antiaging potential from microorganisms in marine sponges. Su et al. (2015) obtained LipA from a metagenomic library of the sponge Ircinia sp. Yuan et al. (2016) expressed the MAS1 gene derived from marine Streptomyces strain W007 in P. pastoris and demonstrated that MAS1 is a heat-resistant and alkali-resistant lipase. These results provide us with ideas for the exploitation of marine microbial resources. In addition, we know that most of the natural products of marine micro-organisms have multiple functions. For example, collagen not only has the functions of antioxidation and anti-UV radiation but also has a moisturizing function. The combination of these functions provides it with an antiaging function (Swatschek et al., 2002). Moreover, using marine species to produce more green and environmentally friendly products through biotransformation has become a new choice for cosmetic manufacturers. In developing these types of materials in cosmetics, we can combine a variety of non-interfering substances to produce greater functionality. These active substances produced by marine microbes will be brought to market safely and effectively.
Author Contributions
JD and BW are responsible for writing the whole passage. LC is responsible for giving suggestions on revision and review. All authors contributed to the article and approved the submitted version.
Funding
This work was supported by Fuzhou University Testing Fund of precious apparatus (Grant/Award Number: 2022T040), Natural Science Foundation of Fujian Province (Grant/Award Number: 2021Y0094, 2021Y0095, 2021J01607), Fujian Provincial Clinical Research Center for Cancer Radiotherapy and Immunotherapy (Grant/Award Number: 2020Y2012), Joint Funds for the innovation of science and Technology, Fujian province (Grant/Award Number: 2018Y9105), Sponsored by Fuzhou health technology project (Grant/Award Number: 2021S263).
Conflict of Interest
The authors declare that the research was conducted in the absence of any commercial or financial relationships that could be construed as a potential conflict of interest.
Publisher’s Note
All claims expressed in this article are solely those of the authors and do not necessarily represent those of their affiliated organizations, or those of the publisher, the editors and the reviewers. Any product that may be evaluated in this article, or claim that may be made by its manufacturer, is not guaranteed or endorsed by the publisher.
Supplementary Material
The Supplementary Material for this article can be found online at: https://www.frontiersin.org/articles/10.3389/fmicb.2022.892505/full#supplementary-material
References
Abd Elrazak, A., Ward, A. C., and Glassey, J. (2013). Polyunsaturated fatty acid production by marine bacteria. Bioprocess Biosyst. Eng. 36, 1641–1652. doi: 10.1007/s00449-013-0936-0
Agrawal, S., Adholeya, A., Barrow, C. J., and Deshmukh, S. K. (2018). Marine fungi: an untapped bioresource for future cosmeceuticals. Phytochem. Lett. 23, 15–20. doi: 10.1016/j.phytol.2017.11.003
Alves, A., Sousa, E., Kijjoa, A., and Pinto, M. (2020). Marine-derived compounds with potential use as cosmeceuticals and nutricosmetics. Molecules 25:2536. doi: 10.3390/molecules25112536
Ambati, R. R., Gogisetty, D., Aswathanarayana, R. G., Ravi, S., Bikkina, P. N., Bo, L., et al. (2018). Industrial potential of carotenoid pigments from microalgae: current trends and future prospects. Crit. Rev. Food Sci. Nutr. 59, 1880–1902. doi: 10.1080/10408398.2018.1432561
Ambati, R., Phang, S. M., Ravi, S., and Aswathanarayana, R. (2014). Astaxanthin: sources, extraction, stability, biological activities and its commercial applications—a review. Mar. Drugs 12, 128–152. doi: 10.3390/md12010128
Arad, S., and Yaron, A. (1992). Natural pigments from red microalgae for use in foods and cosmetics. Trends Food Sci. Technol. 3, 92–97. doi: 10.1016/0924-2244(92)90145-m
Balasubramaniam, V., Gunasegavan, R. D.-N., Mustar, S., Lee, J. C., and Mohd Noh, M. F. (2021). Isolation of industrial important bioactive compounds from microalgae. Molecules 26:943. doi: 10.3390/molecules26040943
Barrett, J. R. (2005). Chemical exposures: the ugly side of beauty products. Environ. Health Perspect. 113:A24. doi: 10.1289/ehp.113-a24
Battistin, M., Dissette, V., Bonetto, A., Durini, E., Manfredini, S., Marcomini, A., et al. (2020). A new approach to uv protection by direct surface functionalization of TiO2 with the antioxidant polyphenol dihydroxyphenyl benzimidazole carboxylic acid. J. Nanomater. 10:231. doi: 10.3390/nano10020231
Bedoux, G., Hardouin, K., Burlot, A. S., and Bourgougnon, N. (2014). Bioactive components from seaweeds: cosmetic applications and future development. Adv. Bot. Res. 71, 345–378. doi: 10.1016/b978-0-12-408062-1.00012-3
Bermejo, R., Gabriel Acién, F., Ibáñez, M. J., Fernández, J. M., Molina, E., and Alvarez-Pez, J. M. (2003). Preparative purification of β-phycoerythrin from the microalga porphyridium cruentum by expanded-bed adsorption chromatography. J. Chromatogr. B 790, 317–325. doi: 10.1016/s1570-0232(03)00168-5
Borel, M., Lamarque, E., and Loing, E. (2017). Unique natural exopolysaccharides for biomimetic protective effect against urban pollution. J. Cosmet. Sci. 68, 126–132.
Brunt, E. G., and Burgess, J. G. (2018). The promise of marine molecules as cosmetic active ingredients. Int. J. Cosmet. Sci. 40, 1–15. doi: 10.1111/ics.12435
Burger, P., Landreau, A., Azoulay, S., Michel, T., and Fernandez, X. (2016). Skin whitening cosmetics: feedback and challenges in the development of natural skin lighteners. Cosmetics 3:36. doi: 10.3390/cosmetics3040036
Capon, R. J., Stewart, M., Ratnayake, R., Lacey, E., and Gill, J. H. (2007). Citromycetins and Bilains A–C: new aromatic polyketides and diketopiperazines from australian marine-derived and Terrestrial Penicillium spp. J. Nat. Prod. 70, 1746–1752. doi: 10.1021/np0702483
Chaves Lopes, F., Rosa de Castro, M., Caldas Barbosa, S., Primel, E. G., and de Martinez Gaspar Martins, C. (2020). Effect of the UV filter, Benzophenone-3, on biomarkers of the yellow clam (Amarilladesma mactroides) under different pH conditions. Mar. Pollut. Bull. 158:111401. doi: 10.1016/j.marpolbul.2020.111401
Chen, C. L., Liou, S. F., Chen, S. J., and Shih, M. F. (2011). Protective effects of Chlorella-derived peptide on UVB-induced production of MMP-1 and degradation of procollagen genes in human skin fibroblasts. Regul. Toxicol. Pharmacol. 60, 112–119. doi: 10.1016/j.yrtph.2011.03.001
Chrapusta, E., Kaminski, A., Duchnik, K., Bober, B., Adamski, M., and Bialczyk, J. (2017). Mycosporine-like amino acids: potential health and beauty ingredients. Mar. Drugs 15:326. doi: 10.3390/md15100326
Corinaldesi, C., Barone, G., Marcellini, F., Dell' Anno, A., and Danovaro, R. (2017). Marine microbial-derived molecules and their potential use in cosmeceutical and cosmetic products. Mar. Drugs 15:118. doi: 10.3390/md15040118
Costin, G. E., and Hearing, V. J. (2007). Human skin pigmentation: melanocytes modulate skin color in response to stress. FASEB J. 21, 976–994. doi: 10.1096/fj.06-6649rev
Courtois, A., Berthou, C., Guézennec, J., Boisset, C., and Bordron, A. (2014). Exopolysaccharides isolated from hydrothermal vent bacteria can modulate the complement system. PLoS One 9:e94965. doi: 10.1371/journal.pone.0094965
Couteau, C., and Coiffard, L. (2020). Phycocosmetics and other marine cosmetics, specific cosmetics formulated using marine resources. Mar. Drugs 18:322. doi: 10.3390/md18060322
Dash, H. R., Mangwani, N., Chakraborty, J., Kumari, S., and Das, S. (2012). Marine bacteria: potential candidates for enhanced bioremediation. Appl. Microbiol. Biotechnol. 97, 561–571. doi: 10.1007/s00253-012-4584-0
Deering, R. W., Chen, J., Sun, J., Ma, H., Dubert, J., Barja, J. L., et al. (2016). N-acyl dehydrotyrosines, tyrosinase inhibitors from the marine bacterium Thalassotalea sp. PP2-459. J. Nat. Prod. 79, 447–450. doi: 10.1021/acs.jnatprod.5b00972
Dessinioti, C., Stratigos, A. J., Rigopoulos, D., and Katsambas, A. D. (2009). A review of genetic disorders of hypopigmentation: lessons learned from the biology of melanocytes. Exp. Dermatol. 18, 741–749. doi: 10.1111/j.1600-0625.2009.00896.x
Dhale, M. A., and Vijay-Raj, A. S. (2009). Pigment and amylase production in Penicillium sp. NIOM-02 and its radical scavenging activity. Int. J. Food Sci. Technol. 44, 2424–2430. doi: 10.1111/j.1365-2621.2009.01983.x
Downs, C. A., Kramarsky-Winter, E., Segal, R., Fauth, J., Knutson, S., Bronstein, O., et al. (2015). Toxicopathological effects of the sunscreen UV filter, Oxybenzone (Benzophenone-3), on coral planulae and cultured primary cells and its environmental contamination in Hawaii and the U.S. Virgin Islands. Arch. Environ. Contam. Toxicol. 70, 265–288. doi: 10.1007/s00244-015-0227-7
Draelos, Z. D. (2018). Cosmeceuticals what’s real, what’s not. Dermatol. Clin. 37, 107–115. doi: 10.1016/j.det.2018.07.001
Du, L., Zhu, T., Liu, H., Fang, Y., Zhu, W., and Gu, Q. (2008). Cytotoxic polyketides from a marine-derived fungus Aspergillus glaucus. J. Nat. Prod. 71, 1837–1842. doi: 10.1021/np800303t
Duval, B., Shetty, K., and Thomas, W. H. (1999). Phenolic compounds and antioxidant properties in the snow alga Chlamydomonas nivalis after exposure to UV light. J. Appl. Phycol. 11, 559–566. doi: 10.1023/a:1008178208949
Ehling-Schulz, M., Bilger, W., and Scherer, S. (1997). UV-B-induced synthesis of photoprotective pigments and extracellular polysaccharides in the terrestrial cyanobacterium Nostoc commune. J. Bacteriol. 179, 1940–1945. doi: 10.1128/jb.179.6.1940-1945.1997
Elias, P. M., and Friend, D. S. (1975). The permeability barrier in mammalian epidermis. J. Cell Biol. 65, 180–191. doi: 10.1083/jcb.65.1.180
Endly, D. C., and Miller, R. A. (2017). Oily skin: a review of treatment options. J. Clin. Aesthet. Dermatol. 10, 49–55.
Fu, Y., Hoelsch, K., and Weuster-Botz, D. (2012). A novel ene-reductase from Synechococcus sp. PCC 7942 for the asymmetric reduction of alkenes. Process Biochem. 47, 1988–1997. doi: 10.1016/j.procbio.2012.07.009
Galasso, C., Corinaldesi, C., and Sansone, C. (2017). Carotenoids from marine organisms: biological functions and industrial applications. Antioxidants 6:96. doi: 10.3390/antiox6040096
Ganceviciene, R., Liakou, A. I., Theodoridis, A., Makrantonaki, E., and Zouboulis, C. C. (2012). Skin anti-aging strategies, Skin anti-aging strategies. Dermatoendocrinol. 4, 308–319. doi: 10.4161/derm.22804
Ganesh Kumar, C., Sahu, N., Narender Reddy, G., Prasad, R. B. N., Nagesh, N., and Kamal, A. (2013). Production of melanin pigment from Pseudomonas stutzeri isolated from red seaweed Hypnea musciformis. Lett. Appl. Microbiol. 57, 295–302. doi: 10.1111/lam.12111
Ganuza, E., Yang, S., Amezquita, M., Giraldo-Silva, A., and Andersen, R. A. (2019). Genomics, biology and phylogeny aurantiochytrium Acetophilum sp. nov. (thraustrochytriaceae), including first evidence of sexual reproduction. Protist 170, 209–232. doi: 10.1016/j.protis.2019.02.004
Garre, A., Martinez-masana, G., Piquero-casals, J., and Granger, C. (2017). Redefining face contour with a novel anti-aging cosmetic product: an open-label, prospective clinical study. Clin. Cosmet. Investig. Dermatol. 10, 473–482. doi: 10.2147/ccid.s148597
Gomez, C. G., Pérez Lambrecht, M. V., Lozano, J. E., Rinaudo, M., and Villar, M. A. (2009). Influence of the extraction–purification conditions on final properties of alginates obtained from brown algae (Macrocystis pyrifera). Int. J. Biol. Macromol. 44, 365–371. doi: 10.1016/j.ijbiomac.2009.02.005
Greenspoon, J., Ahluwalia, R., Juma, N., and Rosen, C. F. (2013). Allergic and photoallergic contact dermatitis. Dermatitis 24, 29–32. doi: 10.1097/der.0b013e31827edc8b
Guillerme, J. B., Couteau, C., and Coiffard, L. (2017). Applications for marine resources in cosmetics. Cosmetics 4:35. doi: 10.3390/cosmetics4030035
Hamed, I. (2016). The evolution and versatility of microalgal biotechnology: a review. Compr. Rev. Food. Sci. Food Saf. 15, 1104–1123. doi: 10.1111/1541-4337.12227
Haoujar, I., Cacciola, F., Abrini, J., Mangraviti, D., Giuffrida, D., Oulad El Majdoub, Y., et al. (2019). The contribution of carotenoids, phenolic compounds, and flavonoids to the antioxidative properties of marine microalgae isolated from mediterranean Morocco. Molecules 24:4037. doi: 10.3390/molecules24224037
Hasan, F., Shah, A. A., and Hameed, A. (2006). Industrial applications of microbial lipases. Enzym. Microb. Technol. 39, 235–251. doi: 10.1016/j.enzmictec.2005.10.016
Hashtroudi, M. S., Shariatmadari, Z., Riahi, H., and Ghassempour, A. (2013). Analysis of anabaena vaginicola and nostoc calcicola from northern Iran, as rich sources of major carotenoids. Food Chem. 136, 1148–1153. doi: 10.1016/j.foodchem.2012.09.055
He, H., Li, A., Li, S., Tang, J., Li, L., and Xiong, L. (2021). Natural components in sunscreens: topical formulations with sun protection factor (SPF). Biomed. Pharmacother. 134:111161. doi: 10.1016/j.biopha.2020.111161
Hong, J. Y., Park, S. J., Seo, S. J., and Park, K. Y. (2020). Oily sensitive skin: a review of management options. J. Cosmet. Dermatol. 19, 1016–1020. doi: 10.1111/jocd.13347
Jin, M., Chen, Y., He, X., Hou, Y., Chan, Z., and Zeng, R. (2020). Amelioration of androgenetic alopecia by algal oligosaccharides prepared by deep-sea bacterium biodegradation. Front. Microbiol. 11:567060. doi: 10.3389/fmicb.2020.567060
Jϋttner, F., and Hans, R. (1986). The reducing capacities of cyanobacteria for aldehydes and ketones. Appl. Microbiol. Biotechnol. 25, 52–54. doi: 10.1007/bf00252512
Kalra, R., Conlan, X. A., and Goel, M. (2020). Fungi as a potential source of pigments: harnessing filamentous fungi. Front. Chem. 8:369. doi: 10.3389/fchem.2020.00369
Kang, K. H., Qian, Z. J., Ryu, B., Karadeniz, F., Kim, D., and Kim, S. K. (2012). Antioxidant peptides from protein hydrolysate of microalgae Navicula incerta and their protective effects in HepG2/CYP2E1 cells induced by ethanol. Phytother. Res. 26, 1555–1563. doi: 10.1002/ptr.4603
Kang, H. Y., Yoon, T.-J., and Lee, G. J. (2011). Whitening effects of marine pseudomonas extract. Ann. Dermatol. 23, 144–149. doi: 10.5021/ad.2011.23.2.144
Kanlayavattanakul, M., and Lourith, N. (2015). “Biopolysaccharides for skin hydrating cosmetics,” in Polysaccharides, eds. K. G. Ramawat and J.-M. Me´rillon (FL: Springer Cham 2015) 1867–1892.
Kerdudo, A., Burger, P., Merck, F., Dingas, A., Rolland, Y., Michel, T., et al. (2016). Development of a natural ingredient – natural preservative: a case study. C. R. Chim. 19, 1077–1089. doi: 10.1016/j.crci.2016.06.004
Khan, I., Peng, J., Fang, Z., Liu, W., Zhang, W., Zhang, Q., et al. (2021). Cylindromicin from arctic-derived fungus Tolypocladium sp. SCSIO 26:1080. doi: 10.3390/molecules26041080
Kim, K., Leutou, A. S., Jeong, H., Kim, D., Seong, C. N., Nam, S.-J., et al. (2017). Anti-pigmentary effect of (−)-4-hydroxysattabacin from the marine-derived bacterium Bacillus sp. Mar. Drugs 15:138. doi: 10.3390/md15050138
Kim, S. K., Ravichandran, Y. D., Khan, S. B., and Kim, Y. T. (2008). Prospective of the cosmeceuticals derived from marine organisms. Biotechnol. Bioprocess Eng. 13, 511–523. doi: 10.1007/s12257-008-0113-5
Kogej, T., Gostinčar, C., Volkmann, M., Gorbushina, A. A., and Gunde-Cimerman, N. (2006). Mycosporines in extremophilic fungi—novel complementary osmolytes? Environ. Chem. 3, 105–110. doi: 10.1071/en06012
Kostyuk, V., Potapovich, A., Albuhaydar, A. R., Mayer, W., De Luca, C., and Korkina, L. (2018). Natural substances for prevention of skin photoaging: screening systems in the development of sunscreen and rejuvenation cosmetics. Rejuvenation Res. 21, 91–101. doi: 10.1089/rej.2017.1931
Kuang, J., Chen, Y., Chu, G., Li, Y., and Yi, G. (2019). The extraction process of astaxanthin and its application in cosmetics were reviewed. Guangdong Chem. 46, 79–81.
Lee, K. E., Nho, Y. H., Yun, S. K., Park, S. M., Kang, S., and Yeo, H. (2020). Caviar extract and its constituent DHA inhibits UVB-irradiated skin aging by inducing adiponectin production. Int. J. Mol. Sci. 21:3383. doi: 10.3390/ijms21093383
Letsiou, S., Bakea, A., Goff, G. L., Lopes, P., Gardikis, K., Weis, M., et al. (2020). Marine fungus Aspergillus chevalieri TM2-S6 extract protects skin fibroblasts from oxidative stress. Mar. Drugs 18:460. doi: 10.3390/md18090460
Leya, T., Rahn, A., Lütz, C., and Remias, D. (2009). Response of arctic snow and permafrost algae to high light and nitrogen stress by changes in pigment composition and applied aspects for biotechnology. FEMS Microbiol. Ecol. 67, 432–443. doi: 10.1111/j.1574-6941.2008.00641.x
Li, J., Hu, Q., Li, Y., and Xu, Y. (2015). Purification and characterization of cold-adapted beta-agarase from an Antarctic psychrophilic strain. Braz. J. Microbiol. 46, 683–690. doi: 10.1590/s1517-838246320131289
Li, X., Jeong, J. H., Lee, K. T., Rho, J. R., Choi, H. D., Kang, J. S., et al. (2003). Gamma-Pyrone derivatives, kojic acid methyl ethers from a marine-derived fungus Altenaria sp. Arch. Pharm. Res. 26, 532–534. doi: 10.1007/bf02976876
Li, X., Kim, M. K., Lee, U., Kim, S.-K., Kang, J. S., Choi, H. D., et al. (2005). Myrothenones A and B, Cyclopentenone derivatives with tyrosinase inhibitory activity from the marine-derived fungus Myrothecium sp. Chem. Pharm. Bull. 36:39. doi: 10.1002/chin.200539194
Li, J., Lee, Y. M., Hong, J., Bae, K. S., Choi, J. S., and Jung, J. H. (2011). A new antioxidant from the marine sponge-derived fungus Aspergillus versicolor. Nat. Prod. Sci. 17, 14–18.
Li, Y., Li, X., Kim, S.-K., Kang, J. S., Choi, H. D., Rho, J. R., et al. (2004). Golmaenone, a new Diketopiperazine alkaloid from the marine-derived fungus Aspergillus sp. Chem. Pharm. Bull. 52, 375–376. doi: 10.1248/cpb.52.375
Lim, S.-J., Min, D.-J., Kim, S., Lee, J., Lee, E.-S., Kim, H., et al. (2021). Pseudoalteromone A, a ubiquinone derivative from marine Pseudoalteromonas spp., suppresses melanogenesis. Mar. Drugs 19:612. doi: 10.3390/md19110612
Liu, Q., Chen, C., and Zhao, S. (2016). Research, development and prospect of enzyme cosmetics. Pharm. Biotechnol. 23, 462–466. doi: 10.19526/j.cnki.1005-8915.2016.05.019
Liu, X., and Osawa, T. (2007). Cis astaxanthin and especially 9-cis astaxanthin exhibits a higher antioxidant activity in vitro compared to the all-trans isomer. Biochem. Biophys. Res. Commun. 357, 187–193. doi: 10.1016/j.bbrc.2007.03.120
Llewellyn, C. A., and Airs, R. L. (2010). Distribution and abundance of MAAs in 33 species of microalgae across 13 classes. Mar. Drugs 8, 1273–1291. doi: 10.3390/md8041273
Long, M., Yu, Z., and Xu, X. (2010). A novel beta-agarase with high pH stability from marine Agarivorans sp. LQ48. Mar. Biotechnol. 12, 62–69. doi: 10.1007/s10126-009-9200-7
Longeon, A., Copp, B. R., Quévrain, E., Roué, M., Kientz, B., Cresteil, T., et al. (2011). Bioactive indole derivatives from the South Pacific marine sponges Rhopaloeides odorabile and Hyrtios sp. Mar. Drugs 9, 879–888. doi: 10.3390/md9050879
Lourenço-Lopes, C., Fraga-Corral, M., Jimenez-Lopez, C., Pereira, A. G., Garcia-Oliveira, P., Carpena, M., et al. (2020). Metabolites from macroalgae and its applications in the cosmetic industry: a circular economy approach. Resources 9:101. doi: 10.3390/resources9090101
Macedo, G. A., Lozano, M. M. S., and Pastore, G. M. (2003). Enzymatic synthesis of short chain citronellyl esters by a new lipase from Rhizopus sp. Electron. J. Biotechnol. 6, 72–75. doi: 10.4067/S0717-34582003000100009
Martini, M. C. (2017). Self-tanning and sunless tanning products. Ann. Dermatol. Venereol. 144, 638–644. doi: 10.1016/j.annder.2017.03.021
Martins, A., Vieira, H., Gaspar, H., and Santos, S. (2014). Marketed marine natural products in the pharmaceutical and cosmeceutical industries: tips for success. Mar. Drugs 12, 1066–1101. doi: 10.3390/md12021066
Mateos, R., Pérez-Correa, J. R., and Domínguez, H. (2020). Bioactive properties of marine phenolics. Mar. Drugs 18:501. doi: 10.3390/md18100501
Matsui, M., Tanaka, K., Higashiguchi, N., Okawa, H., Yamada, Y., Tanaka, K., et al. (2016). Protective and therapeutic effects of fucoxanthin against sunburn caused by UV irradiation. J. Pharmacol. Sci. 132, 55–64. doi: 10.1016/j.jphs.2016.08.004
Matsuno, T., and Miki, W. (1990). Biological functions and activities of animal carotenoids. Kagaku Seibutsu. 28, 219–227. doi: 10.1271/kagakutoseibutsu1962.28.219
Meesapyodsuk, D., and Qiu, X. (2016). Biosynthetic mechanism of very long chain polyunsaturated fatty acids in Thraustochytrium sp. 26185. J. Lipid Res. 57, 1854–1864. doi: 10.1194/jlr.m070136
Metzger, J. O., and Bornscheuer, U. (2006). Lipids as renewable resources: current state of chemical and biotechnological conversion and diversification. Appl. Microbiol. Biotechnol. 71, 13–22. doi: 10.1007/s00253-006-0335-4
Mezzomo, N., and Ferreira, S. R. S. (2016). Carotenoids functionality, sources, and processing by supercritical technology: a review. J. Chemother. 2016, 1–16. doi: 10.1155/2016/3164312
Miyamoto, K. T., Komatsu, M., and Ikeda, H. (2014). Discovery of gene cluster for mycosporine-Like amino acid biosynthesis from actinomycetales microorganisms and production of a novel mycosporine-Like amino acid by heterologous expression. Appl. Environ. Microbiol. 80, 5028–5036. doi: 10.1128/aem.00727-14
Morais, T., Cotas, J., Pacheco, D., and Pereira, L. (2021). Seaweeds compounds: an ecosustainable source of cosmetic ingredients? Cosmetics 8:8. doi: 10.3390/cosmetics8010008
Mourelle, M., Gómez, C., and Legido, J. (2017). The potential use of marine microalgae and cyanobacteria in cosmetics and thalassotherapy. Cosmetics 4:46. doi: 10.3390/cosmetics4040046
Mudimu, O., Koopmann, I. K., Rybalka, N., Friedl, T., Schulz, R., and Bilger, W. (2017). Screening of microalgae and cyanobacteria strains for α-tocopherol content at different growth phases and the influence of nitrate reduction on α-tocopherol production. J. Appl. Phycol. 29, 2867–2875. doi: 10.1007/s10811-017-1188-1
Naguib, Y. M. A. (2000). Antioxidant activities of astaxanthin and related carotenoids. J. Agric. Food Chem. 48, 1150–1154. doi: 10.1021/jf991106k
Nalini, S., Sandy Richard, D., Mohammed Riyaz, S. U., Kavitha, G., and Inbakandan, D. (2018). Antibacterial macro molecules from marine organisms. Int. J. Biol. Macromol. 115, 696–710. doi: 10.1016/j.ijbiomac.2018.04.110
Nedeljka, N. R. (2021). Recent advances in the discovery of novel marine natural products and mycosporine-like amino acid UV-absorbing compounds. Appl. Environ. Microbiol. 105, 7053–7067. doi: 10.1007/s00253-021-11467-9
Nishihara, M., Kamata, M., Koyama, T., and Yazawa, K. (2008). New phospholipase A1-producing bacteria from a marine fish. Mar. Biotechnol. 10, 382–387. doi: 10.1007/s10126-007-9074-5
Pangestuti, R., Suryaningtyas, I. T., Siahaan, E. A., and Kim, S. K. (2020). “Cosmetics and cosmeceutical applications of microalgae pigments,” in Pigments From Microalgae Handbook. eds. E. Jacob-Lopes, M. Isabel Queiroz, and L. Queiroz Zepka (Switzerland, AG: Springer Nature), 611–633.
Peng, X., Adachi, K., Chen, C., Kasai, H., Kanoh, K., Shizuri, Y., et al. (2006). Discovery of a marine bacterium producing 4-hydroxybenzoate and its alkyl esters, parabens. Appl. Environ. Microbiol. 72, 5556–5561. doi: 10.1128/aem.00494-06
Pimentel, F., Alves, R., Rodrigues, F., and Oliveira, M. B. P. P. (2017). Macroalgae-derived ingredients for cosmetic industry—an update. Cosmetics 5, 1–18. doi: 10.3390/cosmetics5010002
Proksch, E., Fölster-Holst, R., Bräutigam, M., Sepehrmanesh, M., Pfeiffer, S., and Jensen, J. M. (2009). Role of the epidermal barrier in atopic dermatitis. J. Dtsch. Dermatol. Ges. 7, 899–910. doi: 10.1111/j.1610-0387.2009.07157.x
Raveendran, S., Palaninathan, V., Chauhan, N., Sakamoto, Y., Yoshida, Y., Maekawa, T., et al. (2013). In vitro evaluation of antioxidant defense mechanism and hemocompatibility of mauran. Carbohydr. Polym. 98, 108–115. doi: 10.1016/j.carbpol.2013.04.050
Rehman, S. U., Yang, L.-J., Zhang, Y.-H., Wu, J.-S., Shi, T., Haider, W., et al. (2020). Sorbicillinoid derivatives from sponge-derived fungus Trichoderma reesei (HN-2016-018). Front. Microbiol. 11:1334. doi: 10.3389/fmicb.2020.01334
Resende, D. I. S. P., Ferreira, M., Magalhães, C., Sousa Lobo, J. M., Sousa, E., and Almeida, I. F. (2021). Trends in the use of marine ingredients in anti-aging cosmetics. Algal Res. 55:102273. doi: 10.1016/j.algal.2021.102273
Riad, N., Zahi, M. R., Bouzidi, N., Daghbouche, Y., Touafek, O. E., and Hattab, M. (2021). Occurrence of marine ingredients in fragrance: update on the state of knowledge. Chemistry 3, 1437–1463. doi: 10.3390/chemistry3040103
Rinaudo, M. (2006). Chitin and chitosan: properties and applications. Prog. Polym. Sci. 31, 603–632. doi: 10.1016/j.progpolymsci.2006.06.001
Ryu, B., Himaya, S. W. A., and Kim, S.-K. (2015). “Applications of microalgae-derived active ingredients as cosmeceuticals,” in Handbook of Marine Microalgae. FL: Academic Press, 309–316.
Said Hassane, C., Fouillaud, M., Le Goff, G., Sklirou, A. D., Boyer, J. B., Trougakos, I. P., et al. (2020). Microorganisms associated with the marine sponge scopalina hapalia: a reservoir of bioactive molecules to slow down the aging process. Microorganisms 8:1262. doi: 10.3390/microorganisms8091262
Sang, S. (2017). Screening of ferulic acid esterase from marine source and its biodegradation ability to lignocellulosic materials. Jiangsu Agric. Sci. 45, 275–278. doi: 10.15889/j.issn.1002-1302.2017.20.068
Sang, Z., and Leung, K. S.-Y. (2016). Environmental occurrence and ecological risk assessment of organic UV filters in marine organisms from Hong Kong coastal waters. Sci. Total Environ. 566–567, 489–498. doi: 10.1016/j.scitotenv.2016.05.120
Shanuja, S. K., Iswarya, S., Sridevi, J., and Gnanamani, A. (2018). Exploring the UVB-protective efficacy of melanin precursor extracted from marine imperfect fungus: featuring characterization and application studies under in vitro conditions. Int. Microbiol. 21, 59–71. doi: 10.1007/s10123-018-0005-2
Shen, C. T., Chen, P. Y., Wu, J. J., Lee, T. M., Hsu, S. L., Chang, C. M. J., et al. (2011). Purification of algal anti-tyrosinase zeaxanthin from nannochloropsis oculata using supercritical anti-solvent precipitation. J. Supercrit. Fluids 55, 955–962. doi: 10.1016/j.supflu.2010.10.003
Shindo, K., Kikuta, K., Suzuki, A., Katsuta, A., Kasai, H., Yasumoto-Hirose, M., et al. (2007). Rare carotenoids, (3r)-saproxanthin and (3r,2′s)-myxol, isolated from novel marine bacteria (flavobacteriaceae) and their antioxidative activities. Appl. Microbiol. Biotechnol. 74, 1350–1357. doi: 10.1007/s00253-006-0774-y
Silva Teixeira, C. S., Cerqueira, N. M. F. S. A., and Silva Ferreira, A. C. (2015). Unravelling the olfactory sense: from the gene to odor perception. Chem. Senses 41, 105–121. doi: 10.1093/chemse/bjv075
Sinha, R. P., Singh, S. P., and Häder, D. P. (2007). Database on mycosporines and mycosporine-Like amino acids (MAAs) in fungi, cyanobacteria, macroalgae, phytoplankton and animals. J. Photochem. Photobiol. B 89, 29–35. doi: 10.1016/j.jphotobiol.2007.07.006
Sivakumar, G., Jeong, K., and Lay, J. O. (2014). Biomass and RRR-α-tocopherol production in Stichococcus bacillaris strain siva2011 in a balloon bioreactor. Microb. Cell Factories 13:79. doi: 10.1186/1475-2859-13-79
Stoyneva-Gärtner, M., Uzunov, B., and Gärtner, G. (2020). Enigmatic microalgae from aeroterrestrial and extreme habitats in cosmetics: The potential of the untapped natural sources. Cosmetics 7:27. doi: 10.3390/cosmetics7020027
Su, J., Zhang, F., Sun, W., Karuppiah, V., Zhang, G., Li, Z., et al. (2015). A new alkaline lipase obtained from the metagenome of marine sponge Ircinia sp. World J. Microbiol. Biotechnol. 31, 1093–1102. doi: 10.1007/s11274-015-1859-5
Sun, Y., Zhang, N., Zhou, J., Dong, S., Zhang, X., Guo, L., et al. (2020). Distribution, contents, and types of Mycosporine-Like amino acids (MAAs) in marine macroalgae and a database for MAAs based on these characteristics. Mar. Drugs 18:43. doi: 10.3390/md18010043
Sun, M., Zhao, F., Shi, M., Zhang, X., Zhou, B., Zhang, Y., et al. (2015). Characterization and biotechnological potential analysis of a new exopolysaccharide from the arctic marine bacterium Polaribacter sp. SM1127. Sci. Rep. 5:18435. doi: 10.1038/srep18435
Swatschek, D., Schatton, W., Kellermann, J., Müller, W. E. G., and Kreuter, J. (2002). Marine sponge collagen: isolation, characterization and effects on the skin parameters surface-pH, moisture and sebum. Eur. J. Pharm. Biopharm. 53, 107–113. doi: 10.1016/s0939-6411(01)00192-8
Thiyagarasaiyar, K., Goh, B.-H., Jeon, Y.-J., and Yow, Y.-Y. (2020). Algae metabolites in cosmeceutical: an overview of current applications and challenges. Mar. Drugs 18:323. doi: 10.3390/md18060323
Toledo, T. R., Dejani, N. N., Monnazzi, L. G. S., Kossuga, M. H., Berlinck, R. G. S., Sette, L. D., et al. (2014). Potent anti-inflammatory activity of pyrenocine a isolated from the marine-derived fungus Penicillium paxilli Ma(G)K. Mediat. Inflamm. 2014:767061. doi: 10.1155/2014/767061
Tončić, R. J., Kezić, S., Hadžavdić, S. L., and Marinović, B. (2018). Skin barrier and dry skin in the mature patient. Clin. Dermatol. 36, 109–115. doi: 10.1016/j.clindermatol.2017.10.002
Tsuchiya, T., Yamada, K., Minoura, K., Miyamoto, K., Usami, Y., Kobayashi, T., et al. (2008). Purification and determination of the chemical structure of the tyrosinase inhibitor produced by trichoderma viride strain H1-7 from a marine environment. Biol. Pharm. Bull. 31, 1618–1620. doi: 10.1248/bpb.31.1618
Verdier-Sévrain, S., and Bonté, F. (2007). Skin hydration: a review on its molecular mechanisms. J. Cosmet. Dermatol. 6, 75–82. doi: 10.1111/j.1473-2165.2007.00300.x
Vílchez, C., Forján, E., Cuaresma, M., Bédmar, F., Garbayo, I., and Vega, J. M. (2011). Marine carotenoids: biological functions and commercial applications. Mar. Drugs 9, 319–333. doi: 10.3390/md9030319
Wang, Z. (2016). Study on Enzymatic Properties of novel Marine Microbial lipase and its Catalytic Synthesis of Cinnamyl Acetate. (Guizhou, China: University of Guizhou).
Wang, H. M. D., Chen, C. C., Huynh, P., and Chang, J. S. (2015). Exploring the potential of using algae in cosmetics. Bioresour. Technol. 184, 355–362. doi: 10.1016/j.biortech.2014.12.001
Wang, J., Jin, W., Hou, Y., Niu, X., Zhang, H., and Zhang, Q. (2013). Chemical composition and moisture-absorption/retention ability of polysaccharides extracted from five algae. Int. J. Biol. Macromol. 57, 26–29. doi: 10.1016/j.ijbiomac.2013.03.001
Wu, B., Wu, X., Sun, M., and Li, M. (2013). Two novel tyrosinase inhibitory sesquiterpenes induced by cucl2 from a marine-derived fungus Pestalotiopsis sp. Z233. Mar. Drugs 11, 2713–2721. doi: 10.3390/md11082713
Xia, E., Zhu, X., Gao, X., Ni, J., and Guo, H. (2021). Antiaging potential of peptides from underused marine bioresources. Mar. Drugs 19:513. doi: 10.3390/md19090513
Xu, J., Nakazawa, T., Ukai, K., Kobayashi, H., Mangindaan, R. E. P., Wewengkang, D. S., et al. (2008). Tetrahydrobostrycin and 1-Deoxytetrahydrobostrycin, two new hexahydroanthrone derivatives, from a marine-derived fungus Aspergillus sp. J. Antibiot. 61, 415–419. doi: 10.1038/ja.2008.57
Yarkent, Ç., Gürlek, C., and Oncel, S. S. (2020). Potential of microalgal compounds in trending natural cosmetics: a review. Sustain. Chem. Pharm. 17:100304. doi: 10.1016/j.scp.2020.100304
Youn, S. W. (2010). The role of facial sebum secretion in acne pathogenesis: facts and controversies. Clin. Dermatol. 28, 8–11. doi: 10.1016/j.clindermatol.2009.03.011
Yuan, D., Lan, D., Xin, R., Yang, B., and Wang, Y. (2016). Screening and characterization of a thermostable lipase from marineStreptomycessp. Strain W007. Biotechnol. Appl. Biochem. 63, 41–50. doi: 10.1002/bab.1338
Yvergnaux, F. (2017). Lipases: particularly effective biocatalysts for cosmetic active ingredients. OCL 24:D408. doi: 10.1051/ocl/2017013
Zhang, X. (2019). Applications of Extracellular Polysaccharidle Produced by Marine Bacterium Polaribacter sp. SM127. (Shandong, China: University of ShanDong)
Zhang, S., and Duan, E. (2018). Fighting against skin aging: the way from bench to bedside. Cell Transplant. 27, 729–738. doi: 10.1177/0963689717725755
Zhang, D., Yang, X., Kang, J. S., Choi, H. D., and Son, B. W. (2008). Circumdatin I, a new ultraviolet-a protecting benzodiazepine alkaloid from a marine isolate of the fungus exophiala. J. Antibiot. 61, 40–42. doi: 10.1038/ja.2008.108
Zhang, J., Zeng, R., and Labes, A. (2021). Editorial: marine microbial-derived molecules and their potential medical and cosmetic applications. Front. Microbiol. 12:706152. doi: 10.3389/fmicb.2021.706152
Zheng, J., Piao, M. J., Keum, Y. S., Kim, H. S., and Hyun, J. W. (2013). Fucoxanthin protects cultured human keratinocytes against oxidative stress by blocking free radicals and inhibiting apoptosis. Biomol. Ther. 21, 270–276. doi: 10.4062/biomolther.2013.030
Keywords: marine microbes, natural products, cosmetic applications, metagenomic technology, biotransformation
Citation: Ding J, Wu B and Chen L (2022) Application of Marine Microbial Natural Products in Cosmetics. Front. Microbiol. 13:892505. doi: 10.3389/fmicb.2022.892505
Edited by:
Jinwei Zhang, University of Exeter, United KingdomReviewed by:
Leonel Pereira, University of Coimbra, PortugalVimala Balasubramaniam, Ministry of Health (Malasiya), Malaysia
Copyright © 2022 Ding, Wu and Chen. This is an open-access article distributed under the terms of the Creative Commons Attribution License (CC BY). The use, distribution or reproduction in other forums is permitted, provided the original author(s) and the copyright owner(s) are credited and that the original publication in this journal is cited, in accordance with accepted academic practice. No use, distribution or reproduction is permitted which does not comply with these terms.
*Correspondence: Liqun Chen, lqchen@fzu.edu.cn
†These authors share first authorship