- 1Department of Biotechnology, Pukyong National University, Busan, South Korea
- 2Aquaculture Research Division, National Institute of Fisheries Science, Busan, South Korea
- 3Biopharmaceutical Engineering Major, Division of Applied Bioengineering, Dong-Eui University, Busan, South Korea
- 4Aquaculture and Applied Life Sciences Major, Division of Fisheries Life Sciences, Pukyong National University, Busan, South Korea
- 5Department of Fisheries Biology, Pukyong National University, Busan, South Korea
- 6Department of Biology Education College of Education, Chonnam National University, Gwangju, South Korea
This study was performed to investigate the effect of microbial supplementation diet on the survival rate and microbiota composition of artificially produced eel larvae. Microorganisms supplemented in the diet were isolated from wild glass eel intestines and identified as Bacillus sp. through 16S rRNA sequencing analysis. In vitro tests confirmed that the strain had no hemolytic activity and virulence genes. Microbial supplemental feeding significantly increased the survival rate of artificially produced eel larvae for 30 days post-hatchling compared with that of the control group. It also caused changes in the α-diversity, β-diversity, and relative abundance of the bacterial communities. Analysis via phylogenetic investigation of communities by reconstruction of unobserved states predicted that these microbial community changes would significantly increase the carbohydrate metabolism, membrane transport, and cellular community pathway of the microbial supplementation group. Therefore, microbial supplementation feeding for eel aquaculture could increase the viability of artificially produced eel larvae and alter the microbial composition to induce metabolic changes.
Introduction
Japanese eel (Anguilla japonica) is a typical catadromous fish found in East Asian countries, such as Korea, Japan, China, and Taiwan (Hsu et al., 2015). Although it is considered a commercially valuable aquaculture species (Okamoto et al., 2009), eel aquaculture generally involves catching glass eels from the wild as aquaculture seeds because commercial-scale artificial breeding techniques have yet to be developed (Okamoto et al., 2009; Hsu et al., 2015). Consequently, eel aquaculture is highly dependent on the capture of glass eels. However, natural eel stock is rapidly depleting because of various factors, such as changes in the marine environment and climate, habitat destruction, and overfishing (Tsukamoto et al., 2009; Chen et al., 2014). Therefore, commercial-scale artificial propagation technology should be developed to protect natural eel resources and stabilize the aquaculture industry (Tsukamoto, 2014; Hsu et al., 2015).
Hormonally treated eels can yield consistently abundant eggs and sperm (Ohta et al., 1996a,b), which can be used for leptocephalus production and subsequent metamorphosis into glass eels (Tanaka et al., 2001, 2003). However, egg quality is still unstable (Unuma et al., 2005), and the growth and survival rates of artificially produced larvae are low (Ishikawa et al., 2001; Okamura et al., 2014; Tsukamoto, 2014). Therefore, further studies on maturation induction and larval rearing methods are needed to overcome these deficiencies (Okamoto et al., 2009).
Marine snow is composed of particulate organic matters, including dead or dying animals and phytoplankton, bacteria, and fecal matter; it can be an important food source for various organisms (Decho and Gutierrez, 2017; Shin et al., 2021). In the wild, it has also been suggested that eel larvae may feed on marine snow consistently present on the ocean surface (Otake et al., 1993; Mochioka and Iwamizu, 1996; Miller et al., 2013). They use various nutrients and substances produced by microorganisms and organic matters that constitute marine snow (Kim et al., 2018). Thus far, the most suitable feed for artificially produced eel larvae is a slurry-type feed containing shark-egg powder developed by Tanaka et al. (2001, 2003). However, it causes some problems. For instance, it is not digested and absorbed well in the intestine of the eel larvae; consequently, larvae grow slowly and have a low survival rate (Hsu et al., 2015).
Fish gut microbiota play an important role in nutrient digestion and immunological processes (Jang et al., 2021). For example, the gut microbiota of eel larvae or microbiota in marine snow may help produce monosaccharides required for the synthesis of hyaluronan, which is the main component of the bodies of preleptocephali and leptocephali (Pfeiler, 1991; Hsu et al., 2015). However, the microbiota of Japanese eel are poorly studied, and the effects of microbial supplementation on survival rate at early developmental stages are yet to be explored. Therefore, the present study is conducted to investigate the effect of a microbial supplementation diet on the survival rate and gut microbiota composition at early developmental stages of eel larvae.
Materials and Methods
Bacterial Isolation, Identification, and Characterization
Bacteria were isolated from the intestines of wild glass eels (5.1 ± 0.3 cm) caught in the Yeongsan River (34°46′05.2′′N 126°20′58.1′′E). Afterward, the intestines were separated, homogenized, and serially diluted with 0.85% saline solution. The suspension was spread on an LB agar plate and incubated at 25°C for 48 h. A single colony was isolated, cultured in liquid medium, and identified through 16S rRNA sequencing analysis. The isolated strain was evaluated to determine its viability at various temperatures, pH, and salinity. Hemolytic activity was examined by incubating bacteria on a blood agar base plate (Kisan Bio, South Korea) at 37°C for 48 h. Toxicity gene analysis was performed via PCR by using the primers shown in Supplementary Table 1. PCR was performed with a Veriti 96-Well Thermal Cycler (Applied Biosystems, Waltham, MA, United States) at Dong-Eui University Core Facility Center (Busan, South Korea).
Experimental Diet Preparation
For the experimental feed, a slurry-type diet containing shark egg as the main raw material was used (Tanaka et al., 2001; Kim et al., 2014). Shark-egg (50 g), krill meal (6 g), Soybean peptide (3 g), fishmeal (3 g), and vitamin mix (0.3 g) were mixed at 1,200 rpm for 3 min and filtered at 90 μm before use.
Artificial Production of Eel Larvae
Larvae were produced using a previously described method (Kagawa et al., 2005; Kim et al., 2007). Briefly, female (3 years old, 400–500 g) and male (3 years old, 300–400 g) eels were matured by administering salmon pituitary extract and human chorionic gonadotropin, respectively. Ovulation of female eels was induced with 17α, 20β-dihydroxy-4-pregnen-3-one and fertilized with artificially produced sperm.
Rearing System and Condition for Feeding Trial
Fertilized eggs were cultured in a 1 t square tank equipped with a cylindrical net (50 cm diameter); after hatching, they were cultured in a round tank (1.5 m height and 50 cm diameter) for 6 days. A feeding trial was conducted in 6 U-shaped tanks (20 L) (Supplementary Figure 1), and each tank dispensed 500 larvae. Water temperature and flow were maintained at 23 ± 0.1°C and 0.99–1.13 L/min, respectively. Experimental feed was given five times a day (10 ml/time). The survival rate 30 days after hatching was calculated using the following equation: Survival rate (%) = number of surviving larvae/number of hatched larvae × 100.
Microbiota Analysis
After the feeding trial, the larvae in each group were collected and washed thrice with filtered water; then, 100 larvae per group were pooled and homogenized. Bacterial DNA was isolated using FavorPrep™ Tissue Genomic DNA Extraction Mini Kit (Favorgen Biotech Corp., Taiwan) in accordance with the manufacturer’s instructions. The V3–V4 region of the isolated total DNA was amplified using primers containing the Illumina overhang adapter sequence. Afterward, library quantification, quality control, and sequencing were conducted at the Moagen (Daejeon, Republic of Korea). Data were analyzed using the EzBioCloud server.1
Bioinformatics and Statistical Analysis
Normality and homogeneity of variance of all data were assessed using Shaprio–Wilk and Levene tests, respectively. Data were analyzed using IBM’s Statistical Package for the Social Sciences software (SPSS Inc., Chicago, IL, United States) following Student’s t-test. Statistical significance was determined at P < 0.05. Data were presented as means ± standard deviations (SD). Principal coordinate analysis was based on the weighted unifrac metrics of bacterial operational taxonomic units between the different diets. Linear discriminant analytical effect size (LEfSe) was applied to identify differentially displayed taxa (biomarkers) among groups with linear discriminate analysis (LDA) score > 4.0 as the threshold. PICRUSt was used to predict the functional profiling of the intestinal microflora.
Results
Bacterial Isolation and Identification
The 16S rRNA sequences of the isolated bacteria shared 99.86, 99.59, 99.39, and 99.32% homology with the following Bacillus species: B. sonorensis NBRC 101234T (AYTN01000016), B. haynesii NRRL B-41327T (MRBL01000076), B. licheniformis ATCC 14580T (AE017333), and B. paralicheniformis KJ-16T (KY694465), respectively). The isolated strain was named Bacillus sp. FEB-1. In general, Bacillus sp. FEB-1 can survive at 20–50°C, pH 6–8, and 0–4% salinity. Optimal growth conditions were 35°C and pH 7. In addition, no virulence genes (Supplementary Figure 2) and hemolytic activity based on the pathogenic strain B. cereus KCTC 3624 were detected
Survival Rate and Growth of Anguilla japonica Larvae
Table 1 shows the survival rate, body length, and depth (vertical measurement) 30 days after hatching according to diet. The survival rate was significantly increased in the group supplemented with Bacillus sp. FEB-1 (BAC) compared with that of the control group (Con). However, body length and depth did not significantly differ between the two groups.
Microbiota Analysis
The diversity estimates of the BAC group significantly differed from those of the Con group. The Shannon value of the BAC group (2.84 ± 0.11) decreased compared with that of the Con (3.60 ± 0.13) groups. The Simpson value of the BAC group (0.21 ± 0.02) increased compared with that of the Con (0.05 ± 0.01) groups. The richness estimates of the two groups did not significantly vary (Table 2).
Figure 1 shows the results of β-diversity analysis at the genus level based on the UniFrac metric using principal coordinate analysis. The Con and BAC groups had relatively long distances, indicating low similarity, whereas each sample in the same group had a relatively close distance. Therefore, Principal coordinate analysis elucidated clear differences between the groups.
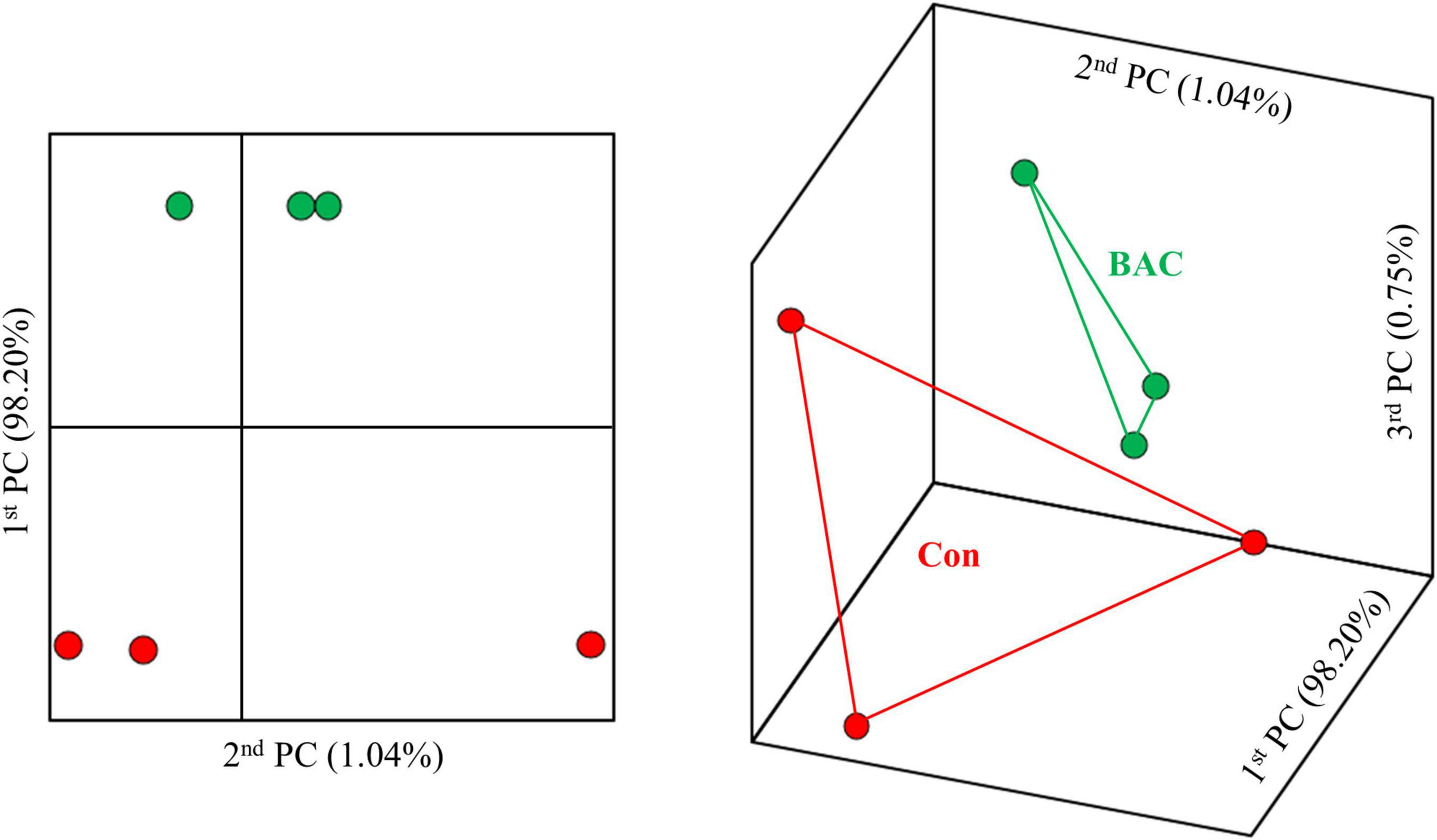
Figure 1. Principal coordinate analysis based on the weighted unifrac metrics of bacterial operational taxonomic units between the different diets.
The comparison of the relative abundance within the groups at the phylum level revealed that both groups were abundant in the order of Proteobacteria and Bacteroidetes. However, Proteobacteria accounted for a high rate of 78.48% in the Con group and a relatively low rate of 49.29% in the BAC group. The proportions of Bacteroidetes, Actinobacteria, and Acidobacteria in the BAC group were higher than those in the Con group. At the order level, Cellvibrionales was relatively abundant in the Con group, and Flavobacteriales and Rhodobacterales were abundant in the BAC group. At the genus level, Spongiibacter, Ruthia family (unclassified), and Crocinitomix were abundant in the Con group, and Tenacibaculum and Psychrobacter were relatively abundant in the BAC group (Figure 2).
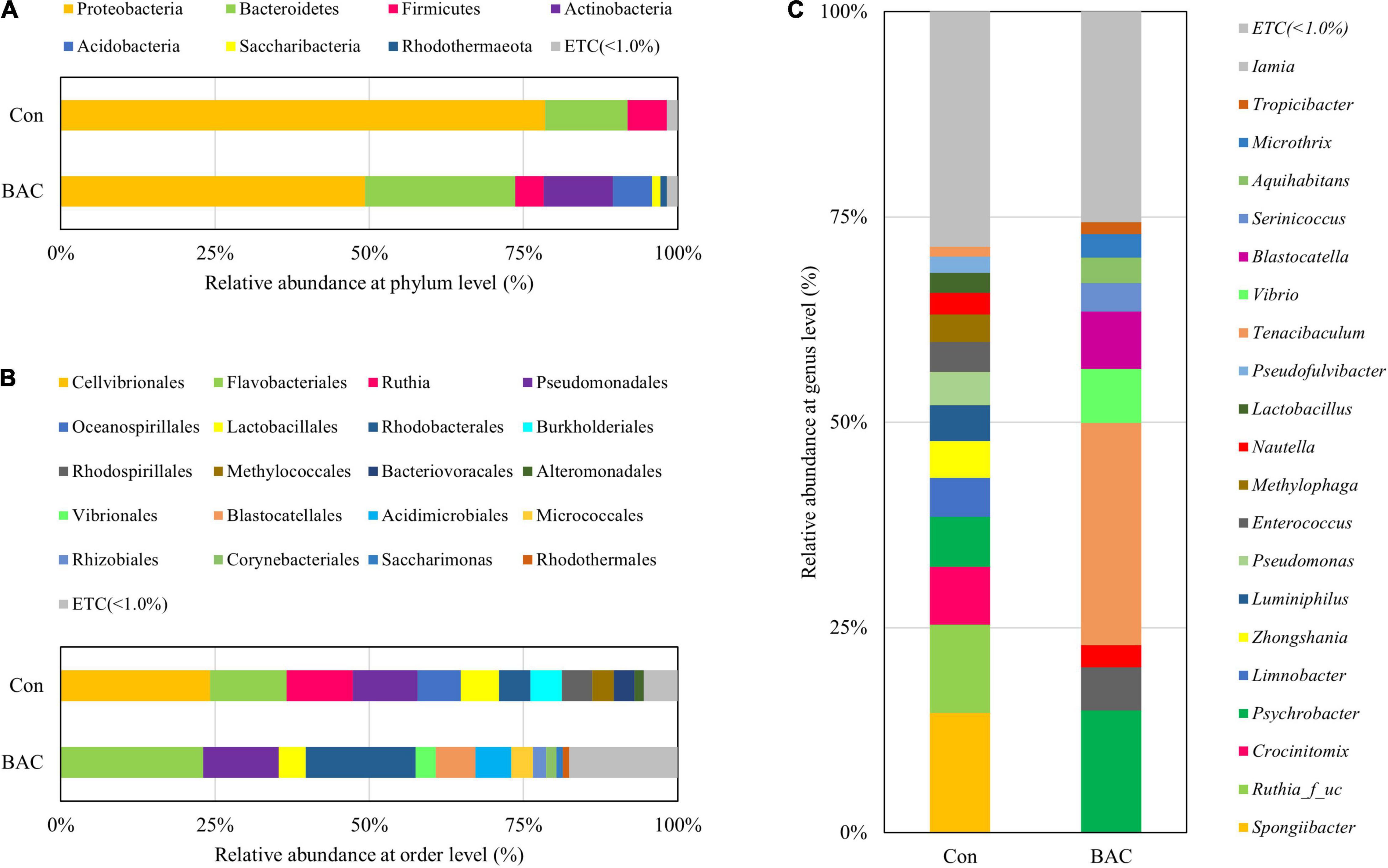
Figure 2. Average composition and relative abundance of bacterial communities of Anguilla japonica fed different diets at the phylum (A), order (B), and genus (C) levels.
The heatmap analysis highlighting the relatively high or low levels of the top 30 selected genera is shown in Figure 3. Microbial abundance evidently differed between the two groups. In particular, 14 genera, including Flavobacteriaceae_uc and Nautella, were relatively abundant in the Con group. Conversely, 16 genera, including Microthrix and Serinicoccus, were abundant in the BAC group.
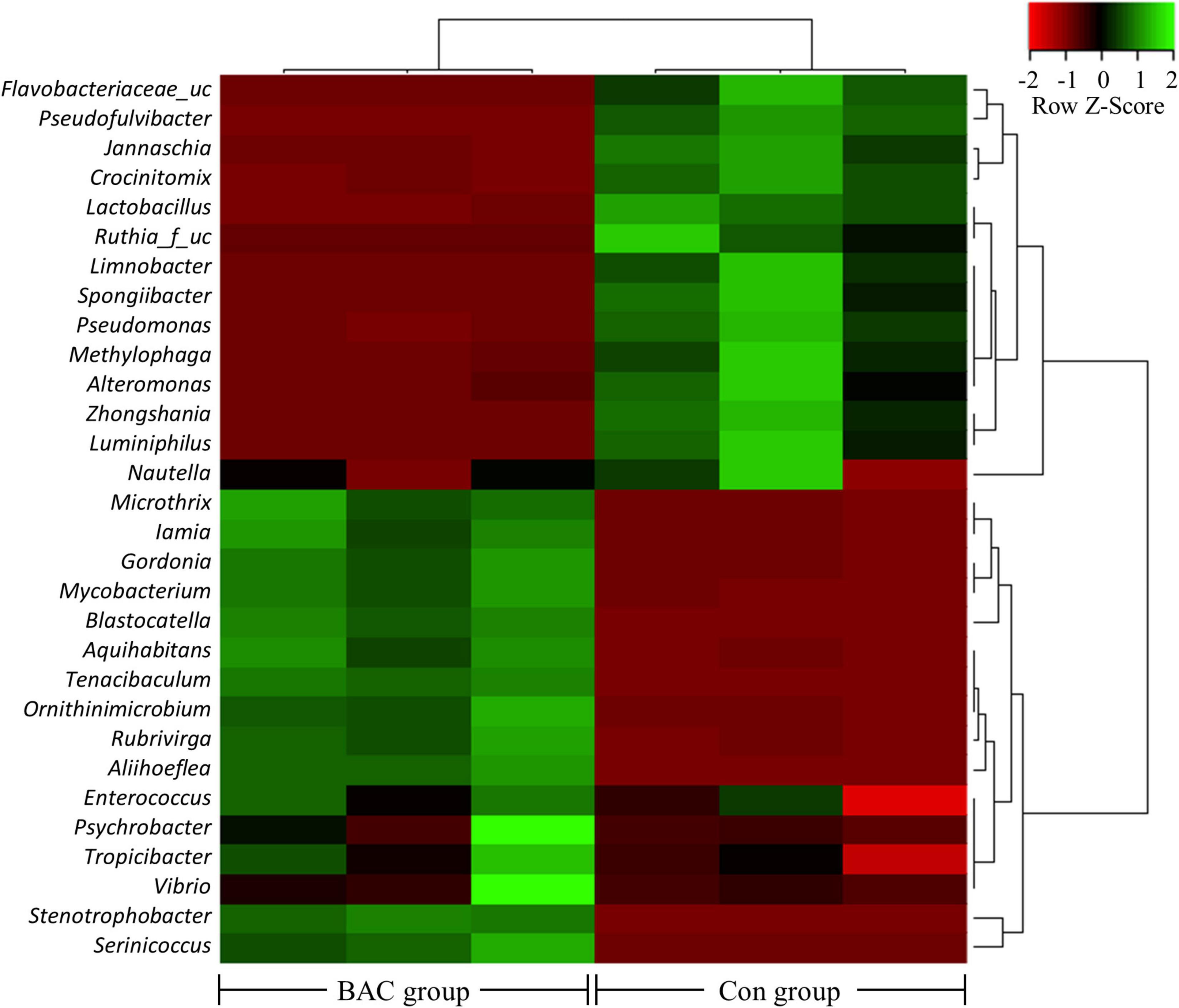
Figure 3. Heatmap analysis of the genus abundance within the A. japonica microbiota from each group. Green represents the more abundant genus in the corresponding sample and red represents the less abundant genus.
Linear discriminant analysis effect size was used to identify significant differences in the taxa of the intestinal microbiota of A. japonica larvae. The Con group showed significant differences in Proteobacteria (phylum), Gammaproteobacteria (class), Cellvibrionales (order), Spongiibacteraceae (Family), Spongiibacter (genus), Ruthia (order to genus), Crocinitomicaceae (Family), and Crocinitomix (genus). the BAC group showed significant differences in the Acidimicrobiia (class), Acidimicrobiales (order), Acidobacteria (phylum), Blastocatellia (class), Blastocatellales (order), Blastocatellaceae (Family), Actinobacteria (phylum), Bacteroidetes (phylum), Flavobacteriales (order), Flavobacteria (class), Flavobacteriaceae (Family), and Tenacibaculum (genus) (Figure 4).
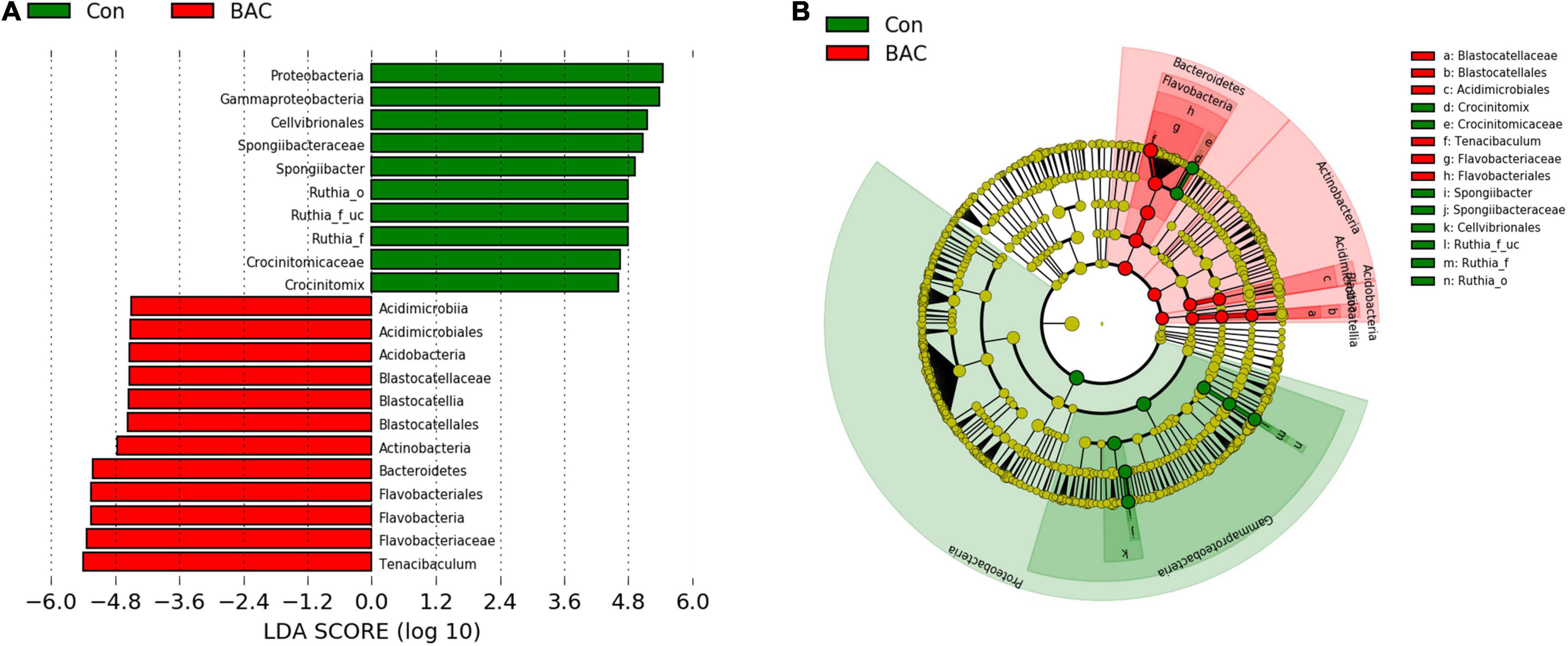
Figure 4. Linear discriminant analysis effect size (LEfSe) analysis of differential abundance of taxa within A. japonica microbiota from each group. (A) Linear discriminant analysis (LDA) score of abundance of taxa; (B) cladogram showing differentially abundant taxa between the two groups of phylum to genus.
Changes in the presumptive metabolic functions of the microbiota of A. japonica larvae were analyzed on the basis of metagenome prediction via PICRUSt. The metabolic function significantly increased in the BAC group (Figure 5). The proportions of starch and sucrose metabolism, amino sugar and nucleotide sugar metabolism, galactose metabolism, pentose and glucuronate interconversions, ABC transporters, phosphotransferase system, and quorum sensing pathways in the BAC group were significantly higher than those in the Con group.
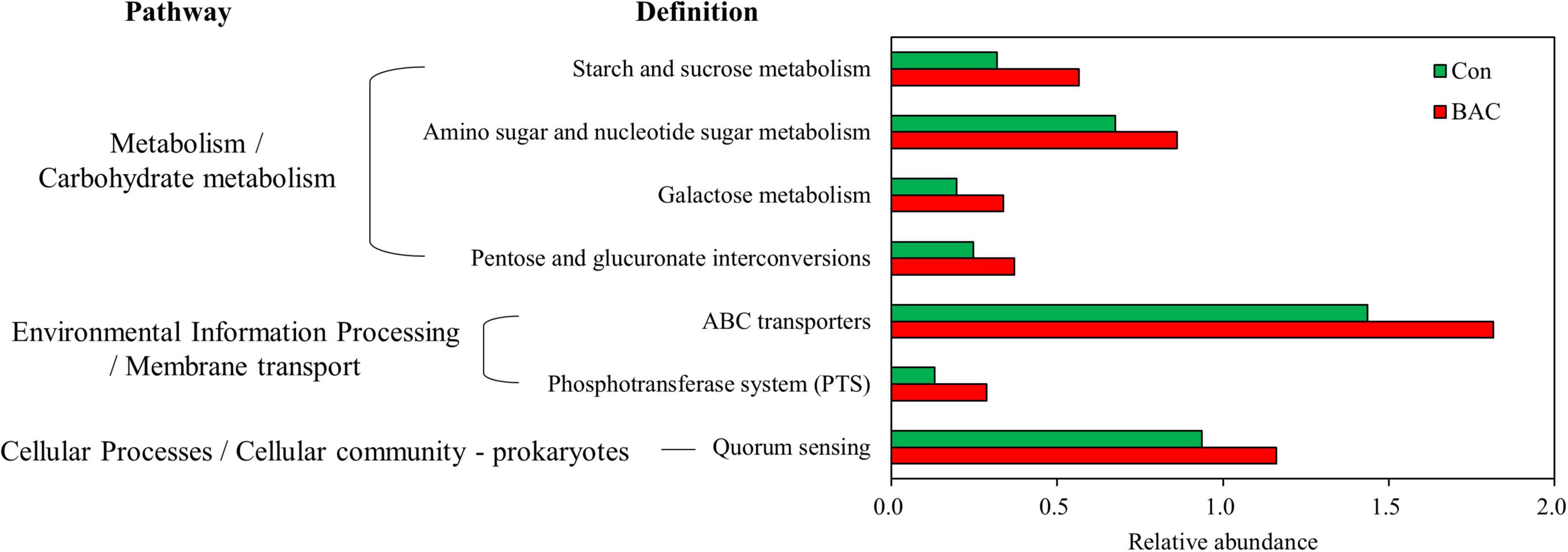
Figure 5. Presumptive metabolism functions of microbiota in A. japonica with different diets. Kyoto Encyclopedia of Genes and Genomes (KEGG) pathway was obtained from 16S metagenomic sequences using PICRUSt.
Discussion
In this study, bacteria were isolated from the intestine of wild glass eel, and the isolated strain was identified through 16S rRNA sequencing analysis as a Bacillus sp. Bacillus spp. are abundant in fish intestines and known to provide various beneficial effects to their host (Jang et al., 2021; Santos et al., 2021). For example, they enhance digestive and antioxidant enzyme activity, immunity, and stress-related gene expression; they also produce natural antibacterial compounds that antagonize pathogens, thereby improving the ability of fish to resist pathogenic microbes (Nayak, 2010; Cha et al., 2013; Buruiană et al., 2014; Kuebutornye et al., 2019; Santos et al., 2021). With these advantages, Bacillus spp. can be used as probiotics in aquaculture. Many studies have reported the positive effects of Bacillus as probiotics on various fish species (Hasan et al., 2019; Jang et al., 2021).
Van Doan et al. (2020) defined host-associated probiotics (HAPs) as bacteria originally isolated from rearing water or the gastrointestinal tract of hosts for improving the growth and health of hosts. When used as probiotics, they may have superior functionality because they can evade their hosts’ defense system and are better adapted to the host gut environment (Van Doan et al., 2020; Jang et al., 2021). Bacillus sp. FEB-1 isolated from the intestines of wild glass eels is also a HAP and may provide beneficial effects on the intestines of farmed eels.
Thus far, the most suitable feed for eel larvae is a slurry-type diet based on shark-egg powder developed by Tanaka et al. (2003). Although many studies have been conducted since then, further studies on feed ingredients and composition more suitable than slurry-type diet based on shark-egg powder have yet to be performed (Kim et al., 2014). However, this slurry-type diet is not well digested and absorbed in the intestine of eel larvae; consequently, their growth is slow, and their survival rate is low (Hsu et al., 2015). Therefore, research on feed development for the advancement of artificial propagation technology on a commercial scale is important (Shin et al., 2021).
Marine snow, known as eel food in nature, contains a high amount of carbohydrates belonging to various monosaccharides and polysaccharides, such as glucose and galactose (Cowen and Holloway, 1996; Skoog et al., 2008). Saccharides are necessary for the synthesis of hyaluronan, the main component of the bodies of preleptocephali and leptocephali; they are also important for larval growth (Pfeiler, 1991; Hsu et al., 2015). Hsu et al. (2015) conducted transcriptome analysis and reported that preleptocephalus and leptocephalus stages have low transcript levels of carbohydrate-digesting enzymes. They suggested that the gut microbiota may play an important role in low nutrient digestion and immune processes in fish (Nayak, 2010; Hsu et al., 2015). They further highlighted the importance of the activity of enzymes such as glucosidase detected in bacteria isolated from marine snow (Rath and Herndl, 1994; Hsu et al., 2015). In the present study, FEB-1 supplementation likely changed the microbiota composition of eels, possibly increasing metabolism related to various carbohydrates, such as starch, sucrose, galactose, and glucuronate. These metabolic changes might have increased the survival rate of the larvae. However, further studies involving transcriptome and western blot analysis are needed to confirm whether microbial supplementation actually induces alterations in carbohydrate metabolism in eel larvae.
Other studies have used microorganisms to increase the digestibility and survival rate of larvae. Kim et al. (2018) used biofloc technology (BFT) involving Bacillus species. They fed larvae with biofloc similar to marine snow and investigated its effects on survival and growth. They found that the growth and survival rates of larvae fed with the BFT diet were lower than those fed with a conventional slurry-type diet. Kim et al. (2018) reported that these results may be due to differences in the composition and content of carbon compounds. Tarnecki et al. (2019) investigated the effect of Bacillus supplementation on common snook (Centropomus undecimalis) larvae. They reported that Bacillus supplementation can enhance the survival rate, but it cannot be accounted for the rapid larval growth (Tarnecki et al., 2019). Similarly, our result revealed that the survival rate of eel larvae increased, but their overall length did not significantly differ. Tarnecki et al. (2019) noted that Bacillus supplementation positively affects the fish immune system, but further studies are needed to identify this protective mechanism.
The gut microbiota composition of fish is influenced by numerous factors, such as habitat, water quality, water temperature, growth stage, and feed (Jang et al., 2021). Changes in microbiota composition can affect fish metabolism and ultimately health (Ye et al., 2011; Semova et al., 2012; Ni et al., 2014). In this study, notable changes in microbial composition at the genus level was observed in Tenacibaculum. For example, T. maritimum is a well-known pathogenic bacterium in wild and farmed marine fish (Pérez-Pascual et al., 2017). However, information about its virulence mechanisms is limited (Avendaño-Herrera et al., 2006). In this study, we could not suggest a clear association between the increased survival rate of eel larvae and increased Tenacibaculum in the microbiota of eel larvae by Bacillus supplementation. However, we found that T. maritimum possesses genes encoding the biosynthesis of exopolysaccharide, protease, and glycoside hydrolase (Pérez-Pascual et al., 2017). This finding suggested that these genes may influence the nutrient digestion and absorption of eel larvae.
Conclusion
Bacillus sp. FEB-1 supplementation could improve the survival rate of artificially produced eel larvae and increase carbohydrate metabolism in eel larvae by changing the microbiota composition. Microbial supplementation might be used to increase survival rate in artificially produced eel larva aquaculture.
Data Availability Statement
The original contributions presented in this study are included in the article/Supplementary Material, further inquiries can be directed to the corresponding authors.
Author Contributions
WJJ: conceptualization, methodology, investigation, data curation, writing original draft, and formal analysis. S-KK: methodology, data curation, and funding acquisition. S-JL and HK: methodology, investigation, and data curation. Y-WR and MGS: conceptualization and funding acquisition. JML: conceptualization, methodology, data curation, and resources. K-BL: conceptualization, methodology, and writing – review and editing. E-WL: conceptualization, methodology, resources, supervision, project administration, funding acquisition, and writing – review and editing. All authors contributed to the article and approved the submitted version.
Funding
This work was financially supported by the grant (R2022009) from the National Institute of Fisheries Science, South Korea.
Conflict of Interest
The authors declare that the research was conducted in the absence of any commercial or financial relationships that could be construed as a potential conflict of interest.
Publisher’s Note
All claims expressed in this article are solely those of the authors and do not necessarily represent those of their affiliated organizations, or those of the publisher, the editors and the reviewers. Any product that may be evaluated in this article, or claim that may be made by its manufacturer, is not guaranteed or endorsed by the publisher.
Acknowledgments
We gratefully acknowledge to Moo-Sang Kim (the Moagen Co., South Korea) for providing useful information on NGS analysis.
Supplementary Material
The Supplementary Material for this article can be found online at: https://www.frontiersin.org/articles/10.3389/fmicb.2022.891070/full#supplementary-material
Footnotes
References
Avendaño-Herrera, R., Toranzo, A. E., and Magariños, B. (2006). Tenacibaculosis infection in marine fish caused by Tenacibaculum maritimum: a review. Dis. Aquat. Org. 71, 255–266. doi: 10.3354/dao071255
Buruiană, C. T., Profir, A. G., and Vizireanu, C. (2014). Effects of probiotic Bacillus species in aquaculture–an overview. Ann. Univ. Dunarea Jos Galati 38, 9–17.
Cha, J. H., Rahimnejad, S., Yang, S. Y., Kim, K. W., and Lee, K. J. (2013). Evaluations of Bacillus spp. as dietary additives on growth performance, innate immunity and disease resistance of olive flounder (Paralichthys olivaceus) against Streptococcus iniae and as water additives. Aquaculture 402, 50–57. doi: 10.1016/j.aquaculture.2013.03.030
Chen, J. Z., Huang, S. L., and Han, Y. S. (2014). Impact of long-term habitat loss on the Japanese eel Anguilla japonica. Estuar. Coast. Shelf Sci. 151, 361–369. doi: 10.1016/j.ecss.2014.06.004
Cowen, J. P., and Holloway, C. F. (1996). Structural and chemical analysis of marine aggregates: in situ macrophotography and laser confocal and electron microscopy. Oceanogr. Lit. Rev. 8:821.
Decho, A. W., and Gutierrez, T. (2017). Microbial extracellular polymeric substances (EPSs) in ocean systems. Front. Microbiol. 8:922. doi: 10.3389/fmicb.2017.00922
Hasan, M. T., Je Jang, W., Lee, J. M., Lee, B. J., Hur, S. W., and Gu Lim, S. (2019). Effects of immunostimulants, prebiotics, probiotics, synbiotics, and potentially immunoreactive feed additives on olive flounder (Paralichthys olivaceus): a review. Rev. Fish. Sci. Aquac. 27, 417–437. doi: 10.1080/23308249.2019.1622510
Hsu, H. Y., Chen, S. H., Cha, Y. R., Tsukamoto, K., Lin, C. Y., and Han, Y. S. (2015). De novo assembly of the whole transcriptome of the wild embryo, preleptocephalus, leptocephalus, and glass eel of Anguilla japonica and deciphering the digestive and absorptive capacities during early development. PLoS One 10:e0139105. doi: 10.1371/journal.pone.0139105
Ishikawa, S., Suzuki, K., Inagaki, T., Watanabe, S., Kimura, Y., Okamura, A., et al. (2001). Spawning time and place of the Japanese eel Anguilla japonica in the North Equatorial Current of the western North Pacific Ocean. Fish. Sci. 67, 1097–1103. doi: 10.1046/j.1444-2906.2001.00366.x
Jang, W. J., Lee, S. J., Jeon, M. H., Kim, T. Y., Lee, J. M., Hasan, M. T., et al. (2021). Characterization of a Bacillus sp. KRF-7 isolated from the intestine of rockfish and effects of dietary supplementation with mannan oligosaccharide in rockfish aquaculture. Fish. Shell. Immunol. 119, 182–192. doi: 10.1016/j.fsi.2021.09.039
Kagawa, H., Tanaka, H., Ohta, H., Unuma, T., and Nomura, K. (2005). The first success of glass eel production in the world: basic biology on fish reproduction advances new applied technology in aquaculture. Fish Physiol. Biochem. 31, 193–199. doi: 10.1007/s10695-006-0024-3
Kim, D. J., Kang, E. J., Bae, J. Y., Park, M. W., and Kim, E. O. (2007). Development of the eggs and pre-leptocephalus Larvae by natural spawning of artificially-matured Japanese eel, Anguilla japonica. J. Aquac. 20, 160–167.
Kim, H. W., Kim, J. H., Kim, M. H., Kim, K. H., Park, J. C., Park, H. G., et al. (2018). Fermented organic matter as possible food for rearing Anguilla japonica Leptocephali. J. Life Sci. 28, 1424–1431. doi: 10.5352/JLS.2018.28.12.1424
Kim, S. K., Lee, B. I., Kim, D. J., and Lee, N. S. (2014). Development of the slurry type diet for the growing leptocephalus, eel larvae (Anguilla japonica). J. Fish. Mar. Sci. Edu. 26, 1209–1216. doi: 10.13000/JFMSE.2014.26.6.1209
Kuebutornye, F. K., Abarike, E. D., and Lu, Y. (2019). A review on the application of Bacillus as probiotics in aquaculture. Fish. Shellfish. Immunol. 87, 820–828. doi: 10.1016/j.fsi.2019.02.010
Miller, M. J., Chikaraishi, Y., Ogawa, N. O., Yamada, Y., Tsukamoto, K., and Ohkouchi, N. (2013). A low trophic position of Japanese eel larvae indicates feeding on marine snow. Biol. Lett. 9:20120826. doi: 10.1098/rsbl.2012.0826
Mochioka, N., and Iwamizu, M. (1996). Diet of anguilloid larvae: leptocephali feed selectively on larvacean houses and fecal pellets. Mar. Biol. 125, 447–452. doi: 10.1007/BF00353257
Nayak, S. K. (2010). Probiotics and immunity: a fish perspective. Fish. Shellfish. Immunol. 29, 2–14. doi: 10.1016/j.fsi.2010.02.017
Ni, J., Yan, Q., Yu, Y., and Zhang, T. (2014). Factors influencing the grass carp gut microbiome and its effect on metabolism. FEMS Microbiol. Ecol. 87, 704–714. doi: 10.1111/1574-6941.12256
Ohta, H., Kagawa, H., Tanaka, H., Okuzawa, K., and Hirose, K. (1996a). Changes in fertilization and hatching rates with time after ovulation induced by 17, 20β-dihydroxy-4-pregnen-3-one in the Japanese eel, Anguilla japonica. Aquaculture 139, 291–301. doi: 10.1016/0044-8486(95)01167-6
Ohta, H., Kagawa, H., Tanaka, H., Okuzawa, K., and Hirose, K. (1996b). Milt production in the Japanese eel Anguilla japonica induced by repeated injections of human chorionic gonadotropin. Fish. Sci. 62, 44–49. doi: 10.2331/fishsci.62.44
Okamoto, T., Kurokawa, T., Gen, K., Murashita, K., Nomura, K., Kim, S. K., et al. (2009). Influence of salinity on morphological deformities in cultured larvae of Japanese eel, Anguilla japonica, at completion of yolk resorption. Aquaculture 293, 113–118. doi: 10.1016/j.aquaculture.2009.04.005
Okamura, A., Horie, N., Mikawa, N., Yamada, Y., and Tsukamoto, K. (2014). Recent advances in artificial production of glass eels for conservation of anguillid eel populations. Ecol. Freshw. Fish 23, 95–110. doi: 10.1111/eff.12086
Otake, T., Nogami, K., and Maruyama, K. (1993). Dissolved and particulate organic matter as possible food sources for eel leptocephali. Mar. Ecol. Prog. Ser. 92, 27–34.
Pérez-Pascual, D., Lunazzi, A., Magdelenat, G., Rouy, Z., Roulet, A., and Lopez-Roques, C. (2017). The complete genome sequence of the fish pathogen Tenacibaculum maritimum provides insights into virulence mechanisms. Front. Microbiol. 8:1542. doi: 10.3389/fmicb.2017.01542
Pfeiler, E. (1991). Glycosaminoglycan composition of anguilliform and elopiform leptocephali. J. Fish Biol. 38, 533–540. doi: 10.1111/j.1095-8649.1991.tb03140.x
Rath, J., and Herndl, G. J. (1994). Characteristics and diversity of β-D-glucosidase (EC 3.2. 1.21) activity in marine snow. Appl. Environ. Microbiol. 60, 807–813. doi: 10.1128/aem.60.3.807-813.1994
Santos, R. A., Oliva-Teles, A., Pousão-Ferreira, P., Jerusik, R., Saavedra, M. J., and Enes, P. (2021). Isolation and characterization of fish-gut Bacillus spp. as source of natural antimicrobial compounds to fight aquaculture bacterial diseases. Mar. Biotechnol. 23, 276–293. doi: 10.1007/s10126-021-10022-x
Semova, I., Carten, J. D., Stombaugh, J., Mackey, L. C., Knight, R., and Farber, S. A. (2012). Microbiota regulate intestinal absorption and metabolism of fatty acids in the zebrafish. Cell Host Microbe 12, 277–288. doi: 10.1016/j.chom.2012.08.003
Shin, M. G., Kim, S. K., Lee, B. I., Choi, Y. H., and Ryu, Y. (2021). Histological development of the digestive system in artificially produced Anguilla japonica larvae. Korean J. Fish. Aquat. Sci. 54, 298–310. doi: 10.5657/KFAS.2021.0298
Skoog, A., Alldredge, A., Passow, U., Dunne, J., and Murray, J. (2008). Neutral aldoses as source indicators for marine snow. Mar. Chem. 108, 195–206. doi: 10.1016/j.marchem.2007.11.008
Tanaka, H., Kagawa, H., and Ohta, H. (2001). Production of leptocephali of Japanese eel (Anguilla japonica) in captivity. Aquaculture 201, 51–60. doi: 10.1016/S0044-8486(01)00553-1
Tanaka, H., Kagawa, H., Ohta, H., Unuma, T., and Nomura, K. (2003). The first production of glass eel in captivity: fish reproductive physiology facilitates great progress in aquaculture. Fish Physiol. Biochem. 28, 493–497. doi: 10.1023/B:FISH.0000030638.56031.ed
Tarnecki, A. M., Wafapoor, M., Phillips, R. N., and Rhody, N. R. (2019). Benefits of a Bacillus probiotic to larval fish survival and transport stress resistance. Sci. Rep. 9:4892. doi: 10.1038/s41598-019-39316-w
Tsukamoto, K. (2014). “Aquaculture production of glass eels as a possible conservation measure for freshwater eels,” in 144th Annual Meeting of the American Fisheries Society. (Bethesda, MD: American fisheries society).
Tsukamoto, K., Aoyama, J., and Miller, M. J. (2009). “Present status of the Japanese eel: resources and recent research,” in Eels at the Edge: American Fisheries Society, Symposium, (Bethesda, MD: American Fisheries Society), 21–35. doi: 10.1186/2193-1801-3-534
Unuma, T., Kondo, S., Tanaka, H., Kagawa, H., Nomura, K., and Ohta, H. (2005). Relationship between egg specific gravity and egg quality in the Japanese eel, Anguilla japonica. Aquaculture 246, 493–500. doi: 10.1016/j.aquaculture.2005.01.011
Van Doan, H., Hoseinifar, S. H., Ringø, E., Ángeles Esteban, M., Dadar, M., and Dawood, M. A. (2020). Host-associated probiotics: a key factor in sustainable aquaculture. Rev. Fish. Sci. Aquac. 28, 16–42. doi: 10.1080/23308249.2019.1643288
Ye, J. D., Wang, K., Li, F. D., and Sun, Y. Z. (2011). Single or combined effects of fructo-and mannan oligosaccharide supplements and Bacillus clausii on the growth, feed utilization, body composition, digestive enzyme activity, innate immune response and lipid metabolism of the Japanese flounder Paralichthys olivaceus. Aquac. Nutr. 17, e902–e911. doi: 10.1111/j.1365-2095.2011.00863.x
Keywords: Anguilla japonica, eel, leptocephalus, survival, microbiota
Citation: Jang WJ, Kim S-K, Lee S-J, Kim H, Ryu Y-W, Shin MG, Lee JM, Lee K-B and Lee E-W (2022) Effect of Bacillus sp. Supplementation Diet on Survival Rate and Microbiota Composition in Artificially Produced Eel Larvae (Anguilla japonica). Front. Microbiol. 13:891070. doi: 10.3389/fmicb.2022.891070
Received: 07 March 2022; Accepted: 16 May 2022;
Published: 10 June 2022.
Edited by:
Catarina Magalhães, University of Porto, PortugalReviewed by:
Wensheng Lan, Technology Center for Animal and Plant Inspection and Quarantine, Shenzhen Customs, ChinaTakashi Yada, Japan Fisheries Research and Education Agency (FRA), Japan
Copyright © 2022 Jang, Kim, Lee, Kim, Ryu, Shin, Lee, Lee and Lee. This is an open-access article distributed under the terms of the Creative Commons Attribution License (CC BY). The use, distribution or reproduction in other forums is permitted, provided the original author(s) and the copyright owner(s) are credited and that the original publication in this journal is cited, in accordance with accepted academic practice. No use, distribution or reproduction is permitted which does not comply with these terms.
*Correspondence: Kyung-Bon Lee, kblee@jnu.ac.kr; Eun-Woo Lee, ewlee@deu.ac.kr
†These authors have contributed equally to this work