- 1School of Chemical Engineering, Yeungnam University, Gyeongsan, South Korea
- 2School of Food & Biological Engineering, Shaanxi University of Science and Technology, Xi'an, China
Fatty acids have diverse functions in the vast majority of cells. At high doses, they act as antimicrobials while, at low doses, they exhibit antibiofilm and antivirulence activities. In this study, the synergistic antibacterial and antibiofilm activities of 30 fatty acids and 11 antibiotics were investigated against methicillin-sensitive and methicillin-resistant Staphylococcus aureus strains. Of the 15 saturated and 15 unsaturated fatty acids examined, 16 enhanced the antibacterial activity of tobramycin. Combinatorial treatment with myristoleic acid (the most active) at 10 μg/ml and tobramycin at 10 μg/ml decreased cell survival by >4 log as compared with tobramycin treatment alone. Notably, aminoglycoside antibiotics, such as tobramycin, kanamycin, gentamicin, and streptomycin exhibited antimicrobial synergy with myristoleic acid. Co-treatment with myristoleic acid and antibiotics markedly decreased biofilm formation. Interestingly, co-treatment with tobramycin and myristoleic acid induced a reduction in S. aureus cell size. These results suggest that fatty acids, particularly myristoleic acid, can be used as aminoglycoside antibiotic adjuvants against recalcitrant S. aureus infections.
Introduction
Fatty acids (FAs), both free and in complex lipids, are ubiquitously distributed in animals, plants, and microbes. FAs are carboxylic acids with a saturated or unsaturated aliphatic chain and have diverse functions in cells, for example, they act as cell membrane structural components, suppliers of energy, and signaling molecules (de Carvalho and Caramujo, 2018). Recently, FAs were suggested to be potential antimicrobial agents at high doses (Desbois and Smith, 2010) and to act as antibiofilm and antivirulence agents at low doses (Kumar et al., 2020). The mechanism responsible for their antimicrobial activities presumably involves cell membrane disruption due to their amphipathic characters (Desbois and Smith, 2010), and the mechanism responsible for their antivirulence activities probably involves interference with bacterial signaling (Kumar et al., 2020). However, details of the molecular mechanisms involved remain to be revealed. Several reports have shown some FAs enhance the antibacterial activities of antibiotics (Hess et al., 2014; Chan et al., 2015; Casillas-Vargas et al., 2021; Kim et al., 2021a). However, no comprehensive study has been undertaken to compare the synergistic antibacterial and antibiofilm activities of a series of FAs.
Staphylococcus aureus is a major pathogen of acute and chronic infections and is responsible for worldwide outbreaks of nosocomial infections (Lowy, 1998). Staphylococcus aureus often exhibits antibiotic resistance, and its biofilms on medical devices and host surfaces play a critical role in antibiotic tolerance (Stewart and Costerton, 2001; Seo et al., 2021). Lauric acid (Hess et al., 2014), linoleic and oleic acids (Chan et al., 2015), and myristic acid (Kim et al., 2021b) have been reported to act synergistically with antibiotics to enhance antibacterial efficacy against S. aureus. Therefore, we investigated the effects of FAs and antibiotic co-treatments on the antibacterial and antibiofilm activities of S. aureus strains.
In this study, 30 natural FAs (two short-chain, seven medium-chain, 16 long-chain, and five very long-chain) were initially screened for their bactericidal activities when co-administered with tobramycin against S. aureus. The synergistic antibacterial and antibiofilm activities of the most active FA, myristoleic acid, were confirmed in combination with four aminoglycoside antibiotics, namely, tobramycin, kanamycin, gentamicin, and streptomycin. Conventional microscopy and scanning electron microscopy (SEM) were used to observe changes in biofilm formation by S. aureus.
Materials and Methods
Strains, Chemicals, and Culture Materials
In this study, we used methicillin-sensitive S. aureus (MSSA; ATCC 6538) and methicillin-resistant S. aureus (MRSA; MW2) strains. All experiments were performed at 37°C in Luria-Bertani medium (Silber et al., 2020). Thirty FAs, namely butanoic acid (C4:0), pentanoic acid (C5:0), hexanoic acid (C6:0), heptanoic acid (C7:0), octanoic acid (C8:0), nonanoic acid (C9:0), decanoic acid (C10:0), undecanoic acid (C11:0), lauric acid (C12:0), myristic acid (C14:0), myristoleic acid (C14:1), palmitic acid (C16:0), palmitoleic acid (C16:1 ω-5), heptadecanoic acid (C17:0), stearic acid (C18:0), vaccenic acid (C18:1 ω-7), oleic acid (C18:1 ω-9, cis), elaidic acid (C 18:1 ω-9, trans), petroselinic acid (C18:1 ω-12), linoleic acid (C18:2 ω-6), conjugated linoleic acid (C18:2 ω-6), α-linolenic acid (C18:3 ω-3), γ-linolenic acid (C18:3 ω-6), arachidonic acid (C20:4 ω-6), eicosapentaenoic acid (C20:5 ω-3), behenic acid (C22:0), erucic acid (C22:1 ω-9), docosahexaenoic acid (C22:6 ω-3), tricosanoic acid (C23:0), and nervonic acid (C24:1 ω-9) were purchased from Sigma-Aldrich (St. Louis, United States) or TCI Co. (Tokyo, Japan). All FAs except butanoic acid were dissolved in dimethyl sulfoxide (DMSO); butanoic acid was dissolved in water. DMSO at 0.1% v/v, which had no effect on bacterial growth or biofilm formation, was used as a control. Antibiotics viz. tobramycin, kanamycin, streptomycin, amoxicillin, vancomycin, tetracycline, erythromycin, rifampicin, gentamicin, ciprofloxacin, and methicillin were obtained from Sigma-Aldrich.
Planktonic cell growths and turbidities were measured at 600 nm using an Optizen 2120 UV spectrophotometer (Mecasys Co. Ltd., Daejeon, Korea). MIC was defined as the lowest concentration that visually inhibited the growth of planktonic cells, and MICs were also confirmed by colony counting and droplet assay modified from previous publication (Sieuwerts et al., 2008).
Co-treatments With Fatty Acids and Antimicrobial Agents
The combinatorial efficacies of FAs and antimicrobial agents were analyzed using the two S. aureus strains. Briefly, overnight cultures at an initial turbidity of OD 0.05 at 600 nm were inoculated into proper culture media (final volume 1,000 μl) with antibiotics and/or FAs. Cells were incubated with shaking for 1 h at 37°C, and cell mixtures were diluted serially in PBS (phosphate-buffered saline) and plated on LB agar plates. CFUs were determined by colony counting after incubation for 24 h at 37°C. Experiments were performed using two independent cultures in quadruplicate.
Crystal Violet Biofilm Assay
Biofilm formation by the two S. aureus strains was quantified in 96-well polystyrene plates (SPL Life Sciences, Korea), as previously described (Lee et al., 2019). Briefly, cells were inoculated into LB broth (300 μl) at an initial turbidity of 0.05 (3.5 × 109 CFU/ml) at 600 nm and FAs were added at different concentrations and incubated for 24 h at 37°C in a static state. To quantify biofilm formation, cell cultures were rinsed three times with water (300 μl), and then biofilms were tinted with 0.1% crystal violet (300 μl) for 20 min, and dissolved in 300 μl of 95% ethanol. Absorbances were measured at 570 nm (OD570) using a Multiskan EX microplate reader (Thermo Fisher Scientific, Waltham, USA). Static biofilm formation results are the averages of six replicate wells of two representative independent experiments.
Biofilm Observations by Live Imaging Microscopy and SEM
To analyze biofilms, they were produced, as mentioned above, over 24 h at 37°C. After incubation, planktonic cells were discarded by gentle washing with distilled water three times and biofilms were analyzed by live imaging microscopy using the iRiS™ Digital Cell Imaging System (Logos BioSystems, Anyang, Korea) at different magnifications. Biofilm images were regenerated as color-coded 2D and 3D pictures using ImageJ.1 Staphylococcus aureus biofilms on nylon filter membranes were also examined by SEM, as previously described (Kim et al., 2016). Briefly, a nylon filter membrane (Merck Millipore, Burlington, United States) was cut into 0.4 cm × 0.4 cm pieces, autoclaved, and placed in each well of 96-well plates containing cell culture and incubated with or without myristoleic acid and/or tobramycin for 24 h at 37°C. Biofilm cells on nylon membranes were washed with PBS, fixed with glutaraldehyde (2.5%) and formaldehyde (2%) for 24 h, post-fixed with OsO4 (Osmium tetroxide), and dehydrated using an ethanol series (50%, 70%, 80%, 90%, 95%, and 100%) and isoamyl acetate. After critical-point drying and sputter coating, cells on membranes were imaged using an S-4800 scanning electron microscope (Hitachi, Tokyo, Japan) at 15 kV.
Statistical Analysis
Replication numbers for assays are provided above and results are expressed as means ± standard deviations. The statistical analysis was performed by one-way ANOVA followed by Dunnett’s test using SPSS Ver. 23 (SPSS Inc., Chicago, United States). The values of p < 0.05 were considered as significant, and asterisks indicate significant differences between treated and untreated samples.
Results
Combinatorial Antimicrobial Activities of Fatty Acids
The combinatorial antimicrobial activities of the 30 FAs (15 saturated and 15 unsaturated FAs) at 10 μg/ml were initially investigated against a methicillin-sensitive S. aureus strain (MSSA; ATCC 6538) in combination with the aminoglycoside tobramycin. Tobramycin treatment alone at a concentration of 10 μg/ml in 1 h decreased cell survival by 92%, whereas treatment with any of the FAs alone at the same concentration did not affect cell survival (Figure 1). Interestingly, co-treatment with tobramycin and FAs showed some synergistic antimicrobial effects with widely different efficiencies. Sixteen FAs, namely pentanoic (C5:0), heptanoic (C6:0), undecanoic (C11:0), lauric (C12:0), myristic (C14:0), myristoleic (C14:1), palmitoleic (C16:1 ω-5), oleic (C18:1 ω-9, cis), elaidic (C18:1 ω-9, trans), linoleic (C18:2 ω-6), conjugated linoleic (C18:2 ω-6), α-linolenic (C18:3 ω-3), γ-linolenic (C18:3 ω-6), arachidonic (C20:4 ω-6), erucic (C22:1 ω-9), and docosahexaenoic acid (C22:6 ω-3) significantly decreased cell survival as compared with tobramycin treatment alone (Figure 1A). Most notably, myristoleic acid plus tobramycin decreased cell survival by >5 log as compared with 1 log reduction by tobramycin alone. The synergistic antimicrobial efficacies of the six active FAs, namely, undecanoic, lauric, myristic, myristoleic, palmitoleic, and arachidonic acid, were also observed on a methicillin-resistant S. aureus strain (MRSA; MW2) and reduced cell survival by >1–3 log vs. tobramycin alone (Figure 1B). Hence, in subsequent studies, we focused on the effects of the most active FA, myristoleic acid, on both S. aureus strains.
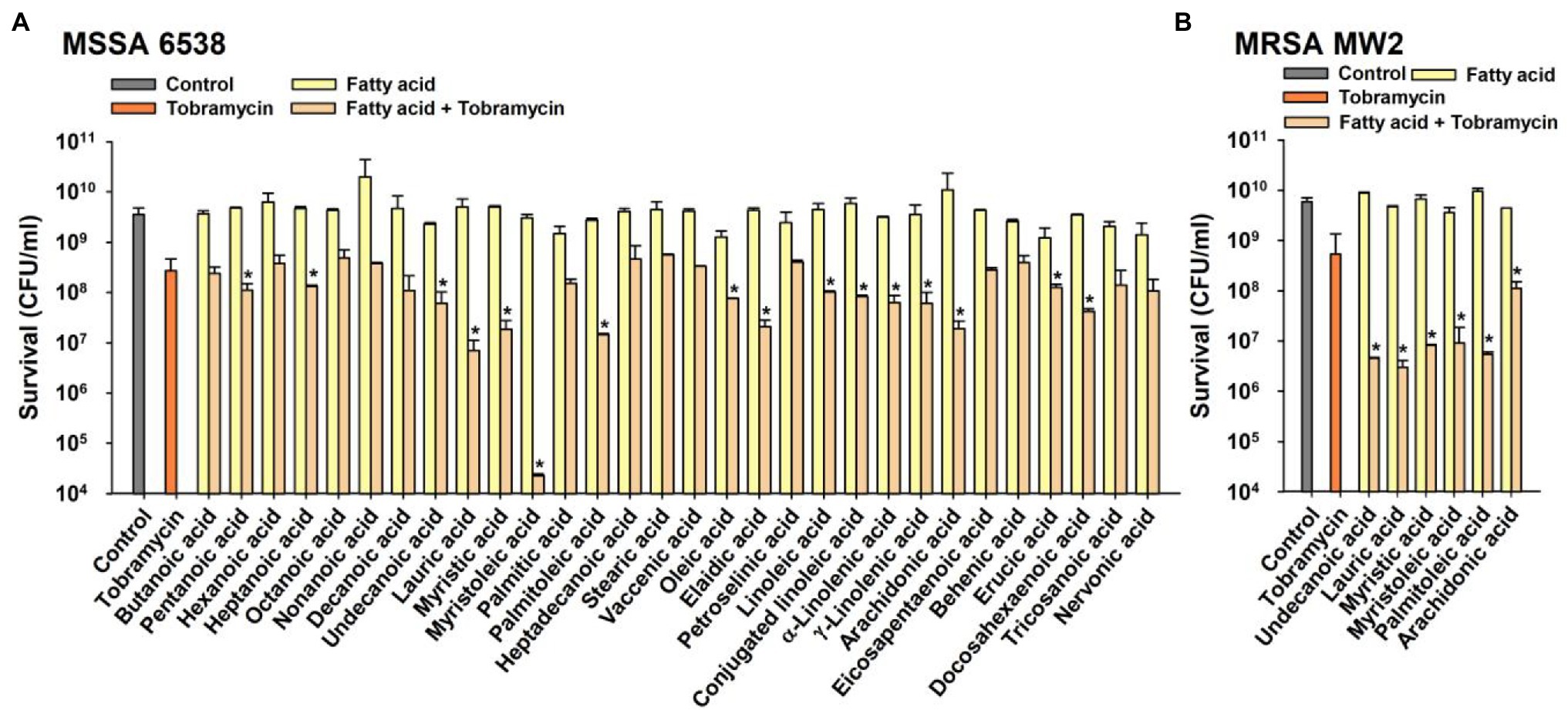
Figure 1. Combinatorial antimicrobial activities of fatty acids and tobramycin against Staphylococcus aureus. Cell survivals were measured after treating MSSA 6538 (A) or MRSA MW2 (B) with tobramycin (10 μg/ml) and/or a FA (10 μg/ml) for 1 h. *p < 0.05 vs. tobramycin-treated controls. Control; untreated control.
The MICs of the 16 active FAs against MSSA ATCC 6538 are shown in Table 1. While the MICs of most FAs were > 400 μg/ml, the MICs of undecanoic, myristoleic, and linoleic acid were 200, 100, and 200 μg/ml, respectively. These results suggest that the antibacterial synergistic effect of FAs at 10 μg/ml was due to synergism between antibiotics and FAs but not to their antimicrobial activities.
Antibacterial Synergy Between Myristoleic Acid and Aminoglycosides
To investigate which antibiotics, exhibit synergism with FAs, S. aureus was treated with 10 common antibiotics, namely kanamycin, streptomycin, amoxicillin, vancomycin, tetracycline, erythromycin, rifampicin, gentamicin, ciprofloxacin, and methicillin up to 200 μg/ml for 1 h. Three aminoglycosides, namely, kanamycin, gentamicin, and streptomycin significantly decreased cell survival, but the other seven antibiotics did not, indicating that these three had bactericidal effects (Supplementary Figure 1). Interestingly, unlike other antibiotics, tobramycin, kanamycin, gentamicin, and streptomycin are all aminoglycoside antibiotics.
As was expected, myristoleic acid in combination with kanamycin, gentamicin, or streptomycin dose-dependently decreased cell survival as compared with tobramycin treatment alone (Figure 2). For example, myristoleic acid (10 μg/ml) plus kanamycin (50 or 100 μg/ml) or gentamicin (5 or 10 μg/ml) decreased cell survival by >2 log while the synergistic effect of myristoleic acid was less when administered with streptomycin.
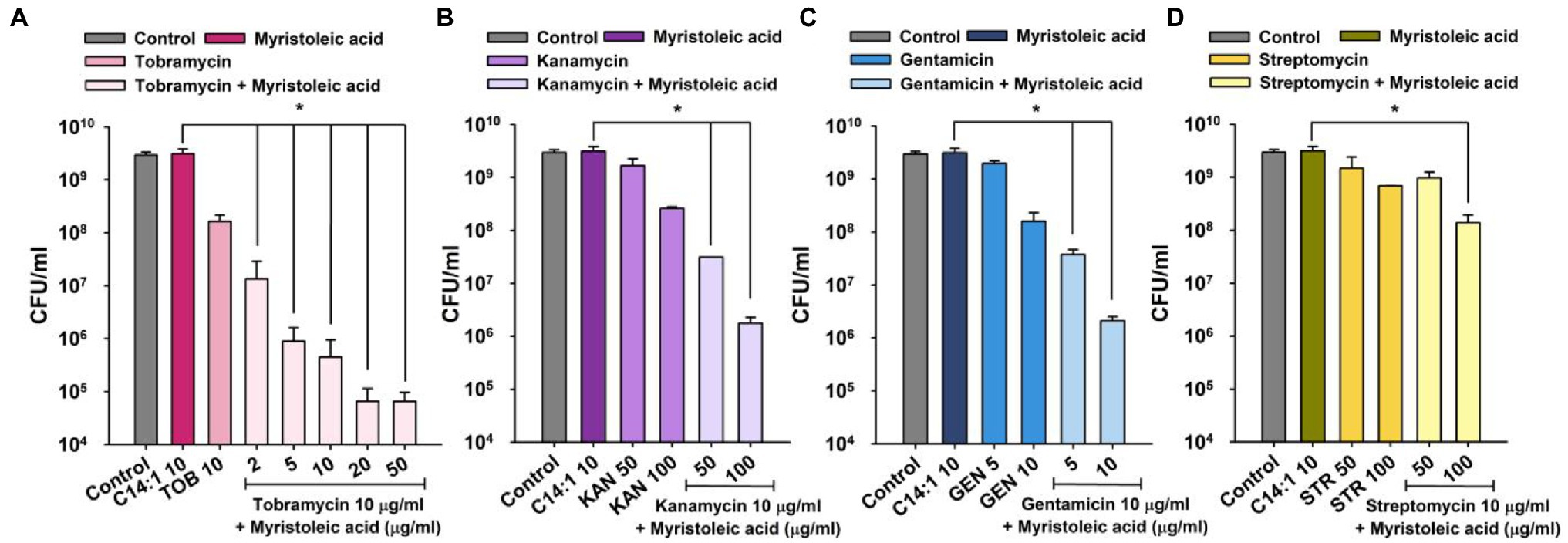
Figure 2. Synergistic antimicrobial activities of myristoleic acid and aminoglycoside antibiotics against Staphylococcus aureus. Cell survivals were measured 1 h after treating MSSA 6538 with myristoleic acid (10 μg/ml) and/or tobramycin (A), kanamycin (B), gentamicin (C), or streptomycin (D). *p < 0.05 vs. myristoleic acid-treated controls. TOB; tobramycin, KAN; kanamycin, GEN; gentamicin, STR; streptomycin, C14:1; myristoleic acid, Control; untreated control.
Synergistic Antibiofilm Effects of Myristoleic Acid and Aminoglycoside Antibiotics
Staphylococcus aureus biofilm formation plays important roles in antimicrobial resistance and a variety of device-related infections (Stewart and Costerton, 2001), and sub-inhibitory concentrations of aminoglycosides often induce biofilm formation (Hoffman et al., 2005). Hence, we investigated the antibiofilm activities of tobramycin, kanamycin, gentamicin, and streptomycin alone and in combination with myristoleic acid. Treatment of MSSA ATCC 6538 with myristoleic acid alone dose-dependently reduced biofilm formation (Figure 3A), which is in agreement with a recent study which reported that myristoleic acid inhibited biofilm formation by Cutibacterium acnes alone or with S. aureus (Kim et al., 2021a). Low concentrations of myristoleic acid, tobramycin, and streptomycin slightly increased biofilm formation by S. aureus, whereas high doses decreased biofilm formation (Figure 3A). For example, streptomycin at 1 or 10 μg/ml increased S. aureus biofilm formation by 22% and 26%, respectively (Figure 3A).
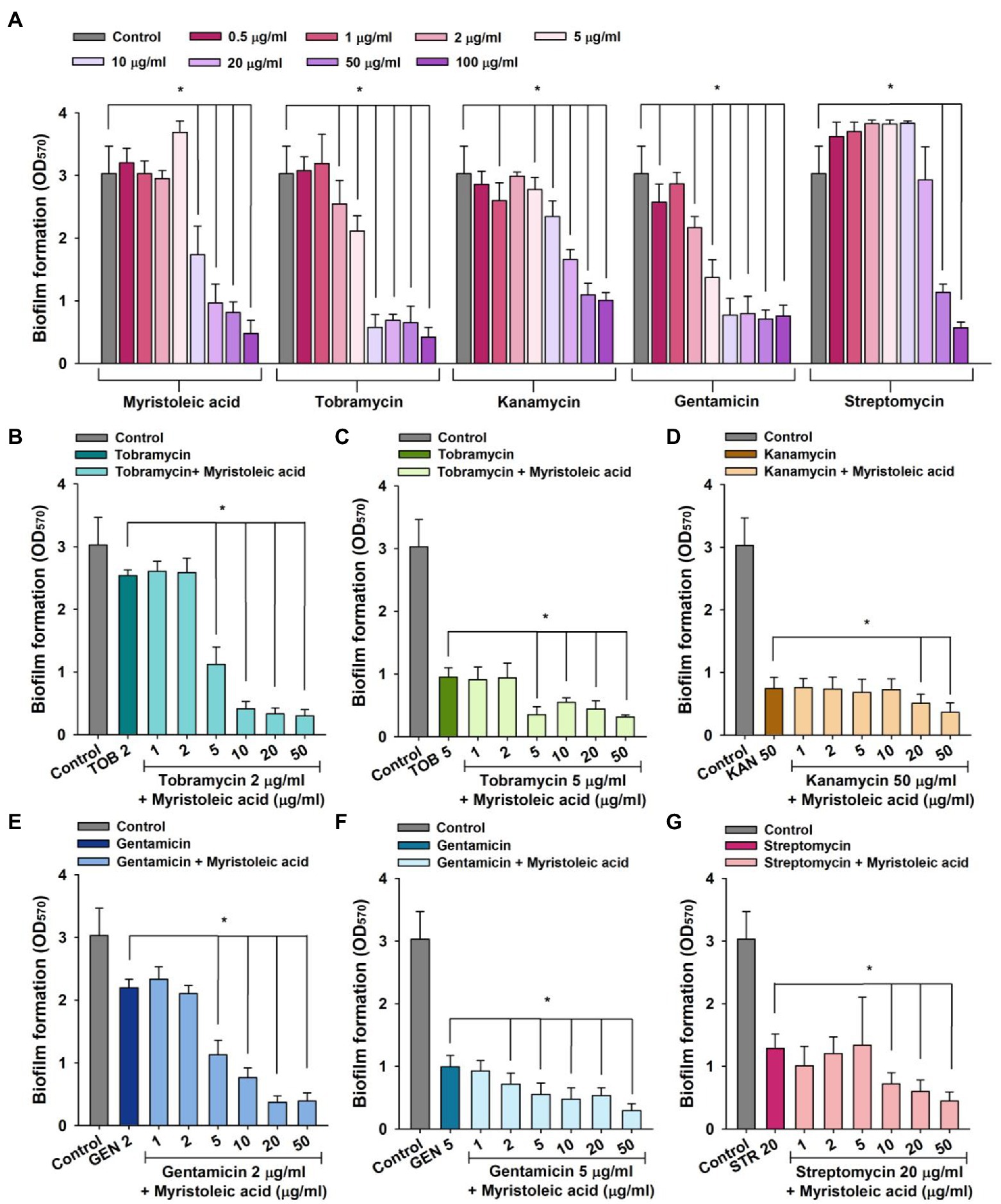
Figure 3. Staphylococcus aureus biofilm inhibition by antibiotics in the presence or absence of fatty acids. Biofilm inhibitions by myristoleic acid, tobramycin, kanamycin, gentamicin, or streptomycin (A) were determined after incubation for 24 h in 96-well plates at 37°C under static conditions. Combinatorial antibiofilm activities of tobramycin (B,C), kanamycin (D), gentamicin (E,F), or streptomycin (G) in the presence of myristoleic acid were measured after incubation under identical conditions. Error bars indicate SD. *p < 0.05 vs. untreated controls (A). *p < 0.05 vs. antibiotic-treated controls (B–G). TOB; tobramycin, KAN; kanamycin, GEN; gentamicin, STR; streptomycin, Control; untreated control.
To study the antibiofilm efficacies of myristoleic acid and antibiotic co-treatments, we administered antibiotics at concentrations that reduced biofilm formation by 16 to 75%. For example, tobramycin at 2 or 5 μg/ml, kanamycin at 50 μg/ml, gentamicin at 2 or 5 μg/ml, and streptomycin at 20 μg/ml were subjected to combinatorial assays.
Co-treatment with myristoleic acid and antibiotics enhanced antibiofilm activities. For example, tobramycin alone at 2 μg/ml reduced biofilm formation by 16%, whereas treatment with tobramycin (2 μg/ml) and myristoleic acid (5 μg/ml) reduced biofilm formation by 63% (Figure 3B). Similar results were obtained for tobramycin at 5 μg/ml (Figure 3C). Gentamicin alone at 2 μg/ml reduced biofilm formation by 28% and treatment with gentamicin (2 μg/ml) and myristoleic acid (5 μg/ml) reduced biofilm formation by 63% (Figure 3E). Similar results were also obtained for gentamicin at 5 ug/ml (Figure 3F). However, myristoleic acid had a smaller synergistic effect on the antibiofilm activities of kanamycin (Figure 3D) and streptomycin (Figure 3G). Fractional inhibitory concentration indices (FICI) for the antibiofilm activity were calculated to check the synergistic effects of myristoleic acid/antibiotic combinations. FICI values of tobramycin, gentamicin, kanamycin, and streptomycin were 0.2–0.3, 0.3–0.52, 1.5, and 0.6, respectively. Hence, combinatory effects were synergistic for tobramycin and gentamicin, additive for streptomycin, and indifferent for kanamycin.
In addition, combinatory antibiofilm efficacy trends of myristoleic acid and antibiotics against MRSA MW2 and MSSA ATCC 6538 were found to be similar, although MRSA MW2 produced less biofilm than MSSA ATCC 6538 (Figure 4).
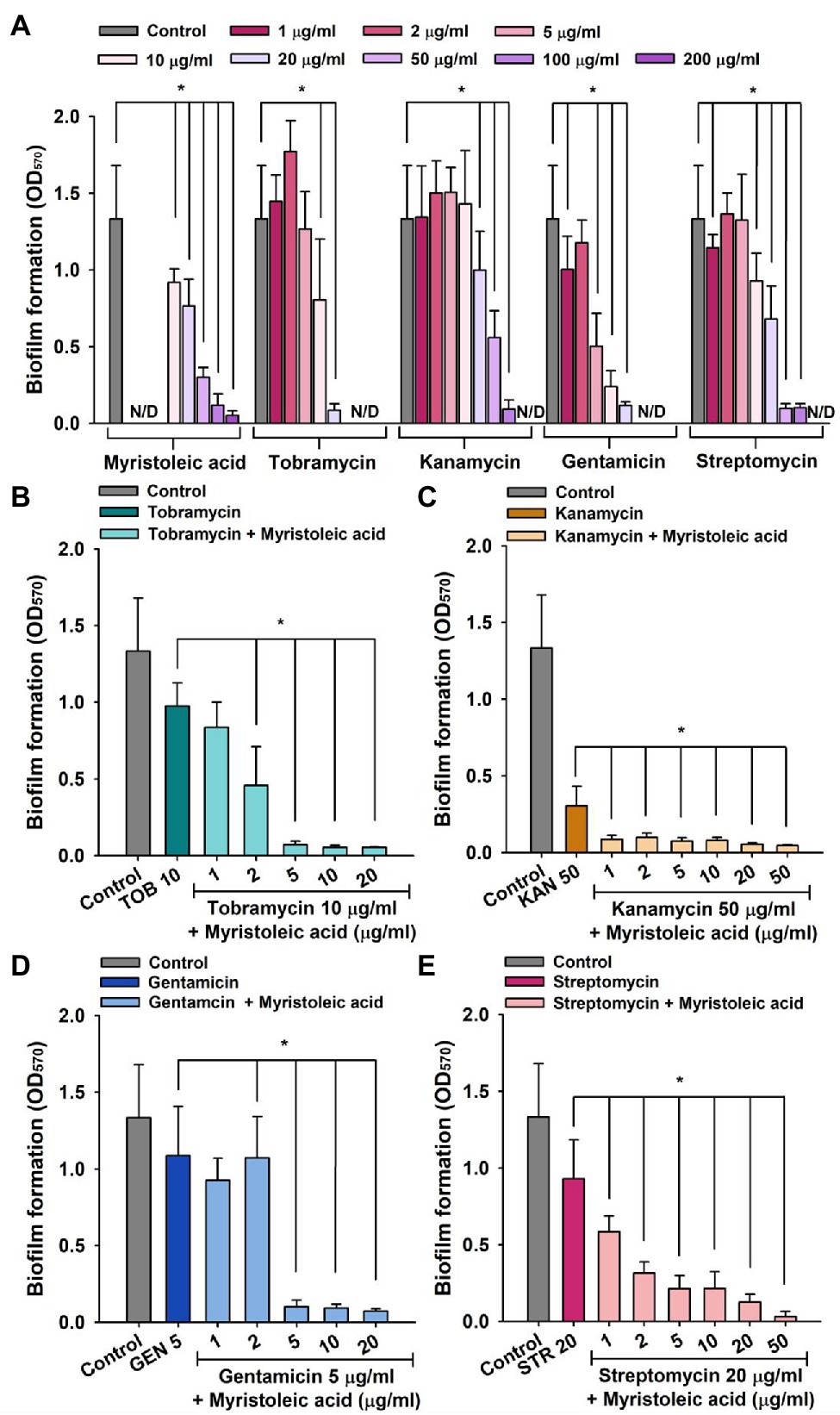
Figure 4. MRSA biofilm inhibition by antibiotics in the presence or absence of fatty acid. Biofilm inhibitions by myristoleic acid, tobramycin, kanamycin, gentamicin, and streptomycin (A) were measured after incubating in 96-well plates for 24 h at 37°C under static conditions. Antibiofilm activities of tobramycin (B), kanamycin (C), gentamicin (D), or streptomycin (E) plus myristoleic acid were measured after incubation under identical conditions. Error bars indicate standard deviations. *p < 0.05 vs. untreated controls (A). *p < 0.05 vs. antibiotic-treated controls (B–E). TOB; tobramycin, KAN; kanamycin, GEN; gentamicin, STR; streptomycin, Control; untreated control. N/D indicates “Not Determined.”
Microscopic Observations of Staphylococcus aureus Biofilm Inhibition by Myristoleic Acid and/or Tobramycin
Biofilm inhibition was analyzed using a live cell imaging system and by SEM. 3-D color mesh plots showed that tobramycin at 5 μg/ml or myristoleic acid at 20 μg/ml inhibited biofilm formation by S. aureus, but that in combination they had a markedly greater effect (Figure 5A). SEM analysis confirmed the inhibitory effect of myristoleic acid along with tobramycin and also showed the shape of S. aureus biofilm cells on the surface of nylon filter membrane. Treatment of myristoleic acid, tobramycin, or their combination markedly reduced the numbers of S. aureus cells. Tobramycin at 5 μg/ml or myristoleic acid at 20 μg/ml did not cause any surface defects or membrane damages. Interestingly, tobramycin or myristoleic acid produced few small cells, but in combination they produced many smaller cells (Figure 5B). Taken together, myristoleic acid co-treatment enhanced the antibiofilm effects of antibiotics on S. aureus.
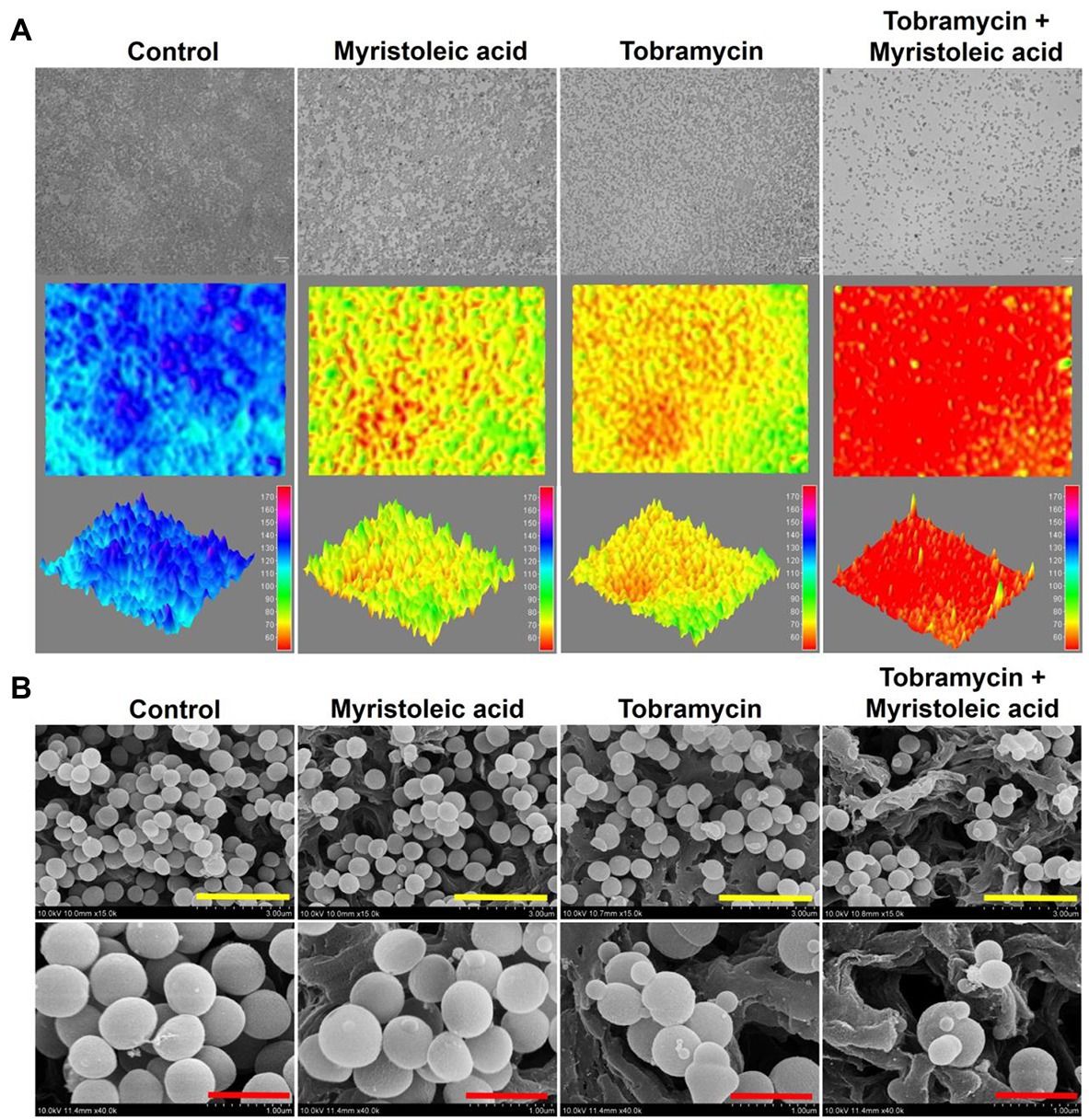
Figure 5. Biofilm cells of Staphylococcus aureus grown in the presence or absence of myristoleic acid and/or tobramycin. Biofilm inhibitions by myristoleic acid (20 μg/ml) and/or tobramycin (5 μg/ml) were determined after culture for 24 h under static conditions. Re-created color-coded 2D and 3D images of Staphylococcus aureus biofilms (A). SEM images of Staphylococcus aureus biofilms formed in the presence or absence of myristoleic acid (20 μg/ml) and/or tobramycin (5 μg/ml; B). Yellow and red scale bars represent 3 and 1 μm, respectively. Control; untreated control.
Discussion
Since penicillin was discovered in 1940, various antibiotics have been used as treatments of choice to treat infectious diseases. However, the overuse of antibiotics has caused the worldwide emergence of drug-resistant pathogens. Also, bacterial biofilms are tolerant of conventional antimicrobial therapeutics and the host immune system. Hence, alternative approaches are needed to control drug resistance and biofilm formation, and antibiotic adjuvants provide one such alternative. This study presents that several FAs, particularly myristoleic acid, enhance the bactericidal activities of four aminoglycoside antibiotics, namely, kanamycin, gentamicin, streptomycin, and tobramycin against S. aureus and that combinatory treatment of myristoleic acid and these antibiotics significantly decreases biofilm formation by S. aureus, which suggests the possible use of myristoleic acid as an antibiotic adjuvant.
Antibiotic adjuvants include: (1) anti-resistance drugs such as β-lactamase inhibitors pump inhibitors, membrane permeabilizers, and biofilm inhibitors; (2) antivirulence drugs, such as quorum-sensing inhibitors, toxin inhibitors, and secretion system inhibitors; (3) host-directed therapies such as innate immune system agonists and immunomodulatory peptides; and (4) other therapies such as phage, probiotic, and oral rehydration therapies (Gill et al., 2015). Various synthetic and natural compounds such as prazolopyridazine derivatives (Schaenzer et al., 2018), isoflavonoids (Abreu et al., 2017), plant extracts (Abreu et al., 2016), cationic peptides (Geitani et al., 2019), cannabidiol (Wassmann et al., 2022), marine bisindole alkaloid (Campana et al., 2020), cinnamonitrile (Speri et al., 2019), amine oxide alkyl derivatives (Fagnani et al., 2021), and brominated carbazoles (Berndsen et al., 2022) have been recently proposed to be used as antibiotic adjuvants against S. aureus strains.
The FAs identified in this study are potential anti-resistance agents since they enhanced antibiotic efficacy and inhibited biofilm formation. Recent studies have suggested that FAs could be used as antibiotic adjuvants (Kim et al., 2018; Kumar et al., 2020; Yuyama et al., 2020). In particular, several FAs, such as lauric acid plus gentamicin or streptomycin (but not ampicillin or vancomycin; Hess et al., 2014), linoleic or oleic acids with erythromycin (Chan et al., 2015), and lauric or myristic acids with the aminoglycosides gentamicin, kanamycin, or tobramycin (Kim et al., 2021b) showed synergistic antibacterial efficacy. However, the action mechanisms responsible for this antibacterial synergy conferred by FAs are unknown. It has long been reported that FAs at high concentrations have antimicrobial activities, probably because they alter cell membrane permeability, disrupt membranes, and cause leakage of intracellular metabolites (Desbois and Smith, 2010). However, a few reasonable mechanisms have been suggested to explain the antibacterial synergistic effects of FAs. In particular, it was suggested that the synergistic antibacterial activities of linoleic and oleic acids on the activity of the macrolide erythromycin were probably due to efflux MsrA pump interference in S. aureus (Chan et al., 2015). In another study, it was speculated that the synergistic antibiofilm effect of lauric acid on the antibiofilm effects of gentamicin and streptomycin were due to the facilitation of biofilm matrix penetration due to surfactant effects (Hess et al., 2014).
Interestingly, in the present study, myristoleic acid alone at low doses did not exhibit antibacterial activity but had synergistic effects on the bactericidal activities of four aminoglycosides (Figure 2). Although speculative, myristoleic acid, like linoleic and oleic acids, may target efflux MsrA pump and possibly interfere with protein synthesis via the 30S ribosomal subunit, which is the action mechanism of aminoglycosides. Intriguingly, small size S. aureus cells were produced by treating S. aureus with tobramycin and/or myristoleic acid (Figure 5). Furthermore, it was mentioned in a previous report that small S. aureus cells were produced by treatment with the antibiotic nitrofuran (Koike et al., 1974), whereas long or large cells were often observed after the inhibition of cell division via the major cell division protein FtsZ (Silber et al., 2020). Hence, it is possible that low doses of antibiotics plus myristoleic acid disturb the regulation of cell division in S. aureus. In addition, we suggest that since aminoglycosides exhibit bactericidal activity against most Gram-negative bacilli, our findings may be applicable to other Gram-negative bacteria. Recently, several FAs were reported to exhibit antibiofilm activity (Kim et al., 2018; Kumar et al., 2020; Yuyama et al., 2020). In. S. aureus, oleic acid, cis-11-eicosenoic acid, and two long chain omega-3 FAs inhibited S. aureus biofilm formation and hemolytic activity by suppressing the alpha-hemolysin hla gene (Lee et al., 2017; Kim et al., 2018). Kim et al. reported myristoleic acid inhibited the formations of C. acnes and S. aureus biofilms and mixed C. acnes/S. aureus biofilms, and that lauric and myristic acids inhibited polymicrobial biofilm formation by S. aureus, Escherichia coli O157:H7, and Candida albicans (Kim et al., 2021a). These observations indicate that FAs, such as myristoleic acid, could be used as antibiotic adjuvants with antibiotics to target many species of microbes.
Data Availability Statement
The original contributions presented in the study are included in the article/Supplementary Material, further inquiries can be directed to the corresponding author.
Author Contributions
SP and JL: conceptualization, data curation, writing of the manuscript, and visualization. SP, J-HL, and Y-GK: methodology, validation, formal analysis, and investigation. SP and J-HL: software. JL: resources. J-HL and JL: project administration. All authors contributed to the article and approved the submitted version.
Funding
This research was supported by the Basic Science Research Program of the National Research Foundation of Korea (NRF) funded by the Ministry of Education (2021R1I1A3A04037486 to J-HL), the NRF funded by the Korea government (MSIT; 2021R1A2C1008368), and by the Priority Research Center Program of the NRF funded by the Ministry of Education (2014R1A6A1031189).
Conflict of Interest
The authors declare that the research was conducted in the absence of any commercial or financial relationships that could be construed as a potential conflict of interest.
Publisher’s Note
All claims expressed in this article are solely those of the authors and do not necessarily represent those of their affiliated organizations, or those of the publisher, the editors and the reviewers. Any product that may be evaluated in this article, or claim that may be made by its manufacturer, is not guaranteed or endorsed by the publisher.
Supplementary Material
The Supplementary Material for this article can be found online at: https://www.frontiersin.org/articles/10.3389/fmicb.2022.876932/full#supplementary-material
Footnotes
References
Abreu, A. C., Coqueiro, A., Sultan, A. R., Lemmens, N., Kim, H. K., Verpoorte, R., et al. (2017). Looking to nature for a new concept in antimicrobial treatments: isoflavonoids from Cytisus striatus as antibiotic adjuvants against MRSA. Sci. Rep. 7:3777. doi: 10.1038/s41598-017-03716-7
Abreu, A., Paulet, D., Coqueiro, A., Malheiro, J., Borges, A., Saavedra, M., et al. (2016). Antibiotic adjuvants from Buxus sempervirens to promote effective treatment of drug-resistant Staphylococcus aureus biofilms. RCS Advances 6, 95000–95009. doi: 10.1039/C6RA21137B
Berndsen, R., Cunningham, T., Kaelin, L., Callender, M., Boldog, W. D., Viering, B., et al. (2022). Identification and evaluation of brominated carbazoles as a novel antibiotic adjuvant scaffold in MRSA. ACS Med. Chem. Lett. 13, 483–491. doi: 10.1021/acsmedchemlett.1c00680
Campana, R., Mangiaterra, G., Tiboni, M., Frangipani, E., Biavasco, F., Lucarini, S., et al. (2020). A fluorinated analogue of marine bisindole alkaloid 2,2-Bis(6-bromo-1H-indol-3-yl)ethanamine as potential anti-biofilm agent and antibiotic adjuvant against Staphylococcus aureus. Pharmaceuticals 13:210. doi: 10.3390/ph13090210
Casillas-Vargas, G., Ocasio-Malavé, C., Medina, S., Morales-Guzmán, C., Del Valle, R. G., Carballeira, N. M., et al. (2021). Antibacterial fatty acids: An update of possible mechanisms of action and implications in the development of the next-generation of antibacterial agents. Prog. Lipid Res. 82:101093. doi: 10.1016/j.plipres.2021.101093
Chan, B. C., Han, X. Q., Lui, S. L., Wong, C. W., Wang, T. B., Cheung, D. W., et al. (2015). Combating against methicillin-resistant Staphylococcus aureus - two fatty acids from Purslane (Portulaca oleracea L.) exhibit synergistic effects with erythromycin. J. Pharm. Pharmacol. 67, 107–116. doi: 10.1111/jphp.12315
de Carvalho, C., and Caramujo, M. J. (2018). The various roles of fatty acids. Molecules 23:2583. doi: 10.3390/molecules23102583
Desbois, A. P., and Smith, V. J. (2010). Antibacterial free fatty acids: activities, mechanisms of action and biotechnological potential. Appl. Microbiol. Biotechnol. 85, 1629–1642. doi: 10.1007/s00253-009-2355-3
Fagnani, L., Nazzicone, L., Brisdelli, F., Giansanti, L., Battista, S., Iorio, R., et al. (2021). Cyclic and acyclic amine oxide alkyl derivatives as potential adjuvants in antimicrobial chemotherapy against methicillin-resistant Staphylococcus aureus with an MDR profile. Antibiotics 10:952. doi: 10.3390/antibiotics10080952
Geitani, R., Ayoub Moubareck, C., Touqui, L., and Karam Sarkis, D. (2019). Cationic antimicrobial peptides: alternatives and/or adjuvants to antibiotics active against methicillin-resistant Staphylococcus aureus and multidrug-resistant Pseudomonas aeruginosa. BMC Microbiol. 19:54. doi: 10.1186/s12866-019-1416-8
Gill, E. E., Franco, O. L., and Hancock, R. E. (2015). Antibiotic adjuvants: diverse strategies for controlling drug-resistant pathogens. Chem. Biol. Drug Des. 85, 56–78. doi: 10.1111/cbdd.12478
Hess, D. J., Henry-Stanley, M. J., and Wells, C. L. (2014). Antibacterial synergy of glycerol monolaurate and aminoglycosides in Staphylococcus aureus biofilms. Antimicrob. Agents Chemother. 58, 6970–6973. doi: 10.1128/aac.03672-14
Hoffman, L. R., D'Argenio, D. A., MacCoss, M. J., Zhang, Z., Jones, R. A., and Miller, S. I. (2005). Aminoglycoside antibiotics induce bacterial biofilm formation. Nature 436, 1171–1175. doi: 10.1038/nature03912
Kim, Y.-G., Lee, J.-H., Gwon, G., Kim, S.-I., Park, J. G., and Lee, J. (2016). Essential oils and eugenols inhibit biofilm formation and the virulence of Escherichia coli O157:H7. Sci. Rep. 6:36377. doi: 10.1038/srep36377
Kim, Y.-G., Lee, J.-H., and Lee, J. (2021a). Antibiofilm activities of fatty acids including myristoleic acid against Cutibacterium acnes via reduced cell hydrophobicity. Phytomedicine 91:153710. doi: 10.1016/j.phymed.2021.153710
Kim, Y.-G., Lee, J.-H., Park, S., and Kim, S. (2021b). Inhibition of polymicrobial biofilm formation by saw palmetto oil, lauric acid and myristic acid. Microb. Biotechnol. 15:Lee, J., 590–602. doi: 10.1111/1751-7915.13864
Kim, Y.-G., Lee, J.-H., Raorane, C. J., Oh, S. T., Park, J. G., and Lee, J. (2018). Herring oil and omega fatty acids inhibit Staphylococcus aureus biofilm formation and virulence. Front. Microbiol. 9:1241. doi: 10.3389/fmicb.2018.01241
Koike, M., Iida, K., Nakashima, K., and Tokunaga, J. (1974). Small-cell formation of Staphylococcus aureus by subinhibitory concentrations of nitrofuran derivatives. J. Bacteriol. 120, 524–526. doi: 10.1128/jb.120.1.524-526.1974
Kumar, P., Lee, J.-H., Beyenal, H., and Lee, J. (2020). Fatty acids as antibiofilm and antivirulence agents. Trends Microbiol. 28, 753–768. doi: 10.1016/j.tim.2020.03.014
Lee, J.-H., Kim, Y.-G., Khadke, S. K., Yamano, A., Watanabe, A., and Lee, J. (2019). Inhibition of biofilm formation by Candida albicans and polymicrobial microorganisms by nepodin via hyphal-growth suppression. ACS Infect. Dis. 5, 1177–1187. doi: 10.1021/acsinfecdis.9b00033
Lee, J.-H., Kim, Y.-G., Park, J. G., and Lee, J. (2017). Supercritical fluid extracts of Moringa oleifera and their unsaturated fatty acid components inhibit biofilm formation by Staphylococcus aureus. Food Control 80, 74–82. doi: 10.1016/j.foodcont.2017.04.035
Lowy, F. D. (1998). Staphylococcus aureus infections. N. Engl. J. Med. 339, 520–532. doi: 10.1056/nejm199808203390806
Schaenzer, A. J., Wlodarchak, N., Drewry, D. H., Zuercher, W. J., Rose, W. E., Ferrer, C. A., et al. (2018). GW779439X and its pyrazolopyridazine derivatives inhibit the serine/threonine kinase Stk1 and act as antibiotic adjuvants against β-lactam-resistant Staphylococcus aureus. ACS Infect. Dis. 4, 1508–1518. doi: 10.1021/acsinfecdis.8b00136
Seo, S., Jung, J., Kim, C. Y., Kang, H., and Lee, I. H. (2021). Antimicrobial peptides encounter resistance of aureolysin during their action on Staphylococcus aureus biofilm. Biotechnol. Bioprocess Eng. 26, 216–222. doi: 10.1007/s12257-020-0384-z
Sieuwerts, S., de Bok, F. A., Mols, E., de vos, W. M., and Vlieg, J. E. (2008). A simple and fast method for determining colony forming units. Lett. Appl. Microbiol. 47, 275–278. doi: 10.1111/j.1472-765X.2008.02417.x
Silber, N., Matos de Opitz, C. L., Mayer, C., and Sass, P. (2020). Cell division protein FtsZ: from structure and mechanism to antibiotic target. Future Microbiol. 15, 801–831. doi: 10.2217/fmb-2019-0348
Speri, E., Kim, C., De Benedetti, S., Qian, Y., Lastochkin, E., Fishovitz, J., et al. (2019). Cinnamonitrile adjuvants restore susceptibility to β-lactams against methicillin-resistant Staphylococcus aureus. ACS Med. Chem. Lett. 10, 1148–1153. doi: 10.1021/acsmedchemlett.9b00169
Stewart, P. S., and Costerton, J. W. (2001). Antibiotic resistance of bacteria in biofilms. Lancet 358, 135–138. doi: 10.1016/s0140-6736(01)05321-1
Wassmann, C. S., Rolsted, A. P., Lyngsie, M. C., Torres-Puig, S., Kronborg, T., Vestergaard, M., et al. (2022). The menaquinone pathway is important for susceptibility of Staphylococcus aureus to the antibiotic adjuvant, cannabidiol. Microbiol. Res. 257:126974. doi: 10.1016/j.micres.2022.126974
Keywords: aminoglycoside antibiotics, biofilm, fatty acids, myristoleic acid, S. aureus
Citation: Park S, Lee J-H, Kim Y-G, Hu L and Lee J (2022) Fatty Acids as Aminoglycoside Antibiotic Adjuvants Against Staphylococcus aureus. Front. Microbiol. 13:876932. doi: 10.3389/fmicb.2022.876932
Edited by:
Mariano Martinez-Vazquez, Universidad Nacional Autónoma de México, MexicoReviewed by:
Maria José Saavedra, Universidade de Trás os Montes e Alto Douro, PortugalArunachalam Muthaiyan, University of New Mexico Gallup, United States
Copyright © 2022 Park, Lee, Kim, Hu and Lee. This is an open-access article distributed under the terms of the Creative Commons Attribution License (CC BY). The use, distribution or reproduction in other forums is permitted, provided the original author(s) and the copyright owner(s) are credited and that the original publication in this journal is cited, in accordance with accepted academic practice. No use, distribution or reproduction is permitted which does not comply with these terms.
*Correspondence: Jintae Lee, jtlee@ynu.ac.kr
†These authors have contributed equally to this work