- 1Hunan Engineering Technology Research Center of Featured Aquatic Resources Utilization, College of Animal Science and Technology, Hunan Agricultural University, Changsha, China
- 2Hunan Agriculture Product Processing Institute, Hunan Academy of Agricultural Sciences, Changsha, China
Aeromonas hydrophila is one of the important pathogenic bacteria in aquaculture causing serious losses every year. Essential oils are usually used as natural antimicrobial agents to reduce or replace the use of antibiotics. The aim of this study was to evaluate the antibacterial activity and explore the mechanisms of essential oil from satsuma mandarin (Citrus unshiu Marc.) (SMEO) against A. hydrophila. The results of the gas chromatography-mass spectrometer demonstrated that SMEO contains 79 chemical components with the highest proportion of limonene (70.22%). SMEO exhibited strong antibacterial activity against A. hydrophila in vitro, the diameter of the inhibition zone was 31.22 ± 0.46 mm, and the MIC and MBC values were all 1% (v/v). Intracellular material release, scanning electron microscopy (SEM), transmission electron microscopy (TEM), and flow cytometry analysis revealed the dynamic antibacterial process of SMEO, the morphological changes of bacterial cells, and the leakage process of intracellular components. These results demonstrated that SMEO disrupted the extracellular membrane permeability. Our study demonstrated that SEMO has the potential to be used to control and prevent A. hydrophila infections in aquaculture.
Introduction
In recent years, with the rapid development and expansion of the aquaculture industry in the world, outbreaks of diseases in aquatic animals are increasing, which have become serious threats to the sustainable development of aquaculture. Compared with other pathogens (e.g., viruses, fungi, and parasites), diseases caused by bacteria have become a major obstacle to aquaculture, because bacteria can survive independently in the aquatic environment without the presence of a host. Among these bacterial pathogens, diseases caused by Aeromonas hydrophila have caused huge economic losses to aquaculture in the world (Vivekanandhan et al., 2002; Rico et al., 2013; Stratev and Odeyemi, 2017). A. hydrophila belongs to Vibrionaceae and Aeromonas genus, which is a type strain of Aeromonas (Hamid et al., 2016). It is an opportunistic pathogenic bacterium with a wide range of hosts, including fish, mollusks, crustaceans, amphibians, reptiles, poultry, mammals, and humans (Janda and Abbott, 2010; Parker and Shaw, 2011). A. hydrophila is widely present in water, soil, silt and biological body surfaces, digestive tracts, and feces (Yardimci and Aydin, 2011; Rasmussen-Ivey et al., 2016; Fernández-Bravo and Figueras, 2020). The main symptoms of infection include local damage, necrosis, surface hemorrhage, edema, and abdominal distension (Sapkota et al., 2008; Rico and Van den Brink, 2014). At present, the methods for the control and prevention of A. hydrophila in aquaculture mainly include medical, immune, and biological therapies. Indeed, medical therapies, especially antibiotics, are still the main method used for the prevention of A. hydrophila.
However, the abuse or overuse of antibiotics is widespread in aquaculture, which increases the resistance of microorganisms (antimicrobial resistance, AMR), and ultimately leads to the emergence of drug-resistant microorganisms (Yang et al., 2019). Several studies have shown that the abuse or overuse of antibiotics [such as tetracycline (Nawaz et al., 2006), enrofloxacin (Zhu et al., 2017), ampicillin (Erdem et al., 2010)] increased the drug resistance of A. hydrophila. Meanwhile, multiple drug-resistant strains of A. hydrophila were found in a different variety of fishes, which may enter the food chain through aquatic products and infect humans (Vivekanandhan et al., 2005). Moreover, the overuse of antibiotics may also cause antibiotic residues in aquatic animals and the environment, which is a serious threat to food safety and human health (Majolo et al., 2017; Guo et al., 2018; Gao et al., 2020).
Over the years, the problems caused by drug-resistant microorganisms have caused widespread concern around the world. Many countries have issued the “National Action Plan for Antimicrobial Resistance (Shallcross and Davies, 2014),” which aimed to deal with the risks and challenges posed by microbial resistance. One of the main measures in the “Plan” pointed out that the development of “antibiotic alternatives” is an important way to solve the problem of drug resistance. Essential oils (EOs), one of the promising antibiotic alternatives, is a kind of volatile oily liquid substance extracted from different parts of a plant, such as fruits, seeds, peels, flowers, and leaves (El Asbahani et al., 2015). Many investigations indicated that EOs have antimicrobial, insecticidal, antioxidant, and anti-inflammatory activities (Bakkali et al., 2008). When focusing on the antimicrobial part, EOs are widely used as human drugs, veterinary drugs, and food preservatives (Idris et al., 2017; Trifan et al., 2020). But until now, the studies focusing on the antimicrobial activity of EOs in aquaculture are very limited.
China is the world’s largest producer of citrus. In addition to fresh food, citrus is mainly used for processing (such as juice and canned food), but this processing may produce a mass of citrus peels (Gómez-Mejía et al., 2019). Extracting EO from the peels is a promising way for its comprehensive utilization. Thus, in this study, EO was extracted from one of the major citrus cultivars in China, Satsuma mandarin (Citrus unshiu Marc.). Furthermore, its antibacterial activity and mechanism of action against A. hydrophila were investigated. We aimed to develop a natural antibiotic alternative (EO) to solve the problems of drug resistance and antibiotic residues and provide a new method for the prevention and treatment of A. hydrophila in aquaculture.
Materials and Methods
Microorganism and Culture Conditions
Aeromonas hydrophila (PRJNA808687) used in this study was from our lab, which was isolated from grass carp (Ctenopharyngodon Idella) in 2018. A. hydrophil (CICC25017) was purchased from the China Center of Industrial Culture Collection. A. hydrophila was streaked on Tryptone Soy Agar (TSA, Guangdong Huankai Microbial Sci. & Tech. Co., Ltd., China) and incubated at 28°C for 24 h, and then 5 colonies were transferred to 5 ml of Tryptone Soy Broth (TSB, Guangdong Huankai Microbial Sci. & Tech. Co., Ltd., China) and incubated for 8 h at 28°C with shaking.
Steam Distillation of Essential Oil From Satsuma Mandarin
Satsuma mandarin was collected from Neijiang city, Sichuan Province, China. In the Clevenger apparatus, 500 g of satsuma mandarin peels were suspended in 2,000 ml of distilled water and subjected to steam distillation. The extraction was carried out for 3 h, and the obtained EO was collected, dried by anhydrous sodium sulfate (Na2SO4) for 24 h, and then stored at 4°C in brown glass vials.
Gas Chromatography-Mass Spectrometry
The GC-MS analysis was carried out by using an Agilent 7890A GC equipped with a Gerstel MPS autosampler, coupled with an Agilent 5975C MSD detector. The chromatographic separation was performed on an HP-5MS capillary column 30 m × 0.25 mm i.d., 0.25 μm, the GC oven was operated at 40°C held for 1 min, increased to 220°C at a rate of 3°C/min, held at 220°C for 25 min, increased to 250°C at a rate of 5°C/min, and finally held for 10 min. Helium was used as the carrier gas at a flow rate of 1 ml/min. The injector and detector temperatures were set at 250 and 280°C, respectively. The mass spectrometer was operated in the 70 eV EI mode with scanning from 35 to 350 amu/s, and the ion source was set at 230°C. The EO components were identified by matching their recorded mass spectra with the data bank NIST 08 (National Institute of Standards and Technology).
Agar Diffusion Method
A volume of 100 μl bacterial dilution (PRJNA808687 and CICC25017, 1 × 106 CFU/ml) was evenly smeared on Mueller-Hinton Agar (MHA). Following that, filter paper disks (6 mm in diameter) containing 6 μl SMEO were placed on the surface of the agar plates. After standing for 10 min, the plates were incubated at 28°C for 12 h. Meanwhile, antibiotics (florfenicol and amoxicillin) and water + 1% Tween were used as positive and negative controls, respectively. Finally, the diameters of the inhibition zone (DIZ) were measured (Oonmetta-aree et al., 2006).
Determination of Minimum Inhibitory Concentration and Minimum Bactericidal Concentration
Serial dilutions of SMEO ranging from 0.0625 to 16% were prepared in TSB (with 1% Tween 20). A volume of 100 μl of each SMEO serial dilution was dispensed into 96 microtiter plates, respectively. Afterward, 100 μl of the bacterial suspension (PRJNA808687, 2 × 106 CFU/ml) was treated with the dilutions of SMEO. Then, the microtiter plates were cultured at 28°C for 120 rpm. After 24 h incubation of the bacterial suspension, the lowest concentration of SMEO with no bacterial growth was determined as MIC. Furthermore, to determine MBC, 10 μl of the above solutions (from the concentration of MIC to 16%) were placed on TSA plates and incubated at 28°C for 24 h. MBC was the lowest concentration of SMEO without any visible colonies on the plates.
Bacterial Growth Kinetics
Bacterial growth kinetics was measured by the effect of SMEO on bacterial growth (Sureshkumar et al., 2010). According to the above experimental data, 0 × MIC, 0.0625 × MIC, 0.125 × MIC, 0.25 × MIC, 0.5 × MIC, and 1 × MIC of SMEO were diluted with 40 ml bacterial suspension (PRJNA808687, 1 × 106 CFU/ml). The suspensions were incubated at 28°C for shaking (120 rpm), and the absorbance was measured by a spectrophotometer (UV759S, INESA Analytical Instrument Co., Ltd., China) at an optical density of 600 nm at the following time points (t/h): 0, 2, 4, 6, 8, 10, 12, 14, 16, 18, 20, 22, 24, 26, 28, 30, 36, and 48 h.
Leakage of Cellular Components Assay
A total of 3–4 colonies of A. hydrophila were transferred into 10 ml TSB and incubated at 28°C overnight. After incubation, cells were collected by centrifugation at 8,000 rpm for 10 min and adjusted to the concentration of 1 × 109 CFU/ml (PRJNA808687) in 10 ml of phosphate-buffered solution (PBS). Then, SMEO at the concentration of 1 × MIC was added to the abovementioned solutions and treated at 28°C for 4, 8, and 12 h. Finally, the supernatants were separated from bacterial cells by centrifugation (8,000 rpm) and filtration (with 0.22 μm filter, Sigma-Aldrich, United States), which will be used for the nucleic acid and protein assay as described. At the same time, SMEO treatment for 0 h was used as the zeroing sample for UV spectroscopic detection.
For the nucleic acid assay, the absorbance of the supernatant samples was measured by a spectrophotometer (UV759S, INESA Analytical Instrument Co., Ltd., China) at the optical density of 260 nm.
For the protein assay, a Total Protein Kit, Micro (Sigma-Aldrich, United States) was used to measure the concentration of protein. According to the operating instructions, the protein assay solution was mixed with the supernatant samples. After approximately 2 min, the absorbance of the sample was also measured by a spectrophotometer at the optical density of 595 nm (UV759S, INESA Analytical Instrument Co., Ltd., China).
Scanning Electron Microscope and Transmission Electron Microscope
Aeromonas hydrophila (PRJNA808687) was incubated to log phase as the conditions mentioned in the “Microorganism and culture conditions” section; after incubation, bacterial suspensions were adjusted to 1 × 108 CFU/ml. Cells (2 ml suspensions) were collected by centrifugation at 8,000 rpm for 10 min, and the bacterial pellet was carefully washed with PBS and resuspended. Later, 1 × MIC SMEO (with 1% Tween 20) was added to the resuspension and treated for 4, 8, and 12 h. Finally, the samples were collected by centrifugation, washed three times with PBS, and fixed with 2.5% glutaraldehyde at 4°C for 4 h or overnight. Next, the samples were prepared for SEM and TEM assay as described.
For SEM, the glutaraldehyde solution was removed and the bacteria samples were washed with PBS for three times, and then dehydrated with 10, 30, 50, 70, and 90% ethanol; in turn, 15 min each time, and dehydrated two times with 100% ethanol, 20 min each time. After dehydration, ethanol was replaced with tertiary butanol two times for 20 min each, then the samples were vacuum freeze-dried, sprayed with gold, and observed by scanning electron microscope (Hitachi S-4800, Hitachi, Japan).
For TEM, the fixed solution was removed, and the samples were washed three times with PBS, then the bacteria samples were placed on the copper mesh, air-dried for 5 min, and negatively stained with 1% phosphotungstic acid. After that, the samples were observed by a transmission electron microscope (Hitachi HT-7700, Hitachi, Japan).
Flow Cytometry Analysis
Flow cytometry analysis was carried out to investigate the effects of SMEO on A. hydrophila (PRJNA808687). The method for SMEO treatment was the same as mentioned in the “Leakage of Cellular Components Assay” section, but the treatment time is 8 h. Meanwhile, PBS and ethanol treatment were used as negative and positive controls, respectively. After treatment, bacterial cells were collected by centrifugation and adjusted to the concentration of 1 × 106 CFU/ml; then different staining procedures proceeded as follows.
Membrane Permeability [Thiazole Orange and Propidium Iodide Staining]
Thiazole orange (TO, Sigma-Aldrich, United States) and propidium iodide (PI, Sigma-Aldrich, United States) were used to evaluate the membrane permeability of cells. For TO staining, 1-μl TO solution was added to 1-ml bacterial suspensions (final concentration of TO: 10 μg/ml in DMSO), and then incubated at room temperature for 15 min. For PI staining, 1-μg PI was added to 1 ml bacterial suspensions (final concentration of PI: 1 μg/ml in PBS), and then incubated at 37°C for 15 min.
Membrane Potential [Bis-1,3-Dibutylbarbutiric Acid (BOX) and Propidium Iodide Staining]
Bis-1,3-dibutylbarbutiric acid (BOX, Sigma-Aldrich, United States) and PI were used to evaluate the membrane potential of cells. For BOX staining, 2.5-μg BOX was added to 1-ml bacterial suspensions (final concentration of BOX: 2.5 μg/ml in PBS with 4 mM EDTA), and then incubated at 37°C for 15 min. For PI staining, the procedure was the same as mentioned in the “Membrane Permeability (TO and PI Staining)” section.
Efflux Activity [Ethidium Bromide Staining]
Ethidium bromide (EB, Sigma-Aldrich, United States) was used to evaluate the efflux activity of cells. For EB staining, 10-μg EB was added to 1-ml bacterial suspensions (final concentration of EB: 10 μg/ml in DMSO) and then incubated at 37°C for 15 min.
After finishing these staining procedures, samples were washed three times with PBS, and the concentration of bacterial suspensions was adjusted to about OD600 = 0.1. Then, the samples were placed on ice for flow cytometry analysis by a flow cytometer (BD Accuri C6 plus, BD, United States), green fluorescence was collected in the FL1 channel (533 nm), and red fluorescence in the FL3 channel (>670 nm). Fluorescence signals were collected by FL1 (TO and BOX) and FL3 (PI, EB) bandpass filters. Bacterial cells were gated per the FSC/SSC parameters and a total of 5,000 events were acquired for each sample.
Statistical Analysis
All the experiments were performed in triplicate. Statistical analysis was carried out using SPSS and GraphPad Prism 7 for the t-tests. All asterisks indicate significant differences (p < 0.05).
Results and Discussion
The Chemical Composition of Essential Oil From Satsuma Mandarin
The chemical composition of SMEO is summarized in Table 1. A total of 79 components were identified, which accounted for the percentage of 99.9997%. Meanwhile, 96.3238% of SEMO constituents were monoterpenes. Limonene was the most abundant component with a percentage of 70.2252%, followed by γ-terpinene (7.8955%), β-myrcene (5.0086%), l-α-pinene (3.8957%), β-terpinene (1.7055%), linalool (1.5054%), and α-terpineol (1.0265%); these seven components account for 91.2624% of all the components. The percentage of the other remaining 72 components was below 1% (0.0097–0.8700%).
The Antibacterial Activity of SMEO
As shown in Figure 1A and Table 2, SMEO florfenicol exhibited strong antibacterial activity against A. hydrophila in vitro (PRJNA808687 and CICC25017). Among them, the inhibition diameter of SEMO against A. hydrophila was 31.22 ± 0.46 mm. The bacteria were completely killed when the SEMO concentration was 1%. During bacterial growth period, A. hydrophila was exposed to SEMO to confirm whether the environmental adaptations of cells were affected. The antibacterial kinetic curves of SMEO were demonstrated in Figure 1B, which reflected the kinetic character of SMEO at different concentrations (0.0625 × MIC, 0.125 × MIC, 0.25MIC, 0.5 × MIC, and 1 × MIC) and treated times. In the 0.0625 × MIC, 0.125 × MIC, 0.25 × MIC, and 0.5 × MIC groups, the increasing lag phase time and decreasing concentration of bacteria during the stationary phase indicated that the cells were more sensitive to the environmental stress in which SMEO was located. Meantime, bacterial growth was not observed at the concentration of 1 × MIC.
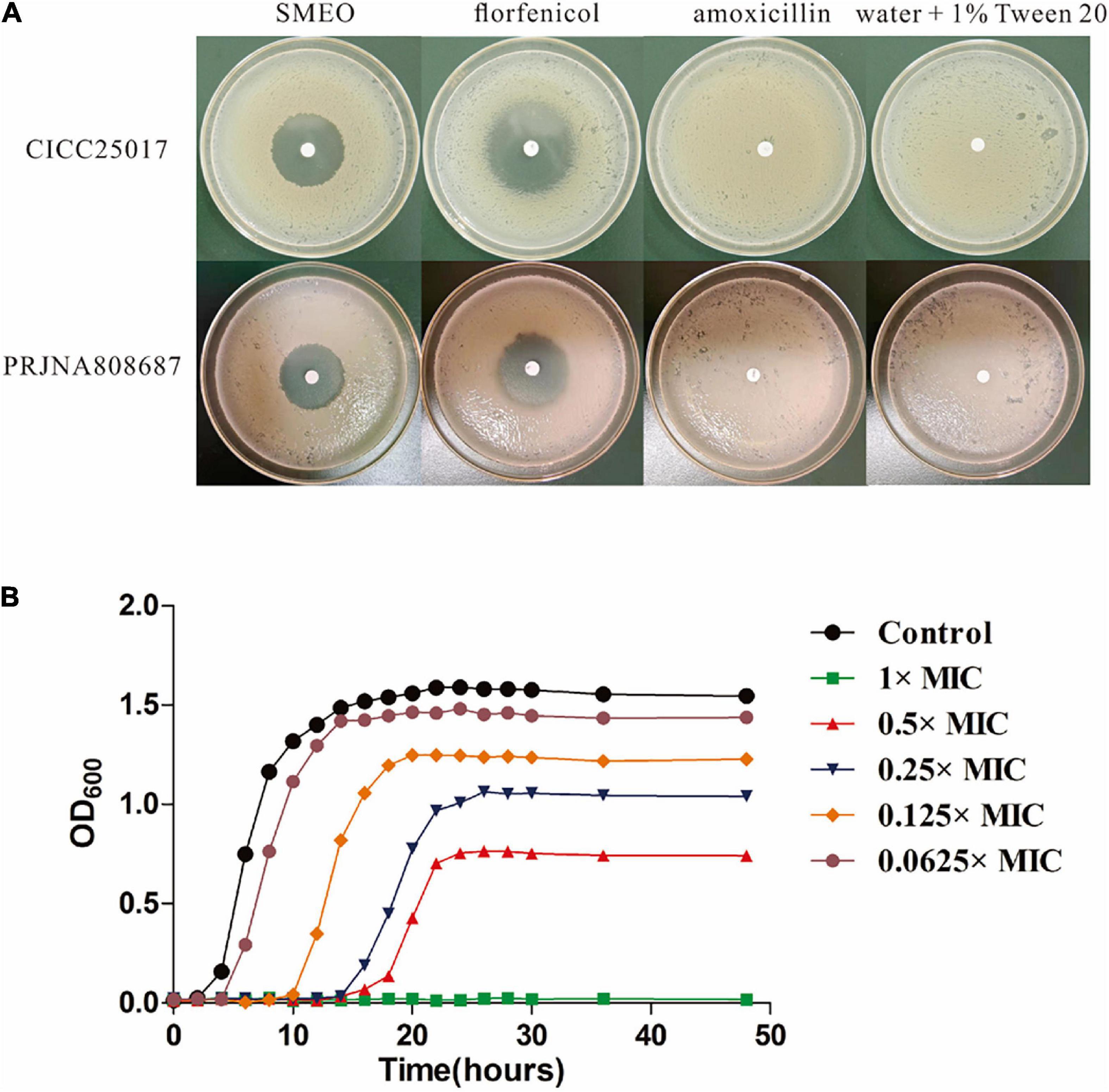
Figure 1. The antibacterial activity of SMEO against Aeromonas hydrophila. (A) The diameter zone of inhibition (mm). (B) A. hydrophila growth curves with or without different concentrations of SMEO.
The Release of Intracellular Material
The release of the bacterial intracellular material was observed by measuring changes in the composition of cell supernatant. Figure 2A shows the changes in nucleic acids. When the cells were exposed to SMEO at the concentrations of 2 × MIC for 4, 8, and 12 h, the values of A260 were 0.1950 ± 0.0324, 0.8163 ± 0.0573, and 1.0992 ± 0.0529, respectively. The results demonstrated that the concentration of nucleic acids in the supernatant increased with the increase of exposure time. Figure 2B shows the protein changes by Coomassie blue staining, which indicated that the concentration of proteins increased significantly (p < 0.05) with the increase of exposure time, with values of 0.0237 ± 0.0015, 0.0513 ± 0.0032, and 0.1157 ± 0.0061 in A595, respectively. Meanwhile, the changes in protein and nucleic acid concentrations in the extracellular supernatant demonstrate that SMEO was able to rupture bacterial cell membranes.
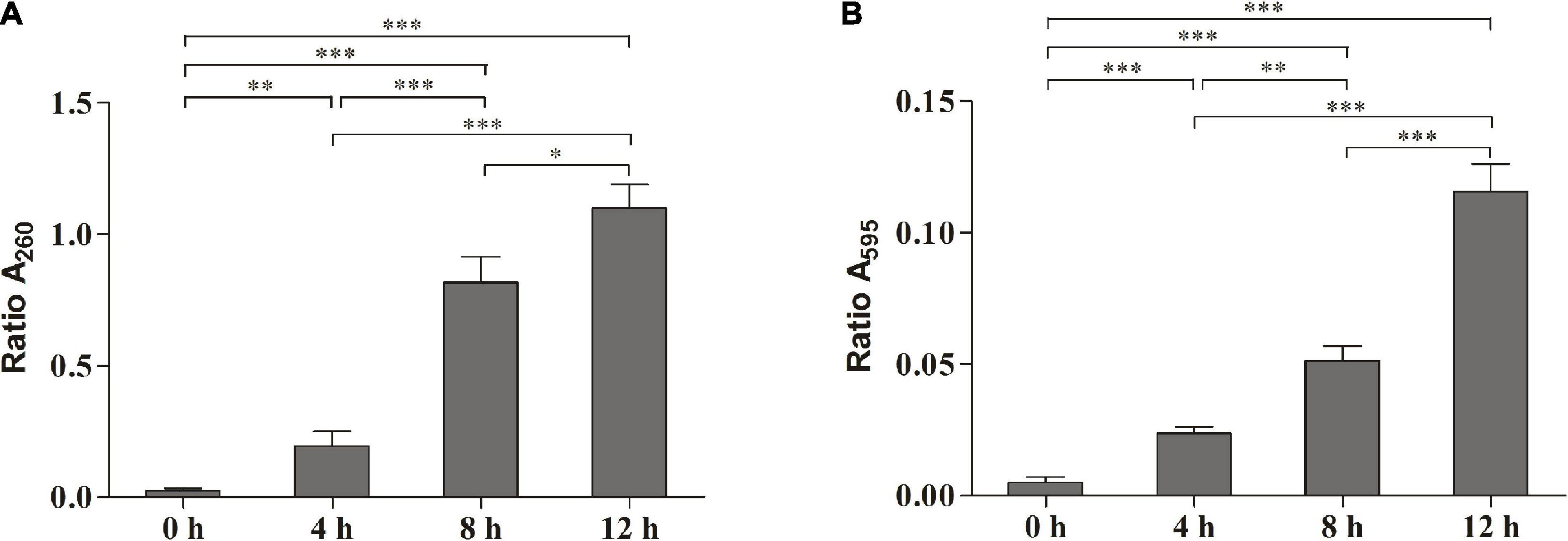
Figure 2. The release of the intracellular material of A. hydrophila at different treatment times (0, 4, 8, and 12 h) in 1 × minimum inhibitory concentration (MIC) SMEO. (A) Change of the optical absorbance (A260) of cell supernatant, which is an indicator of nucleic acids. (B) Change of the optical absorbance (A280) of cell supernatant, which is an indicator of protein. ∗, ∗∗, and *** represent P < 0.05, P < 0.01, and P < 0.001.
Scanning Electron Microscopy Observations
Scanning electron microscopy was used to reveal the changes in themorphology of A. hydrophila with and without the treatment of SMEO. As shown in Figure 3, cells in the control group were rod-shaped with smooth surfaces and intact structures, about 1–2 μm in length. Compared with the control group, the morphology of cells in those three experimental groups showed significant changes, wrinkled surfaces (pointed by the red arrow, Figure 3, 4h), and collapsed cells (pointed by the blue circle, Figure 3, 4,8h), indicating the huge damage caused by SMEO. The degree of SMEO-induced morphological changes and the quantity of affected cells enhanced with the increase in treatment time. First, after a 6-h treatment (Figure 3, 4 h), bacterial cells began to collapse, and some of the folded cells remained rod-shaped. Further, after an 8-h treatment (Figure 3), 8 h the surfaces of the cells were more densely collapsed and wrinkled, and the cell morphology changed significantly. Moreover, after a 12-h treatment, no intact cells existed and only a fraction of cells could be observed, which indicated the whole lysis of cells.
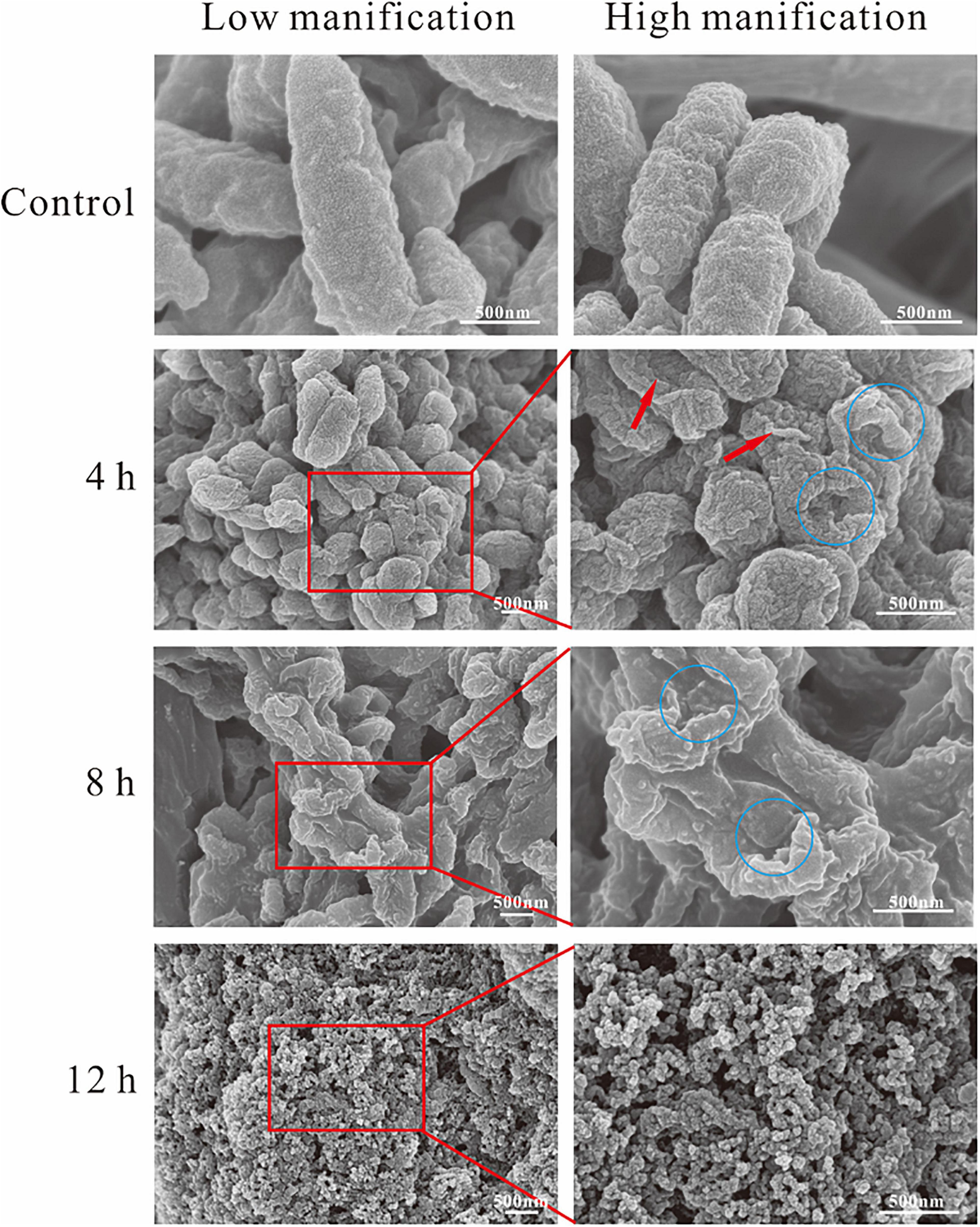
Figure 3. Scanning electron microscopy (SEM) photography of A. hydrophila planktonic cell. (Control) Untreated group; (6, 12, and 18 h) bacteria treated by SMEO at 1 × MIC for 6, 12, and 18 h, respectively. The red arrows and blue circles indicate wrinkled surfaces and cell collapse.
Transmission Electron Microscopy Observations
Transmission electron microscopy was also used to observe more details. As shown in Figure 4, in the control group, the cell membrane and cell wall were intact, and the cytoplasm was evenly dispersed. Compared with the control group, the cell morphology of the other three experimental groups changed significantly, and wrinkled surfaces (pointed by the red arrow, Figure 4), 4 h vacuoles inside the cytoplasm (pointed by the purple arrow, Figure 4), 4, 8, 12 h and the leakage of cytoplasm (pointed by the blue arrow, Figure 4), 8 and 12 h were clearly observed. Meanwhile, the boundaries of the cell membrane and cell wall became vague, and the distribution of cytoplasm became heterogeneous. Moreover, some extreme phenomena were also observed after the treatment of SMEO, such as the bare cell wall without cytoplasm inside it (pointed by the green arrow, Figure 4), 12 h and the individual cytoplasm without the protection of the cell wall (pointed by the blue arrow, Figure 4, 12 h).
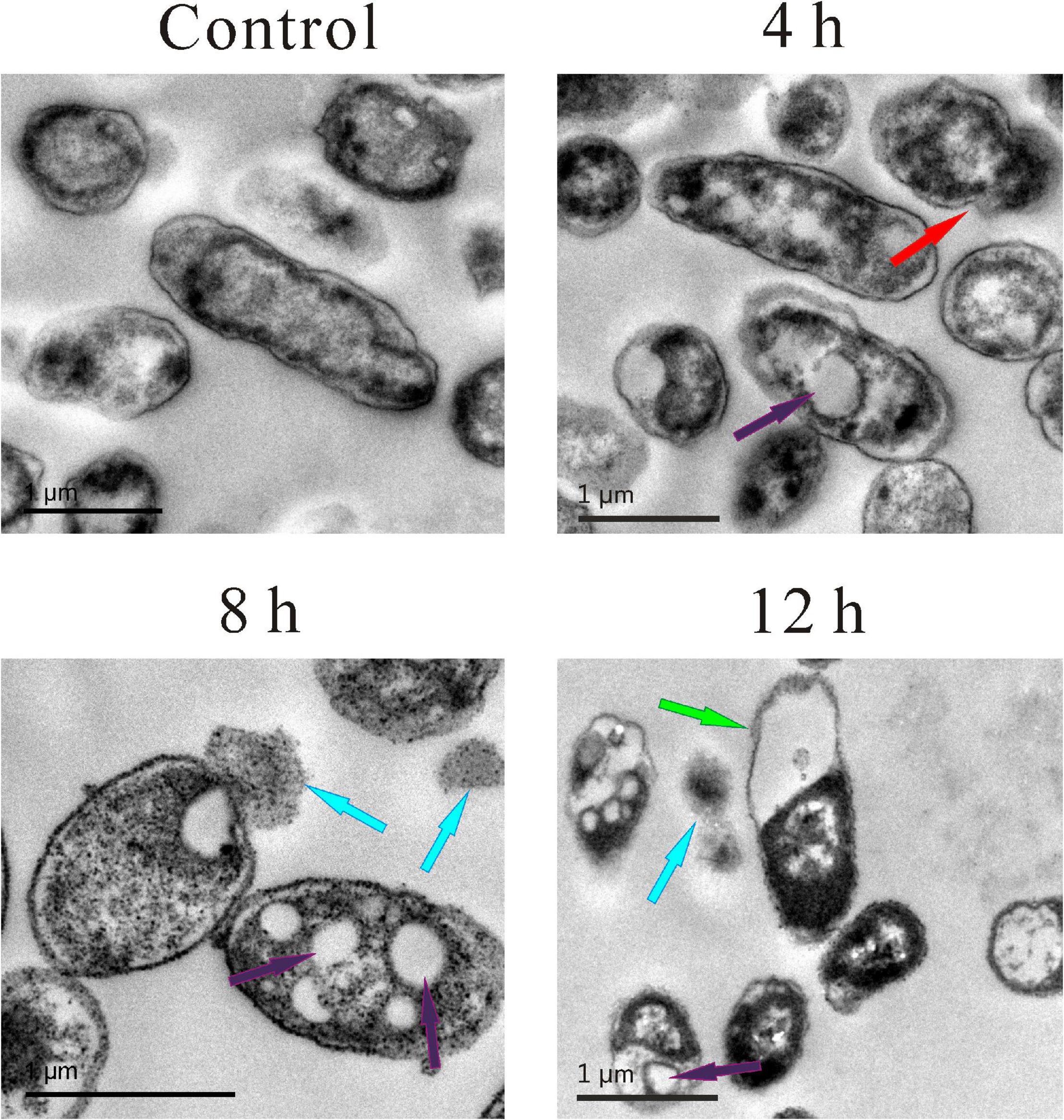
Figure 4. Transmission electron microscopy (TEM) photography of A. hydrophila planktonic cell. (Control) Untreated group; (6, 12, 18 h) bacteria treated by SMEO at 1 × MIC for 6, 12, and 18 h, respectively. The red, purple, blue, and green arrows indicate wrinkled surfaces, vacuoles inside the cytoplasm, the leakage of cytoplasm, and the cell wall without cytoplasm inside, respectively.
Flow Cytometry Analysis
Four fluorescent dyes (TO, PI, BOX, and EB) were used to evaluate several vital biological functions in A. hydrophila cells by flow cytometry analysis.
Membrane integrity was evaluated by double staining of TO and PI as shown in Figure 5 (the first row). In the control group, 73.2% of the cells were located in plot Q1 (TO + and PI–), which represented cells with intact cell membranes. Approximately 99.1% of the cells in the positive control group were located in plot Q2 (TO + and PI +), which represented cells with permeabilized cell membranes. In the SMEO-treated group, 75.6% of the cells were located in plot Q2 (TO + and PI +). Moreover, 11.9% of the cells were located in plot Q4 (TO- and PI-), which represented cells with damaged DNA or RNA.
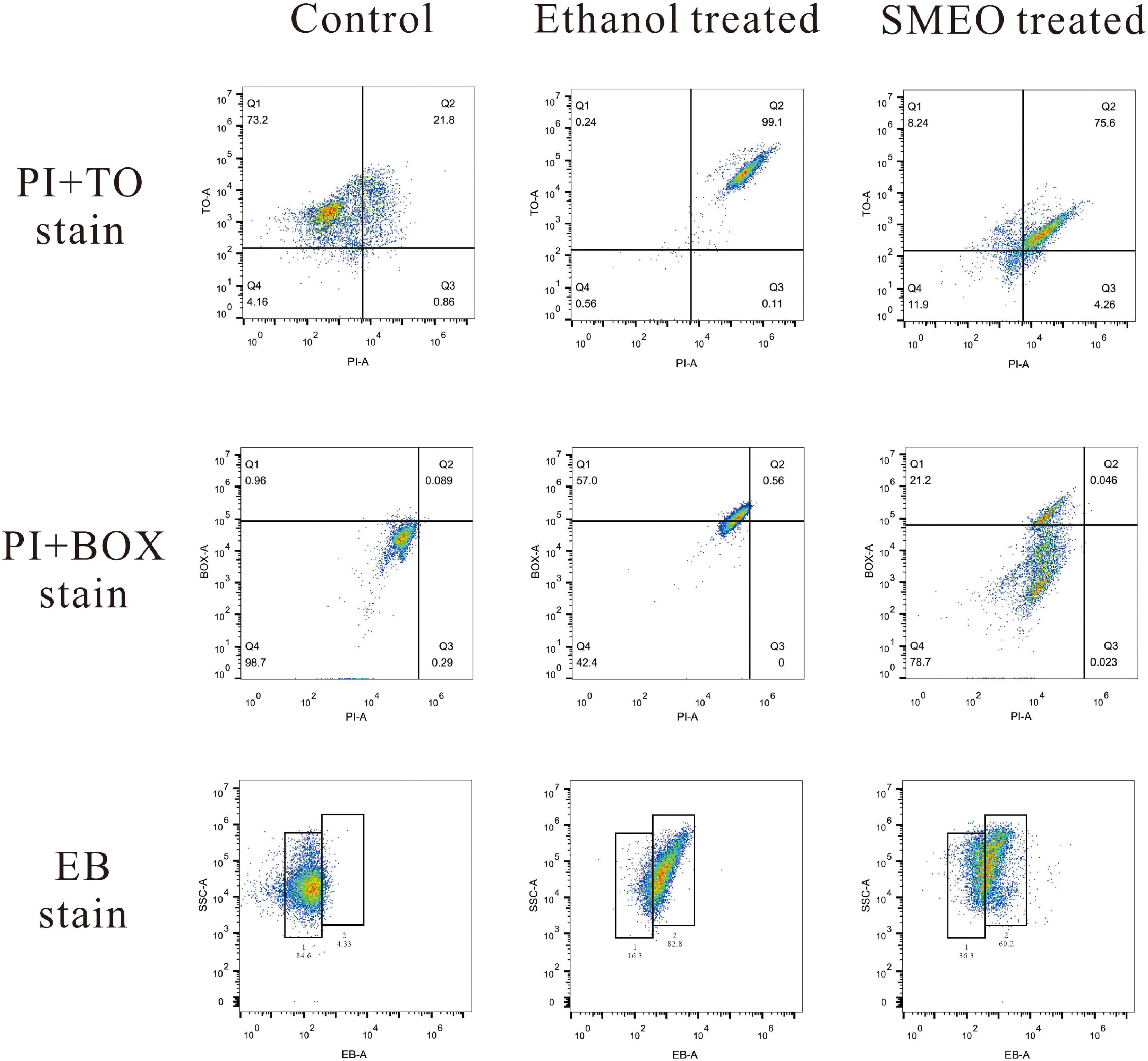
Figure 5. Fluorescence density plots of A. hydrophila treated with ethanol, SMEO, and PBS (Control), stained with propidium iodide (PI) and thiazole orange (TO), PI and bis-1,3-dibutylbutyric acid (BOX) and ethidium bromide (EB).
Membrane potential was evaluated by double staining of BOX and PI as shown in Figure 5 (the second row). In the control group, 98.7% of the cells were located in plot Q4 (BOX- and PI-), which represented cells with polarized membranes. By contrast, in the SMEO-treated group and ethanol-treated group, 21.2% of the cells and 57.0% of the cells were located in plot Q1 (BOX + and PI-), respectively, which represented cells with depolarized membrane. Efflux activity was evaluated by EB staining as shown in Figure 5 (the third row). EB represented that the efflux pump functioned properly, while EB + meant the malfunction of the efflux pump. The percentage of EB + cells in the control and treated groups was 4.33 and 84.6%, and the percentage of EB- cells in these two groups was 60.2 and 36.3%.
Discussion
Many species in Citrus L. are industrial crops and have a wide range of planting areas. In this study, SMEO was composed of 89 components, and the main chemical component was limonene (70.2252%). Meanwhile, other studies have exhibited that the content of limonene in the EO extracted from Citrus unshiu Marc. occupied a high percentage of content (Espina et al., 2011; Elmaci and Onoğur, 2012; Ioannou et al., 2012). Although not absolute, the extraction of Citrus unshiu Marc. by solid-phase micro-extraction showed that EO was not only composed of limonene but also linalool, γ-terpinene and β-elemene, p-cymene, and other components (Azam et al., 2013). The different composition of Citrus unshiu Marc. EO might be caused by different harvesting periods and extraction processes (Settanni et al., 2014).
As bacterial resistance has become a hindrance to antibiotics, the antibacterial activity of citrus leaf and peel extracts are prerequisites as potential alternatives to antibiotic drugs. This work indicated that SMEO has a bacteriostatic effect on A. hydrophila. Furthermore, studies have also indicated that Citrus unshiu Marc. EO has antibacterial activity against food-borne bacteria (Espina et al., 2011). In addition to Citrus unshiu Marc. EO, a variety of plant EOs exhibited the ability to inhibit the growth of A. hydrophila (Iturriaga et al., 2012; Ruiz-Navajas et al., 2012; Majolo et al., 2017). For example, Thymus vulgaris, Eugenia caryophyllus, and Tee Tree EO inhibited the growth of A. hydrophila (Assane et al., 2021). Screening of EOs of different plant-derived varieties indicated that 14 of them were found to be active against A. hydrophila (Kot et al., 2019). Therefore, SMEO has a great potential to reduce the use of antibiotics in aquaculture.
At present, there are limited details on the mechanism of how Citrus unshiu Marc. EO affects A. hydrophila. The cell membrane maintains the relative homeostasis of the intracellular environment to allow biochemical reactions to proceed normally in the cell. Therefore, the damage of the cell membrane is extremely detrimental to the survival of bacteria (Ali et al., 2016; Lu et al., 2016). Antimicrobial peptides from Erythroculter ilishaeformis killed bacteria by disrupting the integrity of A. hydrophila cell membranes (Chen et al., 2020). The mustard (Brassica spp.) EO affected the membrane permeability of Escherichia coli and Salmonella typhi (Turgis et al., 2009). In the present study, the absence of the cell protective function of the cell membrane was demonstrated by the changes in proteins and nucleic acids. Meanwhile, the results of microscopic observation also indicated that the cell membrane of A. hydrophila was severely deformed.
Flow cytometry, which facilitates the acquisition of data and the analysis of multiparameter rapidly, is an effective method widely used to evaluate antimicrobial activity and mechanism of action (Turgis et al., 2009; Wang et al., 2010). In this study, membrane integrity was evaluated by double staining of TO and PI. The results showed that, after the treatment of SMEO, the cell membrane of most of the A. hydrophila cells became unintegrated and permeable, with a certain degree of DNA or RNA damage, which was consistent with the phenomena observed by SEM and TEM. Membrane potential was evaluated by double staining of BOX and PI. Nearly all the cells in the control group had polarized membranes, but 21.2% of the cells in the treated groups had depolarized membranes. The loss of membrane potential might be explained by the change of ion concentrations inside and outside of the cell membrane, which were induced by the increase of cell permeability (Mirzoeva et al., 1997). These abovementioned results together with those observations of SEM and TEM indicated that the cell membrane should be an important target for SMEO and that the cell membrane was seriously damaged after SMEO treatment, finally resulting in the leakage of the cell content.
Conclusion
Citrus EOs are applied in a variety of fields due to their various biological properties. In this study, SMEO was prepared and determined for composition. SMEO showed strong antibacterial activity against A. hydrophila. Intracellular material release, SEM, TEM, and flow cytometry analysis indicated that SMEO was capable of destabilizing the cell membrane. Therefore, the cell membrane was an important drug target for SMEO against A. hydrophila. Moreover, transcriptome and proteome technics will be used to explore the mechanisms at gene and protein levels.
Data Availability Statement
The original contributions presented in the study are included in the article/supplementary material, further inquiries can be directed to the corresponding authors.
Author Contributions
WZ: investigation and writing original draft preparation. KC and SJ: investigation. LY: visualization. TT: methodology. JG: validation and writing—review and editing. ZG: supervision, funding acquisition, and writing—review and editing. All authors contributed to the article and approved the submitted version.
Funding
This research was funded by the National Natural Science Foundation of China (32073020), the Key Projects of Hunan Education Department (20A238), the Changsha Municipal Natural Science Foundation (kq2014070 and kq2202332), the Key Research and Development Program of Hunan Province (2021NK2025), and the Key Laboratory of Agro-Products Processing, Ministry of Agriculture and Rural Affairs of China (S2021KFKT-22).
Conflict of Interest
The authors declare that the research was conducted in the absence of any commercial or financial relationships that could be construed as a potential conflict of interest.
Publisher’s Note
All claims expressed in this article are solely those of the authors and do not necessarily represent those of their affiliated organizations, or those of the publisher, the editors and the reviewers. Any product that may be evaluated in this article, or claim that may be made by its manufacturer, is not guaranteed or endorsed by the publisher.
References
Ali, S. S., Shaaban, M. T., Abomohra, A. E.-F., and El-Safity, K. (2016). Macroalgal activity against multiple drug resistant Aeromonas hydrophila: a novel treatment study towards enhancement of fish growth performance. Microb. Pathog. 101, 89–95. doi: 10.1016/j.micpath.2016.10.026
Assane, I. M., Valladao, G. M., and Pilarski, F. (2021). Chemical composition, cytotoxicity and antimicrobial activity of selected plant-derived essential oils against fish pathogens. Aquac. Res. 52, 793–809. doi: 10.1111/are.14935
Azam, M., Jiang, Q., Zhang, B., Xu, C., and Chen, K. (2013). Citrus leaf volatiles as affected by developmental stage and genetic type. Int. J. Mol. Sci. 14, 17744–17766. doi: 10.3390/ijms140917744
Bakkali, F., Averbeck, S., Averbeck, D., and Idaomar, M. (2008). Biological effects of essential oils–a review. Food Chem. Toxicol. 46, 446–475. doi: 10.1016/j.fct.2007.09.106
Chen, Y., Wu, J., Cheng, H., Dai, Y., Wang, Y., Yang, H., et al. (2020). Anti-infective effects of a fish-derived antimicrobial peptide against drug-resistant bacteria and its synergistic effects with antibiotic. Front. Microbiol. 11:2942. doi: 10.3389/fmicb.2020.602412
El Asbahani, A., Miladi, K., Badri, W., Sala, M., Addi, E. A., Casabianca, H., et al. (2015). Essential oils: From extraction to encapsulation. I Int. J. Pharm. 483, 220–243. doi: 10.1016/j.ijpharm.2014.12.069
Elmaci, Y., and Onoğur, T. (2012). Mandarin peel aroma: estimation by using headspace/GC/MS and descriptive analysis techniques. Acta Aliment. 41, 131–139. doi: 10.1556/AAlim.41.2012.1.15
Erdem, B., Kariptaş, E., and Kaya, T. (2010). Siderophore, hemolytic, protease, and pyrazinamidase activities and antibiotic resistance in motile Aeromonas isolated from fish. Turk. J. Biol. 34, 453–462. doi: 10.3906/biy-0901-20
Espina, L., Somolinos, M., Lorán, S., Conchello, P., García, D., and Pagán, R. (2011). Chemical composition of commercial citrus fruit essential oils and evaluation of their antimicrobial activity acting alone or in combined processes. Food Control 22, 896–902. doi: 10.1016/j.foodcont.2010.11.021
Fernández-Bravo, A., and Figueras, M. J. (2020). An update on the genus Aeromonas: taxonomy, epidemiology, and pathogenicity. Microorganisms 8:129. doi: 10.3390/microorganisms8010129
Gao, Z., Zhong, W., Chen, K., Tang, P., and Guo, J. (2020). Chemical composition and anti-biofilm activity of essential oil from Citrus medica L. var. sarcodactylis Swingle against Listeria monocytogenes. Ind. Crop. Prod. 144:112036. doi: 10.1016/j.indcrop.2019.112036
Gómez-Mejía, E., Rosales-Conrado, N., León-González, M. E., and Madrid, Y. (2019). Citrus peels waste as a source of value-added compounds: extraction and quantification of bioactive polyphenols. Food Chem. 295, 289–299. doi: 10.1016/j.foodchem.2019.05.136
Guo, J.-J., Gao, Z.-P., Xia, J.-L., Ritenour, M. A., Li, G.-Y., and Shan, Y. (2018). Comparative analysis of chemical composition, antimicrobial and antioxidant activity of citrus essential oils from the main cultivated varieties in China. LWT-Food Sci. Technol. 97, 825–839. doi: 10.1016/j.lwt.2018.07.060
Hamid, R., Ahmad, A., and Usup, G. (2016). Pathogenicity of Aeromonas hydrophila isolated from the Malaysian Sea against coral (Turbinaria sp.) and sea bass (Lates calcarifer). Environ. Sci. Pollut. Res. 23, 17269–17276. doi: 10.1007/s11356-016-6655-8
Idris, M., Abbas, R., Masood, S., Rehman, T., Farooq, U., Babar, W., et al. (2017). The potential of antioxidant rich essential oils against avian coccidiosis. Worlds Poult. Sci. J. 73, 89–104. doi: 10.1017/S0043933916000787
Ioannou, C. S., Papadopoulos, N. T., Kouloussis, N. A., Tananaki, C. I., and Katsoyannos, B. I. (2012). Essential oils of citrus fruit stimulate oviposition in the Mediterranean fruit fly Ceratitis capitata (Diptera: Tephritidae). Physiol. Entomol. 37, 330–339. doi: 10.1111/j.1365-3032.2012.00847.x
Iturriaga, L., Olabarrieta, I., and de Marañón, I. M. (2012). Antimicrobial assays of natural extracts and their inhibitory effect against Listeria innocua and fish spoilage bacteria, after incorporation into biopolymer edible films. Int. J. Food Microbiol. 158, 58–64. doi: 10.1016/j.ijfoodmicro.2012.07.001
Janda, J. M., and Abbott, S. L. (2010). The genus Aeromonas: taxonomy, pathogenicity, and infection. Clin. Microbiol. Rev. 23, 35–73. doi: 10.1128/CMR.00039-09
Kot, B., Kwiatek, K., Janiuk, J., Witeska, M., and Pȩkala-Safińska, A. (2019). Antibacterial activity of commercial phytochemicals against Aeromonas species isolated from fish. Pathogens 8:142. doi: 10.3390/pathogens8030142
Lu, J., Wang, Z., Ren, M., Huang, G., Fang, B., Bu, X., et al. (2016). Antibacterial effect of gallic acid against Aeromonas hydrophila and Aeromonas sobria through damaging membrane integrity. Curr. Pharm. Biotechnol. 17, 1153–1158. doi: 10.2174/1389201017666161022235759
Majolo, C., da Rocha, S. I. B., Chagas, E. C., Chaves, F. C. M., and Bizzo, H. R. (2017). Chemical composition of Lippia spp. essential oil and antimicrobial activity against Aeromonas hydrophila. Aquac. Res. 48, 2380–2387. doi: 10.1111/are.13073
Mirzoeva, O., Grishanin, R., and Calder, P. (1997). Antimicrobial action of propolis and some of its components: the effects on growth, membrane potential and motility of bacteria. Microbiol. Res. 152, 239–246. doi: 10.1016/s0944-5013(97)80034-1
Nawaz, M., Sung, K., Khan, S. A., Khan, A. A., and Steele, R. (2006). Biochemical and molecular characterization of tetracycline-resistant Aeromonas veronii isolates from catfish. Appl. Environ. Microbiol. 72, 6461–6466. doi: 10.1128/AEM.00271-06
Oonmetta-aree, J., Suzuki, T., Gasaluck, P., and Eumkeb, G. (2006). Antimicrobial properties and action of galangal (Alpinia galanga Linn.) on Staphylococcus aureus. LWT-Food Sci. Technol. 39, 1214–1220. doi: 10.1016/j.lwt.2005.06.015
Parker, J. L., and Shaw, J. G. (2011). Aeromonas spp. clinical microbiology and disease. J. Infect. 62, 109–118. doi: 10.1016/j.jinf.2010.12.003
Rasmussen-Ivey, C. R., Hossain, M. J., Odom, S. E., Terhune, J. S., Hemstreet, W. G., Shoemaker, C. A., et al. (2016). Classification of a hypervirulent Aeromonas hydrophila pathotype responsible for epidemic outbreaks in warm-water fishes. Front. Microbiol. 7:1615. doi: 10.3389/fmicb.2016.01615
Rico, A., Phu, T. M., Satapornvanit, K., Min, J., Shahabuddin, A., Henriksson, P. J., et al. (2013). Use of veterinary medicines, feed additives and probiotics in four major internationally traded aquaculture species farmed in Asia. Aquaculture 412, 231–243. doi: 10.1016/j.aquaculture.2013.07.028
Rico, A., and Van den Brink, P. J. (2014). Probabilistic risk assessment of veterinary medicines applied to four major aquaculture species produced in Asia. Sci. Total Environ. 468, 630–641. doi: 10.1016/j.scitotenv.2013.08.063
Ruiz-Navajas, Y., Viuda-Martos, M., Sendra, E., Perez-Alvarez, J., and Fernández-López, J. (2012). Chemical characterization and antibacterial activity of Thymus moroderi and Thymus piperella essential oils, two Thymus endemic species from southeast of Spain. Food Control 27, 294–299. doi: 10.1016/j.foodcont.2012.04.005
Sapkota, A., Sapkota, A. R., Kucharski, M., Burke, J., McKenzie, S., Walker, P., et al. (2008). Aquaculture practices and potential human health risks: current knowledge and future priorities. Environ. Int. 34, 1215–1226. doi: 10.1016/j.envint.2008.04.009
Settanni, L., Randazzo, W., Palazzolo, E., Moschetti, M., Aleo, A., Guarrasi, V., et al. (2014). Seasonal variations of antimicrobial activity and chemical composition of essential oils extracted from three Citrus limon L. Burm. cultivars. Nat. Prod. Res. 28, 383–391. doi: 10.1080/14786419.2013.871544
Shallcross, L. J., and Davies, S. C. (2014). The World Health Assembly resolution on antimicrobial resistance. J. Antimicrob. Chemother. 69, 2883–2885. doi: 10.1093/jac/dku346
Stratev, D., and Odeyemi, O. A. (2017). An overview of motile Aeromonas septicaemia management. Aquac. Int. 25, 1095–1105. doi: 10.1007/s10499-016-0100-3
Sureshkumar, M., Siswanto, D. Y., and Lee, C.-K. (2010). Magnetic antimicrobial nanocomposite based on bacterial cellulose and silver nanoparticles. J. Mater. Chem. 20, 6948–6955. doi: 10.1039/c0jm00565g
Trifan, A., Luca, S. V., Greige-Gerges, H., Miron, A., Gille, E., and Aprotosoaie, A. C. (2020). Recent advances in tackling microbial multidrug resistance with essential oils: Combinatorial and nano-based strategies. Crit. Rev. Microbiol. 46, 338–357. doi: 10.1080/1040841X.2020.1782339
Turgis, M., Han, J., Caillet, S., and Lacroix, M. (2009). Antimicrobial activity of mustard essential oil against Escherichia coli O157: H7 and Salmonella typhi. Food Control 20, 1073–1079. doi: 10.1016/j.foodcont.2009.02.001
Vivekanandhan, G., Hatha, A., and Lakshmanaperumalsamy, P. (2005). Prevalence of Aeromonas hydrophila in fish and prawns from the seafood market of Coimbatore. South India. Food Microbiol. 22, 133–137. doi: 10.1016/j.fm.2004.01.015
Vivekanandhan, G., Savithamani, K., Hatha, A., and Lakshmanaperumalsamy, P. (2002). Antibiotic resistance of Aeromonas hydrophila isolated from marketed fish and prawn of South India. Int. J. Food Microbiol. 76, 165–168. doi: 10.1016/S0168-1605(02)00009-0
Wang, Y., Hammes, F., De Roy, K., Verstraete, W., and Boon, N. (2010). Past, present and future applications of flow cytometry in aquatic microbiology. Trends Biotechnol. 28, 416–424. doi: 10.1016/j.tibtech.2010.04.006
Yang, S., He, T., Sun, J., and Sun, S. (2019). Distinct antimicrobial resistance profiling of clinically important Aeromonas spp. in southwest China: a seven-year surveillance study. Infect. Drug Resistance 12:2971. doi: 10.2147/IDR.S216926
Yardimci, B., and Aydin, Y. (2011). Pathological findings of experimental Aeromonas hydrophila infection in Nile tilapia (Oreochromis niloticus). Ank. Univ. Vet. Fak. Derg. 58, 47–54. doi: 10.1501/Vetfak_0000002448
Keywords: Citrus unshiu Marc. essential oil, chemical composition, antibacterial activity, Aeromonas hydrophila, mode of action
Citation: Zhong W, Chen K, Yang L, Tang T, Jiang S, Guo J and Gao Z (2022) Essential Oils From Citrus unshiu Marc. Effectively Kill Aeromonas hydrophila by Destroying Cell Membrane Integrity, Influencing Cell Potential, and Leaking Intracellular Substances. Front. Microbiol. 13:869953. doi: 10.3389/fmicb.2022.869953
Received: 05 February 2022; Accepted: 01 June 2022;
Published: 28 June 2022.
Edited by:
Miklos Fuzi, Independent Researcher, Budapest, HungaryReviewed by:
Tim W. Overton, University of Birmingham, United KingdomAjay Sharma, Delhi Pharmaceutical Sciences and Research University, India
Md. Amdadul Huq, Chung-Ang University, South Korea
Copyright © 2022 Zhong, Chen, Yang, Tang, Jiang, Guo and Gao. This is an open-access article distributed under the terms of the Creative Commons Attribution License (CC BY). The use, distribution or reproduction in other forums is permitted, provided the original author(s) and the copyright owner(s) are credited and that the original publication in this journal is cited, in accordance with accepted academic practice. No use, distribution or reproduction is permitted which does not comply with these terms.
*Correspondence: Jiajing Guo, guojiajing1986@163.com; Zhipeng Gao, gaozhipeng627@163.com
†These authors share first authorship