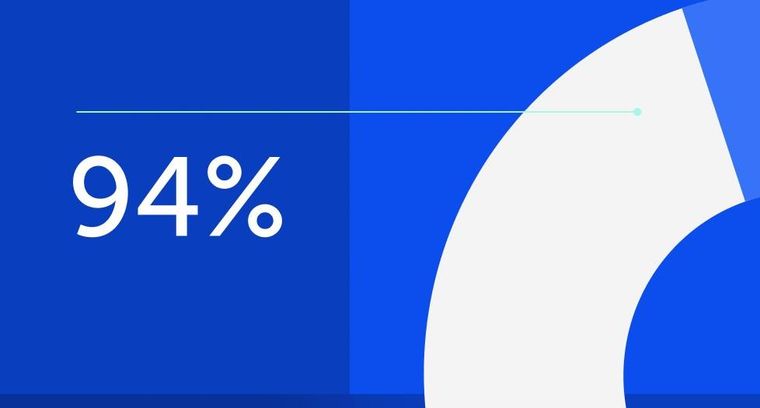
94% of researchers rate our articles as excellent or good
Learn more about the work of our research integrity team to safeguard the quality of each article we publish.
Find out more
ORIGINAL RESEARCH article
Front. Microbiol., 07 April 2022
Sec. Infectious Agents and Disease
Volume 13 - 2022 | https://doi.org/10.3389/fmicb.2022.866893
The diagnosis of orthopedic implant-associated infections (OIAIs) caused by the slow-growing anaerobic bacterium Cutibacterium acnes is challenging. The mild clinical presentations of this low-virulent bacterium along with its ubiquitous presence on human skin and human-dominated environments often make it difficult to differentiate true infection from contamination. Previous studies have applied C. acnes phylotyping as a potential avenue to distinguish contamination from infection; several studies reported a prevalence of phylotypes IB [corresponding to type H in the single-locus sequence typing (SLST) scheme] and II (SLST type K) in OIAIs, while a few others found phylotype IA1 (more specifically SLST type A) to be abundant. However, phylotype determination has mainly been done in a culture-dependent manner on randomly selected C. acnes isolates. Here, we used a culture-independent amplicon-based next-generation sequencing (aNGS) approach to determine the presence and relative abundances of C. acnes phylotypes in clinical OIAI specimens. As amplicon, the SLST target was used, a genomic fragment that is present in all C. acnes strains known to date. The aNGS approach was applied to 30 sonication fluid (SF) samples obtained from implants removed during revision surgeries, including 17 C. acnes culture-positive and 13 culture-negative SF specimens. In 53% of the culture-positive samples, SLST types were identified: relative abundances were highest for K-type C. acnes, followed by H- and D-type C. acnes. Other types, including A- and C-type C. acnes that are more prevalent on human skin, had low relative abundances. The aNGS results were compared with, and confirmed by a culture-dependent approach, which included the isolation, whole genome sequencing (WGS) and phylotyping of 36 strains of C. acnes obtained from these SF samples. Besides serving as a powerful adjunct to identify C. acnes phylotypes, the aNGS approach could also distinguish mono- from heterotypic infections, i.e., infections caused by more than one phylotype of C. acnes: in eight out of nine culture-positive SF samples multiple C. acnes types were detected. We propose that the aNGS approach, along with the patient’s clinical information, tissue and SF cultures and WGS, could help differentiate C. acnes contamination from true infection.
Cutibacterium acnes (C. acnes, formerly known as Propionibacterium acnes) is a slow-growing Gram-positive anaerobic bacterium (SGAB) that is found preferentially in the pilosebaceous glands of the human skin (McDowell et al., 2013; Aubin et al., 2014). It is increasingly being reported from various implant-associated infections, including orthopedic implant-associated infections (OIAI; Portillo et al., 2013; Achermann et al., 2014; Aubin et al., 2014; Ponraj et al., 2021). It is most frequently isolated from prosthetic joint infections (PJI) of the shoulder, but has also been implicated in OIAI of spine, hip, knee, elbow, and other joints (Sampedro et al., 2009; Bacle et al., 2017; Renz et al., 2018a; Namdari et al., 2019).
The diagnosis of C. acnes OIAI is not easy. The mild clinical presentation, muted immune responses to this low-virulent organism and the lack of a gold standard make the diagnosis challenging (Renz et al., 2018a; Pruijn et al., 2021). The ubiquitous nature of C. acnes on human skin and human-dominated environments increases the risk of contamination of diagnostic specimens during surgery and/or specimen processing. Some studies reported the recovery of C. acnes from deep tissues during primary surgery despite antibiotic prophylaxis and skin preparation with chlorhexidine and isopropyl alcohol (Matsen et al., 2015; Torrens et al., 2019). Moreover, C. acnes have been detected intracellularly within stromal cells and macrophages of intraarticular tissue samples of patients undergoing primary shoulder surgery, suggesting the possibility of it being an intraarticular commensal (Hudek et al., 2021). Thus, when C. acnes is isolated in patients without obvious clinical or laboratory features of OIAI, it is difficult to determine its clinical significance, and if it should be treated (Dorrestijn and Pruijn, 2021; Falstie-Jensen et al., 2021; Neufeld et al., 2021; Pruijn et al., 2021; Spek et al., 2021). There is therefore a risk of over- or undertreatment.
Cutibacterium acnes is a multiphyletic species with six distinct phylogenetic lineages, namely IA1, IA2, IB, IC, II, and III, based on multi-locus sequence typing (MLST) that has been applied in culture-dependent studies (McDowell et al., 2005, 2012; Kilian et al., 2012). In addition, to determine the population structure of C. acnes in culture-dependent and culture-independent studies, a single-locus sequence typing (SLST) scheme based on core genome phylogeny was developed that can distinguish 10 main SLST types (Scholz et al., 2014). The SLST types A–E represent phylotype IA1 and SLST types F–L correspond to phylotypes IA2, IC, IB, II, and III, respectively. Previous attempts to distinguish infection and contamination based on C. acnes phylotyping yielded conflicting results. In several studies, C. acnes strains of types IB (SLST type H) and II (SLST type K) were found to be overrepresented in suspected cases of OIAI (Sampedro et al., 2009; McDowell et al., 2013; Aubin et al., 2017; Salar-Vidal et al., 2021), while other studies found phylotype IA1 (in particular SLST type A and also SLST type D) to be more prevalent (El Sayed et al., 2019; Liew-Littorin et al., 2019). On the skin surface, strains of phylotype IA are usually predominant (McDowell et al., 2013; McLaughlin et al., 2019; Paetzold et al., 2019).
This study was conducted to evaluate the possibility of using a culture-independent phylotyping method on clinical specimens to identify OIAI-causing C. acnes phylotypes, namely amplicon-based next-generation sequencing (aNGS) using the SLST fragment as amplicon target. The method is based on the SLST amplicon sequencing approach developed by Scholz et al. (2014). The method was subsequently adapted for Illumina sequencing (Paetzold et al., 2019). Recently, a slightly modified approach, also adapted for Illumina sequencing, was used to determine the C. acnes phylotype distribution on skin of patients undergoing primary and revision shoulder arthroplasty surgeries (Hsu et al., 2020a). In addition, the study aimed at the distinction between monotypic infections, i.e., infections due to a single C. acnes phylotype only, and heterotypic infections. Previous studies, based on C. acnes cultivation and subsequent random selection of colonies, have suggested that C. acnes might be able to cause heterotypic infections (El Sayed et al., 2019; Bumgarner et al., 2020).
Here, implants removed during revision surgeries were collected and sonicated to maximize bacterial recovery. The obtained sonication fluid (SF) samples were cultured, and all C. acnes isolates were phylotyped and genome-sequenced. In addition, SF samples from 30 implants (17 culture-positive for C. acnes and 13 culture-negative) were subjected to aNGS to determine the presence and relative abundance of all C. acnes SLST types. A high relative abundance of strains belonging to H- and K-type C. acnes was found; these were detected in heterotypic infections. In addition, based on the combined evaluation of clinical and molecular methods our study suggests that only a fraction of C. acnes culture-positive cases represent true OIAIs.
Patients who underwent revision surgery between August 2019 and September 2020 at the Department of Orthopedic Surgery, Aarhus University Hospital, were included in the study. The removed orthopedic implants were collected, irrespective of the anatomic location (shoulder, knee, hip, elbow, and ankle), type of implant (plates, screws, liners, nails, and prostheses), or indication for revision (hardware irritation, dislocation of implant, suspected, or overt infection). Processing of all implants was performed at the Department of Biomedicine, Aarhus University. A priori, it was decided to include 100 implants in the study. At the discretion of the surgeons, for some patients, tissue samples for culture were sent to the Department of Clinical Microbiology, Aarhus University Hospital. Tissue culture results, when available, were accessed from the patient’s records. They were considered positive, if two or more of the five tissue cultures showed growth of the same bacteria. Informed consent was obtained from 85 patients to access data from the patient’s record. The study was registered with Region Midtjylland with reference number 661624. The Central Denmark Region ethical committee waived the need for ethical approval. Patient data were collected and managed using REDCap electronic data capture tools hosted at Aarhus University (Harris et al., 2019).
Orthopedic implants from patients included in the study were collected in the operating room (OR) during revision surgery performed under standard aseptic protocols for implant-associated surgical procedures, and directly placed in sterile, single-use, air-tight, plastic containers (LocknLock, South Korea) by the scrub nurse. If more than one implant was extracted from the same surgical site, they were placed in the same container. The implant was covered at least 90% with sterile saline before the container was sealed in the OR. The sealed containers were stored for maximum about 2 h at room temperature and at 4°C from 2 h to maximum about 24 h until retrieval and implant processing. All implants were subsequently processed by the same person.
The implants were processed by a previously described vortex-sonication method (Borens et al., 2013). In brief, the method included the following steps: 30 s of vigorous shaking of the sealed container with the implant and sterile saline, followed by 60 s of sonication at 100% power (BactoSonic, Bandelin electronic, Berlin, Germany) and another 30 s of vigorous shaking. Opening of the sealed containers and all further processing of SF were done inside a biological safety class II cabinet (ScanLaf class 2 cabinets: Mars, LaboGene) using standard biosafety level-2 laboratory practices. Separate sterile pipettes were used to harvest SF to two sterile tubes for use in culture-dependent and culture-independent investigations, respectively.
For culture-dependent analysis, aliquots of 100 μl of SF were inoculated on three different agar media: sheep blood agar (BA), fastidious anaerobic agar (FAA) with horse blood (Thermo Scientific™), and reinforced clostridial agar (RCA; Oxoid, Thermo Scientific™). In addition, the remaining SF was centrifuged at 10,000 g (rotor—F 13–14 × 50 Cy, Thermo Scientific™ Sorvall™ RC 6 Plus Centrifuge) for 20 min at 4°C. The pellet was resuspended in 1 ml remaining supernatant and aliquots of 10 and 1 μl of the resuspended pellet were inoculated on BA, FAA, and RCA plates. The plates were incubated anaerobically (Whitley A35 anaerobic workstation) for up to 28 days. The plates were checked for visible microbial growth daily in the first week and thereafter weekly. One additional BA plate inoculated with 100 μl of uncentrifuged SF was incubated aerobically for up to 3 days.
In case of visible bacterial growth, the colony-forming unit count (CFU/ml SF) was determined for each colony morphology type, since different colony morphologies could represent different C. acnes phylotypes. As per the latest guidelines of The European Bone and Joint Infection Society (EBJIS), growth of >50 CFU/ml from uncentrifuged SF and >200 CFU/ml from centrifuged SF was considered significant (McNally et al., 2021).
Bacterial colonies were subcultured on the same media as used for primary growth. Crude extract of bacterial DNA was prepared as previously described (Scholz et al., 2014). 16S rRNA gene amplification was performed using the B4 and B5 primers that amplify the V1–V3 region (Mikkelsen et al., 2000). A PCR reaction mixture of 25 μl containing 8 μl sterile PCR grade water, 2 μl primer mix (5 μmol each of B4 and B5 primers), 5 μl of the 1:100 diluted DNA, and 10 μl of 5Prime Hotmaster mix (Quanta Bio) was prepared. The following thermocycling scheme was used: 94°C for 5 min (1 cycle), 94°C for 1 min, 60°C for 1 min (30 cycles), 72°C for 2 min, and 72°C for 8 min (1 cycle). The PCR products were verified on agarose gels. Sequencing of the PCR products using the B4 and B5 primers was done at Eurofins Genomics (Ebersberg, Germany), and sequence comparison with the NR database at NCBI was done using blastn. A sequence identity >99% of the amplicon sequence with a database entry led to species assignment.
For genomic DNA extraction of C. acnes strains, the MasterPure™ Gram-Positive DNA Purification Kit (Lucigen) was used as per manufacturer’s instructions. Concentration and purity of the isolated DNA were first checked with a NanoDrop ND-1000 (Peqlab, Erlangen, Germany); concentrations were determined using the Qubit® dsDNA HS Assay Kit as recommended by the manufacturer (Life Technologies GmbH, Darmstadt, Germany). Illumina shotgun libraries were prepared using the Nextera XT DNA Sample Preparation Kit and subsequently sequenced on a MiSeq system using the v3 reagent kit with 600 cycles (Illumina, San Diego, CA, United States) as recommended by the manufacturer. Quality filtering was done with version 0.36 of Trimmomatic (Bolger et al., 2014). Assembly was performed with version 3.13.0 of the SPAdes genome assembler software (Bankevich et al., 2012). Version 2.2.1 of Qualimap was used to validate the assembly and determine the sequence coverage (García-Alcalde et al., 2012). In total, 36 C. acnes genomes were sequenced with a genome coverage of 31- to 225-fold (in average 139-fold), and all genome sequences were deposited in GenBank.
Gene prediction and annotation of all genomes were performed with PGAP (Tatusova et al., 2016). For phylogenomic analyses, the core genome was identified and aligned with the Parsnp program from the Harvest software package (Treangen et al., 2014). All C. acnes genomes available from GenBank (status October 2021, n = 286) were used along with the 36 C. acnes genomes from this study to build a core genome-based phylogeny. Reliable core genome single-nucleotide variants (SNVs) identified by Parsnp were used for the reconstruction of genome-based phylogeny. Phylogenetic trees were visualized using the Interactive Tree Of Life (Letunic and Bork, 2021). The SLST type assignment based on the genome sequence was done using the SLST assignment tool on http://medbac.dk/slst/pacnes.
For culture-independent analysis, 30 SF specimens were selected. They included all 17 samples that showed C. acnes growth in SF culture, as well as 13 SF specimens that were randomly chosen from implants that were SF culture-negative for C. acnes.
About 40 ml of SF per implant that was stored at −20°C until processing was thawed overnight at 4°C and then processed as follows: concentration of SF was done by centrifugation at 15,000 × g (rotor—F 13–14 × 50 Cy, Thermo Scientific™ Sorvall™ RC 6 Plus Centrifuge) for 1 h at 16°C. All but approximately 1 ml of the supernatant was discarded, and the pellet was resuspended in the remaining supernatant. DNA was extracted using the DNeasy PowerSoil Kit (QIAGEN, Hilden, Germany) following the manufacturer’s protocol. DNA concentrations were measured using the Qubit dsDNA HS Assay (ThermoFisher Scientific, Waltham, MA, United States) with a Qubit fluorometer following the manufacturer’s instructions.
The SLST fragment was amplified using the primers: 5′-TTGCTCGCAACTGCAAGCA-3′ and 5′-CCGGCTGGCAAATGAGGCAT-3′. PCR reaction mixtures were made in a total volume of 25 μl and comprised 5 μl of DNA sample, 2.5 μl AccuPrime PCR Buffer II (Invitrogen, Waltham, MA, United States), 1.5 μl of each primer (10 μM; DNA Technology, Risskov, Denmark), 0.15 μl AccuPrime Taq DNA Polymerase High Fidelity (Invitrogen, Waltham, MA, United States), and 14.35 μl of PCR grade water. The PCR reaction was performed using the following cycle conditions: initial denaturation at 94°C for 2 min, 35 cycles of denaturation at 94°C for 20 s, annealing at 55°C for 30 s, elongation at 68°C for 1 min, and final elongation step at 72°C for 5 min. PCR products were verified on an agarose gel and purified using the Qiagen Generead™ Size Selection kit (Qiagen, Hilden, Germany). The concentration of the purified PCR products was measured with a NanoDrop 2000 spectrophotometer (ThermoFisher Scientific, Waltham, MA, United States).
PCR products were used to attach indices and Illumina sequencing adapters using the Nextera XT Index kit (Illumina, San Diego, CA, United States). Index PCR was performed using 5 μl of template PCR product, 2.5 μl of each index primer, 12.5 μl of 2x KAPA HiFi HotStart ReadyMix, and 2.5 μl PCR grade water. The thermal cycling scheme was as follows: 95°C for 3 min, 8 cycles of 30 s at 95°C, 30 s at 55°C, and 30 s at 72°C, and a final extension at 72°C for 5 min. Quantification of the products was performed using the Quant-iT dsDNA HS assay kit (ThermoFisher Scientific, Waltham, MA, United States) and a Qubit fluorometer, following the manufacturer’s instructions. MagSi-NGSPREP Plus Magnetic beads (Steinbrenner Laborsysteme GmbH, Wiesenbach, Germany) were used for purification of the indexed products as recommended by the manufacturer, and normalization was performed using the Janus Automated Workstation from Perkin Elmer (Perkin Elmer, Waltham, MA, United States). Sequencing was conducted using an Illumina MiSeq platform with dual indexing and the MiSeq reagent kit v3 (600 cycles), as recommended by the manufacturer.
FASTQ sequences obtained after demultiplexing the reads and trimming the primers (Cutadapt v. 3.7; Martin, 2011) were imported into QIIME2 (v. 2021.2; Bolyen et al., 2019). Sequences with an average quality score lower than 20 or containing unresolved nucleotides were removed from the dataset. The paired-end reads were denoised and chimeras removed with DADA2 via q2-dada2 (Callahan et al., 2016), and a feature table was generated. These features were then clustered with VSEARCH (Rognes et al., 2016) using q2-vsearch (Rideout et al., 2014) at a threshold of 99% identity against the SLST allele database (accessible via: http://medbac.dk/slst/ pacnes: December 2021). Figures were prepared in R (v. 4.0.5) with the packages ggplot2 (v. 3.3.3) and gplots (v. 3.1.1).
A total of 100 implants from 99 patients were collected for this study. Shoulder prostheses were the most common implants, followed by knee and hip prostheses. In addition, plates and screws from these joints as well as elbow and ankle joints were also included in the study. The location and type of implants included in the study are shown in Supplementary Figure S1A. For 85 patients, we had access to their clinical files. The median age of these patients was 70 years (range 26–91 years) and majority of them were female (61.2%). Aseptic loosening (20.0%), aseptic failure (17.7%) and pain from plates/screws (14.1%) were the three most common reasons as defined by the surgeon responsible for implant removal, while OIAI or suspicion of OIAI was the reason for implant removal in 10.6%. Periprosthetic tissue biopsy samples were sent for culture to the Department of Clinical Microbiology at Aarhus University Hospital in 69 of 85 patients (81.2%), of whom 16 (23.2%) were tissue culture-positive. The demographic and clinical data of these patients are given in Supplementary Figure S1B.
All 100 removed implants were processed by the vortex-sonication method (Borens et al., 2013), and the resultant SF of the implants was subjected to culture-dependent and culture-independent analyses. An overview of the workflow and applied analyses is given in Figure 1.
Figure 1. Illustration of the workflow regarding 100 implants processed in this study. Hundred implants were first processed using the culture-dependent method. After obtaining these results, 30 SF specimens were selected (SF from 17 C. acnes culture-positive and 13 culture-negative implants) for culture-independent analysis. The final evaluation was based on the integration of patients’ clinical features, periprosthetic tissue culture results and results from the culture-dependent and culture-independent detection in SF. *: number of implants; **: number of bacterial isolates. SF, sonication fluid; WGS, whole genome sequencing; SLST, single-locus sequence typing; aNGS, amplicon-based next-generation sequencing.
Sonication fluid was cultivated under aerobic and anaerobic conditions on three different agar media. Visible bacterial growth was seen in SF from 44 implants (44%). Multiple colony morphology types, either on the same or different agar media were noted in SF culture of 26 implants. In total, 89 bacterial isolates were obtained from 44 culture-positive implants. All bacterial isolates were assigned to species level by 16S rRNA gene sequencing (Supplementary Table S1). In total, 42 isolates were assigned to C. acnes, derived from 21 implants; 10 of these implants showed growth of C. acnes isolates with more than one colony morphology. CFU counts were determined for each colony morphology type. Based on the EBJIS cut-off values (McNally et al., 2021), 23 of the 42 C. acnes isolates (54.8%) had significant CFU counts.
To investigate the C. acnes isolates in more detail, genome sequencing and SLST type assignment of the 42 isolates were performed. WGS data analysis revealed that five isolates from four implants had to be reclassified as Cutibacterium modestum (data not shown). This shows that the applied species assignment method, relying on 16S rRNA gene sequencing, is not optimal to differentiate C. acnes from C. modestum, as previously noted (Goldenberger et al., 2021). The remaining 36 C. acnes isolates from 17 implants were used in subsequent analyses. The details of the 36 C. acnes genomes, including size, coverage, and contig numbers as well as their SLST types are given in Supplementary Table S2.
The SLST type assignment for the 36 strains revealed that 14 isolates belonged to the K type (five K1, three K8, three K30, two K2, and one K7), eight isolates to the H type (six H1 and two H14), seven to A1, four to D1, two to C2, and one to F26 (Figure 2A). Multiple SLST types of C. acnes were isolated from five implants. Two implants harbored three SLST types each: K2, K1, and D1 in one implant and K8, H1, and K1 in another implant. Three implants were associated with two SLST types each: K1 and A1, A1 and K7, and H1 and D1, respectively.
Figure 2. Assignment of SLST types and core genome-based phylogeny of C. acnes isolates obtained in this study. (A) The SLST types of 36 C. acnes isolates are shown. Phylotype II (SLST type K) strains were most often found (39%), followed by strains of phylotypes IA1 (SLST types A, C, D; 36%), IB (SLST type H; 22%), and IA2 (SLST type F; 3%). (B) The core genome-based phylogeny of C. acnes is shown, using all genomes available at GenBank (n = 286; status October 2021) and the 36 C. acnes genomes sequenced here (labeled in red). The SLST types of the main phylogenetic clades are given as letters. A high-resolution version of the figure is available as Supplementary Figure S2.
Next, a phylogenetic analysis was conducted, based on SNVs in the aligned core genome. The phylogenetic tree showed the expected clustering of strains according to their determined SLST types (Figure 2B; Supplementary Figure S2). There was no obvious separation of the strains based on the anatomical location or type of implant. The analysis revealed that several strains were found to be clonal (Table 1); clonal strains were defined by less than 30 core genome-located SNVs. As expected, most clonal strains were from the same implant but isolated on different agar media. However, also two (SLST type) C2 strains, isolated from two different implants, were clonal. Similarly, four A1 strains were found to be clonal, even though they were all isolated from different implants. This raises the possibility that these C2 and A1 strains are potential contaminants that were acquired during specimen processing.
Table 1. Sequenced Cutibacterium acnes strains with clonal structure based on numbers of single-nucleotide variants (SNVs).
Next, we applied a previously developed aNGS approach that can detect all SLST types of C. acnes in a given sample and determine their relative abundances without the need for cultivation (Scholz et al., 2014). The first step of this approach is a PCR on DNA extracted from SF to amplify the SLST fragment, a genomic fragment that is present in all strains of C. acnes known to date. Around 30 SF specimens were selected, including all 17 samples that exhibited growth of C. acnes in SF culture, as well as 13 SF specimens that were randomly chosen from implants that were SF culture-negative for C. acnes. All but one of the 13 culture-negative implants (implants no. 18–30) were also negative for the SLST PCR, indicating that these culture-negative samples usually do not contain uncultivatable C. acnes or free DNA derived from C. acnes (Figure 3A).
Figure 3. Results of the culture-independent aNGS analysis on 30 sonication fluid specimens. (A) Around 30 samples were analyzed by aNGS, including 17 and 13 C. acnes culture-positive and -negative implants, respectively. The detected SLST types of the C. acnes strains obtained from the 17 culture-positive implants are listed. The SF of 10 implants was amplicon PCR-positive, the respective SLST types determined by aNGS are listed (relative abundances in %). (B) The relative abundances of all detected SLST types are shown from the 10 PCR-positive SF samples that were subjected to aNGS and subsequent SLST type assignment. (C) The average relative abundance for the main SLST types, determined by aNGS, was calculated across the 10 samples, showing that overall K-type C. acnes had the highest relative abundance, followed by H- and D-type C. acnes.
Only nine out of the 17 culture-positive implants were positive in the SLST PCR (53%). All amplicons were sequenced and the sequencing data statistics are given in Supplementary Table S3. SLST types H1 and D1 (average abundances 28.8 and 33.1%, respectively) were detected in six implants, while types A1 and K1 (average abundances 10.5 and 19.9%, respectively) were detected in five implants. The other SLST types were less frequently detected (Figure 3B). Summarizing the data according to the main phylotypes, K-type C. acnes (phylotype II) had in average the highest relative abundance (30.5%), followed by H-type (IB; 23.9%), D-type (IA1; 19.8%), F-type (IA2; 9.9%), A-type (IA1; 6.6%), and L-type C. acnes (III; 3.9%; Figure 3C).
Surprisingly, multiple SLST types were detected in all but one of the nine SF specimens that were PCR-positive, indicating that heterotypic infections might be common. In contrast, in the culture-dependent approach, multiple SLST types were isolated in only five of these nine implants. Regarding these five samples, the predominant SLST type detected by aNGS was also detected by SF culture and subsequent WGS, confirming that the culture-dependent and culture-independent methods are complementary (Figure 3A).
Eight out of the 17 C. acnes culture-positive implants were SLST PCR-negative (47%). This indicates that in these cases, C. acnes bacteria might have contaminated the sample after implant sonication, e.g., in the cultivation or incubation step. The SLST types of the C. acnes isolates in these eight implants included three A1, two H1, two C2, and one H14 strain (Figure 3A). Only one culture-negative implant was positive by SLST PCR (implant no. 18). This could be due to the presence of a strain that is difficult to culture or a potential contamination during the SLST PCR.
To further study the origin of the clonal strains and to assess if they could be derived from contamination, skin swabs for cultivation were taken from the person who processed the implants. Colonies that resembled C. acnes were isolated and SLST type assignment was done for 10 selected colonies. Strains belonging to the SLST types A1 and C2 were obtained and further genome-sequenced. Genome comparison of these strains with the C2- and A1-type strains obtained from several implants (no. 1, 2, 5, 6, 7, 8, and 17) showed that the A1- and C2-type C. acnes strains were clonal, as judged from the low number of SNVs in the core genome (data not shown). This strongly suggests that these A1 and C2 strains were indeed contaminants.
All obtained data of the 17 implants that were SF culture-positive for C. acnes, i.e., the details of the patients’ clinical features, SF and periprosthetic tissue culture results and aNGS results were combined and analyzed (Supplementary Table S4). Based on the combined data, we categorized the patient cases with the 17 implants in three groups (Table 2).
Table 2. Group 1: Infection unlikely, Group 2: Infection likely, and Group 3: Undetermined, respectively.
Eight implants were assigned to this group, including seven implants that were SF culture-positive but SLST PCR-negative (Table 2A). This indicated contamination after sonication, likely during the cultivation or incubation step. This group also contained the implants from which C2- (implant no. 1 and 2) and A1- (implant no. 5, 6, 7 and 8) type C. acnes strains were isolated; these were identified as clonal by WGS and subsequent genome comparison. It is likely that these contaminant strains originated from a single source, possibly from a source in the laboratory. In addition, SF specimens in this group had CFU counts that were below the EBJIS cut-off, or the C. acnes isolates took more than 14 days to grow. None of the patients had clinical features of OIAI, and tissue culture (if available) did not show any bacterial growth.
This group contained five implants, all of which were SF culture- and SLST PCR-positive (Table 2B). Three of them (implant no. 9, 10, and 13) had significant amounts of C. acnes in SF culture that grew within 7 days, as well as growth of C. acnes in tissue culture. Implant no. 12 had low growth of C. acnes in SF culture, but C. acnes was isolated from all five tissue biopsies. Regarding implant no. 11, tissue samples were not sent for culture, but SF culture revealed significant amounts of C. acnes SLST types D1 and H1, both of which were also detected in aNGS (relative abundance: D1, 69% and H1, 13%).
Four implants could not be clearly classified in either the “infection unlikely” or “infection likely” group (Table 2C). Three of them were considered possible infections (implant no. 14, 15, and 16). Tissue and SF cultures were positive for C. acnes in implant no. 14, but the SLST PCR was negative. This could potentially be due to a false negative result in the SLST PCR. Implant no. 15 had low growth of three SLST types of C. acnes, all of which were also detected by aNGS. However, no tissue biopsies were sent for culture. Implant no. 16 was a suspected case of OIAI, but none of the tissue cultures showed growth of C. acnes. SF culture showed low growth of C. acnes SLST type F26 and aNGS also showed the presence of F26 as the sole SLST type. Implant no. 17 was considered as a possible contamination. SF culture of implant no. 17 revealed two SLST types, K1 and A1. Only A1-type C. acnes showed significant growth, but it was not detected by aNGS, suggesting that this A1 strain was likely a contaminant.
The increasing awareness of OIAIs caused by C. acnes has fueled debate of whether C. acnes isolation from orthopedic implants and periprosthetic tissues represents true infection or contamination or commensal colonization (Hudek et al., 2014, 2021; Patel et al., 2020; Dorrestijn and Pruijn, 2021; Torrens et al., 2022). Here, we applied an aNGS approach that, in combination with other clinical and laboratory investigations, can potentially help to differentiate between C. acnes infection and contamination in cases of suspected OIAI.
In the current study, we included 100 implants, irrespective of the reason for implant removal. This was done to minimize the chance of missing any potential C. acnes infection that does not present with (classical) features of OIAI. Differentiation of C. acnes OIAI from non-infectious causes of implant failure based on clinical symptoms alone is difficult, as they often present with non-specific clinical symptoms like joint stiffness or pain (Achermann et al., 2014; Renz et al., 2018a).
The cultivation time has previously been stated as a criterion to differentiate C. acnes contaminants from infection-causing isolates (Butler-Wu et al., 2011; Frangiamore et al., 2015; El Sayed et al., 2021). In the culture-dependent part of our study, agar plates were incubated for up to 28 days. Cutibacterium acnes isolates from all five cases of the “infection likely” group as well as the three possible infection cases of the “undetermined” group grew within 7 days, with one exception. However, four of the eight C. acnes isolates from cases of the “infection unlikely” group took more than 13 days to grow. This is in agreement with the results of previous studies that used thresholds of 11 and 13 days, respectively: it was observed that all C. acnes true-positives grew within these time periods; in contrast, 21.7–44% of contaminants grew beyond the cut-off time limits (Butler-Wu et al., 2011; Frangiamore et al., 2015).
The CFU count of bacteria isolated from SF is another important criterion used to determine the clinical relevance of the bacterial isolate. While previous studies have used different cut-offs, the latest EBJIS guidelines suggested a threshold of >200 or >50 CFU/ml from centrifuged and uncentrifuged SF, respectively (McNally et al., 2021). However, these thresholds may not be applicable concerning SGAB like C. acnes. Two of the five cases in the “infection likely” as well as all three possible infection cases of the “undetermined” group were associated with CFU counts below the recommended thresholds, while two out of eight cases of the “infection unlikely” group showed growth above the CFU count thresholds. This suggests that the CFU count is not a reliable criterion regarding SGAB like C. acnes.
Several previous studies have noted that C. acnes phylotypes IB (SLST type H) and II (SLST type K) tend to be more predominant in OIAIs (Sampedro et al., 2009; McDowell et al., 2013; Aubin et al., 2017; Lee et al., 2020; Salar-Vidal et al., 2021). The present study shows similar results. Concerning the culture-dependent results, all five implants in the “infection likely” group showed growth of either C. acnes phylotypes IB or II, and one implant showing growth of both phylotypes. In contrast, there was a predominance of phylotype IA1 C. acnes in the cases of the “infection unlikely” group: six out of eight cases showed only growth of A1- and C2-type C. acnes strains. A similar result was also found by the aNGS analysis: among all identified C. acnes types the relative abundances are in average highest for K- and H-type C. acnes in the 10 SLST PCR-positive cases (Figure 3C). Moreover, aNGS identified K- and/or H-type C. acnes in all but one of the 10 SLST PCR-positive cases. In seven cases, K- or H-type C. acnes were the dominating types in terms of relative abundance. This could suggest that strains of phylotypes IB and II have a higher probability to cause OIAI, whereas strains belonging to phylotype IA1 (with the possible exception of D1-type C. acnes) are more likely to represent contaminants or, possibly, non-involved commensals. However, it remains to be experimentally proven if strains of phylotypes IB and II have a higher OIAI-causing potential. Moreover, the association of type IB/II phylotypes with OIAI might not be so evident, as a few studies have found conflicting results, and reported a predominance of phylotype IA1 in OIAI (El Sayed et al., 2019; Liew-Littorin et al., 2019). It should be noted here that previous studies almost exclusively applied phylotyping to C. acnes isolates obtained by culture-dependent methods. Thus, additional studies, especially those based on culture-independent analyses of C. acnes phylotypes, are needed before it can be evaluated if phylotyping is a useful and reliable method to differentiate infectious strains of C. acnes from contaminants. Another interesting result from the current study was the finding that C. acnes OIAI appears to primarily be a heterotypic infection, i.e., an infection that is caused by more than one phylotype/SLST type of C. acnes. In many cases of the “infection likely” and “undetermined” groups multiple C. acnes types were found. Out of the nine implants in these two groups, five had heterotypic growth on culture, while aNGS detected heterotypic C. acnes populations in eight cases. As previously reported, C. acnes strains belonging to different SLST types can show similar colony morphologies (Bumgarner et al., 2020). Thus, heterotypic C. acnes infections could easily be missed in routine clinical diagnosis based on culture-dependent methods alone, where single colonies are randomly selected for further analysis. Interestingly, a study has reported that mono- and heterotypic C. acnes OIAIs appear to be two different clinical entities with different clinical histories and immune responses (El Sayed et al., 2019). While current treatment for both types of infection is the same, it could potentially differ in the future.
The present study also shows that several C. acnes strains isolated from SF culture were contaminants. Cutibacterium acnes detected in clinical samples could originate from the patients’ own skin during surgical incision, as the bacteria have been proven to persist on skin, despite standard surgical skin preparation (Lee et al., 2014; Hsu et al., 2020b). It could also originate from the OR, as C. acnes has been detected by culture from swabs exposed to air in the OR (Namdari et al., 2020a). Interestingly, regarding the 17 samples that exhibited growth of C. acnes in SF culture, all samples derived from hip (n = 2) and knee (n = 3) specimens were SLST PCR-negative cases and assigned to the “infection unlikely” group. This could indicate a higher risk of culture-dependent contamination associated with hip and knee surgery compared to shoulder and elbow surgery. Contamination may also occur in the laboratory, despite adequate precautions as demonstrated in this present study. The high risk of contamination due to the ubiquity of this microorganism needs to be carefully considered when interpreting positive C. acnes cultures.
Our study has a few important limitations. First, the study included a limited number of implants, with only 17 being culture-positive for C. acnes. This was due to the prospective nature of the study. Secondly, histopathology to detect the presence of neutrophils, which is an important criterion for diagnosis of OIAI is not done in Denmark and hence could not be included in the final evaluation. Thirdly, intraoperative tissue biopsy specimens were only sent for culture in 70.6% (12 of 17) of the cases; moreover, C. acnes isolates from tissue culture were not available for further analyses, e.g., for comparison with the C. acnes strains cultured from SF.
Other culture-independent methods could be used in future studies to support the diagnosis of C. acnes OIAI, including multiplex PCR and species- or even phylotype-specific PCR approaches (Sampedro et al., 2010; Morgenstern et al., 2018; Renz et al., 2018b; Sigmund et al., 2019). Metagenomic NGS (mNGS), which is an untargeted approach unlike aNGS, has recently been applied. While some studies (Weaver et al., 2019) showed that mNGS was superior to standard culture, especially in polymicrobial infections, others have shown poor correlation between mNGS and culture (Namdari et al., 2020b). In summary, we applied an aNGS approach to identify all SLST types/phylotypes of C. acnes in SF specimens and determine their relative abundances, with the aim to distinguish contaminant strains of C. acnes from OIAI-causing isolates. Our study showed the advantage of using a combination of clinical, laboratory, and microbiological methods, including culture-dependent and culture-independent analyses. An important finding was that not all C. acnes culture-positive cases represented true infections. This can potentially prevent overdiagnosis and unnecessary antibiotic treatment for the patients. However, additional studies, including the use of aNGS on tissue biopsy samples, are needed before the conundrum of C. acnes in OIAI can be entirely resolved.
The datasets presented in this study can be found in online repositories. The names of the repository/repositories and accession number(s) can be found at: https://www.ncbi.nlm.nih.gov/bioproject/PRJNA769547 and https://www.ncbi.nlm.nih.gov/bioproject/PRJNA801471.
The studies involving human participants were reviewed and approved by Ethical Committee Region Midtjylland, Denmark. The patients/participants provided their written informed consent to participate in this study.
DSP, JL, TF-J, NPJ, CR, and HB contributed to the conception and design of the study. DSP performed wet lab benchwork and analyzed the data. AP and HB contributed to sequence data generation and analyses. DSP, JL, and HB wrote the manuscript. All authors contributed to the article and approved the submitted version.
This research was supported with funds from the A. P. Møller foundation (no. 30903) for running costs and the “Fabrikant Vilhelm Pedersen og Hustrus Legat” (by the recommendation from the Novo Nordisk Foundation) for running costs and personnel (no. 30658).
The authors declare that the research was conducted in the absence of any commercial or financial relationships that could be construed as a potential conflict of interest.
All claims expressed in this article are solely those of the authors and do not necessarily represent those of their affiliated organizations, or those of the publisher, the editors and the reviewers. Any product that may be evaluated in this article, or claim that may be made by its manufacturer, is not guaranteed or endorsed by the publisher.
The authors would like to thank the OR nurses from the Department of Orthopedic Surgery, Aarhus University Hospital, for help with collection of implants, Lise Hald Schultz for her excellent technical assistance and Michael Lund for help with bioinformatics analyses.
The Supplementary Material for this article can be found online at: https://www.frontiersin.org/articles/10.3389/fmicb.2022.866893/full#supplementary-material
Supplementary Figure S1 | Implant characteristics and patient data. (A) Around 100 implants were processed in this study, including different types of implants from different locations. (B) Demographic and clinical data of 85 patients are listed.
Supplementary Figure S2 | High-resolution figure of the core genome-based phylogeny of C. acnes obtained in this study. The core genome-based phylogeny of C. acnes is shown, using all genomes available at GenBank (n = 286; status June 2021) and the 36 C. acnes genomes sequenced here (in red).
aNGS, amplicon-based next-generation sequencing; CFU, colony-forming unit; OIAI, orthopedic implant-associated infection; MLST, multi-locus sequence typing; OR, operating room; PJI, prosthetic joint infection; SF, sonication fluid; SGAB, slow-growing Gram-positive anaerobic bacterium; SLST, single-locus sequence typing; SNV, single-nucleotide variant; WGS, whole genome sequencing.
Achermann, Y., Goldstein, E. J., Coenye, T., and Shirtliff, M. E. (2014). Propionibacterium acnes: from commensal to opportunistic biofilm-associated implant pathogen. Clin. Microbiol. Rev. 27, 419–440. doi: 10.1128/CMR.00092-13
Aubin, G. G., Lavigne, J. P., Foucher, Y., Delliere, S., Lepelletier, D., Gouin, F., et al. (2017). Tropism and virulence of Cutibacterium (formerly Propionibacterium) acnes involved in implant-associated infection. Anaerobe 47, 73–78. doi: 10.1016/j.anaerobe.2017.04.009
Aubin, G. G., Portillo, M. E., Trampuz, A., and Corvec, S. (2014). Propionibacterium acnes, an emerging pathogen: from acne to implant-infections, from phylotype to resistance. Med. Mal. Infect. 44, 241–250. doi: 10.1016/j.medmal.2014.02.004
Bacle, G., Sikora, S. K., and Ek, E. T. H. (2017). Propionibacterium acnes infection of a Metacarpophalangeal joint Arthroplasty. J. Hand. Surg. [Am.] 42, 394.e1–394.e6. doi: 10.1016/j.jhsa.2017.01.023
Bankevich, A., Nurk, S., Antipov, D., Gurevich, A. A., Dvorkin, M., Kulikov, A. S., et al. (2012). SPAdes: a new genome assembly algorithm and its applications to single-cell sequencing. J. Comput. Biol. 19, 455–477. doi: 10.1089/cmb.2012.0021
Bolger, A. M., Lohse, M., and Usadel, B. (2014). Trimmomatic: a flexible trimmer for Illumina sequence data. Bioinformatics 30, 2114–2120. doi: 10.1093/bioinformatics/btu170
Bolyen, E., Rideout, J. R., Dillon, M. R., Bokulich, N. A., Abnet, C. C., Al-Ghalith, G. A., et al. (2019). Reproducible, interactive, scalable and extensible microbiome data science using QIIME 2. Nat. Biotechnol. 37, 852–857. doi: 10.1038/s41587-019-0209-9
Borens, O., Yusuf, E., Steinrücken, J., and Trampuz, A. (2013). Accurate and early diagnosis of orthopedic device-related infection by microbial heat production and sonication. J. Orthop. Res. 31, 1700–1703. doi: 10.1002/jor.22419
Bumgarner, R. E., Harrison, D., and Hsu, J. E. (2020). Cutibacterium acnes isolates from deep tissue specimens retrieved during revision shoulder Arthroplasty: similar Colony morphology does not indicate Clonality. J. Clin. Microbiol. 58, e00121–e00119. doi: 10.1128/JCM.00121-19
Butler-Wu, S. M., Burns, E. M., Pottinger, P. S., Magaret, A. S., Rakeman, J. L., Matsen, F. A. 3rd, et al. (2011). Optimization of periprosthetic culture for diagnosis of Propionibacterium acnes prosthetic joint infection. J. Clin. Microbiol. 49, 2490–2495. doi: 10.1128/JCM.00450-11
Callahan, B. J., McMurdie, P. J., Rosen, M. J., Han, A. W., Johnson, A. J., and Holmes, S. P. (2016). DADA2: high-resolution sample inference from Illumina amplicon data. Nat. Methods 13, 581–583. doi: 10.1038/nmeth.3869
Dorrestijn, O., and Pruijn, N. (2021). Reply: low-grade Cutibacterium acnes shoulder infections do exist!: In response to the letter to the editor by Reinier WA Spek, job N Doornberg, David Ring and Michel PJ van den Bekerom. Should. Elb. 13, 151–153. doi: 10.1177/1758573220979906
El Sayed, F., Jeverica, S., Roux, A. L., Bauer, T., Nkam, L., Sivadon-Tardy, V., et al. (2021). Cutibacterium acnes clonal complexes display various growth rates in blood culture vials used for diagnosing orthopedic device-related infections. Anaerobe 72:102469. doi: 10.1016/j.anaerobe.2021.102469:102469
El Sayed, F., Roux, A. L., Sapriel, G., Salomon, E., Bauer, T., Gaillard, J. L., et al. (2019). Molecular typing of multiple isolates is essential to diagnose Cutibacterium acnes orthopedic device-related infection. Clin. Infect. Dis. 68, 1942–1945. doi: 10.1093/cid/ciy952
Falstie-Jensen, T., Lange, J., Daugaard, H., Sørensen, A. K. B., Ovesen, J., and Søballe, K. (2021). Unexpected positive cultures after revision shoulder arthroplasty: does it affect outcome? J. Shoulder Elb. Surg. 30, 1299–1308. doi: 10.1016/j.jse.2020.12.014
Frangiamore, S. J., Saleh, A., Grosso, M. J., Alolabi, B., Bauer, T. W., Iannotti, J. P., et al. (2015). Early versus late culture growth of Propionibacterium acnes in revision shoulder Arthroplasty. J. Bone Joint Surg. Am. 97, 1149–1158. doi: 10.2106/JBJS.N.00881
García-Alcalde, F., Okonechnikov, K., Carbonell, J., Cruz, L. M., Götz, S., Tarazona, S., et al. (2012). Qualimap: evaluating next-generation sequencing alignment data. Bioinformatics 28, 2678–2679. doi: 10.1093/bioinformatics/bts503
Goldenberger, D., Søgaard, K. K., Cuénod, A., Seth-Smith, H., de Menezes, D., Vandamme, P., et al. (2021). Cutibacterium modestum and “Propionibacterium humerusii” represent the same species that is commonly misidentified as Cutibacterium acnes. Antonie Van Leeuwenhoek 114, 1315–1320. doi: 10.1007/s10482-021-01589-5
Harris, P. A., Taylor, R., Minor, B. L., Elliott, V., Fernandez, M., O’Neal, L., et al. (2019). The REDCap consortium: building an international community of software platform partners. J. Biomed. Inform. 95:103208. doi: 10.1016/j.jbi.2019.103208
Hsu, J. E., Matsen, F. A. 3rd, Whitson, A. J., and Bumgarner, R. E. (2020a). Cutibacterium subtype distribution on the skin of primary and revision shoulder arthroplasty patients. J. Shoulder Elb. Surg. 29, 2051–2055. doi: 10.1016/j.jse.2020.02.007
Hsu, J. E., Whitson, A. J., Woodhead, B. M., Napierala, M. A., Gong, D., and Matsen, F. A. 3rd. (2020b). Randomized controlled trial of chlorhexidine wash versus benzoyl peroxide soap for home surgical preparation: neither is effective in removing Cutibacterium from the skin of shoulder arthroplasty patients. Int. Orthop. 44, 1325–1329. doi: 10.1007/s00264-020-04594-x
Hudek, R., Brobeil, A., Brüggemann, H., Sommer, F., Gattenlöhner, S., and Gohlke, F. (2021). Cutibacterium acnes is an intracellular and intra-articular commensal of the human shoulder joint. J. Shoulder Elb. Surg. 30, 16–26. doi: 10.1016/j.jse.2020.04.020
Hudek, R., Sommer, F., Kerwat, M., Abdelkawi, A. F., Loos, F., and Gohlke, F. (2014). Propionibacterium acnes in shoulder surgery: true infection, contamination, or commensal of the deep tissue? J. Shoulder Elb. Surg. 23, 1763–1771. doi: 10.1016/j.jse.2014.05.024
Kilian, M., Scholz, C. F., and Lomholt, H. B. (2012). Multilocus sequence typing and phylogenetic analysis of Propionibacterium acnes. J. Clin. Microbiol. 50, 1158–1165. doi: 10.1128/JCM.r06129-11
Lee, J., Greenwood Quaintance, K. E., Schuetz, A. N., Shukla, D. R., Cofield, R. H., Sperling, J. W., et al. (2020). Correlation between hemolytic profile and phylotype of Cutibacterium acnes (formerly Propionibacterium acnes) and orthopedic implant infection. Should. Elb. 12, 390–398. doi: 10.1177/1758573219865884
Lee, M. J., Pottinger, P. S., Butler-Wu, S., Bumgarner, R. E., Russ, S. M., and Matsen, F. A. 3rd. (2014). Propionibacterium persists in the skin despite standard surgical preparation. J. Bone Joint Surg. Am. 96, 1447–1450. doi: 10.2106/JBJS.M.01474
Letunic, I., and Bork, P. (2021). Interactive tree Of life (iTOL) v5: an online tool for phylogenetic tree display and annotation. Nucleic Acids Res. 49, W293–W296. doi: 10.1093/nar/gkab301
Liew-Littorin, C., Brüggemann, H., Davidsson, S., Nilsdotter-Augustinsson, Å., Hellmark, B., and Söderquist, B. (2019). Clonal diversity of Cutibacterium acnes (formerly Propionibacterium acnes) in prosthetic joint infections. Anaerobe 59, 54–60. doi: 10.1016/j.anaerobe.2019.04.011
Martin, M. (2011). Cutadapt removes adapter sequences from high-throughput sequencing reads. EMBnet J. 17, 10–12. doi: 10.14806/ej.17.1.200
Matsen, F. A., Russ, S. M., Bertelsen, A., Butler-Wu, S., and Pottinger, P. S. (2015). Propionibacterium can be isolated from deep cultures obtained at primary arthroplasty despite intravenous antimicrobial prophylaxis. J. Shoulder Elb. Surg. 24, 844–847. doi: 10.1016/j.jse.2014.10.016
McDowell, A., Barnard, E., Nagy, I., Gao, A., Tomida, S., Li, H., et al. (2012). An expanded multilocus sequence typing scheme for Propionibacterium acnes: investigation of ‘pathogenic’, ‘commensal’ and antibiotic resistant strains. PLoS One 7:e41480. doi: 10.1371/journal.pone.0041480
McDowell, A., Nagy, I., Magyari, M., Barnard, E., and Patrick, S. (2013). The opportunistic pathogen Propionibacterium acnes: insights into typing, human disease, clonal diversification and CAMP factor evolution. PLoS One 8:e70897. doi: 10.1371/journal.pone.0070897
McDowell, A., Valanne, S., Ramage, G., Tunney, M. M., Glenn, J. V., McLorinan, G. C., et al. (2005). Propionibacterium acnes types I and II represent phylogenetically distinct groups. J. Clin. Microbiol. 43, 326–334. doi: 10.1128/JCM.43.1.326-334.2005
McLaughlin, J., Watterson, S., Layton, A. M., Bjourson, A. J., Barnard, E., and McDowell, A. (2019). Propionibacterium acnes and acne vulgaris: new insights from the integration of population genetic, multi-Omic. Biochem. Host Microbe Stud. Microorgan. 7:128. doi: 10.3390/microorganisms7050128
McNally, M., Sousa, R., Wouthuyzen-Bakker, M., Chen, A. F., Soriano, A., Vogely, H. C., et al. (2021). The EBJIS definition of periprosthetic joint infection. Bone Joint J. 103-b, 18–25. doi: 10.1302/0301-620X.103B1.BJJ-2020-1381.R1
Mikkelsen, L., Theilade, E., and Poulsen, K. (2000). Abiotrophia species in early dental plaque. Oral Microbiol. Immunol. 15, 263–268. doi: 10.1034/j.1399-302x.2000.150409.x
Morgenstern, C., Cabric, S., Perka, C., Trampuz, A., and Renz, N. (2018). Synovial fluid multiplex PCR is superior to culture for detection of low-virulent pathogens causing periprosthetic joint infection. Diagn. Microbiol. Infect. Dis. 90, 115–119. doi: 10.1016/j.diagmicrobio.2017.10.016
Namdari, S., Nicholson, T., Abboud, J., Lazarus, M., Ramsey, M. L., Williams, G., et al. (2019). Comparative study of cultures and next-generation sequencing in the diagnosis of shoulder prosthetic joint infections. J. Shoulder Elb. Surg. 28, 1–8. doi: 10.1016/j.jse.2018.08.048
Namdari, S., Nicholson, T., Abboud, J., Lazarus, M., Ramsey, M. L., Williams, G., et al. (2020b). Cutibacterium acnes is less commonly identified by next-generation sequencing than culture in primary shoulder surgery. Should. Elb. 12, 170–177. doi: 10.1177/1758573219842160
Namdari, S., Nicholson, T., and Parvizi, J. (2020a). Cutibacterium acnes is isolated from air swabs: time to doubt the value of traditional cultures in shoulder surgery? Arch. Bone Joint. Surg. 8, 506–510. doi: 10.22038/abjs.2020.40642.2095
Neufeld, M. E., Lanting, B. A., Shehata, M., Howard, J. L., MacDonald, S. J., Teeter, M. G., et al. (2021). Prevalence and outcomes of unexpected positive intraoperative cultures in presumed aseptic revision hip arthroplasty. Bone Joint Surg. Am. 103, 1392–1401. doi: 10.2106/JBJS.20.01559
Paetzold, B., Willis, J. R., Pereira de Lima, J., Knödlseder, N., Brüggemann, H., Quist, S. R., et al. (2019). Skin microbiome modulation induced by probiotic solutions. Microbiome 7:95. doi: 10.1186/s40168-019-0709-3
Patel, M. S., Singh, A. M., Gregori, P., Horneff, J. G., Namdari, S., and Lazarus, M. D. (2020). Cutibacterium acnes: a threat to shoulder surgery or an orthopedic red herring? J. Shoulder Elb. Surg. 29, 1920–1927. doi: 10.1016/j.jse.2020.02.020
Ponraj, D. S., Falstie-Jensen, T., Jørgensen, N. P., Ravn, C., Brüggemann, H., and Lange, J. (2021). Diagnosis of orthopaedic-implant-associated infections caused by slow-growing gram-positive anaerobic bacteria—a clinical perspective. J. Bone Joint Infect. 6, 367–378. doi: 10.5194/jbji-6-367-2021
Portillo, M. E., Corvec, S., Borens, O., and Trampuz, A. (2013). Propionibacterium acnes: an underestimated pathogen in implant-associated infections. Biomed. Res. Int. 2013:804391. doi: 10.1155/2013/804391
Pruijn, N., Schuncken, A. C., Kosse, N. M., Hofstad, C. J., and Dorrestijn, O. (2021). Pre- and peroperative diagnosis of Cutibacterium acnes infections in shoulder surgery: a systematic review. Should. Elb. 13, 131–148. doi: 10.1177/1758573220913243
Renz, N., Cabric, S., Morgenstern, C., Schuetz, M. A., and Trampuz, A. (2018b). Value of PCR in sonication fluid for the diagnosis of orthopedic hardware-associated infections: has the molecular era arrived? Injury 49, 806–811. doi: 10.1016/j.injury.2018.02.018
Renz, N., Mudrovcic, S., Perka, C., and Trampuz, A. (2018a). Orthopedic implant-associated infections caused by Cutibacterium spp.—A remaining diagnostic challenge. PLoS One 13:e0202639. doi: 10.1371/journal.pone.0202639
Rideout, J. R., He, Y., Navas-Molina, J. A., Walters, W. A., Ursell, L. K., Gibbons, S. M., et al. (2014). Subsampled open-reference clustering creates consistent, comprehensive OTU definitions and scales to billions of sequences. PeerJ 2:e545. doi: 10.7717/peerj.545
Rognes, T., Flouri, T., Nichols, B., Quince, C., and Mahé, F. (2016). VSEARCH: a versatile open source tool for metagenomics. PeerJ 4:e2584. doi: 10.7717/peerj.2584
Salar-Vidal, L., Achermann, Y., Aguilera-Correa, J. J., Poehlein, A., Esteban, J., Brüggemann, H., et al. (2021). Genomic analysis of Cutibacterium acnes strains isolated from prosthetic joint infections. Microorganisms 9:1500. doi: 10.3390/microorganisms9071500
Sampedro, M. F., Huddleston, P. M., Piper, K. E., Karau, M. J., Dekutoski, M. B., Yaszemski, M. J., et al. (2010). A biofilm approach to detect bacteria on removed spinal implants. Spine 35, 1218–1224. doi: 10.1097/BRS.0b013e3181c3b2f3
Sampedro, M. F., Piper, K. E., McDowell, A., Patrick, S., Mandrekar, J. N., Rouse, M. S., et al. (2009). Species of Propionibacterium and Propionibacterium acnes phylotypes associated with orthopedic implants. Diagn. Microbiol. Infect. Dis. 64, 138–145. doi: 10.1016/j.diagmicrobio.2009.01.024
Scholz, C. F., Jensen, A., Lomholt, H. B., Brüggemann, H., and Kilian, M. (2014). A novel high-resolution single locus sequence typing scheme for mixed populations of Propionibacterium acnes in vivo. PLoS One 9:e104199. doi: 10.1371/journal.pone.0104199
Sigmund, I. K., Windhager, R., Sevelda, F., Staats, K., Puchner, S. E., Stenicka, S., et al. (2019). Multiplex PCR Unyvero i60 ITI application improves detection of low-virulent microorganisms in periprosthetic joint infections. Int. Orthop. 43, 1891–1898. doi: 10.1007/s00264-018-4136-z
Spek, R. W., Doornberg, J. N., Ring, D., and van den Bekerom, M. P. (2021). Can surgeons differentiate between painful shoulders that grow Cutibacterium acnes and infection benefitting from treatment? Should. Elb. 13, 149–150. doi: 10.1177/1758573220979905
Tatusova, T., DiCuccio, M., Badretdin, A., Chetvernin, V., Nawrocki, E. P., Zaslavsky, L., et al. (2016). NCBI prokaryotic genome annotation pipeline. Nucleic Acids Res. 44, 6614–6624. doi: 10.1093/nar/gkw569
Torrens, C., Bellosillo, B., Gibert, J., Alier, A., Santana, F., Prim, N., et al. (2022). Are Cutibacterium acnes present at the end of primary shoulder prosthetic surgeries responsible for infection? Prospective study. Eur. J. Clin. Microbiol. Infect. Dis. 41, 169–173. doi: 10.1007/s10096-021-04348-6
Torrens, C., Mari, R., Alier, A., Puig, L., Santana, F., and Corvec, S. (2019). Cutibacterium acnes in primary reverse shoulder arthroplasty: from skin to deep layers. J. Shoulder Elb. Surg. 28, 839–846. doi: 10.1016/j.jse.2018.10.016
Treangen, T. J., Ondov, B. D., Koren, S., and Phillippy, A. M. (2014). The harvest suite for rapid core-genome alignment and visualization of thousands of intraspecific microbial genomes. Genome Biol. 15:524. doi: 10.1186/s13059-014-0524-x
Weaver, A. A., Hasan, N. A., Klaassen, M., Karathia, H., Colwell, R. R., and Shrout, J. D. (2019). Prosthetic joint infections present diverse and unique microbial communities using combined whole-genome shotgun sequencing and culturing methods. J. Med. Microbiol. 68, 1507–1516. doi: 10.1099/jmm.0.001068
Keywords: Cutibacterium acnes, orthopedic implant-associated infections, prosthetic joint infections, single-locus sequence typing, amplicon-based next-generation sequencing, sonication fluid
Citation: Ponraj DS, Lange J, Falstie-Jensen T, Jørgensen NP, Ravn C, Poehlein A and Brüggemann H (2022) Amplicon-Based Next-Generation Sequencing as a Diagnostic Tool for the Detection of Phylotypes of Cutibacterium acnes in Orthopedic Implant-Associated Infections. Front. Microbiol. 13:866893. doi: 10.3389/fmicb.2022.866893
Received: 31 January 2022; Accepted: 21 March 2022;
Published: 07 April 2022.
Edited by:
Jozsef Soki, University of Szeged, HungaryReviewed by:
Roger Eugene Bumgarner, University of Washington, United StatesCopyright © 2022 Ponraj, Lange, Falstie-Jensen, Jørgensen, Ravn, Poehlein and Brüggemann. This is an open-access article distributed under the terms of the Creative Commons Attribution License (CC BY). The use, distribution or reproduction in other forums is permitted, provided the original author(s) and the copyright owner(s) are credited and that the original publication in this journal is cited, in accordance with accepted academic practice. No use, distribution or reproduction is permitted which does not comply with these terms.
*Correspondence: Holger Brüggemann, YnJ1ZWdnZW1hbm5AYmlvbWVkLmF1LmRr
Disclaimer: All claims expressed in this article are solely those of the authors and do not necessarily represent those of their affiliated organizations, or those of the publisher, the editors and the reviewers. Any product that may be evaluated in this article or claim that may be made by its manufacturer is not guaranteed or endorsed by the publisher.
Research integrity at Frontiers
Learn more about the work of our research integrity team to safeguard the quality of each article we publish.