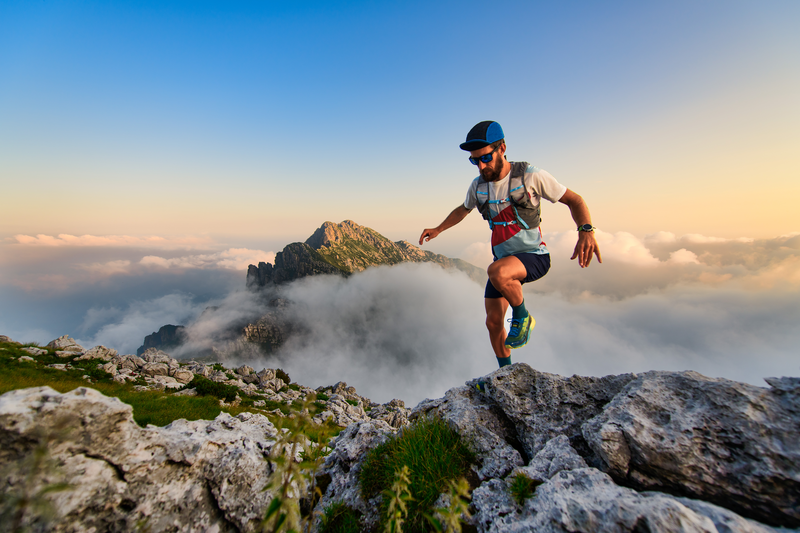
94% of researchers rate our articles as excellent or good
Learn more about the work of our research integrity team to safeguard the quality of each article we publish.
Find out more
ORIGINAL RESEARCH article
Front. Microbiol. , 23 June 2022
Sec. Antimicrobials, Resistance and Chemotherapy
Volume 13 - 2022 | https://doi.org/10.3389/fmicb.2022.866674
This article is part of the Research Topic From Genomics to Antibiotic Resistance in Emerging Pathogens View all 10 articles
This study aimed to characterize the changes in fecal carriage of Extended-Spectrum β-Lactamase (ESBL) producing Enterobacterales (ESBL-PE) in a single Dutch veal calves. During the rearing period at the Dutch veal farm, a decrease in fecal carriage of cefotaxime-resistant Escherichia coli isolates was observed after 2 weeks at the veal farm, while an increase of cefotaxime-resistant Klebsiella pneumoniae isolates was demonstrated. E. coli and K. pneumoniae were isolated from rectal swabs collected from 110 veal calves in week 2, 6, 10, 18, and 24 after their arrival at the farm. ESBL-PE isolates were selectively cultured and identified by MALDI-TOF. ESBL genes were characterized by RT-PCR, PCRs, and amplicon sequencing. A total of 80 E. coli and 174 K. pneumoniae strains were isolated from 104 out of 110 veal calves. The prevalence of ESBL-E. coli decreased from week 2 (61%) to week 6 (7%), while an unexpected increase in ESBL-K. pneumoniae colonization was detected in week 6 (80%). The predominant ESBL genes detected in E. coli isolates were blaCTX-M-15 and the non-ESBL gene blaTEM-1a, while in K. pneumoniae blaCTX-M-14 gene was detected in all isolates. Four cefotaxime-resistant K. pneumoniae isolates were randomly selected and characterized in deep by transformation, PCR-based replicon typing, and whole-genome sequencing (WGS). The clonal relatedness of a subgroup of nine animals carrying K. pneumoniae ESBL genes was investigated by Multi Locus sequence typing (MLST). In four ESBL-K. pneumoniae isolates, blaCTX-M-14 was located on IncFIIK and IncFIINK plasmid replicons and the isolates were multi-drug resistant (MDR). MLST demonstrated a clonal spread of ESBL-K. pneumoniae ST107. To the best of our knowledge, this is the first study to report a change in fecal carriage of ESBL-PE over time in the same veal calf during the rearing period.
Fecal carriage of antibiotic resistant-bacteria represents an important reservoir for the transmission and dissemination of resistance genes within and between commensal bacteria and to pathogens (Munk et al., 2018). Extended-spectrum β-lactamase (ESBLs) producing Enterobacterales (ESBL-PE) constitutes an important group of multidrug-resistant bacteria reported all over the world in humans and animals (Bush and Fisher, 2011). Over the past years, the potential role of food-producing animals as a reservoir of ESBL genes has been described (European Food Safety Authority (EFSA), 2011; Liebana et al., 2013; Schmid et al., 2013). Moreover, food products contaminated by ESBL-PE have also been identified as a source for the dissemination of antibiotic-resistant bacteria to humans through food consumption and/or manipulation (Overdevest et al., 2011). Likewise, plasmid similarities between ESBL-PE isolates obtained from humans and food-producing animals have been described (Kurittu et al., 2021).
The Enterobacterales family inhabit the gastrointestinal tract of humans and several animal species in a symbiotic relationship. Members of this family, particularly Escherichia coli and Klebsiella pneumoniae, are commonly associated with a variety of severe infections in humans and animals. In dairy cattle and veal calves, these bacteria can cause mastitis as well as respiratory and gastrointestinal infections (Schukken et al., 2012).
In dairy and veal farms, the use of antimicrobials, particularly third- and fourth-generation cephalosporin β-lactam antibiotics, provides a selective pressure for the emergence of resistant bacteria and the increase of antibiotic resistance by the production of ESBLs (Liebana et al., 2013). The usage of these antimicrobials was greatly reduced in the Netherlands which has led to a reduction in the prevalence of ESBLs (NETMAP_MARAN, 2021). The ESBL genes are commonly located on mobile elements including plasmids, facilitating the dissemination of the antibiotic resistance genes between bacteria (Rozwandowicz et al., 2018). Plasmid-encoded ESBL enzymes inactivate a large variety of β-lactam antibiotics including third-generation cephalosporins such as cefotaxime. The CTX-M family is the most predominant ESBL in Enterobacterales isolates from livestock in Europe (Horton et al., 2011; D’Andrea et al., 2013; Waade et al., 2021). In the Netherlands, the CTX-M-1 group (mainly blaCTX-M-1 and blaCTX-M-15) and CTX-M-9 group (mainly blaCTX-M-14) are the most common ESBLs genes identified in E. coli isolates obtained from veal calves (NETMAP_MARAN, 2021).
In a previous study, it was reported that usage of antimicrobials, differences in farm management practices, and the environment contribute to the selection and co-selection of antibiotic resistance in veal calves (Hordijk et al., 2013). In the production system in the Netherlands, veal calves are collected from different dairy farms and mixed before they are distributed among veal farms which provides an ideal scenario for the acquisition and transmission of antibiotic-resistant bacteria among the population of calves. During the rearing period at the veal farm, fecal shedding facilitates the dissemination of antibiotic-resistant bacteria including potential pathogens such as K. pneumoniae (Genomic Epidemiology Organization Server, n.d.).
The majority of the studies on the occurrence and prevalence of ESBL-K. pneumoniae in dairy cattle and veal calves have been confined within raw milk, food products, and bovine mastitis cases (Dahmen et al., 2013; Diab et al., 2017). Nevertheless, CTX-M genes (blaCTX-M-1, blaCTX-M-15, and blaCTX-M-14) detected in diseased calves have also been reported in healthy calves (Pubmlst Server, n.d.; Tshitshi et al., 2020). Despite that, limited data are available on the prevalence of fecal carriage of ESBL-K. pneumoniae in veal farms during the rearing period.
Between March 2019 and May 2020, we conducted a large longitudinal study on the prevalence of ESBL-E. coli in Netherlands. Rectal swabs were collected from calves born in 13 dairy farms and subsequently transported to 8 veal farms across the country where the animals were followed until slaughter. Samples were collected before transportation of the animals from the dairy farm to the veal farm and subsequently at five different time points at the veal farm (2, 6, 10, 18, and 24 weeks; manuscript submitted). In one particular veal farm, a decrease in fecal carriage of cefotaxime-resistant E. coli isolates was observed 2 weeks after the arrival of calves at the veal farm, while an increase of cefotaxime-resistant K. pneumoniae isolates was demonstrated from week 6 until slaughter.
In the present study, we aimed to: (a) identify the resistance genes in 80 cefotaxime-resistant E. coli and 174 cefotaxime-resistant K. pneumoniae isolates from veal calves obtained during the rearing period in one particular veal farm; (b) follow-up the fecal carriage of ESBL-K. pneumoniae isolates from a subgroup of nine animals and one animal carrying ESBL-E. coli over time, to identify the clonal relatedness between the isolates recovered, and (c) identify the mobile elements present in four ESBL-K. pneumoniae isolates randomly selected by whole-genome sequencing (WGS)-based analyses.
A total of 80 E. coli and 174 cefotaxime-resistant K. pneumoniae isolates were identified from 104 out of 110 calves in week 2, 6, 10, 18, and 24 during the rearing period at the veal farm, see Marcato et al. (2022) for the complete experimental setup. The remaining six animals were culture negative during all time points. In brief, rectal swabs were placed in 3 ml of Buffer Peptone Water (BPW; Becton Dickinson GmbH, Heidelberg, Germany) and incubated overnight at 37°C. After incubation, an aliquot (10 ul) of the enriched solution was plated on MacConkey agar plates with 1 mg/L of cefotaxime and incubated overnight at 44°C (European Reference Laboratory-Antimicrobial Resistance, n.d.).1 A single random pink colony was streaked onto Heart Infusion Agar (HIS; Becton Dickinson GmbH, Heidelberg, Germany) supplemented with 5% sheep blood and incubated at 37°C for 24 h to obtain a pure culture. Bacterial isolates were subsequently identified by Matrix-Assisted Laser Desorption Ionization-Time of Light mass spectrometry (MALDI-TOF MS; Bruker Daltonik, Germany). All the isolates were preserved at −80°C for further analysis.
The E. coli and cefotaxime-resistant K. pneumoniae isolates were further analyzed by a Real-time PCR assay on a light cycler System (Applied Biosystems, 7500 Fast Real-Time PCR System) for the detection of ESBL genes blaCTX-M-1 group, blaCMY, blaTEM, and blaSHV as previously described, using bacteria cell boiled lysate method as DNA template (Geurts et al., 2017; Veldman et al., 2018). In case of negative results, single PCRs for blaCTX-M-2 group, blaCTX-M-8/25, blaCTX-M-9 group and chromosomal blaAmpC were performed (Dierikx et al., 2012; Liakopoulos et al., 2016a). Well-defined strains with known ESBLs genes were included as positive controls in the PCR assays. The identification of the ESBL detected by PCR was confirmed by DNA Sanger sequencing using the PCR product by QIAquick® PCR Purification kit (Qiagen®). Subsequently, the PCR product was purified using Sephadex (Merck) and used for the DNA Sanger sequencing (3130 Genetic Analyzer) as previously described (Liakopoulos et al., 2016a). The sequences were compared with reference sequences obtained from GenBank using the Sequencher 5.4.6 software.
Antimicrobial susceptibility tests of four cefotaxime-resistant K. pneumoniae isolates randomly selected from the earliest and latest time point possible (n = 2 week 6 and n = 2 week 24) were tested by broth microdilution using standard European antibiotic panels EUVSEC and EUVSEC2 (Thermo Fisher, “Sensititre™ Gram-Negative MIC Plate” n.d.) (World Health Organization (WHO), n.d.). E. coli ATCC 25922 was used as a control reference strain. The results were interpreted using the EUCAST ECOFFs (v7.1),2 in case epidemiological cut-off values (ECOFFs) for K. pneumoniae were lacking, we used E. coli ECOFFs for the interpretation. The K. pneumoniae isolates, two from week 6 and one from week 24, were obtained from animals that were initially colonized with ESBL-E. coli at week 2 carrying blaCTX_M-15 and non-ESBL blaTEM-1a gene, while the additional K. pneumoniae isolates included from week 24 were colonized with K. pneumoniae in week 6 and 10 and negative culture in week 2.
The same four ESBL-K. pneumoniae isolates used for antimicrobial susceptibility testing were characterized in depth using molecular methods. Plasmids carrying ESBLs genes were extracted from pure culture using a miniprep method and transformed by electroporation into competent DH10B cells (Thermo Scientific, United States) as previously described (Liakopoulos et al., 2016a). The obtained transformants were selected on Luria Bertani (LB) agar plates supplemented with cefotaxime (1 mg/L) and confirmed for the presence of the ESBL gene using PCR. The plasmid typing was performed using the PCR-Based Replicon Typing (PBRT) 2.0 Kit (DIATHEVA, Fano, Italy) as previously described (Carattoli et al., 2005). To confirm the location of the identified genes on the plasmids in the four K. pneumoniae selected isolates, WGS was performed. The K. pneumoniae DNA was isolated and purified using the Qiagen Blood and tissue DNA isolation kit, and DNA concentration was measured with a CLARIOstar Plus (BMG Labtech). The isolated DNA was used for library preparation using the KAPA HyperPlus Kit (KAPA BIOSYSTEMS). DNA was loaded onto the MiSeq (Illumina) sequencer using the MiSeq Reagent kit v3 (Illumina) with pair-end reads, generating 250–300-bp read length.
In addition, MinION long read sequencing was performed using a single K. pneumoniae isolate as representative of the other three isolates randomly selected. DNA extraction was conducted using the Gentra Puregene Blood Kit (Qiagen). The preparation of the DNA for sequencing using 500 ng of purified DNA was performed using the Genomic DNA Ligation kit (SQK-LSK109, Oxford Nanopore Technologies, United Kingdom). The DNA sample was barcoded using the Native barcoding genomic DNA kits (EXP-NBD104 Oxford Nanopore Technologies, United Kingdom). The run of the samples was performed in a Flongle flow cell (FLO-FLG001, Oxford Nanopore Technologies, United Kingdom; Software v19.06.8). Base-calling was set on High-Accuracy base calling, and adapter trimming was performed through Porechop v0.2.3. After demultiplexing and adapter trimming, the Hybrid assemblies of short sequencing reads of the four randomly selected isolates and long-read sequencing reads of the single selected representative isolate were performed using Unicycler v0.4.7. The assembled genome was analyzed using tools from the “Center for genomic Epidemiology” (CGE) website.3
A subgroup of nine animals (n = 27 isolates) carrying ESBL-K. pneumoniae were followed-up over time to determine whether or not these ESBL-K. pneumoniae isolates were clonally related (Table 1). Multi Locus Sequencing Typing (MLST) was carried out according to the protocol previously described in the pubmlst web server.4 The sequence obtained was compared with the sequences available on the Genomic Epidemiology website. In addition, the only animal colonized with ESBL-E. coli (n = 4 isolates) over time along with the ESBL-K. pneumoniae isolates was also included in the MLST analysis. No additional ESBL-E. coli isolates were selected for MLST analysis.
Table 1. Distribution of fecal carriage of ESBL-Klebsiella pneumoniae isolates per time point (week 6 until week 24 after arrival of calves at a Dutch veal farm) selected for MLST analysis.
The Illumina (NGS) sequence data sets generated and analyzed in this study have been deposited in the European Nucleotide Archive (ENA) at EMBL-EBI under accession number PRJEB50519.
A t-test assuming equal variance statistical test was performed to indicate if a significant change was observed over time between animals colonized with cefotaxime-resistant E. coli and K. pneumoniae.
Veal calves were followed longitudinally from the dairy farms to the veal farm as previously described. A total of 80 E. coli and 174 cefotaxime-resistant K. pneumoniae isolates from rectal swabs obtained from 104 out of 110 veal calves located in the same veal farm in the Netherlands were analyzed. In this particular farm, a decrease of fecal carriage of cefotaxime-resistant E. coli isolates was observed from week 2 after transportation of calves from the dairy farms to the veal farm (61.3%) to week 6 at the veal farm (7.3%). Instead, an increase of fecal carriage of cefotaxime-resistant K. pneumoniae isolates was observed from week 6 until week 24 (Figure 1; p > 0.05). We did not detect cefotaxime-resistant K. pneumoniae isolates previous to week 6. The prevalence of cefotaxime-resistant K. pneumoniae isolates fluctuated during the rearing period, where the highest prevalence was detected in week 6 (80%) and the lowest in week 18 (7.3%). Calves were individually treated with antibiotics [β-Lactam (ampicillin, benzylpenicillin), amphenicol (florfenicol) and, aminoglycoside (gentamicin)] in week 2 (n = 18), week 6 (n = 32), week 10 (n = 78), week 18 (n = 31) and week 24 (n = 30) due to a respiratory disease (unspecified) for at least 8 weeks. In addition, a nonsteroidal anti-inflammatory drug was given in combination with the antibiotics during the same period. Three batch antibiotic treatments at herd level included tetracycline and aminoglycosides which were provided via the milk for 10 feedings within the first 6 weeks after arrival to the veal farms. Since the differences in the production system and management were considered minimal between all the veal farms, no data for risk factor analysis were included at this level.
Figure 1. Percentage of animals colonized by cefotaxime-resistant Escherichia coli and Klebsiella pneumoniae isolates recovered over time during the rearing period at the veal farm from week 2 after arrival of calves at the veal farm until week 24.
The blaCTX-M-1 group and blaTEM genes were detected by RT-PCRs in all of the cefotaxime-resistant E. coli isolates. The sequencing results showed that blaCTX_M-15 was present in all the E. coli isolates and in combination with the non-ESBL blaTEM-1a in 74 E. coli isolates (Supplementary Table 1), the other six E. coli isolates contains only the blaCTX-M-15 gene.
Furthermore, the blaSHV and blaTEM were detected by RT-PCRs in all the cefotaxime-resistant K. pneumoniae isolates. The sequencing results showed that a non-ESBL allelic variant identified as blaTEM-1b and a novel chromosomal variant sharing 99.5% identity to blaSHV-1-8 and other variants was identified, which results in a synonymous amino acid sequence. We also obtained positive PCR products for blaCTX-M-9 and blaCTX-M-14 genes. The sequencing analysis indicates that the fragment sequence corresponds to blaCTX-M-14. Furthermore, all the cefotaxime-resistant K. pneumoniae isolates were tested by PCR and the sequencing results indicates the presence of the blaCTX-M-14 in all the isolates (Supplementary Table 1).
The antimicrobial susceptibility test of four randomly selected K. pneumoniae isolates showed an identical resistance profile. The isolates were susceptible to colistin, carbapenems (imipenem, meropenem, ertapenem), azithromycin, chloramphenicol, nalidixic acid, tigecycline, and resistant to ciprofloxacin, gentamicin, sulfamethoxazole, trimethoprim, and tetracycline (Table 2). The ESBL phenotype was confirmed by showing resistance to cefotaxime and ceftazidime, susceptibility to cefoxitin, and synergy with clavulanic acid in combination with cefotaxime and ceftazidime.
Table 2. Antimicrobial susceptibility testing expressing the Minimal Inhibitory Concentration (MIC) determined in four Klebsiella pneumoniae isolates collected from calves in week 6 and week 24 at the veal farm.
Transformation experiments were performed using competent E. coli to determine if the blaCTX-M-14 gene was present on a transferable plasmid. Using the four ESBL-K. pneumoniae isolates showed a successful transferability of the blaCTX-M-14 gene. The plasmid-based replicon typing analysis detected the presence of two replicons in the transformants: IncFIIK and IncFIBKN type. Hybrid analysis of long-short read sequencing showed that the blaCTX-M-14 gene and the plasmids replicons previously identified were located in a 258.971 bp contig in K. pneumoniae isolates 2 week 24. The other three isolates were sequenced with short-read sequencing only which resulted in blaCTX-M-14 and the plasmid replicons in separate smaller contigs. Additionally, plasmid-mediated quinolone resistance (PMQR) genes qnrS1 and OqxAB were identified in the four K. pneumoniae isolates, along with genes conferring resistance to aminoglycosides, sulfamethoxazole, fosfomycin, tetracycline, trimethoprim and quaternary ammonium compound-resistance protein (Table 3).
Table 3. Molecular profile of fecal carriage of ESBL-Klebsiella pneumoniae obtained from calves during the rearing period at a Dutch veal farm.
MLST analysis was carried out on a set of 27 ESBL-K. pneumoniae isolates from nine veal calves that were colonized at multiple time-points to determine the clonality of the K. pneumoniae. All 27 ESBL-K. pneumoniae isolates presented an identical sequence type, all belonging to ST 107. Similarly, all the 4 ESBL-E. coli isolates obtained from a single animal colonized at the same rearing period as the animals colonized by ESBL-K. pneumoniae present an identical sequence type, all belonging to ST 46.
The World Health Organization (WHO) has included third-generation cephalosporin-resistant E. coli and K. pneumoniae into the group of “critical pathogens” due to the increasing challenges for infection treatment, highlighting the importance of the monitoring and prevention of infections in humans and animals. In humans, Meijs et al. (2021) have recently reported a high prevalence of fecal carriage of ESBL-E. coli and ESBL-K. pneumoniae in veterinary healthcare workers in the Netherlands compared to the general Dutch population (9.8% and 5%, respectively) (Meijs et al., 2021). Likewise, in Dutch hospitals, the prevalence of ESBL-K. pneumoniae in 2020 increased up to 15% compared to 2019 (12%) (NETMAP_MARAN, 2021). In the longitudinal study reported in the present manuscript, an unexpected increase in fecal carriage of ESBL-K. pneumoniae was detected in veal calves. This increase occurred in one single farm, 6 weeks after arrival of the calves to the veal farm and occurred along with a decrease in ESBL-E. coli which is typical in many farms at this time point (submitted manuscript). A previous study showed a similar low prevalence of ESBL-E. coli after 10 weeks at the veal farm (Hordijk et al., 2013). Previously, Adler et al. (2015) showed that the prevalence of ESBL-PE was high in calves (age, < 4 months) compared to adult cows (age > 25 months) (Adler et al., 2015). At the slaughterhouses, a high prevalence of ESBL producers has been reported in France (29.4%) (Haenni et al., 2014) and Switzerland (25%) (Geser et al., 2012), whereas a shift in enteric bacteria species carrying ESBL producers in the same calves has not been reported previously. Therefore, we assessed the characterization of fecal carriage ESBL-E. coli and ESBL-K. pneumoniae isolates from selected veal calves and the distribution of the ESBL genes present in the calves’ population over time.
In our study, the blaCTX-M-15 was the dominant ESBL gene detected in E. coli. In the Netherlands, the percentage of ESBL-E. coli reported in calves at slaughter age was 47% in 2018 and decreased to 38.1% in 2020, in which the CTX-M-1 group was the most dominant ESBL type detected (NETMAP_MARAN, 2021). The global spread of blaCTX-M-15 has been associated with particular E. coli clones such as clonal complex ST131 (Nicolas-Chanoine et al., 2014) and ST46 (Mshana et al., 2011). The E. coli clone ST46 harboring blaCTX-M-15 was detected in our study. The ST46 harboring blaCTX-M-15 has been previously identified in healthy chickens and pigs in Nigeria (Chah et al., 2018), in bovine feces in Tunisia (Hassen et al., 2019) and in the aquatic environment in Bangladesh (Rashid et al., 2015), suggesting that ESBL_E. coli clonal spread occurs in different environments across the world.
Furthermore, in the K. pneumoniae-cefotaxime resistance isolates in our study, the blaCTX-M-14 gene was identified in all the isolates. From those, four isolates were randomly selected to determine their antimicrobial susceptibility phenotype, indicating that those isolates were multidrug-resistant and displayed a typical ESBL phenotype. In addition, the non-ESBL blaTEM-1b and a novel chromosomal variant of blaSHV were detected by PCR and amplicon sequencing and confirmed by WGS. Previous studies also reported the presence of non-ESBLs blaTEM and blaSHV allelic variants in K. pneumoniae strains in clinical and non-clinical isolates including water, soil and animals raised for food production (Liakopoulos et al., 2016b; Shahraki-Zahedani et al., 2016). In addition to beta-lactams, it is concerning that resistance genes to other antibiotic classes including fluoroquinolones, tetracycline and aminoglycosides were also detected here, but considering results of a recent study in human K. pneumoniae isolates in the Netherlands, our results are not surprising (Hendrickx et al., 2020).
Several studies reported that the dissemination of the blaCTX-M-15 gene among Enterobacterales has been facilitated by the IncFII plasmids (Peirano and Pitout, 2010; Mansour et al., 2015; Stercz et al., 2021). In our study we showed that the blaCTX-M-14 gene dissemination was facilitated by the IncFIIK and IncFIBKN plasmid replicons in four ESBL-K. pneumoniae isolates tested. Previous studies showed the presence of K. pneumoniae isolates harboring blaCTX-M-14 located on IncFIBK and IncFIIK obtained from healthy red kangaroos (Wang et al., 2020) and from a clinical isolate in China (Zhang et al., 2017). Likewise, the spread of the blaCTX-M-14 gene in Enterobacterales has been predominantly associated with IncFII and IncK plasmid replicons in humans and animals including cattle in several countries (Hou et al., 2012; Stokes et al., 2012).
Several clonal lineages of K. pneumoniae have been reported widely in the United States and Europe such as ST258 and are currently present in several European countries (Woodford et al., 2011; Rodrigues et al., 2014; Ríos et al., 2017), supporting the epidemic potential of these clones. Our results showed that ST107 was the only ST type identified among ESBL-K. pneumoniae isolates in a single veal farm. The K. pneumoniae ST107 has been previously identified in K. pneumoniae isolates harboring blaKPC-2 from a hospital environment (Fu et al., 2018), K. pneumoniae producing NDM (NDM-9) from a human clinical isolate in China (Wang et al., 2014), and in K. pneumoniae isolates harboring blaSHV-11 from pigs and blaCTX-M-1 from cattle (Klaper et al., 2021).
We aimed to determine if the isolates were clonally related by calculating the pairwise Single-Nucleotide Polymorphism (SNPs) distances based on the core genome predicted genes. The high number of SNPs detected exclude the possibility to confirm this hypothesis (data not shown). However, it seems that these isolates may have been present at the farm beyond the time of a single production round. To confirm this hypothesis additional sampling of the farm, a bigger sample size and molecular analysis will need to be performed.
The low diversity of ESBL-PE reported in this study and the clonal spread of ESBL-K. pneumoniae during the rearing period highlight the importance to reinforce the hygiene and management practices in farms as previously reported (Bokma et al., 2019; Damiaans et al., 2019). Recently, Atterby et al. (2019) showed that direct contact with animal manure and animal slaughter products are potential risk factors for fecal carriage of ESBL-E. coli and ESBL-K. pneumoniae in humans and animals. Additionally, the authors indicated that daily removal of animal manure could decrease the environmental exposure to antibiotic-resistant bacteria (Atterby et al., 2019). In the current study, the fecal carriage of ESBL-K. pneumoniae started to spread rapidly from week 6 after the calves were transferred from the dairy farm to the veal farm, suggesting that the ESBL-K. pneumoniae originated from the veal farm environment, although colonization of one of the animals before transport from the dairy to the veal farm or contact with other calves or animals present in the farm cannot be ruled out. While the pathogen that was responsible for clinical respiratory problems on this farm has not been investigated, K. pneumoniae could have caused these symptoms while the usage of antimicrobials may have increased transmission. As such, an increase in hygiene measures on farms in which respiratory disease is seen could lead to a decrease in intestinal colonization which in turn could lead to a decrease in environmental contamination. We also hypothesis that the administration of nonsteroidal anti-inflammatory drug (NSAID) for at least 2 weeks continuously in combination with antibiotics to control the respiratory infection, could perhaps contribute to the presence of K. pneumoniae in feces because the use of this drug can cause gastrointestinal upset such as irritation, therefore affecting the colonization resistance population present in the gastrointestinal tract. Further research is needed to better understand the relationship between animals, farmworkers, and the environment to further assess and limit the exposure and risk of fecal carriage of antibiotic-resistant bacteria.
The datasets presented in this study can be found in online repositories. The names of the repository/repositories and accession number(s) can be found at: https://www.ebi.ac.uk/ena, PRJEB50519.
The animal study was reviewed and approved by The Central Committee on Animal Experiments (the Hague, Netherlands; approval number 2017.D-0029). Written informed consent was obtained from the owners for the participation of their animals in this study.
MB and KvR designed the study. FM collected the samples and data. TB performed the sample analysis, processed the data, and wrote the manuscript. AK and QD contributed with the laboratory work. MB, KV, FM, and KvR contributed to review and editing the manuscript. All authors contributed to the article and approved the submitted version.
The collection of isolates that were used for this study was funded by the Dutch Ministry of Agriculture, Nature and Food Quality grant BO-43-111-011. Funding for the analysis of the isolates was received from the European Union’s Horizon 2020 research and innovation programme through One Health EJP Project Full-Force [grant agreement number 773830] with co-funding from TKI bureau AgriFood.
The authors declare that the research was conducted in the absence of any commercial or financial relationships that could be construed as a potential conflict of interest.
All claims expressed in this article are solely those of the authors and do not necessarily represent those of their affiliated organizations, or those of the publisher, the editors and the reviewers. Any product that may be evaluated in this article, or claim that may be made by its manufacturer, is not guaranteed or endorsed by the publisher.
The Supplementary Material for this article can be found online at: https://www.frontiersin.org/articles/10.3389/fmicb.2022.866674/full#supplementary-material
Adler, A., Sturlesi, N., Fallach, N., Zilberman-Barzilai, D., Hussein, O., Blum, S. E., et al. (2015). Prevalence, risk factors, and transmission dynamics of extended-spectrum-β-lactamase-producing enterobacteriaceae: a national survey of cattle farms in Israel in 2013. J. Clin. Microbiol. 53, 3515–3521. doi: 10.1128/JCM.01915-15
Atterby, C., Osbjer, K., Tepper, V., Rajala, E., Hernandez, J., Seng, S., et al. (2019). Carriage of carbapenemase- and extended-spectrum cephalosporinase-producing Escherichia coli and Klebsiella pneumoniae in humans and livestock in rural Cambodia; gender and age differences and detection of blaOXA-48in humans. Zoonoses Public Health 66, 603–617. doi: 10.1111/zph.12612
Bokma, J., Boone, R., Deprez, P., and Pardon, B. (2019). Risk factors for antimicrobial use in veal calves and the association with mortality. J. Dairy Sci. 102, 607–618. doi: 10.3168/jds.2018-15211
Bush, K., and Fisher, J. F. (2011). Epidemiological expansion, structural studies, and clinical challenges of new β-lactamases from gram-negative bacteria. Annu. Rev. Microbiol. 65, 455–478. doi: 10.1146/annurev-micro-090110-102911
Carattoli, A., Bertini, A., Villa, L., Falbo, V., Hopkins, K. L., and Threlfall, E. J. (2005). Identification of plasmids by PCR-based replicon typing. J. Microbiol. Methods 63, 219–228. doi: 10.1016/j.mimet.2005.03.018
Chah, K. F., Ugwu, I. C., Okpala, A., Adamu, K. Y., Alonso, C. A., Ceballos, S., et al. (2018). Detection and molecular characterisation of extended-spectrum β-lactamase-producing enteric bacteria from pigs and chickens in Nsukka, Nigeria. J. Glob. Antimicrob. Resist 15, 36–40. doi: 10.1016/j.jgar.2018.06.002
D’Andrea, M. M., Arena, F., Pallecchi, L., and Rossolini, G. M. (2013). CTX-M-type β-lactamases: a successful story of antibiotic resistance. Int. J. Med. Microbiol. 303, 305–317. doi: 10.1016/j.ijmm.2013.02.008
Dahmen, S., Métayer, V., Gay, E., Madec, J. Y., and Haenni, M. (2013). Characterization of extended-spectrum beta-lactamase (ESBL)-carrying plasmids and clones of Enterobacteriaceae causing cattle mastitis in France. Vet. Microbiol. 162, 793–799. doi: 10.1016/j.vetmic.2012.10.015
Damiaans, B., Renault, V., Sarrazin, S., Berge, A. C., Pardon, B., Ribbens, S., et al. (2019). Biosecurity practices in Belgian veal calf farming: level of implementation, attitudes, strengths, weaknesses and constraints. Prev. Vet. Med. 172:104768. doi: 10.1016/j.prevetmed.2019.104768
Diab, M., Hamze, M., Bonnet, R., Saras, E., Madec, J. Y., and Haenni, M. (2017). OXA-48 and CTX-M-15 extended-spectrum beta-lactamases in raw milk in Lebanon: epidemic spread of dominant Klebsiella pneumoniae clones. J. Med. Microbiol. 66, 1688–1691. doi: 10.1099/jmm.0.000620
Dierikx, C. M., van Duijkeren, E., Schoormans, A. H. W., van Essen-Zandbergen, A., veldman, K., Kant, A., et al. (2012). Occurrence and characteristics of extended-spectrum-β-lactamase- and AmpC-producing clinical isolates derived from companion animals and horses. J. Antimicrob. Chemother. 67, 1368–1374. doi: 10.1093/jac/dks049
European Food Safety Authority (EFSA) (2011). Scientific opinion on the public health risks of bacterial strains producing extended-spectrum β-lactamases and/or AmpC βlactamases in food and food-producing animals. EFSA J. 9:2322. doi: 10.2903/j.efsa.2011.2322
European Reference Laboratory-Antimicrobial Resistance (n.d.). Available at: https://www.eurlar.eu/CustomerData/Files/Folders/21-protocols/390_protocol-for-validation-of-macconkey-and-ctx-agar-plates-final03112017.pdf
Fu, L., Huang, M., Zhang, X. Z., Yang, X. Y., Liu, Y., Zhang, L. H., et al. (2018). Frequency of virulence factors in high biofilm formation blaKPC-2 producing Klebsiella pneumoniae strains from hospitals. Microb. Pathog. 116, 168–172. doi: 10.1016/j.micpath.2018.01.030
Genomic Epidemiology Organization Server (n.d.). Available at: http://genomicepidemiology.org/
Geser, N., Stephan, R., and Hächler, H. (2012). Occurrence and characteristics of extended-spectrum β-lactamase (ESBL) producing Enterobacteriaceae in food producing animals, minced meat and raw milk. BMC Vet. Res. 8:21. doi: 10.1186/1746-6148-8-21
Geurts, Y., Brouwer, M., Noorman, K., Kant, A., Ceccarelli, D., Veldman, K., et al. (2017). “Development of sensitive and cost-effective real-time PCR assays for rapid detection of the beta-lactamases genes CTX-M1, SHV, TEM and Amp C gene CMY2 in Enterobacteriaceae.” in Proceeding of the 27th European Congress of Clinical Microbiology and Infectious Diseases 2017. April 22, 2017. Vienna (European Society for Clinical Microbiology and Infectious Diseases).
Haenni, M., Châtre, P., Métayer, V., Bour, M., Signol, E., Madec, J. Y., et al. (2014). Comparative prevalence and characterization of ESBL-producing Enterobacteriaceae in dominant versus subdominant enteric flora in veal calves at slaughterhouse, France. Vet. Microbiol. 171, 321–327. doi: 10.1016/j.vetmic.2014.02.023
Hassen, B., Saloua, B., Abbassi, M. S., Ruiz-Ripa, L., Mama, O. M., Hassen, A., et al. (2019). Mcr-1 encoding colistin resistance in CTX-M-1/CTX-M-15- producing Escherichia coli isolates of bovine and caprine origins in Tunisia. First report of CTX-M-15-ST394/D E. coli from goats. Comp. Immunol. Microbiol. Infect. Dis. 67:101366. doi: 10.1016/j.cimid.2019.101366
Hendrickx, A., Landman, F., de Haan, A., Borst, D., Witteveen, S., van Santen-Verheuvel, M. G., et al. (2020). Plasmid diversity among genetically related Klebsiella pneumoniae blaKPC-2 and blaKPC-3 isolates collected in the Dutch national surveillance. Sci. Rep. 10:16778. doi: 10.1038/s41598-020-73440-2
Hordijk, J., Mevius, D. J., Kant, A., Bos, M. E. H., Graveland, H., Bosman, A. B., et al. (2013). Within-farm dynamics of ESBL/AmpC-producing escherichia coli in veal calves: a longitudinal approach. J. Antimicrob. Chemother. 68, 2468–2476. doi: 10.1093/jac/dkt219
Horton, R. A., Randall, L. P., Snary, E. L., Cockrem, H., Lotz, S., Wearing, H., et al. (2011). Fecal carriage and shedding density of CTX-M extended-spectrum β-lactamase-producing Escherichia coli in cattle, chickens, and pigs: implications for environmental contamination and food production. Appl. Environ. Microbiol. 77, 3715–3719. doi: 10.1128/AEM.02831-10
Hou, J., Huang, X., Deng, Y., He, L., Yang, T., Zeng, Z., et al. (2012). Dissemination of the fosfomycin resistance gene fosA3 with CTX-M β-lactamase genes and rmtB carried on incfII plasmids among Escherichia coli isolates from pets in China. Antimicrob. Agents Chemother. 56, 2135–2138. doi: 10.1128/AAC.05104-11
Klaper, K., Hammerl, J. A., Rau, J., Pfeifer, Y., and Werner, G. (2021). Genome-based analysis of klebsiella spp. Isolates from animals and food products in germany, 2013–2017. Pathogens 10:573. doi: 10.3390/pathogens10050573
Kurittu, P., Khakipoor, B., Aarnio, M., Nykäsenoja, S., Brouwer, M., Myllyniemi, A. L., et al. (2021). Plasmid-borne and chromosomal ESBL/AmpC genes in Escherichia coli and Klebsiella pneumoniae in global food products. Front. Microbiol. 12:592291. doi: 10.3389/fmicb.2021.592291
Liakopoulos, A., Geurts, Y., Dierikx, C. M., Brouwer, M. S. M., Kant, A., Wit, B., et al. (2016a). Extended-spectrum cephalosporin- resistant Salmonella enterica serovar Heidelberg strains, the Netherlands. Emerg. Infect. Dis. 22, 1257–1261. doi: 10.3201/eid2207.151377
Liakopoulos, A., Mevius, D., and Ceccarelli, D. (2016b). A review of SHV extended-spectrum β-lactamases: neglected yet ubiquitous. Front. Microbiol. 7:1374. doi: 10.3389/fmicb.2016.01374
Liebana, E., Carattoli, A., Coque, T. M., Hasman, H., Magiorakos, A. P., Mevius, D., et al. (2013). Public health risks of enterobacterial isolates producing extended-spectrum β-lactamases or AmpC β-lactamases in food and food-producing animals: an EU perspective of epidemiology, analytical methods, risk factors, and control options. Clin. Infect. Dis. 56, 1030–1037. doi: 10.1093/cid/cis1043
Mansour, W., Grami, R., Ben Haj Khalifa, A., Dahmen, S., Châtre, P., Haenni, M., et al. (2015). Dissemination of multidrug-resistant blaCTX-M-15/IncFIIk plasmids in Klebsiella pneumoniae isolates from hospital- and community-acquired human infections in Tunisia. Diagn. Microbiol. Infect. Dis. 83, 298–304. doi: 10.1016/J.DIAGMICROBIO.2015.07.023
Marcato, F., van den Brand, H., Kemp, B., Engel, B., Schnabel, S. K., Jansen, C. A., et al. (2022). Calf and dam characteristics and calf transport age affect immunoglobulin titers and hematological parameters of veal calves. J. Dairy Sci. 105, 1432–1451. doi: 10.3168/jds.2021-20636
Meijs, A. P., Gijsbers, E. F., Hengeveld, P. D., Dierikx, C. M., de Greeff, S. C., and van Duijkeren, E. (2021). ESBL/pAmpC-producing Escherichia coli and Klebsiella pneumoniae carriage among veterinary healthcare workers in the Netherlands. Antimicrob. Resist. Infect. Control 10:147. doi: 10.1186/s13756-021-01012-8
Mshana, S. E., Imirzalioglu, C., Hain, T., Domann, E., Lyamuya, E. F., and Chakraborty, T. (2011). Multiple ST clonal complexes, with a predominance of ST131, of Escherichia coli harbouring blaCTX-M-15 in a tertiary hospital in Tanzania. Clin. Microbiol. Infect. 17, 1279–1282. doi: 10.1111/j.1469-0691.2011.03518.x
Munk, P., Knudsen, B. E., Lukjacenko, O., Duarte, A. S. R., van Gompel, L., Luiken, R. E. C., et al. (2018). Abundance and diversity of the faecal resistome in slaughter pigs and broilers in nine European countries. Nat. Microbiol. 3, 898–908. doi: 10.1038/s41564-018-0192-9
NETMAP_MARAN (2021). Available at: https://www.wur.nl/nl/show/Nethmap-MARAN-2021.htm (Accessed January, 2022).
Nicolas-Chanoine, M. H., Bertrand, X., and Madec, J. Y. (2014). Escherichia coli st131, an intriguing clonal group. Clin. Microbiol. Rev. 27, 543–574. doi: 10.1128/CMR.00125-13
Overdevest, I., Willemsen, I., Rijnsburger, M., Eustace, A., Xu, L., Hawkey, P., et al. (2011). Extended-spectrum β-lactamase genes of Escherichia coli in chicken meat and humans, the Netherlands. Emerg. Infect. Dis. 17, 1216–1222. doi: 10.3201/eid1707.110209
Peirano, G., and Pitout, J. D. D. (2010). Molecular epidemiology of Escherichia coli producing CTX-M β-lactamases: the worldwide emergence of clone ST131 O25:H4. Int. J. Antimicrob. Agents 35, 316–321. doi: 10.1016/j.ijantimicag.2009.11.003
Pubmlst Server (n.d.). Available at: https://pubmlst.org/
Rashid, M., Rakib, M. M., and Hasan, B. (2015). Antimicrobial-resistant and ESBL-producing Escherichia coli in different ecological niches in Bangladesh. Infect. Ecol. Epidemiol. 5:26712. doi: 10.3402/iee.v5.26712
Ríos, E., López, M. C., Rodríguez-Avial, I., Culebras, E., and Picazo, J. J. (2017). Detection of Escherichia coli ST131 clonal complex (ST705) and Klebsiella pneumoniae ST15 among faecal carriage of extended-spectrum β-lactamase- and carbapenemase-producing Enterobacteriaceae. J. Med. Microbiol. 66, 169–174. doi: 10.1099/jmm.0.000399
Rodrigues, C., Machado, E., Ramos, H., Peixe, L., and Novais, Â. (2014). Expansion of ESBL-producing Klebsiella pneumoniae in hospitalized patients: a successful story of international clones (ST15, ST147, ST336) and epidemic plasmids (IncR, IncFIIK). Int. J. Med. Microbiol. 304, 1100–1108. doi: 10.1016/j.ijmm.2014.08.003
Rozwandowicz, M., Brouwer, M. S. M., Fischer, J., Wagenaar, J. A., Gonzalez-Zorn, B., Guerra, B., et al. (2018). Plasmids carrying antimicrobial resistance genes in Enterobacteriaceae. J. Antimicrob. Chemother. 73, 1121–1137. doi: 10.1093/jac/dkx488
Schmid, A., Hörmansdorfer, S., Messelhäusser, U., Käsbohrer, A., Sauter-Louis, C., and Mansfeld, R. (2013). Prevalence of extended-spectrum β-lactamase-producing Escherichia coli on Bavarian dairy and beef cattle farms. Appl. Environ. Microbiol. 79, 3027–3032. doi: 10.1128/AEM.00204-13
Schukken, Y., Chuff, M., Moroni, P., Gurjar, A., Santisteban, C., Welcome, F., et al. (2012). The “other” gram-negative Bacteria in mastitis. Klebsiella, Serratia, and more. Vet. Clin. North Am. Food Anim. Pract. 28, 239–256. doi: 10.1016/j.cvfa.2012.04.001
Shahraki-Zahedani, S., Rigi, S., Bokaeian, M., Ansari-Moghaddam, A., and Moghadampour, M. (2016). First report of TEM-104-, SHV-99-, SHV-108-, and SHV-110-producing Klebsiella pneumoniae from Iran. Rev. Soc. Bras. Med. Trop. 49, 441–445. doi: 10.1590/0037-8682-0114-2016
Stercz, B., Farkas, F. B., Tóth, Á., Gajdács, M., Domokos, J., Horváth, V., et al. (2021). The influence of antibiotics on transitory resistome during gut colonization with CTX-M-15 and OXA-162 producing Klebsiella pneumoniae ST15. Sci. Rep. 11:6335. doi: 10.1038/s41598-021-85766-6
Stokes, M. O., Cottell, J. L., Piddock, L. J. V., Wu, G., Wootton, M., Mevius, D. J., et al. (2012). Detection and characterization of pCT-like plasmid vectors for Bla ctx-M-14 in Escherichia coli isolates from humans, turkeys and cattle in England and Wales. J. Antimicrob. Chemother. 67, 1639–1644. doi: 10.1093/jac/dks126
Tshitshi, L., Manganyi, M. C., Montso, P. K., Mbewe, M., and Ateba, C. N. (2020). Extended spectrum beta-lactamase-resistant determinants among carbapenem-resistant Enterobacteriaceae from beef cattle in the north West Province, South Africa: a critical assessment of their possible public health implications. Antibiotics 9:820. doi: 10.3390/antibiotics9110820
Veldman, K., Swanenburg, M., Ceccarelli, D., and Mevius, D. (2018). Monitoring of Antimicrobial Resistance and Antibiotic Usage in Animals (MARAN) in the Netherlands in 2017. Lelystad: Wageningen Bioveterinary Research.
Waade, J., Seibt, U., Honscha, W., Rachidi, F., Starke, A., Speck, S., et al. (2021). Multidrug-resistant enterobacteria in newborn dairy calves in Germany. PLoS One 16:e0248291. doi: 10.1371/journal.pone.0248291
Wang, X., Kang, Q., Zhao, J., Liu, Z., Ji, F., Li, J., et al. (2020). Characteristics and epidemiology of extended-Spectrum β-lactamase-producing multidrug-resistant Klebsiella pneumoniae from red kangaroo. China. Front. Microbiol. 11:560474. doi: 10.3389/fmicb.2020.560474
Wang, X., Li, H., Zhao, C., Chen, H., Liu, J., Wang, Z., et al. (2014). Novel NDM-9 metallo-β-lactamase identified from a ST107 Klebsiella pneumoniae strain isolated in China. Int. J. Antimicrob. Agents 44, 90–91. doi: 10.1016/j.ijantimicag.2014.04.010
Woodford, N., Turton, J. F., and Livermore, D. M. (2011). Multiresistant gram-negative bacteria: the role of high-risk clones in the dissemination of antibiotic resistance. FEMS Microbiol. Rev. 35, 736–755. doi: 10.1111/j.1574-6976.2011.00268.x
World Health Organization (WHO) (n.d.). Available at: https://www.who.int/medicines/publications/WHO-PPL-Short_Summary_25Feb-ET_NM_WHO.pdf
Keywords: veal calves, fecal carriage, Klebsiella pneumoniae, clonal spread, extended-spectrum β-lactamase producing Enterobacterales
Citation: Bello Gonzalez TdJ, Kant A, Dijkstra Q, Marcato F, van Reenen K, Veldman KT and Brouwer MSM (2022) Changes in Fecal Carriage of Extended-Spectrum β-Lactamase Producing Enterobacterales in Dutch Veal Calves by Clonal Spread of Klebsiella pneumoniae. Front. Microbiol. 13:866674. doi: 10.3389/fmicb.2022.866674
Received: 31 January 2022; Accepted: 31 May 2022;
Published: 23 June 2022.
Edited by:
Ravi kant, University of Helsinki, FinlandReviewed by:
Lixin Zhang, Michigan State University, United StatesCopyright © 2022 Bello Gonzalez, Kant, Dijkstra, Marcato, van Reenen, Veldman and Brouwer. This is an open-access article distributed under the terms of the Creative Commons Attribution License (CC BY). The use, distribution or reproduction in other forums is permitted, provided the original author(s) and the copyright owner(s) are credited and that the original publication in this journal is cited, in accordance with accepted academic practice. No use, distribution or reproduction is permitted which does not comply with these terms.
*Correspondence: Teresita d.J. Bello Gonzalez, dGVyZXNpdGEuYmVsbG9nb256YWxlekB3dXIubmw=
Disclaimer: All claims expressed in this article are solely those of the authors and do not necessarily represent those of their affiliated organizations, or those of the publisher, the editors and the reviewers. Any product that may be evaluated in this article or claim that may be made by its manufacturer is not guaranteed or endorsed by the publisher.
Research integrity at Frontiers
Learn more about the work of our research integrity team to safeguard the quality of each article we publish.