- 1College of Forestry, Shandong Agriculture University, Taian, China
- 2Key Laboratory of National Forestry and Grassland Administration on Silviculture of the Lower Yellow River, Shandong Agricultural University, Taian, China
- 3College of Plant Protection, Shandong Agricultural University, Taian, China
- 4College of Biological and Agricultural Engineering, Weifang University, Weifang, China
Meloidogyne incognita is one of the most destructive soil pests, causing serious economic losses in tomato production. Here, in vitro experiments demonstrated that the Bacillus licheniformis strain JF-22 has the potential to prevent M. incognita infection. A pot experiment confirmed that B. licheniformis strain JF-22 isolated from the tomato rhizosphere soil and planted in the tomato root-knot nematode disease area effectively prevented and controlled M. incognita, reducing its negative effect on tomato growth. Additionally, the composition of volatile substances secreted by B. licheniformis strain JF-22 was analyzed using solid-phase microextraction and gas chromatography–mass spectrometry. We detected acetoin, 2,3-Butanediol, [R-(R*,R*) ]-, and hexamethyl cyclotrisiloxane as the main components among these volatiles. Using MiSeq sequencing technology and bioinformatics, we analyzed the influence of B. licheniformis strain JF-22 on the microbial community of the tomato rhizosphere. B. licheniformis strain JF-22 changed the composition of the microbial community; particularly, it significantly reduced the diversity of the fungal community. Furthermore, using the FUNGuild and PICRUSt databases, we predicted the effect of JF-22 on microbial community function. In conclusion, B. licheniformis strain JF-22 may be considered as a potential biocontrol agent against M. incognita.
Introduction
The tomato root-knot nematode disease caused by Meloidogyne incognita is a devastating disease that affects tomatoes (Siddiqui et al., 2006). M. incognita absorbs key nutrients after infecting plant roots and can easily induce secondary infections by a range of soil pathogens, thereby negatively affecting the plant growth and, ultimately, yield. M. incognita is transmitted via the soil, water sources, and diseased plants (Roberts et al., 1981). Specifically, under greenhouse conditions, the disease may occur continuously because of repeated cropping. Moreover, it has developed into a disaster in some large vegetable-growing areas (Fravel, 2005).
Plant growth-promoting rhizobacteria (PGPR) are an important biological resource (Bhattacharyya and Jha, 2012). Studies have shown that many PGPR, such as Bacillus subtilis, Bacillus megaterium, Bacillus coagulans, and Pseudomonas fluorescens, can control M. incognita (Xiang et al., 2018). Indeed, PGPR can reportedly induce plant resistance to M. incognita (Siddiqui and Shaukat, 2002). For example, rhizobacterial consortia can be used to prevent parasitic nematodes in grapevines (Aballay et al., 2020). Similarly, Phanerochaete chrysosporium can inhibit the growth of J2s (second stage juveniles) of M. incognita (Du et al., 2020). Furthermore, studies have shown that Bacillus was the main genus responsible for the higher mortality of M. incognita (Xiang et al., 2017). Bacillus not only directly stimulates the plant growth by enhancing nutrient acquisition or stimulating the host-plant defense mechanism; but, additionally, it inhibits the growth of pathogenic microorganisms (Kumar et al., 2001). For example, Bacillus halotolerans, Bacillus kochii, Bacillus oceanisediminis, Bacillus pumilus, Bacillus toyonensis, Bacillus cereus, Pseudomonas aeruginosa, and Bacillus pseudomycoides can control the M. incognita (Liu et al., 2020). Further, single or multiple rhizosphere bacteria can control the root-knot nematodes. For example, the combination of Bacillus amyloliquefaciens and B. subtilis strains can reduce the number of M. incognita specimens in the soil (Burkett et al., 2008).
The control strategies for M. incognita mainly include biological and chemical methods. However, currently, chemical control methods are predominantly in use for the control of plant-parasitic nematodes. Chemical agents have been used for a long time because of their rapid and obvious control effects. However, this method can lead to problems such as environmental pollution and drug resistance in M. incognita. Therefore, biological control is now receiving increasing attention (Zhang et al., 2022), particularly because the social awareness of the urgency for environmental protection is increasing. Rhizosphere microorganisms interact with phytopathogens, other indigenous microorganisms, and host plants by competing for nutrients, producing antimicrobial substances, and secreting volatile organic compounds to better adapt to the rhizosphere environment. While doing so, rhizosphere microorganisms perform a disease-suppressing function (Hinsinger et al., 2009). In this study, strain JF-22 of Bacillus licheniformis was isolated from the rhizosphere soil in an area where healthy tomato plants were grown in the presence of tomato root-knot nematode. The effect of B. licheniformis strain JF-22 on M. incognita was studied using in vitro tests and pot experiments. Solid phase microextraction (SPME), gas chromatography–mass spectrometry (GC–MS), MiSeq sequencing technology, and bioinformatics analytical methods were used to analyze the composition of volatile substances produced by strain JF-22 and the influence of strain JF-22 on the structure of the rhizosphere microbial community.
Materials and Methods
Isolation and Screening of Bacterial Strains
We followed the method described by Wang et al. (2021) to isolate the soil bacteria. Rhizosphere soil in which healthy tomato (Lycopersicon esculentum) plants grew was collected from areas infested with tomato root-knot nematode disease. Soil samples (5 g) were suspended in 50 ml of sterile distilled water and mixed on a table concentrator for 30 min. These soil samples were serially diluted (up to 10–7-fold), plated on potato dextrose agar (PDA), and incubated at 30 ± 2°C for 2–3 days. Bacterial colonies growing on the plates were isolated according to their morphological characteristics for further study. Subsequently, the isolated strains were screened using in vitro tests, for which purpose, they were transferred to an LB solid medium plate. After activation at 30°C, purified single colonies were picked using a sterile inoculating loop and placed in a 250 ml conical flask containing 50 ml LB liquid medium. After 24 h of cultivation at 30°C, with stirring at 200 rpm, the inoculum was connected to the 50 ml LB culture medium at a ratio of 2% and cultured at 30°C and 200 rpm in a 250 ml conical flask for 48 h to prepare a fermentation broth. This broth was then centrifuged at 13,000 rpm for 10 min and filtered through a 0.22 μm sterile syringe filter. Lastly, 0.8 ml of the filtrates was placed in 1.5 ml centrifuge tubes before adding 100 s-stage juveniles (J2s) of M. incognita to each centrifuge tube. The corrected mortality of the J2s of M. incognita was calculated at 24 h and again at 48 h. Each treatment was repeated six times.
Identification of JF-22
Strain JF-22 of B. licheniformis was preliminarily identified based on its morphological characteristics and then inoculated on PDA medium and observed for colony morphological characteristics after incubation at 32°C for 5 days. The strain was subsequently cultured for 24 h in LB liquid medium prior to Gram staining. Morphology was observed and photographed under a microscope. Further identification of JF-22 was achieved through the analysis of its 16S rDNA and gyrB gene sequences. The DNA of strain JF-22 was extracted with the Tiangen bacterial DNA extraction kit and stored at 4°C. 16S rDNA was amplified by the polymerase chain reaction (PCR) using the bacterial universal primers 27F (5′-AGAGTTTGATCCTGGCTCAG-3′) and 1492R (5′-GGTTACCTTGTTACGACTT-3′). The gyrB gene was amplified by PCR using specific primers G3F (5′-GAAGTCATCATGACCGTTCTGCAYGCNGGNGGNAARTTY GA-3′) and G3L(5′-AGCAGGGTACGGATGTGCGAGCCRTCN ACRTCNGCRTCNGTCAT-3′). The reaction conditions for 16S rDNA amplification were as follows: 95°C for 5 min; 33 cycles at 95°C for 1 min, at 58°C for 30 s, and at 72°C for 2 min, and a final extension at 72°C for 10 min. The reaction conditions of gyrB gene amplification were as follows: 96°C for 5 min, 32 cycles at 96°C for 30 s, at 59°C for 30 s, and at 72°C for 1 min, and a final extension at 72°C for 10 min. Then, the PCR products were purified and sequenced by a commercial sequencing company (Sangon Biotech, Shanghai, China). Nucleotide sequences were analyzed and compared with sequences in the GenBank database using BLAST. A phylogenetic tree of the JF-22 strain was constructed with 16S rDNA and gyrB gene sequences using the neighbor-joining method in Mega 7.0.21.
Pot Test
Meloidogyne incognita was isolated and cultured according to the method described by Jing et al. (2020). Tomato seeds of the tested variety were sown in a nursery tray, and when the seedlings reached the fourth true-leaf stage they were transplanted into a plastic pot 20 cm in diameter and infested with 300 J2s specimens of M. incognita per plant. Two days after transplanting, each tomato plant was watered with 100 ml of JF-22 bacterial suspension at a concentration of 108 CFU/ml, and the same amount of sterile water was used as a control (CK). After inoculation, pots were randomly placed on the operating platform of a greenhouse. Each treatment was repeated 60 times. Tomato plants were uprooted separately to determine plant biomass indices. Disease severity was determined using the method of Lu et al. (2017), and the incidence index was determined. Rhizosphere soil around the tomato roots was collected and stored at −80°C for analysis of microbial community structure.
Analysis of Volatile Organic Compounds by Gas Chromatography–Mass Spectrometry
The volatile composition of strain JF-22 was determined using SPME–GC–MS as described by Wang et al. (2021). Strain JF-22 was inoculated on PDA slants placed in triangular flasks, which were then sealed with foil and incubated at 30°C. After 3 days, polydimethylsiloxane-divinylbenzene (65 μm) was used to collect volatile organic compounds (VOCs), and an RTX-5MS capillary column (60 × 0⋅25 μm ID × 0⋅25-μm thick film) was used to separate VOCs. Helium was used as the carrier gas at a rate of 1 ml min–1 to analyze VOCs by GC–MS using the following procedure: desorb SPME-DVB fiber at 200°C for 3 min. For VOCs analysis, first, the oven temperature was raised to 40°C for 3 min, and then increased to 160°C at a rate of 8°C min–1; it was maintained at that point for 2 min, and finally increased to 240°C at a rate of 15°C min–1 and maintained at that point for 3 min. The mass spectrometer was set to 70 eV electron ionization mode, with a source temperature of 200°C. Continuous scanning from 45 to 500 m/z was used. VOCs were identified by comparison with data provided in the National Institute of Standards and Technology guidelines (number 14).
DNA Extraction and Illumina MiSeq High-Throughput Sequencing
Total DNA extraction from the soil was performed using the BIO-TEK OMEGA Soil DNA Kit (Omega Bio-tek, Norcross, GA, United States). Concomitantly, the bacterial 16S rDNA V3-V4 region and the fungal rDNA-ITS gene were amplified. PCR amplification was performed as previously described (Sui et al., 2019). After mixing the PCR products of the same sample, they were separated using 2% agarose gels, and the recovered products were purified using AxyPrep DNA Gel Extraction Kit (Axygen Biosciences, Union City, CA, United States), and detected by 2% agarose gel electrophoresis. The recovered products were quantified using a Quantus fluorometer (Promega, United States). Furthermore, the NEXTFLEX Rapid DNA-Seq Kit was used for the DNA library construction. The library was constructed following these steps: (1) Adapter linking; (2) Magnetic bead screening to remove adapter self-ligating fragments; (3) PCR amplification to enrich library templates; (4) Magnetic beads recovery of PCR products to produce the final library. Sequencing was performed on the Illumina MiSeq PE300 platform. The raw sequences were quality controlled using the Trimmomatic software and spliced using the FLASH software as follows: (1) the 300 bp reads were truncated at any site receiving an average quality score of < 20 over a 50 bp sliding window, and the truncated reads shorter than 50 bp were discarded, reads containing ambiguous characters were also discarded; (2) according to the overlap relationship between PE reads, paired reads were merged into a sequence, and the minimum overlap length was 10 bp; (3) maximum mismatch ratio allowed in the overlap region of the spliced sequence was 0.2, and the non-conforming sequences were screened; and (4) samples were distinguished according to the barcodes and primers at the beginning and end of the sequence, and the sequence direction was adjusted. The number of mismatches allowed by the barcode was zero, and the maximum number of primer mismatches was 2. All reads were clustered with a 97% similarity cut-off using UPARSE (ver. 7.1)1, and chimeric sequences were identified and removed using UCHIME (Zheng et al., 2015). The taxonomy of each 16S rDNA and ITS rDNA gene sequence was analyzed using the RDP Classifier against the Silva (SSU123) 16S rDNA database (Amato et al., 2013) and the UNITE 7.0/ITS database (Abarenkov et al., 2010), respectively, using a confidence threshold of 70%. Bacterial population functions were analyzed using the PICRUSt 2 database. Fungal ecosystem analyses were performed using the FUNGuild database (Nguyen et al., 2015). Raw reads were deposited in the NCBI Sequence Read Archive database (accession numbers: PRJNA803317 and PRJNA803323).
Statistical Analysis
Data were analyzed using Microsoft Excel and SPSS 22.0 (SPSS Inc., Chicago, IL, United States). Duncan’s multi-range test and Student’s t-test were performed to compare the significance of the differences at p < 0.05.
Results
Isolation and Screening of Bacterial Strains
For the selection of potential biocontrol agents against M. incognita, 15 bacterial strains were isolated, purified, and screened using in vitro assays to test the toxicity of the isolated fermentation supernatant on J2s of M. incognita. The results showed that the fermentation supernatant of four bacterial strains had toxic effects on M. incognita J2s. Among these, specifically, the fermentation supernatant of JF-22 had the strongest toxic effect on M. incognita J2s. When J2s of M. incognita were treated for 24 h with the fermentation supernatant of JF-22, the death rate of the J2s was 77%, and when they were treated for 48 h, mortality reached 89%. This indicated that JF-22 has a strong ability to kill M. incognita at the J2s developmental stage (Table 1).
Identification of B. licheniformis JF-22
The colony of strain JF-22 was round, white, and non-transparent, with a wrinkled surface, irregular edges, and a central bulge. Under the microscope, strain JF-22 was found to be a rod-shaped, gram-positive bacterium. Based on phylogenetic analysis of 16S rDNA and gyrB (Figure 1A), strain JF-22 was identified as B. licheniformis (Figure 1B).
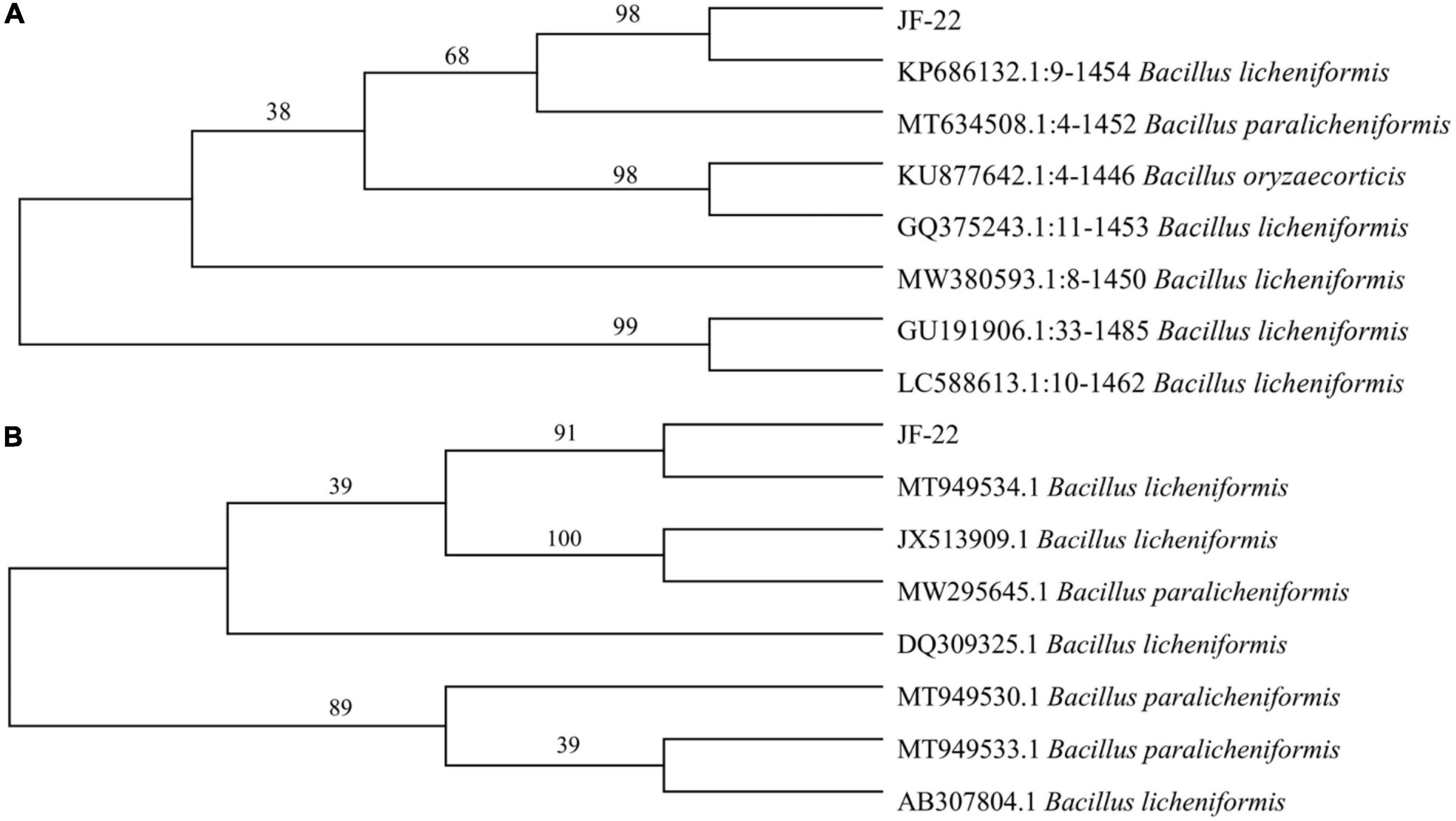
Figure 1. Phylogenetic tree shows relationship between JF-22 and other strains. (A) Phylogenetic tree constructed based on 16S rDNA sequences of strain JF-22 and 7 other Bacillus strains; (B) Phylogenetic tree constructed based on gyrB gene sequences of strain JF-22 and 7 other Bacillus strains. Bootstrap values (%) presented at the branches were calculated from 1,000 replications.
Effects of JF-22 on Plant Growth and Development
The biological functions of B. licheniformis strain JF-22 were verified by a pot test. Compared with the control treatment CK, JF-22 significantly increased tomato biomass by 18.41%. The application of B. licheniformis strain JF-22 significantly reduced the severity of the root-nematode disease, indicating that B. licheniformis effectively controlled M. incognita (Table 2).
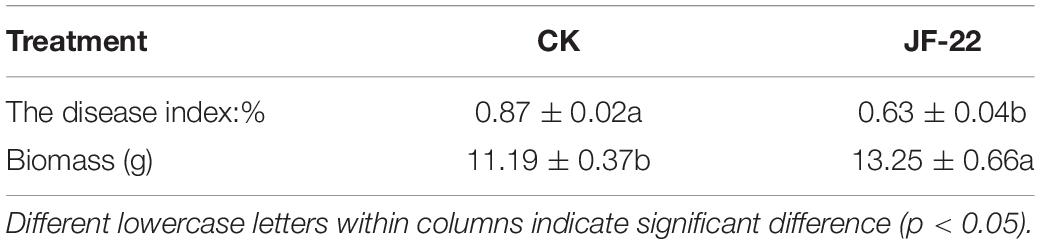
Table 2. Effects of Bacillus licheniformis strain JF-22 on tomato plant growth and root-nematode disease severity.
Analysis of Volatile Organic Compounds by Gas Chromatography–Mass Spectrometry
The types and relative contents of VOCs produced by the JF-22 fraction were analyzed using SPME–GC–MS. Figure 2 and Table 3 show SPME–GC–MS results for VOCs produced by JF-22 strain. Volatiles produced by JF-22 were identified using NIST17 and NIST17s (National Institute of Standards and Technology) standard mass spectrometry libraries. Among VOCs produced by JF-22, acetoin and 2,3-Butanediol, [R-(R*,R*)]- were the main components, with the relative peak areas of 64.2 and 7.89%, respectively, followed by cyclotrisiloxane, hexamethyl-; cyclotetrasiloxane, octamethyl-; 3-Hexanol, 2-methyl-; cyclopentasiloxane, decamethyl-; and 3-Pentanol and silanediol, dimethyl-.
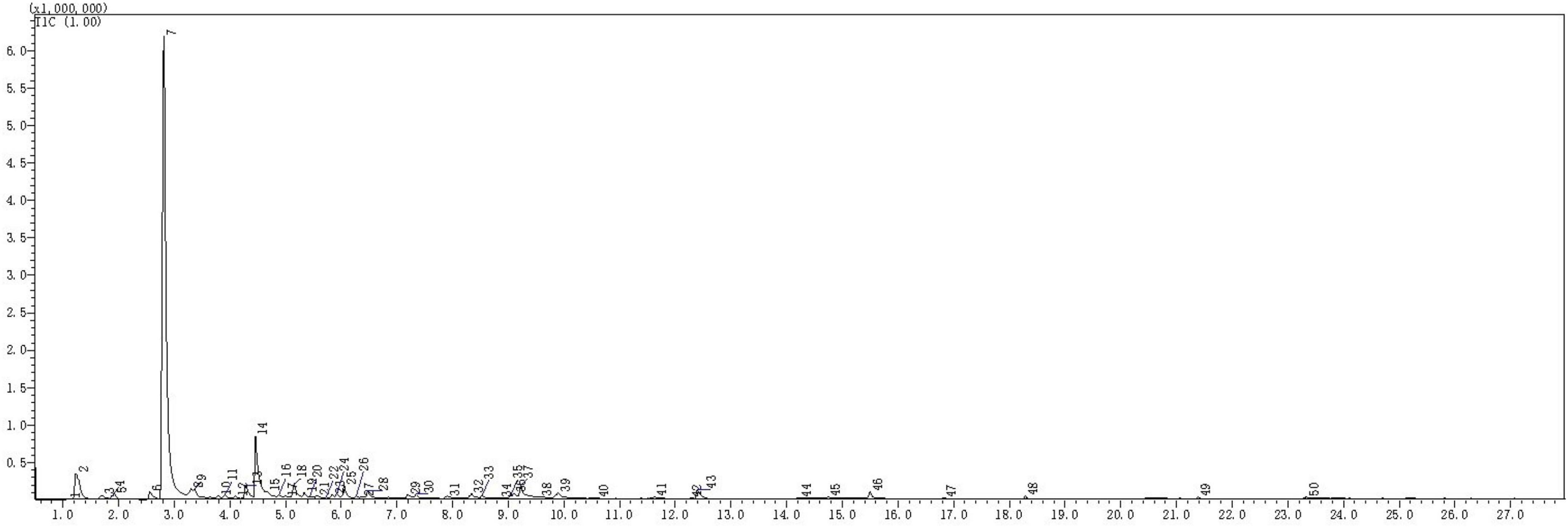
Figure 2. Gas chromatography–mass spectrometry (GC–MS) chromatogram of volatile organic compounds (VOCs) produced by JF-22 strain.
Analysis of Data Characteristic of Soil Samples and Microbial Community Diversity
After sequence optimization, there were 252,803 sequences in the two treatment groups, with an average of 42,134 in each soil sample of bacteria. And there were a total of 234,239 ITS sequences, with an average of 39,040 in each soil sample. The sequence generated by sequencing was clustered and segmented at a similarity level of 97%, and corresponding bacterial and fungal operational taxonomic units (OTUs) were generated, respectively. The bacterial community structure diversity indexes Shannon and Chao in the CK and JF-22 groups didn’t change significantly. However, in the richness index of fungal community, the fungal diversity indexes Shannon and Chao of the JF-22 group decreased significantly compared to CK (Table 4).
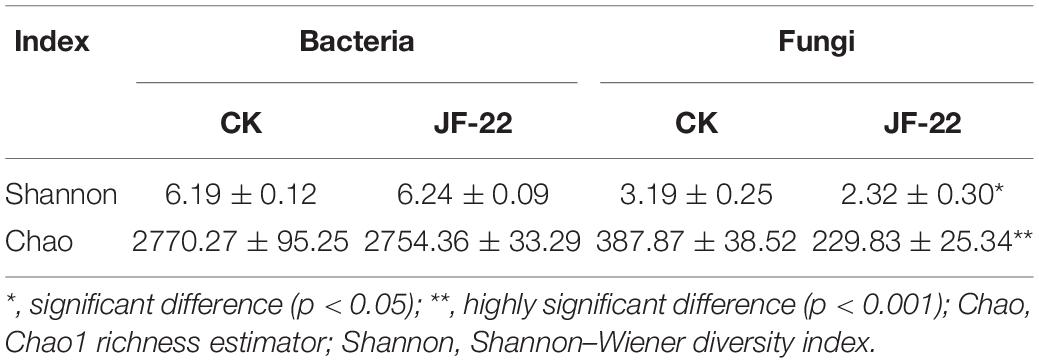
Table 4. Diversity and richness indices of microbial communities from the CK and JF-22 experimental groups.
Comparative Analysis of Microbial Community Composition Between JF-22-Treated and Untreated Soils
Using high-throughput sequencing, we analyzed the effects of JF-22 inoculants on the tomato–rhizosphere microbial communities. PCoA analysis based on unweighted UniFrac distance analysis showed that B. licheniformis strain JF-22 changed the composition of the microbial community in the tomato rhizosphere, particularly, that of the fungal communities (Figures 3A,B). Further, the bacterial community in the tomato rhizosphere soil across treatments was mainly composed of 25 families whose members were the same across treatments, although the relative abundance of each member differed. Thus, for example, abundance of Norank_c_Subgroup_6 and Nocardioidaceae differed greatly among treatments. In the CK treatment, the relative abundance of norank_c_Subgroup_6 was 3.6%, but in the JF-22-treated pots, the relative abundance of norank_c_Subgroup_6 was 6.6%, and the relative abundance of Nocardioidaceae was 6.3, and 3.9% in the CK and the JF-22 treatment groups, respectively (Figure 3C).
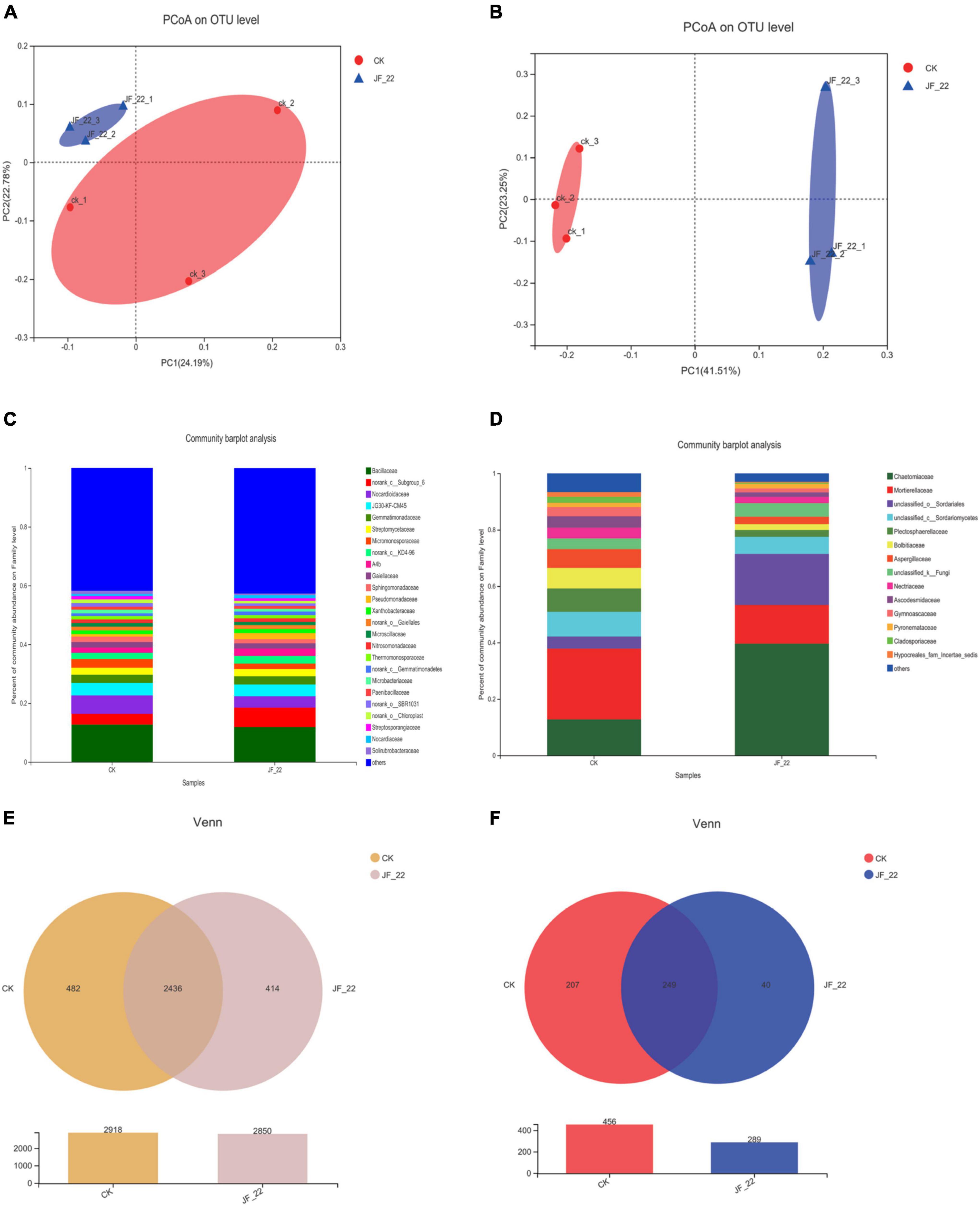
Figure 3. The effect of JF-22 to tomato rhizosphere soil microbial community. (A) PCoA analysis based on the unweighted unifrac distances of bacterial community. (B) PCoA analysis based on unweighted unifrac distances of fungal community. (C) The relative abundance in family of tomato rhizosphere bacterial. (D) The relative abundance in family of tomato rhizosphere fungi. (E) Venn diagram of OTUs between CK- and JF-22 relate bacterial; (F) Venn diagram of OTUs between CK and JF-22 relate fungi.
The fungal community in the tomato rhizosphere was mainly composed of 14 families across treatments. The overall composition of the rhizosphere fungal communities was similar in all treatments, but the abundance of each member was different. Specifically, Chaetomiaceae showed the largest difference between treatments. In CK pots, the relative abundance of Chaetomiaceae was 12.6%, while it was 39.7% in JF-22-treated pots. Similarly, Mortierellaceae and unclassified _o_Sordariales differed between treatments. In CK, the relative abundance of Mortierellaceae was 25.2%, while it was 13.6% in JF-22-treated pots. Furthermore, the relative abundance of unclassified _o_Sordariales was 4.3 and 18.2% in the CK and JF-22-treated pots, respectively (Figure 3D).
The number of unique and common OTUs in the multiple samples is shown in a Venn diagram. In the case of bacteria, 2,918 OTUs were identified in CK and 2,850 OTUs were found in JF-22, among which, 2,436 were common to both CK and JF-22-treated pots, while 482 were unique to CK and 414 to JF-22-treated pots (Figure 3E). As for fungi, 456 OTUs were identified in CK and 289 in JF-22-treated pots. Among them, 249 were common to both CK and JF-22-treated pots (Figure 3F). These findings indicated that the application of JF-22 can change the composition of the tomato rhizosphere–soil microbial community.
Prediction of Rhizosphere Microbial-Community Function
FUNGuild was used to analyze the functional guild annotation of the fungi present across treatments. In the CK group, the number of plant pathogens was 2,516, and the number of Dung Saprotrophs and Soil Saprotrophs was 1,221; meanwhile, in the JF-22 group, the number of plant pathogens was 739, and the number of Dung Saprotrophs and Soil Saprotrophs was 737, indicating that B. licheniformis strain JF-22 may reduce the number of plant pathogens, pathogenic bacteria, dung saprotrophs, and soil saprotrophs (Figure 4A). Compared to the results of FUNGuild, the results of the CoG function classification did not differ much between the experimental treatment groups (Figure 4B). All samples were rich in energy production and conversion (relative abundance 7.41–7.46%); amino acid transport and metabolism (8.85–8.95%); nucleotide transport and metabolism (2.36–2.40%); carbohydrate transport and metabolism (6.31–6.37%); coenzyme transport and metabolism (4.12–4.13%); lipid transport and metabolism (4.63–4.81%); translation, ribosomal structure, and biogenesis (5.13–5.14%); transcription (6.82–6.96%); replication recombination and repair (5.40–5.46%); cell wall/membrane/envelope biogenesis (5.98–6.14%); post-translational modification, protein turnover, chaperones (3.96–4.00%); inorganic ion transport and metabolism (5.69–5.69%); secondary metabolites biosynthesis, transport, and catabolism (2.69–2.79%); general function prediction only (8.71–8.74%); and signal transduction mechanisms (6.35–6.50%). These COG functional classification results indicate that the microbial communities of tomato rhizosphere–soil bacteria maintained similar biological functions in all treatment groups.
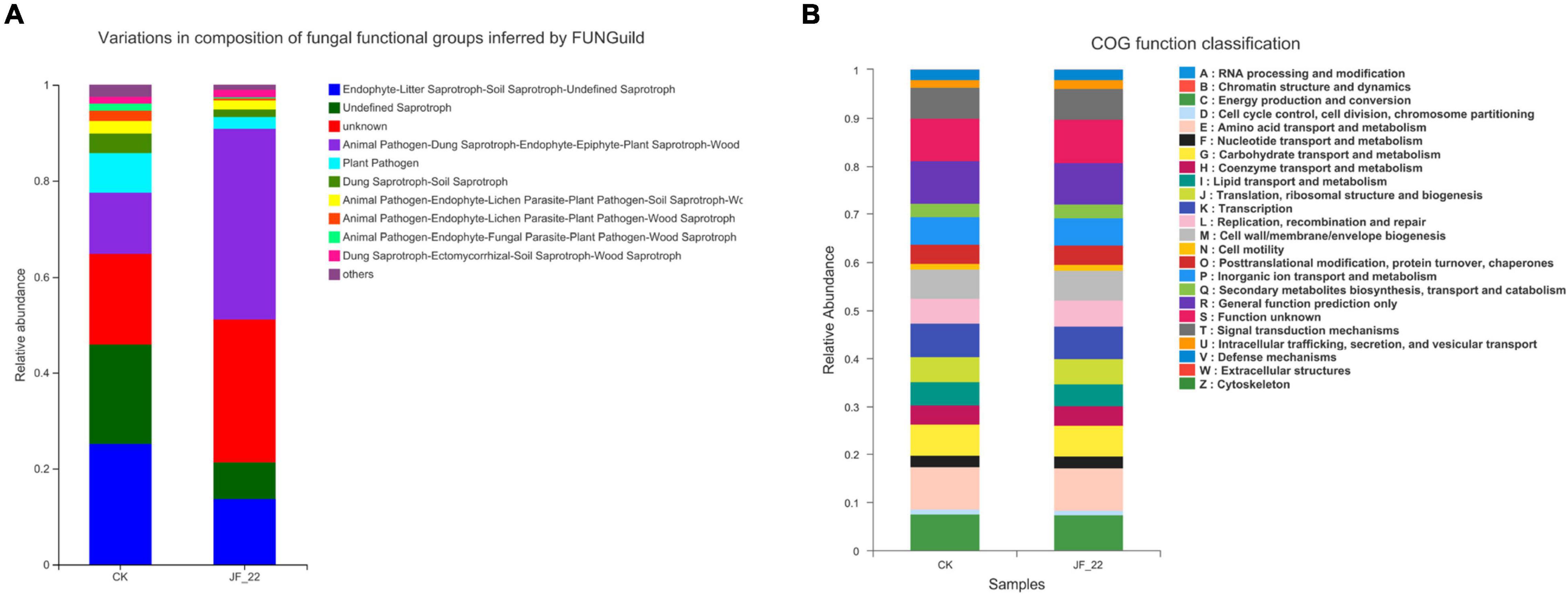
Figure 4. Analysis of the function of the rhizosphere microbial community. (A) COG function classification analysis, and (B) FUNGuide functional classification statistical histogram.
Discussion
Plant growth-promoting rhizobacteria are important and beneficial rhizosphere microorganisms (Babalola, 2010). The volatile substances produced by PGPR can reduce the incidence of root-knot nematode disease by poisoning nematodes and via other mechanisms (Siddiqui and Mahmood, 1999). B. cereus, B. licheniformis, Lactobacillus sphaeroides, P. fluorescens, and P. brassicae were used in a greenhouse test against M. incognita infection, and the results showed that B. licheniformis and P. fluorescens significantly reduced the infection of tomato roots by second-stage juveniles of M. incognita (Colagiero et al., 2017). The endophytic bacterium B. cereus BCM2 can affect the rhizosphere secretions (2,4-di-tert-butylphenol, 3,3-dimethyloctane, and n-tridecane), thereby inhibiting the second-stage juveniles of M. incognita and reducing the number of nematode specimens in the soil (Li et al., 2019). Similarly, Bacillus aryabhattai A08 can reduce the number of M. incognita specimens in the soil (Viljoen et al., 2019). As for the experiments reported herein, our data clearly demonstrated a toxic effect of B. licheniformis JF-22 on M. incognita. Specifically, our pot experiment showed that the JF-22 strain of B. licheniformis effectively prevented infection by M. incognita and alleviated the negative effects of M. incognita infection on tomato growth. Beneficial microorganisms can effectively prevent soil-borne diseases through the secretion of metabolites. For example, Bacillus velezensis FZB42 significantly inhibited the plant pathogen Ralstonia solanacearum by secreting benzaldehyde and 1,3-butadiene (Borriss et al., 2019).
This study analyzed the types of VOCs produced by JF-22 using SPME–GC–MS and found many ingredients, such as cyclotrisiloxane and hexamethyl- which reportedly can be toxic to the root-knot Meloidogyne (Hamouda, 2013). Further, acetoin can produce 2,3-Butanediol via a series of enzymatic reactions (Cui et al., 2021). In turn, 2,3-Butanediol can induce the stomatal closure under stress conditions. Additionally, it induces plant system tolerance; affects salicylic acid, jasmonic acid, and ethylene signaling channels; and promotes plant growth by regulating the expression of genes related to cell wall structure (Yi et al., 2016; Wu et al., 2018). Consistently, the application of Sphingomonas sp. Cra20 changes the rhizosphere native bacterial community and promotes the growth of Arabidopsis thaliana by driving root developmental plasticity, thereby stimulating the growth of lateral roots and root hairs (Luo et al., 2019). Thokchom demonstrated that the inoculation with PGPR and plant age can affect bacterial communities in root tissues and rhizosphere soil (Thokchom et al., 2017).
Rhizosphere microorganisms are important because they can manage nutrient transformation, nutrient acquisition and use, and crop sustainability (Prasad et al., 2017). Rhizosphere microflora enhances the plant growth under abiotic stress through nitrogen fixation, plant hormone production, mineral solubilization, and iron carrier and HCN production. Furthermore, it activates the plant defense mechanisms against different bacterial and fungal pathogens (Mukhtar et al., 2019). Thus, the composition of rhizosphere–soil microbial communities plays an important role in the stability of plant, soil, and rhizosphere microbial communities (Lu et al., 2018). Further, syringic acid reportedly changes the community composition of bacteria and fungi in the cucumber rhizosphere, which may negatively impact the growth of cucumber seedlings by inhibiting the growth of plant-beneficial microorganisms (Wang et al., 2018). In contrast, PGPR can affect the microbial community succession and increase the plant yields (Zhang et al., 2019). Rhizosphere microorganisms play an important role in most ecosystem processes, and different crop management strategies applied to agricultural production can change the soil microbial composition. Further, VOCs produced by the beneficial microorganisms in the rhizosphere soil affect the rhizosphere microbial communities (Netzker et al., 2020). The present study shows that, in addition to inhibiting M. incognita infection, strain JF-22 changed the composition of the microbial community in the tomato rhizosphere and significantly altered the diversity of the fungal community. Such change was likely caused by volatile substances secreted by JF-22. However, the role of volatile organic compounds in the microbial community of strain JF-22 needs to be further studied to reveal the mechanism of strain JF-22-microbial community.
Conclusion
This study used high-throughput and bioinformatics technology to study the effect of the JF-22 strain of B. licheniformis on the rhizosphere-soil microbial community and tomato plant growth. Strain JF-22 changed the composition of the microbial community in the tomato rhizosphere and significantly altered the diversity of the fungal community. Such change was likely caused by volatile substances secreted by JF-22. Using the FUNGuild and PICRUSt databases, we predicted the effect of JF-22 on microbial community function. Based on the demonstrated ability of JF-22 to control M. incognita, we believe that strain JF-22 of B. licheniformis can be considered a potential biocontrol agent for M. incognita.
Data Availability Statement
The datasets presented in this study can be found in online repositories. The names of the repository/repositories and accession number(s) can be found below: NCBI SRA database, accession numbers PRJNA803317 and PRJNA803323.
Author Contributions
XL, JD, and QG conceived and designed the experiment. XL, JD, QG, and CJ performed the experiment. XL, JD, QG, CJ, XS, YL, HL, CL, PZ, and JL analyzed the data. XL, JD, and QG wrote the manuscript. All authors read and approved the final manuscript.
Funding
This research was funded by the Shandong forestry science and technology innovation project (2019ly003-5), the West Coast Science and Technology Foundation of Qingdao (2019-23), Major scientific and technological innovation project of Shandong Province (2019jzzy020614).
Conflict of Interest
The authors declare that the research was conducted in the absence of any commercial or financial relationships that could be construed as a potential conflict of interest.
Publisher’s Note
All claims expressed in this article are solely those of the authors and do not necessarily represent those of their affiliated organizations, or those of the publisher, the editors and the reviewers. Any product that may be evaluated in this article, or claim that may be made by its manufacturer, is not guaranteed or endorsed by the publisher.
Footnotes
References
Aballay, E., Prodan, S., Correa, P., and Allende, J. (2020). Assessment of rhizobacterial consortia to manage plant parasitic nematodes of grapevine. Crop Prot. 131:105103. doi: 10.1016/j.cropro.2020.105103
Abarenkov, K., Henrik Nilsson, R., Larsson, K.-H., Alexander, I. J., Eberhardt, U., Erland, S., et al. (2010). The UNITE database for molecular identification of fungi – recent updates and future perspectives. New Phytol. 186, 281–285. doi: 10.1111/j.1469-8137.2009.03160.x
Amato, K., Yeoman, C., Kent, A., Righini, N., Carbonero, F., Estrada, A., et al. (2013). Habitat degradation impacts black howler monkey (Alouatta pigra) gastrointestinal microbiomes. ISME. J. 7:1344. doi: 10.1038/ismej.2013.16
Babalola, O. O. (2010). Beneficial bacteria of agricultural importance. Biotechnol. Lett. 32, 1559–1570. doi: 10.1007/s10529-010-0347-0
Bhattacharyya, P. N., and Jha, D. K. (2012). Plant growth-promoting rhizobacteria (PGPR): emergence in agriculture. World J. Microbiol. Biotechnol. 28, 1327–1350. doi: 10.1007/s11274-011-0979-9
Borriss, R., Wang, C., Song, X., Ding, X., Wu, L., Wu, H., et al. (2019). Corrigendum: Bacillus velezensis FZB42 in 2018: the gram-positive model strain for plant growth promotion and biocontrol. Front. Microbiol. 10:1279. doi: 10.3389/fmicb.2019.01279
Burkett, M., Kokalis-Burelle, N., Lawrence, K., van Santen, E., and Kloepper, J. (2008). Suppressiveness of root-knot nematodes mediated by rhizobacteria. Biol. Control 47, 55–59. doi: 10.1016/j.biocontrol.2008.07.008
Colagiero, M., Rosso, L., and Ciancio, d. A. (2017). Diversity and biocontrol potential of bacterial consortia associated to root-knot nematodes. Biol. Control 120, 11–16. doi: 10.1016/j.biocontrol.2017.07.010
Cui, Z., Wang, Z., Zheng, M., and Chen, T. (2021). Advances in biological production of acetoin: a comprehensive overview. Crit. Rev. Biotechnol. 21, 1–22. doi: 10.1080/07388551.2021.1995319
Du, B., Xu, Y. M., Dong, H., Li, Y., and Wang, J. (2020). Phanerochaete chrysosporium strain B-22, a nematophagous fungus parasitizing Meloidogyne incognita. PLoS One 15:e0216688. doi: 10.1371/journal.pone.0216688
Fravel, D. R. (2005). Commercialization and implementation of biocontrol 1. Ann. Rev. phytopathol. 43, 337–359. doi: 10.1146/annurev.phyto.43.032904.092924
Hamouda, R. (2013). Biocontrol of root knot nematode, Meloidogyne incognita infected banana plants by Cyanobacteria. Egypt. J. Agronematol 12, 113–129.
Hinsinger, P., Bengough, A., Vetterlein, D., and Young, I. (2009). Rhizosphere: biophysics, biogeochemistry and ecological relevance. Plant Soil 321, 117–152. doi: 10.1007/s11104-008-9885-9
Jing, T.-f, Zhang, D.-x, Pan, S., Liu, G., Mu, W., Hou, Y., et al. (2020). Phenyl isocyanate-Modified avermectin b1a improves the efficacy against plant-parasitic nematode diseases by facilitating its soil mobility. ACS Sustain. Chem. Eng. 8, 2310–2319. doi: 10.1021/acssuschemeng.9b07243
Kumar, A., Prakash, A., and Johri, B. N. (2001). “Bacillus as PGPR in crop ecosystem,” in Bacteria in Agrobiology:Crop Ecosystems, ed. D. K. Maheswari (Berlin: Springer), 37–59. doi: 10.1007/978-3-642-18357-7_2
Li, X., Hu, H.-J., Li, J.-Y., Wang, C., Chen, S.-L., and Yan, S.-Z. (2019). Effects of the endophytic bacteria bacillus cereus bcm2 on tomato root exudates and meloidogyne incognita infection. Plant Dis. 103, 1551–1558. doi: 10.1094/PDIS-11-18-2016-RE
Liu, G., Lin, X., Xu, S., Liu, G., Liu, F., and Mu, W. (2020). Screening, identification and application of soil bacteria with nematicidal activity against root-knot nematode (Meloidogyne incognita) on tomato. Pest Manage. Sci. 76, 2217–2224. doi: 10.1002/ps.5759
Lu, H., Xu, S., Zhang, W., Xu, C., Li, B., Zhang, D., et al. (2017). Nematicidal Activity of trans-2-hexenal against southern root-knot nematode (Meloidogyne incognita) on tomato plants. J. Agri. Food Chem. 65, 544–550. doi: 10.1021/acs.jafc.6b04091
Lu, S., Lepo, J. E., Song, H.-X., Guan, C.-Y., and Zhang, Z.-H. (2018). Increased rice yield in long-term crop rotation regimes through improved soil structure, rhizosphere microbial communities, and nutrient bioavailability in paddy soil. Biol. Fertil. Soils 54, 909–923. doi: 10.1007/s00374-018-1315-4
Luo, Y., Wang, F., Huang, Y., Zhou, M., Gao, J., Yan, T., et al. (2019). Sphingomonas sp. Cra20 increases plant growth rate and alters rhizosphere microbial community structure of Arabidopsis thaliana under drought stress. Front. Microbiol. 10:1221. doi: 10.3389/fmicb.2019.01221
Mukhtar, S., Mehnaz, S., and Malik, K. A. (2019). Microbial diversity in the rhizosphere of plants growing under extreme environments and its impact on crop improvement. Environ. Sustain. 2, 329–338. doi: 10.1007/s42398-019-00061-5
Netzker, T., Shepherdson, E. M. F., Zambri, M. P., and Elliot, M. A. (2020). Bacterial Volatile Compounds: Functions in Communication. Cooperation, and Competition. Ann. Rev. Microbiol. 74, 409–430. doi: 10.1146/annurev-micro-011320-015542
Nguyen, N., Song, Z., Bates, S., Branco, S., Tedersoo, L., Menke, J., et al. (2015). FUNGuild: an open annotation tool for parsing fungal community datasets by ecological guild. Fungal Ecol. 20, 241–248. doi: 10.1016/j.funeco.2015.06.006
Prasad, M., Chaudhary, M., Choudhary, M., Kumar, T., Jat, L., Meena, et al. (2017). “Rhizosphere microorganisms towards soil sustainability and nutrient acquisition,” in Agriculturally Important Microbes for Sustainable Agriculture (Singapore: Springer), 31–49. doi: 10.3390/ijms22136852
Roberts, P., Gundy, S., and McKinney, H. (1981). Effects of Soil Temperature and Planting Date of Wheat on Meloidogyne incognita Reproduction. Soil Populations, and Grain Yield. J. Nematol. 13, 338–345.
Siddiqui, I. A., and Shaukat, S. (2002). Rhizobacteria-mediated Induction of Systemic Resistance (ISR) in Tomato against Meloidogyne javanica. J.Phytopathol. 150, 469–473. doi: 10.1046/j.1439-0434.2002.00784.x
Siddiqui, I. A., Shaukat, S. S., Sheikh, I. H., and Khan, A. (2006). Role of cyanide production by Pseudomonas fluorescens CHA0 in the suppression of root-knot nematode. Meloidogyne javanica in tomato. World J.Microbiol. Biotechnol. 22, 641–650. doi: 10.1007/s11274-005-9084-2
Siddiqui, Z. A., and Mahmood, I. (1999). Role of bacteria in the management of plant parasitic nematodes: a review. Bioresour. Technol. 69, 167–179. doi: 10.1016/s0960-8524(98)00122-9
Sui, J., Ji, C., Wang, X., Liu, Z., Sa, R., Hu, Y., et al. (2019). A plant growth-promoting bacterium alters the microbial community of continuous cropping poplar trees’ rhizosphere. J. Appl. Microbiol. 126, 1209–1220. doi: 10.1111/jam.14194
Thokchom, E., Thakuria, D., Kalita, M., Sharma, C., and Talukdar, N. C. T. (2017). Root colonization by host-specific rhizobacteria alters indigenous root endophyte and rhizosphere soil bacterial communities and promotes the growth of mandarin orange. Eur. J. Soil Biol. 79, 48–56. doi: 10.1016/j.ejsobi.2017.02.003
Viljoen, J. J. F., Labuschagne, N., Fourie, H., and Sikora, R. A. (2019). Biological control of the root-knot nematode Meloidogyne incognita on tomatoes and carrots by plant growth-promoting rhizobacteria. Trop. Plant Pathol. 44, 284–291. doi: 10.1007/s40858-019-00283-2
Wang, X., Xiao, C., Ji, C., Liu, Z., Song, X., Liu, Y., et al. (2021). Isolation and characterization of endophytic bacteria for controlling root rot disease of Chinese jujube. J. App.l Microbiol. 130, 926–936. doi: 10.1111/jam.14818
Wang, Z., Zhang, J., Wu, F., and Zhou, X. (2018). Changes in rhizosphere microbial communities in potted cucumber seedlings treated with syringic acid. PLoS One 13:e0200007. doi: 10.1371/journal.pone.0200007
Wu, L., Li, X., Ma, L., Borriss, R., Wu, Z., and Gao, X. (2018). Acetoin and 2,3-butanediol from Bacillus amyloliquefaciens induce stomatal closure in Arabidopsis thaliana and Nicotiana benthamiana. J. Exp. Bot. 69, 5625–5635. doi: 10.1093/jxb/ery326
Xiang, N., Lawrence, K. S., and Donald, P. A. (2018). Biological control potential of plant growth-promoting rhizobacteria suppression of Meloidogyne incognita on cotton and Heterodera glycines on soybean: a review. J. Phytopathol. 166, 449–458. doi: 10.1111/jph.12712
Xiang, N., Lawrence, K. S., Kloepper, J. W., Donald, P. A., McInroy, J. A., and Lawrence, G. W. (2017). Biological Control of Meloidogyne incognita by spore-forming plant growth-promoting rhizobacteria on cotton. Plant Dis. 101, 774–784. doi: 10.1094/PDIS-09-16-1369-RE
Yi, H.-S., Ahn, Y.-R., Song, G., Ghim, S.-Y., Lee, S., Lee, G., et al. (2016). Impact of a bacterial volatile 2,3-butanediol on Bacillus subtilis rhizosphere robustness. Front. Microbiol. 7:993. doi: 10.3389/fmicb.2016.00993
Zhang, D., Shen, X., Zhang, H., Huang, X., He, H., Ye, J., et al. (2022). Integrated transcriptomic and metabolic analyses reveal that ethylene enhances peach susceptibility to Lasiodiplodia theobromae-induced gummosis. Hortic. Res. 9:uhab019. doi: 10.1093/hr/uhab019
Zhang, Y., Gao, X., Shen, Z., Zhu, C., Jiao, Z., Rong, L., et al. (2019). Pre-colonization of PGPR triggers rhizosphere microbiota succession associated with crop yield enhancement. Plant Soil 439, 553–567. doi: 10.1007/s11104-019-04055-4
Keywords: Bacillus licheniformis, microbial community, volatile substances, Meloidogyne incognita, microbial community composition
Citation: Du J, Gao Q, Ji C, Song X, Liu Y, Li H, Li C, Zhang P, Li J and Liu X (2022) Bacillus licheniformis JF-22 to Control Meloidogyne incognita and Its Effect on Tomato Rhizosphere Microbial Community. Front. Microbiol. 13:863341. doi: 10.3389/fmicb.2022.863341
Received: 27 January 2022; Accepted: 07 March 2022;
Published: 07 April 2022.
Edited by:
Amin Uddin Mridha, University of Chittagong, BangladeshReviewed by:
Laith Khalil Tawfeeq Al-Ani, Universiti Sains Malaysia, MalaysiaAnastasia Venieraki, Agricultural University of Athens, Greece
Copyright © 2022 Du, Gao, Ji, Song, Liu, Li, Li, Zhang, Li and Liu. This is an open-access article distributed under the terms of the Creative Commons Attribution License (CC BY). The use, distribution or reproduction in other forums is permitted, provided the original author(s) and the copyright owner(s) are credited and that the original publication in this journal is cited, in accordance with accepted academic practice. No use, distribution or reproduction is permitted which does not comply with these terms.
*Correspondence: Xunli Liu, xlliu@sdau.edu.cn
†These authors have contributed equally to this work