- 1Plant Microbe Interaction Laboratory, Department of Microbiology, Maharshi Dayanand University, Rohtak, India
- 2Centre for Medical Biotechnology, Maharshi Dayanand University, Rohtak, India
Plant growth and development are positively regulated by the endophytic microbiome via both direct and indirect perspectives. Endophytes use phytohormone production to promote plant health along with other added benefits such as nutrient acquisition, nitrogen fixation, and survival under abiotic and biotic stress conditions. The ability of endophytes to penetrate the plant tissues, reside and interact with the host in multiple ways makes them unique. The common assumption that these endophytes interact with plants in a similar manner as the rhizospheric bacteria is a deterring factor to go deeper into their study, and more focus was on symbiotic associations and plant–pathogen reactions. The current focus has shifted on the complexity of relationships between host plants and their endophytic counterparts. It would be gripping to inspect how endophytes influence host gene expression and can be utilized to climb the ladder of “Sustainable agriculture.” Advancements in various molecular techniques have provided an impetus to elucidate the complexity of endophytic microbiome. The present review is focused on canvassing different aspects concerned with the multidimensional interaction of endophytes with plants along with their application.
Introduction
Earth has been kneeling under the pressure of a rapidly increasing population which has exerted a lot of stress on the stakeholders, namely, farmers, scientists, and other intermediaries alike. What the world needs right now is extensive, yet a nature-friendly system of agriculture using modern tools along with systems sans application of chemical fertilizers (Lareen et al., 2016). The current system of agriculture is based on the application of chemical fertilizers and other inputs to enhance productivity, thereby leading to destruction of soil nutrients, groundwater contamination, eutrophication, and production of greenhouse gasses, thereby, impacting the overall environment and playing havoc to the health of consumers both humans and animals alike. To overcome this, microbes with plant growth promoting (PGP) traits are being explored to develop potential bioinoculants for sustainable and eco-friendly agriculture.
The plants and microorganisms are well known to interact by various natural amalgams that serve as signaling and nutritive substances for microbes to act upon and influence the nature of the plant microbiome. Plants are naturally associated with microorganisms in rhizosphere, phyllosphere, and endosphere (Dong et al., 2019). The rhizosphere is the tapered region of soil regulated by plant root secretions and associated microbial community termed as root microbiome (Turner et al., 2013; Suneja et al., 2016). Phyllosphere is the microbial habitat on the exterior of above-ground plant organs and the most abundant microbial ecosystem on earth (Vorholt, 2012). Microorganisms living and growing within their host plants are termed endophytes, constituting the plant endosphere. Endophytic bacteria usually complete their life cycle inside host plants without causing any harm to them (Dudeja et al., 2012). However, these bacteria flourish copiously in the rhizosphere due to sufficient nutrition supply by plant root exudates (Canarini et al., 2019).
The abundance of microorganisms in the rhizosphere is known since the beginning of the 20th Century but the endosphere region has not been explored much (Sessitsch et al., 2011). Earlier, the endosphere was known mainly for the fungal group and as a result, our preliminary information about bacterial endophytes remained circumscribed. Various other factors restricted our understanding regarding the action of bacterial endophytes, which includes culturing hitches and lack of pioneering identification techniques. However, endophytic bacteria have attracted a lot of attention since the last two decades owing to recognition of their ability to promote plant growth and their biocontrol potential (Vasileva et al., 2019). This review discusses the PGP endophytic bacteria, their interaction with host plants leading to variations in colonization patterns and diversity, mechanisms of plant growth promotion under normal as well as stress conditions along with omics-led revolution in the field of exploring their bioactive metabolites.
Plant Growth-Promoting Endophytic Bacteria–Host Plant: Interaction and Colonization
Endophytes (either bacteria or fungi) are defined as colonizers of the internal plant tissues without causing any disease or hostile symptoms and obtained from surface-sterilized tissue of plant (Santoyo et al., 2016; Afzal et al., 2019). Bacterial endophytes are known to be present in every plant part, namely, seeds, rhizomes, roots, nodules, stems, and leaves (Alibrandi et al., 2018). It has been anticipated that endophytic bacteria referred to as the subclass of rhizospheric bacteria or seed-borne bacterial communities, commonly termed as PGP rhizobacteria, established the ability to enter into the host plant (Khare et al., 2018). They possess all vital PGP traits as present in rhizobacteria, but their effect on host plants is typically more significant than rhizobacteria owing to the better adaptation during stress conditions resulting in augmentation of plant growth (Hardoim et al., 2008; Afzal et al., 2019).
The rhizosphere is the interaction point between roots and soil microorganisms (Bulgarelli et al., 2012). Plants release exudates such as organic acids, amino acids, and proteins from their roots, which serve as pre-communication signals between bacterial endophytes and host plants (Kawasaki et al., 2016). Colonization of bacteria into roots occurs through root hairs and, to some extent, through the stem and leaves (Maela, 2019). Some studies have reported that endophytes also colonize through flowers and fruits of the anthosphere and carposphere (Frank et al., 2017). A few regular hotspots have been observed for bacterial colonization such as emergence sites of lateral roots, outer layers of cells, and root cortex (dos Santos et al., 2018). Endophytic bacterial colonization is a multi-stage process that involves (a) chemotactic movement toward roots, (b) root surface attachment, (c) entry inside the root, and (d) movement and localization (Gupta et al., 2012; Kandel et al., 2017a). Table 1 cites various genes involved in the colonization of endophytes.
Bacteria, in the vicinity of the roots, receive chemical signals from root exudates and move toward them. Saleh et al. (2020) reported that citric acid, a root exudate of Brachypodium distachyon, acting as a strong chemoattractant for PGP bacterial strains. The hypothesis of Streptomyces species being attracted by the root exudates was tested and confirmed by Worsley et al. (2021) on the root exudates of Arabidopsis thaliana. The study demonstrated that phytohormone, salicylate, plays a specific role in this process. The genes for proteins encoding motility, chemotaxis, and adhesion are upregulated in response to root exudates, indicating a two-way interaction between the endophyte and its host plant (Jha et al., 2018). Chemotaxis is a significant event in the rhizosphere and the interior parts of roots, for both movement and colonization (Kawasaki et al., 2016). Mutant strains of Azorhizobium caulinodans lacking chemotaxis gene cluster (che) were reported to undergo defective colonization owing to its significant role in biofilm formation and exopolysaccharides (EPSs) production (Liu X. et al., 2018; Liu W. et al., 2018, Table 1). Bacterial endophytes primarily bind to the root surface (rhizoplane) and detect the possible entry sites for accessing internal plant tissues (Kandel et al., 2017a). The entry points used by endophytes to reach the host plant are the gaps present in the roots where root hairs or lateral roots arise, as well as the holes in the shoots, wounds, stomata, and hydathodes (Hardoim et al., 2015). Figure 1 illustrates distinct steps of colonization.
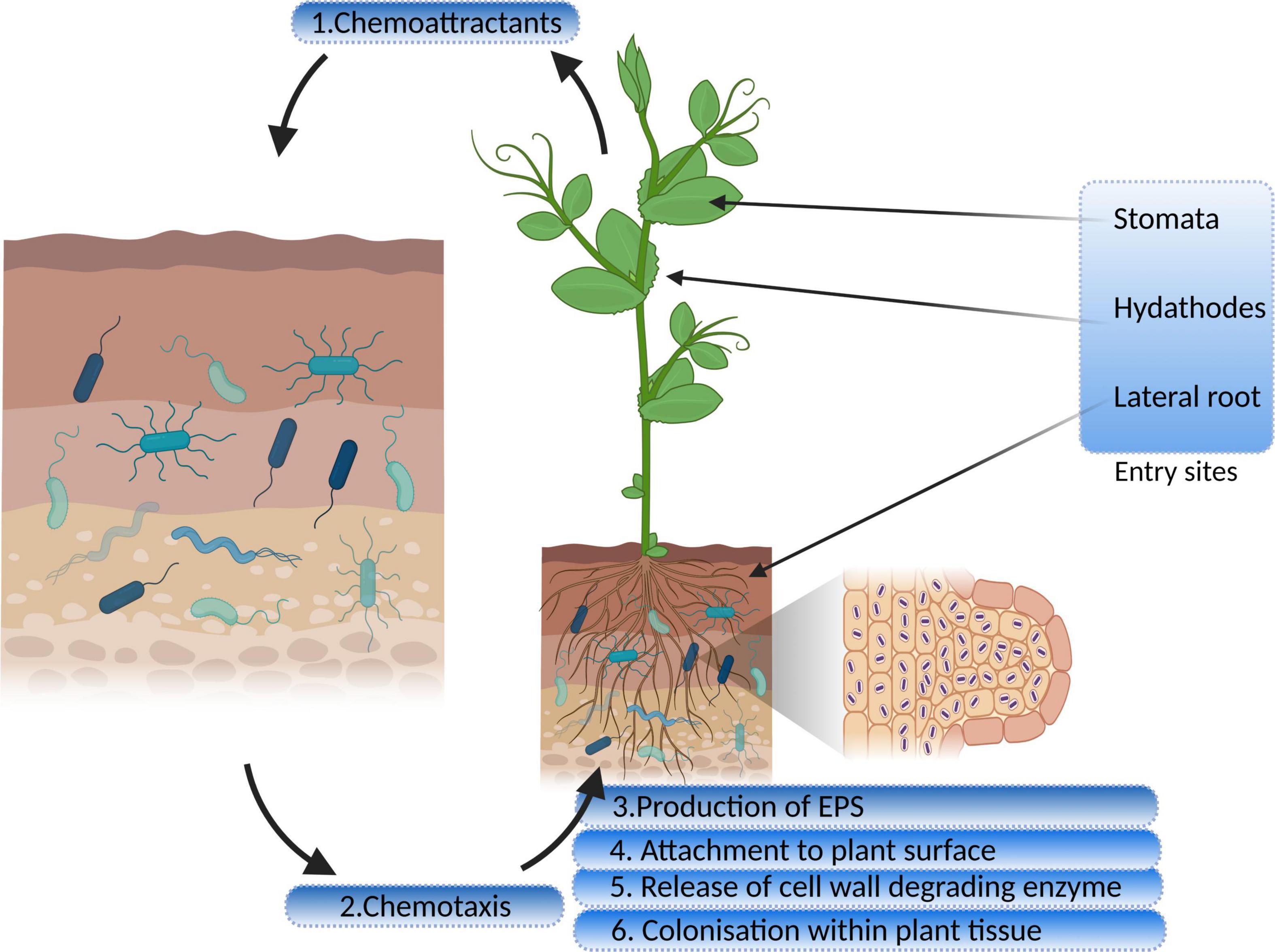
Figure 1. Interaction and colonization of plant growth-promoting endophytic bacteria in the host plant (EPS, exopolysaccharides).
Many researchers have stated that extensive bacterial endophyte colonization occurs at the secondary root emergence site. It is because of rapid endophytic penetration at epidermal breakage-point, colonizing at the cortex and subsequent spreading through the endodermis to the vascular tissue (Mahaffee, 1994; Lodewyckx et al., 2002). Endophytes release the cell wall–degrading enzymes such as pectinases, xylanases, cellulases, and endoglucanases before colonizing the roots (Naveed et al., 2013; Maela, 2019). This phenomenon facilitates the entry of bacteria within plant tissues (Kandel et al., 2017a). Various gene sequences have been deduced by comparative genomics, engaged in biofilm formation, adhesion, and motility, leading to plant colonization and maintaining healthy plant–microbe interaction. Bacterial cells synthesize EPSs during the early colonization phase which help the cells to adhere to the root surface. The endophytic strain Gluconacetobacter diazotrophicus produces EPS that serves as a critical factor for adhesion and colonization in rice roots (Meneses et al., 2011). The study showed that EPS production by G. diazotrophicus shielded the bacterial cells from oxidative damage, and also decreased the concentrations of free radicals. Colonization was found to be reduced in the case of EPS knockout strain of G. diazotrophicus, further rescued by the application of wild-type strain (Meneses et al., 2017). Fernández-Llamosas et al. (2021) used homologous recombination for insertional disruption of epsF genes in the genome of Azoarcus sp. CIB depicting their role in the efficient colonization of rice roots. Xu et al. (2021) identified EPS biosynthesis gene, blr2358, in Bradyrhizobium diazoefficiens USDA110, the mutant of which resulted in a reduced capacity to induce nodules. Other than playing a significant role in plant–endophyte interactions, they exhibit antioxidant, anti-inflammatory, anti-tumor, and prebiotic activities (Liu W. et al., 2018). Lipopolysaccharide machinery is involved in the attachment and proliferation of endophyte colonization that includes the development of flagella and pili, quorum sensing, and movement of bacteria within the host plants (Rocío Suaìrez-Moreno et al., 2010; Scharf et al., 2016). The role of cell wall degrading enzymes in entering and spreading within the host tissue is also very well established. Fan et al. (2016) highlighted the importance of endo-β-1, 4-glucanase in penetration of Bacillus amyloliquefaciens into the host tissue. The disruption mutant of eglS gene encoding this enzyme halted colonization; however, overexpression of the same resulted in a substantial increase in the endophyte population. Mechanism of bacterial endophyte attachment with plant surface, entry, survival, is mediated by the cross-talk between host and microorganism, and a lot is to be studied in this regard.
Endophytism vs. Pathogenicity: Thin Line Between Two Lifestyles
The prevalence of endophytes is decided by chance and genetic indicators of bacteria that promote intermodulation between bacteria and plants, contributing to an active colonization (Dong et al., 2019). Endophytes maintain a smaller cell density to prevent a systemic reaction in comparison to pathogens (Zinniel et al., 2002). They also produce lesser quantities of cell wall degrading enzymes as compared to the phytopathogens that secrete deleteriously large amounts of these enzymes, thereby, preventing the trigger of plant defense systems (Elbeltagy et al., 2000; Afzal et al., 2019). Anabolism-related genes are found to be more diverse and in abundance among the endophytes unlike the phytopathogens having catabolism genes prominently (Hardoim et al., 2015). Endophytes undergo several mechanisms to protect themselves from the plant defense system. Microbe-/pathogen-associated molecular patterns (MAMPs/PAMPs) are the characteristics of microbes recognized by pattern recognition receptors (PRRs) present on the surface of plant cells (Newman et al., 2013). Endophytic bacteria produce MAMPs which either remain unrecognized by plant’s PRRs or induce only a weak reaction as a response in comparison to the pathogenic interactions (Vandenkoornhuyse et al., 2015). They produce enzymes of antioxidant machinery such as superoxide dismutase (SOD), catalase (CAT), peroxidase (POD), glutathione S-transferases (GSTs), and alkyl-hydroperoxide reductase C (AhpC), to mitigate the oxidative burst (Zeidler et al., 2004). Bacterial virulence factors are delivered in the extracellular environment or directly into the host by the secretion system (Depluverez et al., 2016). Type-III and Type-VI protein secretion systems, necessary to deliver effector proteins into the plant by pathogens, are altogether absent or present scarcely in endophytic bacteria (Liu W. et al., 2018). Endophytes have been found to undergo a reduction in genome size, which is associated with differences in niche specialization (Lòpez-Fernàndez et al., 2015; Zin et al., 2021). Some bacterial endophytes also downregulate flagella biosynthesis and upregulate functions related to flagellar motor rotation to mask up their flagellin PAMPs and move fast within plants during colonization.
Endophytes have been reported to undergo a change in their lifestyles from endophytes to pathogenic as a result of any imbalance in the host–microbe interaction (Mengistu, 2020). Strategies employed by the plants to distinguish endophytes from pathogens are still a matter of active research. Plett and Martin (2018) have indicated that LysM receptor-like kinases (LysM-RLKs) can differentiate pathogenic signals from those secreted by the mutualistic microbes. It has been suggested that different groups of genes are regulated during colonization in the plants to facilitate the same. The majority of pathways targeted by miRNAs of plant defense system are turned off. These microRNAs otherwise remain stable and can be used as a pathogenicity signal by the plants (Wang et al., 2017). Plants undergo nutrient monitoring to identify parasites and manipulate the ratio of MAMP/DAMP signals to identify the mutualistic signals. However, there are many such receptor/perceptor systems present throughout the plant kingdom that are yet to be studied (Plett and Martin, 2018). Many studies have pointed toward downregulation of plant defense during colonization by mutualistic partners (Khare et al., 2018). In the recruitment of an endophytic companion, the plant host often plays a pivotal role, where the release of specific root exudates and a selective host plant defense response are considered as crucial factors in choosing individual endophytes (Kumar A. et al., 2020).
Apprehending the Endophytes
Enormous benefits provided by endophytes have led to robust research in this field world over. Harnessing their potential to the fullest and large scale application requires a more clear and better understanding of endophytes. It is no less than a challenge as the methods available for detection, isolation, and identification are not sufficient to provide the entire picture of the host–parasite interaction. Cultivation-based studies omit several microbes because it is not possible to reproduce and maintain the optimal conditions required for the growth of most of the microbes (Santoyo et al., 2016). However, the study of endophytes has come a long way from the typical isolation and cultivation methods to more sophisticated ones such as advanced microscopic techniques and “omics”-based studies (Table 2). The amalgamation of two or more techniques helps to significantly increase the discriminatory power of the analysis and a better overview of diversity. Therefore, a combination of techniques is employed to complement each other and to enrich our understanding of the detection and patterns of colonization as shown in Figure 2.
Microscopy is the sole direct method to observe the endophytes, which helps in understanding the mode of infection, tissue-specific concentration, and the extent of colonization along with the plant response (Johnston et al., 2006). Both light and electron microscopy can reveal the exact location of endophytes within the plant tissue (Kuldau and Yates, 2000). Electron microscopy provides us with the ultrastructural analysis of the endophytes (Pimentel Esposito-Polesi et al., 2017). Transmission electron microscopy (TEM) and scanning electron microscopy (SEM) yield valuable information about the inner structure and surface of the sample, respectively (Ramanujam et al., 2016). Electron microscopy has provided a lot of assistance in the detection of endophytes, the extent of colonization, interaction with the host, and establishment within the plant environment (War Nongkhlaw and Joshi, 2017). Fluorescence in situ hybridization is a powerful technique to analyze the microorganisms and screen various microbial communities employing group-specific probes. It involves targeting 16S rRNA gene’s conserved region or species-specific probe to observe the individual cells of endophytic bacteria (Castanheira et al., 2017). Green fluorescent protein (GFP) tagging and β-glucuronidase (GUS) staining rely on the broad host plasmids containing constitutively expressed GUS or GFP genes for tracking bacteria within the endosphere (Robertson-Albertyn et al., 2017). GFP-tagged endophytes fluoresce in the presence of UV or blue light and oxygen thus unfolding the information concerned with the success of colonization as well as the sites of entry (Reinhold-Hurek and Hurek, 2011).
Reactive oxygen species (ROS) staining is a more convenient and cost-effective method than the visualization techniques, for instance, TEM and Fluorescent microscopy. Similar to ROS, hydrogen peroxide (H2O2) and superoxide (O2–) radicals associated with the microbial invasion of eukaryotic cells are stained by 3,3′-diaminobenzidine tetrachloride (DAB) and nitroblue tetrazolium (NBT), respectively. DAB results in brown coloration, indicating the presence of endophytes in tissues (White et al., 2014). NBT stains superoxide radicals, which reduce NBT and it results in dark blue and water-insoluble formazan (Shinogi et al., 2003). It is used to detect endophytes in different plant tissues unlike DAB, which is not able to detect endophytes in shoot tissues because of the inability of DAB to penetrate the same.
Genomics-based studies such as next-generation sequencing and continued development in bioinformatics have allowed a significant improvement in our understanding of plant–endophyte interactions. Metagenomics and transcriptomics in particular are proving to be extremely useful for analyzing the functional characteristics of endophyte species (Kaul et al., 2016). Molecular methods are being readily employed to cultivate bacteria for their identification and enable to distinguish the bacterial populations in plant tissues (Afzal et al., 2019). These have allowed a more rigorous analysis of endophytic bacteria’s abundance and community composition (Santoyo et al., 2016). Different molecular methods used to characterize the endophytic bacteria include 16S rDNA sequencing, randomly amplified polymorphic DNA (RAPD), BOX-A1R-based repetitive extragenic palindromic PCR, amplified ribosomal DNA restriction analysis, enterobacterial repetitive intergeneric consensus, denaturing gradient gel electrophoresis (PCR-DGGE) and repetitive extra-genic palindromic sequence.
Ten bacterial endophytes were isolated and identified from three different cereals, Triticum aestivum, Oryza sativa, and Zea mays (Liu et al., 2017a). These isolates were classified by 879F-RAPD and 16S rDNA sequencing followed by clustering into seven groups signifying a clonal origin and assigned into four genera, Paenibacillus, Enterobacter, Pantoea, and Klebsiella. Recently, Zheng et al. (2020) identified root nodule endophytes from Sesbania cannabina and Glycine soja using PacBio’s circular consensus sequencing of full-length 16S rDNA gene for more accurate taxonomic information. These nodule isolates were assigned to 18 genera and 55 species, Ensifer being the predominant genera. PacBio technology helps in less ambiguous classification and provides finer taxonomic details. This technique has also recently been used to explore microbial communities in different samples (Singer et al., 2016; Motooka et al., 2017; Pootakham et al., 2017). Lastochkina et al. (2020) performed RAPD-PCR analysis to confirm the identity of Bacillus subtilis within the internal tissues of T. aestivum L. The endophytic diversity and detailed analysis of endophytic bacterial composition from the commercial crop (Paullinia cupana) of Brazil using PCR-DGGE were studied. The study disclosed the presence of phyla Firmicutes, Proteobacteria, Actinobacteria, Bacteroidetes, and Acidobacteria, Firmicutes being the predominant phylum (Bogas et al., 2015). The endophytic community of Distichlis spicata, Plucheaab sinthiodes, Gaultheria mucronate, and Hieracium pilosella growing in extreme environments of Chile (Atacama desert and Patagonia) was studied. The composition and diversity were analyzed using quantitative PCR and high-throughput gene sequencing of 16S rDNA. The endophytes from both the regions were categorized into phylum Proteobacteria, Firmicutes, Actinobacteria, and Bacteroidetes (Zhang et al., 2019). Upon extensive data mining of endophytic diversity from various plants, it has been observed that the members of phylum Proteobacteria, Actinobacteria, and Firmicutes were the most dominant (Rana et al., 2020; Bhutani et al., 2021).
Plant Growth Promoting Endophytic Bacteria: The Base of “Sustainable Agroecosystem”
Plant growth promoting endophytic bacteria (PGPEB) are well known to enhance the growth of plants directly and indirectly. They benefit directly to host by the concerted activity of biological nitrogen fixation, phytohormones production, phosphate solubilization, and modulation of 1-aminocyclopropane-1-carboxylic acid (ACC) deaminase expression for better growth under normal and stress conditions. Endophytes being in direct association with plants provide nitrogen in the functional form to their host either by fixing atmospheric nitrogen or by producing ammonia (Marques et al., 2010; Brígido et al., 2019). The frequent usage of nitrogen in the form of chemical fertilizers predominantly increases the cost of crop production. Hence, ammonia production by bacterial endophytes is an essential attribute for the selection of desirable bioinoculants (Li et al., 2017).
Solubilization and mineralization of phosphate are accomplished by bacterial endophytes, which assist in lowering the pH by releasing various organic acids that break Ca-bonded phosphorus in the bound form of soil (Sharma et al., 2013). Members of several genera have been reported as efficient phosphate solubilizers, such as Rhizobium, Bacillus, Serratia, Arthrobacter, Burkholderia, Pseudomonas, Erwinia, and Microbacterium (Oteino et al., 2015; Li et al., 2017; Srinivasan et al., 2018). The production of phytohormones by PGPEB is another mechanism that significantly boosts the growth of plants and alters the plant morphology (de Souza et al., 2015). IAA is a commonly produced auxin by endophytic bacteria that controls various growth processes in plants, including cell division, elongation, differentiation, gravity, and light responses (Bhutani et al., 2018a). Thus, it aids the host plant in nutrient absorption (Dhungana and Itoh, 2019). There have been numerous studies documenting IAA-producing endophytic bacteria in addition to endogenous IAA in plants (Khan and Doty, 2009; Mohite, 2013; Etesami et al., 2015; Bhutani et al., 2018b; Maheshwari et al., 2021). The application of IAA-producing bacteria to plants has demonstrated substantial upsurge in growth and yield. In addition to this, microbial IAA has been reported as a signaling molecule in several interactions between plants and microbes (Duca et al., 2014).
Gaseous phytohormone, ethylene and its precursor 1-aminocyclopropane 1-carboxylic acid (ACC), play a significant role in response to a wide range of stresses (Glick, 2014). Symbiotic bacteria ease the negative impact of ethylene on plants by expressing the ACC deaminase (ACCD). A variety of endophytic bacteria such as Azospirillum, Ralstonia, Pseudomonas, Rhizobium, Agrobacterium, Enterobacter, Achromobacter, and Bacillus mojavensis possess ACC deaminase gene and are characterized for ACCD activity (Blaha et al., 2006; Glick et al., 2007; Maheshwari et al., 2020). Transgenic varieties of plants have been developed which, by expressing the bacterial ACCD gene, have improved stress tolerance mechanisms (Stearns and Glick, 2003; Singh et al., 2015). But the addition of ACCD-producing endophytic bacteria could be more cost-effective, readily available, and environmentally sustainable, with higher acceptability compared to transgenics (Glick, 2012; Barnawal et al., 2016).
Plant growth-promoting endophytic bacteria–based biofertilizers can be envisioned as the future nutrient delivery system for plants. This approach, if carried out effectively, can bring about “real” green revolution, which will be more sustainable and reliable (Lugtenberg and Kamilova, 2009; Liu et al., 2017b). To develop these endophytes as microbial inoculants in agriculture, their functional characterization based on PGP traits and in vivo evaluation to check their efficacy should be the first prerequisite (de Souza et al., 2015; Alori and Babalola, 2018). Ferchichi et al. (2019) evaluated in vitro and in vivo efficacy of bacterial endophytes from three species of Lupinus. Two endophytes Paenibacillus glycanilyticus and Pseudomonas brenneri possessed desired functional characters and promoted plant growth in vivo and in vitro that can be developed as eco-friendly biofertilizers to boost up Lupinus productivity. Recently, the multifunctional potential of Paenibacillus polymyxa isolated from bulbs of Lilium lancifolium was assessed (Khan M. S. et al., 2020). The bacterial isolate possessed various PGP traits, including the production of IAA, siderophores, ACCD, fixation of nitrogen, and solubilization of phosphate. It also promoted the plant growth of different Lilium varieties under greenhouse conditions. The study demonstrated that the potential of P. polymyxa can be evaluated as an effective bioinoculant. Before any ex-planta application for the agricultural crop, some pre-requisites need to be fulfilled for selection as an inoculant. These factors include colonization ability in plant roots, competition with other microflora and their survival in soil, upsurge exudate production which acts as a bridge between plant and bacteria, and improvement of soil health (Beauregard et al., 2013; Carvalhais et al., 2013; Ríos-Ruiz et al., 2019). Seven bacterial endophytes were isolated from different legume crops, namely, Glycine max, Vigna unguiculata, Vigna mungo, Vigna radiata, and Arachis hypogaea and categorized on the basis of PGP traits. These isolates were used to bacterize the seeds of A. hypogaea for plant growth stimulation experiments using gnotobiotic systems and in pots. The results depicted a positive influence on A. hypogaea growth. Additional treatment given along with chemical fertilizer at 50% recommended dose, positively affected A. hypogaea growth, but the negative effect was seen over the bacterial population when the dose of fertilizers exceeded more than 50%. Their results suggested that root nodules harbor the endophytic population, which augments the growth of a plant, and the addition of fertilizers adversely affects their population and activity (Dhole et al., 2016). The 28 bacterial endophytes were isolated from dry and germinating seeds of Cicer arietinum and characterized for PGP attributes. Molecular identification analysis showed that these endophytes belong to Pseudomonas sp., Enterobacter sp., Bacillus sp., Mixta sp., and Pantoea sp. These were applied to C. arietinum roots and led to an increase in plant length, biomass, and chlorophyll content along with biocontrol activity against Fusarium oxysporum (Mukherjee et al., 2020). Maheshwari et al. (2019a) isolated and investigated endophytic bacteria from C. arietinum and Pisum sativum for PGP attributes. Most efficient isolates were identified as Pantoea agglomerans, Bacillus cereus, Bacillus sonorensis, Bacillus subtilis, Pseudomonas chlororaphis, Ornithibacillus sp., and Ochrobactrum sp. These studies convey direct evidence for the occurrence of valuable endophytes, which can be further harnessed as bioinoculants for improving plant health.
Inducing Climate Change Resilience in Flora
Plant community throughout the world is suffering in terms of growth, development, and yield as a result of climate change–induced environmental stress manifested in the form of drought, flood, temperature extremes, salinity, heavy metal toxicity combined with biotic stresses caused due to herbivores, pathogens, and so on. Enough studies have reported the significant role played by endophytes in mitigating abiotic and biotic stress (Rani et al., 2021). Different mechanisms have been deciphered revealing the complex regulation involved in the stress tolerance conferred by the endophytes to the host plants. Drought, salinity, and temperature extremes have been reported as the most devastating abiotic stresses for crops as far as the yield is concerned. Plants respond to drought and salinity via stomatal closure, reduced turgidity, osmotic stress, ultimately reducing growth and yield (Van Zelm et al., 2020). Increased evaporation induced by high temperature leads to water loss resulting in the formation of protein aggregates as a result of protein folding inhibition, whereas low temperature leads to the formation of ice crystals causing permanent damage to cells (Lamers et al., 2020). Extreme temperature alters membrane fluidity (Nicolson, 2014).
Endophytes alleviate these stresses by upregulating aquaporins, improving the level of abscisic acid, ACCD activity, enhancing enzymatic and non-enzymatic ROS scavenging machinery and osmolytes, higher expression of ion channels KAT1 and KAT2 resulting in decreased Na+/K+, adjusting gene expression, and reducing malondialdehyde (MDA) content (Cohen et al., 2015; Gond et al., 2015; Barnawal et al., 2016; Abdelaziz et al., 2017; Xu et al., 2017; Zhang et al., 2017; Sapre et al., 2018; Wang et al., 2018). Likewise, toxic metal ions evoke oxidative stress by generating ROS which promote DNA damage/impairment of DNA repair mechanisms, membrane functional integrity, nutrient homeostasis, and perturb protein function and activity (Tamás et al., 2014). Endophytes induce heavy metal tolerance to their hosts by reducing the mobility of heavy metals by chelation or intracellular sequestration and limiting the translocation of heavy metal ions from roots to shoots (Leonhardt et al., 2014). Table 3 describes various endophytes conferring abiotic stress protection to their hosts by different mechanisms.
Plant Growth Promoting Endophytic Bacteria-Assisted Biocontrol of Phytopathogens
Several microbes (viruses, bacteria, and fungi), nematodes, and insects are responsible for infecting plants leading to biotic stress. Physical barriers such as cuticle, wax, trichomes, etc., form first line of defense for the plants (Iqbal et al., 2021). Promoting the availability and absorption of nutrients, augmentation of stress tolerance and disease resistance of disease are the key means of plant disease control by endophytic bacteria (Hamilton et al., 2012). The most commonly reported bacterial genera with biocontrol activity are Bacillus, Actinobacteria, Enterobacter, Pseudomonas, Paenibacillus, and Serratia (Ek-Ramos et al., 2019). These mechanisms can be broadly grouped into direct and indirect biocontrol activities (Figure 3).
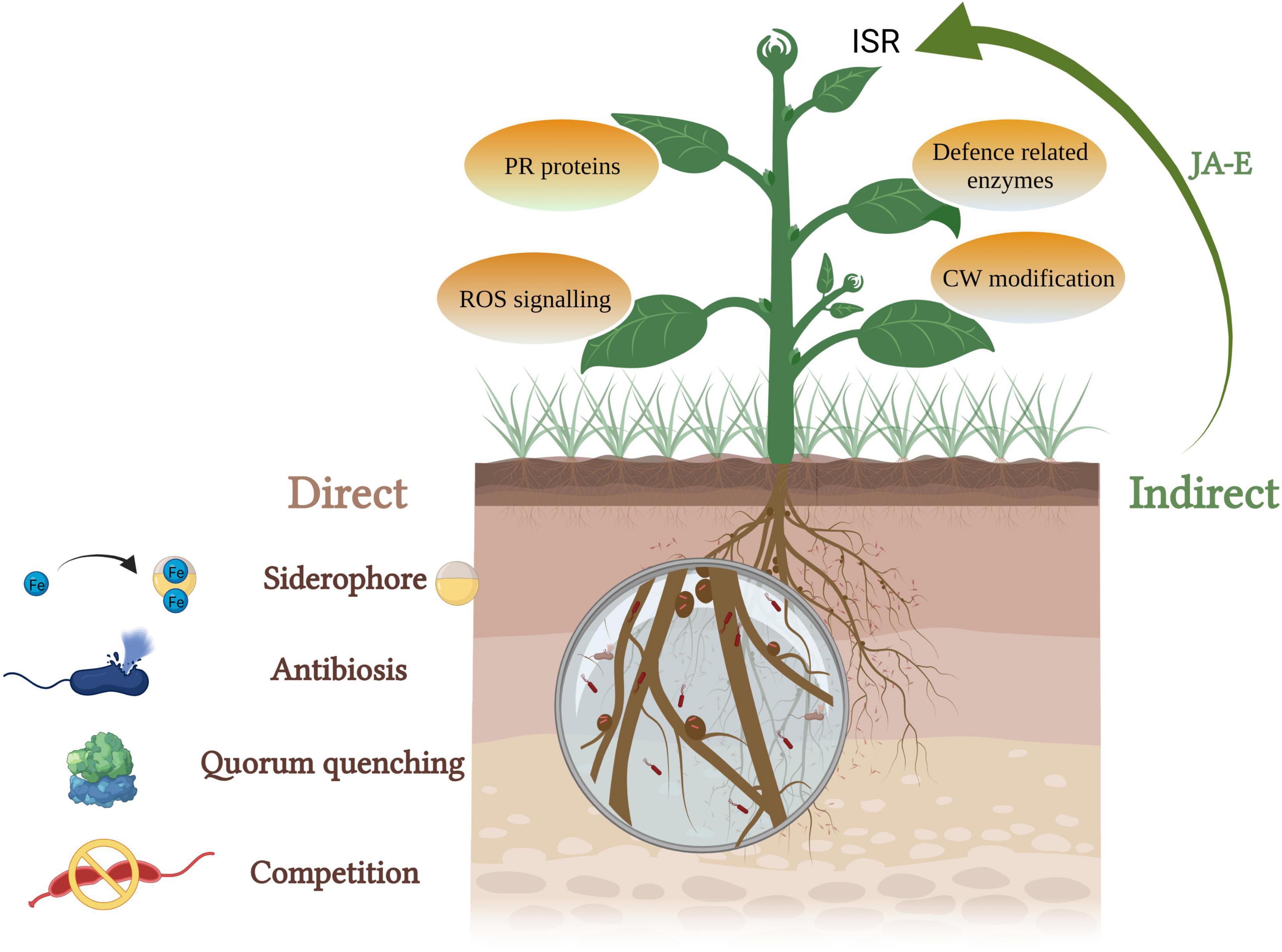
Figure 3. PGPEB-mediated direct and indirect biocontrol of phytopathogens (JA, jasmonic acid; E, ethylene; ISR, induced systemic resistance; PR, pathogenesis related; ROS, reactive oxygen species; CW, cell wall).
Direct biocontrol involves the production of growth-inhibiting compounds such as siderophores, hydrogen cyanide, cell wall–degrading enzymes, and quorum-sensing inhibitors. Siderophore complex provides Fe to plants during the scarcity and assists them in iron acquisition (Dimkpa, 2016). A lot of work has been done to screen siderophore-producing endophytic bacteria–mediated improvement in plant growth along with biocontrol of phytopathogens (Lacava et al., 2008; Pandey et al., 2015; Passari et al., 2016; Walitang et al., 2017; Yan et al., 2018; Maheshwari et al., 2019b). A number of studies have demonstrated that siderophore-producing bacteria support the plants to endure both biotic and abiotic stresses. In a study by Ruiz et al. (2015), Pseudomonas protegens strain survived in the presence of Fusaric acid (mycotoxin)-producing Fusarium strains by producing metal scavenging siderophores (pyoverdine and pyochelin). Recently, Butaite et al. (2017) found that non- and low-pyoverdine siderophore producers coexist in various natural populations. The non-siderophore producers with suitable siderophore receptors can utilize external siderophores. The producers are different in the types of pyoverdine they secrete and offer protection against exploitation by non-producers and acquisition of iron unaccessible to opposing strains lacking the proper receptors.
Hydrogen cyanide (HCN) production leads to the growth inhibition of pathogens due to biogenic cyanogenesis. Cyanide, a metabolic inhibitor, inhibits the cytochrome C oxidase and the other metalloenzymes of the pathogen and thus helps the plant to combat against soil-borne diseases (Voisard et al., 1989; Blumer and Haas, 2000; Dheeman et al., 2019; Maheshwari et al., 2019a; Swarnalakshmi et al., 2019). Dubey et al. (2014) reported Bacillus subtilis strain producing HCN and other metabolites that inhibit the growth of phytopathogen Fusarium oxysporum. HCN-producing endophytes isolated from Glycine max exhibited in vitro antagonism against a wide range of phytopathogens, namely, Sclerotium rolfsii, Rhizoctonia solani, Alternaria alternata, F. oxysporum, Macrophomina phaseolina, and Colletotrichum truncatum (Dalal et al., 2015). The bacterial endophytes obtained from the medicinal plant Clerodendrum colebrookianum Walp possessed in vitro PGP activities including HCN production. These endophytes exhibited in vitro and in vivo biocontrol activity against various phytopathogenic fungi (Passari et al., 2016). Quorum sensing is mediated by small diffusible signaling molecules called autoinducers which mediate the regulation of diverse functions such as virulence and biofilm formation. Endophytic bacteria can interfere in quorum sensing using quorum sensing inhibitors and quorum quenching enzymes to control bacterial infections as a result of suppressing the formation of biofilm (Zhou et al., 2020). Many endophytic bacteria have been reported to produce lactonases and acylases which results in quorum quenching by the inactivation/degradation of major signaling molecules involved in quorum sensing, N-acyl homoserine lactones (Rashid et al., 2012).
Indirectly, endophytic bacteria are known to trigger jasmonic acid and ethylene-mediated induced systemic resistance which induces a defense mechanism and protects the plants from future attacks of plant pathogens (Miliute et al., 2015). It leads to the production of pathogenesis-related proteins, phytoalexins, defense-related enzymes such as polyphenol oxidase (PPO) and phenylalanine ammonia lyase, formation of physical barriers such as cuticles and modification of cell wall (Wiesel et al., 2014). Mao et al. (2019) demonstrated endophytic bacterial strain REB01 to induce disease resistance against rice sheath blight caused by R. solani via enhancing the activity of PPO, POD enzymes, and reducing the MDA content. Kim et al. (2019) isolated and selected pine endophytic bacteria on the basis of the relative expression of defense-related genes, Pseudomonas putida 16 YSM-E48, Curtobacterium pusillum 16YSM-P180, and Stenotrophomonas rhizophila 16YSM-P3G, effective against Bursaphelenchus xylophilus (pinewood nematode) causing pine wilt disease. Asghari et al. (2020) reported induction of systemic resistance in Vitis vinifera, by Pseudomonas sp. Sn48 and Pantoea sp. Sa14 against Agrobacterium tumefaciens through improvement in the levels of PR1, PR2, and PR4 gene expression levels of plantlets.
Some of the endophytic bacteria undergo rhizophagy, biphasic cycle of alternation between the root intracellular phase (nutrients extracted by plants) and a free-living soil phase (acquisition of nutrients by bacteria) (Dudeja et al., 2021). “Endobiome interference” is the term used to describe the phenomenon in which other endophytes interfere with rhizophagy and extract the nutrients from native microbes post-colonization. Although the mechanism behind this remains poorly understood, it has been hypothesized that the oxidative resistance of microbes reduces the capacity of host cells to control the intracellular microbes using ROS produced by NOX enzymes on the root cell plasma membranes. They can be explored to develop bioherbicides to target competitive weeds (White et al., 2019). This way they enhance stress in the host and inhibit their growth leading to an eco-friendly biocontrol option (Verma et al., 2021). Kowalski et al. (2018) explored endobiome interference by the application of a bacterial endophyte, Micrococcus luteus, isolated from the seedling root hairs of Lycopersicon esculentum, to arrest the growth of a weedy plant sp., Phragmites australis, by targeting its growth promotional native microbiome. It also illustrates a vital precaution to be taken before applying any exogenous endophytes, that is, to analyze the interactions between the endophytes being applied and the native microflora (Verma et al., 2021). Table 4 describes numerous studies depicting the biocontrol potential of the bacterial endophytes.
Harnessing “Omics” for Enhancing the Bioactive Metabolites
Bioactive compounds are mostly secondary metabolites produced by the microbes in an active culture cultivation process. Their unique properties have led to lots of research regarding their applications in healthcare as feed supplements, pharmaceuticals, and so on (Singh nee’ Nigam, 2009). Endophytes have been reported to produce many secondary metabolites similar to their host under in vitro systems. This ability can lessen our dependency on endangered plants for the extraction of metabolites (Sharma et al., 2021). Several approaches have been employed to harness novel metabolites or to enhance the production of already known ones to support and flourish their large scale application (Du and van Wezel, 2018). Co-culture engineering by culturing more than one type of endophyte together can make us exploit the intermicrobial communications for the enhanced production of bioactive metabolites. A number of studies have reported the production of new metabolites by this method (Stierle et al., 2017; Arora et al., 2018; Gautam et al., 2019). The associated disadvantages such as compatibility issues, competition for substrates, and data acquisition problems pose remarkable challenges (Padmaperuma et al., 2017; Jawed et al., 2019). A number of reports have demonstrated the precise effects of endophytes on the production of secondary metabolites of the host (Khare et al., 2018). Table 5 sums up recent studies dealing with the enhancement of bioactive compounds of the host owing to the endophytes. Other than enhancing the bioactive compounds of the host, endophytes serve as a great treasure of new metabolites which remains largely unexplored looking at the vast diversity of endophytic flora.
Some approaches have been developed in the recent past to trigger the expression of Biosynthetic gene clusters (BGCs) present in the genome of microbes (endophytes) that can yield some valuable secondary metabolites but remain either silent or poorly expressed. In most cases, BGCs remain silent under laboratory conditions due to complex regulation involved at transcriptional, translational, and post-translational levels. Therefore, the study of changes in gene expression at various levels needs to be done. Recent progress made in bioinformatics, especially genome mining tools have pushed the boundaries of “omics” technologies toward new horizons. It has revolutionized our understanding of the pathways controlling the expression of BGCs.
Genome mining is a powerful approach that can estimate the genetic potential of microbial strain by scanning genomes of interest and identifying the metabolites encoded by BGCs (Ziemert et al., 2016). Whole-genome sequencing and its comparative analysis yield the reconstruction of primary and secondary metabolic pathways that help in suggesting the key metabolic genes to be utilized for metabolic engineering (Palazzotto and Weber, 2018). Metabolic and genetic engineering involves the modulation of biosynthetic enzymes at cellular level/upregulation and downregulation of transcription and translation genes/knock-out and knock-in of desired genes and have been effective in enhancing the production of specific metabolites (Stephens et al., 2015). Mining tools such as antibodies and secondary metabolites analysis shell) (antiSMASH), generalized retro-biosynthetic assembly prediction engine (GRAPE), prediction informatics for secondary metabolomes (PRISM3) have been successful in overcoming the drawbacks associated with manual analyses to some extent. A number of natural products encoded by BGCs remain uncharacterized owing to the complicated regulations occurring at transcriptional, translational, and post-translational levels.
Transcriptome-based studies provide a comparative profile of gene expression and help to assess the key regulators which are in turn used for manifesting the designer strains having the ability to overproduce secondary metabolites (Chaudhary et al., 2013). Proteomics complements other two omics approaches, namely, transcriptomics and genomics, yielding information on differential pathways regulation highlighting key players in the biosynthesis of natural products, which can be used to target for rational engineering (Palazzotto and Weber, 2018). Comparative transcriptomics and proteomics are used to identify the alterations in gene expression associated with the overproduction which are subsequently re-engineered into the organism of interest by considering key genes involved in complex mechanisms. However, its success depends on the reproducibility of the overproducing mechanism in the new target strains (Chaudhary et al., 2013). One of the most important tools of system biology toolbox is metabolomics which catalogs all small metabolites in a biological sample. NMR- and MS-based metabolomic analysis facilitates measurement of low–molecular weight metabolites allowing the metabolic comparison of various biological samples leading to the identification of secondary metabolites from orphan BGCs. A comprehensive picture of metabolic networks helps to engineer the primary metabolism via cofactors and precursors for the biosynthesis of any secondary metabolite (Nguyen et al., 2012). Metagenomics is the most commonly used approach to study the chemistry of uncultivated bacteria. It provides a culture-independent approach to exploring the hidden potential of microorganisms.
Omics analysis in isolation is unable to completely unfurl the complexities involved in microbial metabolism associated with the production of secondary metabolites. Therefore, it is necessary to undertake their integration to get better insights into the same (Chaudhary et al., 2013). The combined use of multi-meta-omics approaches such as metabologenomics involves a combination of genome sequencing and automated gene clusters prediction with MS-based metabolomics. It provides us a complete picture of microbial metabolism shedding light on the silent BGCs and the role of natural products (Palazzotto and Weber, 2018). Precision engineering is another modern approach that integrates information from different sources, transcriptome profiling (DNA microarrays), proteome profiling (2D gel electrophoresis), and metabolic profiling (HPLC), thus enabling a more precise identification of key genetic targets and pathways engineered for strain improvement (Gao et al., 2010). Many microbes engineered by metabolic engineering are being used in industrial-scale processes; however, it is associated with challenges such as titers, yields, and productivities required for commercial viability. Different aspects of microbial physiology can also create obstacles for metabolic engineering (Montaño López et al., 2022).
Limitations and Challenges
Word “endophyte” searched on Google scholar (January 05, 2022) showed 80,100 results indicating extensive research happening in this arena. However, some lacunae need to be filled with regard to the research on endophytes. A sufficient number of studies have not been conducted to study the variations such as plant–microbe interactions on the field induced by a range of environmental and physiological conditions unlike in vitro. Information about the synergistic interaction between different microbial taxa such as bacteria, archaea, and fungi, is sparse with most of the studies focusing on each taxa separately.
Although the importance of biofertilizers and biocontrol potential of endophytes over conventional and environment degrading chemical pesticides are well known, some drawbacks of biopesticides are responsible for our slow speed on this eco-friendly path. High production cost and limited period of activity as compared to the chemical ones along with lower potency make it difficult for the farmers to opt for it. Owing to their target-specific nature, they control a specific portion of pests in the treated area and may leave the other damage-causing pests unaffected (Kawalekar, 2013). Lesser insights are available into the overlaps present in the metabolic pathways of endophytes and host plants, which leads to the production of a particular bioactive compound (Mishra et al., 2021). More research targeted at unfurling the genetic controls involved in stress tolerance conferring potential as well as bioactive compounds accumulation capability of the microbe is to be undertaken to unfold the molecular mechanisms behind the same.
Conclusion
Plant and endophytes exist in close interaction with each other and provide increased productivity as a bonus. The effects and functions of these associations have not been understood fully thereby calling for a more in-depth study. It would be more beneficial if more knowledge of endophytes’ ecology and their molecular interaction is made available for harnessing and application in agriculture. This research will have a progressive impact on the environment in the direction of chemical fertilizer–free cultivation and better contribution to the economy. Also, the optimization of growth conditions as well as nutrient media for the endophytes having enormous potential to be applied particularly in pharmaceutical and agricultural sectors needs to be done at the earliest. “Omics” combined with recent computational data mining tools can help unravel the functions of complex plant microbiome, which can provide us with more competent microbes as far as stress tolerance and enhancing the bioactive metabolites is concerned.
Author Contributions
PS and AD conceptualized the theme of this review. SR wrote and compiled the original draft. PK, PD, and RM drafted the figures and compiled tables. All authors have made intellectual and substantial contribution and approved it for publication.
Conflict of Interest
The authors declare that the research was conducted in the absence of any commercial or financial relationships that could be construed as a potential conflict of interest.
Publisher’s Note
All claims expressed in this article are solely those of the authors and do not necessarily represent those of their affiliated organizations, or those of the publisher, the editors and the reviewers. Any product that may be evaluated in this article, or claim that may be made by its manufacturer, is not guaranteed or endorsed by the publisher.
Acknowledgments
SR, PK, and PD would like to acknowledge the Council of Scientific & Industrial Research, New Delhi, India, for CSIR fellowship. PS acknowledges support from the Department of Science and Technology (DST), Government of India, New Delhi. The authors would like to acknowledge the Department of Science and Technology, Government of India, for providing FIST grant (Grant No. 1196 SR/FST/LS-I/2017/4) to the Department of Microbiology, MDU, Rohtak.
References
Abdelaziz, M. E., Kim, D., Ali, S., Fedoroff, N. V., and Al-Babili, S. (2017). The endophytic fungus Piriformospora indica enhances Arabidopsis thaliana growth and modulates Na+/K+ homeostasis under salt stress conditions. Plant Sci. 263, 107–115. doi: 10.1016/J.PLANTSCI.2017.07.006
Achari, G. A., and Ramesh, R. (2019). Colonization of Eggplant by endophytic bacteria antagonistic to Ralstonia solanacearum, the Bacterial Wilt Pathogen. Proc. Natl. Acad. Sci. India Sect. B Biol. Sci. 89, 585–593. doi: 10.1007/S40011-018-0972-2/FIGURES/3
Afzal, I., Iqrar, I., Shinwari Zabta, K., and Yasmin, A. (2017). Plant growth-promoting potential of endophytic bacteria isolated from roots of wild Dodonaea viscosa L. Plant Growth Regul. 81, 399–408. doi: 10.1007/s10725-016-0216-5/Published
Afzal, I., Shinwari, Z. K., Sikandar, S., and Shahzad, S. (2019). Plant beneficial endophytic bacteria: mechanisms, diversity, host range and genetic determinants. Microbiol. Res. 221, 36–49. doi: 10.1016/J.MICRES.2019.02.001
Afzal, S., Begum, N., Zhao, H., Fang, Z., Lou, L., and Cai, Q. (2017). Influence of endophytic root bacteria on the growth, cadmium tolerance and uptake of switchgrass (Panicum virgatum L.). J. Appl. Microbiol. 123, 498–510. doi: 10.1111/JAM.13505
Agisha, V. N., Eapen, J. S., Bhai, S., and Aundy, K. (2017). Detecting and monitoring endophytic colonization by Pseudomonas putida BP25 in black pepper (Piper nigrum L.) using quantitative real-time PCR exploitation of antimicrobial and growth promotive traits of Methylobacterium for the production and protection. Artic. J. Spices Aromat. Crops 26, 1–7. doi: 10.25081/josac.2017.v26.i1.812
Akbari Kiarood, S. L., Rahnama, K., Golmohammadi, M., and Nasrollanejad, S. (2020). Quorum-quenching endophytic bacteria inhibit disease caused by Pseudomonas syringae pv. syringae in citrus cultivars. J. Basic Microbiol. 60, 746–757. doi: 10.1002/JOBM.202000038
Ali, S., Charles, T. C., and Glick, B. R. (2014). Amelioration of high salinity stress damage by plant growth-promoting bacterial endophytes that contain ACC deaminase. Plant Physiol. Biochem. 80, 160–167. doi: 10.1016/J.PLAPHY.2014.04.003
Alibrandi, P., Cardinale, M., Rahman, M. M., Strati, F., Ciná, P., de Viana, M. L., et al. (2018). The seed endosphere of Anadenanthera colubrina is inhabited by a complex microbiota, including Methylobacterium spp. and Staphylococcus spp. with potential plant-growth promoting activities. Plant Soil 422, 81–99. doi: 10.1007/S11104-017-3182-4/FIGURES/5
Alijani, Z., Amini, J., Ashengroph, M., and Bahramnejad, B. (2019). Antifungal activity of volatile compounds produced by Staphylococcus sciuri strain MarR44 and its potential for the biocontrol of Colletotrichum nymphaeae, causal agent strawberry anthracnose. Int. J. Food Microbiol. 307:108276. doi: 10.1016/J.IJFOODMICRO.2019.108276
Alori, E. T., and Babalola, O. O. (2018). Microbial inoculants for improving crop quality and human health in Africa. Front. Microbiol. 9:2213. doi: 10.3389/FMICB.2018.02213/BIBTEX
Arora, D., Chashoo, G., Singamaneni, V., Sharma, N., Gupta, P., and Jaglan, S. (2018). Bacillus amyloliquefaciens induces production of a novel blennolide K in coculture of Setophoma terrestris. J. Appl. Microbiol. 124, 730–739. doi: 10.1111/JAM.13683
Arora, M., Saxena, P., Choudhary, D. K., Abdin, M. Z., and Varma, A. (2016). Dual symbiosis between Piriformospora indica and Azotobacter chroococcum enhances the artemisinin content in Artemisia annua L. World J. Microbiol. Biotechnol. 32, 19. doi: 10.1007/s11274-015-1972-5
Asghari, S., Harighi, B., Ashengroph, M., Clement, C., Aziz, A., Esmaeel, Q., et al. (2020). Induction of systemic resistance to Agrobacterium tumefaciens by endophytic bacteria in grapevine. Plant Pathol. 69, 827–837. doi: 10.1111/PPA.13175
Aydi Ben Abdallah, R., Mokni-Tlili, S., Nefzi, A., Jabnoun-Khiareddine, H., and Daami-Remadi, M. (2016). Biocontrol of Fusarium wilt and growth promotion of tomato plants using endophytic bacteria isolated from Nicotiana glauca organs. Biol. Control 97, 80–88. doi: 10.1016/J.BIOCONTROL.2016.03.005
Babu, A. G., Shea, P. J., Sudhakar, D., Jung, I. B., and Oh, B. T. (2015). Potential use of Pseudomonas koreensis AGB-1 in association with Miscanthus sinensis to remediate heavy metal(loid)-contaminated mining site soil. J. Environ. Manage. 151, 160–166. doi: 10.1016/J.JENVMAN.2014.12.045
Bagde, U. S., Prasad, R., and Varma, A. (2014). Impact of culture filtrate of Piriformospora indica on biomass and biosynthesis of active ingredient aristolochic acid in Aristolochia elegans Mart. Int. J. Biol. 6, 29–37. doi: 10.5539/ijb.v6n1p29
Bai, F., Morimoto, Y. V., Yoshimura, S. D. J., Hara, N., Kami-Ike, N., Namba, K., et al. (2014). Assembly dynamics and the roles of FliI ATPase of the bacterial flagellar export apparatus. Sci. Rep. 4:6528. doi: 10.1038/srep06528
Balsanelli, E., Tadra-Sfeir, M. Z., Faoro, H., Pankievicz, V. C., de Baura, V. A., Pedrosa, F. O., et al. (2016). Molecular adaptations of Herbaspirillum seropedicae during colonization of the maize rhizosphere. Environ. Microbiol. 18, 2343–2356. doi: 10.1111/1462-2920.12887/SUPPINFO
Balsanelli, E., Tuleski, T. R., de Baura, V. A., Yates, M. G., Chubatsu, L. S., de Oliveira Pedrosa, F., et al. (2013). Maize root lectins mediate the interaction with Herbaspirillum seropedicae via N-Acetyl glucosamine residues of Lipopolysaccharides. PLoS One 8:e77001. doi: 10.1371/JOURNAL.PONE.0077001
Ban, Y., Xu, Z., Yang, Y., Zhang, H., Chen, H., and Tang, M. (2017). Effect of dark septate endophytic fungus Gaeumannomyces cylindrosporus on plant growth, photosynthesis and Pb tolerance of maize (Zea mays L.). Pedosphere 27, 283–292. doi: 10.1016/S1002-0160(17)60316-3
Banik, A., Mukhopadhaya, S. K., Sahana, A., Das, D., and Dangar, T. K. (2016). Fluorescence resonance energy transfer (FRET)-based technique for tracking of endophytic bacteria in rice roots. Biol. Fertil. Soils 52, 277–282. doi: 10.1007/S00374-015-1064-6/FIGURES/4
Barnawal, D., Bharti, N., Pandey, S. S., Pandey, A., Chanotiya, C. S., and Kalra, A. (2017). Plant growth-promoting rhizobacteria enhance wheat salt and drought stress tolerance by altering endogenous phytohormone levels and TaCTR1/TaDREB2 expression. Physiol. Plant. 161, 502–514. doi: 10.1111/PPL.12614
Barnawal, D., Bharti, N., Tripathi, A., Pandey, S. S., Chandan, S., and Kalra, A. (2016). ACC-deaminase-producing endophyte brachybacterium paraconglomeratum strain SMR20 ameliorates chlorophytum salinity stress via altering phytohormone generation. J. Plant Growth Regul. 35, 553–564. doi: 10.1007/s00344-015-9560-3
Bashir, S., Iqbal, A., Hasnain, S., and White, J. F. (2021). Screening of sunflower associated bacteria as biocontrol agents for plant growth promotion. Arch. Microbiol. 203, 4901–4912. doi: 10.1007/S00203-021-02463-8/FIGURES/5
Beauregard, P. B., Chai, Y., Vlamakis, H., Losick, R., and Kolter, R. (2013). Bacillus subtilis biofilm induction by plant polysaccharides. Proc. Natl. Acad. Sci. U.S.A. 110, E1621–E1630. doi: 10.1073/PNAS.1218984110/-/DCSUPPLEMENTAL
Bharti, N., Pandey, S. S., Barnawal, D., Patel, V. K., and Kalra, A. (2016). Plant growth promoting rhizobacteria Dietzia natronolimnaea modulates the expression of stress responsive genes providing protection of wheat from salinity stress. Sci. Rep. 6, 34768. doi: 10.1038/srep34768
Bhise, K. K., Bhagwat, P. K., and Dandge, P. B. (2017). Synergistic effect of Chryseobacterium gleum sp. SUK with ACC deaminase activity in alleviation of salt stress and plant growth promotion in Triticum aestivum L. 3 Biotech 7, 1–13. doi: 10.1007/S13205-017-0739-0/TABLES/3
Bhutani, N., Maheshwari, R., Negi, M., and Suneja, P. (2018a). Optimization of IAA production by endophytic Bacillus spp. from Vigna radiata for their potential use as plant growth promoters. Isr. J. Plant Sci. 65, 83–96. doi: 10.1163/22238980-00001025
Bhutani, N., Maheshwari, R., and Suneja, P. (2018b). Isolation and characterization of plant growth promoting endophytic bacteria isolated from Vigna radiata-Indian Journals. Indian J. Agric. Res. 52, 593–603.
Bhutani, N., Maheshwari, R., Kumar, P., and Suneja, P. (2021). Bioprospecting of endophytic bacteria from nodules and roots of Vigna radiata, Vigna unguiculata and Cajanus cajan for their potential use as bioinoculants. Plant Gene 28, 100326. doi: 10.1016/J.PLGENE.2021.100326
Bilal, S., Khan, A. L., Shahzad, R., Kim, Y. H., Imran, M., Khan, M. J., et al. (2018). Mechanisms of Cr (VI) resistance by endophytic Sphingomonas sp. LK11 and its Cr(VI) phytotoxic mitigating effects in soybean (Glycine max L.). Ecotoxicol. Environ. Saf. 164, 648–658. doi: 10.1016/J.ECOENV.2018.08.043
Blaha, D., Prigent-Combaret, C., Mirza, M. S., and Moënne-Loccoz, Y. (2006). Phylogeny of the 1-aminocyclopropane-1-carboxylic acid deaminase-encoding gene acdS in phytobeneficial and pathogenic Proteobacteria and relation with strain biogeography. FEMS Microbiol. Ecol. 56, 455–470. doi: 10.1111/J.1574-6941.2006.00082.X
Blumer, C., and Haas, D. (2000). Mechanism, regulation, and ecological role of bacterial cyanide biosynthesis. Arch. Microbiol. 173, 170–177. doi: 10.1007/S002039900127
Bogas, A. C., Ferreira, A. J., Araújo, W. L., Astolfi-Filho, S., Kitajima, E. W., Lacava, P. T., et al. (2015). Endophytic bacterial diversity in the phyllosphere of Amazon Paullinia cupana associated with asymptomatic and symptomatic anthracnose. Springerplus 4, 1–13. doi: 10.1186/S40064-015-1037-0/FIGURES/8
Bonilla, A., Sarria, A. L. F., Algar, E., Muñoz Ledesma, F. J., Ramos Solano, B., Fernandes, J. B., et al. (2014). Microbe associated molecular patterns from rhizosphere bacteria trigger germination and Papaver somniferum metabolism under greenhouse conditions. Plant Physiol. Biochem. 74, 133–140. doi: 10.1016/J.PLAPHY.2013.11.012
Brígido, C., Menéndez, E., Paço, A., Glick, B. R., Belo, A., Félix, M. R., et al. (2019). Mediterranean native leguminous plants: a reservoir of endophytic bacteria with potential to enhance chickpea growth under stress conditions. Microorganisms 7:392. doi: 10.3390/MICROORGANISMS7100392
Bulgarelli, D., Rott, M., Schlaeppi, K., Ver Loren van Themaat, E., Ahmadinejad, N., Assenza, F., et al. (2012). Revealing structure and assembly cues for Arabidopsis root-inhabiting bacterial microbiota. Nature 488, 91–95. doi: 10.1038/nature11336
Buschart, A., Sachs, S., Chen, X., Herglotz, J., Krause, A., and Reinhold-Hurek, B. (2012). Flagella mediate endophytic competence rather than act as MAMPS in rice–Azoarcus sp. Strain BH72 interactions. Mol. Plant Microbe Interact. 25, 191–199. doi: 10.1094/MPMI-05-11-0138
Butaite, E., Baumgartner, M., Wyder, S., and Kümmerli, R. (2017). Siderophore cheating and cheating resistance shape competition for iron in soil and freshwater Pseudomonas communities. Nat. Commun. 8, 414. doi: 10.1038/s41467-017-00509-4
Canarini, A., Kaiser, C., Merchant, A., Richter, A., and Wanek, W. (2019). Root exudation of primary metabolites: mechanisms and their roles in plant responses to environmental stimuli. Front. Plant Sci. 10:157. doi: 10.3389/FPLS.2019.00157/BIBTEX
Carvalhais, L. C., Muzzi, F., Tan, C. H., Hsien-Choo, J., and Schenk, P. M. (2013). Plant growth in Arabidopsis is assisted by compost soil-derived microbial communities. Front. Plant Sci. 4:235. doi: 10.3389/FPLS.2013.00235/ABSTRACT
Castanheira, N. L., Dourado, A. C., Pais, I., Semedo, J., Scotti-Campos, P., Borges, N., et al. (2017). Colonization and beneficial effects on annual ryegrass by mixed inoculation with plant growth promoting bacteria. Microbiol. Res. 198, 47–55. doi: 10.1016/J.MICRES.2017.01.009
Castanheira, N., Dourado, A. C., Kruz, S., Alves, P. I. L., Delgado-Rodríguez, A. I., Pais, I., et al. (2016). Plant growth-promoting Burkholderia species isolated from annual ryegrass in Portuguese soils. J. Appl. Microbiol. 120, 724–739. doi: 10.1111/JAM.13025
Chaudhary, A. K., Dhakal, D., and Sohng, J. K. (2013). An insight into the “-Omics” based engineering of streptomycetes for secondary metabolite overproduction. Biomed. Res. Int. 2013:968518. doi: 10.1155/2013/968518
Chen, Z. J., Sheng, X. F., He, L. Y., Huang, Z., and Zhang, W. H. (2013). Effects of root inoculation with bacteria on the growth, Cd uptake and bacterial communities associated with rape grown in Cd-contaminated soil. J. Hazard. Mater. 244–245, 709–717. doi: 10.1016/J.JHAZMAT.2012.10.063
Cohen, A. C., Bottini, R., Pontin, M., Berli, F. J., Moreno, D., Boccanlandro, H., et al. (2015). Azospirillum brasilense ameliorates the response of Arabidopsis thaliana to drought mainly via enhancement of ABA levels. Physiol. Plant. 153, 79–90. doi: 10.1111/PPL.12221
Dal Cortivo, C., Barion, G., Visioli, G., Mattarozzi, M., Mosca, G., and Vamerali, T. (2017). Increased root growth and nitrogen accumulation in common wheat following PGPR inoculation: assessment of plant-microbe interactions by ESEM. Agric. Ecosyst. Environ. 247, 396–408. doi: 10.1016/J.AGEE.2017.07.006
Dalal, J. M., Kulkarni, N. S., and Bodhankar, M. G. (2015). Utilization of indigenous endophytic microbes for induction of systemic resistance (ISR) in soybean (Glycine max (L) Merril) against challenge inoculation with F. oxysporum. Res. Biotechnol. 6, 10–25.
de Souza, R., Ambrosini, A., and Passaglia, L. M. P. (2015). Plant growth-promoting bacteria as inoculants in agricultural soils. Genet. Mol. Biol. 38, 401–419. doi: 10.1590/S1415-475738420150053
Depluverez, S., Devos, S., and Devreese, B. (2016). The role of bacterial secretion systems in the virulence of gram-negative airway pathogens associated with cystic fibrosis. Front. Microbiol. 7:1336. doi: 10.3389/FMICB.2016.01336/BIBTEX
Dheeman, S., Maheshwari, K., Dubey, R. C., Kumar, S., Baliyan, N., Dhiman, S., et al. (2019). “Harnessing beneficial Bacillus in Productivity improvement of food security crops of Himalayan agro-climatic zones,” in Field Crops: Sustainable Management by PGPR, eds D. K. Maheshwari and S. Dheeman (Cham: Springer), 105–143. doi: 10.1007/978-3-030-30926-8_5
Dhole, A., Shelat, H., Vyas, R., Jhala, Y., and Bhange, M. (2016). Endophytic occupation of legume root nodules by nifH-positive non-rhizobial bacteria, and their efficacy in the groundnut (Arachis hypogaea). Ann. Microbiol. 66, 1397–1407. doi: 10.1007/S13213-016-1227-1/FIGURES/3
Dhungana, S. A., and Itoh, K. (2019). Effects of co-inoculation of indole-3-acetic acid-producing and -degrading bacterial endophytes on plant growth. Horticulturae 5:17. doi: 10.3390/HORTICULTURAE5010017
Dimkpa, C. (2016). Endocytobiosis and cell research microbial siderophores: production, detection and application in agriculture and environment. Endocytobiosis Cell Res. 27, 7–16.
Dong, C. J., Wang, L. L., Li, Q., and Shang, Q. M. (2019). Bacterial communities in the rhizosphere, phyllosphere and endosphere of tomato plants. PLoS One 14:e0223847. doi: 10.1371/JOURNAL.PONE.0223847
dos Santos, M. L., Berlitz, D. L., Wiest, S. L. F., Schünemann, R., Knaak, N., and Fiuza, L. M. (2018). Benefits associated with the interaction of endophytic bacteria and plants. Braz. Arch. Biol. Technol. 61, 1–11. doi: 10.1590/1678-4324-2018160431
Du, C., and van Wezel, G. P. (2018). Mining for microbial gems: integrating proteomics in the postgenomic natural product discovery pipeline. Proteomics 18:1700332. doi: 10.1002/PMIC.201700332
Dubey, A., Saiyam, D., Kumar, A., Hashem, A., Abduallah, E. F., and Khan, M. L. (2021). Bacterial root endophytes: characterization of their competence and plant growth promotion in soybean (Glycine max (L.) Merr.) under drought stress. Int. J. Environ. Res. Public Health 18:931. doi: 10.3390/IJERPH18030931
Dubey, R. C., Khare, S., Kumar, P., and Maheshwari, D. K. (2014). Combined effect of chemical fertilisers and rhizosphere-competent Bacillus subtilis BSK17 on yield of Cicer arietinum. Arch. Phytopathol. Plant Prot. 47, 2305–2318. doi: 10.1080/03235408.2013.876744
Duca, D., Lorv, J., Patten, C. L., Rose, D., and Glick, B. R. (2014). Indole-3-acetic acid in plant-microbe interactions. Antonie Van Leeuwenhoek 106, 85–125. doi: 10.1007/S10482-013-0095-Y/FIGURES/8
Dudeja, S. S., Giri, R., Saini, R., Suneja-Madan, P., and Kothe, E. (2012). Interaction of endophytic microbes with legumes. J. Basic Microbiol. 52, 248–260. doi: 10.1002/JOBM.201100063
Dudeja, S. S., Suneja-Madan, P., Paul, M., Maheswari, R., and Kothe, E. (2021). Bacterial endophytes: molecular interactions with their hosts. J. Basic Microbiol. 61, 475–505. doi: 10.1002/JOBM.202000657
Egamberdieva, D., Wirth, S. J., Shurigin, V. V., Hashem, A., and Abd Allah, E. F. (2017). Endophytic bacteria improve plant growth, symbiotic performance of chickpea (Cicer arietinum L.) and induce suppression of root rot caused by Fusarium solani under salt stress. Front. Microbiol. 8:1887. doi: 10.3389/FMICB.2017.01887/BIBTEX
Ek-Ramos, M. J., Gomez-Flores, R., Orozco-Flores, A. A., Rodríguez-Padilla, C., González-Ochoa, G., and Tamez-Guerra, P. (2019). Bioactive products from plant-endophytic gram-positive bacteria. Front. Microbiol. 10:463. doi: 10.3389/FMICB.2019.00463/BIBTEX
Elbeltagy, A., Nishioka, K., Suzuki, H., Sato, T., Sato, Y. I., Morisaki, H., et al. (2000). Isolation and characterization of endophytic bacteria from wild and traditionally cultivated rice varieties. Soil Sci. Plant Nutr. 46, 617–629. doi: 10.1080/00380768.2000.10409127
Etesami, H., Alikhani, H. A., and Hosseini, H. M. (2015). Indole-3-acetic acid (IAA) production trait, a useful screening to select endophytic and rhizosphere competent bacteria for rice growth promoting agents. MethodsX 2, 72–78. doi: 10.1016/J.MEX.2015.02.008
Faddetta, T., Abbate, L., Alibrandi, P., Arancio, W., Siino, D., Strati, F., et al. (2021). The endophytic microbiota of Citrus limon is transmitted from seed to shoot highlighting differences of bacterial and fungal community structures. Sci. Rep. 11:7078. doi: 10.1038/s41598-021-86399-5
Fan, B., Chen, X. H., Budiharjo, A., Bleiss, W., Vater, J., and Borriss, R. (2011). Efficient colonization of plant roots by the plant growth promoting bacterium Bacillus amyloliquefaciens FZB42, engineered to express green fluorescent protein. J. Biotechnol. 151, 303–311. doi: 10.1016/J.JBIOTEC.2010.12.022
Fan, X., Yang, R., Qiu, S., Cai, X., Zou, H., and Hu, F. (2016). The Endo-β-1,4-glucanase of Bacillus amyloliquefaciens is required for optimum endophytic colonization of plants. J. Microbiol. Biotechnol. 26, 946–952. doi: 10.4014/JMB.1512.12055
Fard, E. M., Ghabooli, M., Mehri, N., and Bakhshi, B. (2017). Regulation of miR159 and miR396 mediated by Piriformospora indica confer drought tolerance in rice. J. Plant Mol. Breed. 5, 10–18. doi: 10.22058/JPMB.2017.60864.1129
Ferchichi, N., Toukabri, W., Boularess, M., Smaoui, A., Mhamdi, R., and Trabelsi, D. (2019). Isolation, identification and plant growth promotion ability of endophytic bacteria associated with lupine root nodule grown in Tunisian soil. Arch. Microbiol. 201, 1333–1349. doi: 10.1007/S00203-019-01702-3/FIGURES/5
Fernández-Llamosas, H., Díaz, E., and Carmona, M. (2021). Motility, adhesion and c-di-GMP influence the endophytic colonization of rice by Azoarcus sp. CIB. Microorganisms 9:554. doi: 10.3390/MICROORGANISMS9030554
Frank, A. C., Guzmán, J. P. S., and Shay, J. E. (2017). Transmission of bacterial endophytes. Microorganisms 5:70. doi: 10.3390/MICROORGANISMS5040070
Gao, H., Zhou, X., Gou, Z., Zhuo, Y., Fu, C., Liu, M., et al. (2010). Rational design for over-production of desirable microbial metabolites by precision engineering. Antonie van Leeuwenhoek 98, 151–163. doi: 10.1007/S10482-010-9442-4/FIGURES/5
Gao, Y., Liu, Q., Zang, P., Li, X., Ji, Q., He, Z., et al. (2015). An endophytic bacterium isolated from Panax ginseng C.A. Meyer enhances growth, reduces morbidity, and stimulates ginsenoside biosynthesis. Phytochem. Lett. 11, 132–138. doi: 10.1016/J.PHYTOL.2014.12.007
Gautam, K., Tripathi, J. K., Pareek, A., and Sharma, D. K. (2019). Growth and secretome analysis of possible synergistic interaction between green algae and cyanobacteria. J. Biosci. Bioeng. 127, 213–221. doi: 10.1016/J.JBIOSC.2018.07.005
Ghaffari, M. R., Mirzaei, M., Ghabooli, M., Khatabi, B., Wu, Y., Zabet-Moghaddam, M., et al. (2019). Root endophytic fungus Piriformospora indica improves drought stress adaptation in barley by metabolic and proteomic reprogramming. Environ. Exp. Bot. 157, 197–210. doi: 10.1016/J.ENVEXPBOT.2018.10.002
Glassner, H., Zchori-Fein, E., Yaron, S., Sessitsch, A., Sauer, U., and Compant, S. (2018). Bacterial niches inside seeds of Cucumis melo L. Plant Soil 422, 101–113. doi: 10.1007/S11104-017-3175-3/FIGURES/7
Glick, B. R. (2012). Plant growth-promoting bacteria: mechanisms and applications. Scientifica (Cairo) 2012, 1–15. doi: 10.6064/2012/963401
Glick, B. R. (2014). Bacteria with ACC deaminase can promote plant growth and help to feed the world. Microbiol. Res. 169, 30–39. doi: 10.1016/J.MICRES.2013.09.009
Glick, B. R., Cheng, Z., Czarny, J., and Duan, J. (2007). “Promotion of plant growth by ACC deaminase-producing soil bacteria,” in New Perspect. Approaches Plant Growth-Promoting Rhizobacteria Research, eds P. A. H. M. Bakker, J. M. Raaijmakers, G. Bloemberg, M. Höfte, P. Lemanceau, and B. M. Cooke (Dordrecht: Springer), 329–339. doi: 10.1007/978-1-4020-6776-1_8
Gond, S. K., Bergen, M. S., Torres, M. S., White, J. F., and Kharwar, R. N. (2015). Effect of bacterial endophyte on expression of defense genes in Indian popcorn against Fusarium moniliforme. Symbiosis 66, 133–140. doi: 10.1007/S13199-015-0348-9/FIGURES/5
Gorai, P. S., Ghosh, R., Mandal, S., Ghosh, S., Chatterjee, S., Gond, S. K., et al. (2021). Bacillus siamensis CNE6- a multifaceted plant growth promoting endophyte of Cicer arietinum L. having broad spectrum antifungal activities and host colonizing potential. Microbiol. Res. 252:126859. doi: 10.1016/J.MICRES.2021.126859
Gupta, G., Panwar, J., Akhtar, M. S., and Jha, P. N. (2012). Endophytic nitrogen-fixing bacteria as biofertilizer. Sustain. Agric. Rev. 11, 183–221. doi: 10.1007/978-94-007-5449-2_8
Hamilton, C. E., Gundel, P. E., Helander, M., and Saikkonen, K. (2012). Endophytic mediation of reactive oxygen species and antioxidant activity in plants: a review. Fungal Divers. 54, 1–10. doi: 10.1007/S13225-012-0158-9
Hardoim, P. R., van Overbeek, L. S., and van Elsas, J. D. (2008). Properties of bacterial endophytes and their proposed role in plant growth. Trends Microbiol. 16, 463–471. doi: 10.1016/J.TIM.2008.07.008
Hardoim, P. R., van Overbeek, L. S., Berg, G., Pirttilä, A. M., Compant, S., Campisano, A., et al. (2015). The hidden world within plants: ecological and evolutionary considerations for defining functioning of microbial endophytes. Microbiol. Mol. Biol. Rev. 79, 293–320. doi: 10.1128/MMBR.00050-14
Hazarika, D. J., Goswami, G., Gautom, T., Parveen, A., Das, P., Barooah, M., et al. (2019). Lipopeptide mediated biocontrol activity of endophytic Bacillus subtilis against fungal phytopathogens. BMC Microbiol. 19:71. doi: 10.1186/S12866-019-1440-8/FIGURES/4
Hernández-Soberano, C., Ruíz-Herrera, L. F., and Valencia-Cantero, E. (2020). Endophytic bacteria Arthrobacter agilis UMCV2 and Bacillus methylotrophicus M4-96 stimulate achene germination, in vitro growth, and greenhouse yield of strawberry (Fragaria × ananassa). Sci. Hortic. (Amsterdam) 261:109005. doi: 10.1016/J.SCIENTA.2019.109005
Hu, H., Gao, Y., Li, X., Chen, S., Yan, S., and Tian, X. (2020). Identification and nematicidal characterization of proteases secreted by endophytic bacteria Bacillus cereus BCM2. Phytopathology 110, 336–344. doi: 10.1094/PHYTO-05-19-0164-R/ASSET/IMAGES/LARGE/PHYTO-05-19-0164-R_F7.JPEG
Iqbal, Z., Iqbal, M. S., Hashem, A., Abd-Allah, E. F., and Ansari, M. I. (2021). Plant defense responses to biotic stress and its interplay with fluctuating dark/light conditions. Front. Plant Sci. 12:297. doi: 10.3389/FPLS.2021.631810/BIBTEX
Irizarry, I., and White, J. F. (2018). Bacillus amyloliquefaciens alters gene expression, ROS production and lignin synthesis in cotton seedling roots. J. Appl. Microbiol. 124, 1589–1603. doi: 10.1111/JAM.13744
Issa, A., Esmaeel, Q., Sanchez, L., Courteaux, B., Guise, J. F., Gibon, Y., et al. (2018). Impacts of Paraburkholderia phytofirmans strain PsJN on tomato (Lycopersicon esculentum L.) under high temperature. Front. Plant Sci. 871:1397. doi: 10.3389/FPLS.2018.01397/BIBTEX
Jan, R., Khan, M. A., Asaf, S., Lubna, Lee, I. J., and Kim, K. M. (2019). Metal resistant endophytic bacteria reduces cadmium, nickel toxicity, and enhances expression of metal stress related genes with improved growth of Oryza sativa, via regulating its antioxidant machinery and endogenous hormones. Plants 8:363. doi: 10.3390/PLANTS8100363
Jawed, K., Yazdani, S. S., and Koffas, M. A. (2019). Advances in the development and application of microbial consortia for metabolic engineering. Metab. Eng. Commun. 9:e00095. doi: 10.1016/J.MEC.2019.E00095
Jha, P., Panwar, J., and Jha, P. N. (2018). Mechanistic insights on plant root colonization by bacterial endophytes: a symbiotic relationship for sustainable agriculture. Environ. Sustain. 1, 25–38. doi: 10.1007/S42398-018-0011-5
Johnston, P. R., Sutherland, P. W., and Joshee, S. (2006). Visualising endophytic fungi within leaves by detection of (1→3)-ß-d-glucans in fungal cell walls. Mycologist 20, 159–162. doi: 10.1016/J.MYCOL.2006.10.003
Kandel, S. L., Joubert, P. M., and Doty, S. L. (2017a). Bacterial endophyte colonization and distribution within plants. Microorganisms 5:77. doi: 10.3390/MICROORGANISMS5040077
Kandel, S. L., Firrincieli, A., Joubert, P. M., Okubara, P. A., Leston, N. D., McGeorge, K. M., et al. (2017b). An in vitro study of bio-control and plant growth promotion potential of salicaceae endophytes. Front. Microbiol. 8:386. doi: 10.3389/FMICB.2017.00386/BIBTEX
Kandel, S. L., Herschberger, N., Kim, S. H., and Doty, S. L. (2015). Diazotrophic endophytes of poplar and willow for growth promotion of rice plants in nitrogen-limited conditions. Crop Sci. 55, 1765–1772. doi: 10.2135/CROPSCI2014.08.0570
Kaul, S., Sharma, T., and Dhar, M. K. (2016). “Omics” tools for better understanding the plant–endophyte interactions. Front. Plant Sci. 7:955. doi: 10.3389/FPLS.2016.00955/BIBTEX
Kawalekar, J. (2013). Role of biofertilizers and biopesticides for sustainable agriculture. J. Bio Innov. 2, 73–78.
Kawasaki, A., Donn, S., Ryan, P. R., Mathesius, U., Devilla, R., Jones, A., et al. (2016). Microbiome and exudates of the root and rhizosphere of Brachypodium distachyon, a model for wheat. PLoS One 11:e0164533. doi: 10.1371/JOURNAL.PONE.0164533
Khan, A., Sirajuddin, Zhao, X. Q., Javed, M. T., Khan, K. S., Bano, A., et al. (2016). Bacillus pumilus enhances tolerance in rice (Oryza sativa L.) to combined stresses of NaCl and high boron due to limited uptake of Na+. Environ. Exp. Bot. 124, 120–129. doi: 10.1016/J.ENVEXPBOT.2015.12.011
Khan, M. A., Asaf, S., Khan, A. L., Jan, R., Kang, S. M., Kim, K. M., et al. (2020). Thermotolerance effect of plant growth-promoting Bacillus cereus SA1 on soybean during heat stress. BMC Microbiol. 20:175. doi: 10.1186/S12866-020-01822-7/FIGURES/6
Khan, M. S., Gao, J., Chen, X., Zhang, M., Yang, F., Du, Y., et al. (2020). Isolation and characterization of plant growth-promoting endophytic bacteria Paenibacillus polymyxa SK1 from Lilium lancifolium. Biomed. Res. Int. 2020:8650957. doi: 10.1155/2020/8650957
Khan, Z., and Doty, S. L. (2009). Characterization of bacterial endophytes of sweet potato plants. Plant Soil 322, 197–207. doi: 10.1007/S11104-009-9908-1/TABLES/3
Khan, Z., Rho, H., Firrincieli, A., Hung, S. H., Luna, V., Masciarelli, O., et al. (2016). Growth enhancement and drought tolerance of hybrid poplar upon inoculation with endophyte consortia. Curr. Plant Biol. 6, 38–47. doi: 10.1016/J.CPB.2016.08.001
Khare, E., Mishra, J., and Arora, N. K. (2018). Multifaceted interactions between endophytes and plant: developments and Prospects. Front. Microbiol. 9:2732. doi: 10.3389/FMICB.2018.02732/BIBTEX
Kilam, D., Saifi, M., Abdin, M. Z., Agnihotri, A., and Varma, A. (2017). Endophytic root fungus Piriformospora indica affects transcription of steviol biosynthesis genes and enhances production of steviol glycosides in Stevia rebaudiana. Physiol. Mol. Plant Pathol. 97, 40–48. doi: 10.1016/J.PMPP.2016.12.003
Kim, N., Jeon, H. W., Mannaa, M., Jeong, S. I., Kim, J., Kim, J., et al. (2019). Induction of resistance against pine wilt disease caused by Bursaphelenchus xylophilus using selected pine endophytic bacteria. Plant Pathol. 68, 434–444. doi: 10.1111/PPA.12960
Kowalski, K., Kingsley, K., Butterworth, S., Elmore, M., Brindisi, L., and White, J. (2018). Endobiome Interference” As a Strategy to Curtail Aggressiveness of Invasive Plant Species. Available online at: https://eco.confex.com/eco/2018/meetingapp.cgi/Paper/72836 (Accessed January 4, 2022).
Kuldau, G. A., and Yates, I. E. (2000). “Evidence for Fusarium endophytes in cultivated and wild plants,” in Microbial endophytes, eds C. W. Bacon and J. F. White Jr. (New York, NY: Marcel Dekker), 85–117.
Kumar, A., Droby, S., Singh, V. K., Singh, S. K., and White, J. F. (2020). Entry, colonization, and distribution of endophytic microorganisms in plants. Microb. Endophytes 1–33. doi: 10.1016/B978-0-12-819654-0.00001-6
Kumar, A., Vandana, Singh, M., Singh, P. P., Singh, S. K., Singh, P. K., et al. (2016). Isolation of plant growth promoting rhizobacteria and their impact on growth and curcumin content in Curcuma longa L. Biocatal. Agric. Biotechnol. 8, 1–7. doi: 10.1016/J.BCAB.2016.07.002
Kumar, V., Jain, L., Jain, S. K., Chaturvedi, S., and Kaushal, P. (2020). Bacterial endophytes of rice (Oryza sativa L.) and their potential for plant growth promotion and antagonistic activities. S. Af. J. Bot. 134, 50–63. doi: 10.1016/J.SAJB.2020.02.017
Kusari, S., Lamshöft, M., Kusari, P., Gottfried, S., Zühlke, S., Louven, K., et al. (2014). Endophytes are hidden producers of maytansine in Putterlickia roots. J. Nat. Prod. 77, 2577–2584. doi: 10.1021/NP500219A/SUPPL_FILE/NP500219A_SI_001.PDF
Labanca, E. R. G., Andrade, S. A. L., Kuramae, E. E., and Silveira, A. P. D. (2020). The modulation of sugarcane growth and nutritional profile under aluminum stress is dependent on beneficial endophytic bacteria and plantlet origin. Appl. Soil Ecol. 156, 103715. doi: 10.1016/J.APSOIL.2020.103715
Lacava, P. T., Silva-Stenico, M. E., Araújo, W. L., Simionato, A. V. C., Carrilho, E., Siu, M. T., et al. (2008). Detection of siderophores in endophytic bacteria Methylobacterium spp. associated with Xylella fastidiosa subsp. pauca. Pesqui. Agropecuária Bras. 43, 521–528. doi: 10.1590/S0100-204X2008000400011
Lamers, J., Van Der Meer, T., and Testerink, C. (2020). How plants sense and respond to stressful environments. Plant Physiol. 182, 1624–1635. doi: 10.1104/PP.19.01464
Lareen, A., Burton, F., and Schäfer, P. (2016). Plant root-microbe communication in shaping root microbiomes. Plant Mol. Biol. 90, 575–587. doi: 10.1007/S11103-015-0417-8
Lastochkina, O., Garshina, D., Allagulova, C., Fedorova, K., Koryakov, I., and Vladimirova, A. (2020). Application of endophytic Bacillus subtilis and salicylic acid to improve wheat growth and tolerance under combined drought and Fusarium root rot stresses. Agronomy 10:1343. doi: 10.3390/agronomy10091343
Leonhardt, T., Sáckı, J., Šimek, P., Šantrùèek, J., and Kotrba, P. (2014). Metallothionein-like peptides involved in sequestration of Zn in the Zn-accumulating ectomycorrhizal fungus Russula atropurpurea. Metallomics 6, 1693–1701. doi: 10.1039/C4MT00141A
Li, Y., Cheng, C., and An, D. (2017). Characterisation of endophytic bacteria from a desert plant Lepidium perfoliatum L. Plant Prot. Sci. 53, 32–43. doi: 10.17221/14/2016-PPS
Lima, A. S., Prieto, K. R., Santos, C. S., Paula Valerio, H., Garcia-Ochoa, E. Y., Huerta-Robles, A., et al. (2018). In-vivo electrochemical monitoring of H2O2 production induced by root-inoculated endophytic bacteria in Agave tequilana leaves. Biosens. Bioelectron. 99, 108–114. doi: 10.1016/J.BIOS.2017.07.039
Liscombe, D. K., and Facchini, P. J. (2008). Evolutionary and cellular webs in benzylisoquinoline alkaloid biosynthesis. Curr. Opin. Biotechnol. 19, 173–180. doi: 10.1016/J.COPBIO.2008.02.012
Liu, H., Zhang, L., Meng, A., Zhang, J., Xie, M., Qin, Y., et al. (2017a). Isolation and molecular identification of endophytic diazotrophs from seeds and stems of three cereal crops. PLoS One 12:e0187383. doi: 10.1371/JOURNAL.PONE.0187383
Liu, H., Carvalhais, L. C., Crawford, M., Singh, E., Dennis, P. G., Pieterse, C. M. J., et al. (2017b). Inner plant values: diversity, colonization and benefits from endophytic bacteria. Front. Microbiol. 8:2552. doi: 10.3389/FMICB.2017.02552/BIBTEX
Liu, W., Sun, Y., Shen, R., Dang, X., Liu, X., Sui, F., et al. (2018). A chemotaxis-like pathway of Azorhizobium caulinodans controls flagella-driven motility, which regulates biofilm formation, exopolysaccharide biosynthesis, and competitive nodulation. Mol. Plant Microbe Interact. 31, 737–749. doi: 10.1094/MPMI-12-17-0290-R
Liu, X., Liu, W., Sun, Y., Xia, C., Elmerich, C., and Xie, Z. (2018). A cheZ-like gene in Azorhizobium caulinodans is a key gene in the control of chemotaxis and colonization of the host plant. Appl. Environ. Microbiol. 84, 1827–1844. doi: 10.1128/AEM.01827-17
Lodewyckx, C., Vangronsveld, J., Porteous, F., Moore, E. R. B., Taghavi, S., Mezgeay, M., et al. (2002). Endophytic bacteria and their potential applications. Crit. Rev. Plant Sci. 21, 583–606. doi: 10.1080/0735-260291044377
Lòpez-Fernàndez, S., Sonego, P., Moretto, M., Pancher, M., Engelen, K., Pertot, I., et al. (2015). Whole-genome comparative analysis of virulence genes unveils similarities and differences between endophytes and other symbiotic bacteria. Front. Microbiol. 6:419. doi: 10.3389/FMICB.2015.00419/ABSTRACT
Lugtenberg, B., and Kamilova, F. (2009). Plant-growth-promoting rhizobacteria. Annu. Rev. Microbiol. 63, 541–556. doi: 10.1146/ANNUREV.MICRO.62.081307.162918
Maela, P. M. (2019). Current understanding of bacterial endophytes, their diversity, colonization and their roles in promoting plant growth. Appl. Microbiol. 5, 1–12. doi: 10.4172/2471-9315.1000157
Mahaffee, W. F. (1994). Endophytic colonization of Phaseolus vulgaris by Pseudomonas fluorescens strain 89B-27 and Enterobacter asburiae strain JM22. Improv. Plant Product. Rhizobacteria. Available online at: https://ci.nii.ac.jp/naid/10015091111/ (accessed March 29, 2022).
Maheshwari, R., Bhutani, N., Bhardwaj, A., and Suneja, P. (2019a). Functional diversity of cultivable endophytes from Cicer arietinum and Pisum sativum: bioprospecting their plant growth potential. Biocatal. Agric. Biotechnol. 20:101229. doi: 10.1016/J.BCAB.2019.101229
Maheshwari, R., Bhutani, N., and Suneja, P. (2019b). Screening and characterization of siderophore producing endophytic bacteria from Cicer arietinum and Pisum sativum plants. J. Appl. Biol. Biotechnol. 7, 7–14. doi: 10.7324/JABB.2019.70502
Maheshwari, R., Bhutani, N., and Suneja, P. (2020). Isolation and characterization of ACC deaminase producing endophytic Bacillus mojavensis PRN2 from Pisum sativum. Iran. J. Biotechnol. 18:e2308. doi: 10.30498/IJB.2020.137279.2308
Maheshwari, R., Bhutani, N., Kumar, P., and Suneja, P. (2021). Plant growth promoting potential of multifarious endophytic Pseudomonas lini strain isolated from Cicer arietinum L. Isr. J. Plant Sci. 1, 1–11. doi: 10.1163/22238980-BJA10047
Mao, J., Gong, M., and Guan, Q. (2019). Induced disease resistance of endophytic bacteria REB01 to bacterial blight of rice. AIP Conf. Proc. 2079:020017. doi: 10.1063/1.5092395
Marques, A. P. G. C., Pires, C., Moreira, H., Rangel, A. O. S. S., and Castro, P. M. L. (2010). Assessment of the plant growth promotion abilities of six bacterial isolates using Zea mays as indicator plant. Soil Biol. Biochem. 42, 1229–1235. doi: 10.1016/J.SOILBIO.2010.04.014
Martínez-Gil, M., Yousef-Coronado, F., and Espinosa-Urgel, M. (2010). LapF, the second largest Pseudomonas putida protein, contributes to plant root colonization and determines biofilm architecture. Mol. Microbiol. 77, 549–561. doi: 10.1111/J.1365-2958.2010.07249.X
Mastan, A., Vivek Babu, C. S., Hiremath, C., Srinivas, K. V. N. S., Kumar, A. N., and Kumar, J. K. (2019). Treatments with native Coleus forskohlii endophytes improve fitness and secondary metabolite production of some medicinal and aromatic plants. Int. Microbiol. 23, 345–354. doi: 10.1007/S10123-019-00108-X
Meneses, C. H. S. G., Rouws, L. F. M., Simões-Araújo, J. L., Vidal, M. S., and Baldani, J. I. (2011). Exopolysaccharide production is required for biofilm formation and plant colonization by the nitrogen-fixing endophyte Gluconacetobacter diazotrophicus. Mol. Plant Microbe Interact. 24, 1448–1458. doi: 10.1094/MPMI-05-11-0127
Meneses, C., Gonçalves, T., Alquéres, S., Rouws, L., Serrato, R., Vidal, M., et al. (2017). Gluconacetobacter diazotrophicus exopolysaccharide protects bacterial cells against oxidative stress in vitro and during rice plant colonization. Plant Soil 416, 133–147. doi: 10.1007/S11104-017-3201-5/FIGURES/8
Mengistu, A. A. (2020). Endophytes: colonization, behaviour, and their role in defense mechanism. Int. J. Microbiol. 2020:6927219. doi: 10.1155/2020/6927219
Miliute, I., Buzaite, O., Baniulis, D., Baniulis, D., and Stanys, V. (2015). Bacterial endophytes in agricultural crops and their role in stress tolerance: a review. Zemdirb. Agric. 102, 465–478. doi: 10.13080/z-a.2015.102.060
Minamino, T., Morimoto, Y. V., Hara, N., Aldridge, P. D., and Namba, K. (2016). The bacterial flagellar type III export gate complex is a dual fuel engine that can use Both H+ and Na+ for flagellar protein export. PLoS Pathog. 12:e1005495. doi: 10.1371/JOURNAL.PPAT.1005495
Mishra, S., Sahu, P. K., Agarwal, V., and Singh, N. (2021). Exploiting endophytic microbes as micro-factories for plant secondary metabolite production. Appl. Microbiol. Biotechnol. 105, 6579–6596. doi: 10.1007/S00253-021-11527-0
Mohite, B. (2013). Isolation and characterization of indole acetic acid (IAA) producing bacteria from rhizospheric soil and its effect on plant growth. J. Soil Sci. Plant Nutr. 13, 638–649. doi: 10.4067/S0718-95162013005000051
Montaño López, J., Duran, L., and Avalos, J. L. (2022). Physiological limitations and opportunities in microbial metabolic engineering. Nat. Rev. Microbiol. 20, 35–48. doi: 10.1038/s41579-021-00600-0
Monteiro, R. A., Balsanelli, E., Tuleski, T., Faoro, H., Cruz, L. M., Wassem, R., et al. (2012). Genomic comparison of the endophyte Herbaspirillum seropedicae SmR1 and the phytopathogen Herbaspirillum rubrisubalbicans M1 by suppressive subtractive hybridization and partial genome sequencing. FEMS Microbiol. Ecol. 80, 441–451. doi: 10.1111/J.1574-6941.2012.01309.X
Motooka, D., Fujimoto, K., Tanaka, R., Yaguchi, T., Gotoh, K., Maeda, Y., et al. (2017). Fungal ITS1 deep-sequencing strategies to reconstruct the composition of a 26-species community and evaluation of the gut mycobiota of healthy Japanese individuals. Front. Microbiol. 8:238. doi: 10.3389/FMICB.2017.00238/BIBTEX
Mukherjee, A., Singh, B. K., and Verma, J. P. (2020). Harnessing chickpea (Cicer arietinum L.) seed endophytes for enhancing plant growth attributes and bio-controlling against Fusarium sp. Microbiol. Res. 237:126469. doi: 10.1016/J.MICRES.2020.126469
Naveed, M., Mitter, B., Yousaf, S., Pastar, M., Afzal, M., and Sessitsch, A. (2013). The endophyte Enterobacter sp. FD17: a maize growth enhancer selected based on rigorous testing of plant beneficial traits and colonization characteristics. Biol. Fertil. Soils 50, 249–262. doi: 10.1007/S00374-013-0854-Y/TABLES/5
Neumann, S., Grosse, K., and Sourjik, V. (2012). Chemotactic signaling via carbohydrate phosphotransferase systems in Escherichia coli. Proc. Natl. Acad. Sci. U.S.A. 109, 12159–12164. doi: 10.1073/PNAS.1205307109/-/DCSUPPLEMENTAL
Newman, M. A., Sundelin, T., Nielsen, J. T., and Erbs, G. (2013). MAMP (microbe-associated molecular pattern) triggered immunity in plants. Front. Plant Sci. 4:139. doi: 10.3389/FPLS.2013.00139/BIBTEX
Nguyen, Q. T., Merlo, M. E., Medema, M. H., Jankevics, A., Breitling, R., and Takano, E. (2012). Metabolomics methods for the synthetic biology of secondary metabolism. FEBS Lett. 586, 2177–2183. doi: 10.1016/J.FEBSLET.2012.02.008
Nicolson, G. L. (2014). The Fluid—Mosaic Model of Membrane Structure: Still relevant to understanding the structure, function and dynamics of biological membranes after more than 40 years. Biochim. Biophys. Acta Biomembr. 1838, 1451–1466. doi: 10.1016/J.BBAMEM.2013.10.019
Oteino, N., Lally, R. D., Kiwanuka, S., Lloyd, A., Ryan, D., Germaine, K. J., et al. (2015). Plant growth promotion induced by phosphate solubilizing endophytic Pseudomonas isolates. Front. Microbiol. 6:745. doi: 10.3389/FMICB.2015.00745/BIBTEX
Padmaperuma, G., Kapoore, R. V., Gilmour, D. J., and Vaidyanathan, S. (2017). Microbial consortia: a critical look at microalgae co-cultures for enhanced biomanufacturing. Crit. Rev. Biotechnol. 38, 690–703. doi: 10.1080/07388551.2017.1390728
Palazzotto, E., and Weber, T. (2018). Omics and multi-omics approaches to study the biosynthesis of secondary metabolites in microorganisms. Curr. Opin. Microbiol. 45, 109–116. doi: 10.1016/J.MIB.2018.03.004
Pandey, P. K., Samanta, R., and Yadav, R. N. S. (2015). Plant beneficial endophytic bacteria from the ethnomedicinal Mussaenda roxburghii (Akshap) of Eastern Himalayan Province, India. Adv. Biol. 2015, 1–8. doi: 10.1155/2015/580510
Pankievicz, V. C. S., Camilios-Neto, D., Bonato, P., Balsanelli, E., Tadra-Sfeir, M. Z., Faoro, H., et al. (2016). RNA-seq transcriptional profiling of Herbaspirillum seropedicae colonizing wheat (Triticum aestivum) roots. Plant Mol. Biol. 90, 589–603. doi: 10.1007/s11103-016-0430-6
Passari, A. K., Mishra, V. K., Leo, V. V., Gupta, V. K., and Singh, B. P. (2016). Phytohormone production endowed with antagonistic potential and plant growth promoting abilities of culturable endophytic bacteria isolated from Clerodendrum colebrookianum Walp. Microbiol. Res. 193, 57–73. doi: 10.1016/J.MICRES.2016.09.006
Patel, J. K., and Archana, G. (2017). Diverse culturable diazotrophic endophytic bacteria from Poaceae plants show cross-colonization and plant growth promotion in wheat. Plant Soil 417, 99–116. doi: 10.1007/S11104-017-3244-7/FIGURES/5
Patel, J. K., Gohel, K., Patel, H., and Solanki, T. (2021). Wheat growth dependent succession of culturable endophytic bacteria and their plant growth promoting traits. Curr. Microbiol. 78, 4103–4114. doi: 10.1007/S00284-021-02668-6/FIGURES/2
Pimentel Esposito-Polesi, N., Fiori De Abreu-Tarazi, M., Vieira De Almeida, C., Tsai, S. M., and De Almeida, M. (2017). Investigation of endophytic bacterial community in supposedly axenic cultures of pineapple and orchids with evidence on abundant intracellular bacteria. Curr. Microbiol. 74, 103–113. doi: 10.1007/s00284-016-1163-0
Plett, J. M., and Martin, F. M. (2018). Know your enemy, embrace your friend: using omics to understand how plants respond differently to pathogenic and mutualistic microorganisms. Plant J. 93, 729–746. doi: 10.1111/TPJ.13802
Ponpandian, L. N., Rim, S. O., Shanmugam, G., Jeon, J., Park, Y. H., Lee, S. K., et al. (2019). Phylogenetic characterization of bacterial endophytes from four Pinus species and their nematicidal activity against the pine wood nematode. Sci. Rep. 9:12457. doi: 10.1038/s41598-019-48745-6
Pootakham, W., Mhuantong, W., Yoocha, T., Putchim, L., Sonthirod, C., Naktang, C., et al. (2017). High resolution profiling of coral-associated bacterial communities using full-length 16S rRNA sequence data from PacBio SMRT sequencing system. Sci. Rep. 7:2774. doi: 10.1038/s41598-017-03139-4
Qiang, X., Ding, J., Lin, W., Li, Q., Xu, C., Zheng, Q., et al. (2019). Alleviation of the detrimental effect of water deficit on wheat (Triticum aestivum L.) growth by an indole acetic acid-producing endophytic fungus. Plant Soil 439, 373–391. doi: 10.1007/S11104-019-04028-7/FIGURES/10
Qin, D., Wang, L., Han, M., Wang, J., Song, H., Yan, X., et al. (2018). Effects of an endophytic fungus Umbelopsis dimorphaon the secondary metabolites of host-plant Kadsura angustifolia. Front. Microbiol. 9:2845. doi: 10.3389/FMICB.2018.02845/BIBTEX
Ramanujam, B., Renuka, S., and Shylesha, A. N. (2016). Electron microscopic studies for confirmation of endophytic colonization of Beauveria bassiana in maize. J. Pure Appl. Microbiol. 10, 3017–3021. doi: 10.22207/JPAM.10.4.72
Rana, K. L., Kour, D., Kaur, T., Devi, R., Yadav, A. N., Yadav, N., et al. (2020). Endophytic microbes: biodiversity, plant growth-promoting mechanisms and potential applications for agricultural sustainability Kusam Lata Rana and Divjot Kour contributed equally to the present review. Antonie Van Leeuwenhoek 113, 1075–1107. doi: 10.1007/s10482-020-01429-y
Rani, S., Kumar, P., and Suneja, P. (2021). Biotechnological interventions for inducing abiotic stress tolerance in crops. Plant Gene 27:100315. doi: 10.1016/J.PLGENE.2021.100315
Rashid, S., Charles, T. C., and Glick, B. R. (2012). Isolation and characterization of new plant growth-promoting bacterial endophytes. Appl. Soil Ecol. 61, 217–224. doi: 10.1016/J.APSOIL.2011.09.011
Reimer, A., Maffenbeier, V., Dubey, M., Sentchilo, V., Tavares, D., Gil, M. H., et al. (2017). Complete alanine scanning of the Escherichia coli RbsB ribose binding protein reveals residues important for chemoreceptor signaling and periplasmic abundance. Sci. Rep. 7:8245. doi: 10.1038/s41598-017-08035-5
Reinhold-Hurek, B., and Hurek, T. (2011). Living inside plants: bacterial endophytes. Curr. Opin. Plant Biol. 14, 435–443. doi: 10.1016/J.PBI.2011.04.004
Ríos-Ruiz, W. F., Alfredo Valdez-Nuñez, R., Bedmar, E. J., Castellano-Hinojosa, A., Ríos-Ruiz, W. F., Valdez-Nuñez, R. A., et al. (2019). “Utilization of endophytic bacteria isolated from legume root nodules for plant growth promotion,” in Field Crops: Sustainable Management by PGPR, eds D. K. Maheshwari and S. Dheeman (Cham: Springer), 145–176. doi: 10.1007/978-3-030-30926-8_6
Robertson-Albertyn, S., Terrazas, R. A., Balbirnie, K., Blank, M., Janiak, A., Szarejko, I., et al. (2017). Root hair mutations displace the barley rhizosphere microbiota. Front. Plant Sci. 8:1094. doi: 10.3389/FPLS.2017.01094/BIBTEX
Ruiz, J. A., Bernar, E. M., and Jung, K. (2015). Production of siderophores increases resistance to fusaric acid in Pseudomonas protegens Pf-5. PLoS One 10:e0117040. doi: 10.1371/JOURNAL.PONE.0117040
Saleh, D., Sharma, M., Seguin, P., and Jabaji, S. (2020). Organic acids and root exudates of Brachypodium distachyon: effects on chemotaxis and biofilm formation of endophytic bacteria. Can. J. Microbiol. 66, 562–575. doi: 10.1139/cjm-2020-0041
Samanta, D., Widom, J., Borbat, P. P., Freed, J. H., and Crane, B. R. (2016). Bacterial energy sensor aer modulates the activity of the chemotaxis kinase CheA based on the redox state of the flavin cofactor *. J. Biol. Chem. 291, 25809–25814. doi: 10.1074/JBC.C116.757492
Sangwan, P., Raj, K., Wati, L., and Kumar, A. (2021). Isolation and evaluation of bacterial endophytes against Sclerospora graminicola (Sacc.) schroet, the causal of pearl millet downy mildew. Egypt. J. Biol. Pest Control 31, 1–11. doi: 10.1186/S41938-021-00468-5/TABLES/4
Santoyo, G., Moreno-Hagelsieb, G., del Carmen Orozco-Mosqueda, M., and Glick, B. R. (2016). Plant growth-promoting bacterial endophytes. Microbiol. Res. 183, 92–99. doi: 10.1016/J.MICRES.2015.11.008
Sapre, S., Gontia-Mishra, I., and Tiwari, S. (2018). Klebsiella sp. confers enhanced tolerance to salinity and plant growth promotion in oat seedlings (Avena sativa). Microbiol. Res. 206, 25–32. doi: 10.1016/J.MICRES.2017.09.009
Sarkar, A., Pramanik, K., Mitra, S., Soren, T., and Maiti, T. K. (2018). Enhancement of growth and salt tolerance of rice seedlings by ACC deaminase-producing Burkholderia sp. MTCC 12259. J. Plant Physiol. 231, 434–442. doi: 10.1016/J.JPLPH.2018.10.010
Scharf, B. E., Hynes, M. F., and Alexandre, G. M. (2016). Chemotaxis signaling systems in model beneficial plant-bacteria associations. Plant Mol. Biol. 90, 549–559. doi: 10.1007/s11103-016-0432-4
Schmidt, R., Köberl, M., Mostafa, A., Ramadan, E. M., Monschein, M., Jensen, K. B., et al. (2014). Effects of bacterial inoculants on the indigenous microbiome and secondary metabolites of chamomile plants. Front. Microbiol. 5:64. doi: 10.3389/FMICB.2014.00064/ABSTRACT
Senthilkumar, M., Pushpakanth, P., Arul Jose, P., Krishnamoorthy, R., and Anandham, R. (2021). Diversity and functional characterization of endophytic Methylobacterium isolated from banana cultivars of South India and its impact on early growth of tissue culture banana plantlets. J. Appl. Microbiol. 131, 2448–2465. doi: 10.1111/JAM.15112
Sessitsch, A., Hardoim, P., Döring, J., Weilharter, A., Krause, A., Woyke, T., et al. (2011). Functional characteristics of an endophyte community colonizing rice roots as revealed by metagenomic analysis. Mol. Plant Microbe Interact. 25, 28–36. doi: 10.1094/MPMI-08-11-0204
Shah, A. U., Rajab, H., Jalal, A., Ajmal, M., Bangash, S. A. K., Ahmad, D., et al. (2020). Inoculation of Brassica napus L. genotypes with endophytic bacteria promote growth and alleviate cadmium toxicity. APS J. Anim. Plant Sci. 30, 1187–1193.
Shanmugam, G., Lee, S. K., and Jeon, J. (2018). Identification of potential nematicidal compounds against the pine wood nematode, Bursaphelenchus xylophilus through an in silico approach. Molecules. 23:1828. doi: 10.3390/MOLECULES23071828
Sharifazizi, M., Harighi, B., and Sadeghi, A. (2017). Evaluation of biological control of Erwinia amylovora, causal agent of fire blight disease of pear by antagonistic bacteria. Biol. Control 104, 28–34. doi: 10.1016/J.BIOCONTROL.2016.10.007
Sharma, H., Rai, A. K., Dahiya, D., Chettri, R., Nigam, P. S., Sharma, H., et al. (2021). Exploring endophytes for in vitro synthesis of bioactive compounds similar to metabolites produced in vivo by host plants. AIMS Microbiol. 7, 175–199. doi: 10.3934/MICROBIOL.2021012
Sharma, P., Kharkwal, A. C., Abdin, M. Z., and Varma, A. (2014). Piriformospora indica improves micropropagation, growth and phytochemical content of Aloe vera L. plants. Symbiosis 64, 11–23. doi: 10.1007/S13199-014-0298-7/FIGURES/8
Sharma, S. B., Sayyed, R. Z., Trivedi, M. H., and Gobi, T. A. (2013). Phosphate solubilizing microbes: sustainable approach for managing phosphorus deficiency in agricultural soils. Springerplus 2, 1–14. doi: 10.1186/2193-1801-2-587/FIGURES/3
Shastry, R. P., Dolan, S. K., Abdelhamid, Y., Vittal, R. R., and Welch, M. (2018). Purification and characterisation of a quorum quenching AHL-lactonase from the endophytic bacterium Enterobacter sp. CS66. FEMS Microbiol. Lett. 365:54. doi: 10.1093/FEMSLE/FNY054
Sheoran, N., Kumar, A., Munjal, V., Nadakkakath, A. V., and Eapen, S. J. (2016). Pseudomonas putida BP25 alters root phenotype and triggers salicylic acid signaling as a feedback loop in regulating endophytic colonization in Arabidopsis thaliana. Physiol. Mol. Plant Pathol. 93, 99–111. doi: 10.1016/J.PMPP.2016.01.008
Shi, P., Zhu, K., Zhang, Y., and Chai, T. (2016). Growth and cadmium accumulation of Solanum nigrum L. Seedling were enhanced by heavy metal-tolerant strains of Pseudomonas aeruginosa. Water. Air Soil Pollut. 227, 1–11. doi: 10.1007/S11270-016-3167-6/FIGURES/4
Shidore, T., Dinse, T., Öhrlein, J., Becker, A., and Reinhold-Hurek, B. (2012). Transcriptomic analysis of responses to exudates reveal genes required for rhizosphere competence of the endophyte Azoarcus sp. strain BH72. Environ. Microbiol. 14, 2775–2787. doi: 10.1111/J.1462-2920.2012.02777.X
Shinogi, T., Suzuki, T., Kurihara, T., Narusaka, Y., Park, P., Shinogi, T., et al. (2003). Microscopic detection of reactive oxygen species generation in the compatible and incompatible interactions of Alternaria alternata Japanese pear pathotype and host plants. J. Gen. Plant Pathol. 69, 7–16. doi: 10.1007/S10327-002-0013-Z
Singer, E., Bushnell, B., Coleman-Derr, D., Bowman, B., Bowers, R. M., Levy, A., et al. (2016). High-resolution phylogenetic microbial community profiling. ISME J. 10, 2020–2032. doi: 10.1038/ismej.2015.249
Singh, B., and Sharma, R. A. (2016). Yield enhancement of phytochemicals by Azotobacter chroococcum biotization in hairy roots of Arnebia hispidissima. Ind. Crops Prod. 81, 169–175. doi: 10.1016/J.INDCROP.2015.11.068
Singh, R. P., Shelke, G. M., Kumar, A., and Jha, P. N. (2015). Biochemistry and genetics of ACC deaminase: a weapon to “stress ethylene” produced in plants. Front. Microbiol. 6:937. doi: 10.3389/FMICB.2015.00937/BIBTEX
Singh nee’ Nigam, P. (2009). “Production of bioactive secondary metabolites,” in Biotechnology for Agro-Industrial Residues Utilisation, eds P. Singh nee’ Nigam and A. Pandey (Dordrecht: Springer), 129–145. doi: 10.1007/978-1-4020-9942-7_7
Soldan, R., Mapelli, F., Crotti, E., Schnell, S., Daffonchio, D., Marasco, R., et al. (2019). Bacterial endophytes of mangrove propagules elicit early establishment of the natural host and promote growth of cereal crops under salt stress. Microbiol. Res. 223–225, 33–43. doi: 10.1016/J.MICRES.2019.03.008
Srinivasan, R., Kantwa, S. R., Sharma, K. K., Chaudhary, M., Prasad, M., and Radhakrishna, A. (2018). Development and evaluation of phosphate solubilising microbial inoculants for fodder production in problem soils-Indian Journals. Range Manag. Agrofor. 39, 77–89.
Stearns, J. C., and Glick, B. R. (2003). Transgenic plants with altered ethylene biosynthesis or perception. Biotechnol. Adv. 21, 193–210. doi: 10.1016/S0734-9750(03)00024-7
Stephens, E., Wolf, J., Oey, M., Zhang, E., Hankamer, B., Ross, I. L., et al. (2015). “Genetic engineering for microalgae strain improvement in relation to biocrude production systems,” in Biomass and Biofuels from Microalga, eds N. Moheimani, M. McHenry, K. de Boer, and P. Bahri (Cham: Springer), 191–249. doi: 10.1007/978-3-319-16640-7_11
Stierle, A. A., Stierle, D. B., Decato, D., Priestley, N. D., Alverson, J. B., Hoody, J., et al. (2017). The berkeleylactones, antibiotic macrolides from fungal coculture. J. Nat. Prod. 80, 1150–1160. doi: 10.1021/ACS.JNATPROD.7B00133
Su, F., Jacquard, C., Villaume, S., Michel, J., Rabenoelina, F., Clément, C., et al. (2015). Burkholderia phytofirmans PsJN reduces impact of freezing temperatures on photosynthesis in Arabidopsis thaliana. Front. Plant Sci. 6:810. doi: 10.3389/FPLS.2015.00810/BIBTEX
Su, L., Shen, Z., Ruan, Y., Tao, C., Chao, Y., Li, R., et al. (2017). Isolation of antagonistic endophytes from banana roots against Meloidogyne javanica and their effects on soil nematode community. Front. Microbiol. 8:2070. doi: 10.3389/FMICB.2017.02070/BIBTEX
Suaìrez-Moreno, Z. R., Devescovi, G., Myers, M., Hallack, L., Mendonça-Previato, L., Caballero-Mellado, J., et al. (2010). Commonalities and differences in regulation of N -Acyl homoserine lactone quorum sensing in the beneficial plant-associated Burkholderia species cluster. Appl. Environ. Microbiol. 76, 4302–4317. doi: 10.1128/AEM.03086-09
Subramanian, P., Mageswari, A., Kim, K., Lee, Y., and Sa, T. (2015). Psychrotolerant endophytic Pseudomonas sp. strains OB155 and OS261 induced chilling resistance in tomato plants (Solanum lycopersicum Mill.) by activation of their antioxidant capacity. Mol. Plant Microbe Interact. 28, 1073–1081. doi: 10.1094/MPMI-01-15-0021-R
Suneja, P., Singh Dudeja, S., and Dahiya, P. (2016). Deciphering the phylogenetic relationships among rhizobia nodulating chickpea: a review. J. Appl. Biol. Biotechnol. 4, 61–070. doi: 10.7324/JABB.2016.40310
Swarnalakshmi, K., Rajkhowa, S., Dhar, D. W., Senthilkumar, M., Swarnalakshmi, K., Rajkhowa, S., et al. (2019). “Influence of endophytic bacteria on growth promotion and protection against diseases in associated plants,” in Microbial Interventions in Agriculture and Environment: Volume 3: Soil and Crop Health Management, eds D. P. Singh and R. Prabha (Berlin: Springer). doi: 10.1007/978-981-32-9084-6_12
Tamás, M. J., Sharma, S. K., Ibstedt, S., Jacobson, T., and Christen, P. (2014). Heavy metals and metalloids as a cause for protein misfolding and aggregation. Biomolecules 4, 252–267. doi: 10.3390/BIOM4010252
Tang, K., Li, B., and Guo, S. (2014). An active endophytic fungus promoting growth and increasing salvianolic acid content of Salvia miltiorrhiza. Mycosystema 33, 594–600.
Tashi-Oshnoei, F., Harighi, B., and Abdollahzadeh, J. (2017). Isolation and identification of endophytic bacteria with plant growth promoting and biocontrol potential from oak trees. For. Pathol. 47:e12360. doi: 10.1111/EFP.12360
Taulé, C., Castillo, A., Villar, S., Olivares, F., and Battistoni, F. (2016). Endophytic colonization of sugarcane (Saccharum officinarum) by the novel diazotrophs Shinella sp. UYSO24 and Enterobacter sp. UYSO10. Plant Soil 403, 403–418. doi: 10.1007/S11104-016-2813-5
Thokchom, E., Thakuria, D., Kalita, M. C., Sharma, C. K., and Talukdar, N. C. (2017). Root colonization by host-specific rhizobacteria alters indigenous root endophyte and rhizosphere soil bacterial communities and promotes the growth of mandarin orange. Eur. J. Soil Biol. 79, 48–56. doi: 10.1016/J.EJSOBI.2017.02.003
Toumatia, O., Compant, S., Yekkour, A., Goudjal, Y., Sabaou, N., Mathieu, F., et al. (2016). Biocontrol and plant growth promoting properties of Streptomyces mutabilis strain IA1 isolated from a Saharan soil on wheat seedlings and visualization of its niches of colonization. S. Afr. J. Bot. 105, 234–239. doi: 10.1016/J.SAJB.2016.03.020
Turner, T. R., James, E. K., and Poole, P. S. (2013). The plant microbiome. Genome Biol. 14, 1–10. doi: 10.1186/GB-2013-14-6-209/FIGURES/1
Ullah, I., Al-Johny, B. O., Al-Ghamdi, K. M. S., Al-Zahrani, H. A. A., Anwar, Y., Firoz, A., et al. (2019). Endophytic bacteria isolated from Solanum nigrum L., alleviate cadmium (Cd) stress response by their antioxidant potentials, including SOD synthesis by sodA gene. Ecotoxicol. Environ. Saf. 174, 197–207. doi: 10.1016/J.ECOENV.2019.02.074
Van Zelm, E., Zhang, Y., and Testerink, C. (2020). Salt tolerance mechanisms of plants. Annu Rev Plant Biol. 71, 403–433. doi: 10.1146/ANNUREV-ARPLANT-050718-100005
Vandenkoornhuyse, P., Quaiser, A., Duhamel, M., Le Van, A., and Dufresne, A. (2015). The importance of the microbiome of the plant holobiont. New Phytol. 206, 1196–1206. doi: 10.1111/NPH.13312
Vasileva, E. N., B. E., Akhtemova, G. A., A. Γ, Zhukov, V. A., B, et al. (2019). Endophytic microorganisms in fundamental research and agriculture. Ecol. Genet. 17, 19–32. doi: 10.17816/ecogen17119-32
Verma, H., Kumar, D., Kumar, V., Kumari, M., Singh, S. K., Sharma, V. K., et al. (2021). The potential application of endophytes in management of stress from drought and salinity in crop plants. Microorganisms 9:1729. doi: 10.3390/MICROORGANISMS9081729
Verma, S. K., Kingsley, K., Bergen, M., English, C., Elmore, M., Kharwar, R. N., et al. (2018). Bacterial endophytes from rice cut grass (Leersia oryzoides L.) increase growth, promote root gravitropic response, stimulate root hair formation, and protect rice seedlings from disease. Plant Soil 422, 223–238. doi: 10.1007/S11104-017-3339-1/FIGURES/6
Vimal, S. R., Patel, V. K., and Singh, J. S. (2019). Plant growth promoting Curtobacterium albidum strain SRV4: an agriculturally important microbe to alleviate salinity stress in paddy plants. Ecol. Indic. 105, 553–562. doi: 10.1016/J.ECOLIND.2018.05.014
Viscardi, S., Ventorino, V., Duran, P., Maggio, A., De Pascale, S., Mora, M. L., et al. (2016). Assessment of plant growth promoting activities and abiotic stress tolerance of Azotobacter chroococcum strains for a potential use in sustainable agriculture. J. Soil Sci. Plant Nutr. 16, 848–863. doi: 10.4067/S0718-95162016005000060
Voisard, C., Keel, C., Haas, D., and Defago, G. (1989). Cyanide production by Pseudomonas fluorescens helps suppress black root rot of tobacco under gnotobiotic conditions. EMBO J. 8, 351–358. doi: 10.1002/J.1460-2075.1989.TB03384.X
Vorholt, J. A. (2012). Microbial life in the phyllosphere. Nat. Rev. Microbiol. 10, 828–840. doi: 10.1038/nrmicro2910
Vurukonda, S. S. K. P., Giovanardi, D., and Stefani, E. (2021). Identification, evaluation and selection of a bacterial endophyte able to colonize tomato plants, enhance their growth and control Xanthomonas vesicatoria, the causal agent of the spot disease. Can. J. Plant Pathol. 44, 1–16. doi: 10.1080/07060661.2021.1980822
Walitang, D. I., Kim, K., Madhaiyan, M., Kim, Y. K., Kang, Y., and Sa, T. (2017). Characterizing endophytic competence and plant growth promotion of bacterial endophytes inhabiting the seed endosphere of Rice. BMC Microbiol. 17:209. doi: 10.1186/S12866-017-1117-0/FIGURES/4
Wang, C., Wang, C., Gao, Y.-L., Wang, Y.-P., and Guo, J.-H. (2016). A consortium of three plant growth-promoting rhizobacterium strains acclimates Lycopersicon esculentum and confers a better tolerance to chilling stress. J. Plant Growth Regul. 35, 54–64. doi: 10.1007/s00344-015-9506-9
Wang, M., Weiberg, A., Dellota, E., Yamane, D., and Jin, H. (2017). Botrytis small RNA Bc-siR37 suppresses plant defense genes by cross-kingdom RNAi. RNA Biol. 14, 421–428. doi: 10.1080/15476286.2017.1291112
Wang, W., Wu, Z., He, Y., Huang, Y., Li, X., and Ye, B. C. (2018). Plant growth promotion and alleviation of salinity stress in Capsicum annuum L. by Bacillus isolated from saline soil in Xinjiang. Ecotoxicol. Environ. Saf. 164, 520–529. doi: 10.1016/J.ECOENV.2018.08.070
Wani, Z. A., Kumar, A., Sultan, P., Bindu, K., Riyaz-Ul-Hassan, S., and Ashraf, N. (2017). Mortierella alpina CS10E4, an oleaginous fungal endophyte of Crocus sativus L. enhances apocarotenoid biosynthesis and stress tolerance in the host plant. Sci. Rep 7:8598. doi: 10.1038/s41598-017-08974-z
War Nongkhlaw, F., and Joshi, S. (2017). Microscopic study on colonization and antimicrobial property of endophytic bacteria associated with ethnomedicinal plants of Meghalaya. J. Microsc. Ultrastruct. 5, 132–139. doi: 10.1016/J.JMAU.2016.09.002
White, J. F., Kingsley, K. L., Zhang, Q., Verma, R., Obi, N., Dvinskikh, S., et al. (2019). Review: endophytic microbes and their potential applications in crop management. Pest Manag. Sci. 75, 2558–2565. doi: 10.1002/PS.5527
White, J. F., Torres, M. S., Somu, M. P., Johnson, H., Irizarry, I., Chen, Q., et al. (2014). Hydrogen peroxide staining to visualize intracellular bacterial infections of seedling root cells. Microsc. Res. Tech. 77, 566–573. doi: 10.1002/JEMT.22375
Wiesel, L., Newton, A. C., Elliott, I., Booty, D., Gilroy, E. M., Birch, P. R. J., et al. (2014). Molecular effects of resistance elicitors from biological origin and their potential for crop protection. Front. Plant Sci. 5:655. doi: 10.3389/FPLS.2014.00655/ABSTRACT
Worsley, S. F., Macey, M. C., Prudence, S. M., Wilkinson, B., Murrell, J. C., and Hutchings, M. I. (2021). Investigating the role of root exudates in recruiting Streptomyces bacteria to the Arabidopsis thaliana microbiome. Mol. Front. J. 8:541. doi: 10.3389/fmolb.2021.686110
Xu, C., Ruan, H., Cai, W., Staehelin, C., and Dai, W. (2021). Identification of an exopolysaccharide biosynthesis gene in Bradyrhizobium diazoefficiens USDA110. Microorganisms 9, 2490. doi: 10.3390/MICROORGANISMS9122490
Xu, J. X., Li, Z. Y., Lv, X., Yan, H., Zhou, G. Y., Cao, L. X., et al. (2020). Isolation and characterization of Bacillus subtilis strain 1-L-29, an endophytic bacteria from Camellia oleifera with antimicrobial activity and efficient plant-root colonization. PLoS One 15:e0232096. doi: 10.1371/JOURNAL.PONE.0232096
Xu, L., Wang, A., Wang, J., Wei, Q., and Zhang, W. (2017). Piriformospora indica confers drought tolerance on Zea mays L. through enhancement of antioxidant activity and expression of drought-related genes. Crop J. 5, 251–258. doi: 10.1016/J.CJ.2016.10.002
Yan, X., Wang, Z., Mei, Y., Wang, L., Wang, X., Xu, Q., et al. (2018). Isolation, diversity, and growth-promoting activities of endophytic bacteria from tea cultivars of Zijuan and Yunkang-10. Front. Microbiol. 9:1848. doi: 10.3389/FMICB.2018.01848/BIBTEX
Zachow, C., Jahanshah, G., De Bruijn, I., Song, C., Ianni, F., Pataj, Z., et al. (2015). The novel lipopeptide poaeamide of the endophyte Pseudomonas poae RE*1-1-14 is involved in pathogen suppression and root colonization. Mol. Plant Microbe Interact. 28, 800–810. doi: 10.1094/MPMI-12-14-0406-R
Zeidler, D., Zähringer, U., Gerber, I., Dubery, I., Hartung, T., Bors, W., et al. (2004). Innate immunity in Arabidopsis thaliana: lipopolysaccharides activate nitric oxide synthase (NOS) and induce defense genes. Proc. Natl. Acad. Sci. U.S.A. 101, 15811–15816. doi: 10.1073/PNAS.0404536101
Zerrouk, I. Z., Benchabane, M., Khelifi, L., Yokawa, K., Ludwig-Müller, J., and Baluska, F. (2016). A Pseudomonas strain isolated from date-palm rhizospheres improves root growth and promotes root formation in maize exposed to salt and aluminum stress. J. Plant Physiol. 191, 111–119. doi: 10.1016/J.JPLPH.2015.12.009
Zhai, X., Luo, D., Li, X., Han, T., Jia, M., Kong, Z., et al. (2018). Endophyte Chaetomium globosum D38 promotes bioactive constituents accumulation and root production in Salvia miltiorrhiza. Front. Microbiol. 8:2694. doi: 10.3389/FMICB.2017.02694/BIBTEX
Zhang, C., Li, C., Liu, J., Lv, Y., Yu, C., Li, H., et al. (2017). The OsABF1 transcription factor improves drought tolerance by activating the transcription of COR413-TM1 in rice. J. Exp. Bot. 68, 4695–4707. doi: 10.1093/JXB/ERX260
Zhang, Q., Acuña, J. J., Inostroza, N. G., Mora, M. L., Radic, S., Sadowsky, M. J., et al. (2019). Endophytic bacterial communities associated with roots and leaves of plants growing in chilean extreme environments. Sci. Rep. 9:4950. doi: 10.1038/s41598-019-41160-x
Zheng, Y., Liang, J., Zhao, D. L., Meng, C., Xu, Z. C., Xie, Z. H., et al. (2020). The root nodule microbiome of cultivated and wild halophytic legumes showed similar diversity but distinct community structure in Yellow River delta saline soils. Microorganisms 8:207. doi: 10.3390/MICROORGANISMS8020207
Zhou, L., Zhang, Y., Ge, Y., Zhu, X., and Pan, J. (2020). Regulatory mechanisms and promising applications of quorum sensing-inhibiting agents in control of bacterial biofilm formation. Front. Microbiol. 11:2558. doi: 10.3389/fmicb.2020.589640
Ziemert, N., Alanjary, M., and Weber, T. (2016). The evolution of genome mining in microbes – a review. Nat. Prod. Rep. 33, 988–1005. doi: 10.1039/C6NP00025H
Zin, N. M., Ismail, A., Mark, D. R., Westrop, G., Schniete, J. K., and Herron, P. R. (2021). Adaptation to endophytic lifestyle through genome reduction by Kitasatospora sp. SUK42. Front. Bioeng. Biotechnol. 9:740722. doi: 10.3389/FBIOE.2021.740722/FULL
Zinniel, D. K., Lambrecht, P., Harris, N. B., Feng, Z., Kuczmarski, D., Higley, P., et al. (2002). Isolation and characterization of endophytic colonizing bacteria from agronomic crops and prairie plants. Appl. Environ. Microbiol. 68, 2198–2208. doi: 10.1128/AEM.68.5.2198-2208.2002
Zúñiga, A., Poupin, M. J., Donoso, R., Ledger, T., Guiliani, N., Gutiérrez, R. A., et al. (2013). Quorum sensing and indole-3-acetic acid degradation play a role in colonization and plant growth promotion of Arabidopsis thaliana by Burkholderia phytofirmans PsJN. Mol. Plant Microbe Interact. 26, 546–553. doi: 10.1094/MPMI-10-12-0241-R
Keywords: abiotic stress, biocontrol, detection of PGPEB, endophytism, sustainable agriculture
Citation: Rani S, Kumar P, Dahiya P, Maheshwari R, Dang AS and Suneja P (2022) Endophytism: A Multidimensional Approach to Plant–Prokaryotic Microbe Interaction. Front. Microbiol. 13:861235. doi: 10.3389/fmicb.2022.861235
Received: 24 January 2022; Accepted: 11 March 2022;
Published: 12 May 2022.
Edited by:
Ying Ma, University of Coimbra, PortugalReviewed by:
Rupali Gupta, Institute of Plant Protection, Agricultural Research Organization, Volcani Center, IsraelDivjot Kour, Eternal University, India
Copyright © 2022 Rani, Kumar, Dahiya, Maheshwari, Dang and Suneja. This is an open-access article distributed under the terms of the Creative Commons Attribution License (CC BY). The use, distribution or reproduction in other forums is permitted, provided the original author(s) and the copyright owner(s) are credited and that the original publication in this journal is cited, in accordance with accepted academic practice. No use, distribution or reproduction is permitted which does not comply with these terms.
*Correspondence: Pooja Suneja, poojapavit@gmail.com