- 1Department of Aquaculture, College of Animal Science and Technology, Sichuan Agricultural University, Chengdu, China
- 2College of Life Science, Sichuan Agricultural University, Ya’an, China
- 3Fisheries Institute, Sichuan Academy of Agricultural Sciences, Chengdu, China
Heat stress induced by global warming has damaged the well-being of aquatic animals. The skin tissue plays a crucial role as a defense barrier to protect organism, however, little is known about the effect of heat stress on fish skin, particularly in cold-water fish species. Here, we investigated the effects of mild heat stress (24°C, MS) and high heat stress (28°C, HS) on Siberian sturgeon skin using RNA-seq, histological observation, and microbial diversity analysis. In RNA-seq, 8,819 differentially expressed genes (DEGs) in MS vs. C group and 12,814 DEGs in HS vs. C group were acquired, of which the MS vs. C and HS vs. C groups shared 3,903 DEGs, but only 1,652 DEGs were successfully annotated. The shared DEGs were significantly enriched in pathways associating with mucins synthesis. Histological observation showed that the heat stresses significantly reduced the number of skin mucous cells and induced the damages of epidermis. The microbial diversity analysis elicited that heat stress markedly disrupted the diversity and abundance of skin microbiota by increasing of potential pathogens (Vibrionimonas, Mesorhizobium, and Phyllobacterium) and decreasing of probiotics (Bradyrhizobium and Methylovirgula). In conclusion, this study reveals that heat stress causes adverse effects on sturgeon skin, reflecting in decreasing the mucus secretion and disordering the mucosal microbiota, which may contribute to develop the preventive strategy for heat stress caused by global warming.
Introduction
In the past few decades, the increasingly pronounced global warming has gradually warmed the Earth’s water temperature (Rossati, 2017; Alfonso et al., 2021). The increasing water temperature is devastating for fish species, particularly in cold-water fish species that are poikilothermic vertebrates and particularly vulnerable to the change of environmental temperature. Excessively high or low water temperature can cause physiological dysfunction and even death in fishes (Little et al., 2020). In this case, the heat stress caused by global warming restricts the development of aquaculture primarily by impacting fisheries ecosystem and economic benefit (Brander, 2007; Ng’onga et al., 2019).
In aquatic environment, fish health is susceptible to the change of environmental factors like water temperature. To cope the possible threats in water environment, fishes have generated an important immune barrier skin (Glover et al., 2013; Gomez et al., 2013). Skin tissue, including its surface, is the first defender of contacting fish body to water environment, which contributes to combat against the adverse effects, such as stresses and pathogens (Llewellyn et al., 2014). Skin mucus is a strong sticky substance secreted by epidermal mucous cells, which covers the surface of fish skin, forming an effective protective barrier (Fæste et al., 2020). And, skin mucous is colonized by a complex community of microbiota that plays an essential role to resist environmental pathogens (Ellis, 2001; Minniti et al., 2017). Fish skin mucosal microbiota gives the contact of fish with surrounding water, but variations in water physico-chemistry drives microbial dysbiosis (Sylvain et al., 2016). Mohammed and Arias (2015) reported that potassium permanganate caused changes in the skin microbiota of catfish and raises susceptibility to columnar disease, and Sylvain et al. (2016) study found that a drop in pH caused changes in the skin microbiota of Amazonian fish, suggesting that skin mucosal microbiota can be used as a marker to monitor fish health and improve their growth performance through rapid diagnosis. Unfortunately, the heat stress-derived variations in mucus secretion and mucosal microbiota in fish skin are poorly understood.
Siberian sturgeon (Acipenser baerii) is widely farmed around the world because of richness of its flesh in unsaturated fatty acids and protein and high quality caviar (Bronzi and Rosenthal, 2014; Bronzi et al., 2019). The optimal living temperature for Siberian sturgeon is around 20°C (Secor and Gunderson, 1998; Kappenman et al., 2009; Silvestre et al., 2010). Warm water (24°C), or excessive temperature water (28°C), was proved to derive stress response in Siberian sturgeon, eventually leading to the collapse of immunity and even death (Castellano et al., 2017; Yang et al., 2021). However, whether and how heat stress poses a threat to the skin of cold-water fish, such as sturgeon, remains to be explored. In this study, we firstly performed RNA-Seq to uncover the gene expression features in skin tissue of Siberian sturgeon suffered from heat stresses at 24 and 28°C. The histological observation was carried out to assess the pathological change in the skin of Siberian sturgeon under the heat stresses. Additionally, we analyzed the community structure of the skin microbiota by high throughput sequencing. This study aims to reveal the preliminary mechanism of the effect of heat stress on fish skin.
Materials and Methods
Experimental Fishes
A total of 90 healthy juvenile Siberian sturgeon (143.6 ± 4.6 g) were supplied from a sturgeon aquafarms (Hongya Donglong Sturgeon Industry Co., Ltd., Leshan, China). The fishes were then bred in circular glass tanks (1.5 m diameter and 1 m height) in laboratory. During fish domestication, water quality parameters including temperature, dissolved oxygen and pH were maintained at 20 ± 1.0°C, 7.1 ± 0.8 mg⋅L–1 and 7.8 ± 0.3, respectively, and fishes were fed with commercial diet (3% of fish weight) three times per day (at 8:00 am, 14:00 pm, and 20:00 pm, respectively). All animal handling procedures were approved by the Animal Care and Use Committee of Sichuan Agricultural University, following the guidelines of animal experiments of Sichuan Agricultural University under permit number AW-2020302179.
Heat Stress Treatment
The optimum growth status for Siberian sturgeon has been reported to be about 20°C (Aidos et al., 2020), but the living temperature raising to 24 and 28°C caused distinct stress response and thus effected the survival rate during sturgeon farming (Castellano et al., 2017; Aidos et al., 2020; Yang et al., 2021). After 2 weeks acclimatization under laboratory condition, 90 fishes were randomly divided into three groups, each group includes three tanks, 10 fishes per tank. The first group was the control group (C), the water temperature was kept at 20.0 ± 1.0°C. Fishes in the second and third groups underwent mimetic long-term heat stresses, mild heat stress (MS) and high heat stress (HS), by raising slowly from 20°C ± 1.0°C to 24°C ± 1.0°C and 28°C ± 1.0°C in 1 week, respectively, and kept the temperatures for 1 week.
Sample Collection
After the heat stress processes, the skin tissues of all fishes were aseptically collected. Briefly, six fishes from each group were randomly anesthetized by MS-222. The skin tissues were aseptically excised from both the dorsal side and ventral side and stored at −80°C until for use. Of these six skin samples, three were used for RNA-seq, while the rest were used for microbial diversity analysis. To preserve the integrity of skin epithelial cells, the skin samples jointed with subcutaneous muscle from random three fishes were collected and fixed in 10% neutral buffered formalin for at least 24 h, until for histological observation.
Histopathological Observation
The fixed skin tissues were dehydrated through a conventional alcohol gradient, then cleared in xylene and embedded in paraffin wax. Sections of 5 μm were prepared and mounted on slide for hematoxylin and eosin (H&E) and periodic acid Schiff (PAS) staining. After neutral sealing with glue, the slides were observed under an optical microscope (Nikon, Tokyo, Japan). The number of mucous cells was measured in 10 visual fields and then calculated the average.
To evaluate the effects of heat stress on the skin of Siberian sturgeon, we evaluated the skin damage based on a scoring system proposed by Baums et al. (2013). Briefly, abnormal changes in the epithelial layer of the skin, such as hyperplasia, necrosis, deposits, hypertrophy, hyperemia, or atrophy, were assessed with using a score of 0–5: (0) unchanged; (1) mildly altered; (3) moderately altered; and (5) severely altered (diffuse necrosis).
RNA-Seq
RNA Extraction, Library Construction, and Sequencing
To explore the gene expression features of skin under the heat stresses, RNA-seq was performed. According to the manufacturer’s instructions (Invitrogen, Carlsbad, CA, United States), TRIzol®reagent was used to extract total RNA from the skin samples, and DNase I (Takara, Dalian, China) was used to remove genomic DNA. Then, the RNA quality and quantification were determined by 2100 Bioanalyzer (Agilent Technologies, Santa Clara, CA, United States) and ND-2000 (NanoDrop Technologies, Shanghai, China), respectively. High-quality RNA samples were selected to construct sequencing libraries by TruSeq™ RNA sample preparation Kit (San Diego, CA, United States). The libraries were sequenced on an Illumina NovaSeq 6000 sequencing platform (Shanghai Biozeron Biotechnology Co., Ltd).
De novo Assembly and Annotation
The raw paired end reads were firstly trimmed and controlled the quality by Trim Galore and Fast QC software to acquire clean data. Then, the clean data from all samples were used to de novo assembly by Trinity1. All the assembled transcripts were searched against the NCBI protein non-redundant (NR), String, and KEGG databases using BLASTX to identify the proteins that had the highest sequence similarity with the given transcripts to retrieve their function annotations and a typical cut-off E-values less than 1.0 × 10––5 was set up. Blast2GO program2 was used to get Gene Ontology (GO) annotations of unique assembled transcripts for describing biological processes, molecular functions, and cellular components. Metabolic pathway analysis was performed using the Kyoto Encyclopedia of Genes and Genomes (KEGG)3.
Differential Expression and Functional Enrichment Analyses
Reads per kilobase of exon per million mapped reads (RPKM) value was used for identification of differentially expressed genes (DEGs) amongst the treatments. RSEM software was used to quantify gene and isoform abundances. Differential expression analysis was performed using the DEGSeq R statistical package software. In addition, GO functional enrichment analysis of DEGs and KEGG pathway analysis were implemented by Goatools4 and KOBAS5.
Microbial Diversity Analysis
DNA Extraction and Purification
Microbial DNA was extracted from Siberian sturgeon skin using the E.Z.N.A.®DNA Kit (Omega Bio-Tek, Norcross, GA, United States), according to manufacturer’s protocols. After extraction, the V1-V9 region of samples were amplified by polymerase chain reaction (PCR) with the forward primers (27F: 5′-AGRGTTYGATYMTGGCTCAG-3′) and the reverse primer (1492R: 5′-RGYTACCTTGTTACGACTT-3′). Then PCR product was extracted from 2% agarose gel and purified with the AxyPrep DNA Gel Extraction Kit (Axygen Biosciences, Union City, CA, United States) according to the manufacturer’s instructions.
Sequencing and Processing
The libraries were prepared from the amplified DNA by blunt-ligation according to the manufacturer’s instructions (Pacific Biosciences). Purified libraries from the Zymo and HMP mock communities were sequenced on dedicated PacBio Sequel II 8M cells using the Sequencing Kit 2.0 chemistry. All amplicon sequencing was performed by Shanghai Biozeron Biotechnology Co., Ltd. (Shanghai, China). PacBio raw reads were processed using the SMRT Link Analysis software (version 9.0), the dataset was prepared for analysis by excluding that: (i) shorter than 50 bp, (ii) <10 bp in libraries, (iii) ambiguous nucleotides that constituted over 20% of the sequence. The remaining sequences were clustered into operational taxonomic units (OTUs) with 98.65% similarity cutoff using UPARSE (version 7.1)6 and chimeric sequences were identified and removed using UCHIME. The phylogenetic affiliation of each 16S rRNA gene sequence was analyzed by RDP Classifier7 against the SILVA (SSU132) 16S rRNA database using confidence threshold of 70% (Amato et al., 2013).
Data Analysis
All data are expressed as mean ± standard deviation. Significances between groups were analyzed by one-way analysis and t-test. Statistical analyses were performed using SPSS 22.0 software (IBM Corp., Armonk, NY, United States). P-value < 0.05 was considered significant for all tests.
Results
Characteristics of RNA-Seq Data and Assembly Annotation
Nine RNA-seq libraries were constructed from the skin of Siberian sturgeon under the heat stresses, and three biological replicates were set up for each group. After filtering, the number of clean reads in each library ranged from 24 to 34 million, and the clean Q30 values ranged from 93.17 to 93.85% per sample, indicating that the sequencing data were of high quality and of significance for further analysis (Table 1). All datasets from the Illumina sequencing platform can be found in the National Center for Biotechnology Information Short Read Archive Database under Accession number (PRJNA731649).
To fully understand the gene functional information, the unigenes were annotated in five databases (Supplementary Figure 1B). Finally, 172,158 unigenes with annotation information were obtained. The GO annotation statistic showed that most unigenes were annotated to biological processes and cellular components (Supplementary Figure 1C). The KEGG pathway analysis revealed that the majority of the unigenes were annotated to signal transduction and endocrine system (Supplementary Figure 1D).
Identification of Differentially Expressed Genes and Gene Ontology Enrichment Analysis
A total of 28,072 differentially expressed genes (DEGs) were detected in the three comparison groups (MS vs. C, HS vs. C and HS vs. MS). 4,182 upregulated DEGs and 4,636 downregulated DEGs were detected in the MS vs. C group, while 4,673 upregulated DEGs and 8,140 downregulated DEGs were detected in the HS vs. C group. In addition, 2,692 upregulated DEGs and 3,749 downregulated DEGs were detected in the HS vs. MS group (Supplementary Figure 2). To elaborate the effects of the heat stresses on Siberian sturgeon skin, we selected the top 30 significantly enriched terms of the GO enrichment for analysis. A large number of DEGs in the MS vs. C group were categorized to “responses to cAMP,” “keratinocyte differentiation,” “keratinization,” “responses to unfolded proteins,” and “defense responses to bacteria.” The “regulation of chemokine production,” “responses to unfolded protein,” “keratinocyte differentiation,” “cellular responses to a mechanical stimulus,” and “skin development categories” dominated the HS vs. C group. In HS vs. MS group, the categories “regulation of sequestering of calcium ion” and “response to calcium ion” were highly enriched (Supplementary Figure 3).
Kyoto Encyclopedia of Genes and Genomes Pathway Analysis
In order to analyze the genes that responded to different temperatures, the Venn diagrams were drawn (Figure 1A). Two comparing groups shared 3,903 DEGs that included 1,652 function annotated genes. The KEGG enrichment of these annotated genes was then visualized by bubble chart according to their FPKM values (Figure 1B). Notably, the genes related to protein synthesis (e.g., “protein digestion and absorption” and “protein processing in the endoplasmic reticulum”) were significantly enriched in both MS vs. C and HS vs. C groups. We also analyzed the expression difference of the non-shared annotated genes after stimulation with mild heat stress and high heat stress, 1,494 and 3,032 non-shared annotated genes were identified, respectively (Figure 2A). The KEGG enrichment of the non-shared genes was then visualized by bubble chart based on their FPKM values (Figure 2B). Interestingly, lots of genes were enriched in “IL-17 signaling pathway,” “Osteoclast differentiation,” and “TNF signaling pathway” in MS vs. C group but not in HS vs. C group. And, “Cardiac muscle contraction,” “Apelin signaling pathway,” and “Tight junction” pathway were only enriched in HS vs. C group (Figure 2C).
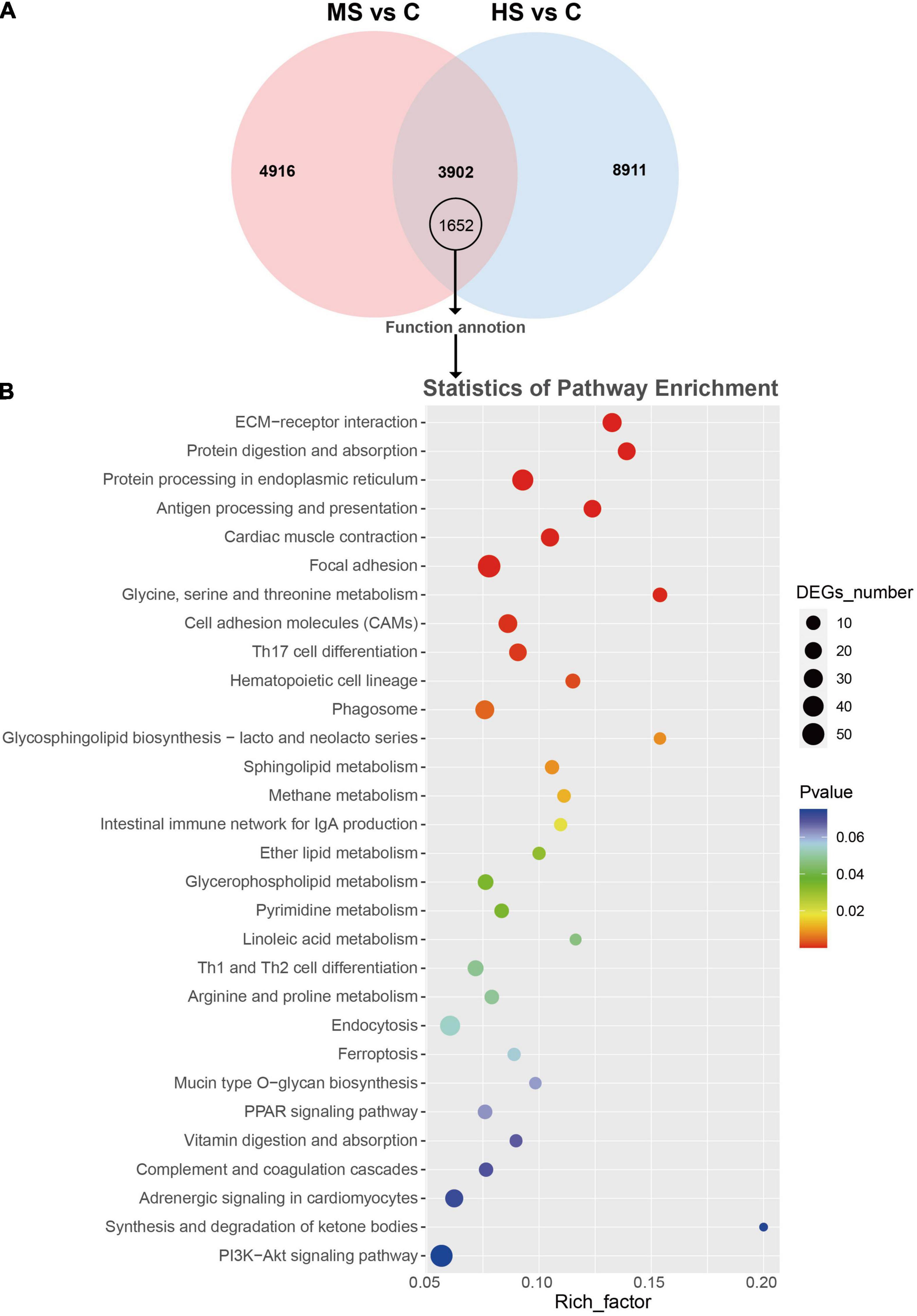
Figure 1. Venn diagram, KEGG enrichment analysis of the shared DEGs in MS vs. C and HS vs. C groups. (A) Venn diagram showed the 8,818 in MS vs. C group and 12,813 DEGs in HS vs. C group, and 1,652 shared annotated DEGs. (B) Scatter diagram of KEGG enrichment analysis of the shared DEGs. X-axis (enrichment factor) indicates the enrichment level of DEGs in each pathway, while Y-axis represents the reliability of enrichment analysis of each pathway.
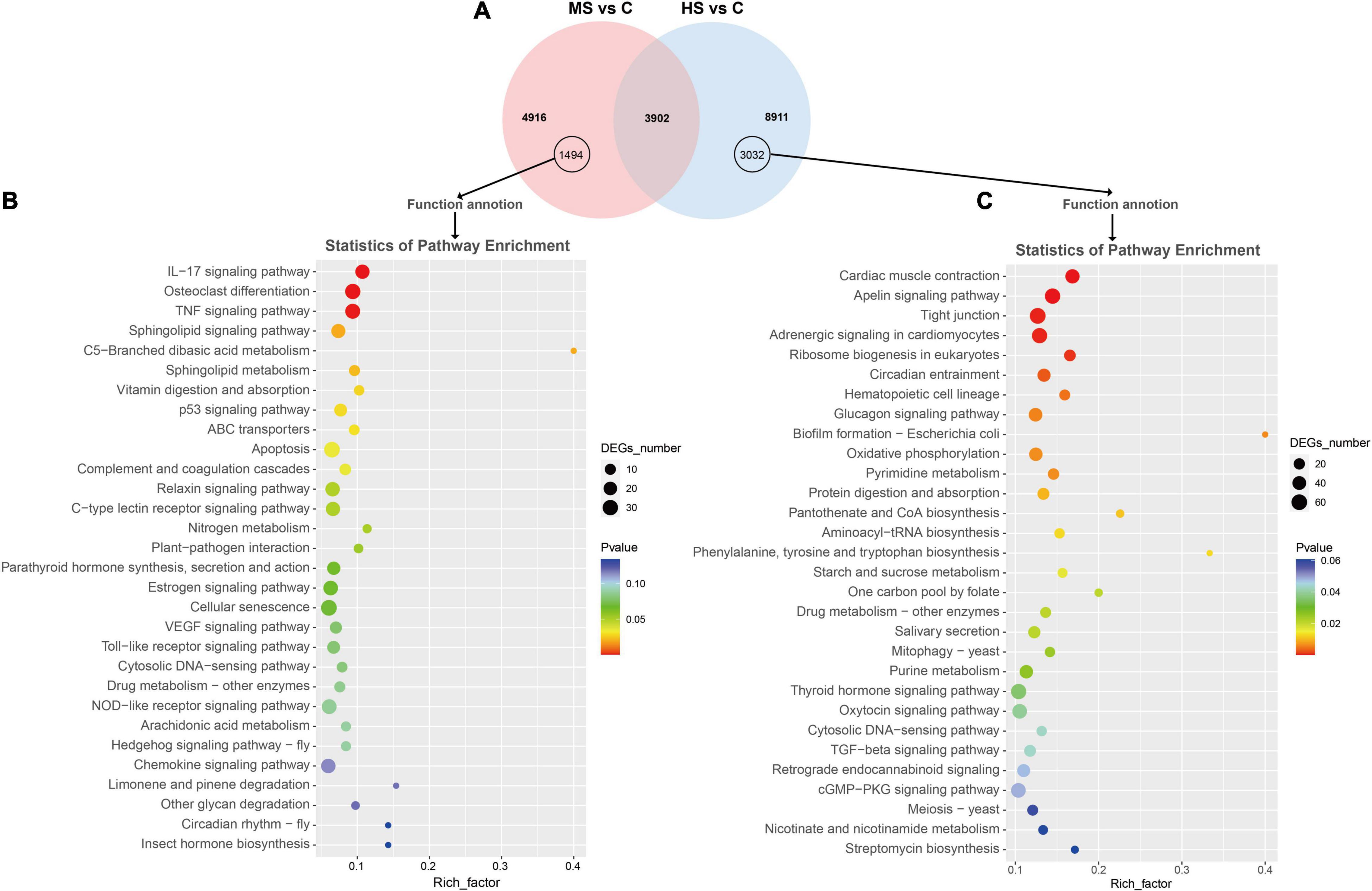
Figure 2. Venn diagram and KEGG enrichment analysis of the non-shared DEGs in MS vs. C and HS vs. C groups. (A) Venn diagram showed 1,494 and 3,032 non-shared annotated DEGs in MS vs. C and HS vs. C groups, respectively. (B,C) Scatter diagram of KEGG enrichment analysis. X-axis (enrichment factor) indicates the enrichment level of DEGs in each pathway, while Y-axis represents the reliability of enrichment analysis of each pathway.
Heat Stress Damages the Skin Epidermis and Decreases the Number of Mucous Cells
To investigate the effect of heat stress on Siberian sturgeon, skin samples were collected for histological observation. The histological observation of the skin samples are shown in Figure 3. The results showed that the epithelial layer of the MS group was exfoliating and necrotizing with a raise of cell debris compared to the control group (Figure 3B). The epidermal layer of the skin in the HS group was partially broken, with slight dissociation and epidermal shriveling (Figure 3C). The pathological scores also indicated significant pathological changes in the MS and HS groups (Figure 3D). PAS staining revealed that the number of mucous cells had decreased significantly in the MS and HS groups compared to the control group (Figures 3E–G). Significant difference in the number of mucous cells from different group also confirmed these results (Figure 3H).
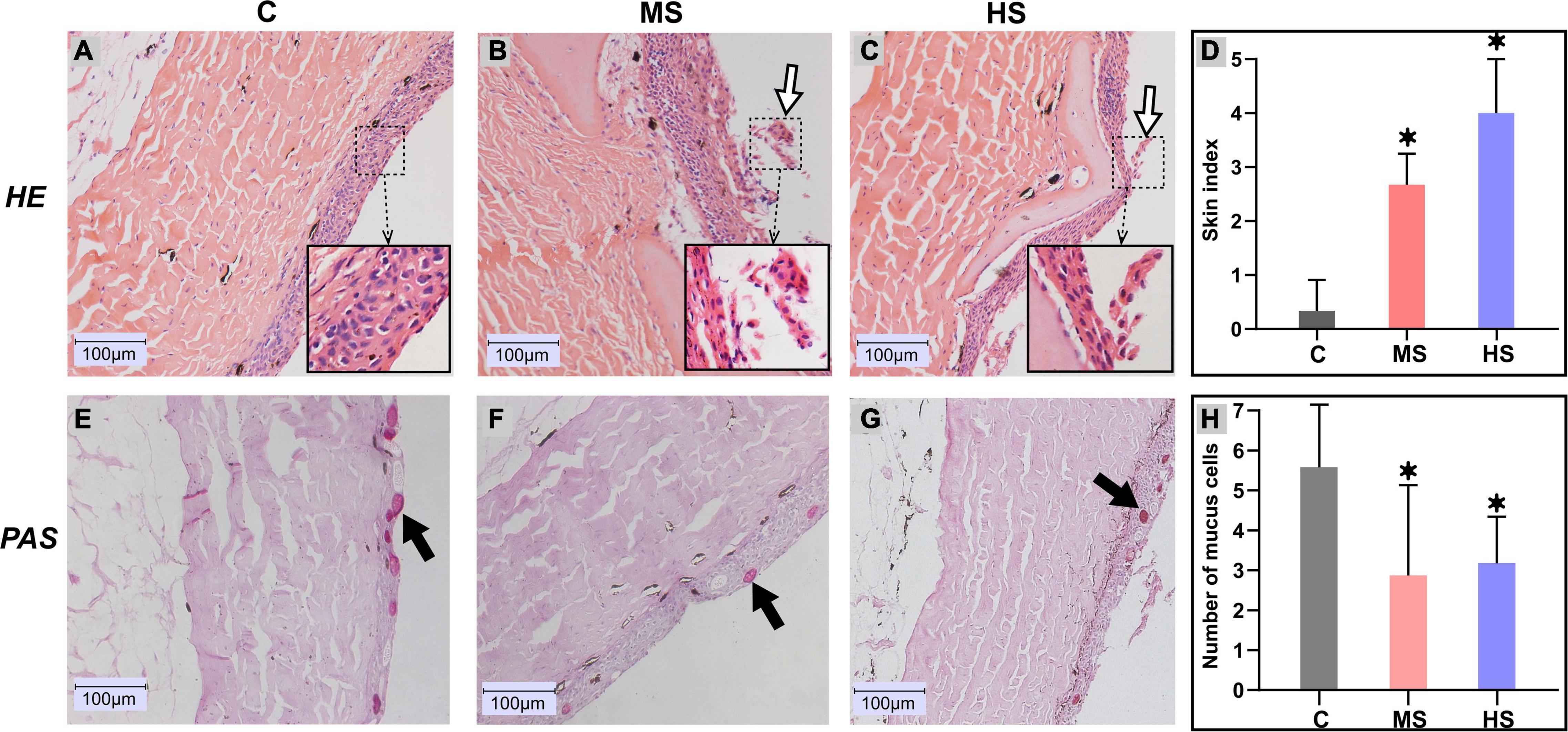
Figure 3. Histological changes in the skin of Siberian sturgeon after exposure to the heat stresses. (A–C) indicates hematoxylin and eosin (H&E) staining, (D–F) represents Periodic Acid-Schiff (PAS) staining. (A,E) 20°C group, (B,F) 24°C group, (C,G) 28°C group, scale bar = 100 μm. The exfoliated cells are shown by the white arrows, black arrows delegate mucus cells. (D) Skin health status (organ index) of sturgeon in different groups. (H) Mucus cell count between different groups, *p < 0.05 indicates a significant difference compared to the control group.
Characteristics of 16S rRNA Sequencing and the Operational Taxonomic Unit Distribution
A total of 107,557 valid sequences were obtained from the nine samples. In addition, the number of valid sequences in the skin samples ranged from 11,673 to 22,761. The rarefaction curve reached the plateau phase and the rank abundance curve was a long and flat broken line, indicating that the sequencing depth, richness, and evenness met the sequencing requirements (Supplementary Figure 4). The raw 16S rRNA sequencing data have been submitted to the National Center for Biotechnology Information (NCBI) with Accession number PRJNA768965.
The distribution of OTUs in different groups is shown in Figure 4. Seventy-seven OTUs were shared by sturgeon under the three temperatures, and microbial diversity decreased slightly in group MS compared to the control group, while HS group was similar to the control group (Figure 4A). The β-diversity analysis revealed that all samples were divided into three relatively independent categories, indicating that the diversity of microorganisms varied between the groups (Figure 4B), which was consistent with the Venn diagram results.
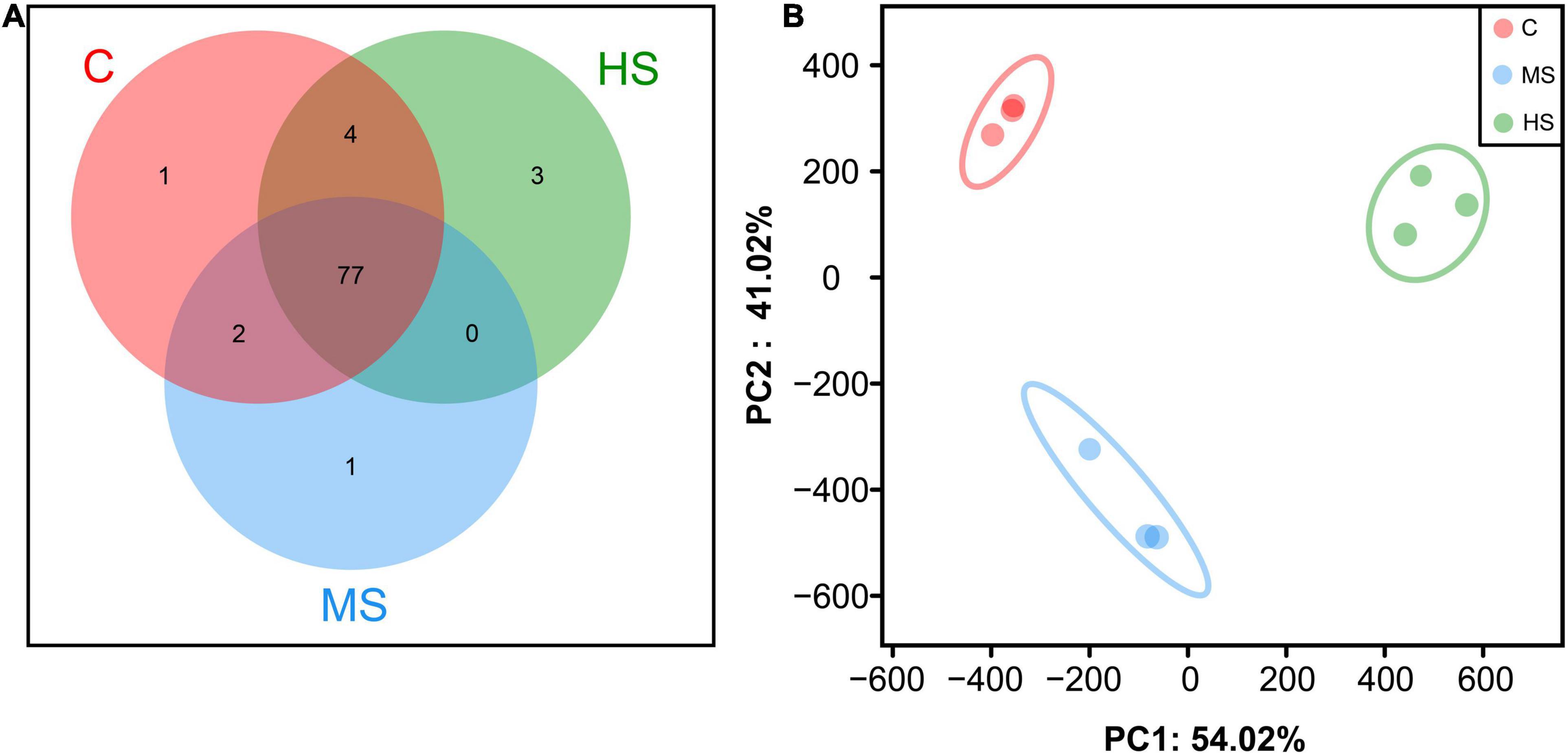
Figure 4. Species composition analysis among different groups. (A) Venn comparison diagram of different groups. (B) Principal Component Analysis (PCA).
Heat Stress Disrupts the Composition of the Skin Microbiota
Analysis of microbial composition showed that the heat stresses induced significant changes in skin microbial composition. The most abundant bacterial types at the phylum level were Proteobacteria and Bacteroidota under the heat stresses (Figure 5A). At the genus level, we observed a notable decrease in Methylovirgula after heat stress, while the Rhodanobacter was significantly higher, particularly in group HS after heating up, with a relative abundance of 11% (Figure 5B).
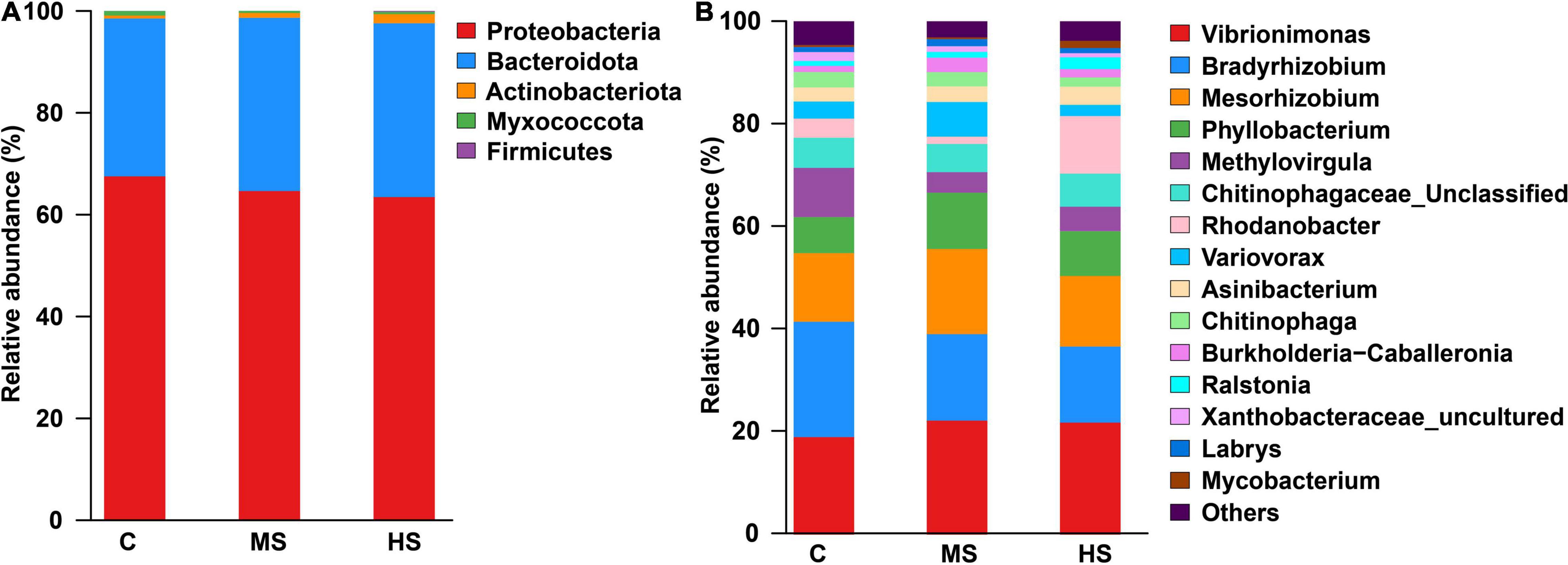
Figure 5. The relative abundance of phylum (A) and genus (B) under the heat stresses, only classes that are present at abundances >1% in at least one sample are shown.
Heat Stress Reduces the Abundance of Probiotics and Raises the Abundance of Potential Pathogen
Linear discriminant analysis effect size analysis was carried out to further evaluate the differences in the structure of the skin microbiota in Siberian sturgeon under the heat stresses. The results showed significant differences in the skin microbiota between the heat stress groups and the control group (Figures 6A,B). We screened the microbiota with relative abundances >5% into two major categories of potential pathogen and probiotics according to their functions to further verify this difference at the species level (Figure 6C). Although there were exceptions in the minority of the microbiota, the relative abundance of potential pathogen (e.g., Vibrionimonas, Mesorhizobium, and Phyllobacterium) tended to increase, while the relative abundance of the dominant probiotic (Bradyrhizobium and Methylovirgula) decreased significantly.
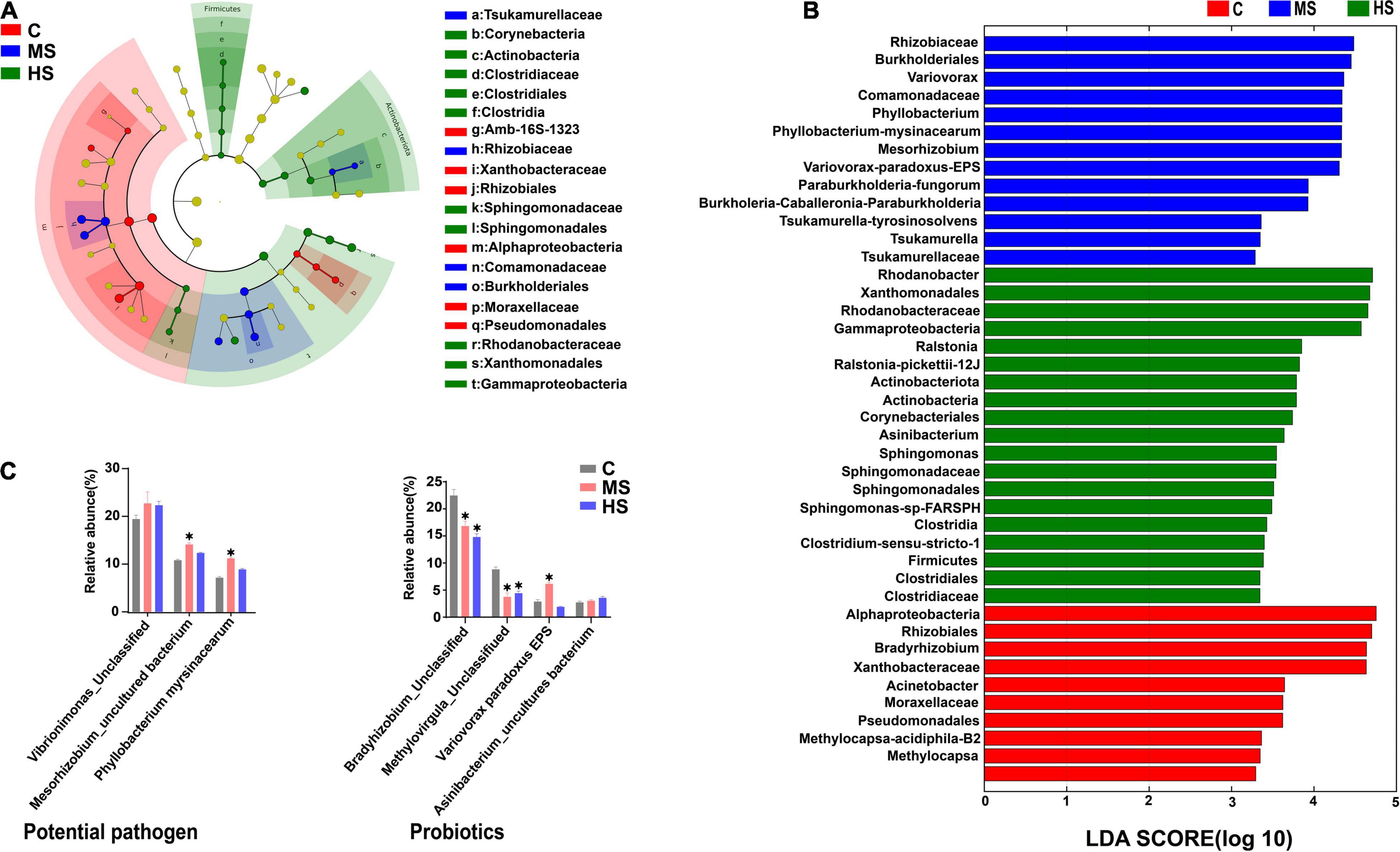
Figure 6. LEfSe analysis of the differences in skin microbiota of Siberian sturgeon. (A) Taxonomic characterization of differences in skin microbiota of Siberian sturgeon under different temperature treatments, the concentric circles from the inside to the outside stand for different taxonomic classes (phylum to family). Different color areas point to different groups (the red nodes in the branches represented microbial taxa that play an important role in the control group, the blue nodes indicate microbial taxa that plays a vital role in the MS group, the green nodes mean microbial taxa that play a key role in the HS group, while the yellow nodes show no significant difference). Abundance of skin microbiota were revealed by the size of each node. (B) Linear discriminant analysis (LDA) for three groups, scores for remarkable abundant of skin microbiota. (C) The relative proportion of species under different temperatures, only classes that are present at abundances >1% in at least one sample are shown. *p < 0.05, indicates a significant difference compared to the group C.
Discussion
In this study, transcriptome, histopathological observation and microbiota were analyzed after the heat stresses in the skin of Siberian sturgeon. Transcriptome analysis determined that “keratinocyte differentiation” and “keratinization” terms were highly enriched in the GO enrichment analysis of DEGs of MS vs. C group (Supplementary Figure 3). Differentiation of keratinocytes is a process in which epidermal cells convert into protective stratum corneum, providing skin with the ability to retain moisture (Elias, 2007; Sumitomo et al., 2019). Thus, heat stress may directly affect the formation of stratum corneum, but the mechanism needs more evidences to be uncovered. In addition, keratinocytes produce countless antimicrobial peptides and chemokines that form an effective immune barrier against environmental pathogens (Furue et al., 2020). In HS vs. C group, the term “regulation of chemokine production” was significantly enriched in the GO enrichment analysis of DEGs. Therefore, it is reasonable to conclude that heat stress may also damage the skin immune system by affecting the secretion of chemokines in Siberian sturgeon.
In KEGG enrichment analysis, the enrichment results of the shared DEGs between MS vs. C and HS vs. C groups showed that the heat stresses influenced “ECM-receptor interaction” pathway (Figure 1B). “ECM-receptor interaction” pathway is closely related to the maintenance of organismal health (Hwang et al., 2020; Sainio and Järveläinen, 2020). Thus, the enrichment in “ECM-receptor interaction” pathway suggests that heat stress may damage the balance of skin health via ECM-receptor interaction pathway. Moreover, the pathways related to protein synthesis (e.g., protein digestion and absorption, protein processing in the endoplasmic reticulum) were significantly enriched, in the same line, “Glycine, serine, and threonine metabolic” and “Mucin type O-glycan biosynthesis” pathways were also significantly enriched. Mucins are a large family of high molecular weight proteins, which are composed of amino acids (such as glycine, serine, and threonine) (Feller et al., 1990; Chakraborty et al., 2011; Ramsey et al., 2016; Bansil and Turner, 2018). And, Mucin O-glycosylation is a common covalent modification of serine and threonine residues of glycoproteins (Van den Steen et al., 1998; Bagdonaite et al., 2021). Therefore, our results indicate that heat stress may affect the synthesis of mucins via amino acid metabolic and O-glycan biosynthesis.
In histopathological observation, the results showed that the heat stresses caused the damages and decreased the number of mucous cells in epidermis. Previous studies had shown that the number of mucous cells was decreased when fishes were exposed to stressful conditions, such as catching and transport (Szakolczai, 1997) and raised salinity (Shephard, 1994). Our results reveal that, like other stresses, heat stress also decreases the number of mucous cells in epidermis. Besides, it is well-known that mucous cells directly reflect the decrease of mucus generation (Bols et al., 2001). Thus, these results provide a potential mechanism of the mucin decrease that the mucin generation was decreased by the damages of epidermis and therefore to reduce the number of mucous cells in epidermis.
The outermost layer of fish skin is epidermis, which reflects the degree of stress because of the direct contact with the surrounding environment (Ross et al., 2019). The symbiotic microbiota colonized on epidermis is believed to closely relate to health situation of host (Chen et al., 2018). It has been demonstrated that water with chemical pollutions (e.g., hypoxia, ammonia, and antibiotics) alters the diversity and composition of the microbial community in cultured systems and, thereby, to affect the microbiota of fish skin (Navarrete et al., 2008; Boutin et al., 2013; Pindling et al., 2018). However, the effects of heat stress on fish skin microbiota have been rarely reported. Our study explored the response of skin microbiota to the heat stresses in Siberian sturgeon. The skin microbiota shared identical colony categories at the three heat stress conditions, but their proportions were different, suggesting that the heat stresses altered the abundance of skin microbiota. Specifically, we observed an increase in the abundance of the potential pathogen (Vibrio, Mesorhizobium, and Phyllobacterium) under the heat stresses. Vibrio was reported to impair the innate immune system by reducing the mucus protease activity in fish skin (Guardiola et al., 2014; Cámara-Ruiz et al., 2021). And, changes in the abundance of intestinal pathogenic bacteria, Mesorhizobium and Vibrio, were associated to pathogen infection (Yang et al., 2017). Phyllobacterium is believed to negatively affect the colonization of microbial community in skin (Mei et al., 2020). In contrast, significant changes in the abundance of some probiotic bacteria (Bradyrhizobium, Methylovirgula, Variovorax paradoxus and Asinibacterium) were also observed under the heat stresses. Bradyrhizobium is believed to have antagonistic effects against 10 pathogenic bacteria (Gabriella et al., 2017). Methanobacterium and Asinibacterium are considered to produce poly-β-hydroxybutyrates that inhibit the growth of pathogens in the host bacterial community (Defoirdt et al., 2007; Halet et al., 2007; Magalhes et al., 2020). And, Variovorax paradoxus is associated with biofilm formation (Fredendall et al., 2020). In summary, the abundance changes in the potential pathogens and the probiotics indicate that heat stress derives adverse influences, which associate to break the balance between pathogens and probiotics in bacterial community of Siberian sturgeon.
The mucus is one of the most important nutrient supplies for skin microbiota. Previous study proved that the variance of the proteome of Atlantic salmon (Salmo salar) skin mucus induced change of resident bacterial community (Minniti et al., 2019). In this study, the changes of the skin microbial community were happened when the mucus generation was decreased. Thus, instead of the unconnected events, the decrease of mucus generation is closely associated with the changes of microbial community on the skin of Siberian sturgeon under heat stress.
Conclusion
In this study, the damaged epidermal layer and the reduced number of mucous cells in skin, and the decreased feeding rate and protein synthesis, were observed under heat stresses at 24 and 28°C. These events point that heat stress influences skin- and mucin-constructed barriers against the surrounding environment. Two possible mechanisms are that: the heat stresses reduced the mucin synthesis by influencing the energy supplements via intestine intake, and decreased the mucin generation by damaging the epidermis and therefore to reduce the number of mucous cells in epidermis. The decrease of mucus generation is closely associated with the changes of microbial community on the skin, reflecting in reducing the abundance of probiotics and raising the abundance of potential pathogen.
Data Availability Statement
The datasets presented in this study can be found in online repositories. The names of the repository/repositories and accession number(s) can be found below: https://www.ncbi.nlm.nih.gov/, PRJNA731649 and https://www.ncbi.nlm.nih.gov/, PRJNA768965.
Ethics Statement
The animal study was reviewed and approved by the Sichuan Agricultural University Ethics Committee.
Author Contributions
SY, WX, CT, and JD: conceptualization. SY, CT, CZ, and XH: data curation. JW and XD: formal analysis. SY, JW, and GL: funding acquisition. SY, WX, CT, QC, YL, CL, and QG: investigation. SY, WX, ML, and LF: methodology. JW, JJ, and WL: resources. XD: software. GL: supervision. WX, DL, ZD, and XH: validation. CT: visualization. WX and CT: writing – original draft. SY, WX, and JW: writing – review and editing. All authors contributed to the article and approved the submitted version.
Funding
This study was supported by the Project of Provincial 14th Five Years’ Animal Breeding of Sichuan Province (2021YFYZ0015), Science and Technology Achievement Transfer and Transformation Demonstration Project of Sichuan Province (2021ZHCG0065), Applied Basic Research from Technological Office of Sichuan Province, and Two Sides Supporting Plan in Sichuan Agricultural University.
Conflict of Interest
The authors declare that the research was conducted in the absence of any commercial or financial relationships that could be construed as a potential conflict of interest.
Publisher’s Note
All claims expressed in this article are solely those of the authors and do not necessarily represent those of their affiliated organizations, or those of the publisher, the editors and the reviewers. Any product that may be evaluated in this article, or claim that may be made by its manufacturer, is not guaranteed or endorsed by the publisher.
Acknowledgments
We are grateful to the Shanghai Biozeron Biotechnology Co. Ltd., for performing RNA sequencing and assisting with bioinformatics analysis.
Supplementary Material
The Supplementary Material for this article can be found online at: https://www.frontiersin.org/articles/10.3389/fmicb.2022.860079/full#supplementary-material
Footnotes
- ^ http://trinityrnaseq.sourceforge.net/
- ^ http://www.blast2go.com/b2ghome
- ^ http://www.genome.jp/kegg/
- ^ https://github.com/tanghaibao/Goatools
- ^ http://kobas.cbi.pku.edu.cn/home.do
- ^ http://drive5.com/uparse/
- ^ http://rdp.cme.msu.edu/
References
Aidos, L., Cafiso, A., Bertotto, D., Bazzocchi, C., Radaelli, G., and Di Giancamillo, A. (2020). How different rearing temperatures affect growth and stress status of Siberian sturgeon Acipenser baerii larvae. J. Fish. Biol. 96, 913–924. doi: 10.1111/jfb.14280
Alfonso, S., Gesto, M., and Sadoul, B. (2021). Temperature increase and its effects on fish stress physiology in the context of global warming. J. Fish. Biol. 98, 1496–1508. doi: 10.1111/jfb.14599
Amato, K. R., Yeoman, C. J., Kent, A., Righini, N., Carbonero, F., Estrada, A., et al. (2013). Habitat degradation impacts black howler monkey (Alouatta pigra) gastrointestinal microbiomes. ISME J. 7, 1344–1353. doi: 10.1038/ismej.2013.16
Bagdonaite, I., Pallesen, E. M. H., Nielsen, M. I., Bennett, E. P., and Wandall, H. H. (2021). Mucin-Type O-GalNAc Glycosylation in Health and Disease. Adv. Exp. Med. Biol. 1325, 25–60. doi: 10.1007/978-3-030-70115-4_2
Bansil, R., and Turner, B. S. (2018). The biology of mucus: composition, synthesis and organization. Adv. Drug Deliv. Rev. 124, 3–15. doi: 10.1016/j.addr.2017.09.023
Baums, C. G., Hermeyer, K., Leimbach, S., Adamek, M., Czerny, C. P., Horstgen-Schwark, G., et al. (2013). Establishment of a model of Streptococcus iniae meningoencephalitis in Nile tilapia (Oreochromis niloticus). J. Comp. Pathol. 149, 94–102. doi: 10.1016/j.jcpa.2012.10.003
Bols, N. C., Brubacher, J. L., Ganassin, R. C., and Lee, L. E. J. (2001). Ecotoxicology and innate immunity in fish. Dev. Comp. Immunol. 25, 853–873. doi: 10.1016/s0145-305x(01)00040-4
Boutin, S., Bernatchez, L., Audet, C., and Derome, N. (2013). Network analysis highlights complex interactions between pathogen, host and commensal microbiota. PLoS One 8:e84772. doi: 10.1371/journal.pone.0084772
Brander, K. M. (2007). Global fish production and climate change. Proc. Natl. Acad. Sci. U.S.A. 104, 19709–19714. doi: 10.1073/pnas.0702059104
Bronzi, P., Chebanov, M., Michaels, J. T., Wei, Q., Rosenthal, H., and Gessner, J. (2019). Sturgeon meat and caviar production: global update 2017. J. Appl. Ichthyol. 35, 257–266. doi: 10.1111/jai.13870
Bronzi, P., and Rosenthal, H. (2014). Present and future sturgeon and caviar production and marketing: a global market overview. J. Appl. Ichthyol. 30, 1536–1546. doi: 10.1111/jai.12628
Cámara-Ruiz, M., Cerezo, I. M., Guardiola, F. A., García-Beltrán, J. M., Balebona, M. C., Moriñigo, M., et al. (2021). Alteration of the Immune Response and the Microbiota of the Skin during a Natural Infection by Vibrio harveyi in European Seabass (Dicentrarchus labrax). Microorganisms 9:964. doi: 10.3390/microorganisms9050964
Castellano, M., Silva-Álvarez, V., Fernández-López, E., Mauris, V., Conijeski, D., Villarino, A., et al. (2017). Russian sturgeon cultured in a subtropical climate shows weaken innate defences and a chronic stress response. Fish. Shellfish Immunol. 68, 443–451. doi: 10.1016/j.fsi.2017.07.048
Chakraborty, S., Bonthu, N., Swanson, B. J., and Batra, S. K. (2011). Role of mucins in the skin during benign and malignant conditions. Cancer Lett. 301, 127–141. doi: 10.1016/j.canlet.2010.11.004
Chen, Y. E., Fischbach, M. A., and Belkaid, Y. (2018). Skin microbiota-host interactions. Nature 553, 427–436. doi: 10.1038/nature25177
Defoirdt, T., Halet, D., Vervaeren, H., Boon, N., Van de Wiele, T., Sorgeloos, P., et al. (2007). The bacterial storage compound poly-beta-hydroxybutyrate protects Artemia franciscana from pathogenic Vibrio campbellii. Environ. Microbiol. 9, 445–452. doi: 10.1111/j.1462-2920.2006.01161.x
Elias, P. M. (2007). The skin barrier as an innate immune element. Semin. Immunopathol. 29, 3–14. doi: 10.1007/s00281-007-0060-9
Ellis, A. E. (2001). Innate host defense mechanisms of fish against viruses and bacteria. Dev. Comp. Immunol. 25, 827–839. doi: 10.1016/s0145-305x(01)00038-6
Feller, W. F., Henslee, J. G., Kinders, R. J., Manderino, G. L., Tomita, J. T., and Rittenhouse, H. G. (1990). Mucin glycoproteins as tumor markers. Immunol. Ser. 53, 631–672.
Fredendall, R. J., Stone, J. L., Pehl, M. J., and Orwin, P. M. (2020). Transcriptome profiling of Variovorax paradoxus EPS under different growth conditions reveals regulatory and structural novelty in biofilm formation. Access Microbiol. 2:acmi000121. doi: 10.1099/acmi.0.000121
Furue, M., Furue, K., Tsuji, G., and Nakahara, T. (2020). Interleukin-17A and Keratinocytes in Psoriasis. Int. J. Mol. Sci. 21:1275. doi: 10.3390/ijms21041275
Fæste, C. K., Tartor, H., Moen, A., Kristoffersen, A. B., Dhanasiri, A. K. S., Anonsen, J. H., et al. (2020). Proteomic profiling of salmon skin mucus for the comparison of sampling methods. J. Chromatogr. B Analyt. Technol. Biomed. Life Sci. 1138:121965. doi: 10.1016/j.jchromb.2019.121965
Gabriella, D. V. P., Da Cunha, D. G., Pedreira Mourino, J. L., Rodiles, A., Jaramillo-Torres, A., and Merrifield, D. L. (2017). Characterization of microbiota in Arapaima gigas intestine and isolation of potential probiotic bacteria. J. Appl. Microbiol. 123, 1298-1311 doi: 10.1111/jam.13572
Glover, C. N., Bucking, C., and Wood, C. M. (2013). The skin of fish as a transport epithelium: a review. J. Comp. Physiol. B 183, 877–891. doi: 10.1007/s00360-013-0761-4
Gomez, D., Sunyer, J. O., and Salinas, I. (2013). The mucosal immune system of fish: the evolution of tolerating commensals while fighting pathogens. Fish Shellfish Immunol. 35, 1729–1739. doi: 10.1016/j.fsi.2013.09.032
Guardiola, F. A., Cuesta, A., Arizcun, M., Meseguer, J., and Esteban, M. A. (2014). Comparative skin mucus and serum humoral defence mechanisms in the teleost gilthead seabream (Sparus aurata). Fish Shellfish Immunol. 36, 545–551. doi: 10.1016/j.fsi.2014.01.001
Halet, D., Defoirdt, T., Van Damme, P., Vervaeren, H., Forrez, I., Van de Wiele, T., et al. (2007). Poly-beta-hydroxybutyrate-accumulating bacteria protect gnotobiotic Artemia franciscana from pathogenic Vibrio campbellii. FEMS Microbiol. Ecol. 60, 363–369. doi: 10.1111/j.1574-6941.2007.00305.x
Hwang, J., Sullivan, M. O., and Kiick, K. L. (2020). Targeted Drug Delivery via the Use of ECM-Mimetic Materials. Front. Bioeng. Biotechnol. 8:69. doi: 10.3389/fbioe.2020.00069
Kappenman, K. M., Fraser, W. C., Toner, M., Dean, J., and Webb, M. (2009). Effect of Temperature on Growth, Condition, and Survival of Juvenile Shovelnose Sturgeon. Trans. Am. Fish. Soc. 138, 927–937. doi: 10.1577/t07-265.1
Little, A. G., Loughland, I., and Seebacher, F. (2020). What do warming waters mean for fish physiology and fisheries? J. Fish. Biol. 97, 328–340. doi: 10.1111/jfb.14402
Llewellyn, M. S., Boutin, S., Hoseinifar, S. H., and Derome, N. (2014). Teleost microbiomes: the state of the art in their characterization, manipulation and importance in aquaculture and fisheries. Front. Microbiol. 5:207. doi: 10.3389/fmicb.2014.00207
Magalhes, R., Guerreiro, I., Santos, R. A., Coutinho, F., and Oliva-Teles, A. (2020). Oxidative status and intestinal health of gilthead sea bream (Sparus aurata) juveniles fed diets with different ARA/EPA/DHA ratios. Sci. Rep. 10:13824. doi: 10.1038/s41598-020-70716-5
Mei, F., Liu, J., Wu, J., Duan, Z., Chen, M., Meng, K., et al. (2020). Collagen Peptides Isolated from Salmo salar and Tilapia nilotica Skin Accelerate Wound Healing by Altering Cutaneous Microbiome Colonization via Upregulated NOD2 and BD14. J. Agric. Food Chem. 68, 1621–1633. doi: 10.1021/acs.jafc.9b08002
Minniti, G., Hagen, L. H., Porcellato, D., Jørgensen, S. M., Pope, P. B., and Vaaje-Kolstad, G. (2017). The Skin-Mucus Microbial Community of Farmed Atlantic Salmon (Salmo salar). Front. Microbiol. 8:2043. doi: 10.3389/fmicb.2017.02043
Minniti, G., Rod Sandve, S., Padra, J. T., Heldal Hagen, L., Linden, S., Pope, P. B., et al. (2019). The Farmed Atlantic Salmon (Salmo salar) Skin-Mucus Proteome and Its Nutrient Potential for the Resident Bacterial Community. Genes 10:515. doi: 10.3390/genes10070515
Mohammed, H. H., and Arias, C. R. (2015). Potassium permanganate elicits a shift of the external fish microbiome and increases host susceptibility to columnaris disease. Vet. Res. 46:82. doi: 10.1186/s13567-015-0215-y
Navarrete, P., Mardones, P., Opazo, R., Espejo, R., and Romero, J. (2008). Oxytetracycline treatment reduces bacterial diversity of intestinal microbiota of Atlantic salmon. J. Aquat. Anim. Health 20, 177–183. doi: 10.1577/h07-043.1
Ng’onga, M., Kalaba, F. K., Mwitwa, J., and Nyimbiri, B. (2019). The interactive effects of rainfall, temperature and water level on fish yield in Lake Bangweulu fishery. Zambia. J. Therm. Biol. 84, 45–52. doi: 10.1016/j.jtherbio.2019.06.001
Pindling, S., Azulai, D., Zheng, B., Dahan, D., and Perron, G. G. (2018). Dysbiosis and early mortality in zebrafish larvae exposed to subclinical concentrations of streptomycin. FEMS Microbiol. Lett. 365:fny188. doi: 10.1093/femsle/fny188
Ramsey, K. A., Rushton, Z. L., and Ehre, C. (2016). Mucin Agarose Gel Electrophoresis: western Blotting for High-molecular-weight Glycoproteins. J. Vis. Exp. 14:54153. doi: 10.3791/54153
Ross, A. A., Rodrigues Hoffmann, A., and Neufeld, J. D. (2019). The skin microbiome of vertebrates. Microbiome 7:79. doi: 10.1186/s40168-019-0694-6
Rossati, A. (2017). Global Warming and Its Health Impact. Int. J. Occup. Environ. Med. 8, 7–20. doi: 10.15171/ijoem.2017.963
Sainio, A., and Järveläinen, H. (2020). Extracellular matrix-cell interactions: focus on therapeutic applications. Cell Signal. 66:109487. doi: 10.1016/j.cellsig.2019.109487
Secor, D. H., and Gunderson, T. E. (1998). Effects of hypoxia and temperature on survival, growth, and respiration of juvenile Atlantic sturgeon, Acipenser oxyrinchus. Fish. Bull. Natl. Ocean. Atmos. Adm. 96, 603–613.
Shephard, K. L. (1994). Functions for fish mucus. Rev. Fish Biol. Fish. 4, 401–429. doi: 10.1007/bf00042888
Silvestre, F., Linares-Casenave, J., Doroshov, S. I., and Kültz, D. (2010). A proteomic analysis of green and white sturgeon larvae exposed to heat stress and selenium. Sci. Total Environ. 408, 3176–3188. doi: 10.1016/j.scitotenv.2010.04.005
Sumitomo, A., Siriwach, R., Thumkeo, D., Ito, K., Nakagawa, R., Tanaka, N., et al. (2019). LPA Induces Keratinocyte Differentiation and Promotes Skin Barrier Function through the LPAR1/LPAR5-RHO-ROCK-SRF Axis. J. Invest. Dermatol. 139, 1010–1022. doi: 10.1016/j.jid.2018.10.034
Sylvain, F., Cheaib, B., Llewellyn, M., Gabriel Correia, T., Barros Fagundes, D., Luis Val, A., et al. (2016). pH drop impacts differentially skin and gut microbiota of the Amazonian fish tambaqui (Colossoma macropomum). Sci. Rep. 6:32032. doi: 10.1038/srep32032
Szakolczai, J. (1997). Histopathological changes induced by environmental stress in common carp, Japanese coloured carp, European eel, and African catfish. Acta Vet. Hung. 45, 1–10.
Van den Steen, P., Rudd, P. M., Dwek, R. A., and Opdenakker, G. (1998). Concepts and principles of O-linked glycosylation. Crit. Rev. Biochem. Mol. Biol. 33, 151–208. doi: 10.1080/10409239891204198
Yang, H. T., Zou, S. S., Zhai, L. J., Wang, Y., Zhang, F. M., An, L. G., et al. (2017). Pathogen invasion changes the intestinal microbiota composition and induces innate immune responses in the zebrafish intestine. Fish Shellfish Immunol. 71, 35–42. doi: 10.1016/j.fsi.2017.09.075
Keywords: heat stress, Siberian sturgeon, skin, mucous cells, microbial diversity
Citation: Yang S, Xu W, Tan C, Li M, Li D, Zhang C, Feng L, Chen Q, Jiang J, Li Y, Du Z, Luo W, Li C, Gong Q, Huang X, Du X, Du J, Liu G and Wu J (2022) Heat Stress Weakens the Skin Barrier Function in Sturgeon by Decreasing Mucus Secretion and Disrupting the Mucosal Microbiota. Front. Microbiol. 13:860079. doi: 10.3389/fmicb.2022.860079
Received: 22 January 2022; Accepted: 21 March 2022;
Published: 26 April 2022.
Edited by:
Lifeng Zhu, Nanjing Normal University, ChinaReviewed by:
Stefan Oehlers, Agency for Science, Technology and Research (A*STAR), SingaporeR. S. Sethi, Guru Angad Dev Veterinary and Animal Sciences University, India
Copyright © 2022 Yang, Xu, Tan, Li, Li, Zhang, Feng, Chen, Jiang, Li, Du, Luo, Li, Gong, Huang, Du, Du, Liu and Wu. This is an open-access article distributed under the terms of the Creative Commons Attribution License (CC BY). The use, distribution or reproduction in other forums is permitted, provided the original author(s) and the copyright owner(s) are credited and that the original publication in this journal is cited, in accordance with accepted academic practice. No use, distribution or reproduction is permitted which does not comply with these terms.
*Correspondence: Jiayun Wu, YWJjMjAxNHdqeUAxNjMuY29t
†These authors have contributed equally to this work and share first authorship