- 1Department of Neonatology, Hunan Children’s Hospital, Changsha, China
- 2Department of Emergency, Hunan Children’s Hospital, Changsha, China
- 3Department of Medical, Hangzhou Matridx Biotechnology Co., Ltd., Hangzhou, China
Microbial infections cause significant morbidity and mortality in neonates. Metagenomic next-generation sequencing is a hypothesis-free and culture-free test that enables broad identification of pathogens and antimicrobial resistance genes directly from clinical samples within 24 h. In this study, we used mNGS for etiological diagnosis and monitoring the efficacy of antibiotic treatment in a cohort of neonatal patients with severe infections. The median age was 19.5 (3–52) days, median gestational age was 37.96 (31–40+3) weeks, and the median birth weight was 3,261 (1,300–4,300) g. The types of infectious diseases included pneumonia, sepsis, and meningitis. mNGS reported microbial findings in all cases, which led to changes in antibiotic treatment. These included cases of Mycobacterium tuberculosis, Legionella pneumophila, and Bacillus cereus. Eight of ten infants recovered after antibiotic adjustment and showed normal development during follow-up. On the other hand, neurological retardation was seen in two infants with meningitis. mNGS enabled etiological diagnosis and guided antibiotic therapy when all conventional methods failed to discover the culprit. It has the potential to cut down the overall cost and burden of disease management in neonatal infections.
Introduction
Infectious disease is an important cause of morbidity and mortality in neonatal populations (Cailes et al., 2018). Seventeen deaths per 100 live births and 6,700 neonatal deaths per day were reported in 2019 at a global scale (Gu et al., 2019). A total of 48% of neonatal death older than 7 days can be attributed to microbial infections, which is the main cause of death during this period (Collaborators, 2015; Khan et al., 2017). Conventional microbiological tests usually have long turn-around time and low sensitivity (Singh et al., 2021).
The gold standard for microbial identification is culture. However, several factors contributed to the low detection rate of culture in neonatal infections, including limited sample volume, the presence of intermittent bacteremia, and maternal intrapartum antimicrobial exposure. The detection rate of culture ranged from 3–10.29% in early-onset sepsis (EOS) to 16.7–33% in late-onset sepsis (LOS; Abdelhamid, 2017; Marks et al., 2020; Huggard et al., 2021). Other commonly used culture-independent tests such as blood cell count and the immature to total neutrophils (I/T ratio) can be used to infer bacterial infection (Shane et al., 2017). The inflammatory serum markers including C-reactive protein (CRP), Procalcitonin (PCT), and cytokines (interleukin 6, interleukin 8, tumor necrosis factor α, etc.) can also be used to indicate microbial infections, albeit with low specificity (Sharma et al., 2018; Brown et al., 2019). With the advances in molecular biology, polymerase chain reaction (PCR) and 16S/18S rRNA sequencing have been developed, which had limited scope of targets (Cummings et al., 2016; Kosacki et al., 2020). Therefore, an accurate diagnostic test for broad pathogen detection is needed.
Metagenomic Next-Generation Sequencing (mNGS) is culture-independent, which unbiasedly sequences both host and microbial nucleic acids extracted from a variety of clinical specimens (Deurenberg et al., 2017; Miller et al., 2019; Pohl et al., 2019; Wilson et al., 2019; Li et al., 2020). It has the advantage of detecting a broad range of pathogens and antibiotic resistance genes (Gu et al., 2019; Mitchell and Simner, 2019). Although the current mNGS technology has shown several advantages, there are still challenges for the clinical application: (1) the amount of non-microbial DNA presents in clinical samples (mostly derived from human cells) negatively impact the diagnostic sensitivity of microorganisms; (2) microbial read count cannot be compared between samples, making it unlikely to assess the changes in pathogen load if multiple mNGS tests were carried out for the same patient (Gu et al., 2019). Taken the above-mentioned concerns into consideration, we have developed a quantitative metagenomic sequencing approach (Zhou et al., 2021) to monitor the cellularity and microbial abundance of each sample, which was achieved by including a nucleic acid internal control (IC) spiked at constant concentrations into all specimens. In addition to monitoring the host level, we could also use the ratio of microbial reads/spike reads to quantify the abundance of microorganisms. Different microbes have genomes of varying length and GC content. Therefore, it was not likely to directly extrapolate the copy number or CFU based on this ratio. However, this ratio was independent of host nucleic acid and thus might be better than read count (RPM) to represent the pathogen load. In this study, we conducted the quantitative metagenomic sequencing for neonatal patients with severe infections in which conventional methods failed to elucidate the etiology. We reported 10 cases that were successfully and treated according to mNGS.
Materials and Methods
Ethics Statement
This study was performed in compliance with relevant laws and institutional guidelines and was approved by Ethics Committee of Hunan Children’s Hospital (HCHLL-2021-71). Written informed consent was obtained from all patients or their legal guardians.
Patients and Samples
Ten subjects suspected of microbial infections in Neonatal Intensive Care Unit (NICU) were recruited. Fresh specimens including peripheral blood, sputum, bronchoalveolar lavage fluid (BALF), and cerebrospinal fluid were collected for mNGS testing and conventional microbiology tests including bacterial/mycoplasma/chlamydia/fungal culture, antigen test for influenza A/B virus, parainfluenza virus, adenovirus respiratory syncytial virus and antibody test for cytomegalovirus, herpes simplex virus, toxoplasma, and rubella virus in each patient.
Preparation of Spike-in Internal Control
The internal control (IC) DNA was synthesized, amplified by PCR (TAKARA PrimeSTAR® HS DNA Polymerase, Cat# R044), and purified using magnetic beads (Matridx, Cat# MD012). All procedures were performed inside a Biosafety cabinet. Qubit fluorometric quantitation was performed on the amplicons (Thermo Fisher, Qubit™ dsDNA HS Assay Kit, Cat# Q32854). The nucleotide sequences of IC DNA can be found in a previous study (Zhou et al., 2021). ICs were added to each sample prior to nucleic acid extraction at a final concentration of 0.02 ng/μl.
Library Preparation and Sequencing
Whole blood was centrifuged at 1,600 g for 10 min and supernatant was centrifuged at 16,000 g for 10 min to separate plasma. For sputum, BALF and cerebrospinal fluid, DNA, or RNA sequencing were performed. DNA or RNA sequencing PCR-free library preparation was prepared by reverse transcription (for RNA), enzymatic fragmentation (except for plasma), end repairing, terminal adenylation, and adaptor ligation (NGSmaster™ library preparation, Matridx, Cat# MAR002; Luan et al., 2021). Concentration of libraries was quantified by real-time PCR (KAPA) and pooled. Shotgun sequencing was carried out on illumina Nextseq platform. Approximately 20 million of 75 bp single-end reads were generated for each library. For each run, one negative control (artificial plasma mixed with fragmented human genomic DNA) and one positive control (a mixture of inactivated bacteria, fungi, and pseudoviral particles containing synthesized DNA or RNA fragments of adenovirus and influenza A virus, respectively) were included for quality control.
Bioinformatic Pipeline
Raw sequencing data were analyzed by a bioinformatic pipeline, which included the following steps: (1) unnecessary adapter sequences and low-quality bases (Q-score cutoff, 20) were trimmed of in the pipeline; (2) human host sequences were eliminated by mapping to the human reference genome (GRCh38.p13) using BWA (BurrowsWheeler alignment)1; and (3) after removal of low-complexity reads, the remaining sequencing data were simultaneously aligned by BWA to reference database [NCBI nt database and GenBank (Benson et al., 2013)] in order to identify microbial species (Cantalupo and Pipas, 2019).
mNGS Reporting Criteria
Microbial reads identified from a library were reported if: (1) the sequencing data passed quality control filters (library concentration > 50 pM, Q20 > 85%, and Q30 > 80%); (2) negative control (NC) in the same sequencing run does not contain the species or the RPM (sample) /RPM (NC) ≥ 5, which was determined empirically as a cutoff for discriminating true positives from background contaminations.
Statistical Analysis
Patient characteristics were described using frequency, median, mean, range, and summary data with SPSS version 22.0.
Results
Clinical Characteristic and Overall Diagnostic Performance of mNGS
The age of the 10 participants ranged from 2 to 52 days, and 4 participants (40%) were female. The median age was 19.5 (3–52) days, median gestational age was 37.96 (31–40 + 3) weeks, and median birth weight was 3,261 (1,300–4,300) g. The types of infectious diseases included pneumonia, sepsis, and meningitis. The clinical characteristics of enrolled patients were listed in Table 1. The patient samples were analyzed by classical methods (culture, antigen test, and PCR) and mNGS. mNGS reported microbial findings in all cases, which led to changes in antibiotic treatment. These included cases of Mycobacterium tuberculosis, Legionella pneumophila, and Bacillus cereus. Eight of ten infants recovered after antibiotic adjustment and showed normal development. On the other hand, neurological retardation was seen in two infants with meningitis. The mNGS findings were listed in Table 2.
Case Descriptions
Mycobacterium tuberculosis
Patient 1 (19-day-old male) was admitted due to intermittent fever and tachypnea. The patient was born by vaginal delivery to a 30-year-old gravida 4, para 1 mother at 39 weeks of gestation. The Apgar score was 10/10 at 1 and 5 min, respectively. The patient developed fever (39.5°C) and required ventilation on day 16. During a 3-day-stay at a local hospital, the patient’s symptoms rapidly deteriorated and sepsis screen reported a CRP of 153.04 mg/dl and Procalcitonin (PCT) of 16.44 ng/ml. The patient was then transferred to our hospital due to respiratory distress, which needed 70% oxygen supplement to maintain saturation after upgrading antibiotics to meropenem and vancomycin. Microbiological culture of sputum, blood, and cerebrospinal fluid were negative. The biochemical analysis of cerebrospinal fluid (CSF) was also normal. On day 21, we collected peripheral blood for mNGS testing, which reported 59 reads of Mycobacterium tuberculosis complex. This finding was confirmed by T.SPOT-TB test of blood and Xpert MTB/RIF assay of sputum. Chest computed tomography (CT) scan revealed many miliary nodules with different sizes, densities, and shapes which had blurred edges and partly fused into a piece in both lungs (Figure 1). The patient’s mother had abnormal CT scan that was consistent with TB infection, although with no clinical manifestations. We applied antituberculosis treatment (Isoniazid, rifampicin, and pyrazinamide) in combination with oral Isoniazid and rifampicin (Figure 2). Blood count, CRP, growth, and neurological development were normal during the 1-month follow-up.
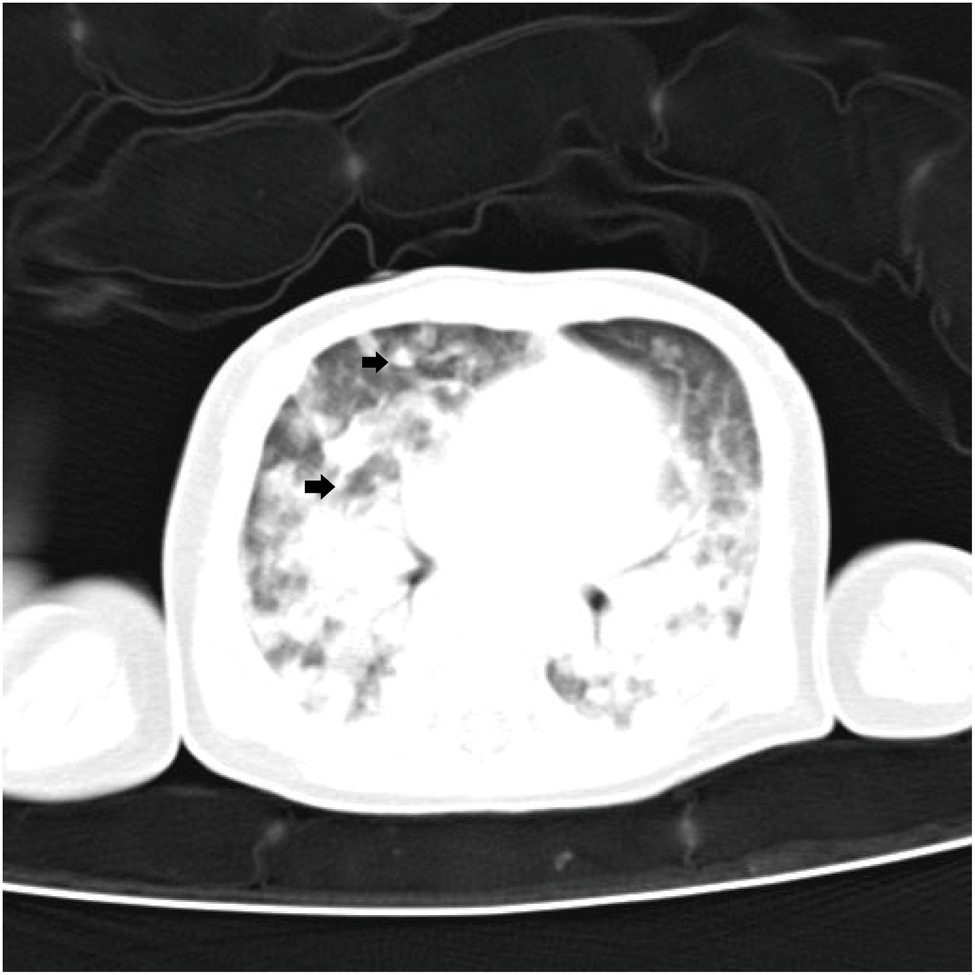
Figure 1. Chest computed tomography image of patient 1 showing many miliary nodules (arrowheads) can be seen in both lungs.
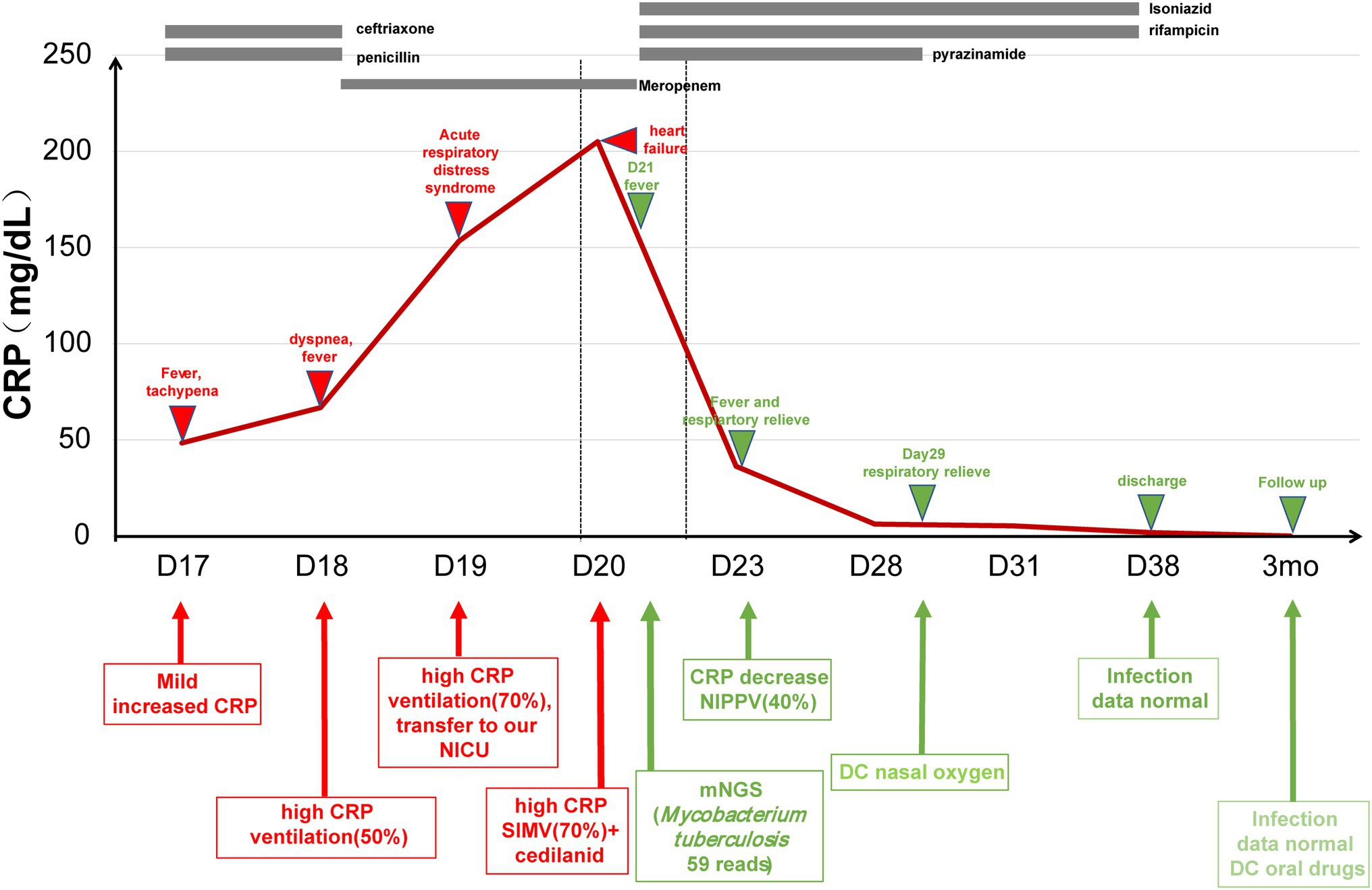
Figure 2. The treatment course in Mycobacterium tuberculosis case. CRP, C-reactive protein; NICU, neonatal intensive care unit; and NIPPV, non-invasive positive pressure ventilation.
Legionella pneumophila
Patient 2 (4-day-old female) was admitted to NICU due to high fever. The patient was born (3,400 g) via spontaneous pregnancy at 40 weeks to a 31-year-old mother (gravida 2, para 2). Apgar score was 10/10 at 1 and 5 min, respectively. After birth, the patient stayed at home for breast feeding using a circulation system. She developed fever and cough on day 4, which rapidly deteriorated. The patient had NCPAP support (35% oxygen) and persistent fever. The blood analysis showed a CRP higher than 320 mg/dl, PCT of 1.07 ng/ml, and lactate level of 4.3 mmol/l. On day 16, peripheral blood was collected for mNGS, which reported 361 reads of Legionella pneumophila while microbiological culture of sputum, blood, CSF, and BALF were negative. To confirm the mNGS findings, a BALF sample was collected and sent for 16s ribosomal RNA (rRNA) sequencing, which also identified L. pneumophila (Figure 3). In addition, the blood Legionella pneumophila IgM antibody was positive. We adjusted antibiotics to intravenous erythrocin and supported therapy (Figure 4) for 33 days. The patient was not discharged until mNGS of blood reported zero reads of L. pneumophila. The growth and neurological development were normal at 2-month follow-up.
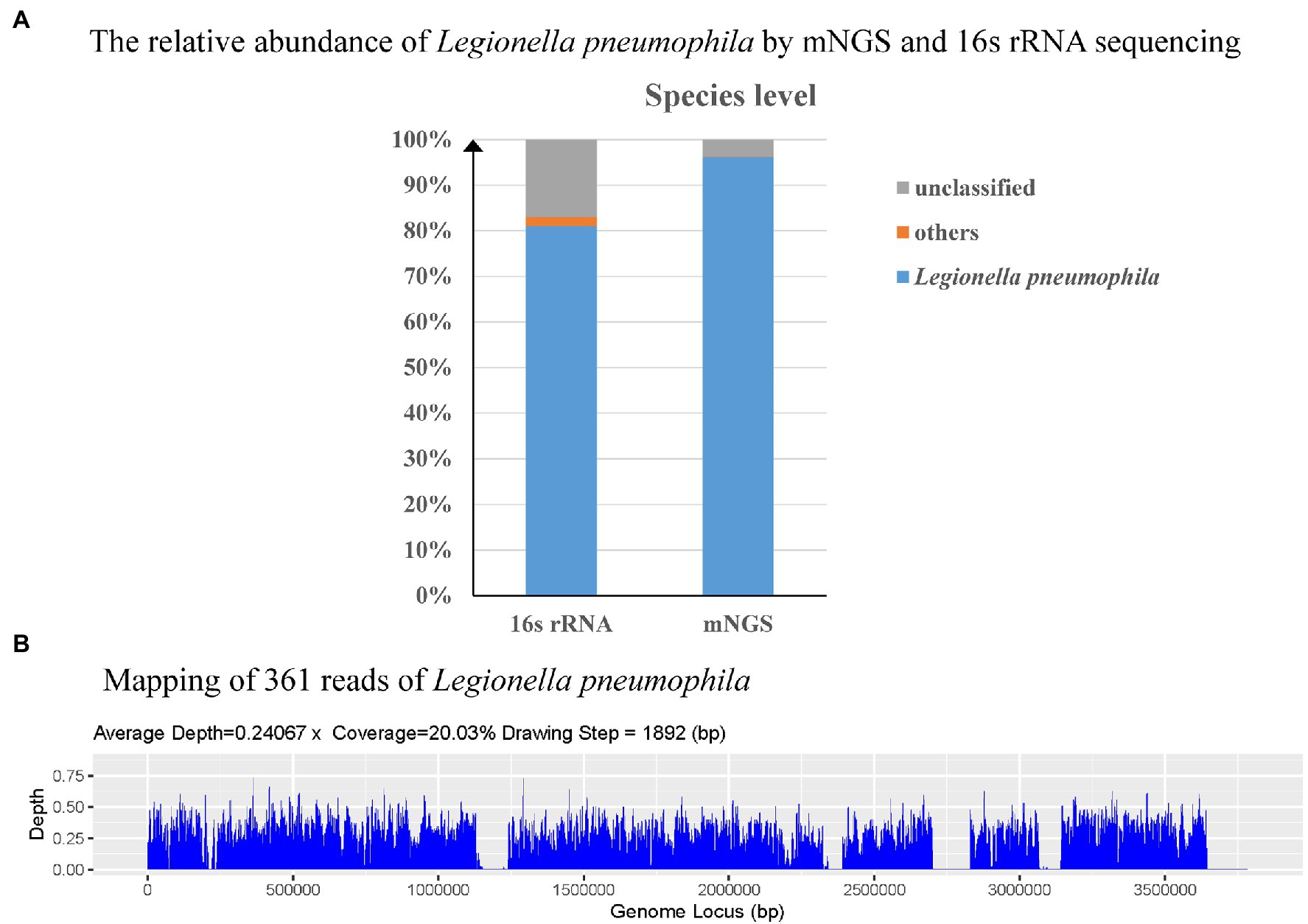
Figure 3. Diagnosis of L. pneumophila infection by means of Unbiased Next-Generation Sequencing (mNGS) and 16s ribosomal RNA (rRNA) sequencing. The relative abundance of L. pneumophila from the patient’s bronchoalveolar lavage fluid (BALF) by mNGS and 16s rRNA sequencing (A). Sequence reads mapped to L. pneumophila by mNGS data (B).
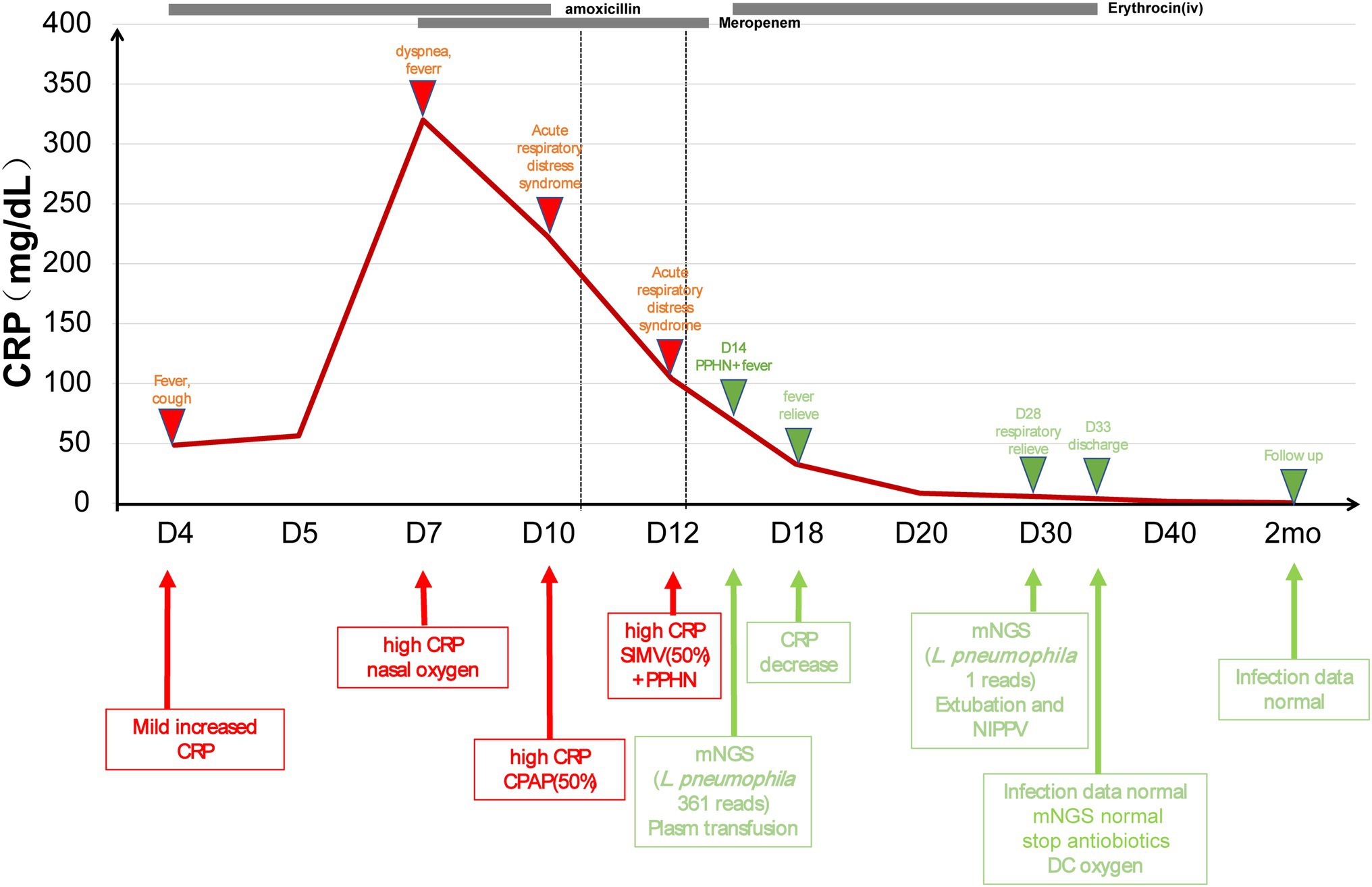
Figure 4. The treatment course in Legionella pneumophila case. CRP, C-reactive protein; NICU, neonatal intensive care unit; L. pneumophila, Legionella pneumophila.
Bacillus cereus
Patient 10 (15-day-old female) presented with tachypnea for 15 days, fever for 3 days, and convulsion for 2 days. She was the younger twin born via caesarean section to a 34-year-old gravida 6, para 3 mother at 34+2 weeks weighed at 1,660 g. The patient was sent to the local neonatal ward for nasal continuous positive airway pressure treatment (NCPAP). She had fever (38°C) and was switched to BiBAP (Fi02 30%) on day 12. Intubation was performed due to apnea on day 14. Blood test reported a CRP of 168.1 mg/dl, PCT of 18.12 ng/ml. CSF test showed white blood cell of 9.65 × 109/L, sugar of 1.02 mmol/L, and protein of 2.64 g/L. On day 16, CSF was collected and sent for mNGS, which reported 61 reads of Bacillus cereus. The same sample was also sent for 16s rRNA sequencing, which also showed Bacillus spp. Brain MRI revealed multiple encephalomalacia foci and venous thrombosis. According to one study (Lotte et al., 2017), we changed antibiotics to gentamicin and vancomycin (Figure 5). The patient was discharged from hospital on day 28 due to request of parents. The blood and CSF tests were normal during a follow-up visit two weeks later, but diffused encephalomalacia was still present.
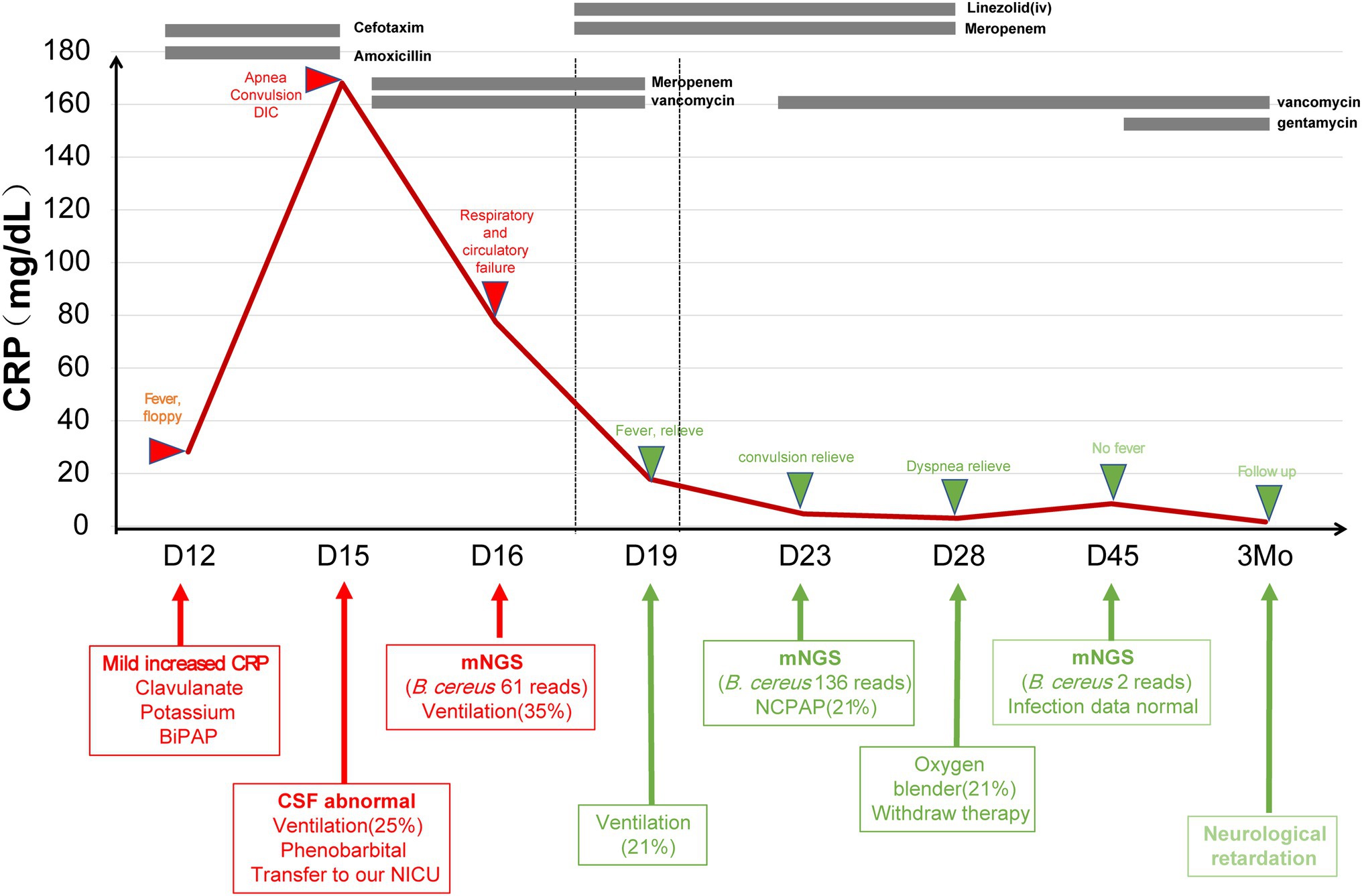
Figure 5. The treatment course in Bacillus cereus case. CRP, C-reactive protein; CSF, cerebral spinal fluid; NICU, neonatal intensive care unit; B. cereus, Bacillus cereus; and NCPAP, nasal continuous positive airway pressure.
Discussion
According to the World Health Organization (WHO) reports in 2018, about 10,000 newborns worldwide were infected with Mycobacterium tuberculosis, of which women and children younger than 15 years old accounted for 32 and 11% of tuberculosis cases, respectively (WHO, 2019). Fetus infected with Mycobacterium tuberculosis intrauterine or during delivery is called congenital tuberculosis, which is a rare type but might leader to a high mortality rate (Jenkins et al., 2017). Literature reviewed reported no more than 400 cases in world limited treatment experience with M. tuberculosis infection (Newberry and Robertson Bell, 2018; Li et al., 2019; Shao et al., 2021). Congenital tuberculosis symptoms cover respiratory, neurologic, and gastrointestinal. The onset of symptoms in infants tends be quicker than in older children and adults. The sickest infants may develop respiratory failure, shock, disseminated intravascular coagulation, and multiple organ failure (Newberry and Robertson Bell, 2018). Congenital tuberculosis is difficult to diagnose due to atypical clinical manifestation (Solórzano Santos, 2015). In clinical practice, due to differences in individuals’ immune functions, the time of symptom onset varied significantly (Schaaf et al., 2010). Varik et al. (2012) reported the symptoms of congenital tuberculosis occurred within 3 weeks after birth, and the average age of onset was 28 days. In our case, the 19-day-old male presented fever and tachypnea as well as signs of respiratory failure. Even after mNGS finding, the Mycobacterium tuberculosis culture of peripheral blood, sputum, and BALF for 21 days was still negative. The etiological diagnosis has shortened the length of stay (mean duration of 36.5 days) and improved the patient’s prognosis (Li et al., 2019).
Legionella pneumophila can cause pneumonia, but rarely seen in neonates (Kleinberg and Antony, 2020). Molly et al. reported a 6-day-old neonate infected by L. pneumophila, which rapidly progressed to severe ARDS (Dorfman et al., 2020). The clinical presentations of Legionnaires’ disease in neonates included fever, cough, tachypnea, pneumonia in chest X-ray, acute respiratory distress, and sepsis. In our case, the patient presented with fever and progressive respiratory insufficiency that needed mechanical ventilation. When diagnosing legionellosis, samples from lower respiratory tract (sputum, BALF etc.) are preferred (Cattan et al., 2019) and the culture generally takes 3–5 days. The urine antigen test is also a first-line diagnostic test, although it is limited to the Legionella pneumophila serogroup 1 (Cunha et al., 2016). In our case, urine antigen and culture of the BALF were negative. The blood IgM antibody, BALF mNGS, and 16s rRNA sequencing were positive for L. pneumophila. Macrolide and quinolone antibiotics are suggested as first-line therapy of severe Legionella pneumonia (Cunha et al., 2016). For our patient, empirical antibiotics were first changed from amoxicillin to meropenem, neither of which was effective against L. pneumophila. Following the etiological diagnosis, erythrocin was administered with satisfying results.
Due to immature immune system and prolonged mechanical ventilation, neonates are particularly susceptible to disseminated infections caused by environmental organisms such as B. cereus (Schelonka and Infante, 1998). B. cereus is usually related to contaminated food and can lead to digestive manifestation and endophthalmitis (Lewin et al., 2019). Despite the small number of reported cases of B. cereus infection in infants, many of which were fatal. Twelve (75%) of the infants died, the majority of which occurred within 3 days of onset. Four infants who survived had developed cerebral palsy (Manickam et al., 2008). Imaging characteristics included hemorrhagic meningoencephalitis that first affected the white matter, and then the cortex and basal ganglia (Lequin et al., 2005). In our case, similar imaging features were observed in encephalomalacia foci in the white matter and then in the cortex and basal ganglia. The CSF, sputum, and blood culture were negative. But CSF mNGS and 16s rRNA sequencing were positive for B. cereus. Due to a beta-lactamase that renders it resistant to most penicillins and cephalosporins, effective antibiotics include chloramphenicol, clindamycin, vancomycin, erythromycin, and aminoglycosides (Drobniewski, 1993).
Our study has several limitations. First, this study was of a small sample size and was unable to sufficiently evaluate the clinical utility and drawbacks of mNGS in neonatal patients. Second, there are challenges associated with mNGS when applied in a clinical setting, such as the cost, complexity of the test, and lack of experimental standards. Third, the interpretation of results was difficult when distinguishing causal pathogens from colonizing or contaminating microorganisms. Last, all patients received empirical antibiotic treatment before mNGS was conducted, which might negatively impact the analytical sensitivity of detection microorganisms, despite that all cases returned positive findings.
Data Availability Statement
The datasets presented in this study can be found in online repositories. The names of the repository/repositories and accession number(s) can be found at: https://www.ncbi.nlm.nih.gov/sra PRJNA808819.
Ethics Statement
The studies involving human participants were reviewed and approved by Ethics Committee of Hunan Children’s Hospital (HCHLL-2021-71). Written informed consent to participate in this study was provided by the participants’ legal guardian/next of kin.
Author Contributions
RZ, X-mP, and CL conceptualized and designed this study. RZ and YZ drafted the paper. Z-hX and C-yL conducted statistical analysis on the data. FZ, W-qH, and MZ gave a lot of assistance and revised manuscript. All authors contributed to the article and approved the submitted version.
Funding
This work was supported by Natural Science Foundation of Hunan Province, China (grant no. 2021JJ40278).
Conflict of Interest
CL and C-yL were employed by Hangzhou Matridx Biotechnology Co., Ltd.
The remaining authors declare that the research was conducted in the absence of any commercial or financial relationships that could be construed as a potential conflict of interest.
Publisher’s Note
All claims expressed in this article are solely those of the authors and do not necessarily represent those of their affiliated organizations, or those of the publisher, the editors and the reviewers. Any product that may be evaluated in this article, or claim that may be made by its manufacturer, is not guaranteed or endorsed by the publisher.
Acknowledgments
We thank the patients for cooperating with our investigation and acknowledge the professionalism and compassion demonstrated by all the healthcare workers involved in clinical care.
Footnotes
References
Abdelhamid, S. M. (2017). Time to positivity and antibiotic sensitivity of neonatal blood cultures. J. Glob. Infect. Dis. 9, 102–107. doi: 10.4103/jgid.jgid_1_17
Benson, D. A., Cavanaugh, M., Clark, K., Karsch-Mizrachi, I., Lipman, D. J., Ostell, J., et al. (2013). GenBank. Nucleic Acids Res. 41, D36–D42. doi: 10.1093/nar/gks1195
Brown, J. V. E., Meader, N., Cleminson, J., and McGuire, W. (2019). C-reactive protein for diagnosing late-onset infection in newborn infants. Cochrane Database Syst. Rev. 2019:Cd012126. doi: 10.1002/14651858.CD012126.pub2
Cailes, B., Kortsalioudaki, C., Buttery, J., Pattnayak, S., Greenough, A., Matthes, J., et al. (2018). Epidemiology of UK neonatal infections: the neonIN infection surveillance network. Arch. Dis. Child. Fetal Neonatal Ed. 103, F547–F553. doi: 10.1136/archdischild-2017-313203
Cantalupo, P. G., and Pipas, J. M. (2019). Detecting viral sequences in NGS data. Curr. Opin. Virol. 39, 41–48. doi: 10.1016/j.coviro.2019.07.010
Cattan, S., Thizy, G., Michon, A., Arlet, J. B., Lanternier, F., Lebeaux, D., et al. (2019). Legionella spp: An update. Rev. Med. Interne 40, 791–798. doi: 10.1016/j.revmed.2019.08.007
Collaborators, G. C. M. (2015). Global, regional, national, and selected subnational levels of stillbirths, neonatal, infant, and under-5 mortality, 1980–2015: a systematic analysis for the global burden of disease study 2015. Lancet 388, 1725–1774. doi: 10.1016/s0140-6736(16)31575-6
Cummings, L. A., Kurosawa, K., Hoogestraat, D. R., SenGupta, D. J., Candra, F., Doyle, M., et al. (2016). Clinical next generation sequencing outperforms standard microbiological culture for characterizing Polymicrobial samples. Clin. Chem. 62, 1465–1473. doi: 10.1373/clinchem.2016.258806
Cunha, B. A., Burillo, A., and Bouza, E. (2016). Legionnaires’ disease. Lancet 387, 376–385. doi: 10.1016/s0140-6736(15)60078-2
Deurenberg, R. H., Bathoorn, E., Chlebowicz, M. A., Couto, N., Ferdous, M., García-Cobos, S., et al. (2017). Application of next generation sequencing in clinical microbiology and infection prevention. J. Biotechnol. 243, 16–24. doi: 10.1016/j.jbiotec.2016.12.022
Dorfman, M. V., Clark, J. D., and Brogan, T. V. (2020). ECLS for legionella: All ages welcome in the ELSO registry. ASAIO J. 66, 226–229. doi: 10.1097/mat.0000000000000985
Drobniewski, F. A. (1993). Bacillus cereus and related species. Clin. Microbiol. Rev. 6, 324–338. doi: 10.1128/cmr.6.4.324
Gu, W., Miller, S., and Chiu, C. Y. (2019). Clinical metagenomic next-generation sequencing for pathogen detection. Annu. Rev. Pathol. 14, 319–338. doi: 10.1146/annurev-pathmechdis-012418-012751
Huggard, D., Powell, J., Kirkham, C., Power, L., O'Connell, N. H., and Philip, R. K. (2021). Time to positivity (TTP) of neonatal blood cultures: a trend analysis over a decade from Ireland. J. Matern. Fetal Neonatal Med. 34, 780–786. doi: 10.1080/14767058.2019.1617687
Jenkins, H. E., Yuen, C. M., Rodriguez, C. A., Nathavitharana, R. R., McLaughlin, M. M., Donald, P., et al. (2017). Mortality in children diagnosed with tuberculosis: a systematic review and meta-analysis. Lancet Infect. Dis. 17, 285–295. doi: 10.1016/s1473-3099(16)30474-1
Khan, A. M., Morris, S. K., and Bhutta, Z. A. (2017). Neonatal and perinatal infections. Pediatr. Clin. N. Am. 64, 785–798. doi: 10.1016/j.pcl.2017.03.008
Kleinberg, K. A., and Antony, S. J. (2020). Legionella pneumophila pneumonia in pregnancy: A case report and review of the literature. Infect. Disord. Drug Targets 20, 247–252. doi: 10.2174/1871526519666190119110657
Kosacki, J., Boisset, S., Maurin, M., Cornut, P. L., Thuret, G., Hubanova, R., et al. (2020). Specific PCR and quantitative real-time PCR in ocular samples from acute and delayed-onset postoperative Endophthalmitis. Am J. Ophthalmol. 212, 34–42. doi: 10.1016/j.ajo.2019.11.026
Lequin, M. H., Vermeulen, J. R., van Elburg, R. M., Barkhof, F., Kornelisse, R. F., Swarte, R., et al. (2005). Bacillus cereus meningoencephalitis in preterm infants: neuroimaging characteristics. AJNR Am. J. Neuroradiol. 26, 2137–2143.
Lewin, A., Quach, C., Rigourd, V., Picaud, J. C., Perreault, T., Frange, P., et al. (2019). Bacillus cereus infection in neonates and the absence of evidence for the role of banked human milk: case reports and literature review. Infect. Control Hosp. Epidemiol. 40, 787–793. doi: 10.1017/ice.2019.110
Li, C., Liu, L., and Tao, Y. (2019). Diagnosis and treatment of congenital tuberculosis: a systematic review of 92 cases. Orphanet J. Rare Dis. 14:131. doi: 10.1186/s13023-019-1101-x
Li, Y., Sun, B., Tang, X., Liu, Y. L., He, H. Y., Li, X. Y., et al. (2020). Application of metagenomic next-generation sequencing for bronchoalveolar lavage diagnostics in critically ill patients. Eur. J. Clin. Microbiol. Infect. Dis. 39, 369–374. doi: 10.1007/s10096-019-03734-5
Lotte, R., Hérissé, A. L., Berrouane, Y., Lotte, L., Casagrande, F., Landraud, L., et al. (2017). Virulence analysis of Bacillus cereus isolated after death of preterm neonates, Nice, France, 2013. Emerg. Infect. Dis. 23, 845–848. doi: 10.3201/eid2305.161788
Luan, Y., Hu, H., Liu, C., Chen, B., Liu, X., Xu, Y., et al. (2021). A proof-of-concept study of an automated solution for clinical metagenomic next-generation sequencing. J. Appl. Microbiol. 131, 1007–1016. doi: 10.1111/jam.15003
Manickam, N., Knorr, A., and Muldrew, K. L. (2008). Neonatal meningoencephalitis caused by Bacillus cereus. Pediatr. Infect. Dis. J. 27, 843–846. doi: 10.1097/INF.0b013e31816feec4
Marks, L., de Waal, K., and Ferguson, J. K. (2020). Time to positive blood culture in early onset neonatal sepsis: A retrospective clinical study and review of the literature. J. Paediatr. Child Health 56, 1371–1375. doi: 10.1111/jpc.14934
Miller, S., Naccache, S. N., Samayoa, E., Messacar, K., Arevalo, S., Federman, S., et al. (2019). Laboratory validation of a clinical metagenomic sequencing assay for pathogen detection in cerebrospinal fluid. Genome Res. 29, 831–842. doi: 10.1101/gr.238170.118
Mitchell, S. L., and Simner, P. J. (2019). Next-generation sequencing in clinical microbiology: are we there yet? Clin. Lab. Med. 39, 405–418. doi: 10.1016/j.cll.2019.05.003
Newberry, D. M., and Robertson Bell, T. (2018). Congenital tuberculosis: A new concern in the neonatal intensive care unit. Adv. Neonatal Care 18, 341–349. doi: 10.1097/anc.0000000000000555
Pohl, D., Keller, P. M., Bordier, V., and Wagner, K. (2019). Review of current diagnostic methods and advances in helicobacter pylori diagnostics in the era of next generation sequencing. World J. Gastroenterol. 25, 4629–4660. doi: 10.3748/wjg.v25.i32.4629
Schaaf, H. S., Collins, A., Bekker, A., and Davies, P. D. (2010). Tuberculosis at extremes of age. Respirology 15, 747–763. doi: 10.1111/j.1440-1843.2010.01784.x
Schelonka, R. L., and Infante, A. J. (1998). Neonatal immunology. Semin. Perinatol. 22, 2–14. doi: 10.1016/s0146-0005(98)80003-7
Shane, A. L., Sánchez, P. J., and Stoll, B. J. (2017). Neonatal sepsis. Lancet 390, 1770–1780. doi: 10.1016/s0140-6736(17)31002-4
Shao, Y., Hageman, J. R., and Shulman, S. T. (2021). Congenital and Perinatal Tuberculosis. Neoreviews 22:e600. doi: 10.1542/neo.22-9-e600
Sharma, D., Farahbakhsh, N., Shastri, S., and Sharma, P. (2018). Biomarkers for diagnosis of neonatal sepsis: a literature review. J. Matern. Fetal Neonatal Med. 31, 1646–1659. doi: 10.1080/14767058.2017.1322060
Singh, M., Alsaleem, M., and Gray, C.P. (2021). Neonatal Sepsis. Treasure Island (FL): StatPearls Publishing.
Solórzano Santos, F. (2015). Congenital tuberculosis: a challenge to overcome. Bol. Med. Hosp. Infant. Mex. 72, 3–4. doi: 10.1016/j.bmhimx.2015.02.006
Varik, R. S., Shubha, A. M., Lewin, M., Alexander, B., Kini, U., and Das, K. (2012). Infantile pulmonary tuberculosis: the great mimic. Pediatr. Surg. Int. 28, 627–633. doi: 10.1007/s00383-012-3084-8
WHO (2019). Global tuberculosis report 2019. Available at: https://www.who.int/teams/global-tuberculosis-programme/tb-reports
Wilson, M. R., Sample, H. A., Zorn, K. C., Arevalo, S., Yu, G., Neuhaus, J., et al. (2019). Clinical metagenomic sequencing for diagnosis of meningitis and encephalitis. N. Engl. J. Med. 380, 2327–2340. doi: 10.1056/NEJMoa1803396
Keywords: neonatal infections, metagenomic next-generation sequencing, Mycobacterium tuberculosis, Legionella pneumophila, Bacillus cereus
Citation: Zhang R, Zhuang Y, Xiao Z-h, Li C-y, Zhang F, Huang W-q, Zhang M, Peng X-m and Liu C (2022) Diagnosis and Surveillance of Neonatal Infections by Metagenomic Next-Generation Sequencing. Front. Microbiol. 13:855988. doi: 10.3389/fmicb.2022.855988
Edited by:
Shishir K. Gupta, University of Wuerzburg, GermanyReviewed by:
Davide Borroni, Riga Stradiņš University, LatviaElena Bencurova, Julius Maximilian University of Würzburg, Germany
Copyright © 2022 Zhang, Zhuang, Xiao, Li, Zhang, Huang, Zhang, Peng and Liu. This is an open-access article distributed under the terms of the Creative Commons Attribution License (CC BY). The use, distribution or reproduction in other forums is permitted, provided the original author(s) and the copyright owner(s) are credited and that the original publication in this journal is cited, in accordance with accepted academic practice. No use, distribution or reproduction is permitted which does not comply with these terms.
*Correspondence: Chao Liu, liuchao@matridx.com; Xiao-ming Peng, pxmprf@163.com